- Universidad Nacional de Colombia, Sede Bogotá, Facultad de Ciencias, Instituto de Ciencias Naturales, Grupo en Conservación y Manejo de Vida Silvestre, Bogotá, Colombia
Tropical dry forests are among the most threatened ecosystems worldwide. Bats' role in those ecosystems is critical because of multiple bat-mediated processes. Such processes are strongly related to bats' functional traits. However, it is poorly known which bat's functional traits could relate to variations in environmental conditions in tropical dry forests. In this study, we tested the hypotheses that bat functional traits would be significantly associated with landscape variables, climatic variables, and land-use intensity. For testing these hypotheses, we used data from phyllostomid and mormoopid bats captured in mist nets and data from non-phyllostomid insectivorous bat species registered by passive acoustic monitoring. We considered six functional traits for phyllostomid and mormoopid bats, and for non-phyllostomid insectivorous bats, we added two echolocation parameters. We measured five environmental variables, two of local climate (daily maximum temperature and wind speed) and three of landscape features (total area of water and closeness, probability of finding caves, and conservation status). The relationships between bat functional traits and environmental variables were evaluated using the RLQ and the fourth-corner analysis. We captured 360 individuals belonging to 14 species with mist nets (Phyllostomidae and Mormoopidae), and we identified 18 species and six sonotypes with acoustic sampling (Emballonuridae, Mormoopidae, Molossidae, Natalidae, Noctilionidae, and Vespertilionidae). We found that bats' functional traits related to environmental conditions were pulse structure, diet, vertical foraging stratification, and trophic level, although these relationships varied among bats' ecological roles. The hematophagous were related to water bodies' closeness, and animalivorous bats, mostly mormoopids, showed a relationship with the probability of finding caves. Insectivorous bats that mostly forage on the canopy and emit qCF calls were significantly related to more conserved sites, and bats that emit qCF pulses were significantly associated with less area covered by water. Our findings provide insights into how bat functional traits vary in their relationships with environmental conditions in harsh environments such as dry forests.
1. Introduction
Tropical dry forests are among the most threatened forests worldwide, and 54% are in South America (Miles et al., 2006). In Colombia, tropical dry forests originally covered about nine million hectares, but currently, only 8% of those forests remain in isolated patches under different conservation statuses (Pizano et al., 2014). Those tropical dry forest remnants are found within the several biogeographic regions described by Hernández-Camacho et al. (1992), mainly in the Caribbean (called Peri-Caribbean Arid Belt), North Andean region (North Andean Province), and some sites in the valleys of Magdalena, Cauca, Chicamocha, and Patia rivers (Pizano et al., 2014). These areas are the most vulnerable to climate change due to their evapotranspiration conditions, their repercussion on the hydrological cycle, their role in variations of annual runoff percentages, and the seasonality of rain and drought (Solano Pita and Pérez Hernández, 2016). In particular, for the dry ecosystems, the Peri-Caribbean Arid Belt is the biogeographic province most vulnerable to such changes. Similarly, 10% of the North Andean Province is considered highly vulnerable to climate change. This area is covered by dry high Andean vegetation, sub-Andean semi-arid vegetation, tropical dry forest, and tropical dry scrub, such as those located toward the Chicamocha River valley (Solano Pita and Pérez Hernández, 2016).
Dry environments pose challenges that demand biological strategies to live in such conditions. However, despite conditions of water limitation, many bat species thrive with success in many arid zones (Conenna et al., 2021). Habitat features are also important for such bat success in dry areas. For example, in hot-dry environments, features such as the availability of caves are important because they provide optimal roost sites, with more humidity and thermal stability (Kunz, 1982). Also, variations in environmental conditions, such as land-use changes (Farneda et al., 2020) and climatic local features, may affect bat species composition. Also, the frequency of some bat species' functional traits may be related to variations in environmental factors. For example, as aridity increases, a rise in wing loading and wind aspect ratio, as well as body size, and a decrease in echolocation frequency were found in a study of bat functional traits in an aridity gradient at a global scale (Conenna et al., 2021).
The vast majority of studies on bat functional ecology have been conducted in moist broadleaf tropical forests (Farneda et al., 2015, 2020; Castillo-Figueroa, 2020). Although some functional information has been provided for bats in dry African areas (Monadjem et al., 2020), in Neotropical dry forests still needs to be better known. Most research on bats from Neotropical arid areas has a taxonomic diversity approach, where the bats' ecological role is only suggested. For instance, most studies indicate that bats from arid areas participate in ecological processes such as seed dispersal (Sánchez et al., 2007; Molinari et al., 2012), columnar cactus pollination (Marinkelle and Cadena, 1972; Petit, 1995; Nassar et al., 1997), and insect predation (García-Morales et al., 2016). Some studies provide actual data on the relationships between cactus pollination and seed dispersal (Locatelli et al., 1997; Naranjo et al., 2003; Arias-Cóyotl et al., 2006). However, more detailed data on bat functional traits related to their functional role in the dry forest are rarely presented. Similarly, some studies suggest that the wing morphology and the echolocation pulse structure are predictive features of bats' ecosystem functionality associated with food resource exploitation (Almeida et al., 2014; Marques et al., 2016; Wordley et al., 2017). Despite the correlation between variations in bat echolocation calls with vegetation structural features (Schnitzler et al., 2003), this topic has been underexplored in Neotropical species inhabiting the dry forest and arid areas. To provide useful information to improve bat conservation in the Neotropics, it is key to better understand the relationships between bat functional traits and the environmental factors that allow some species to persist. This knowledge is critical, especially in endangered ecosystems that still harbor bat species.
In this study, we aimed to identify which bat functional traits are related to environmental conditions in dry areas or northern Colombia and how those relationships vary among bat species with different ecological roles. Due to bat assemblages showing a strong response to land-use transformation, vegetation loss, and environmental variables (García-Morales et al., 2013; Azhar et al., 2015; Farneda et al., 2015; Aguirre et al., 2016; Kahnonitch et al., 2018; Conenna et al., 2021), we hypothesize that the bat functional traits will be significantly related to landscape variables, climatic variables, and land-use intensity. We tested these hypotheses using data from phyllostomid and mormoopid bats captured in mist nets and data from non-phyllostomid insectivorous bat species registered by passive acoustic monitoring in several sites of dry environments in northern Colombia. We expected that animalivorous bat species with higher maneuverability and agility to move within the forest (higher aspect ratio and relative wing loading) would be positively associated with areas with good conservation status and water bodies' closeness. Also, we expected that places with good conservation status and more suitable landscapes (e.g., higher probability of finding caves and easier access to water) would be critical environmental variables to maintain bat functional traits.
2. Materials and methods
2.1. Study area
We conducted fieldwork in 21 sampling points grouped in seven areas which we delimited by polygons based on the bat's mobility limits. Four polygons were located on the Colombian Caribbean coast: Santa Marta (four sampling points), Puerto Colombia (two sampling points), Piojó (four sampling points), and Cartagena (two sampling points) (Figure 1). The remaining three polygons were located in the Chicamocha River Basin: Lagunetas (three sampling points), Jordán (four sampling points), and Cepitá (two sampling points).
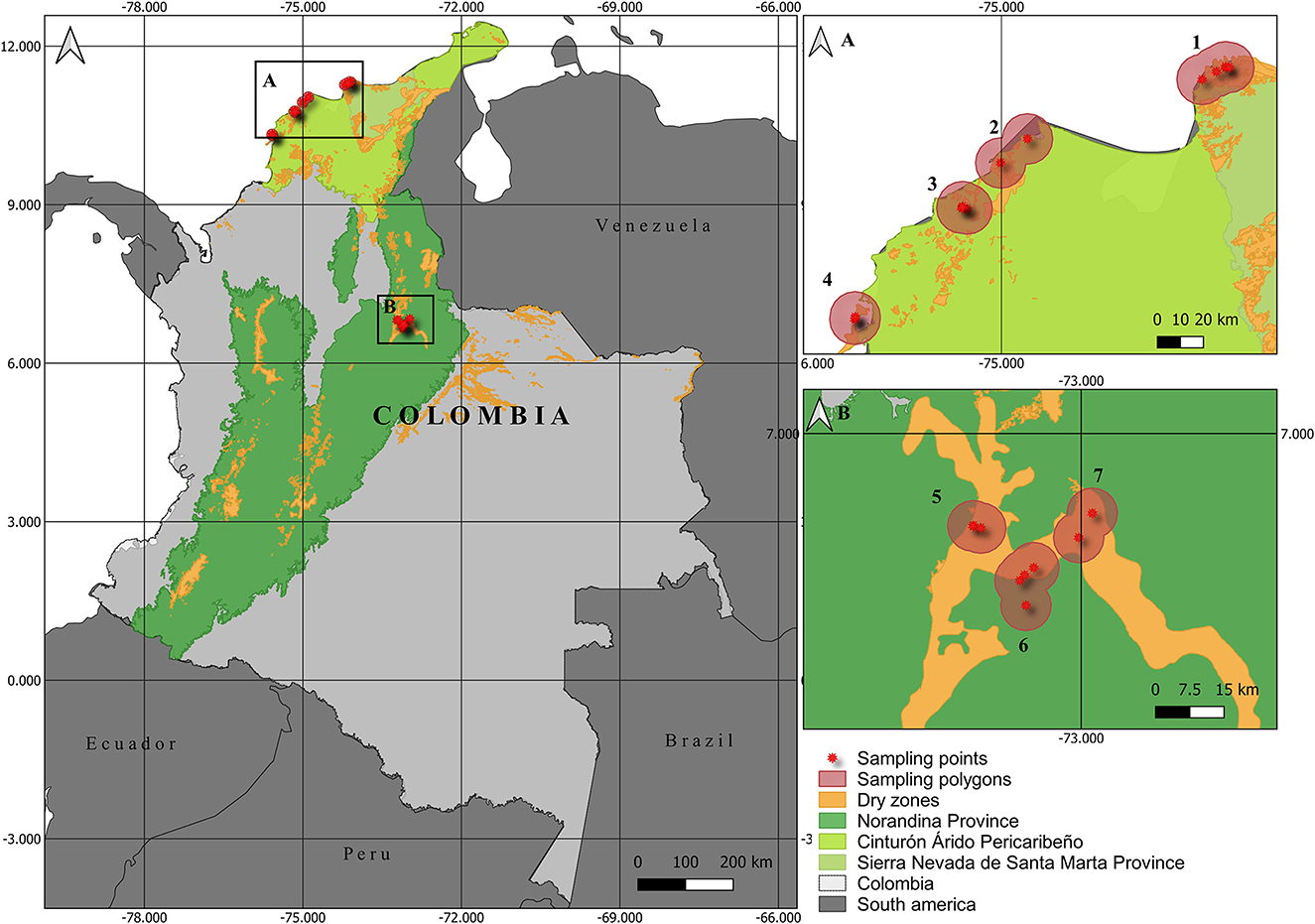
Figure 1. Map of the study area in Colombian dry forests. Sampling points in each polygon in (A) Caribbean Coast: 1. Santa Marta, 2. Puerto Colombia, 3. Piojó, and 4. Cartagena. (B) Chicamocha River Basin: 5. Lagunetas, 6. Jordán, and 7. Cepitá.
The polygons of Santa Marta, Cartagena, Piojó, and Puerto Colombia are located in the biogeographical province of the Peri-Caribbean Arid Belt. A seasonally dry tropical forest dominates this region. The average environmental temperature is 27°C, reaching up to 40°C during the dry season. This biogeographical province has low average yearly rainfall (400 mm), strong winds, and high evaporation rates (Hernández-Camacho et al., 1992).
The Cepitá, Jordán, and Lagunetas polygons are in the Chicamocha River Basin, an arid zone located in the Norandina Province in the Eastern Mountain range at elevations from 500 to 1,500 m.a.s.l. (Figure 1B, Albesiano and Rangel-Churio, 2006). The dominant vegetation of these polygons corresponds to semi-arid vegetation and dry forests (Latorre et al., 2014). The environmental average annual temperature ranges from 15.8 to 35.7°C, and the average yearly rainfall is 731 mm (Albesiano et al., 2003).
2.2. Bat sampling
Phyllostomid and mormoopid bats were captured using mist nets, and insectivorous bat species were recorded from passive acoustic monitoring. For the mist-netting sampling, we used six ground-level mist nets (two 6 m, one 9 m, and three 12 m, 20 mm mesh, ECOTONE, Poland) at each sampling point. Mist nets were active from 18:00 to 00:00 a.m. and were revised every 25 min on average. We calculated the sampling effort as m2 × mist net × hours (m.n.h), reaching a total effort of 12,508 m.n.h. Each polygon was sampled from two to four nights. Captured bats were measured and marked in the wing membrane using tattoos and were released at the same capture site. Capture and handling methods followed the guidelines of the American Society of Mammalogists (Sikes et al., 2011). Bat captures were authorized by licenses from the Environmental Licenses National Agency, ANLA (Resolution 0255, 14/03/2014). Rare species with less than three captures and captured in only one location were excluded, resulting in 14 species for analysis. We captured 360 individuals, from which we processed 290 different individual photographs; 14 species were represented.
Passive acoustic monitoring was carried out using two SM4BAT FS recorders with an omnidirectional SMM-U2 ultrasonic microphone (Wildlife Acoustics Inc., USA; frequency range 12–180 kHz, sampling rate 360 kHz, resolution 16 bits). Microphones were placed at 3 m above the ground level. Both detectors were active from 30 min before sunset to 00:00 at each sampling site for two to four nights per polygon. We used the Kaleidoscope Pro software (ver. 5.4.1, Wildlife Acoustics, Inc, USA) to analyze and classify the audio files. We carried out a manual classification of all recordings to identify bat species calls following Pio et al. (2010), Jung and Kalko (2011), López-Baucells et al. (2016), Arias-Aguilar et al. (2018), and a regional reference library for bat calls gathered by A. Otálora-Ardila (unpublished). In cases where identification to species level was not possible, species with similar calls were grouped in sonotypes (Supplementary Table S1). We analyzed only search phase sequences with at least three consecutive pulses (McKenzie et al., 2002; Lloyd et al., 2006). We estimated the following parameters: pulse structure, bandwidth, frequency of maximum energy (FME), minimum frequency (minF), maximum frequency (maxF), initial and final frequency (initial and final F, respectively), pulse duration (Dur), and pulse interval (PI) (Williams-Guillén and Perfecto, 2011). These measurements were estimated by adjusting the spectrogram parameters: Fast Fourier Transformation of size 1,024, window length equal to 5ms, and a threshold of 10dB in a Hanning window in 5-s files (Torrent et al., 2018; Tuneu-Corral et al., 2020). We recorded 61,259 audio files from passive acoustic monitoring, where 56,172 were analyzed and 5,087 were excluded because they contained noise.
2.3. Functional traits of bats
For phyllostomid and mormoopid bats, we considered six functional traits: body mass, relative wing loading, aspect ratio, diet, trophic level, and vertical stratification. For insectivorous bats, we added two parameters related to their echolocation behavior associated with habitat use and foraging strategies: frequency of maximum energy (FME) and pulse structure (Kalko et al., 1996; Schnitzler et al., 2003; Wordley et al., 2017; Table 1).
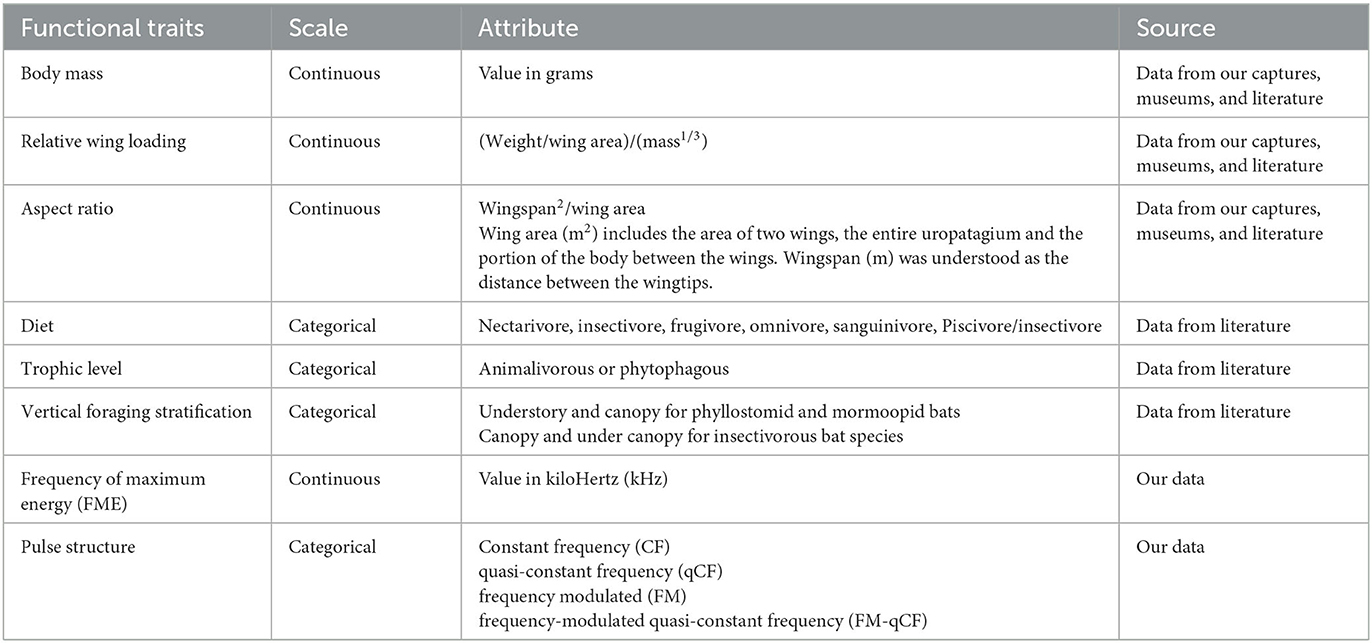
Table 1. Description of the functional traits selected for the bat species captured and registered using acoustic sampling in Colombian dry forests.
Body mass has been used as a body size estimator for bats, and it is related to their flight mobility and agility (Jung and Threlfall, 2018). Wing morphology traits have been demonstrated to correlate with flight speed and maneuverability (Norberg and Rayner, 1987). We estimated relative wing loading and aspect ratio following Norberg and Rayner (1987) and Wordley et al. (2017). We took pictures of the dorsal view of the right wing and body of at least five individuals of each species at each sampling location, except for gestating females. Images were analyzed using ImageJ 1.8.0 software (National Institutes of Health, USA) to estimate the wingspan (E) and total wing area (A). Considering gravity's acceleration, body mass (M) was calculated as the average body weight (AM) multiplied by 9.81 ms2. With these three data, we calculated the relative wing loading (rWL) as rWL= (M/A)/(AM1/3) and the aspect ratio (AR) as AR= E2/A. For insectivorous species that we did not capture in the field, we took these data from specimens deposited in the “Alberto Cadena García” Mammal Collection at Universidad Nacional de Colombia. We measured at least five individuals per species collected in each study polygon. We prioritized specimens preserved in liquid, recently captured, with data about weight at the time of capture. In the case of dry-preserved individuals, the softening of the wings was made following Andrade et al. (2013). Finally, for those insectivorous species not represented in that mammal collection, data were taken from the literature. In total, we analyzed pictures from 302 individuals of 30 species, where 88 were taken from seven species deposited at the “Alberto Cadena García” mammalogy collection and 214 from individuals captured during fieldwork. Measures of the four additional species are taken from the literature.
We established six categories for a diet based on literature: nectarivore, insectivore, frugivore, omnivore, sanguinivore, and piscivore/insectivore (Ramírez-Chaves et al., 2008; Ríos-Blanco and Pérez-Torres, 2015). For the trophic level, we established two broad categories: phytophagous and animalivorous. We determined vertical stratification for phyllostomid and mormoopid bats following Farneda et al. (2015), where we considered three categories: understory, canopy, and no preference. For insectivorous bat species, we followed Kalko et al. (1996) and Marques et al. (2016), where we established two categories for vertical stratification: canopy and under canopy. We considered FME and pulse structure for insectivorous bats following Schnitzler and Kalko (2001), Wordley et al. (2017), and Núñez et al. (2019). We measured these two echolocation variables from a maximum of five recordings per species per hour in 5-s files. Data for FME were estimated for all the species identified. In the case of similar species grouped in sonotypes, we calculated the average FME of all species within each sonotype. We established four categories for pulse structure: (1) constant frequency (CF), usually emitted by species associated with highly cluttered space close to vegetation or the ground, (2) quasi-constant frequency (qCF) emitted by open-space insectivores, (3) frequency modulated (FM) emitted by background-cluttered space aerial insectivores, and (4) frequencymodulated quasi-constant frequency (FM-qCF) emitted by aerial insectivores in highly cluttered spaces.
2.4. Environmental conditions of dry zones
In total, we measured five environmental variables. We included two climatic variables: daily maximum temperature and wind speed. We also considered three landscape variables: total area of water bodies, distance from sampling sites to water bodies, and probability of finding caves. Finally, we estimated the conservation status of each sampled polygon.
Data for maximum temperature were obtained from the closest hydrometeorological stations to the sampling points (CENICAFE, 2021; DIMAR, 2021; IDEAM, 2022). We estimated the median for the single daily maximum temperature value reported from all sampled nights in each polygon. For wind speed, we calculated the median from values obtained every 10 min during each sampling night (18:00–00:00) in each polygon.
We used ESRI ArcMap 10.2 and ERDAS Imagine 10.0 software based on 2020 Sentinel-2A satellite images (10 and 20 m resolution) to measure the landscape variables, such as the total area of water bodies (ha) in each sampling polygon and the distance from sampling sites to water bodies. To determine the probability of finding caves, we identified the presence of caves, sinkholes, karst terrain, coastal erosion, and falling rocks, following Galvis-Gómez (2018) and Posada (2008). Using the algorithm Near in ArcGIS 10.2 software, we estimated the minimum distance of each sampling point to any of the geomorphological features previously mentioned. Once the distances were calculated, we determined the probability of finding caves by establishing four categories based on the Jenks Natural Breaks algorithm in ArcGIS 10.2 (Beyer, 2004): low (0.0 to 1.0 values), medium (2.0 to 3.0 values), high (3.0 to 4.0 values), and very high (higher than 4.0) in each sampling polygon.
We determined the conservation status in each polygon based on the land-use intensity factor in Colombia, following Correa Ayram et al. (2020). To estimate this factor, we considered variables, such as land use, human population density, distance to roads, distance to human settlements, and vegetation percentage (detailed information in Díaz-Beltrán, 2021). All these variables were estimated using ESRI ArcMap 10.2 and ERDAS Imagine 10.0 software based on 2020 Sentinel-2A satellite images (10 and 20 m resolution). Values closer to 0 for the intensity factor indicate low anthropogenic alteration, while values close to 5 indicate higher anthropogenic pressure.
2.5. Data analysis
2.5.1. Bat functional traits and environmental conditions
The relationships between bat functional traits and environmental variables were evaluated using the RLQ and the fourth-corner analysis. The RLQ analysis is a multivariate ordination method that analyzes the covariance between functional traits of the species (Q matrix) and environmental variables (R matrix) mediated by the abundance or occurrence of the species (L matrix). We analyzed table L through a correspondence analysis (CA) and the R and Q matrix through a principal component analysis (PCA). Both PCAs were made following the Hill–Smith method, which allows the inclusion of qualitative and quantitative variables (Legendre et al., 1997). We used the fourth-corner analysis to test the significance between bivariate associations (Dolédec et al., 1996; Dray et al., 2014), such as the relationships between functional traits and environmental variables. The significance of the relationship between bat functional traits and environmental conditions was assessed based on 49,999 permutations. For this analysis, we test model 6, which adjusts the species according to their traits and the imposition of the environment, and it also corrects the level of type 1 error (Dray et al., 2014). We used model 6 which is a combination of model 2 and model 4. Model 2 tests the hypothesis that the distribution of species with fixed traits is not influenced by the environmental conditions, and model 4 tests the hypothesis that the species composition of samples with fixed environmental conditions is not influenced by the species characteristics. Finally, the analysis uses chi-square tests for qualitative variables and Pearson's tests for quantitative variables with an alpha value = 0.05.
We performed RLQ and the fourth-corner analyses for two data sets: one for phyllostomid and mormoopid bats and the other for insectivorous bat species (Emballonuridae, Molossidae, Mormoopidae, Natalidae, Noctilionidae, and Vespertilionidae families). For phyllostomid and mormoopid bats, the matrix L was built using the bat abundance estimated from mist-netting sampling, and traits, such as FME and pulse structure, were not included in the matrix Q. Due to the difficulty in estimating the number of insectivorous bats from passive acoustic sampling, the matrix L was based on the presence–absence of these species. Because all insectivorous bat species have the same trophic level (i.e., animalivorous), this variable was not considered for insectivorous bats. However, in matrix Q, we included functional traits associated with their echolocation calls: FME and call structure. All these analyses were carried out using the ade4 and FD package (Dray and Dufour, 2007; Laliberté et al., 2014) in R version 4.1.1 (R Core Team, 2021).
3. Results
We captured 360 individuals belonging to 14 species with mist nets (Phyllostomidae and Mormoopidae), and we identified 18 species and 6 sonotypes with acoustic sampling (Emballonuridae, Mormoopidae, Molossidae, Natalidae, Noctilionidae, and Vespertilionidae) (Supplementary Table S1).
3.1. Environmental conditions in dry zones
The daily maximum temperature varied between 24 and 34°C, and the wind speed varied between 20 and 33 km/h. The Santa Marta polygon had the highest temperature, while Jordán had the lowest wind speed (Table 2). Regarding the landscape variables, Puerto Colombia had the highest total area covered by water and the shortest average distance between water bodies. In contrast, Cartagena had the largest distance between water bodies. On the other hand, the probability of finding caves was higher in the Jordán and Lagunetas polygons (Table 2). In general, conservation status varied from 0.4 in Piojó to 4.2 in Puerto Colombia. Except for Puerto Colombia, all sampled polygons exhibited good conservation status (Table 2).
3.2. Relation between bat functional traits and environmental conditions
For phyllostomid and mormoopid bats, the first and second axes of the RLQ analysis explained 89.1 and 8.1% of the association between the traits and environmental variables (Table 3A), whereas, for insectivorous bats, they explained 79.6 and 18.9% (Table 3C). The first axis in the Hill–Smith PCA of the tables R (environmental variables) and Q (traits) explained 52.8 and 39.8% for phyllostomid and mormoopid bats and 43.6 and 30.4% for insectivorous bats. Regarding Table L (abundance and presence per site), the first axis of the correspondence analysis explained 38.8% of the variance for phyllostomid and mormoopid bats and 33.4% for insectivorous bats (Tables 3B, D).
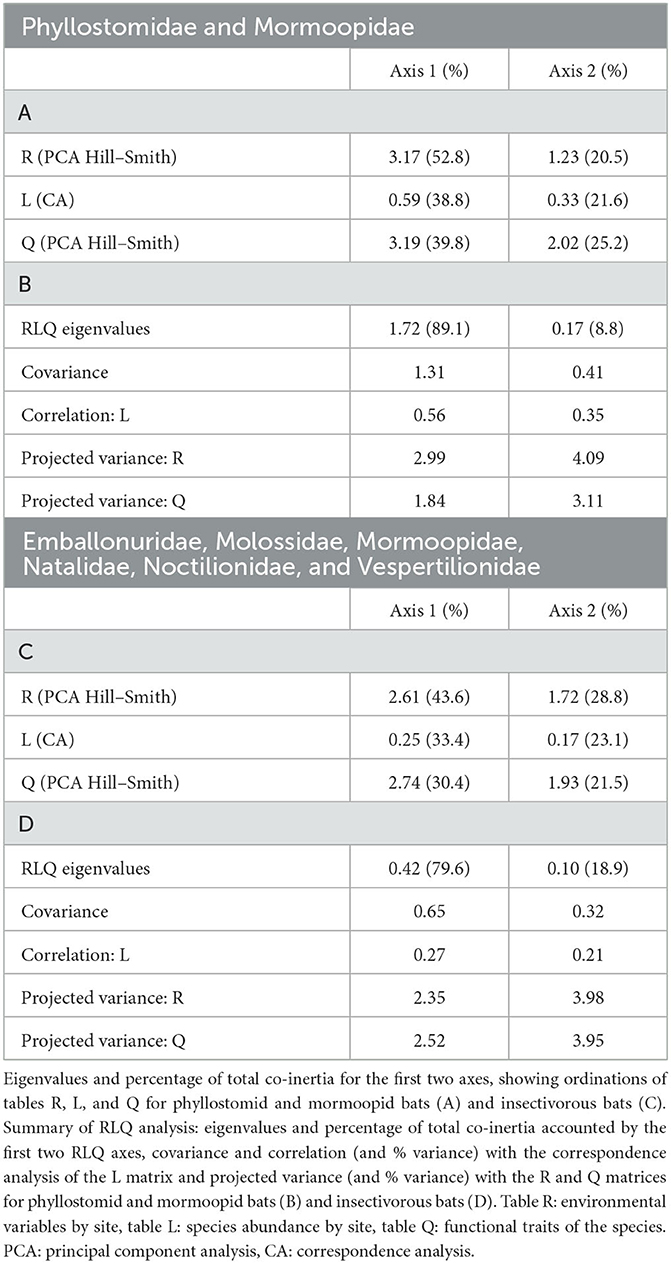
Table 3. Results for the RLQ analysis using bat functional traits and environmental variables in Colombian dry forests.
For phyllostomid and mormoopid bats, Santa Marta and Cartagena polygons formed a distinct group and showed an association with a higher probability of finding caves (Supplementary Figure S1A). The abundance of insectivorous (mostly mormoopids) and nectarivorous bats was related to environmental variables, such as distance to water bodies, temperature, and the probability of finding caves (Figure 2; Supplementary Figures S1A–C). In contrast, frugivorous bats were associated with Piojó and Cepitá polygons, which exhibited higher and intermediate conservation status (Figure 2; Supplementary Figures S1A–C). Regarding the non-phyllostomid insectivorous bats, Santa Marta and Cartagena polygons were grouped and were associated with the highest distance to water bodies (Supplementary Figure S1D). The presence of piscivorous/insectivorous bat species that exhibit an FM-qCF call type was related to a larger area covered by water. This pattern was also observed for insectivorous species with CF pulse structure (Figure 2; Supplementary Figures S1D–F). Insectivorous bat species with qCF pulse structure (molossids and emballonurids) that predominantly forage in the canopy were associated with more distant water bodies (Figure 2; Supplementary Figures S1D–F).
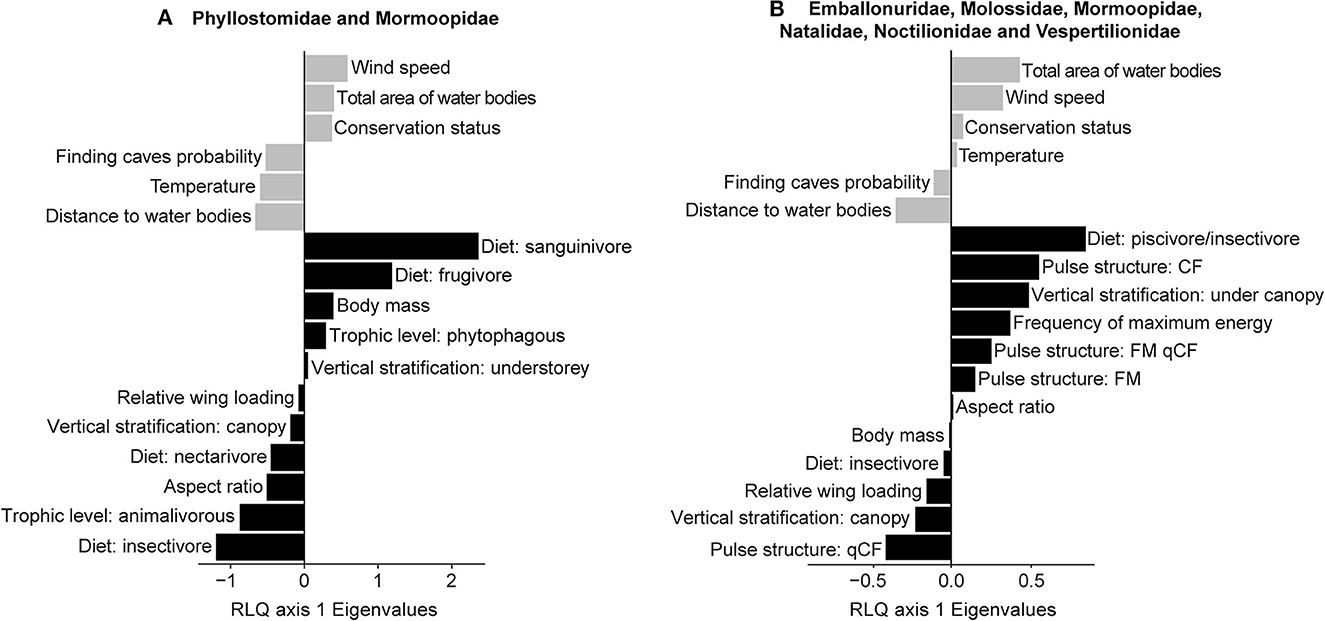
Figure 2. Plot from the RLQ analysis between bat functional traits and environmental variables along RLQ axis 1 in Colombian dry forests. (A) Phyllostomidae and Mormoopidae. (B) Insectivorous bats: Emballonuridae, Molossidae, Mormoopidae, Natalidae, Noctilionidae, Vespertilionidae. Gray: environmental variables. Black: bat species traits.
According to the global test of the fourth-corner analysis, we did not find strong evidence to suggest that species composition depends on the environmental conditions (model 2, p = 0.48) or the species traits (model 4, p = 0.5) for phyllostomid and mormoopid bats. However, there were significant and positive relationships between a higher abundance of hematophagous bats and nearest water bodies and animalivorous bats and a higher and intermediate probability of finding caves (Figure 3). For insectivorous bat species, the global test of the fourth-corner analysis did not find strong evidence to suggest that species composition depends on environmental conditions (model 2, p = 0.37) but showed evidence for the association between species composition and functional traits (model 4, p < 0.001). Nevertheless, there were significant and positive relationships between more conserved sites and the presence of bats that mostly forage on the canopy and emit qCF calls and between bats that emit qCF pulses with intermediate and smaller areas covered by water (Figure 3).
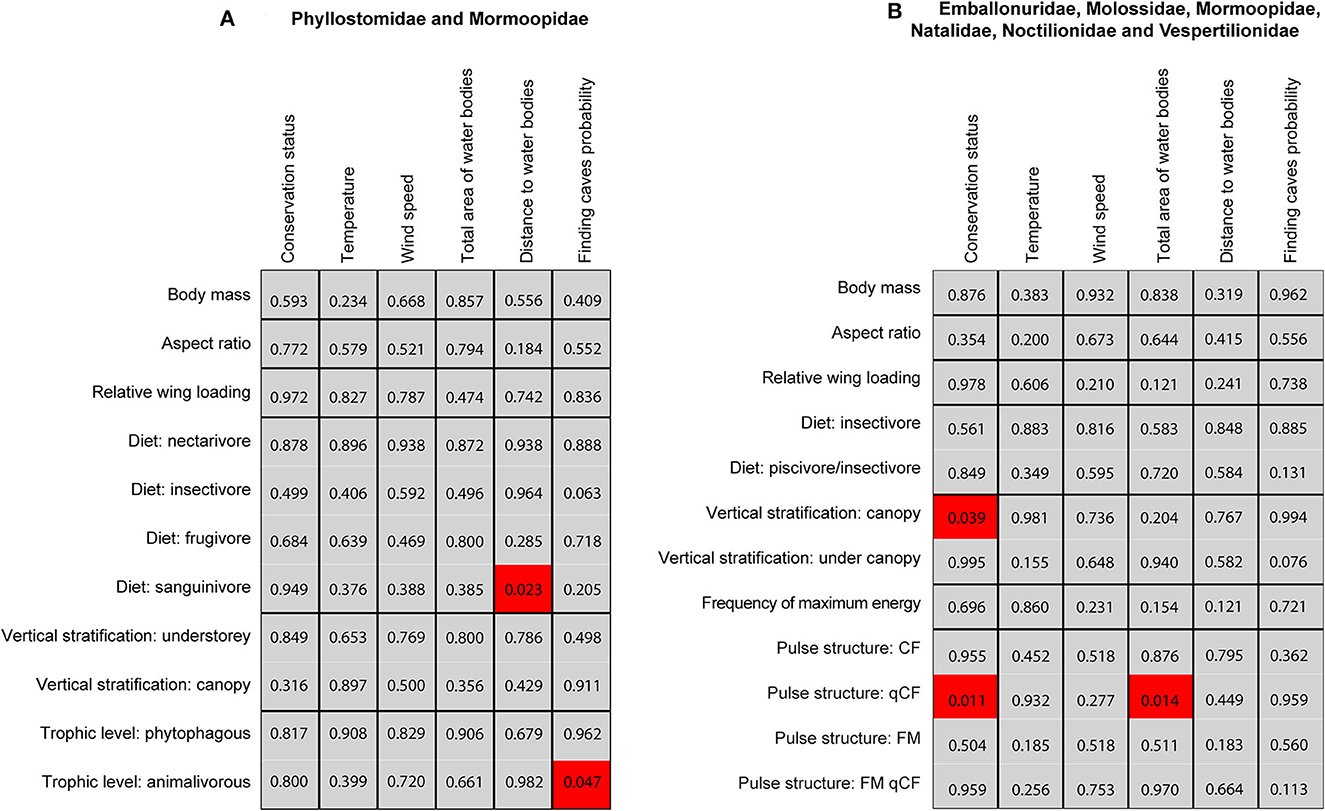
Figure 3. P-values resulting from the fourth-corner test for bivariate associations between bat species traits and environmental variables in Colombian dry forests. (A) Phyllostomidae and Mormoopidae. (B) Insectivorous bats: Emballonuridae, Molossidae, Mormoopidae, Natalidae, Noctilionidae, Vespertilionidae. Red square: significant and positive association; gray square: non-significant association.
4. Discussion
Our findings showed evidence for a significant association between some bat functional traits to landscape variables and land-use intensity, affecting the sensitivity of several bat species. While the fourth-corner analysis did not find evidence that Phyllostomid bats depend on environmental conditions, insectivorous species showed evidence that traits, such as vertical foraging and pulse structure, are related to landscape characteristics and the conservation status of the dry forests. Bat responses to habitat alteration and landscape attributes have been explored more in the Neotropical rainforest ecosystems, and most of them focused on Phyllostomid bats (Farneda et al., 2015, 2020; Meyer et al., 2016). Few studies include data about the functional traits of aerial insectivorous bats and their vulnerability to habitat modification (Núñez et al., 2019). Therefore, our study brings essential information about the sensitivity of both bat groups to habitat disturbances in highly threatened ecosystems like dry forests.
In line with our results, other bat studies in tropical dry ecosystems have found significant relationships between bats' functional traits, environmental conditions, and landscape attributes. Functional traits, such as wing morphology and body size, were related to environmental characteristics such as vertical vegetation stratification (Olaya-Rodrigúez et al., 2019), plant cover type (García-Herrera et al., 2020), and vegetation structure and composition (Martínez-Ferreira et al., 2020). Similarly, in the Amazonian rainforest, trophic level and body mass are good predictors for phyllostomid bats (Farneda et al., 2015). In contrast, for aerial insectivorous bats, the call structure is a functional trait more susceptible to forest fragmentation (Núñez et al., 2019).
In contrast to our prediction, we did not find evidence that the abundance of animalivorous bats was associated with more conserved areas and water bodies' closeness. This pattern could be explained because we captured only five animalivorous species: one phyllostomid and four mormoopids. However, animalivorous bats were positively associated with the probability of finding caves. Caves take relevance in dry forests characterized by extreme climatic conditions because these roosts offer protection against temperature fluctuations and predators and support reproduction, breeding, and other social behaviors (Kunz, 1982). Similarly, other studies show that mormoopids have a strong dependence on caves in Neotropical dry forests or semi-arid ecosystems, where they form large colonies (Bonaccorso et al., 1992; Rodríguez-Durán, 2009; Torres-Flores and López-Wilchis, 2018; Otálora-Ardila et al., 2020), which may reach >10,000 individuals, as registered in our study. Although ca. 40% of worldwide bat species use subterranean habitats as roosts (Frick et al., 2020), it is possible that some species rarely or never use caves as shelter. Consequently, bats that avoid caves could bias the relationship between this landscape feature and the species' composition and traits. However, 70% of the species registered through mist nets and passive acoustic monitoring are considered primary and regular cave-dwelling bats (Arita, 1993).
As expected, we found a positive association between more conserved sites and species traits. Our data showed a positive relationship between the presence of aerial insectivorous bats that mostly forage on the canopy and that emit qCF calls. The polygons with better conservation status were characterized by having more vegetation cover, less intense land use, low population density, and being more distant to populated centers. Similarly, in islands formed by hydroelectric dams in Central Brazilian Amazon and East Asia, species with qCF pulse structure were associated with less disturbed environments like continuous forests and with larger and more connected islands and forest patches (López-Bosch et al., 2022; Colombo et al., 2023). However, other studies have found that bats that emit qCF pulses seem to benefit from fragmentation and the decrease in the size of islands and forest patches (Núñez et al., 2019; Hazard et al., 2023).
Vertical foraging niche as a variable that reflects the impact of habitat transformation has been previously tested in phyllostomid and aerial insectivorous bats. While it had low support for phyllostomids (Farneda et al., 2015), it had a significant relationship with insectivorous that emit qCF and FM-qCF (Núñez et al., 2019). However, it was unclear which vertical stratum was more closely related to insectivorous bat species' foraging activity or pulse structure (Núñez et al., 2019). In our study, the presence of insectivorous bats that emit qCF pulses was positively associated with foraging at the canopy. Similarly, other studies suggest that the activity and richness of insectivorous bats increase at the canopy (Marques et al., 2016; Rojo Cruz et al., 2019), with most of the recorded species emitting qCF calls (Marques et al., 2016). The association between bats that emit qCF calls and canopy is likely due to an increase in insect food resources at this stratum (Jung et al., 2012; Marques et al., 2016). Although insect availability is higher at the canopy in tropical rainforests (Basset et al., 2003; De Souza Amorim et al., 2022), there is scarce and contrasting information on this issue in dry forests (Vega-Badillo et al., 2018; Camero-Rubio et al., 2021). Open spaces between tree crowns at the canopy result in less cluttered habitats, which are more suitable for foraging and flight maneuverability (Marques et al., 2016). Also, there are fewer multidirectional reflections of echolocation calls in uncluttered spaces (Brigham et al., 1997). Therefore, bats tend to make less effort to discriminate between prey and background echoes (Brigham et al., 1997), which could be particularly advantageous for species that emit qCF pulses.
Bats use water bodies for foraging (Ciechanowski, 2002; Razgour et al., 2010), and in dry environments where water is highly fluctuating, it could be an essential resource and determinant in bat survival and diversity (Korine et al., 2016). According to our predictions, places with easier access to water would be critical to maintaining species traits, where we found a significant and positive relationship between a higher abundance of hematophagous bats and the nearest water bodies. Similar patterns have been documented in dry forests in Mexico, where the sanguinivore species Desmodus rotundus was more abundant in pastures close to water-filled sinkholes and riparian forests (MacSwiney et al., 2007; Avila-Cabadilla et al., 2012; Mendoza-Sáenz et al., 2021). Riparian forests and other water resources could promote mobility and provide food and water to native and domestic mammals (Polania Ortiz, 2012; Pineda-Cendales et al., 2020). Therefore, the probability that D. rotundus find food resources near water might increase (Ávila-Flores et al., 2019). Additionally, livestock management observed in the sampled places indicated that cattle or goats frequently use riparian vegetation, streams, or artificial water bodies (Botero et al., 2009).
Our data also indicated that environmental variables such as the total area covered by water are essential to support species traits, where we found a significant and positive relationship between the occurrence of bats that emit qCF pulses and intermediate and smaller areas covered by water. Also, we observed an association between the biggest areas covered by water with the presence of piscivorous/insectivorous bats that emit FM-qCF calls and insectivorous bats with CF pulse structure, although these relationships were not significant. Studies have shown that the richness and activity of insectivorous bats are positively associated with the increment in the size of water bodies in deserts and temperate and tropical forests (MacSwiney et al., 2009; Razgour et al., 2010; López-González et al., 2015; Torrent et al., 2018). It has been suggested that the biggest water bodies could be related to higher insectivorous bats' richness and activity because they could offer more food insect availability (López-González et al., 2015; Straka et al., 2020), larger area for drinking water (Razgour et al., 2010), and more opportunities for the spatial partitioning (MacSwiney et al., 2009; Razgour et al., 2010). Although we did not evaluate the insect diversity, some insects show higher richness in riparian forests or habitats with higher water availability in tropical dry forests (Altamiranda-Saavedra, 2009; Vargas-Zapata et al., 2011; Peña and Reinoso, 2016; Casas-Pinilla et al., 2017; Vega-Badillo et al., 2018). Our results revealed that intermediate or smaller areas covered by water showed a high occurrence of bats that emit qCF calls, which reach between 40 and 60% of all insectivorous species registered. This pattern has been previously observed in tropical rainforests and dry forests, where the 38 and 47% were open-space foragers emitting qCF pulses (MacSwiney et al., 2009; Torrent et al., 2018). Despite the general relationship between the higher richness of insectivorous bat species and the size of water bodies, methodological differences hamper the comparison and interpretation among studies due to geographic scale and the criteria to categorize the size and availability of water bodies. In our case, the total amount of water was calculated on an intermediate geographic scale where polygons with lower amounts reached ~11,000 m2 of water. In contrast, other bat studies considered 200 m2 as small water bodies (Razgour et al., 2010; López-González et al., 2015; Torrent et al., 2018).
Although we did not find any significant relationship for frugivores and nectarivores with the environmental conditions, we found a strong association from RLQ analysis between a higher abundance of heavier frugivorous bats with intermediate conservation status and the biggest area covered by water. Notably, we captured more frugivorous bats in areas covered with primary vegetation varied from 66.6 to 83.5% and with artificial water bodies. Association between the frugivore bats with higher forest coverage has been previously observed in dry forests in Mexico (Avila-Cabadilla et al., 2012) and in Colombia (Ballesteros-Correa and Pérez-Torres, 2022). Frugivorous bats were positively associated with open dry forest and non-forest metrics in Guatemala (Chambers et al., 2016). The association between the higher abundance of frugivore species with more native vegetation and riparian forests could be explained by the high quantity of plants that produce fruits (Avila-Cabadilla et al., 2012) and offer roosts in the foliage (Martínez-Ferreira et al., 2020).
Our data indicated an association between a higher abundance of nectarivorous species with high and intermediate conservation areas and an intermediate probability of finding roosts. Nectarivorous bats are commonly registered in early-stage vegetational succession (~30 years of regeneration) (Farneda et al., 2018) and in open areas and forest edges (Avila-Cabadilla et al., 2014; Otálora-Ardila and López-Arévalo, 2021). Therefore, a higher abundance of nectarivores in intermediate conservation areas could be associated with the preference of species such as Glossophaga soricina, G. longirostris, and Leptonycterys curasoae for foraging in less complex habitats, such as xeric zones, thorn scrub, and arid grasslands (Alvarez et al., 1991; Cole and Wilson, 2006). Due to Glossophaga spp. and Leptonycteris spp. rely on a variety of sensory abilities such as olfactory and visual cues, spatial working memory, and echolocation (Henry and Stoner, 2011; Clare et al., 2014; Moreira-Hernández et al., 2021) to find the floral resources, the preference for foraging in open or less structured ecosystems could facilitate the task of food searching rather than indicating a preference for disturbed habitats. Nectarivore species were associated with an intermediate probability of finding roosts, determined in part by the roosts found. While Glossophaga spp. are considered regular cave dwellers, L. curasoae is a cave-dependent bat (Arita, 1993), where they aggregate in numerous colonies and use them as a nursery (Simal et al., 2015, 2022). Therefore, caves are a vital element that might be associated with high bat abundance, particularly for L. curasoae.
We observed differences in species traits between the Caribbean coast and the Chicamocha River Basin. For phyllostomid and mormoopid bats, the abundance of heavier frugivorous bats was higher in the Chicamocha than in the Caribbean. This pattern might be related to the fact that we captured twice as many individuals of Sturnira lilium (20.1 g) and Artibeus lituratus (65.6 g) in Chicamocha. A similar pattern of species composition in dry forests was observed by Sánchez et al. (2007) and Ballesteros-Correa and Pérez-Torres (2022). These species show changes in niche breadth associated with seasonal food availability in tropical dry forests (Shipley and Twining, 2020). Although they can consume alternative resources, such as pollen/nectar of columnar cacti (Sánchez et al., 2007; MacSwiney et al., 2017), we did not estimate the number of these plants in our study area, but cacti were more abundant in the Chicamocha River Basin than in the Caribbean (Pers. Obs). In contrast, nectarivore and mormoopids were more abundant on the Caribbean coast, particularly in Cartagena's polygon, due to the roosts found, which are used for several nectarivore and mormopid species. Most of these species are considered cave-dwelling bats (Arita, 1993).
Our data indicated that piscivore/insectivore species were mostly recorded in Piojó, Cartagena, and Puerto Colombia, whereas Noctilio leporinus was only recorded in Piojó. This pattern was associated with the larger area covered by water observed on the Caribbean coast, which might offer more resources (MacSwiney et al., 2009; see above, Razgour et al., 2010; López-González et al., 2015; Straka et al., 2020). The insectivore species that forage under the canopy corresponds mainly to mormoopid species that were acoustically registered in the Caribbean polygons and due to several roosts found there. Additionally, we captured three times more phyllostomid and mormoopid bats and recorded more insectivorous species in the Caribbean polygons than in the Chicamocha River Basin. A pattern likely related to the difference in altitude: While the Caribbean coast is between 0 and 199 m.a.s.l., the Chicamocha ranged from 483 to 1,540 m.a.s.l. It is well-documented that Andean bat communities have fewer insectivorous species than lowland forests (Soriano, 2000).
4.1. Potential caveats
Bat sampling covered only the dry season, not gathering information about the climatic variation throughout the year. In dry forests, changes in bat species composition, richness, and abundance were associated with seasonality (Sánchez et al., 2007; Avila-Cabadilla et al., 2014; Ballesteros-Correa and Pérez-Torres, 2022). However, we sampled a wide variety of localities covering a large area in Colombian dry forests and captured a total of 19 species. This species richness was higher or similar to those reported in other studies in this particular ecosystem (Sánchez et al., 2007; Avila-Cabadilla et al., 2014; Martínez-Ferreira et al., 2020). In this context of information loss, we only used presence/absence data of insectivore bat species due to the difficulty in estimating the abundance from passive acoustic sampling. Although our results based on the presence/absence data showed significant trait–environment relationships, the observed patterns could correspond to an underestimation of the community's functional structure. Additionally, some bias may be related to climatic variables, such as temperature and wind speed measured during the bat sampling periods with suitable meteorological conditions, such as no rain or strong winds. Furthermore, we did not include diet-related information for aerial insectivorous bats. Although most studies focusing on functional bat ecology include diet as one relevant trait associated with human-modified landscapes (Cisneros et al., 2016; Farneda et al., 2020), we did not include information about the type of diet due to general lack of information for many insectivorous species (Villalobos-Chaves and Santana, 2022).
4.2. Conservation implications
Our data indicated a positive association between more conserved sites and a more complete set of bat functional traits. Polygons with better conservation status were characterized by having more vegetation cover and less human impact. In the Neotropical dry forests, preserving landscapes that include continuous and large areas is urgent to maintain the ecosystem services bats provide in these ecosystems. Similarly, the conservation and restoration of riparian forests are critical for the long-term preservation of phyllostomid and aerial insectivorous bats in tropical dry forests. In disturbed landscapes, riparian forests have been considered favorable habitats for bats because they offer food and roost resources and promote movement between fragments (Estrada and Coates-Estrada, 2001; Meyer et al., 2016).
Mammals that inhabit dry forests and arid zones must cope with water seasonality and scarcity, particularly during the dry season (Stoner and Timm, 2011). Our data suggested that a large area covered by water was associated with the abundance or presence of many bat species. Therefore, to maintain bat diversity in tropical dry forests, the preservation of water bodies must be promoted. Lakes of different sizes, even the artificial ponds built for livestock management, could sustain and promote several vital resources for insectivores and piscivore/insectivore bats. Large and temporary water bodies are essential because they provide water even during the dry season, when most aquatic resources disappear, have been deviated, drained, or altered. Additionally, larger water areas have the highest probability of remaining, particularly under the current weather changes associated with climate change.
Our data also indicated that caves are essential for the survival of nectarivore and animalivore bats in dry forests. Caves are key landscape elements that are considered biodiversity hotspots (Phelps et al., 2016). The caves in our study area are used by some specialists and vulnerable species that are strongly cave-dependent (e.g., L. curasoae). The large colonies observed in this study of insectivorous bats could be beneficial for nearby agricultural producers due to the significant suppression of insects by bats, including potential crop plagues or human disease vectors (McCracken et al., 2012; Medellín et al., 2017; Puig-Montserrat et al., 2020).
Data availability statement
The original contributions presented in the study are included in the article/Supplementary material, further inquiries can be directed to the corresponding authors.
Author contributions
CD-B, AO-A, and OM conceived and designed the study. AO-A, CD-B, OM, and MV-C designed and developed the fieldwork. AO-A, OM, and HL-A obtained resources and funding acquisition. CD-B drafted the manuscript with edits from OM and AO-A. CD-B, AO-A, and MV-C conducted data curation. All authors contributed to the analysis and writing of the manuscript and approved it for publication.
Funding
This project was funded by the National Geographic Society (Project NGS-61996C-19) to AO-A. The Grupo en Conservación y Manejo de Vida Silvestre of Universidad Nacional de Colombia gave equipment and materials support.
Acknowledgments
We thank PANACHI (Parque Nacional del Chicamocha), Reserva ProAves Cucarachero del Chicamocha, Fundación Batis, and Reserva El Palomar for the logistical support provided, and the staff of Parque Nacional Natural Tayrona and Dirección de Patrimonio y Memoria (Ministerio de Cultura), especially Monica Orduña Monsalve, for the logistical support and help provided. AO-A is grateful for the grant from the National Geographic Society (NGS-61996C-19). We also thank the Grupo en Conservación y Manejo de Vida Silvestre and Universidad Nacional de Colombia, for supporting our research with bats in Colombia, and Hernán Serrano for supporting and advising us on the geographic analysis. Also, we are grateful to Fábio Z. Farneda for his valuable insight.
Conflict of interest
The authors declare that the research was conducted in the absence of any commercial or financial relationships that could be construed as a potential conflict of interest.
Publisher's note
All claims expressed in this article are solely those of the authors and do not necessarily represent those of their affiliated organizations, or those of the publisher, the editors and the reviewers. Any product that may be evaluated in this article, or claim that may be made by its manufacturer, is not guaranteed or endorsed by the publisher.
Supplementary material
The Supplementary Material for this article can be found online at: https://www.frontiersin.org/articles/10.3389/ffgc.2023.1082427/full#supplementary-material
Supplementary Figure S1. Results of the RLQ analysis (two first axis) showing the associations between bat species traits and environmental variables in Colombian dry forests. Eigenvalues and species (eigenvalues showed in the box) for phyllostomidae and mormoopidae (A) and insectivorous bat species (D), principal component analysis (PCA Hill–Smith) showing the covariation of environmental variables for phyllostomidae and mormoopidae (B) and insectivorous bat species (E), and bat's functional traits for phyllostomidae and mormoopidae (C) and insectivorous bat species (F).
Supplementary table S1. Values for the bat species traits in Colombian dry forests. AR, Aspect ratio; rWL, Relative wing loading; VS, Vertical stratification; TL, Trophic level; FME, Frequency of maximum energy; PS, Pulse structure.
References
Aguirre, L. F., Montaño-Centellas, F. A., Gavilanez, M. M., and Stevens, R. D. (2016). Taxonomic and phylogenetic determinants of functional composition of bolivian bat assemblages. PLoS ONE. 11, e0158170. doi: 10.1371/journal.pone.0158170
Albesiano, S., Rangel, C. O., and Cadena, A. (2003). La vegetación del cañón del río Chicamocha (Santander, Colombia). Caldasia 25, 73–99. Available online at: https://revistas.unal.edu.co/index.php/cal/article/view/39402
Albesiano, S., and Rangel-Churio, J. O. (2006). Estructura de la vegetación del Cañón del Río Chicamocha, 500-1200 M; Santander, Colombia: una herramienta para la conservación. Caldasia. 28, 307–325. Available online at: https://revistas.unal.edu.co/index.php/cal/article/view/39291
Almeida, M. H., Ditchfield, A. D., and Tokumaru, R. S. (2014). Habitat characteristics and insectivorous bat activity. Chiropt. Neotrop. 20, 1264–1270.
Altamiranda-Saavedra, M. (2009). Diversidad de libélulas (Insecta-Odonata) para dos usos de suelo, en un bosque seco tropical. Revista Facultad Nacional de Agronomía, Medellín. 62, 5071–5079.
Alvarez, J., Willig, M. R., Jones, J. K., and Webster, W. m. D. (1991). Glossophaga soricina. Mamm. Species. 379, 1–7. doi: 10.2307/3504146
Andrade, C, M. G., Henao Bañol, E. R., and Triviño, P. (2013). Técnicas y procesamiento para la recolección, preservación y montaje de Mariposas en estudios de biodiversidad y conservación. (Lepidoptera: Hesperioidea - Papilionoidea). Revista de la Academia Colombiana de Ciencias Exactas, Físicas y Naturales. 37, 311–325. doi: 10.18257/raccefyn.12
Arias-Aguilar, A., Hintze, F., Aguiar, L. M. S., Rufray, V., Bernard, E., and Pereira, M. J. R. (2018). Who's calling? acoustic identification of Brazilian bats. Mammal Res. 63, 231–253. doi: 10.1007/s13364-018-0367-z
Arias-Cóyotl, E., Stoner, K. E., and Casas, A. (2006). Effectiveness of bats as pollinators of Stenocereus stellatus (Cactaceae) in wild, managed in situ, and cultivated populations in La Mixteca Baja, central Mexico. Am. J. Bot. 93, 1675–1683. doi: 10.3732/ajb.93.11.1675
Arita, H. T. (1993). Conservation biology of the cave bats of Mexico. J. Mammal. 74:693–702. doi: 10.2307/1382291
Avila-Cabadilla, L. D., Sanchez-Azofeifa, G. A., Stoner, K. E., Alvarez-Añorve, M. Y., Quesada, M., and Portillo-Quintero, C. A. (2012). Local and landscape factors determining occurrence of phyllostomid bats in tropical secondary forests. PLoS ONE. 7, e35228. doi: 10.1371/journal.pone.0035228
Avila-Cabadilla, L. D., Stoner, K. E., Nassar, J. M., Espírito-Santo, M. M., Alvarez-Añorve, M. Y., Aranguren, C. I., et al. (2014). Phyllostomid bat occurrence in successional stages of Neotropical dry forests. PLoS ONE. 9, e84572. doi: 10.1371/journal.pone.0084572
Ávila-Flores, R., Bolaina-Badal, A. L., Gallegos-Ruiz, A., and Sánchez-Gómez, W. S. (2019). Use of linear features by the common vampire bat (Desmodus rotundus) in a tropical cattle-ranching landscape. Therya. 10, 229–234. doi: 10.12933/therya-19-890
Azhar, B., Puan, C. L., Aziz, N., Sainuddin, M., Adila, N., Samsuddin, S., et al. (2015). Effects of in situ habitat quality and landscape characteristics in the oil palm agricultural matrix on tropical understory birds, fruit bats and butterflies. Biodivers. Conserv. 24, 3125–3144. doi: 10.1007/s10531-015-1005-6
Ballesteros-Correa, J., and Pérez-Torres, J. (2022). Silvopastoral and conventional management of extensive livestock and the diversity of bats in fragments of tropical dry forest in Córdoba, Colombia. Agroforest. Syst. 96, 589–601. doi: 10.1007/s10457-021-00698-4
Basset, Y., Hammond, P. M., Barrios, H., Holloway, J. D., and Miller, S. E. (2003). “Vertical stratification of arthropod assemblages,” in: Arthropods of Tropical Forests Spatio-Temporal Dynamics and Resource Use in the Canopy, Eds Y. Basset et al. (Cambridge Univ. Press).
Beyer, H. L. (2004). Geospatial Analysis, 6th Edn. Available online at http://www.spatialecology.com/htools (accessed September 30, 2022).
Bonaccorso, F. J., Arends, A., Genoud, M., Cantoni, D., and Morton, T. (1992). Thermal ecology of moustached and ghost-faced bats (Mormoopidae) in Venezuela. J. Mammal. 73:365–378. doi: 10.2307/1382071
Botero, L., De La Ossa, V. J, Espitia, A., and De La Ossa-Lacayo, A. (2009). Importancia de los jagüeyes en las sabanas del caribe colombiano. Rev. Colombiana Cienc. Anim. 1, 71–84. doi: 10.24188/recia.v1.n1.2009.413
Brigham, R. M., Grindal, S. D., Firman, M. C., and Morissette, J. L. (1997). The influence of structural clutter on activity patterns of insectivorous bats. Can. J. Zool. 75, 131–136. doi: 10.1139/z97-017
Camero-Rubio, E., Cárdenas, J. P., and Marin, B. J. M (2021). A vertical stratification of arthropods in the dry ecosystems of Colombian Guyana: morphological patterns and their ecological implications. Revista de Biología Tropical. 69, 1289–1305. doi: 10.15517/rbt.v69i4.48024
Casas-Pinilla, L. C., Mahecha-J, O., Dumar-R, J. C., and Ríos-Málaver, I. C. (2017). Diversidad de mariposas en un paisaje de bosque seco tropical, en la Mesa de los Santos, Santander, Colombia (Lepidoptera: Papilionoidea). SHILAP Revista de Lepidopterologia. Available online at: https://www.redalyc.org/journal/455/45550375010/html/
Castillo-Figueroa, D. (2020). Why bats matters: a critical assessment of bat-mediated ecological processes in the neotropics. Eur. J. Ecol. 6, 77–101. doi: 10.17161/eurojecol.v6i1.13824
CENICAFE (2021). Centro Nacional de Investigaciones de Café. Available online at: https://www.cenicafe.org/es/index.php/nuestras_publicaciones/anuarios_meteorologicos/publicaciones_anu-2020 (accessed January, 2022).
Chambers, C. L., Cushman, S. A., Medina-Fitoria, A., Martínez-Fonseca, J., and Chávez-Velásquez, M. (2016). Influences of scale on bat habitat relationships in a forested landscape in Nicaragua. Landsc. Ecol. 31, 1299–1318. doi: 10.1007/s10980-016-0343-4
Ciechanowski, M. (2002). Community structure and activity of bats (Chiroptera) over different water bodies. Mamm. Biol. 67, 276–285. doi: 10.1078/1616-5047-00042
Cisneros, L. M., Fagan, M. E., and Willig, M. R. (2016). Environmental and spatial drivers of taxonomic, functional, and phylogenetic characteristics of bat communities in human-modified landscapes. PeerJ. 4, e2551 doi: 10.7717/peerj.2551
Clare, E. L., Goerlitz, H. R., Drapeau, V. A., Holderied, M. W., Adams, A. M., Nagel, J., et al. (2014). Trophic niche flexibility in G lossophaga soricina : how a nectar seeker sneaks an insect snack. Funct. Ecol. 28, 632–641. doi: 10.1111/1365-2435.12192
Cole, F. R., and Wilson, D. E. (2006). Leptonycteris curasoae. Mamm. Species. 796, 1–3. doi: 10.1644/796.1
Colombo, G. T., Di Ponzio, R., Benchimol, M., Peres, C. A., and Bobrowiec, P. E. D. (2023). Functional diversity and trait filtering of insectivorous bats on forest islands created by an Amazonian mega dam. Func. Ecol. 37, 99–111. doi: 10.1111/1365-2435.14118
Conenna, I., Santini, L., Rocha, R., Monadjem, A., Cabeza, M., and Russo, D. (2021). Global patterns of functional trait variation along aridity gradients in bats. Glob. Ecol. Biogeogr. 30, 1014–1029. doi: 10.1111/geb.13278
Correa Ayram, C. A., Etter, A., Díaz-Timoté, J., Rodríguez Buriticá, S., Ramírez, W., and Corzo, G. (2020). Spatiotemporal evaluation of the human footprint in Colombia: four decades of anthropic impact in highly biodiverse ecosystems. Ecol. Indic. 117, 106630. doi: 10.1016/j.ecolind.2020.106630
De Souza Amorim, D., Brown, B. V., Boscolo, D., Ale-Rocha, R., Alvarez-Garcia, D. M., Balbi, M. I. P. A., et al. (2022). Vertical stratification of insect abundance and species richness in an Amazonian tropical forest. Sci. Rep. 12:1734. doi: 10.1038/s41598-022-05677-y
Díaz-Beltrán, C. (2021). Patrones de diversidad funcional de murciélagos en zonobiomas secos del norte de Colombia. Universidad Nacional de Colombia.
Dolédec, S., Chessel, D., ter Braak, C. J. F., and Champely, S. (1996). Matching species traits to environmental variables: a new three-table ordination method. Environ. Ecol. Stat. 3, 143–166. doi: 10.1007/BF02427859
Dray, S., Choler, P., Dolédec, S., Peres-Neto, P. R., Thuiller, W., Pavoine, S., et al. (2014). Combining the fourth-corner and the RLQ methods for assessing trait responses to environmental variation. Ecology. 95, 14–21. doi: 10.1890/13-0196.1
Dray, S., and Dufour., A. (2007). The ade4 package: implementing the duality diagram for ecologists. J. Stat. Softw. 22, 1–20. doi: 10.18637/jss.v022.i04
Estrada, A., and Coates-Estrada, R. (2001). Bat species richness in live fences and in corridors of residual rain forest vegetation at Los Tuxtlas, Mexico. Ecography. 24, 94–102. doi: 10.1034/j.1600-0587.2001.240111.x
Farneda, F. Z., Meyer, C. F. J., and Grelle, C. E. V. (2020). Effects of land-use change on functional and taxonomic diversity of Neotropical bats. Biotropica. 52, 120–128. doi: 10.1111/btp.12736
Farneda, F. Z., Rocha, R., López-Baucells, A., Groenenberg, M., Silva, I., Palmeirim, J. M., et al. (2015). Trait-related responses to habitat fragmentation in Amazonian bats. J. Appl. Ecol. 52, 1381–1391. doi: 10.1111/1365-2664.12490
Farneda, F. Z., Rocha, R., López-Baucells, A., Sampaio, E. M., Palmeirim, J. M., Bobrowiec, P. E. D., et al. (2018). Functional recovery of Amazonian bat assemblages following secondary forest succession. Biol. Conserv. 218, 192–199. doi: 10.1016/j.biocon.2017.12.036
Frick, W. F., Kingston, T., and Flanders, J. (2020). A review of the major threats and challenges to global bat conservation. Ann. N. Y. Acad. Sci. 1469, 5–25. doi: 10.1111/nyas.14045
Galvis-Gómez, M. A. (2018). Mapa del potencial kárstico del departamento de Santander. Available online at: https://repository.unimilitar.edu.co/handle/10654/20429
García-Herrera, L. V., Ramírez-Fráncel, L. A., Losada-Prado, S., Reinoso-Flórez, G., Villa-Navarro, F. A., and Guevara, G. (2020). Functional traits of bats associated with the use of wetlands in Colombian tropical dry forests. 22, 283–294. doi: 10.3161/15081109ACC2020.22.2.005
García-Morales, R., Badano, E. I., and Moreno, C. E. (2013). Response of Neotropical bat assemblages to human land use. Conserv. Biol. 27, 1096–1106. doi: 10.1111/cobi.12099
García-Morales, R., Moreno, C. E., Badano, E. I., Zuria, I., Galindo-González, J., Rojas-Martínez, A. E., et al. (2016). Deforestation impacts on bat functional diversity in tropical landscapes. PLoS ONE. 11, e0166765. doi: 10.1371/journal.pone.0166765
Hazard, Q. C., Froidevaux, J. S., Yoh, N., Moore, J., Senawi, J., Gibson, L., et al. (2023). Foraging guild modulates insectivorous bat responses to habitat loss and insular fragmentation in peninsular Malaysia. Biol. Conserv. 281, 110017. doi: 10.1016/j.biocon.2023.110017
Henry, M., and Stoner, K. E. (2011). Relationship between spatial working memory performance and diet specialization in two sympatric nectar bats. PLoS ONE. 6, e23773. doi: 10.1371/journal.pone.0023773
Hernández-Camacho, J., Hurtado, A., Walschburger, T., and Ortiz Quijano, R. (1992). Unidades Biogeográficas de Colombia. La Diversidad Biológica de Iberoamérica I. Available online at: http://www1.inecol.edu.mx/publicaciones/Biodiv/bdcolbig.htm
IDEAM (2022). Instituto de Hidrología, Meteorología y Estudios Ambientales. Available online at: http://dhime.ideam.gov.co/atencionciudadano (accessed January, 2022).
Jung, K., Kaiser, S., Böhm, S., Nieschulze, J., and Kalko, E. K. V. (2012). Moving in three dimensions: effects of structural complexity on occurrence and activity of insectivorous bats in managed forest stands. J. Appl. Ecol. 49, 523–531. doi: 10.1111/j.1365-2664.2012.02116.x
Jung, K., and Kalko, E. K. V. (2011). Adaptability and vulnerability of high flying Neotropical aerial insectivorous bats to urbanization. Divers. Distrib. 17, 262–274. doi: 10.1111/j.1472-4642.2010.00738.x
Jung, K., and Threlfall, C. G. (2018). Trait-dependent tolerance of bats to urbanization: a global meta-analysis. Proc. R. Soc. B: Biol. Sci. 285, 20181222. doi: 10.1098/rspb.2018.1222
Kahnonitch, I., Lubin, Y., and Korine, C. (2018). Insectivorous bats in semi-arid agroecosystems – effects on foraging activity and implications for insect pest control. Agric. Ecosyst. Environ. 261, 80–92. doi: 10.1016/j.agee.2017.11.003
Kalko, E. K. V., Handley, C. O., and Handley, D. (1996). “Organization, diversity, and long-term dynamics of a Neotropical bat community.” in Long-Term Studies of Vertebrate Communities, eds M. Cody and J. Smallwood (San Diego, CA: Academic Press), 503–553. doi: 10.1016/B978-012178075-3/50017-9
Korine, C., Adams, R., Russo, D., Fisher-Phelps, M., and Jacobs, D. (2016). “Bats and water: anthropogenic alterations threaten global bat populations.” in: Bats in the Anthropocene: Conservation of Bats in a Changing World, Eds C. Voigt, T. Kingston (Springer, Cham). doi: 10.1007/978-3-319-25220-9_8
Kunz, T. H. (1982). “Roosting ecology of bats.” in: Ecology of Bats, ed T. H. Kunz (New York, NY: Plenum Press), 425.
Laliberté, E., Legendre, P., and Shipley, B. (2014). FD: Measuring Functional Diversity from Multiple Traits, and Other Tools for Functional Ecology. R Package Version 1.0-12.
Latorre, J. P., Jaramillo, R O, and Corredor, G L. (2014). Atlas del Sistema Nacional de Áreas Protegidas Continentales en Colombia. Parques Nacionales de Colombia. Available online at: https://www.parquesnacionales.gov.co/portal/wp-content/uploads/2019/07/ATLAS-DEL-SISTEMA-NACIONAL-DE-AREAS-PROTEGIDAS.pdf (accessed June 30, 2022).
Legendre, P., Galzin, R., and Harmelin-Vivien, M. L. (1997). Relating behavior to habitat: solutions to the fourth-corner problem. Ecology. 78, 547–562.
Lloyd, A., Law, B., and Goldingay, R. (2006). Bat activity on riparian zones and upper slopes in Australian timber production forests and the effectiveness of riparian buffers. Biol. Conserv. 2, 207–220. doi: 10.1016/j.biocon.2005.10.035
Locatelli, E., Machado, I. C., and Medeiros, P. (1997). Floral Biology and Bat Pollination in Pilosocereus catingicola (Cactaceae) in Northeastern Brazil. 1997, 28–34. doi: 10.25223/brad.n15.1997.a3
López-Baucells, A., Rocha, R., Bobrowiec, P. E. D., Bernard, E., Palmeirim, J. M., and Meyer, C. F. J. (2016). Field Guide to Amazonian Bats. Manaus, Brasil: INPA. Available online at: https://www.researchgate.net/publication/307631357_Field_Guide_to_Amazonian_Bats
López-Bosch, D., Rocha, R., López-Baucells, A., Wang, Y., Si, X., Ding, P., et al. (2022). Passive acoustic monitoring reveals the role of habitat affinity in sensitivity of sub-tropical East Asian bats to fragmentation. Remot. Sens. Ecol. Conserv. 8, 208–221.
López-González, C., Gómez-Ruiz, E. P., Lozano, A., and López-Wilchis, R. (2015). Activity of insectivorous bats associated with cattle ponds at la Michilía biosphere reserve, Durango, Mexico: Implications for conservation. Acta Chiropterologica. 17, 117–129. doi: 10.3161/15081109ACC2015.17.1.010
MacSwiney, G. M. C, Bolívar-Cimé, B., Alfaro-Bates, R., Ortíz-Díaz, J. J., Clarke, F. M., et al. (2017). Pollen movement by the bat Artibeus jamaicensis (Chiroptera) in an agricultural landscape in the Yucatan Peninsula, Mexico. Mamm. Res. 62, 189–193. doi: 10.1007/s13364-016-0306-9
MacSwiney, G. M. C, Vilchis, L. P, Clarke, F. M., and Racey, P. A. (2007). The importance of cenotes in conserving bat assemblages in the Yucatan, Mexico. Biol. Conserv. 136, 499–509. doi: 10.1016/j.biocon.2006.12.021
MacSwiney, G. C., Cim,é, B. B., Clarke, F. M., and Racey, P. A. (2009). Insectivorous bat activity at cenotes in the Yucatan Peninsula, Mexico. Acta Chiropterologica. 11, 139–147. doi: 10.3161/150811009X465758
Marinkelle, C. J., and Cadena, A. (1972). Notes on bats new to the fauna of colombia. Mammalia. 36, 50–58. doi: 10.1515/mamm.1972.36.1.50
Marques, J. T., Ramos Pereira, M. J., and Palmeirim, J. M. (2016). Patterns in the use of rainforest vertical space by Neotropical aerial insectivorous bats: all the action is up in the canopy. Ecography. 39, 476–486. doi: 10.1111/ecog.01453
Martínez-Ferreira, S. R., Alvarez-Añorve, M. Y., Bravo-Monzón, A. E., Montiel-González, C., Flores-Puerto, J. I., Morales-Díaz, S. P., et al. (2020). Taxonomic and functional diversity and composition of bats in a regenerating Neotropical dry forest. Diversity. 12, 332. doi: 10.3390/d12090332
McCracken, G. F., Westbrook, J. K., Brown, V. A., Eldridge, M., Federico, P., and Kunz, T. H. (2012). Bats track and exploit changes in insect pest populations. PLoS ONE. 7, e43839. doi: 10.1371/journal.pone.0043839
McKenzie, N. L., Start, A. N., and Bullen, R. D. (2002). Foraging ecology and organisation of a desert bat fauna. Aust. J. Zool. 50, 529–548. doi: 10.1071/ZO01029
Medellín, R. A., Wiederholt, R., and Lopez-Hoffman, L. (2017). Conservation relevance of bat caves for biodiversity and ecosystem services. Biol. Conserv. 211, 45–50. doi: 10.1016/j.biocon.2017.01.012
Mendoza-Sáenz, V. H., Navarrete-Gutiérrez, D. A., Jiménez-Ferrer, G., Kraker-Castañeda, C., and Saldaña-Vázquez, R. A. (2021). Abundance of the common vampire bat and feeding prevalence on cattle along a gradient of landscape disturbance in southeastern Mexico. Mammal Res. 66, 481–495. doi: 10.1007/s13364-021-00572-9
Meyer, C. F. J., Struebig, M. J., and Willig, M. R. (2016). “Responses of tropical bats to habitat fragmentation, logging and deforestation.” in Bats in the Anthropocene: Conservation of Bats in a Changing World, Eds C. Voigt, T. Kingston (Springer, Cham). doi: 10.1007/978-3-319-25220-9_4
Miles, L., Newton, A. C., DeFries, R. S., Ravilious, C., May, I., Blyth, S., et al. (2006). A global overview of the conservation status of tropical dry forests. J. Biogeogr. 33, 491–505. doi: 10.1111/j.1365-2699.2005.01424.x
Molinari, J., Nassar, J. M., Garcia-Rawlins, A., and Márquez, R. (2012). Singularidad biológica e importancia socioeconómica de los murciélagos cavernícolas de la Península de Paraguaná, Venezuela, con propuestas para su conservación. Revista de Ecología En Latino América.
Monadjem, A., Taylor, P. J., Cotterill, F. P. D., (Woody), and Schoeman, M. C. (2020). Bats of Southern and Central Africa: A Biogeographic and Taxonomic Synthesis, 2nd Edn. Wits University Press. p. 715. doi: 10.18772/22020085829
Moreira-Hernández, J. I., Calderón-Acevedo, C., and Muchhala, N. (2021). “Fur, wings, and flowers: development and progress on nectarivorous bat research in the last 50 years,” in 50 Years of Bat Research, eds B. Lim, M. B. Fenton, R. M. Brigham, Mistry, A. Kurta, Gillam, et al. (Springer). doi: 10.1007/978-3-030-54727-1_9
Naranjo, M. E., Rengifo, C., and Soriano, P. J. (2003). Effect of ingestion by bats and birds on seed germination of Stenocereus griseus and Subpilocereus repandus (Cactaceae). J. Trop. Ecol. 19, 19–25. doi: 10.1017/S0266467403003031
Nassar, J. M., Ramírez, N., and Linares, O. (1997). Comparative pollination biology of Venezuelan columnar cacti and the role of nectar-feeding bats in their sexual reproduction. Am. J. Bot. 84, 918–927. doi: 10.2307/2446282
Norberg, U. M. L., and Rayner, J. (1987). Ecological morphology and flight in bats (Mammalia; Chiroptera): wing adaptations, flight performance, foraging strategy and echolocation. Philosophical Transactions of the Royal Society of London. B, Biological Sciences. 316, 335–427. doi: 10.1098/rstb.1987.0030
Núñez, S. F., López-Baucells, A., Rocha, R., Farneda, F. Z., Bobrowiec, P. E. D., Palmeirim, J. M., et al. (2019). Echolocation and stratum preference: key trait correlates of vulnerability of insectivorous bats to tropical forest fragmentation. Front. Ecol. Evol. 7, 373. doi: 10.3389/fevo.2019.00373
Olaya-Rodrigúez, M. H., Perez-Torres, J., and Londoño-Murcia, M. c. (2019). Use of forest strata by bats according to wing morphology and habitat complexity in a fragment of tropical dry forest (Colombia). Barbastella. 12, 83–91. doi: 10.14709/BarbJ.12.1.2019.11
Otálora-Ardila, A., and López-Arévalo, H. F. (2021). Effect of the matrix-edge-forest interior gradient on the phyllostomid bats assemblage in sub-Andean forest fragments. Caldasia. 43, 274–285. doi: 10.15446/caldasia.v43n2.85071
Otálora-Ardila, A., Marinho Torres, J. M., Barbier, E., Pimentel, N. T., Barbosa Leal, E. S., and Bernard, E. (2020). Thermally-assisted monitoring of bat abundance in an exceptional cave in Brazil's Caatinga drylands. Acta Chiropterologica. 21, 411–423. doi: 10.3161/15081109ACC2019.21.2.016
Peña, C. J. M, and Reinoso, F G. (2016). Mariposas diurnas de tres fragmentos de bosque seco tropical del Alto valle del Magdalena. Tolima-Colombia. RVACCB 28, 57–66.
Petit, S. (1995). The pollinators of two species of columnar cacti on curacao, netherlands antilles. Biotropica. 27, 538. doi: 10.2307/2388971
Phelps, K., Jose, R., Labonite, M., and Kingston, T. (2016). Correlates of cave-roosting bat diversity as an effective tool to identify priority caves. Biol. Conserv. 201, 201–209. doi: 10.1016/j.biocon.2016.06.023
Pineda-Cendales, S., Hernández-Rolong, E., and Carvajal-Cogollo, J. E. (2020). Medium and Large-sized mammals in dry forests of the Colombian Caribbean. Universitas Scientiarum. 25, 435–461. doi: 10.11144/Javeriana.SC25-3.mals
Pio, D. V. V., Clarke, F. M., MacKie, I., and Racey, P. A. (2010). Echolocation calls of the bats of Trinidad, West Indies: Is guild membership reflected in echolocation signal design? Acta Chiropterologica. 12, 217–229. doi: 10.3161/150811010X504716
Pizano, C., González, -M R, López, R., Jurado, R. D., Cuadros, H., et al. (2014). El bosque seco tropical en Colombia. Instituto de Investigación de Recursos Biológicos Alexander von Humboldt (IAvH), Bogotá, DC, Colombia.
Polania Ortiz, Y. E. (2012). Movimiento de vacunos en un paisaje arbolado y su relación con el gasto de energía. [thesis]. Universidad del Tolima, Ibagé.
Posada, B. (2008). Diagnóstico de la erosión en la Zona Costera del Caribe Colombiano. INVEMAR, Serie de Publicaciones Especiales. Available online at: https://aquadocs.org/handle/1834/6682
Puig-Montserrat, X., Flaquer, C., Gómez-Aguilera, N., Burgas, A., Mas, M., Tuneu, C., et al. (2020). Bats actively prey on mosquitoes and other deleterious insects in rice paddies: potential impact on human health and agriculture. Pest Manag. Sci. 76, 3759–3769. doi: 10.1002/ps.5925
R Core Team (2021). R: A language and environment for statistical computing. R Foundation for Statistical Computing, Vienna, Austria. Available online at: https://www.R-project.org/
Ramírez-Chaves, H. E., Mejias, O., and Zambrano-Gonzalez, G. (2008). Anotaciones sobre dieta, estado reprodutivo, actividad e tamaño de colónia del murciélago mastín común (Molossus molossus: Molossidae) en la zona urbana de Popaýan, Departamento del Cauca, Colombia. Chiropt. neotrop. 14, 384–390.
Razgour, O., Korine, C., and Saltz, D. (2010). Pond characteristics as determinants of species diversity and community composition in desert bats. Anim. Conserv. 13, 505–513. doi: 10.1111/j.1469-1795.2010.00371.x
Ríos-Blanco, M. C., and Pérez-Torres, J. (2015). Dieta de las especies dominantes del ensamblaje de murciélagos frugívoros en un Bosque Seco Tropical (Colombia). Mastozoología Neotropical. 22, 103–111. Available online at: https://www.redalyc.org/articulo.oa?id=45739766011
Rodríguez-Durán, A. (2009). “Bat assemblages in the West In dies: the role of caves. Pp. 265–280,” in Island Bats: Evolution, Ecology and Conservation, Eds T. H. Fleming and P. A. Racey (University of Chicago Press, Chicago), 560.
Rojo Cruz, M. A., Zuloaga-Aguilar, S., Cuevas-Guzmán, R., MacSwiney González, M. C., and Iñiguez-Dávalos, L. I. (2019). Influence of vegetation and abiotic factors on habitat use by insectivorous bats in subtropical mountain forests. Mamm. Biol. 95, 93–101. doi: 10.1016/j.mambio.2019.03.004
Sánchez, F., Alvarez, J., Ariza, C., and Cadena, A. (2007). Bat assemblage structure in two dry forests of Colombia: composition, species richness, and relative abundance. Mamm. Biol. 72, 82–92. doi: 10.1016/j.mambio.2006.08.003
Schnitzler, H.-U., and Kalko, E. K. V. (2001). Echolocation by insect-eating bats: we define four distinct functional groups of bats and find differences in signal structure that correlate with the typical echolocation tasks faced by each group. BioScience. 51, 557–569. doi: 10.1641/0006-3568(2001)051(0557:EBIEB)2.0.CO;2
Schnitzler, H. U., Moss, C. F., and Denzinger, A. (2003). From spatial orientation to food acquisition in echolocating bats. Trends Ecol. Evol. 18, 386–394. doi: 10.1016/S0169-5347(03)00185-X
Shipley, J. R., and Twining, C. W. (2020). Seasonal dietary niche contraction in coexisting Neotropical frugivorous bats (Stenodermatinae). Biotropica. 52, 749–757. doi: 10.1111/btp.12784
Sikes, R. S., Gannon, W. L., and the Animal Care Use Committee of the American Society of Mammalogists (2011). Guidelines of the American Society of Mammalogists for the use of wild mammals in research. J. Mammal. 92, 235–253. doi: 10.1644/10-MAMM-F-355.1
Simal, F., de Lannoy, C., García-Smith, L., Doest, O., de Freitas, J. A., Franken, F., et al. (2015). Island–island and island–mainland movements of the Curaçaoan long-nosed bat, Leptonycteris curasoae. J. Mammal. 96, 579–590. doi: 10.1093/jmammal/gyv063
Simal, F., Smith, L., Doest, O., De Lannoy, C., Franken, F., Zaandam, I., et al. (2022). Bat inventories at caves and mines on the islands of aruba, bonaire and curaçao, and proposed conservation actions. Acta Chiropterologica. 23, 455–474. doi: 10.3161/15081109ACC2021.23.2.015
Solano Pita, A. M., and Pérez Hernández, L. M. (2016). Análisis Multicriterio para la Identificación de Prioridades de Conservación para el SINAP. Casos provincias Norandina y Pericaribeña definidas por Parques Nacionales Naturales (PNN). Available online at: https://repository.udistrital.edu.co/handle/11349/3798
Soriano, P. J. (2000). Functional structure of bat communities in tropical rainforests and andean cloud forests. Ecotropicos. 13, 1–20.
Stoner, K. E., and Timm, R. M. (2011). “Seasonally dry tropical forest mammals: Adaptations and seasonal patterns,” in Seasonally Dry Tropical Forests, eds. R. Dirzo, H. S. Young, H. A. Mooney, and G. Ceballos (Washington, DC: Island Press/Center for Resource Economics), 85–106. doi: 10.5822/978-1-61091-021-7_6
Straka, T. M., Lentini, P. E., Lumsden, L. F., Buchholz, S., Wintle, B. A., and van der Ree, R. (2020). Clean and green urban water bodies benefit nocturnal flying insects and their predators, insectivorous bats. Sustainability. 12, 2634. doi: 10.3390/su12072634
Torrent, L., López-Baucells, A., Rocha, R., Bobrowiec, P. E. D., and Meyer, C. F. J. (2018). The importance of lakes for bat conservation in Amazonian rainforests: an assessment using autonomous recorders. Remote Sens. Ecol. Conserv. 4, 339–351. doi: 10.1002/rse2.83
Torres-Flores, J. W., and López-Wilchis, R. (2018). Trophic niche and diet of natalus mexicanus (Chiroptera: natalidae) in a tropical dry forest of Western Mexico. Acta Chiropterologica. 20, 343–350. doi: 10.3161/15081109ACC2018.20.2.006
Tuneu-Corral, C., Puig-Montserrat, X., Flaquer, C., Mas, M., Budinski, I., and López-Baucells, A. (2020). Ecological indices in long-term acoustic bat surveys for assessing and monitoring bats' responses to climatic and land-cover changes. Ecol. Indic. 110, 105849. doi: 10.1016/j.ecolind.2019.105849
Vargas-Zapata, M. A., Gutiérrez-Moreno, L. C., Prince-Chacon, S., and Herrera-Colon, V. (2011). Riqueza y abundancia de Hesperioidea y Papilionoidea (Lepidoptera) en la Reserva Natural Las Delicias, Santa Marta, Magdalena, Colombia. Acta Biológica Colombiana 16, 43–59.
Vega-Badillo, V., Zaragoza-Caballero, S., Moreno, C., and Trejo, I. (2018). Distribución vertical de Coleoptera (Insecta) en un bosque tropical subcaducifolio en Jalisco, México. Revista Mexicana de Biodiversidad. 89, 836–846. doi: 10.22201/ib.20078706e.2018.3.2215
Villalobos-Chaves, D., and Santana, S. E. (2022). Craniodental traits predict feeding performance and dietary hardness in a community of Neotropical free-tailed bats (Chiroptera: molossidae). Funct. Ecol. 36, 1690–1699. doi: 10.1111/1365-2435.14063
Williams-Guillén, K., and Perfecto, I. (2011). Ensemble composition and activity levels of insectivorous bats in response to management intensification in coffee agroforestry systems. PLoS ONE. 6, e16502. doi: 10.1371/journal.pone.0016502
Keywords: Caribbean region, Chiroptera, dry ecosystems, trait-environment relationships, vulnerability, xerophytic areas
Citation: Díaz-B CA, Otálora-Ardila A, Valdés-Cardona MC, López-Arévalo HF and Montenegro OL (2023) Bat functional traits associated with environmental, landscape, and conservation variables in Neotropical dry forests. Front. For. Glob. Change 6:1082427. doi: 10.3389/ffgc.2023.1082427
Received: 28 October 2022; Accepted: 28 June 2023;
Published: 27 July 2023.
Edited by:
David A. Prieto-Torres, National Autonomous University of Mexico, MexicoReviewed by:
Daniel Winkler, University of West Hungary, HungaryJan Zukal, Academy of Sciences of the Czech Republic (ASCR), Czechia
Pablo Hernández Romero, National Autonomous University of Mexico, Mexico
Copyright © 2023 Díaz-B, Otálora-Ardila, Valdés-Cardona, López-Arévalo and Montenegro. This is an open-access article distributed under the terms of the Creative Commons Attribution License (CC BY). The use, distribution or reproduction in other forums is permitted, provided the original author(s) and the copyright owner(s) are credited and that the original publication in this journal is cited, in accordance with accepted academic practice. No use, distribution or reproduction is permitted which does not comply with these terms.
*Correspondence: Camila A. Díaz-B, Y2FkaWF6YmUmI3gwMDA0MDt1bmFsLmVkdS5jbw==; Aída Otálora-Ardila, YW90YWxvcmEmI3gwMDA0MDtnbWFpbC5jb20=
†These authors have contributed equally to this work