- 1College of Horticulture and Landscape Architecture, Zhongkai University of Agriculture and Engineering, Guangzhou, China
- 2Guangdong Provincial Key Laboratory of Chemical Pollution and Environmental Safety, School of Environment, South China Normal University, Guangzhou, China
- 3Institute of Biomass Engineering, South China Agricultural University, Guangzhou, China
Black carbon (BC) is a carbonaceous residue generated by the incomplete combustion of fossil fuels and biomass. It forms an important component of the mangrove carbon pool. Although coastal and marine sediments have long been recognized as important sinks for BC, there are few reports on its distribution in mangrove sediments. This study investigated BC distribution and the associated environmental implications in sediments of various size fractions. Mangrove sediments were collected from 44 sites in the Guangdong, Guangxi, Hainan, and Fujian Provinces of China. Sediments from the Hainan Province were found to have much higher organic carbon (OC) and BC content than those from other provinces. Sediment OC and the BC content showed a significant positive relationship. Sediments from the Guangdong, Guangxi, and Hainan provinces showed significant BC accumulation with an enrichment factor > 1, especially in the size fractions 0.053–0.25 mm and 0.25–2 mm. Sediments from different sampling sites did not show significantly different BC distribution between the various size fractions. A higher mass loading of BC was observed among fine particles in samples from the Fujian Province, and among the larger particles in samples from the Guangdong, Guangxi, and Hainan provinces. Among all the samples, the BC/OC ratio ranged from 0.21 to 0.29, indicating that BC originates from a combination of biomass and mineral combustion. The average δ13C values in the sediments varied between the sampling plots. The highest δ13C values were recorded in the Fujian province, which indicates the proportion of BC from biomass combustion in mangroves in Fujian Province is higher than that in other Provinces.
Introduction
Mangrove wetlands are distributed in the tropical and subtropical intertidal coastal zones. They have unique morphological and structural characteristics, physiological and ecological processes, and strong adaptability to intertidal tidal flat environments, and accounting for 30–40% of tropical forest wetlands worldwide (Perez et al., 2018; Hu et al., 2019). Mangroves have many functions, including attenuating the effects of wind and waves, embankment protection, silt promotion, and water purification. They also serve as important habitats for many sea birds, fish, shrimp, and shellfish (Bouillon et al., 2008; Duarte et al., 2013; Taillardat et al., 2018).
Mangroves, saltmarshes, and seagrasses are recognized as blue carbon ecosystems for their disproportionately large organic carbon (OC) storage. When they are mature, vegetal biomass is deposited and conserved within sediments over millennia (Perez et al., 2018; Taillardat et al., 2018). Mangroves have among the highest carbon sequestration capacity of any ecosystem worldwide. The ecosystem is in a long-term flooded state, with a slow rate of OC decomposition and a long carbon cycle, making it one of the most important coastal zone carbon sinks (Alongi, 2014; Sanders et al., 2014). Mangroves play a vital role in terrestrial and oceanic carbon cycling (Alongi, 2012; Donato et al., 2012; Liu et al., 2014). Although they make up 0.7% of global coastal zones, they contribute 10% of the total net primary production and 25% of the carbon burial in the coastal zone worldwide (Kathiresan and Bingham, 2001; Kathiresan et al., 2013). As a coastal “blue carbon” wetland, mangroves play a vital role in mitigating global climate change and reducing greenhouse gas emissions.
In addition to organic carbon, the black carbon (BC) in the mangrove carbon pool has attracted considerable attention. BC is a carbonaceous residue generated from the incomplete combustion of fossil fuels and biomass (Kuhlbusch, 1998; Masiello and Druffel, 1998; Wang et al., 2022). It predominantly comprises highly polymerized polycyclic aromatic hydrocarbons that are extremely difficult to degrade at ambient temperatures. Owing to this unique property, BC has a considerable influence on the carbon budget and the refractory carbon pool worldwide. It is considered to be an inert carbon pool and an important component of the global carbon sink (Guo et al., 2018). BC is mainly produced on land through natural and anthropogenic activities and is transported to the sea in the form of aerosols and/or through soil erosion and river discharge. It may also accumulate in coastal and marine sediments (Luz et al., 2010). Above formation and storage mechanisms may account for a substantial proportion of the total marine sedimentary organic carbon (Zhang et al., 2022). Mangrove is one of the most carbon-rich coastal wetlands that sequester and store carbon to help mitigate global climate change. BC is very likely to constitute an important component of coastal carbon pool owing to its allochthonous and refractory, and it might have a significant implication on the carbon sequestration in mangrove sediments and then affect the distribution of mangrove carbon stocks and the global blue carbon budget (Perez et al., 2018; Taillardat et al., 2018; Zhang et al., 2022). Therefore, it is necessary to clarify the content and characteristics of BC in mangrove forests. This will also important for us to assess and predict accurately the role of mangroves in mitigating climate change (Chew and Gallagher, 2018). However, relatively few studies have been undertaken on BC in mangrove sediments. Therefore, it is necessary to explore the content and distribution of BC in mangrove sediment. The specific aims of this study were to: (1) determine the BC content of sediments from mangrove wetlands in China, (2) determine the distribution characteristics of BC in sediments of different particle sizes, and (3) identify BC sources in sediments and the associated environmental implications.
Materials and methods
Sediment sampling
Surface sediments were collected in 2018 and 2019 from mangrove wetlands in Shenzhen, Guangzhou, and Zhuhai (Guangdong Province, n = 13); Fangchenggang, Shankou, Qinzhou, and Beicanghe (Guangxi Province, n = 16); Danchang and Huachang (Hainan Province, n = 5); and Quanzhou, Fuding, and Longhai (Fujian Province, n = 10) (Figure 1), In these wetland areas, Sonneratia apetala, Aegiceras corniculatum, Rhizophora stylosa, and Kandelia candel are the dominant mangrove species, respectively. Sediment samples (0–5 cm depth) were collected with a precleaned stainless steel scoop, transported to the laboratory, air-dried, and passed through a 2 mm sieve within 1 week. Roots, rocks, and visible residues were manually removed. If we need to accurately calculate the carbon pool content of sediments in mangrove, the sampling depth should be at least 50 cm, or even 100 cm. However, there is no common protocol for the sampling depth to study the distribution characteristics of BC (Eid and Shaltout, 2016; Chew and Gallagher, 2018; Guo et al., 2018). Our study mainly focuses on the distribution characteristics of BC in mangrove surface sediments in China, therefore, the sampling depth of sediments are at depth of 0–5 cm.
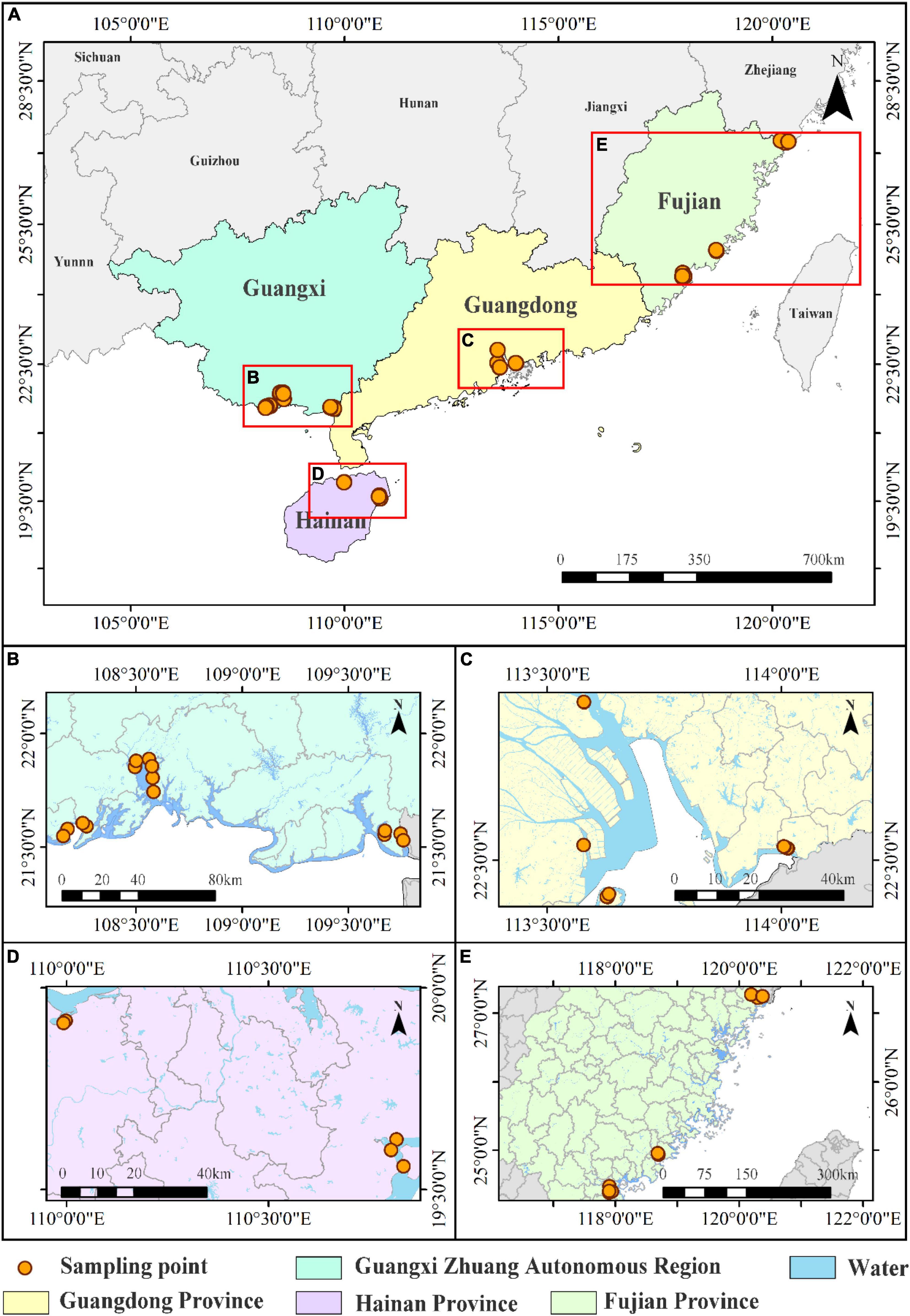
Figure 1. Map of mangrove sampling locations in China used for the study. (A) All sampling locations, (B) sampling locations in Guangxi Province, (C) sampling locations in Guangdong Province, (D) sampling locations in Hainan Province, and (E) sampling locations in Fujian Province.
Laboratory analysis
The OC content of the sediments was measured using the H2SO4-K2Cr2O7 oxidation method (Yeomans and Bremner, 1988). A total of 0.5 g sediment that had been air dried through a 0.149 mm sieve was acidified using a dichromate (H2SO4-K2Cr2O7) solution for 30 min in a Pyrex digestion tube placed within a 40-tube block digester that had been preheated to 170°C. The unreacted dichromate was then estimated using titration of the cooled digest with an acidified solution of ferrous ammonium sulfate using N-phenylanthranilic acid as an indicator. The BC content of the sediments was determined using the wet chemical oxidation method proposed by Lim and Cachier (1996). Briefly, 3 g of air-dried sediment (through a 0.149 mm sieve) was added to a centrifugal tube and then treated with 15 mL of 3 mol L–1 HCl for 24 h to remove the inorganic carbon. After centrifugation, the supernatant was decanted and 15 mL of 10 mol L–1 HCl was added for 24 h to remove the CaF2. The residue was then oxidized with 15 mL of an acid potassium dichromate solution (0.1 mol L–1 K2Cr2O7 and 2 mol L–1 H2SO4, 1:1, v:v) at 55 ± 1°C for 60 h. During the reaction, K2Cr2O7 was added until the color changed to maintain the oxidant in excess, and deionized water was added several times to maintain the solution volume. The carbon residue obtained after this treatment was regarded as BC. The BC content and δ13C values of the residues were determined using an elemental analyzer (FLASH EA-DELTA V; Thermo Fisher Scientific, Waltham, MA, United States).
The sediments were fractionated using modified wet sieving (Smith et al., 2014; Márquez et al., 2019); 100 g of sediment that had been air dried through a 2 mm sieve was placed in a 0.25 mm sieve and then immersed in deionized water for 5 min. It was then agitated vertically at 3 cm in amplitude 50 times within 2 min. The sediments in the 0.25 mm sieve were transferred into aluminum cans and then oven-dried at 60°C to constant weight. Using the same procedure, the remainder of the sediment and the water mixture was passed through a 0.053 mm sieve to remove aggregates of 0.053–0.25 mm. The remaining sediment and water mixture that had previously been passed through the 0.053 mm sieve was then centrifuged and dried to obtain aggregates <0.053 mm. The dried aggregates with differing particle size fractions were used to measure the BC content using this method.
Calculations and statistical analysis
The ratio of BC to OC reflects the sources of BC, calculated as:
The enrichment factor (EF) reflects the addition of BC to the sediments as derived from anthropogenic activity and is calculated as:
where Csample and Cref are the BC content in the sediment sample and the background value for BC, respectively. In the present study, the average BC concentration for undisturbed samples from the bottom of the soil profiles was ascertained, to represent the background value for soil BC, which was calculated as 0.47 g kg–1.
The distribution factor (DF), defined as the ratio of BC concentration between the individual size fraction and bulk sediment sample, is used to estimate the size fraction with which the BC is preferentially enriched, which is calculated as follows (Zong et al., 2016):
where Cfraction and Cbulk represent the BC concentration in a given size fraction and the bulk sediment samples, respectively. A DF value of > 1 indicates that BC has preferentially accumulated in this particular sediment size fraction.
The mass loading of BC is an important index for assessing BC accumulation in grain-size fractions. The mass loading for the grain size fraction (GSFloading) was calculated by combining the BC concentration and the mass percentage in a given size fraction, as follows:
where CMi and GSi are the BC concentration in the individual size fraction (i) and the mass percentage of the individual fraction, respectively. The summation of GSFloading values for the size fractions of an individual sample equaled 100%.
The statistical analyses were performed using SPSS Statistics v.21 (IBM, Armonk, NY, United States). One-way ANOVA was used to explore the differences of BC among different sampling sites. Tukey’s multiple-range test was performed to determine whether significant differences occurred among different sampling sites based on the means at a significance level of 0.05. The figures were plotted using OriginPro 2016 (OriginLab Corporation, Northampton, MA, United States).
Results
Contents and relationship of organic carbon and black carbon in mangrove sediments
The OC content for different mangrove sediments ranged from 1.32 to 5.31 g kg–1. The amount of OC in the sediments varied according to the sampling location. Sediments from the Hainan Province contained considerably more OC than those from the other provinces (F = 3.214, P = 0.033, Figure 2A). The BC content for sediments in our study area ranged from 0.30 to 1.83 g kg–1, and varied according to the sampling province. Sediments from the Hainan Province had substantially higher BC than those from the other provinces (F = 3.239, P = 0.033, Figure 2B). The OC and BC content of our sediments were positively correlated (R2 = 0.951, P < 0.05, Figure 3), indicating that the distribution characteristics of the BC content depend on those of the OC.
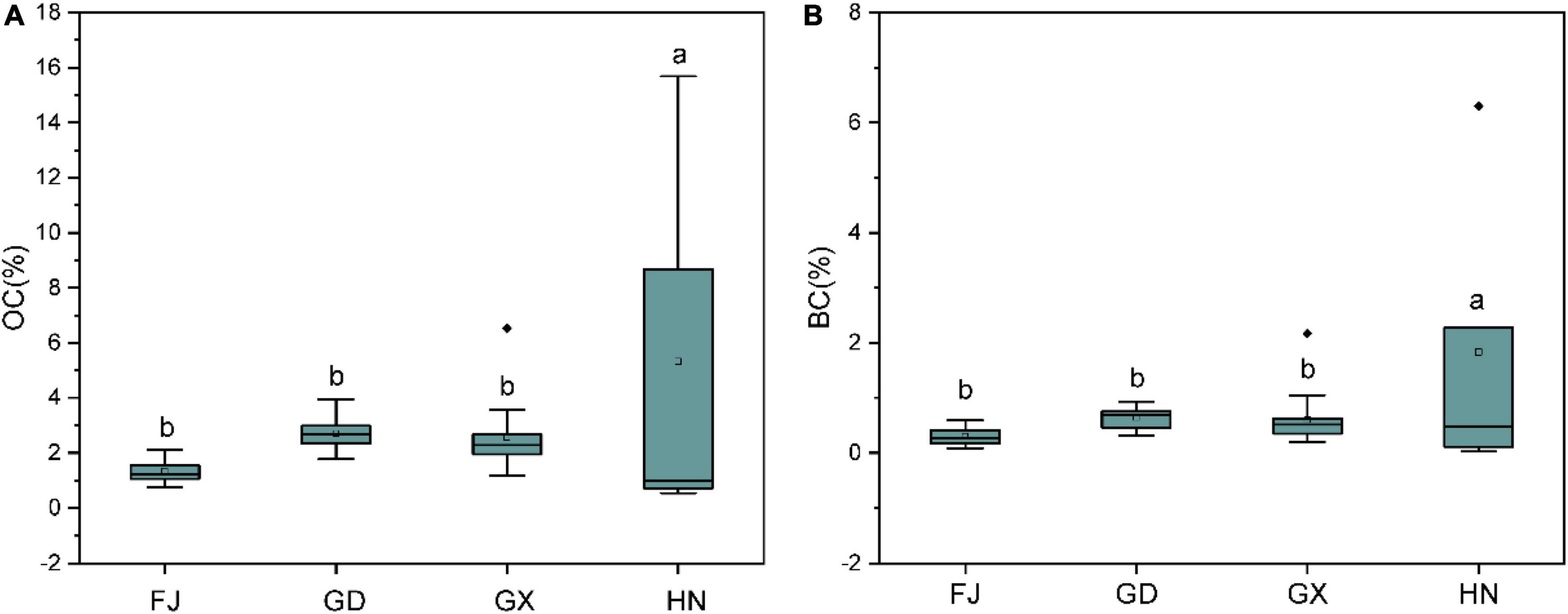
Figure 2. Boxplots showing the organic carbon (OC; A) and black carbon (BC; B) content of sediments from different mangrove wetlands in China. FJ, Fujian; GD, Guangdong; GX, Guangxi; HN, Hainan. Different lowercase letters indicate significant differences (α = 0.05).
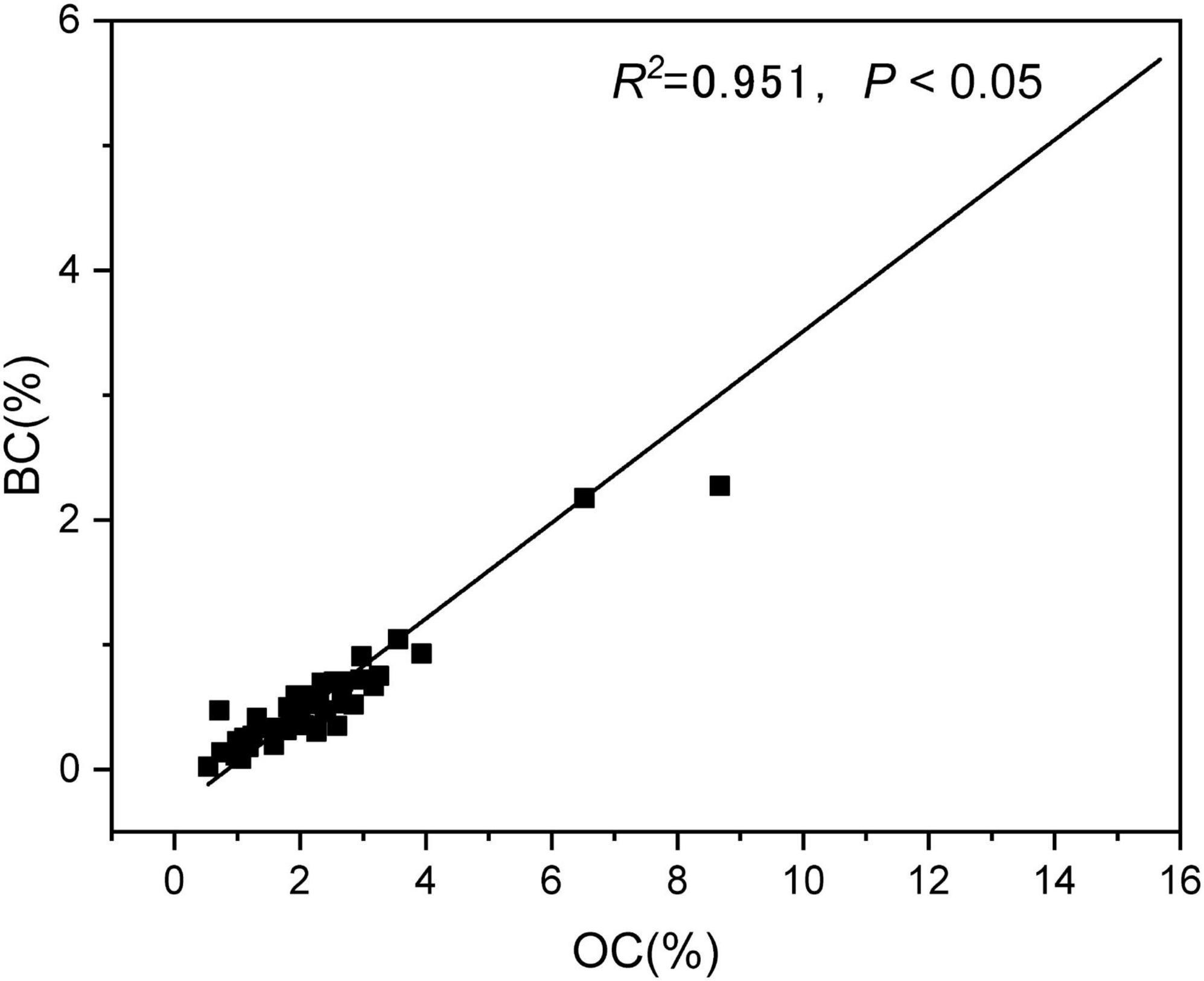
Figure 3. Correlation between organic carbon (OC) and black carbon (BC) content of sediments from different mangrove wetlands in China. R2 and P indicate the fitness and significance of the linear regression models, respectively.
Black carbon enrichment factors in different sediment size fractions
Sediment fractions from the Guangdong, Guangxi, and Hainan provinces showed significant accumulation of BC (EF > 1), especially in the 0.25–2 mm and 0.053–0.25 mm size fractions. The highest concentration was found in the 0.25–2 mm fraction, while the lowest was in the <0.053 mm fraction. Sediments from Fujian (F = 3.338, P = 0.048) and Guangdong (F = 3.530, P = 0.039) showed significantly different BC enrichment factors according to the particle size fraction. The BC concentrations were the highest in the 0.053–0.25 mm fraction from the Fujian Province, and in the 0.25–2 mm fraction from the Guangdong Province. In contrast, the sediments from Guangxi (F = 1.515, P = 0.236) and Hainan (F = 0.406, P = 0.752) showed no significant differences in BC EF among the different sediment size fractions (Figure 4).
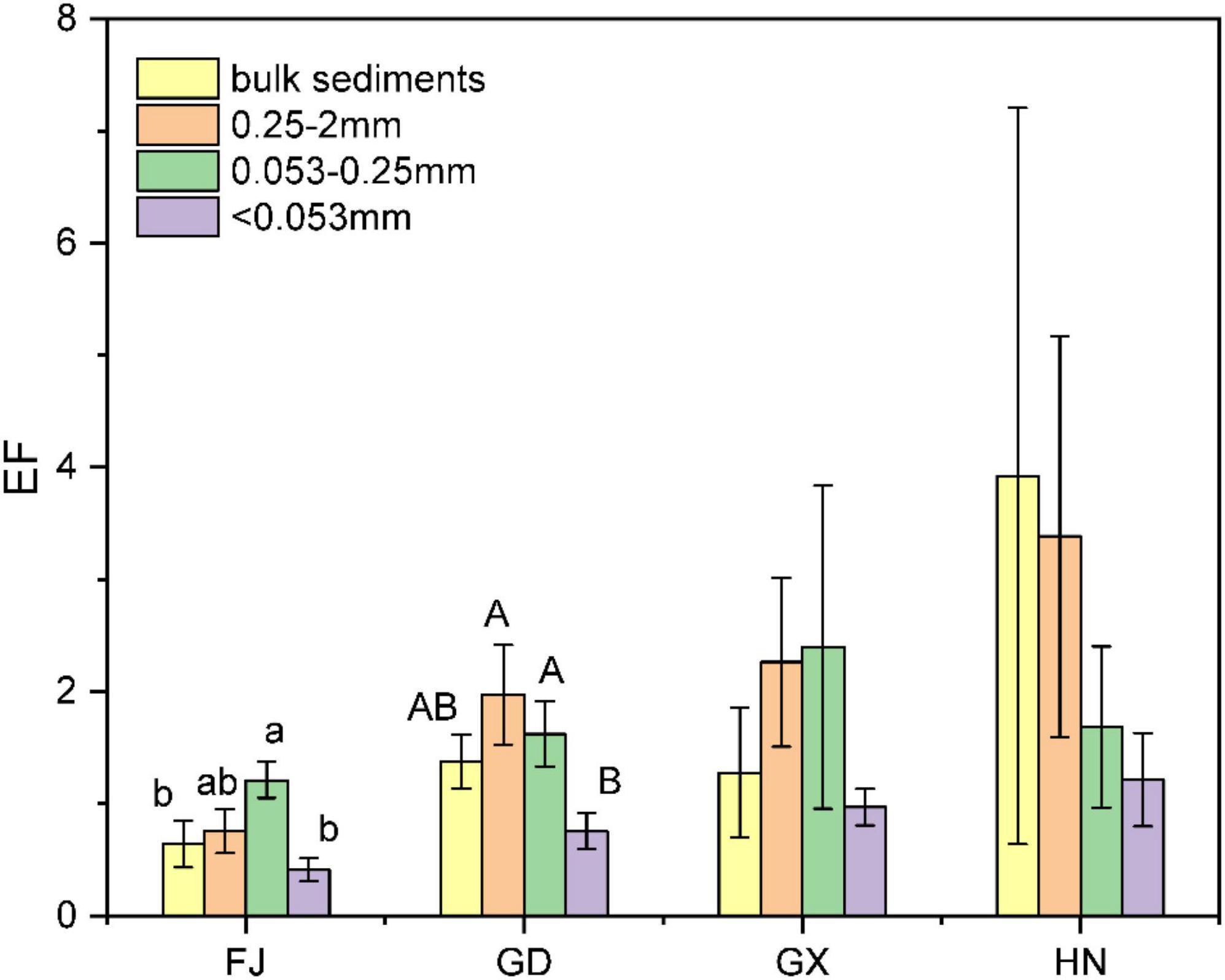
Figure 4. Enrichment factors (EF) for black carbon in sediment fractions from different mangrove wetlands in China. Different lowercase and uppercase letters indicate significant differences (α = 0.05) in the samples from Fujian (FJ) and Guangdong (GD), respectively. GX, Guangxi; HN, Hainan.
Distribution characteristics of black carbon in sediments with different particle sizes
The DF of BC was calculated to estimate the size fractions of BC that were preferentially enriched (see Figure 5A). The DFs of BC in sediments from different sampling sites did not significantly differ among the different size fractions. The DFs for BC were within the ranges of 1.38–2.02 for the 0.25–2 mm fraction, 1.18–2.22 for the 0.053–0.25 mm fraction, and 0.54–1.95 for the <0.053 mm fraction. With the exception of the <0.053 mm fraction from the Hainan Province, the larger sediment fractions (0.053–0.25 mm and 0.25–2 mm) had a higher DF than those with small fractions (<0.053 mm).
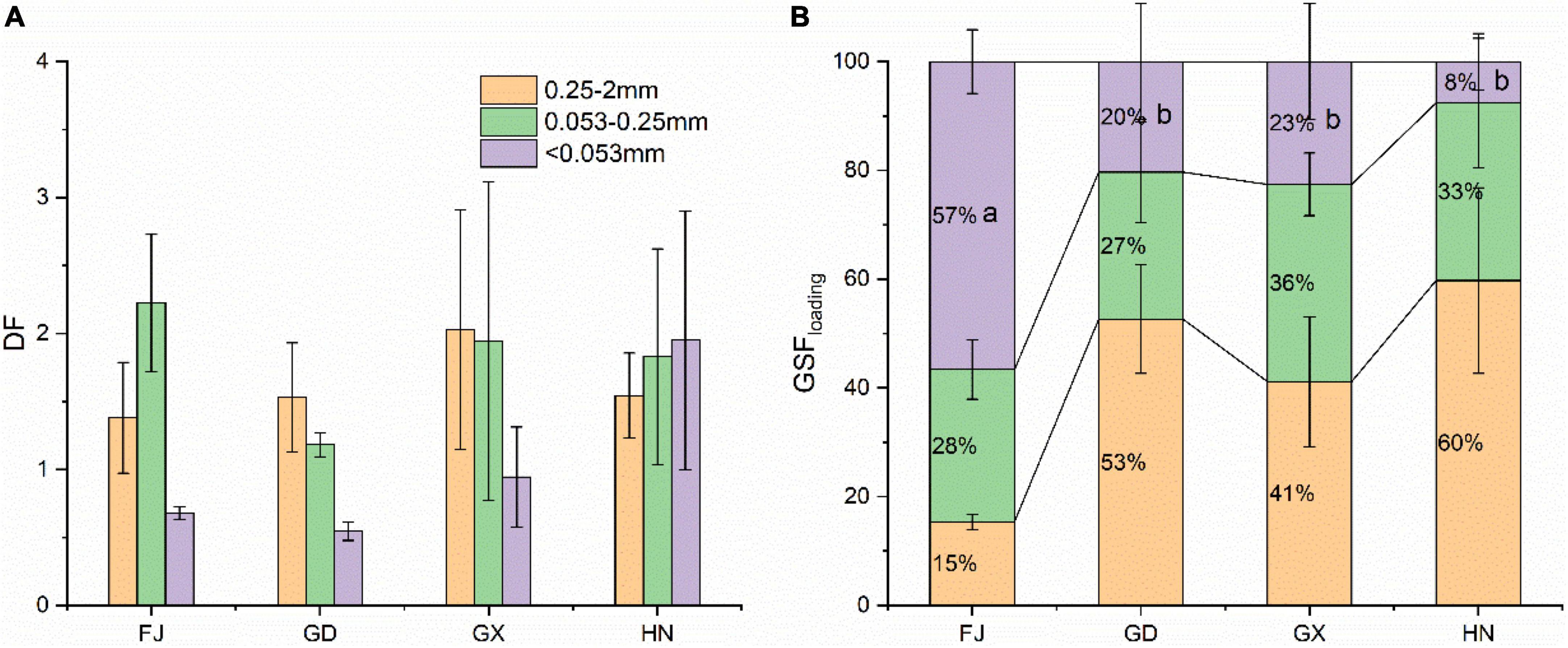
Figure 5. Distribution factors (DF, A) and mass loading (GSFloading, B) for different size sediment fractions from mangrove wetlands in China. Lowercase letters indicate significant differences (α = 0.05). FJ, Fujian; GD, Guangdong; GX, Guangxi; HN, Hainan.
To estimate the contribution from each size fraction to the total BC content of the bulk sediments, mass loading was calculated as shown in Figure 5B. In the sediment from the Fujian Province, the largest BC mass loading with an average of 57% was found in the <0.053 mm fraction, followed by the 0.053–0.25 mm fraction (28%). The three size fractions between 0.25 and 2 mm accounted for 15% of the BC storage in the sediments. In contrast, among the sediments from the Guangdong, Guangxi, and Hainan provinces, the BC mass loading was higher among the larger particles and the lowest among the fine particles.
Correlation between different black carbon indexes and its sources
The BC/OC ratio in our mangrove sediments ranged from 0.21 to 0.29, and there was no difference among the sampling plots (Figure 6A). The BC/OC ratio is linked with the sources of BC accumulation (Wang et al., 2022). A BC/OC value of approximately 0.1 indicates that the BC is mainly derived from biomass combustion, whereas a value of approximately 0.5 indicates that the BC is mainly derived from mineral combustion (Ruellan and Cachier, 2001; Bucheli et al., 2004). The BC/OC ratio in our is higher than 0.1, but lower than 0.5, which indicated that the BC originated from a mixture of biomass and mineral combustion. The average δ13C value across all the sampled sites ranged from –28.07 to –26.26‰. The average value of δ13C in the sediments varied according to the sampling plot, and the sediments from the Fujian Province had considerably higher δ13C values than those from the other provinces (F = 7.36, P = 0.031, Figure 6B), which indicated the proportion of BC from biomass combustion in mangroves in Fujian Province is higher than that in other Provinces.
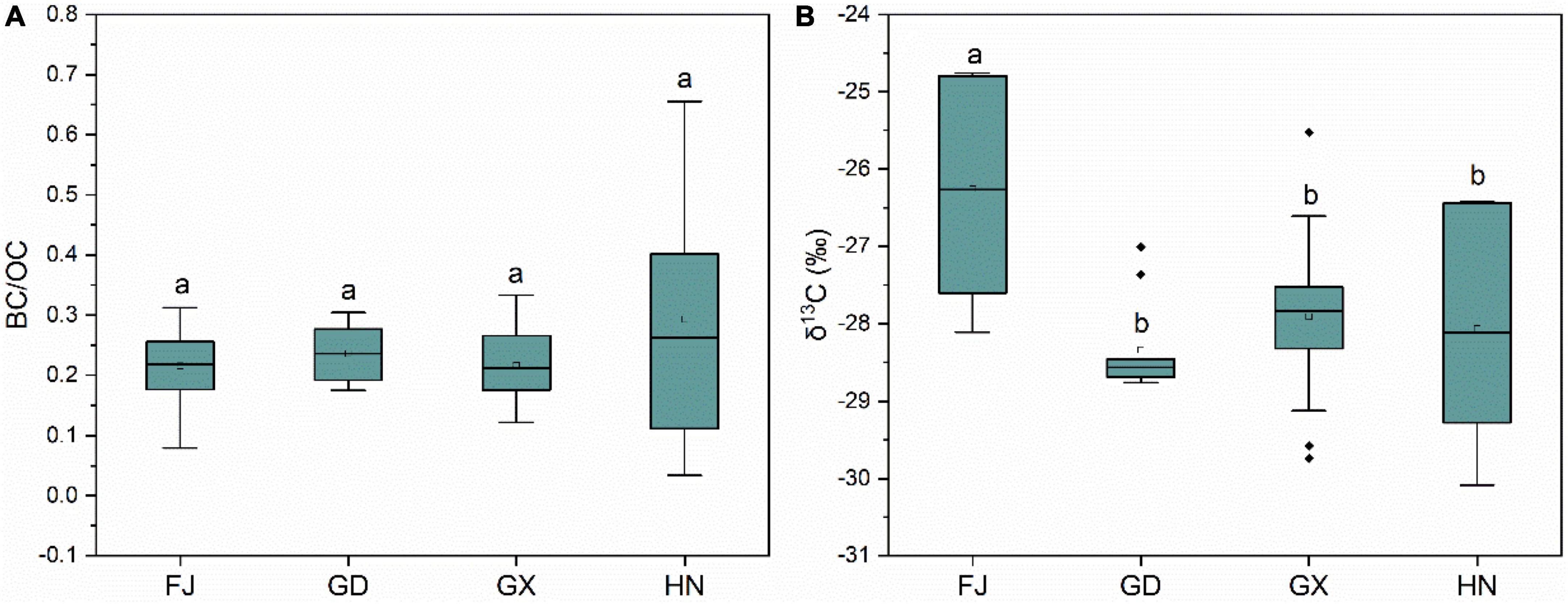
Figure 6. Boxplots showing the ratio of black carbon (BC) to the organic carbon (OC) (A) and δ13C content (B) in the sediments from different mangrove wetlands in China. Different lowercase letters indicate significant differences (α = 0.05). FJ, Fujian; GD, Guangdong; GX, Guangxi; HN, Hainan.
Discussion
Compared with the other regions, the OC content in our study area was lower than those reported for mangrove forests in Australia (Alongi et al., 2000, 2003), Thailand (Alongi et al., 2001, 2002), Brazil (Sanders et al., 2010), and Micronesia (Kauffman et al., 2011). However, they were similar to those reported for Japan (Khan et al., 2007), India (Ray et al., 2011), and Vietnam (Tue et al., 2012). This indicated that there are significant differences in OC concentration among different regions. Previous studies showed that OC content is predominantly influenced by tidal gradient, mangrove forest age, biomass and productivity, species composition, and sedimentation of suspended matter (Gleason and Ewel, 2002; Khan et al., 2007; Cerón-Bretón et al., 2011). In our results, OC and BC contents in Hainan Province where Rhizophora stylosa is a dominant species was significantly higher than other Provinces. Since BC content is affected by mangrove tree species (Guo et al., 2018), this might be the driving factor of a higher accumulation of BC in Hainan province. In addition, mangrove cover, climate factors and anthropogenic disturbances also had impact on BC content in sediments (Charles et al., 2020; Meng et al., 2022). Therefore, these factors mentioned above should be considered in the future researches.
In addition, BC accumulates in all sampling sites because of its high resistance to oxidation (Luz et al., 2010; Guo et al., 2018). Significantly high BC content recorded from the larger size fractions indicates that pronounced accumulation of BC occurred in these fractions. Larger size fractions could enrich more BC than the fine fractions due to their larger pores and easier adsorption and BC wrapping (Zong et al., 2016). The contribution from large particles to the BC content was generally greater than that of the small particles. However, fine particles are easily suspended and can be a source of atmospheric aerosols in the environment, and then might have impact on the air quality of mangrove ecosystems. Therefore, the tendency for BC to be adsorbed into finer fractions should be taken into consideration during environmental risk assessments and atmospheric pollution control (Liu et al., 2019). If we could immobilize airborne BC in mangrove sediments, it would mitigate the effects of BC on climate and human health. However, a higher mass loading of BC was observed among fine particles in samples from the Fujian Province, and among the larger particles in samples from the Guangdong, Guangxi, and Hainan provinces. Previous studies showed that BC produced by biomass would form a well-developed pore structure and specific surface area, while BC produced by fossil fuel would inhibit the formation of its pore structure (King et al., 2008; Edmondson et al., 2015; Gao et al., 2016; Zong et al., 2016). BC with well-developed pore structure and specific surface area is easier to get into fine particles. Our results indicated that the proportion of BC from biomass in mangroves in Fujian Province is higher than that in other Provinces, this might be the reason of a higher accumulation of BC in fine particles Fujian province.
At present, many methods have been applied to identify the source of BC: 14C analysis, 13C analysis, BC/OC ratio, polycyclic aromatic hydrocarbons isomer ratio, particle size and morphological characteristics (Wang et al., 2012). Among them, the BC/OC ratio is often used to distinguish the source of BC due to its high reliability and low cost. However, we cannot determine the main source of BC when the source of BC is a combination of biomass and mineral combustion. Therefore, 13C analysis is often combined with the BC/OC ratio to identify the sources of BC (Wang et al., 2013). Generally, the δ13C value generated by fossil fuel combustion is much lower than that of plant carbon isotopes. For example, the average δ13C value of petroleum is about –28‰, the average δ13C value of coal is about –24.5‰, and the δ13C value of BC particles in motor vehicle exhaust is between –25.9 and –27.6‰ (Guo et al., 2016). Therefore, if a large amount of BC produced by fossil fuel enters the atmosphere or soil, the δ13C value of BC in atmospheric or soil will decrease. The δ13C value of BC in Guangdong, Guangxi and Hainan was lower than that in Fujian, which indicated that the proportion of BC originating from fossil fuels in these three provinces is higher than that in Fujian Province. In addition, the contribution of fossil carbon to the BC pool was spatially heterogeneous, which could be related to differences in the distance to landmass, land cover and socioeconomic development. In conclusion, BC has accumulation in some degree in mangrove area in China. Although BC accumulation is beneficial to mangrove carbon storage, BC will enter the atmosphere firstly, which may aggravate the greenhouse effect and endanger human health. Therefore, we should pay more attention to the BC content in the atmosphere in this area, try to reduce the BC content in the atmosphere, and increase the BC content in the soil and sediments. In addition, BC content generally increases with depth (Guo et al., 2018). However, our sampling depth was only 5 cm, therefore, our results only showed the distribution characteristics of the surface sediments, this is also a limitation of this study.
Conclusion
In this study, the content and distribution of BC was analyzed in mangrove sediments from China. Sediments from the Hainan Province had much higher OC and BC content than those from the other provinces. The sediment OC content showed a significant positive relationship with the BC content. The BC showed a significant accumulation in sediments of differing particle sizes, especially in the size fractions of 0.25–2 mm and 0.053–0.25 mm. The significantly high BC content found in the larger size fractions indicates that pronounced accumulation of BC occurred in these fractions. The contribution of large particles to the BC content was generally greater than that of the small particles. The BC/OC ratio in sediments from different mangroves ranged from 0.21 to 0.29, which indicates that BC originated from a mixture of biomass and mineral combustion. Average δ13C values in the sediments varied between the sampling plots, and sediments from the Fujian Province showed considerably higher δ13C values than those from the other provinces. This indicates the proportion of BC from biomass combustion in mangroves in Fujian Province is higher than other Provinces. Our findings indicate that BC content and its δ13C values in sediments vary between provinces, which provides theoretical support and guidance for future carbon storage estimation and carbon sink increases in mangrove forests. However, we did not estimate the contribution of mangrove BC storage to the sediment carbon pool, which should form the focus of further study.
Data availability statement
The original contributions presented in this study are included in the article/supplementary material, further inquiries can be directed to the corresponding author/s.
Author contributions
MW: conceptualization, methodology, software, and writing-original draft preparation. YS: data curation. HZ and WW: visualization and investigation. LD: software and validation. PT: supervision, writing-reviewing and editing. All authors contributed to the article and approved the submitted version.
Funding
This work was supported by the Meizhou Science and Technology Project (grant no. 2021A0304001) and Guangzhou Science and Technology Project (grant no. 202201011081).
Conflict of interest
The authors declare that the research was conducted in the absence of any commercial or financial relationships that could be construed as a potential conflict of interest.
Publisher’s note
All claims expressed in this article are solely those of the authors and do not necessarily represent those of their affiliated organizations, or those of the publisher, the editors and the reviewers. Any product that may be evaluated in this article, or claim that may be made by its manufacturer, is not guaranteed or endorsed by the publisher.
References
Alongi, D. M. (2012). Carbon sequestration in mangrove forests. Carbon Manag. 3, 313–322. doi: 10.4155/cmt.12.20
Alongi, D. M. (2014). Carbon cycling and storage in mangrove forests. Ann. Rev. Mar. Sci. 6, 195–219. doi: 10.1146/annurev-marine-010213-135020
Alongi, D. M., Clough, B. F., Dixon, P., and Tirendi, F. (2003). Nutrient partitioning and storage in arid-zone forests of the mangroves Rhizophora stylosa and Avicennia marina. Trees 17, 51–60. doi: 10.1007/s00468-002-0206-2
Alongi, D. M., Trott, L., Wattayakorn, G., and Clough, B. F. (2002). Below-ground nitrogen cycling in relation to net canopy production in mangrove forests of southern Thailand. Mar. Biol. 140, 855–864. doi: 10.1007/s00227-001-0757-6
Alongi, D., Tirendi, F., and Clough, B. (2000). Below-ground decomposition of organic matter in forests of the mangroves Rhizophora stylosa and Avicennia marina along the arid coast of Western Australia. Aquat. Bot. 68, 97–122. doi: 10.1016/S0304-3770(00)00110-8
Alongi, D., Wattayakorn, G., Pfitzner, J., Tirendi, F., Zagorskis, I., Brunskill, G., et al. (2001). Organic carbon accumulation and metabolic pathways in sediments of mangrove forests in southern Thailand. Mar. Geol. 179, 85–103. doi: 10.1016/S0025-3227(01)00195-5
Bouillon, S., Borges, A. V., Castañeda-Moya, E., Diele, K., Dittmar, T., Duke, N. C., et al. (2008). Mangrove production and carbon sinks: a revision of global budget estimates. Glob. Biogeochem. cycles 22. doi: 10.1029/2007GB003052
Bucheli, T. D., Blum, F., Desaules, A., and Gustafsson, O. (2004). Polycyclic aromatic hydrocarbons, black carbon, and molecular markers in soils of Switzerland. Chemosphere 56, 1061–1076. doi: 10.1016/j.chemosphere.2004.06.002
Cerón-Bretón, J., Cerón-Bretón, R., Rangel-Marrón, M., Murielgarcia, M., Cordova-Quiroz, A., and Estrella-Cahuich, A. (2011). Determination of carbon sequestration rate in soil of a mangrove forest in Campeche, Mexico. WSEAS Trans. Environ. Dev. 7, 55–64.
Charles, S. P., Kominoski, J. S., Armitage, A. R., Guo, H., Weaver, C. A., and Pennings, S. C. (2020). Quantifying how changing mangrove cover affects ecosystem carbon storage in coastal wetlands. Ecology 101:e02916. doi: 10.1002/ecy.2916
Chew, S. T., and Gallagher, J. B. (2018). Accounting for black carbon lowers estimates of blue carbon storage services. Sci. Rep. 8:2553. doi: 10.1038/s41598-018-20644-2
Donato, D. C., Kauffman, J. B., Mackenzie, R. A., Ainsworth, A., and Pfleeger, A. Z. (2012). Whole-island carbon stocks in the tropical Pacific: implications for mangrove conservation and upland restoration. J. Environ. Manage. 97, 89–96. doi: 10.1016/j.jenvman.2011.12.004
Duarte, C. M., Losada, I. J., Hendriks, I. E., Mazarrasa, I., and Marbà, N. (2013). The role of coastal plant communities for climate change mitigation and adaptation. Nat. Clim. Change 3, 961–968. doi: 10.1038/nclimate1970
Edmondson, J. L., Stott, I., Potter, J., Lopez-Capel, E., Manning, D. A., Gaston, K. J., et al. (2015). Black carbon contribution to organic carbon stocks in urban soil. Environ. Sci. Technol. 49, 8339–8346. doi: 10.1021/acs.est.5b00313
Eid, E. M., and Shaltout, K. H. (2016). Distribution of soil organic carbon in the mangrove Avicennia marina (Forssk.) Vierh. along the Egyptian Red Sea Coast. Reg. Stud. Mar. Sci. 3, 76–82. doi: 10.1016/j.rsma.2015.05.006
Gao, C., Knorr, K.-H., Yu, Z., He, J., Zhang, S., Lu, X., et al. (2016). Black carbon deposition and storage in peat soils of the Changbai Mountain. China. Geoderma. 273, 98–105. doi: 10.1016/j.geoderma.2016.03.021
Gleason, S. M., and Ewel, K. C. (2002). Organic matter dynamics on the forest floor of a micronesian mangrove forest: An investigation of species composition Shifts1. Biotropica 34, 190–198. doi: 10.1111/j.1744-7429.2002.tb00530.x
Guo, P., Sun, Y., Su, H., Wang, M., and Zhang, Y. (2018). Spatial and temporal trends in total organic carbon (TOC), black carbon (BC), and total nitrogen (TN) and their relationships under different planting patterns in a restored coastal mangrove wetland: case study in Fujian, China. Chem. Speciat. Bioavailab. 30, 47–56. doi: 10.1080/09542299.2018.1484673
Guo, Z., Jiang, W., Chen, S., Sun, D., Shi, L., Zeng, G., et al. (2016). Stable isotopic compositions of elemental carbon in PM1. 1 in north suburb of Nanjing Region. China. Atmospheric. Res. 168, 105–111. doi: 10.1016/j.atmosres.2015.09.006
Hu, Y., Pei, N., Sun, Y., Xu, X., Zhang, Z., Li, H., et al. (2019). Halogenated flame retardants in mangrove sediments from the Pearl River Estuary, South China: comparison with historical data and correlation with microbial community. Chemosphere 227, 315–322. doi: 10.1016/j.chemosphere.2019.04.075
Kathiresan, K., Anburaj, R., Gomathi, V., and Saravanakumar, K. (2013). Carbon sequestration potential of Rhizophora mucronata and Avicennia marina as influenced by age, season, growth and sediment characteristics in southeast coast of India. J. Coast. Conserv. 17, 397–408. doi: 10.1007/s11852-013-0236-5
Kathiresan, K., and Bingham, B. L. (2001). Biology of mangroves and mangrove ecosystems. Adv. Mar. Biol. 40, 81–251. doi: 10.1016/S0065-2881(01)40003-4
Kauffman, J. B., Heider, C., Cole, T. G., Dwire, K. A., and Donato, D. C. (2011). Ecosystem carbon stocks of Micronesian mangrove forests. Wetlands 31, 343–352. doi: 10.1007/s13157-011-0148-9
Khan, M., Islam, N., Suwa, R., and Hagihara, A. (2007). Carbon and nitrogen pools in a mangrove stand of Kandelia obovata (S., L.) Yong: vertical distribution in the soil–vegetation system. Wetlands Ecol. Manag. 15, 141–153. doi: 10.1007/s11273-006-9020-8
King, P., Rakesh, N., Lahari, S. B., Kumar, Y. P., and Prasad, V. (2008). Biosorption of zinc onto Syzygium cumini L.: Equilibrium and kinetic studies. Chem. Eng. J. 144, 181–187. doi: 10.1016/j.cej.2008.01.019
Kuhlbusch, T. A. J. (1998). Black carbon and the carbon cycle. Science 280, 1903–1904. doi: 10.1126/science.280.5371.1903
Lim, B., and Cachier, H. (1996). Determination of black carbon by chemical oxidation and thermal treatment in recent marine and lake sediments and Cretaceous-Tertiary clays. Chemical Geology 131, 143–154. doi: 10.1016/0009-2541(96)00031-9
Liu, D., Joshi, R., Wang, J., Yu, C., Allan, J. D., Coe, H., et al. (2019). Contrasting physical properties of black carbon in urban Beijing between winter and summer. Atmos. Chem. Phys. 19, 6749–6769. doi: 10.5194/acp-19-6749-2019
Liu, H., Ren, H., Hui, D., Wang, W., Liao, B., and Cao, Q. (2014). Carbon stocks and potential carbon storage in the mangrove forests of China. J. Environ. Manage. 133, 86–93. doi: 10.1016/j.jenvman.2013.11.037
Luz, L. G., Carreira, R. S., Farias, C. O., Scofield, A. D. L., Nudi, A. H., and Wagener, A. D. L. R. (2010). Trends in PAH and black carbon source and abundance in a tropical mangrove system and possible association with bioavailability. Org. Geochem. 41, 1146–1155. doi: 10.1016/j.orggeochem.2010.06.003
Márquez, C. O., García, V. J., Schultz, R. C., and Isenhart, T. M. (2019). A conceptual framework to study soil aggregate dynamics. Eur. J. Soil Sci. 70, 466–479. doi: 10.1111/ejss.12775
Masiello, C. A., and Druffel, E. M. (1998). Black carbon in deep-sea sediment. Science 280, 1911–1913. doi: 10.1126/science.280.5371.1911
Meng, Y., Gou, R., Bai, J., Moreno-Mateos, D., Davis, C. C., Wan, L., et al. (2022). Spatial patterns and driving factors of carbon stocks in mangrove forests on Hainan Island, China. Glob. Ecol. Biogeogra. 31, 1692–1706. doi: 10.1111/geb.13549
Perez, A., Libardoni, B. G., and Sanders, C. J. (2018). Factors influencing organic carbon accumulation in mangrove ecosystems. Biol. Lett. 14:20180237. doi: 10.1098/rsbl.2018.0237
Ray, R., Ganguly, D., Chowdhury, C., Dey, M., Das, S., Dutta, M., et al. (2011). Carbon sequestration and annual increase of carbon stock in a mangrove forest. Atmos. Environ. 45, 5016–5024. doi: 10.1016/j.atmosenv.2011.04.074
Ruellan, S., and Cachier, H. (2001). Characterisation of fresh particulate vehicular exhausts near a Paris high flow road. Atmos. Environ. 35, 453–468. doi: 10.1016/S1352-2310(00)00110-2
Sanders, C. J., Eyre, B. D. I, Santos, R., Machado, W., Luiz-Silva, W., Smoak, J. M., et al. (2014). Elevated rates of organic carbon, nitrogen, and phosphorus accumulation in a highly impacted mangrove wetland. Geophys. Res. Lett. 41, 2475–2480. doi: 10.1002/2014GL059789
Sanders, C. J., Smoak, J. M., Naidu, A. S., Sanders, L. M., and Patchineelam, S. R. (2010). Organic carbon burial in a mangrove forest, margin and intertidal mud flat. Estuar. Coast. Shelf Sci. 90, 168–172. doi: 10.1016/j.ecss.2010.08.013
Smith, A., Marín-Spiotta, E., De Graaff, M., and Balser, T. (2014). Microbial community structure varies across soil organic matter aggregate pools during tropical land cover change. Soil Biol. Biochem. 77, 292–303. doi: 10.1016/j.soilbio.2014.05.030
Taillardat, P., Friess, D. A., and Lupascu, M. (2018). Mangrove blue carbon strategies for climate change mitigation are most effective at the national scale. Biol. Lett. 14:20180251. doi: 10.1098/rsbl.2018.0251
Tue, N. T., Ngoc, N. T., Quy, T. D., Hamaoka, H., Nhuan, M. T., and Omori, K. (2012). A cross-system analysis of sedimentary organic carbon in the mangrove ecosystems of Xuan Thuy National Park, Vietnam. J. Sea Res. 67, 69–76. doi: 10.1016/j.seares.2011.10.006
Wang, M., Liu, X., Wu, W., Zeng, H., Liu, M., and Xu, G. (2022). Effects of urbanization intensity on the distribution of black carbon in urban surface soil in South China. Forests 13:406. doi: 10.3390/f13030406
Wang, X. S., Zhang, P., Zhou, H. Y., and Fu, J. (2012). Association of black carbon with polycyclic aromatic hydrocarbons and heavy metals in urban topsoils and environmental implications. Int. J. Environ. Stud. 69, 705–713. doi: 10.1080/00207233.2012.702414
Wang, X., Cui, L., Xiao, J., and Ding, Z. (2013). Stable carbon isotope of black carbon in lake sediments as an indicator of terrestrial environmental changes: An evaluation on paleorecord from Daihai Lake. Inner Mongolia, China. Chem. Geol. 347, 123–134. doi: 10.1016/j.chemgeo.2013.03.009
Yeomans, J. C., and Bremner, J. M. (1988). A rapid and precise method for routine determination of organic carbon in soil. Commun. Soil Sci. Plant Anal. 19, 1467–1476. doi: 10.1080/00103628809368027
Zhang, X., Xu, Y., Xiao, W., Zhao, M., Wang, Z., Wang, X., et al. (2022). The hadal zone is an important and heterogeneous sink of black carbon in the ocean. Commun. Earth Environ. 3, 1–9. doi: 10.1038/s43247-022-00351-7
Keywords: mangroves, sediments, black carbon, distribution, organic carbon
Citation: Wang M, Sun Y, Zeng H, Wu W, Deng L and Tu P (2022) Distribution of black carbon in sediments from mangrove wetlands in China. Front. For. Glob. Change 5:989329. doi: 10.3389/ffgc.2022.989329
Received: 08 July 2022; Accepted: 23 August 2022;
Published: 09 September 2022.
Edited by:
Maurizio Ventura, Free University of Bozen-Bolzano, ItalyReviewed by:
Thang Ha, Hue University, VietnamAsep Hidayat, Forest Research and Development Center, Indonesia
Copyright © 2022 Wang, Sun, Zeng, Wu, Deng and Tu. This is an open-access article distributed under the terms of the Creative Commons Attribution License (CC BY). The use, distribution or reproduction in other forums is permitted, provided the original author(s) and the copyright owner(s) are credited and that the original publication in this journal is cited, in accordance with accepted academic practice. No use, distribution or reproduction is permitted which does not comply with these terms.
*Correspondence: Panfeng Tu, dHVwYW5mZW5nQDE2My5jb20=