- 1Department of Forest Ecology and Silviculture, Faculty of Forestry, University of Agriculture in Kraków, Kraków, Poland
- 2Department of Ecological Engineering and Forest Hydrology, Faculty of Forestry, University of Agriculture in Kraków, Kraków, Poland
Today, fires constitute one of the most important factors that can affect soil properties, acting at a rapid pace and on a large scale. They often result from climate change. The present study was aimed at examining how water capacity as well as carbon and nitrogen concentration change during a simulated fire of forest mucky soil under laboratory conditions. The combustion was carried out in two short-time variants—for 3 and 9 min. The results presented herewith show that even a short-term fire of the soil cover contributes to visible changes in the physicochemical parameters of the soil. Thermal decomposition of organic matter caused an increase in total carbon and nitrogen concentrations and a slight increase in the Ct/Nt ratio. The burning of soil samples caused a slight upward trend in soil pH and this was associated with soil organic matter (SOM) deprotonation. The experiment showed the effect of mucky soil burning on the change of its water relations. The 3-min combustion significantly increased the maximum water storage capacity (both after 4 and after 24 h of immersion in water), while the 9-min combustion showed significantly worse results.
Introduction
Forest ecosystems cover two-thirds of all land surfaces, which comprise all native plant species (Pimm and Raven, 2000), and sequester about 12% of human-induced carbon (C) dioxide emissions (Pan et al., 2011). Forest wildfires are a common occurrence in many terrestrial ecosystems in Europe, affecting over half a million hectares of forest ecosystems every year (Gray and Dighton, 2009; Khabarov et al., 2016). Such a large scale of the impact of forest fires causes that they temporarily impact the local C balances (Ribeiro-Kumara et al., 2020). Thus, forest fires contribute to the formation of mosaic complex of ecosystems, differing in the state of ecological succession (De Groot et al., 2013; Ribeiro-Kumara et al., 2020). There is a strong correlation between warming trends and forest wildfires (Dezzeo and Chacón, 2005; Viegas, 2005; Castro et al., 2012); namely, increasing temperatures and periodic droughts make wildfires more likely (Coogan et al., 2019). Also wind is an important natural contributor to wildfires, especially when connected with dry weather and high temperatures (de Rigo et al., 2017). Wildfires are mainly a result of human activity, such as ignition, illegal grass burning, fireworks or smoking, while natural causes (e.g., lightnings or self-ignitions) account for a minority of wildfire causes (Trnka et al., 2021). It is well known that fire can alter forest soil properties (Neill et al., 2007), which influence critical processes such as hydrological and biogeochemical cycling (Neary et al., 1999). A widespread fire in forests can influence emissions of greenhouse gasses and aerosols, vegetation dynamics, and global C budgets (Krawchuk et al., 2009; Pellegrini et al., 2018; Walker et al., 2019), with subsequent feedback to future climate change (Bedia et al., 2015; Harris et al., 2016).
Although wildfires are not the main disturbing factor responsible for shaping forest ecosystem communities in Central Europe, they play important role in dry and moderately moist regions (Leuschner and Ellenberg, 2017). The impact of wildfires on forests in Central Europe has not been widely studied (Dzwonko et al., 2015). In particular, also the response of soils highly susceptible to fire was not thoroughly examined. Poland is classified as third country in Europe for the average yearly number of forest fires (Schmuck et al., 2010), which occur mainly during the summer period of drought and elevated surface temperatures. In Poland, in period 1990–2016, an area (consisting natural ecosystems) of more than 7,300 ha burned annually (San-Miguel-Ayanz et al., 2017). Given that recent climatic forecasts predict more frequent periods of favorable wildfire weather (Khabarov et al., 2016), reported area is likely to increase.
Fire is a major threat across various forest types (Harden et al., 2000; Schoennagel et al., 2017; Pellegrini et al., 2018) because of its effects on the soil’s physical, chemical, and biological properties (Certini, 2005; Boerner et al., 2009; Alcañiz et al., 2018). The abundance, biomass, activity, and biodiversity of microorganisms are all affected by fire (Mataix-Solera et al., 2009). Burning changes soil acidity, water storage capacity, and nutrient concentration by consuming plant biomass, litter layers, and soil organic matter (Certini, 2005; Hubbert et al., 2006; Hamman et al., 2008). There is evidence that fire increases soil water repellency, decreasing permeability and stimulating runoff (Imeson et al., 1992; DeBano, 2003). This is mainly due to the degree of soil dryness, even reaching the “critical soil moisture thresholds” (Dekker and Ritsema, 1996; Doerr and Thomas, 2000). During the fire, the nutrient content of the soil is immediately reduced because of volatilization, smoke, ash, leaching, and erosion (Arocena and Opio, 2003; Wanthongchai et al., 2008). The effects of fire on soil properties depend on several variables, such as fire type, intensity, terrain, region, and fire weather conditions (e.g., temperature, precipitation, and drought) (Certini, 2005; Fonseca et al., 2017; Alcañiz et al., 2018).
Fire changes specific physicochemical properties of burned soil (Wondafrash et al., 2005), and soil heating changes occur in particle size distribution in burned soils by aggregating clay and silt particles into coarse and sand particles (Ketterings et al., 2000; Zavala et al., 2014; Doerr and Santín, 2016). Consumption of organic matter and loss of macro-pores (>0.6 mm) also affect the organic matter content, bulk density, and porosity of soils, particularly in the top few centimeters (Ketterings et al., 2000; Zavala et al., 2014; Doerr and Santín, 2016). Increased bulk density and soil organic matter loss render burnt soils less effective at storing water.
A fire can increase nutrient availability mainly by increasing ash production and mineralization. The heat from the fire can produce ashes with a variety of properties, whereas increased pH values can enhance the availability of nutrients—e.g., the availability of Fe, Mn, and Zn decreases with increasing pH values. Fires, however, can also lead to decreased nutrient availability over time because organic matter and microbial biomass release nutrients into the ecosystem, which are removed by leaching and runoff (Wüthrich et al., 2002; Miesel et al., 2012).
The significance of fire in the terrestrial C cycle and nutrient dynamics has been studied extensively in both indigenous and planted forests in boreal and temperate zones and tropical slash-and-burn agriculture (Carter and Foster, 2004; Geldenhuys and van Wilgen, 2004; Morley et al., 2004; Kashian et al., 2006; Gough et al., 2007; Irvine et al., 2007; Kurz et al., 2008; Meigs et al., 2009). However, there are still a lot of uncertainties in a temperate zone forest of Central Europe, particularly in dry or drained carbon-rich soils subjected to fire. Intended draining of these soils disturbs hydrological relations by lowering the water table position, thus exposing whole ecosystems to more severe and frequent wildfires (Turetsky et al., 2011; Reddy et al., 2015). Fires in forest ecosystems with mucky or peat soils are worrying due to the considerable emissions to atmosphere of terrestrially stabilized carbon (Reddy et al., 2015). Because the effects of fire on soils are so diverse (Ponder et al., 2009; Chen et al., 2018), it is essential to comprehend how fire affects soil carbon and nutrients in temperate zone forests in order to sustain soil fertility in areas where fires have occurred.
This research is conducted in laboratory, with the aim to evaluate the impact of simulated short-term fire durations on physicochemical and hydrological properties of forest dewatered soil in temperate zone forest. We hypothesize that short-time fire has significant impact on (1) chemical properties of forest mucky topsoil (particularly the content of carbon, nitrogen and pH); (2) short-time fire causes deterioration of physiochemical properties of forest mucky topsoil. The obtained results may contribute to the understanding of physiochemical changes in soils particularly susceptible to fires, which are undoubtedly drained peatlands, i.e., mucky soils.
Materials and methods
Study site and sampling
Soil samples were collected from a forest complex in the Krzeszowice Forest District, southern Poland. The forest stand in the study site is multistoried and consists of birch, with a minor contribution of oak, larch, alder, and spruce. The habitat is moderately moist, with rainwater being the dominant moisture regime (Bureau of Forest Management and Geodesy Branch Office in Krakow, 2012). A brief summary of the study site characteristics can be found in Table 1.
According to the World Reference Base of Soil Resources, the soil in the study site is classified as Umbric Gleysol (Arenic) (FAO, 2014). The topsoil in the study plot consisted of muck with an average thickness of about 5 cm. This is due to disturbed water relations associated with past drainage and lowering of water table on the forested area. The soil is developed on Quaternary glacial sands.
The average annual temperature in the study site is about 8°C. The highest average air temperature occurs in July and reaches 18°C, while the lowest average air temperature occurs in January and is –2°C. Annual rainfall is about 600 mm, with a maximum in July (100 mm) and a minimum in February (30 mm) (Bureau of Forest Management and Geodesy Branch Office in Krakow, 2012).
In the study site, six soil cores were taken randomly with a minimum distance of 3 m between each plot. The sampling was performed using steel frames (25 cm width × 25 cm length × 15 cm height) to collect the intact topsoil with organic (O) horizon. The organic overburden consisted mainly of branches and litterfall. Sampling took place in June during summer, when the litter is dry and there is a high risk of wildfire. Moreover, litter derived during the previous fall could undergo the process of initial decomposition. Intact soil samples were stored in plastic bags and transported to the laboratory.
Laboratory analysis
After transporting the frames with intact soil samples from the research site to laboratory, they were immediately subjected to analyzes. Four of the six frames were subjected to burning of the top layer using a propane-butane blow lamp. The average temperature that was used, given by the manufacturer, is 1,700 degrees Celsius. Two frames were left as a control. The burning was divided into two stages, i.e., two frames were burnt for 3 min and two frames were burnt for 9 min (Figure 1). The combustion area was small (625 cm2) compared to large-scale fires; therefore, the burning times were also relatively short.
The choice of 3 and 9 min was due to earlier control tests and the appearance of the sample after a longer and shorter fire simulation time. After 3 min, the top layer of the litter was burnt, and after 9 min, we could observe the symptoms of burning in deeper layers of soil. Frames were burnt separately, after which were left to smolder. The burning was performed from a distance of about 5 cm above the soil surface (Figure 1). Burning was done in regular bands, evenly covering the soil in the frames.
After the burning, 20 intact soil samples were collected from the topsoil of each group (control, 3 min and 9 min of burning). To standardize the sampling procedure, we collected soil samples using 100 mL Kopecky cylinders (5 cm of diameter, 5.1 cm of height).
15 soil samples collected into the cylinders from each group were subjected to a maximum water storage capacity (Smax) analysis. In total, 45 samples were tested. The soil-filled cylinders were closed and weighted. The cylinders were then watered with deionized water and left for 24 h. After 4 and 24 h, the weight of the samples was measured. The samples were then dried at 105°C for 24 h. The dried samples were weighted again. Previously, the empty cylinders were also weighted to know the absolute weight of the samples. The procedure of calculating the maximum water storage capacity is described in section “Statistical analysis.”
Regardless of the Smax analysis, five soil cylinders (15 cylinders in total) were prepared from each of the three groups (control, 3-min burning and 9-min burning), and subjected to chemical analysis. Samples were air-dried at room temperature. After removal of roots, 100 g of the soil was used to determine particle size distribution using the sieving method. The remaining soil was sieved through a 2 mm sieve. From samples thus prepared, subsamples (10 g each) were taken and ground in a ball mill (Fritsch) for the analysis of total carbon (Ct) and total nitrogen (Nt) concentrations in a LECO TrueMac Analyser (Leco, St. Joseph, MI, United States). The remaining sieved soil was used for the estimation of pH and total acidity. The soil pH was measured using a potentiometric method in distilled water (pHH2O) and in 1 M potassium chloride (pHKCl) solution (1:5 mass to volume) (Buurman et al., 1996). The total acidity (TA) was measured after extracting 10 g of soil with 30 mL 1 M calcium acetate [(CH3COO)2Ca], shaken for 1 h and filtered. 25 mL of the extract obtained was titrated by potentiometric titration (using automatic titrator, Mettler Toledo, Inc., Columbus, Ohio) to pH 8.2 with 0.1 M sodium hydroxide (NaOH) (Buurman et al., 1996).
Statistical analysis
To check the normality of the distribution within analyzed groups (control, 3 min of burning, 9 min of burning), the Shapiro-Wilk test was performed. Kruskal-Wallis ANOVA was used to examine the differences in the studied parameters between groups. Duncan’s test was used as a post hoc analysis. Mann-Whitney test was used to compare two groups. In order to evaluate relationships between investigated soil properties, we calculated the Pearson’s correlation coefficients, as well as conducting principal component analysis (PCA). Significance was defined at p < 0.05.
The method for determining the maximum water storage capacity (Smax24) involves calculating the difference in mass between absolutely dry samples and the mass of the same samples fully immersed in water for 24 h.
The maximum water storage capacity was calculated according to the formula (1) (Klamerus-Iwan et al., 2020):
where: Smax—maximum water storage capacity after 24 h of soaking in water; Wgw—water-saturated soil mass after 24 h in water; Wgs—dry soil mass.
In addition, we checked whether the water storage capacity changes during soaking. To accomplish this, soil samples were additionally weighed after 4 h of soaking, which resulted in obtaining the Smax4 parameter.
All statistical analyses were performed using R statistical software, using packages “ggplot2” and “factoextra” (Wickham, 2016; Kassambara and Mundt, 2020; R Core Team, 2022).
Results
In overall, the analyzed soil consisted mainly of medium sand with a small admixture of gravel, silt and clay (Table 2).
No significant differences in Ct concentration were observed between the studied groups (Figure 2A). However, the comparison of the control group with the merged burned samples highlighted the significant effect of burning on Ct concentration (control vs. combustion for 3+9 min, mean ± std. deviation: 45.5 ± 8.4 g kg–1 vs. 56.8 ± 8.1 g kg–1; p < 0.05 according to Mann-Whitney test). Regardless of the duration, burning caused an increase in the concentration of Nt (Figure 2B). The mean concentration of Nt in control group was 3.5 ± 0.4 g kg–1, whereas in the merged burned samples, it was 4.1 ± 0.4 g kg–1 (p < 0.05). The Ct/Nt ratio did not differ between the groups (Figure 2C), despite increasing the value along with burning time (trend near statistical significance, p = 0.09; R2 = 0.2). The pH of the samples, analyzed for all groups, was moderately acidic (pHH2O, mean ± std. deviation: 5.49 ± 0.26; pHKCl 5.05 ± 0.33) (Figures 2D,E). Although combustion did not result in a statistically significant increase in Ct, slightly higher averages were observed in the burned samples (Figure 2A). There were no significant differences between the groups in terms of total acidity values (Figure 2F).
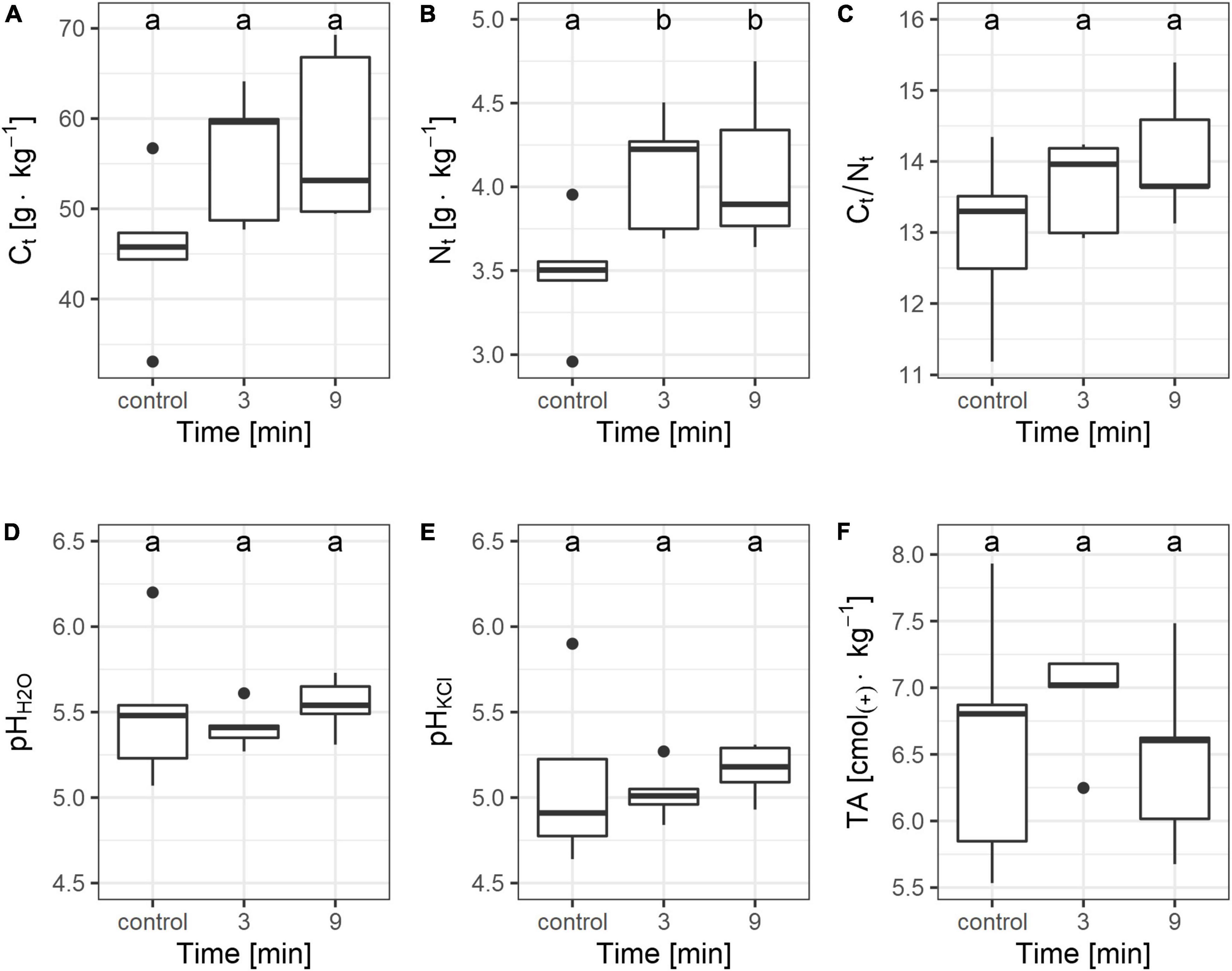
Figure 2. Total C (A), total N (B), Ct/Nt ratio (C), pH in H2O (D) and in KCl (E), total acidity (F) plotted against the time of soil burning. Different letters on this figures indicate significant differences between the groups (according to Duncan test, p < 0.05). The line within the box represents median; the box represents interquartile range; the whiskers represent the largest and the smallest value within 1.5 times interquartile range above 75th and below 25th percentile, respectively; the points represent outliers.
Burning of the topsoil caused an increase in Smax of the tested samples. After 4 h, and again after 24 h, the burned samples had a higher Smax value compared to the control group (Figures 3A,B). The Smax value of the samples burned for 9 min after 4 h (Smax4) was almost equal to the Smax after 24 h of soaking in water (Smax24). In contrast, the Smax24 value of samples burned for 3 min was higher than Smax4.
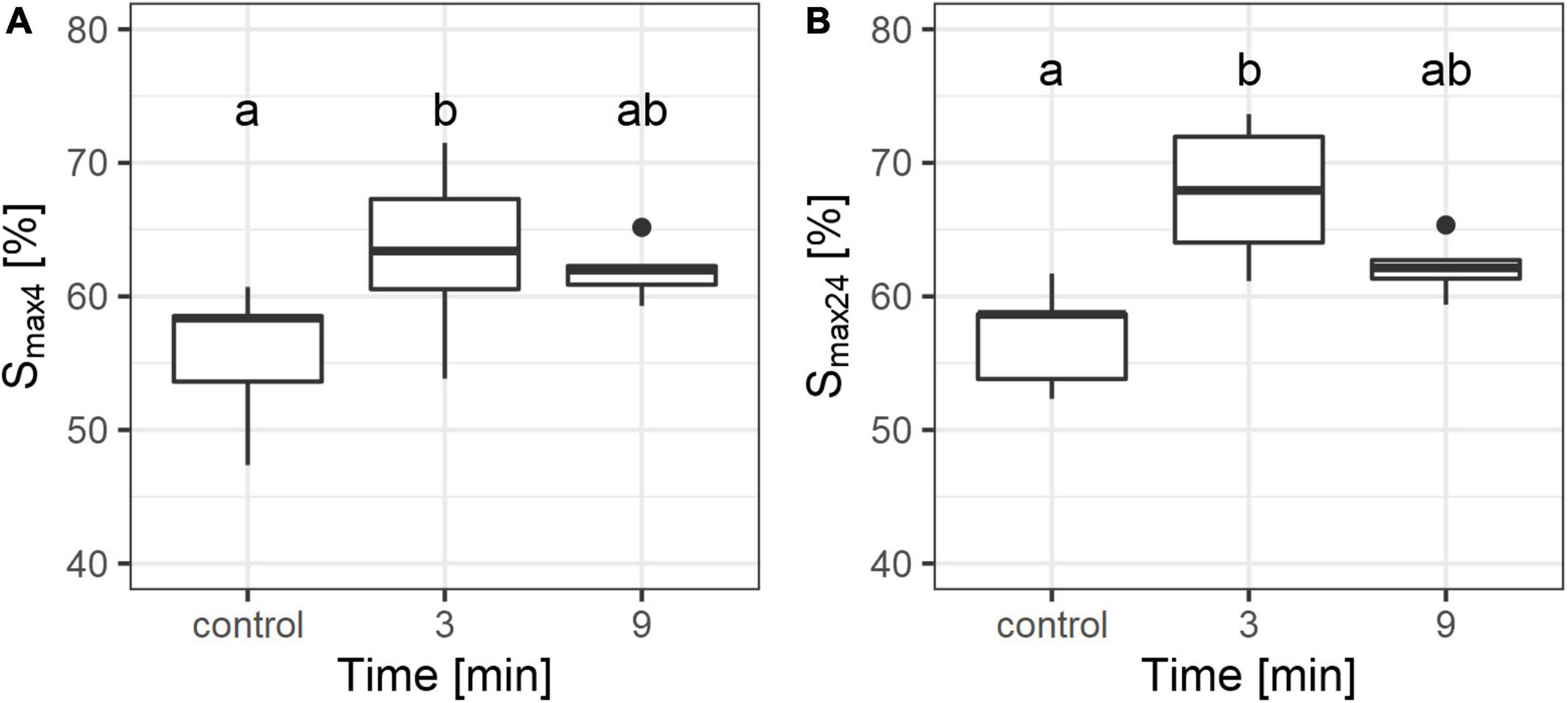
Figure 3. Maximum water storage capacity after 4 h (A) and after 24 h (B) of soil subjected to different combustion times. Different letters on this figures indicate significant differences between the groups (according to Duncan test, p < 0.05). The line within the box represents median; the box represents interquartile range; the whiskers represent the largest and the smallest value within 1.5 times interquartile range above 75th and below 25th percentile, respectively; the points represent outliers.
Analysis of the correlation matrix, presented in Table 3, showed that the burning time of the samples was not significantly correlated with the studied soil physicochemical properties. Smax4 was significantly correlated with Ct and Nt, while Smax24 value was not. However, the PCA provided more precise insights. The first two PCA variables explained altogether 76% of the variance. Projecting the cases onto the component plot showed that the samples of the three analyzed groups were three separate clusters (Figure 4A). Soil samples from the control group occupied the upper and lower left quadrants of the plot. Samples burned for 3 min were the group in the lower right quadrant, and samples burned for 9 min were in the upper right quadrant. A projection of the variables onto the component plot proved a positive correlation of combustion time with the concentration of Ct, Nt and Ct/Nt ratio (Figure 4B). Positive correlation was also found for burning time and Smax, as well as pH values. There was a negligible correlation between the burning time and total acidity.
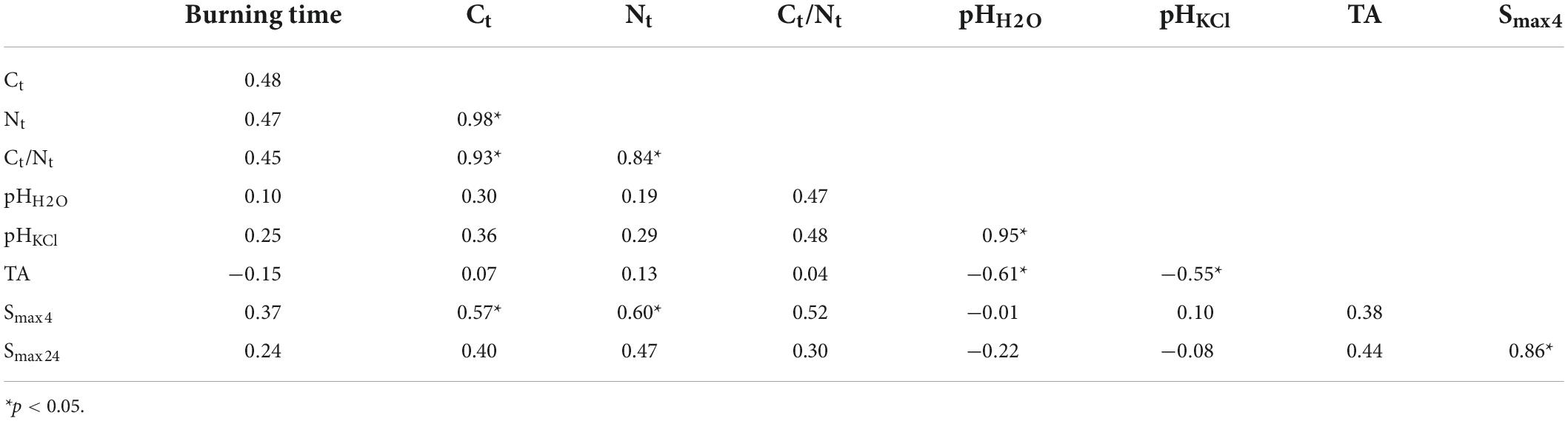
Table 3. Pearson correlation coefficients between the burning time and physicochemical soil properties.
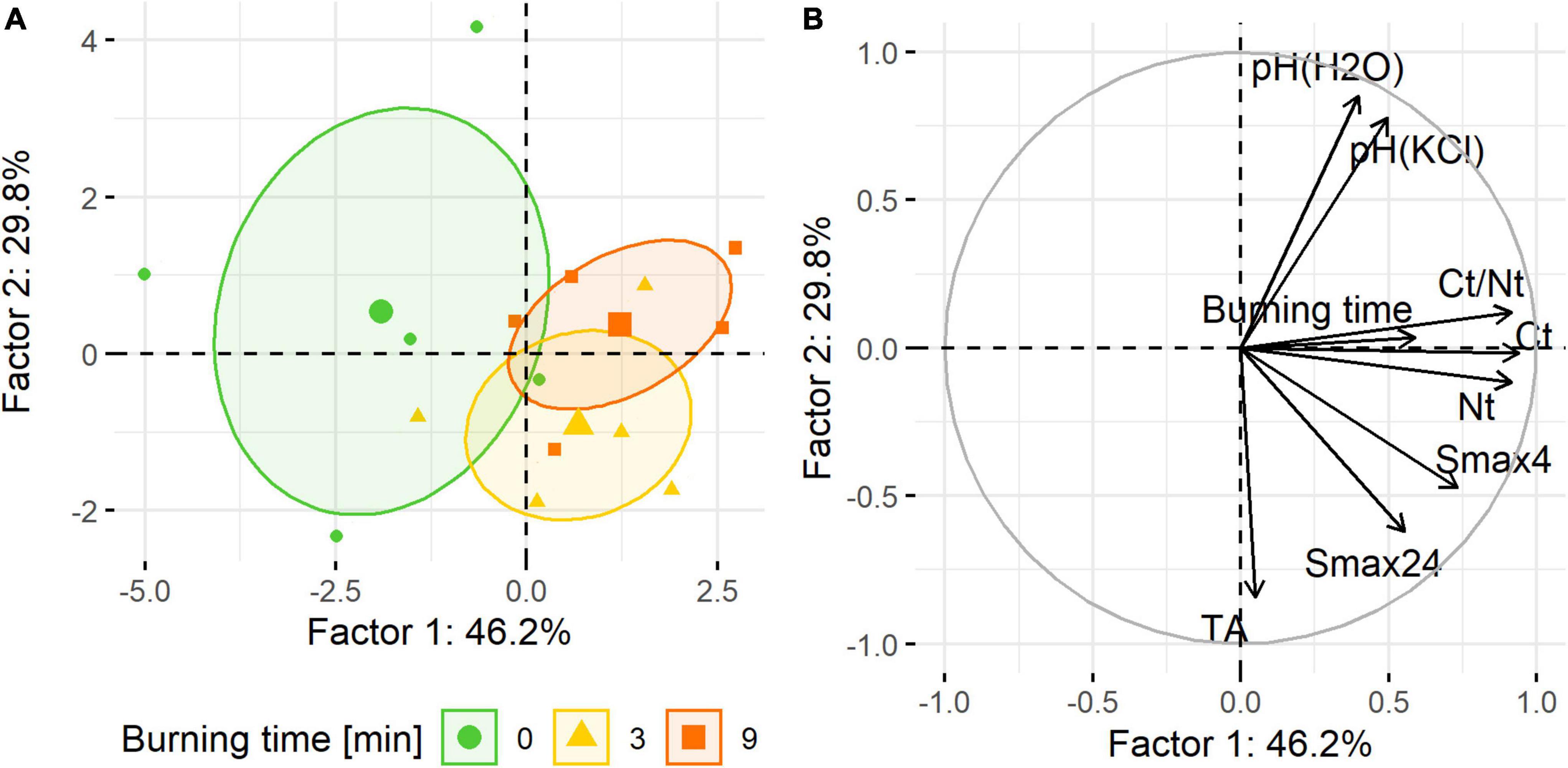
Figure 4. Plot of individuals (PCA) (A) and principal component analysis (B) for the physicochemical properties of soil samples subjected to burning.
Discussion
An impact of fire on soil C and N
Among natural disasters occurring in forests, fires occupy a leading place, and their eradication has a fundamental impact on biocenosis. They cause damage to tree stands, but above all they contribute to changes in the properties of the soil—the component of the ecosystem without which the proper growth and development of phytocenoses is not possible. Fire is a violent, even extreme ecological phenomenon, particularly in the ecosystems exposed to burning due to disrupted hydrological properties. Fires can appear as a result of natural factors such as spontaneous ignition or electrical discharges. At the same time, however, the main perpetrator of fires is man through activities resulting from non-compliance with safety rules, e.g., by burning fires in the forest or burning grass (Wiler and Wcislo, 2007). However, it should be mentioned that wildfires also provide a number of ecosystem services, such as creating new habitats for plant communities, controlling pests, regulate biogeochemical cycles (Pausas and Keeley, 2019).
The effects of a fire in the environment depend on many factors such as duration, severity, weather, forest type, soil moisture or geomorphological situation (Certini, 2005; Benscoter et al., 2011). The research presented in this paper is a brief case study of the effects of wildfires on temperate forest’s dewatered mucky topsoil. In the past, the area from which the soil samples were taken had belonged to peatlands with locally stagnant water. However, land reclamation and anthropogenic disturbances of local water relations, as well as progressing climate changes, caused drainage of the topsoil and lowering of the water table. Therefore, the analyzed soil is even more threatened by fire, thus protection of peatlands and maintenance of their proper water relations are crucial—particularly today, in the era of efforts to sequester CO2 in the soil and to accumulate stable soil organic matter (SOM) fractions (Turetsky et al., 2011).
The greatest intensity of changes in forest ecosystems occurs immediately after the fire, but they are also visible after a few years (Bissett and Parkinson, 1980) or even several dozen years (Monleon and Cromack, 1996; Neary et al., 1999). The results presented in our study demonstrate that even a short-term soil cover fire contributes to visible changes in soil physical and chemical parameters. Thermal decomposition of SOM resulted in increased concentrations of Ct and Nt, as well as a slight increase in the Ct/Nt ratio (Figures 2A–C). The spread, time and intensity of topsoil burning depend precisely on the thickness of the organic layer, and its complete combustion can occur only with unlimited access to oxygen. During the topsoil burning, access to oxygen is significantly limited, which makes the combustion incomplete—on average, 50% of the organic C accumulated in the soil is burned during a fire (Prieto-Fernandez et al., 1998; Santín et al., 2015). Such finding contributes to our results, demonstrating a positive correlation between burning time and Ct concentration in soil (Figures 2A, 4B).
According to Walker et al. (2019), although some part of SOM is released during combustion, the remaining post-fire SOM accumulates in the forest soil. Such SOM (known also as pyrogenic C) is reported to be more resistant to decomposition, which positively induces soil C pools. On the one hand, an increase in Ct is generally a desirable phenomenon because it provides several benefits (e.g., increases soil biodiversity, improves humus quality, increases the share of stable SOM fraction, thus contributing to soil C sequestration) (Lal, 2005, 2013; Bossio et al., 2020; Li et al., 2021). On the other hand, we should note that in the studied samples, the Ct/Nt ratio also increased with burning time. Having said that, the values of this ratio did not exceed 20, therefore it can still be concluded that there were good conditions for SOM decomposition. However, a more significant increase in Ct/Nt ratio could be an important indicator of deterioration of soil fertility and biological activity.
Effect of fire on other soil physio-chemical properties
Burning of the soil samples caused a slight upward trend in soil pH (evident both in pHH2O and in pHKCl). We assume that this phenomenon was related to deprotonation of SOM (dissociation of H+ ions from SOM functional groups), probably connected with the hydrolysis of aluminum, which was reported by some authors (Gruba and Mulder, 2015). In further way, deprotonated SOM functional groups can form organo-mineral complexes with charcoal particles, which significantly contributes to carbon stabilization in soil (Eckmeier et al., 2010). Soil pH also increases after a fire, because OH– groups are lost from clay minerals, oxides are formed, or exchangeable cations are increased in soils (Raison, 1979; Certini, 2005; Zavala et al., 2014). According to various studies, soil pH increases due to the production of potassium (K) and sodium (Na) oxides, hydroxides, and carbonates within the ashes (Ulery and Graham, 1993), as well as the denaturation of organic acids with heating (Scharenbroch et al., 2012). Also, the carbonates and hydroxides derived from burnt plant material can contribute to the increase in soil pH (Schafer and Mack, 2010). Our experiment demonstrated the effect of topsoil burning on changing its water relations but in a more complicated way. A 3-min burning significantly increased the maximum water storage capacity (both after 4 and after 24 h), while a 9-min burning provided visibly worse results (Figures 3A,B). This may indicate that longer simulation of the fire contributed to the (over)drying of the soil, resulting in increased SOM hydrophobicity. Overdrying of the soil and destruction of the natural cover causes frequent occurrence of negative water balance in areas affected by fires. A factor changing the sorption properties of the soil may include the increase in soil density caused by the sealing of pores by ash (Certini, 2005). The end result of sealing soil pores is a decrease in water capacity, a decrease in the rate of infiltration, and thus an increase in surface runoff (DeBano, 2003). Changes in the hydrological properties of the soil as a result of over drying constitute a complicated phenomenon, dependent on many factors. Some laboratory experiments (Doerr and Santín, 2016) indicate that a considerable increase in soil moisture does not necessarily lead to a significant reduction in hydrophobicity. Hydrophobic effect occurs when the surface tension of topsoil is lower than that of water (Zisman, 1964; Doerr and Santín, 2016). The time of combustion influences the degree of thermal decomposition of SOM and oxidation of its functional groups, which is manifested in the change of water, physical and chemical soil parameters. In our experiment, groups of soil samples with different duration of burning (0, 3, and 9 min) constituted three different groups, differing in physiochemical properties, basing on PCA analysis (Figure 4A). We suggest the mechanism that short burning caused in an increase in soil ash content with no increase in SOM hydrophobicity and no significant soil drying, leading to increased water storage capacity.
Further perspectives
Our results show that even short-term forest floor fires can contribute to changes in physiochemical properties of mucky soils in temperate zone forests. Therefore, in order to better understand the impact of fires, among other things, on the hydrological properties of the forest floor, further research is necessary, taking into account the quantity and quality of organic matter, especially since it is the organic matter that usually has a greater capacity to store water (Chen et al., 2018). The research agenda in undertaken topic should consider field experiments, which regard the impact of climatic and environmental factors, that could affect the hydrophysical soil properties. Also, considering different fire regimes in the background of climate change could fill an important gap in the relations between wildfires and ecosystem services (Pausas and Keeley, 2019). Future research should take into account the aforementioned factors as well as the different soil types and longer burning duration. In addition, in order to better understand the impact of fires on soil hydrology under global climate change conditions, further research should consider both the impact of drought on fire occurrence and the consequences of fires such as soil over drying.
Data availability statement
The raw data supporting the conclusions of this article will be made available by the authors, without undue reservation.
Author contributions
AK-I and ES-O: conceptualization. AK-I, ES-O, and DK: methodology. AK-I, ES-O, DK, MK, and AK: validation, writing—original draft preparation, and writing—review and editing. All authors contributed to the article and approved the submitted version.
Funding
This research was financed by the Ministry of Science and Higher Education of the Republic of Poland and the Department of Ecological Engineering and Forest Hydrology.
Acknowledgments
We would like to thank Mateusz Karwan for his assistance with field and laboratory measurements. We also thank reviewers for their helpful suggestions, which improved the manuscript.
Conflict of interest
The authors declare that the research was conducted in the absence of any commercial or financial relationships that could be construed as a potential conflict of interest.
Publisher’s note
All claims expressed in this article are solely those of the authors and do not necessarily represent those of their affiliated organizations, or those of the publisher, the editors and the reviewers. Any product that may be evaluated in this article, or claim that may be made by its manufacturer, is not guaranteed or endorsed by the publisher.
References
Alcañiz, M., Outeiro, L., Francos, M., and Úbeda, X. (2018). Effects of prescribed fires on soil properties: A review. Sci. Total Environ. 613–614, 944–957. doi: 10.1016/j.scitotenv.2017.09.144
Arocena, J. M., and Opio, C. (2003). Prescribed fire-induced changes in properties of sub-boreal forest soils. Geoderma 113, 1–16. doi: 10.1016/S0016-7061(02)00312-9
Bedia, J., Herrera, S., Gutiérrez, J. M., Benali, A., Brands, S., Mota, B., et al. (2015). Global patterns in the sensitivity of burned area to fire-weather: Implications for climate change. Agric. For. Meteorol. 214–215, 369–379. doi: 10.1016/j.agrformet.2015.09.002
Benscoter, B. W., Thompson, D. K., Waddington, J. M., Flannigan, M. D., Wotton, B. M., de Groot, W. J., et al. (2011). Interactive effects of vegetation, soil moisture and bulk density on depth of burning of thick organic soils. J. Wildl. Fire 20, 418–429. doi: 10.1071/WF08183
Bissett, J., and Parkinson, D. (1980). Long-term effects of fire on the composition and activity of the soil microflora of a subalpine, coniferous forest. Can. J. Bot. 58, 1704–1721. doi: 10.1139/b80-199
Boerner, R. E. J., Huang, J., and Hart, S. C. (2009). Impacts of Fire and Fire Surrogate treatments on forest soil properties: A meta-analytical approach. Ecol. Appl. 19, 338–358. doi: 10.1890/07-1767.1
Bossio, D. A., Cook-Patton, S. C., Ellis, P. W., Fargione, J., Sanderman, J., Smith, P., et al. (2020). The role of soil carbon in natural climate solutions. Nat. Sustain. 3, 391–398. doi: 10.1038/s41893-020-0491-z
Bureau of Forest Management and Geodesy Branch Office in Krakow (2012). Forest management plan 2012 – 2021 of Krzeszowice forest district. Krakow: Bureau of Forest Management and Geodesy Branch Office in Krakow. 0
Buurman, P., van Lagen, B., and Velthorst, E. J. (1996). Manual for soil and water analysis. Leiden: Backhuys Publishers.
Carter, M. C., and Foster, C. D. (2004). Prescribed burning and productivity in southern pine forests: A review. For. Ecol. Manage. 191, 93–109. doi: 10.1016/j.foreco.2003.11.006
Castro, A. C. M., Carvalho, J. P., and Meixedo, J. P. (2012). A qualitative description of soil parameters variation due to a prescribed fire in Portuguese northwestern forests using Fuzzy Boolean Nets – The case study of Cabreira mountain. Geoderma 191, 89–96. doi: 10.1016/j.geoderma.2012.02.008
Certini, G. (2005). Effects of fire on properties of forest soils: A review. Oecologia 143, 1–10. doi: 10.1007/s00442-004-1788-8
Chen, S., Cao, T., Tanaka, N., Gao, T., Zhu, L., and Zou, C. B. (2018). Hydrological properties of litter layers in mixed forests in Mt. Qinling, China. IForest 11, 243–250. doi: 10.3832/ifor2535-011
Coogan, S. C. P., Robinne, F. N., Jain, P., and Flannigan, M. D. (2019). Scientists’ warning on wildfire — a canadian perspective. Can. J. For. Res. 49, 1015–1023. doi: 10.1139/cjfr-2019-0094
De Groot, W. J., Cantin, A. S., Flannigan, M. D., Soja, A. J., Gowman, L. M., and Newbery, A. (2013). A comparison of canadian and russian boreal forest fire regimes. For. Ecol. Manage. 294, 23–34. doi: 10.1016/j.foreco.2012.07.033
de Rigo, D., Libertà, G., Houston Durrant, T., Artés Vivancos, T., and San-Miguel-Ayanz, J. (2017). Forest fire danger extremes in Europe under climate change?: Variability and uncertainty. Luxembourg: Publications Office of the European Union. doi: 10.2760/13180
DeBano, L. F. (2003). The role of fire and soil heating on water repellency. Soil Water Repellency Occur. Consequences. Amelior 232, 193–202. doi: 10.1016/B978-0-444-51269-7.50020-5
Dekker, L. W., and Ritsema, C. J. (1996). Variation in water content and wetting patterns in dutch water repellent peaty clay and clayey peat soils. Catena 28, 89–105. doi: 10.1016/S0341-8162(96)00047-1
Dezzeo, N., and Chacón, N. (2005). Carbon and nutrients loos in aboveground biomass along a fire induced forest-savanna gradient in the Gran Sabana, southern Venezuela. For. Ecol. Manage. 209, 343–352. doi: 10.1016/j.foreco.2005.02.008
Doerr, S. H., and Santín, C. (2016). Global trends in wildfire and its impacts: Perceptions versus realities in a changing world. Philos. Trans. R. Soc. B Biol. Sci. 371:20150345. doi: 10.1098/rstb.2015.0345
Doerr, S. H., and Thomas, A. D. (2000). The role of soil moisture in controlling water repellency: New evidence from forest soils in Portugal. J. Hydrol. 231–232, 134–147. doi: 10.1016/S0022-1694(00)00190-6
Dzwonko, Z., Loster, S., and Gawroński, S. (2015). Impact of fire severity on soil properties and the development of tree and shrub species in a Scots pine moist forest site in southern Poland. For. Ecol. Manage. 342, 56–63. doi: 10.1016/j.foreco.2015.01.013
Eckmeier, E., Egli, M., Schmidt, M. W. I., Schlumpf, N., Nötzli, M., Minikus-Stary, N., et al. (2010). Preservation of fire-derived carbon compounds and sorptive stabilisation promote the accumulation of organic matter in black soils of the Southern Alps. Geoderma 159, 147–155. doi: 10.1016/j.geoderma.2010.07.006
Fonseca, F., de Figueiredo, T., Nogueira, C., and Queirós, A. (2017). Effect of prescribed fire on soil properties and soil erosion in a Mediterranean mountain area. Geoderma 307, 172–180. doi: 10.1016/j.geoderma.2017.06.018
Geldenhuys, C. J., and van Wilgen, B. W. (2004). “Fire effects on the maintenance of biodiversity, soil and nutrients,” in Wildland Fire management handbook for sub-sahara Africa, eds J. G. Goldammer and C. de Ronde (Freiburg: Global Fire Monitoring Center (GFMC)), 88–113.
Gough, C. M., Vogel, C. S., Harrold, K. H., George, K., and Curtis, P. S. (2007). The legacy of harvest and fire on ecosystem carbon storage in a north temperate forest. Glob. Chang. Biol. 13, 1935–1949. doi: 10.1111/j.1365-2486.2007.01406.x
Gray, D. M., and Dighton, J. (2009). Nutrient utilization by pine seedlings and soil microbes in oligotrophic pine barrens forest soils subjected to prescribed fire treatment. Soil Biol. Biochem. 41, 1957–1965. doi: 10.1016/j.soilbio.2009.06.021
Gruba, P., and Mulder, J. (2015). Tree species affect cation exchange capacity (CEC) and cation binding properties of organic matter in acid forest soils. Sci. Total Environ. 511, 655–662. doi: 10.1016/j.scitotenv.2015.01.013
Hamman, S. T., Burke, I. C., and Knapp, E. E. (2008). Soil nutrients and microbial activity after early and late season prescribed burns in a Sierra Nevada mixed conifer forest. For. Ecol. Manage. 256, 367–374. doi: 10.1016/j.foreco.2008.04.030
Harden, J. W., Trumbore, S., Stocks, B. J., Hirsch, A., Gower, S. T., O’Neill, K. P., et al. (2000). The role of fire in the boreal carbon budget. Glob. Chang. Biol. 6, 174–184.
Harris, R. M. B., Remenyi, T. A., Williamson, G. J., Bindoff, N. L., and Bowman, D. M. J. S. (2016). Climate–vegetation–fire interactions and feedbacks: Trivial detail or major barrier to projecting the future of the Earth system? Wiley Interdiscip. Rev. Clim. Chang. 7, 910–931. doi: 10.1002/wcc.428
Hubbert, K. R., Preisler, H. K., Wohlgemuth, P. M., Graham, R. C., and Narog, M. G. (2006). Prescribed burning effects on soil physical properties and soil water repellency in a steep chaparral watershed, southern California, USA. Geoderma 130, 284–298. doi: 10.1016/j.geoderma.2005.02.001
Imeson, A. C., Verstraten, J. M., Van Mulligen, E. J., and Sevink, J. (1992). The effects of fire and water repellency on infiltration and runoff under Mediterranean type forest. Catena 19, 345–361.
Irvine, J., Law, B. E., and Hibbard, K. A. (2007). Postfire carbon pools and fluxes in semiarid ponderosa pine in Central Oregon. Glob. Chang. Biol. 13, 1748–1760. doi: 10.1111/j.1365-2486.2007.01368.x
Kashian, D. M., Romme, W. H., Tinker, D. B., Turner, M. G., and Ryan, M. G. (2006). Carbon storage on landscapes with stand-replacing fires. Bioscience 56, 598–606. doi: 10.1641/0006-3568200656
Kassambara, A., and Mundt, F. (2020). Factoextra: Extract and visualize the results of multivariate data analyses. Available online at: https://cran.r-project.org/web/packages/factoextra/ (accessed April 1, 2022).
Ketterings, Q. M., Bigham, J. M., and Laperche, V. (2000). Changes in soil mineralogy and texture caused by slash-and-burn fires in sumatra, Indonesia. Soil Sci. Soc. Am. J. 64, 1108–1117. doi: 10.2136/sssaj2000.6431108x
Khabarov, N., Krasovskii, A., Obersteiner, M., Swart, R., Dosio, A., San-Miguel-Ayanz, J., et al. (2016). Forest fires and adaptation options in Europe. Reg. Environ. Chang. 16, 21–30. doi: 10.1007/s10113-014-0621-0
Klamerus-Iwan, A., Lasota, J., and Błońska, E. (2020). Interspecific variability of water storage capacity and absorbability of deadwood. Forests 11, 1–12. doi: 10.3390/F11050575
Krawchuk, M. A., Moritz, M. A., Parisien, M. A., Van Dorn, J., and Hayhoe, K. (2009). Global pyrogeography: The current and future distribution of wildfire. PLoS One 4:e5102. doi: 10.1371/journal.pone.0005102
Kurz, W. A., Stinson, G., Rampley, G. J., Dymond, C. C., and Neilson, E. T. (2008). Risk of natural disturbances makes future contribution of Canada’s forests to the global carbon cycle highly uncertain. Proc. Natl. Acad. Sci. U.S.A. 105, 1551–1555. doi: 10.1073/pnas.0708133105
Lal, R. (2005). Forest soils and carbon sequestration. For. Ecol. Manage. 220, 242–258. doi: 10.1016/j.foreco.2005.08.015
Lal, R. (2013). Soil carbon management and climate change. Carbon Manag. 4, 439–462. doi: 10.4155/cmt.13.31
Leuschner, C., and Ellenberg, H. (2017). Ecology of central european forests. Cham: Springer International Publishing Switzerland. doi: 10.1007/978-3-319-43042-3
Li, X. Y., Jin, H. J., Wang, H. W., Marchenko, S. S., Shan, W., Luo, D. L., et al. (2021). Influences of forest fires on the permafrost environment: A review. Adv. Clim. Chang. Res. 12, 48–65. doi: 10.1016/j.accre.2021.01.001
Mataix-Solera, J., Guerrero, C., Garcia-Orenes, F., Barcenas, G. M., and Pilar Torres, M. (2009). “Forest fire effects on soil microbiology,” in Fire effects on soils and restoration strategies, ed. A. Cerca (Boca Raton, FL: CRC Press).
Meigs, G. W., Donato, D. C., Campbell, J. L., Martin, J. G., and Law, B. E. (2009). Forest fire impacts on carbon uptake, storage, and emission: The role of burn severity in the Eastern Cascades, Oregon. Ecosystems 12, 1246–1267. doi: 10.1007/s10021-009-9285-x
Miesel, J. R., Goebel, P. C., Corace, R. G., Hix, D. M., Kolka, R., Palik, B., et al. (2012). Fire effects on soils in Lake States forests: A compilation of published research to facilitate long-term investigations. Forests 3, 1034–1070. doi: 10.3390/f3041034
Monleon, V. J., and Cromack, K. (1996). Long-term effects of prescribed underburning on litter decomposition and nutrient release in ponderosa pine stands in central Oregon. For. Ecol. Manage. 81, 143–152. doi: 10.1016/0378-1127(95)03658-x
Morley, S., Grant, C., Hobbs, R., and Cramer, V. (2004). Long-term impact of prescribed burning on the nutrient status and fuel loads of rehabilitated bauxite mines in Western Australia. For. Ecol. Manage. 190, 227–239. doi: 10.1016/j.foreco.2003.10.016
Neary, D. G., Klopatek, C. C., DeBano, L. F., and Ffolliott, P. F. (1999). Fire effects on belowground sustainability: A review and synthesis. For. Ecol. Manage. 122, 51–71. doi: 10.1016/S0378-1127(99)00032-8
Neill, C., Patterson, W. A., and Crary, D. W. (2007). Responses of soil carbon, nitrogen and cations to the frequency and seasonality of prescribed burning in a Cape Cod oak-pine forest. For. Ecol. Manage. 250, 234–243. doi: 10.1016/j.foreco.2007.05.023
Pan, Y., Birdsey, R. A., Fang, J., Houghton, R., Kauppi, P. E., Kurz, W. A., et al. (2011). A large and persistent carbon sink in the world’s forests. Science 333, 988–993. doi: 10.1126/science.1201609
Pausas, J. G., and Keeley, J. E. (2019). Wildfires as an ecosystem service. Front. Ecol. Environ. 17:289–295. doi: 10.1002/fee.2044
Pellegrini, A. F. A., Ahlström, A., Hobbie, S. E., Reich, P. B., Nieradzik, L. P., Staver, A. C., et al. (2018). Fire frequency drives decadal changes in soil carbon and nitrogen and ecosystem productivity. Nature 553, 194–198. doi: 10.1038/nature24668
Pimm, S. L., and Raven, P. (2000). Extinction by numbers. Nature 403, 843–845. doi: 10.1038/35002708
Ponder, F., Tadros, M., and Loewenstein, E. F. (2009). Microbial properties and litter and soil nutrients after two prescribed fires in developing savannas in an upland Missouri Ozark Forest. For. Ecol. Manage. 257, 755–763. doi: 10.1016/j.foreco.2008.10.009
Prieto-Fernandez, A., Acea, M. J., and Carballas, T. (1998). Soil microbial and extractable C and N after wildfire. Biol. Fertil. Soils 27, 132–142.
R Core Team. (2022). R: A language and environment for statistical computing. Available oneline at: https://www.r-project.org/ (accessed April 1, 2022).
Raison, R. J. (1979). Modification of the soil environment by vegetation fires, with particular reference to nitrogen transofrmations: A review. Plant Soil 51, 73–108.
Reddy, A. D., Hawbaker, T. J., Wurster, F., Zhu, Z., Ward, S., Newcomb, D., et al. (2015). Quantifying soil carbon loss and uncertainty from a peatland wildfire using multi-temporal LiDAR. Remote Sens. Environ. 170, 306–316. doi: 10.1016/j.rse.2015.09.017
Ribeiro-Kumara, C., Köster, E., Aaltonen, H., and Köster, K. (2020). How do forest fires affect soil greenhouse gas emissions in upland boreal forests? A Rev. Environ. Res. 184:109328. doi: 10.1016/j.envres.2020.109328
San-Miguel-Ayanz, J., Durrant, T., Boca, R., Libertà, G., Branco, A., de Rigo, D., et al. (2017). Forest fires in europe, middle east and North Africa 2017.. Luxembourg: Publications Office of the European Union.
Santín, C., Doerr, S. H., Preston, C. M., and González-Rodríguez, G. (2015). Pyrogenic organic matter production from wildfires: A missing sink in the global carbon cycle. Glob. Chang. Biol. 21, 1621–1633. doi: 10.1111/gcb.12800
Schafer, J. L., and Mack, M. C. (2010). Short-term effects of fire on soil and plant nutrients in palmetto flatwoods. Plant Soil 334, 433–447. doi: 10.1007/s11104-010-0394-2
Scharenbroch, B. C., Nix, B., Jacobs, K. A., and Bowles, M. L. (2012). Two decades of low-severity prescribed fire increases soil nutrient availability in a Midwestern, USA oak (Quercus) forest. Geoderma 183–184, 80–91. doi: 10.1016/j.geoderma.2012.03.010
Schmuck, G., San-Miguel-Ayanz, J., Camia, A., Tracy, D., Santos De Oliveira, S., Boca, R., et al. (2010). Forest Fires in Europe. Luxemburg: Publication Officeof the European Union. doi: 10.2788/74089
Schoennagel, T., Balch, J. K., Brenkert-Smith, H., Dennison, P. E., Harvey, B. J., Krawchuk, M. A., et al. (2017). Adapt tomore wildfire in western North American forests as climate changes. Proc. Natl. Acad. Sci. U.S.A. 114, 4582–4590. doi: 10.1073/pnas.1617464114
Trnka, M., Možný, M., Jurečka, F., Balek, J., Semerádová, D., Hlavinka, P., et al. (2021). Observed and estimated consequences of climate change for the fire weather regime in the moist-temperate climate of the Czech Republic. Agric. For. Meteorol. 310:108583. doi: 10.1016/j.agrformet.2021.108583
Turetsky, M. R., Donahue, W. F., and Benscoter, B. W. (2011). Experimental drying intensifies burning and carbon losses in a northern peatland. Nat. Commun. 2:514. doi: 10.1038/ncomms1523
Ulery, A. L., and Graham, R. C. (1993). Forest Fire Effects on Soil Color and Texture. Soil Sci. Soc. Am. J. 57, 135–140. doi: 10.2136/sssaj1993.03615995005700010026x
Walker, X. J., Baltzer, J. L., Cumming, S. G., Day, N. J., Ebert, C., Goetz, S., et al. (2019). Increasing wildfires threaten historic carbon sink of boreal forest soils. Nature 572, 520–523. doi: 10.1038/s41586-019-1474-y
Wanthongchai, K., Bauhus, J., and Goldammer, J. G. (2008). Nutrient losses through prescribed burning of aboveground litter and understorey in dry dipterocarp forests of different fire history. Catena 74, 321–332. doi: 10.1016/j.catena.2008.01.003
Wiler, K., and Wcislo, P. (2007). Protection of forests against fires. Warsaw: Polish State Forests Information Center.
Wondafrash, T. T., Sancho, I. M., Miguel, V. G., and Serrano, R. E. (2005). Relationship between soil color and temperature in the surface horizon of Mediterranean soils: A laboratory study. Soil Sci. 170, 495–503.
Wüthrich, C., Schaub, D., and Weber, M. (2002). Soil respiration and soil microbial biomass after fire in a sweet chestnut forest in southern Switzerland. Catena 48, 201–215.
Zavala, L. M., De Celis, R., and Jordán, A. (2014). How wildfires affect soil properties. A brief review. Cuad. Investig. Geográfica 40:311. doi: 10.18172/cig.2522
Keywords: water storage capacity, dewatered soil, temperate forest wildfire, simulated fire, soil organic matter, forest soil
Citation: Kupka D, Khan MO, Kwika A, Słowik-Opoka E and Klamerus-Iwan A (2022) Experimental short-time wildfire simulation—Physicochemical changes of forest mucky topsoil. Front. For. Glob. Change 5:987010. doi: 10.3389/ffgc.2022.987010
Received: 05 July 2022; Accepted: 24 August 2022;
Published: 08 September 2022.
Edited by:
Robert Michael Scheller, North Carolina State University, United StatesReviewed by:
Daniel Winkler, University of West Hungary, HungarySaskia Keesstra, Wageningen University and Research, Netherlands
Copyright © 2022 Kupka, Khan, Kwika, Słowik-Opoka and Klamerus-Iwan. This is an open-access article distributed under the terms of the Creative Commons Attribution License (CC BY). The use, distribution or reproduction in other forums is permitted, provided the original author(s) and the copyright owner(s) are credited and that the original publication in this journal is cited, in accordance with accepted academic practice. No use, distribution or reproduction is permitted which does not comply with these terms.
*Correspondence: Dawid Kupka, dawid.kupka@urk.edu.pl; Anna Klamerus-Iwan, anna.klamerus-iwan@urk.edu.pl