- 1Plant Reproductive Biology, Genetic Diversity and Phytochemistry Research Laboratory, Department of Botany, University of Kashmir, Srinagar, India
- 2Department of Botany, University of Kashmir, Kargil, Ladakh
Phyto-ecological studies are essential in understanding community structure, organization and their response to changes in other environmental factors. In this study we analyzed the phytosociological and soil characteristics of Phytolacca acinosa communities and their correlation. 110 quadrats were laid across ten randomly selected sites in Kashmir Himalaya, India. Soil analysis was done using standard protocols. Overall, 161 species were recorded, belonging to 128 genera and 49 families. The species richness (SR) ranges from 27 to 83. Highest IVI was recorded for Poa angustifolia (60.06) and least for Berberis lycium, Abies pindrow, Plectranthus ragosus, and Ailanthus altissima (0.37 each). P. acinosa showed 100% random associations with other plant species. Soil properties varied significantly across the selected sites. Significant positive correlation was found between species richness (SR), Organic matter (OM) (r = 0.79), Organic carbon (OC) (r = 0.79) and Shannon–Wiener index (H) (r = 0.92). Nitrogen content also showed positive correlation with SR and H. Floristic composition of P. acinosa assemblages was governed by soil properties and habitat characteristics of sampling sites. Areas with highest floral diversity had high soil fertility while areas with low soil fertility possess lower diversity and need restoration. The knowledge may prove helpful in management of these habitats, boost conservation and mitigate the effects of changing climate.
Introduction
Study of vegetation structure dynamics is a vital aspect of ecology that assists in understanding the function and overall structure of a community. Phytosociology or vegetation analysis is the science that deals with the study of the plant assemblages (communities), their characteristics, composition, classification, distribution, and relationships among species. Its primary goal is to delimit and characterize vegetation types based on the whole species (floristic) composition (Dengler, 2016). Phytosociological studies are important in understanding the population dynamics, habitat, species interaction and vegetation structure of an ecosystem (Collins et al., 2020). The vegetation of an area can be classified based on physiognomy and functional combination or on its component species that discriminate the physical appearance of vegetation (Mumshad et al., 2021). Plant communities are formed by those species which share the structural and floristic characteristics at certain level of environmental tolerance. Various biotic and abiotic factors of a region influence the plant population and vegetation heterogeneity (Jiao et al., 2019; Ocon et al., 2021). Furthermore, edaphic factors and topography complexity impact plant performance, vegetation composition and diversity (Chin and Kupfer, 2020). The phyto-ecological studies are essential in understanding the interrelationship between soil, environment and vegetation structure of a specific region. These investigations are vital in ascertaining the ecological status of species in a community (Ray and George, 2009), besides understanding the species diversity, organization of communities and in selecting valuable species from plant communities (Abinaya and Paulsamy, 2015; Wani Z. A. et al., 2022). The functional capacities of an ecosystem as well as its components are evaluated for ecological assessment. This involves assessment of phytosociological parameters (diversity, abundance, frequency, and important value index) of each species and anthropogenic threats to their habitat that may affect the functional and structural integrity of an ecosystem (Manoj et al., 2012).
Kashmir Himalaya, a scenic landscape of Indian sub-continent, an integral part of the Himalayan biodiversity hotspot. Himalayan region harbors stupendously diverse repository of plants with enormous economic and medicinal value (Aryal et al., 2018; Tali et al., 2019). This biodiversity is sustained by the steep climatic gradient characterized in Mountainous range of Himalaya (Rawal et al., 2018; Rana et al., 2021). In Himalayan ecosystems, floral diversity has a crucial role in maintaining ecological, functional integrity and serving indispensable ecosystem services that have enormous scientific utility (Ahmed et al., 2020). Apart from anthropogenic pressures that include deforestation, land cover change and urbanization, mountain ecosystems are also very sensitive to changing climate which eventually leads to the biodiversity loss (Khan S. M. et al., 2012; Paudel et al., 2018; Yadav et al., 2021). Therefore, for maintaining biodiversity and soil fertility, effective management strategies are mandatory. For the benefit of nature and humans, the United Nations Decade on Ecosystem Restoration (2021–2030) has emphasized restoration and protection of ecosystems across all continents. Healthy ecosystems have the ability to alleviate the effects of changing climate and prevent the loss of biodiversity. Phyto-ecological studies are important in understanding the relationship between vegetation, environment and plant responses toward changing climate (Chawla et al., 2012). Vegetation surveys and documentation will assist in monitoring and evaluating climate change that are vital for conservation and management initiatives (Malfasi and Cannone, 2020).
Phytolacca acinosa Roxb. (Phytolaccaceae) is a perennial herb distributed across Himalaya from Hazara to Bhutan. It is native to Himalaya, east and southeast Asia including Myanmar, China, Taiwan, Bhutan, Japan, Tibet, Vietnam, Manchuria, India, and Korea mostly growing along roadsides, inside forests and forest margins at the elevation of 500–3400 m (POWO., 2022). P. acinosa has immense medicinal importance and is widely used as a diuretic in Chinese folk medicine system and treatment of various diseases such as swelling, edema, indigestion, eye disorder, body ache, and sores (Ma et al., 2017; Basnet and Kalauni, 2020). P. acinosa contains many phytochemicals including tannins, flavonoids, polyphenol, triterpenoids, flavones, saponins, tannic and phenolic compounds (Lin et al., 2018; Ma et al., 2019; Tao et al., 2020). It also exhibits substantial antibacterial, antifungal, antiviral and anti-inflammatory activities (He et al., 2004; Manzoor et al., 2017; Abekura et al., 2019). Keeping in view the medicinal importance of the plant species the phytosociology in relation to soil and ecological parameters were carried out. The data generated will not only be helpful for the management of the species but also the habitats as well, which may prove helpful in conservation of this valuable plant species as well help in restoration of these habitats and mitigate the impacts of climate change.
The aim of our study was to address the following specific questions; (i) the composition of plant assemblages associated with Phytolacca acinosa in the Kashmir Himalaya (ii) soil parameters of the study sites (iii) variability of the ecological parameters of associated communities of the P. acinosa and (iv) the correlation between phytosociological and soil parameters in P. acinosa assemblages across the selected sites of Kashmir Himalaya.
Materials and methods
Study area
The current study was conducted in Kashmir Himalaya, India, situated between 32°20’ to 34°50’ N latitude and 73°55’ to 75°35’ E longitude (Tali et al., 2015) encompassing an area of around 15,948 sq. km. The region is a part of the northwest Himalaya in India and comprises of a deep oval shaped valley, bounded by high mountain ranges; the Lesser or Middle Himalaya, known as the Pir Panjal Range, separates the Valley from the Jammu region in the south and southwest, while the Greater Himalaya separates it from the Ladakh region in the north and east (Figure 1). The altitudinal gradient of the valley ranges from 1500 to 1800 m (asl), whereas the average elevation of the adjacent mountains’ ranges from 3000 to 4000 m, with Kolahoi (5420 m) (Romshoo et al., 2020) being the highest peak. Climate of this region exhibits resemblance with continental-Mediterranean type found along temperate latitudes and is characterized by well-defined seasonality. The mean annual temperature of the region varies from a maximum of 35°C in summer to minimum of −10°C during winter (Zaz et al., 2019) and receives an annual precipitation of roughly 1200 mm predominantly in the form of snow (Romshoo et al., 2020). The vegetation of the area is temperate; subalpine and alpine type harboring enormous floristic diversity.
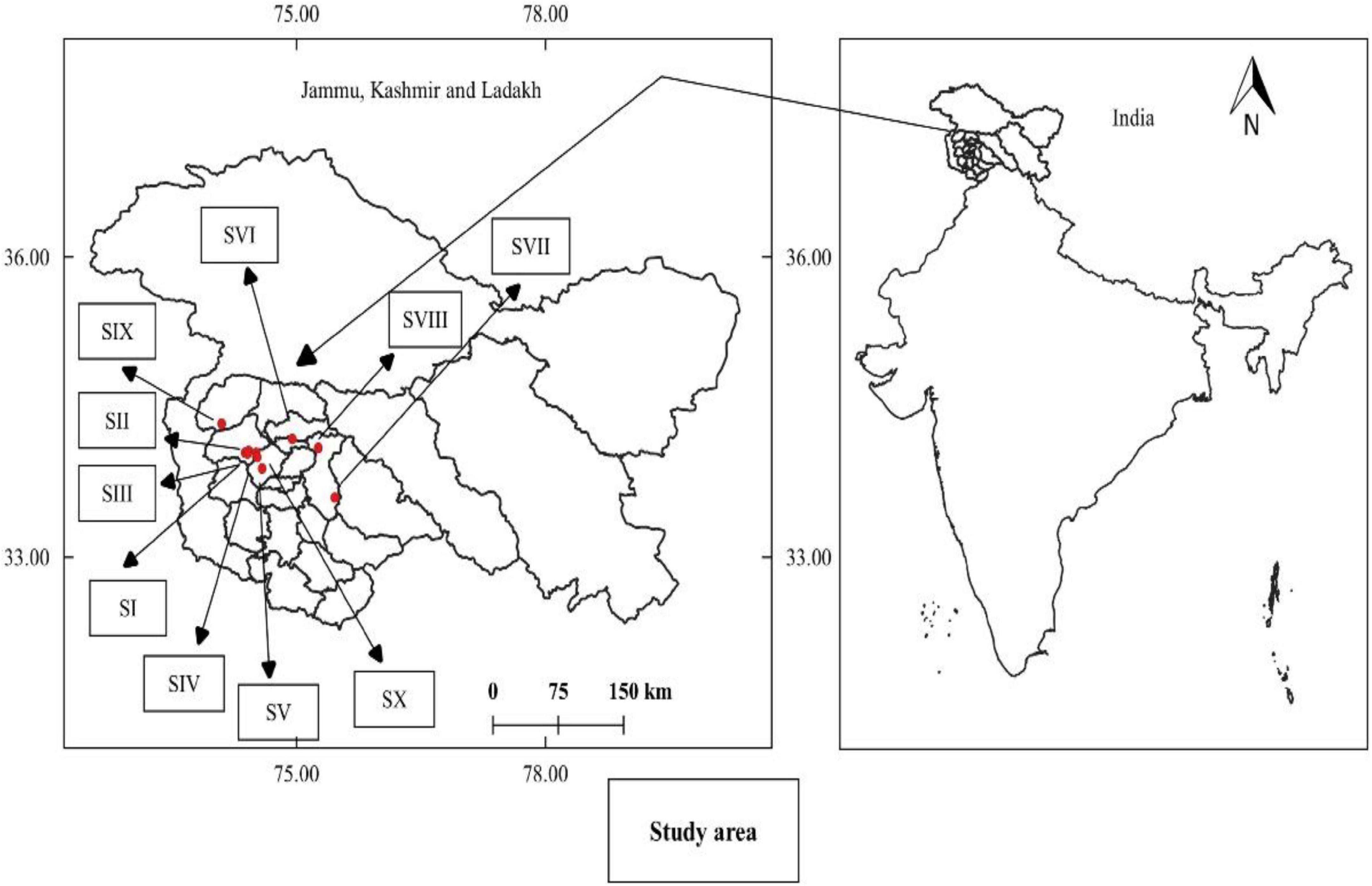
Figure 1. Map showing the study area and sampling sites in Kashmir Himalaya, India. (SI–Tangmarg; SII–Gulmarg; SIII–Drung; SIV–Gogaldara; SV–Doodhpathri; SVI–Dara Harwan; SVII–Daksum; SVIII–Pahalgam; SIX–Bangus; SX–Khag).
Selection of sites
Extensive field surveys were carried out across Kashmir Himalaya and 10 sites were selected based on the presence, abundance and availability of the natural populations of P. acinosa (Figure 1). The selected sites vary visually in vegetation structure and composition. The geo-coordinates and habitat characteristics are given in Table 1.
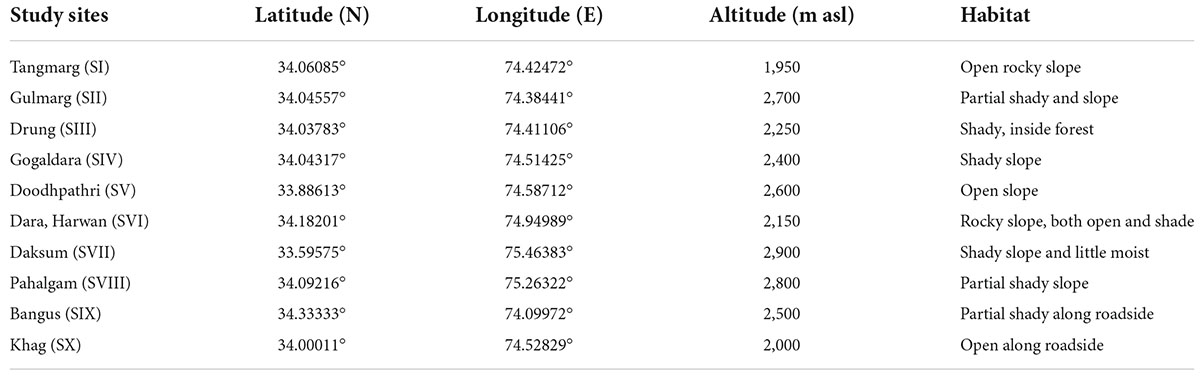
Table 1. Geo-coordinates, altitude and habitat characteristics of the study sites across Kashmir Himalaya, India.
Vegetation sampling
The phytosociological studies were carried out during 2020–2021 across selected sites using the quadrat method (Curtis and McIntosh, 1950). The vegetation diversity was analyzed by using random sampling technique to give the best representation of the floristic composition. A total of 10 study sites (with 110 quadrats) were selected for vegetation sampling. At each site, 8–10 quadrats of 3 m × 3 m size with P. acinosa at center of each quadrat were randomly laid out for sampling. Additional quadrats were laid to distinguish possible unrecognized communities. The shape of quadrat was primarily square but at some instances was modified as per requirement to obtain a homogeneous and representative portrayal of vegetation, e.g., rectangular plots along water streams (Walentowski et al., 2018). Phytosociological characteristics i.e., abundance, frequency, density, relative abundance, relative frequency, relative density, and Importance Value Index (IVI) were a determined at every site for P. acinosa along with constituent plant species (Noreen et al., 2019; Kamran et al., 2020). Distribution levels were noted for other associated species available in the study area. The species richness (SR) was calculated as the total number of species present in a sampling site. Moreover, species diversity was determined using diversity indices such as Simpson’s index (1/D) (Simpson, 1949), Jaccard’s index (J) (Chao et al., 2005), and Shannon–Wiener index (H) (Shannon and Weaver, 1963). All the collected specimens were identified with the help of experts and by perusal the existing literature and online flora (POWO., 2022).
Soil sampling and analysis
For soil analysis, composite soil samples were collected from rhizosphere up to a depth of 15cm from each selected site in triplicates, correctly labeled, air dried in laboratory, and then sieved to remove gravel and debris (Khan et al., 2017; Iqbal et al., 2021). The soil properties—available nitrogen (N), potassium (K), phosphorus (P), soil organic carbon (OC), organic matter (OM), and soil pH were analyzed. The soil samples were analyzed at Soil Testing Laboratory of State Agriculture Department, Lal Mandi, Srinagar, Jammu and Kashmir, India.
The pH of soil was estimated in 1:5 soil–water suspensions using a digital pH meter (Khan S. M. et al., 2012). Likewise, OC in soil was assessed by rapid titration method of Walkley and Black (1934). At each study site, other chemical parameters of soil including available N, K, and P were analyzed using already set methodology of Subbiah and Asija (1956); Black (1968) (flame photometric method); and Olsen et al. (1954) (spectrophotometer method), respectively. Soil organic matter (OM) was computed by multiplying a factor of 1.724 to soil OC (Davies, 1974).
Statistical analysis
All the collected data was analyzed to evaluate the phytosociological attributes of each species, their relationships and effect of various soil properties on the vegetation attributes of the selected area. For further analysis the data related to plant assemblages at all sites (quadrats) was sorted in MS EXCEL as per software requirements. To appraise the impact of edaphic factors on species composition and distribution pattern, the species and soil data were analyzed using correlation analysis in Origin pro 2021b software (version 9.0). The data matrix of species was subjected for cluster analysis to visualize the similarity among the study sites. Bray–Curtis index was employed to estimate the degree of similarity in species composition between the ten selected sites (Ismail and ELawad, 2015) using PAST software (version 4.09). The similarity dendrogram obtained from the result of cluster analysis was plotted. The plant (vegetation) data was also quantitatively analyzed for the determination of frequency, density, abundance as per Mishra et al. (2017); and Curtis and McIntosh (1950) and important value index (IVI) of P. acinosa and associated species at each study site. The IVI of each species was computed by adding up the relative frequency, relative density, and relative abundance following Phillips (1959). Data regarding composition and structure of the natural habitats harboring populations of P. acinosa was recorded across all the selected sites. The sampling was carried out during the flowering period and the data pertaining to P. acinosa assemblages along with the associated plant species was collected from 10 study sites (Table 1). The community composition was analyzed using cooccur package (version 4.0.3) in which co-occurrence data matrix (presence/absence) was envisaged by probabilistic hypergeometric co-occurrence model employed in R Core Team (2020) (Griffith et al., 2016). To extract the species pairings and associated probabilities, we use the prob table() function to retrieve the results element of the cooccur object.
Results
In the present study, 161 plant species were recorded belonging to 128 genera and 49 families (Supplementary Material 1, Figure 2). Out of these plant species, herbs were dominant with 84.47% followed by trees (7.45%), shrubs (5.59%), climber (1.24%), and Fern (1.24%). However, the contribution of different families to the floristic diversity was disproportionate: only 7 families contributed half (50%) of the species pool, while the remaining 50% of the species were contributed by the rest of the 41 families. In terms of the species number, Asteraceae was found as the largest family with 25 species followed by Lamiaceae (13 species), Poaceae and Rosaceae (10 species each), Polygonaceae (8 species), Fabaceae and Caryophyllaceae (7 species each), Ranunculaceae (7 species). There were 21 families represented by a single species recorded at different sites of Kashmir Himalaya (Figure 2). The natural vegetation of a particular region reflects the interaction amongst the associated fauna and flora, edaphic and other environmental factors. The calculated IVI values were used to assign the dominance of species. The highest IVI was recorded for P. angustifolia and least for B. lycium, A. pindrow, P. ragosus, and A. altissima. Based on IVI, Poa angustifolia (IVI 60.06), C. dactylon (53.60), P. pratensis (16.70), U. dioica (30.73), C. iberica (25.04), T. repens (15.17), G. nepalense (24.58), F. nubicola (14.08), and P. aviculare (19.45) were the dominant species in P. acinosa communities across different sampling sites. P. acinosa secured highest and lowest IVI of 11.33 and 4.82 in site II and site X, respectively. Habit-wise, herbaceous flora was dominant with 136 species followed by arboreal flora (12 species), shrubs (9 species), climbers (2 species) and ferns (2 species).
The plant species richness ranged from 27 to 83 and differed between different study sites. C. dactylon and U. dioica were the most common species found across most of the sites. The Shannon-Wiener diversity index (H) was highest at (SVIII)- (4.12). Khag (SX) reported the lowest value of Shanon (H) (2.68), Simpson (1/D) (0.81) and Jaccard’s (J) index (0.9), respectively. The results of current study highlighted variations in species richness (SR) and plant community composition associated with the variability in soil properties across the sampling sites. The highest species richness was found at site SVII (Daksum) while the lowest i.e., 27 species were listed at site SX (Khag).
The phytosociological and soil parameters of selected sites
Site SI (Tangmarg)
The site SI was relatively less diverse having a low value of shannon-weiner index (0.88). Out of 31 plant species, Cynodon dactylon with IVI 53.60 was the dominant one followed by Urtica dioica (IVI 30.73) and Centaurea iberica (25.04). Berberis lycium and Stipa sibirica were the rare species having IVI 2.25 and 2.77, respectively. P. acinosa recorded an IVI of 10.55. The species composition reflects the presence of 30 herbs and a single tree species. The soil at site I was less acidic (pH–6.68) (Table 2). The average OC content at Site I was 3.57% and OM was 6.16%. The value of N, P, and K was 0.02%, 132.43 and 596.59 kg ha–1, respectively.
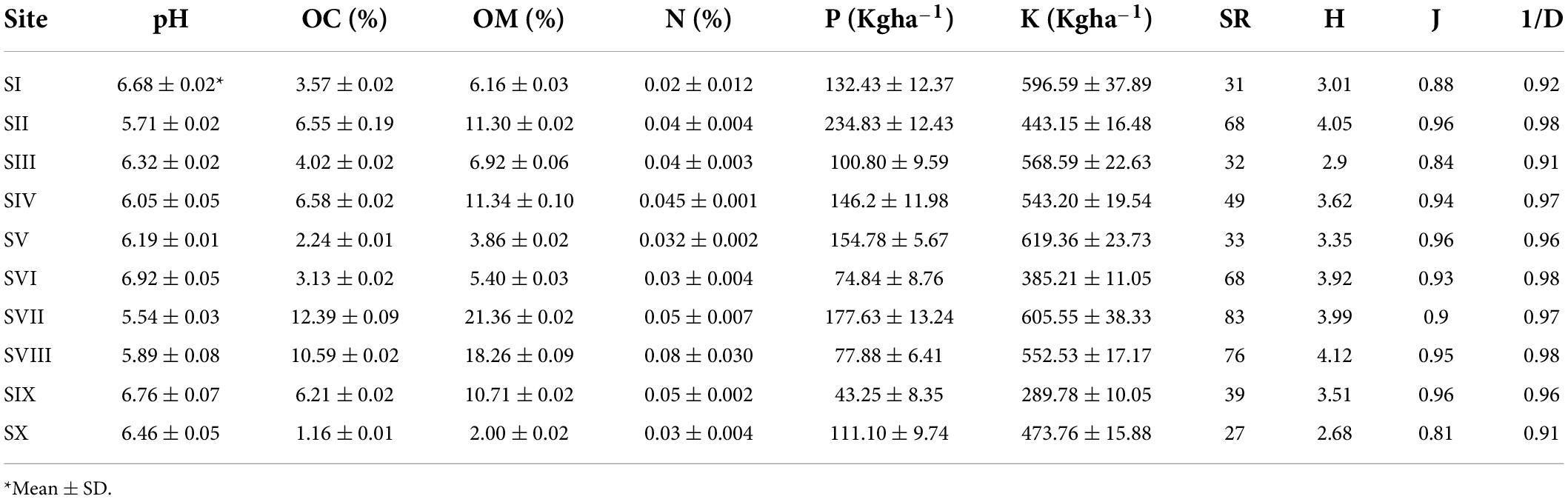
Table 2. Soil chemical properties and floral diversity parameters of selected study sites in Kashmir Himalaya (OC–organic carbon, OM–organic matter, N–Nitrogen, P–phosphorus, K–potassium; SR–species richness, H–Shanon-weiner index, J–Jaccard’s index of evenness, 1/D–Simpson’s index).
Site SII (Gulmarg)
The vegetation analysis of the P. acinosa communities at Site SII (Gulmarg) revealed rich species diversity. This site has high species richness of 68, with C. dactylon (IVI 17.18) being the dominant species followed by P. pratensis (IVI 15.40) while R. webbiana (IVI 1.24) was recorded as rare species. P. acinosa secured the lowest IVI of 4.82 at Gulmarg. Herbs represent 89.71%, shrubs and trees 4.41% each and climbers 1.47% of the total vegetation. The average pH (acidity) and OC of the soil was 5.71 and 6.55%, respectively. Besides, the mean value of N, P, and K were calculated as 0.04%, 234.83 kg ha–1 and 443.15 kg ha–1, respectively.
Site SIII (Drung)
The Site SIII has an altitude of 2200m asl and P. angustifolia having IVI 60.06 was the dominant species followed by C. dactylon (IVI 44.03) while R. canina and R. fruticosa each with IVI 2.43 were rare species. A total of 32 species were recorded. Among these 3.13% were trees, 9.38% shrubs, and 87.50% herbs. The value of the soil pH was 6.32 (Table 2) indicating slightly less acidic nature of soil. Further, the mean value of soil chemical properties including K, P, N, OM and OC was 568.59 kg ha–1, 100.8 kg ha–1, 0.04, 6.92, and 4.02%, respectively.
Site SIV (Gogaldara)
The plant community of sampling Site SIV is represented by 49 species. C. dactylon and P. angustifolia were the dominant species whereas E. canadensis was rare species based on calculated IVI. Vegetation was a blend of herbs (95.92%) shrubs (2.04%) and trees (2.04%). The mean soil pH and OC content was 6.05 and 6.58%, respectively. Moreover, the mean value of P and N in soil was recorded as 146.20 kg ha–1, and 0.045%, respectively.
Site SV (Doodhpathri)
At Doodhpathri, 33 species were found and T. repens was the dominant, followed by U. dioica. The lowest IVI of 2.47 was calculated for A. acuminata. The vegetation was composed of 32 herbs and a single shrub. The soil of Site V was less acidic having pH (6.19). The mean OC and OM content present in soil was 2.24% and 3.86%, respectively. Soil of this site also had maximum average value of 619.36 kg ha–1 for K (Table 2).
Site SVI (Dara)
Site SVI harbors unique and ample floristic diversity with species richness of 68 with herbs, shrubs and trees accounting for 82.35, 8.82, and 8.82%, respectively. U. dioica was the dominant species of this area followed by V. odorata. The calculated IVI of P. acinosa was 4.68. This site has lower content of OM (5.40%) and OC (3.13%) in soil (Table 2). Furthermore, the average P, K, and N content at this site was 74.84 kg ha–1, 385.21 kg ha–1, and 0.03%, respectively.
Site SVII (Daksum)
Site SVII (Daksum) has a high species diversity having Shanon-weiner index of 3.99 and registered the highest species richness (83). Based on calculated IVI, P. aviculare (19.45) and P. pratensis (16.70) were the dominant species of this area. A. pindrow, B. lycium, P. ragosus had lowest IVI. The area is dominated by herbs (90.36%) followed by trees (6.02%) and shrubs (3.61%). The soil was most acidic having pH of 5.84 and quite rich in chemical properties with highest average value of OM and OC content (12.39% and 21.36%), respectively (Table 2). The average value calculated for P, K and N were also high (177.63 kg ha–1 and 605.55 kg ha–1, and 0.05%, respectively).
Site SVIII (Pahalgam)
Phytosociological studies at site SVIII (Pahalgam) revealed the presence of second highest number of species (76) associated with the P. acinosa comprising of 96.05% herbs, 2.63% trees and 1.32% shrubs. T. repens (IVI 12.38) and I. heterantha and R. pseudoacacia (IVI 0.43 each) were the dominant and rare species, respectively. The soil of site VIII had second lowest average pH of 5.89 and highest nitrogen content of 0.08%. The soils also share a rich proportion of OC, OM and K content (10.59%, 18.26%, and 552.53 kg ha–1, respectively) (Table 2).
Site SIX (Bangus)
The Site SIX has a species richness of 39 mostly comprised of herbs (97.44%) and shrubs (2.56%). The highest IVI was recorded for C. dactylon and lowest IVI for S. chrysanthemoides, A. accuminata and T. farfara. The average calculated pH, OC and OM of the soil was 6.76, 6.21% and 10.71%, respectively. The site had the average N content of 0.05% and recorded the lowest value of P content (43.25 kg ha–1) (Table 2).
Site SX (Khag)
At Site SX the plant community was represented by lowest number of species having species richness of 27. As per IVI calculated, U. dioica was the dominant species followed by P. pratensis while as A. pindrow, R. pseudoacacia and P. wallichiana were rare species. The Vegetation found in this site was a blend of all the growth forms: herbs (59.23%), trees (25.93%) and shrubs (14.81%). The soil had nitrogen content of 0.74% and average calculated pH and OM was 6.76. The site has lowest average value of OM (2.00%) and OC (1.16%) (Table 2). Moreover, the value recorded for N, P and K was 0.03%, 111.10 kg ha–1 and 473.76 kg ha–1, respectively.
The present study revealed that plant community composition and species distribution pattern is considerably regulated by the amount and availability of soil nutrients. The correlation plot (Figure 3) indicated that species richness is positively correlated with OC, OM, N, P, Shannon diversity (H) and Simpson diversity (1/D) (r = 0.79, 0.79, 0.58, 0.24, 0.92, and 0.79, respectively), but the resultant correlation was significant only between species richness and OC, OM, Shannon diversity and Simpson index (p < 0.05). Similarly, species richness is negatively correlated with pH (r = −0.56), but the correlation was non-significant. In addition, positive correlation was found between Shannon diversity index and Simpson diversity index (r = 0.95; p < 0.05). A negative corelation was found between potassium and species diversity. SR increased significantly with increasing OC and OM content of soils.
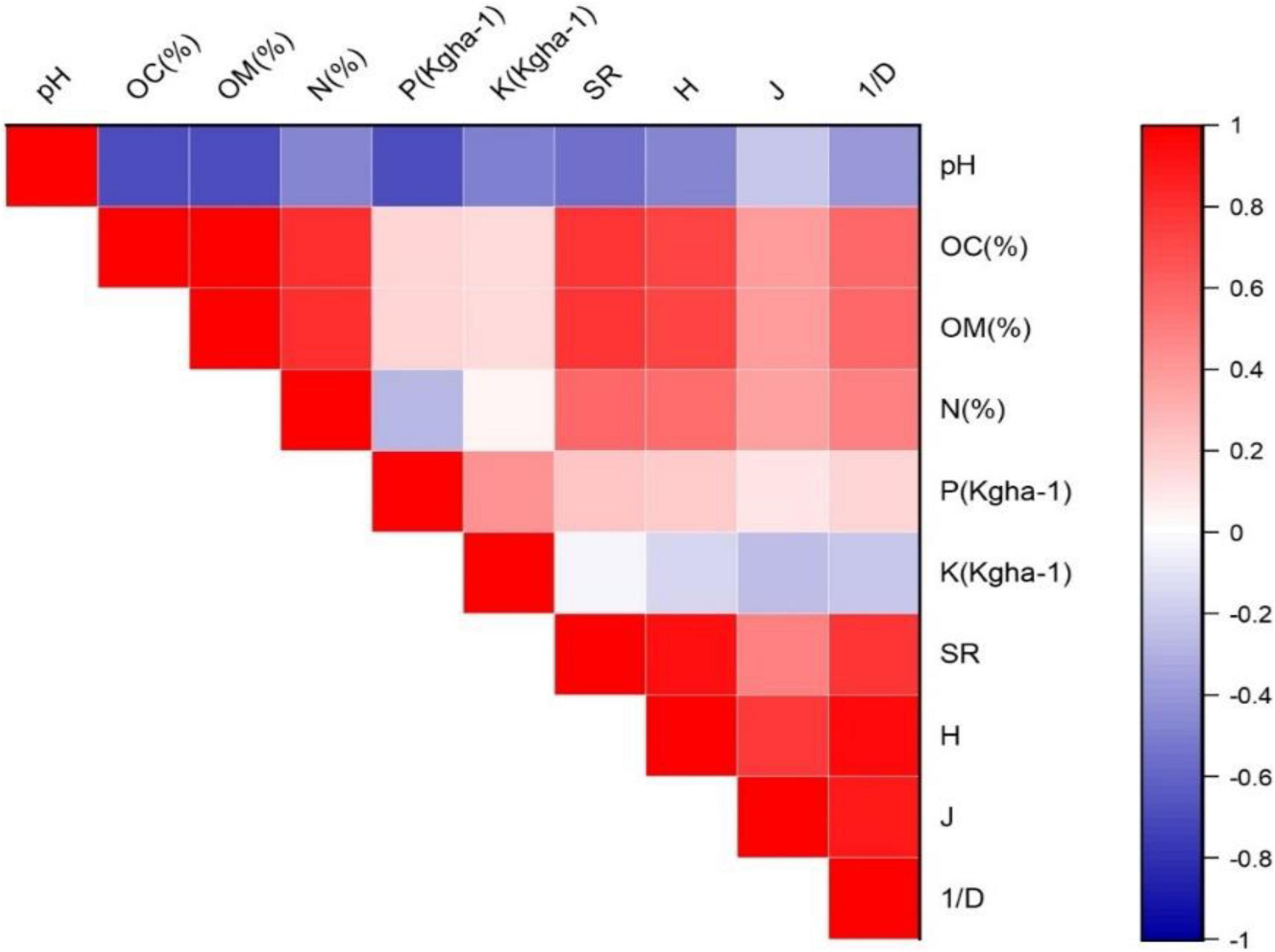
Figure 3. Correlation plot between vegetation and soil parameters. Statistical significance p < 0.05.
Community composition and association
On the basis of community composition, a total of 161 plant species were reported to be associated with P. acinosa. Out of the 12880 species pair combinations, 8006 pairs (62.16%) were eliminated from the analysis as expected co-occurrence was < 1 and 4874 pairs were analyzed. The findings show that the majority of the classifiable species combinations were truly random. The significant non-random associations were mostly positive (524 positive compared to 153 negative).
Cooccurrence Summary:
The plant of interest (Phytolacca acinosa) shows 100% random associations with other species i.e., observed frequency of co-occurrence is not significantly different and roughly equal to expected. Visualizations of all pairwise combinations of species and their co-occurrence signals (positive, negative, and random) (Figure 4). The plot removes any species with non-significant negative or positive correlations, and then ranks the remaining species from the most negative to the most positive relationships (left to right).
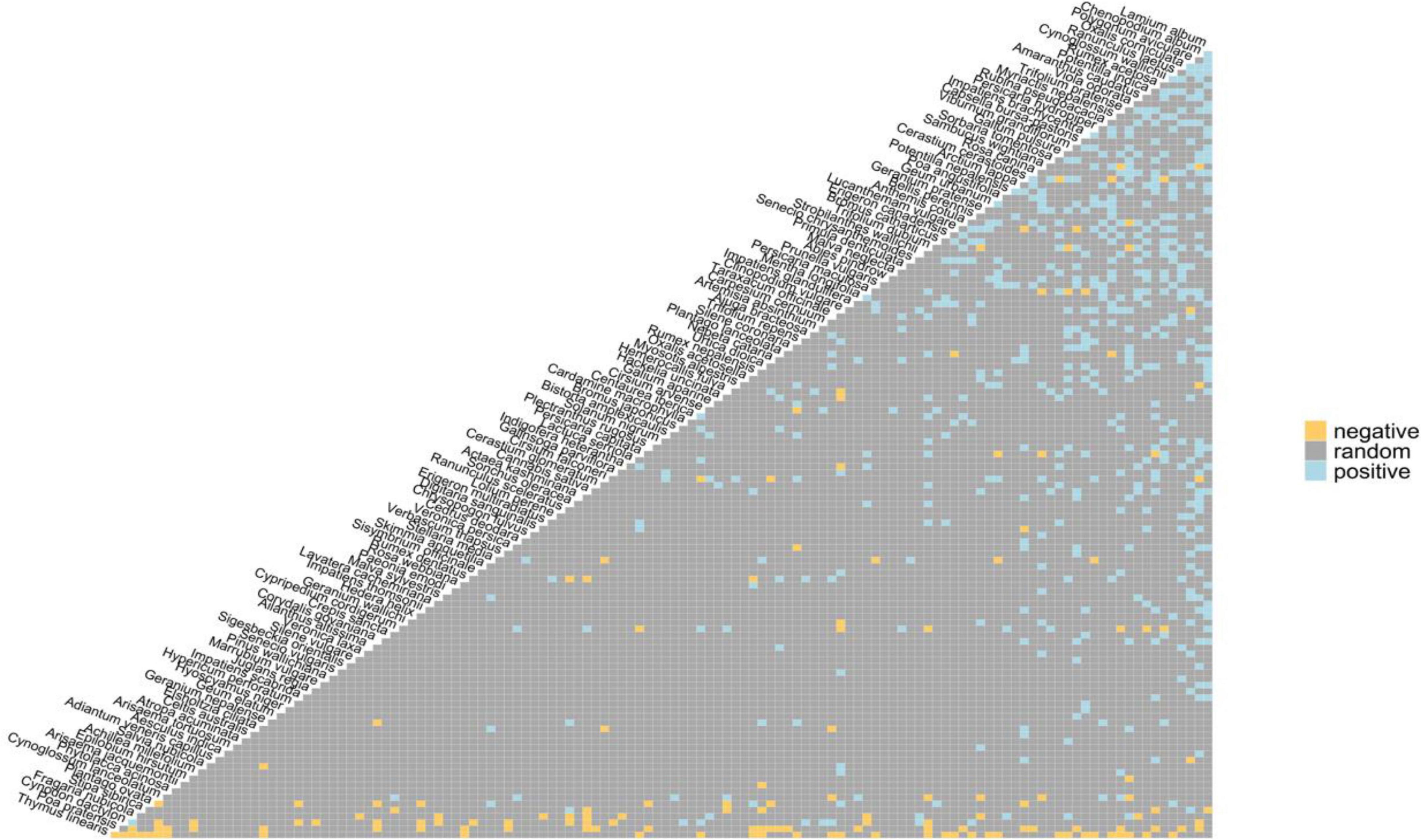
Figure 4. Heat map (co-occurrence plot) portraying the significant random negative and positive species relationships.
Cluster analysis
The cluster analysis revealed highest similarity of 71.39% among SVII (Daksum) at 2900 m asl and SVIII (Pahalgam) at 2800 m asl. On the other hand, site III (Drung) at 2200 m asl inside coniferous forest m asl and site X (Khag) 2000 m asl in disturbed roadside habitat showed the least similarity (37.01%) (Figure 5).
Discussion
During this study, total 161 plant species from 128 genera belonging to 49 plant families were documented from the selected sites of Kashmir Himalaya, India. The dominant families in the region were Asteraceae and Lamiaceae, followed by Rosaceae. The studies are in accordance with Altaf et al. (2021) and Nafeesa et al. (2021), who also recorded the dominance of these families in Kashmir Himalaya. The dominance of the Asteraceae and Lamiaceae families in the Himalayan region is due to their larger ecological amplitude, high adaptive capacity, larger seed output, and presence of different reproductive strategies in diverse environmental conditions (Haq et al., 2021; Sofi et al., 2022; Wani Z. A. et al., 2022).
The IVI gives an idea of socio-ecological interaction of species’ and dominance pattern in a population (Wani Z. A. et al., 2022). Across the study sites, analysis of IVI unveiled diverse species combinations with distinct dominants and co-dominants. The IVI of the constituent species exhibited considerable degree of variation ranging between 0.37 to 60.06. P. angustifolia, P. pratensis, C. dactylon, U. dioica, C. iberica, T. repens, G. nepalense, F. nubicola and P. aviculare have scored highest IVI (more dominant) reflecting their better adaptive mechanism to the conditions of the region (Ge et al., 2005; Shaheen et al., 2012). The majority of dominating species in the selected sites belonged to the Poaceae family. The higher seed vigor, germination, survivability and different means for vegetative reproduction assist these species for their stronger perpetuation (Ismail and ELawad, 2015). The studied species, P. acinosa has IVI ranging between 4.82 (Gulmarg)-11.33 (Khag) reflecting that this species had less perpetuation in the community of Gulmarg while better adaptation in Khag than the other associated species. Community characteristics of plants are highly impacted by the pressure exerted by overgrazing, trampling and varying environmental conditions. These differences in IVI across the sites may be attributed to surrounding environmental conditions and the anthropogenic activities which have already been reported from this area (Ganie et al., 2019). The IVI value of any species is an outcome of prevailing micro environmental conditions as well (Abinaya and Paulsamy, 2015).
The soil across sampling sites was acidic in nature with pH ranging between 5.54 to 6.92. Gairola et al. (2012), also suggested that for steady supply of nutrients the pH of forest soils should be slightly acidic. It has also been reported that the acidic nature of forest soils may be due to the basic cation uptake of by tree roots (Fujii et al., 2018). The pH of soil differed significantly across the study sites (F = 1.976, p = 0.051). This change in pH may be attributed to altitudinal differences among sites (Wani Z. A. et al., 2022). There is significant negative correlation (r = −0.7, p < 0.05) between available P content and pH at the study sites (Figure 3). This is because crystalline and amorphous forms of Al and Fe couple with inorganic P compounds depending on pH of soil (Hedley et al., 1982). Organic matter (OM) is a major constituent of the forest floor supporting microbiological diversity and guarantees a long-term supply of nutrients through decomposition. OM provides a significant portion of soil carbon (C) and regulates soil characteristics (Mishra et al., 2018). Significant variation in both OC (F = 8244.371, p < 0.05) and OM (F = 45985.584, p < 0.05) was found across the study sites, with highest values at SVII (Daksum). This is consistent with the results depicted by Powers and Schlesinger (2002) who reported that soil carbon content varies across different habitats and elevation. According to Agren et al. (2013), litter decomposition is region specific and has a strong correlation with OM mineralization. In addition, other factors such as, species richness (SR) also contributes to the accumulation of OM. Available nitrogen (N) exhibited a significantly positive correlation with OC and OM content (r = 0.8, p < 0.05) (Figure 3). It has been reported that humus content is directly proportional to the amount of essential nutrients in soil. OC and phosphorous (P) content were negatively correlated (Gupta and Sharma, 2009). The nitrogen (N) content is dependent on the amount of OM in the soil (Jha et al., 1984). No significant variation was observed in the N content (%) (F = 1.828, p > 0.05) between the study sites (Table 2). N showed a positive correlation with potassium (K) (Figure 3). Previous studies carried out by Gupta and Sharma, (Gupta and Sharma, 2009); Mishra et al. (2017) also reported a positive correlation between N and K, which in turn are directly related to OM.
The P content varied significantly (F = 3.556, p < 0.05) ranging from 43.25 kg/ha−1 (SIX) to 77.88 kg/ha−1 (SVIII) across the study sites. Highest K content was recorded at SV (619.36 kg ha–1) while SVIII had the lowest content (77.88 kg ha–1). The potassium content in soil is proportional to the parent rock compositions. As the degree of weathering is regulated by altitude and accompanying climatic conditions, variations in K content may be related to habitat features and altitude (Mishra et al., 2017). Further, OC shows a positive correlation with K (r = 0.15) and N (r = 0.8), but this correlation was significant between OC and N (p < 0.05) (Figure 3). Owing to the fact that availability of K is maintained through decomposition of organic matter (Basumatary and Bordoloi, 1992), therefore a positive correlation is present between organic carbon and macronutrients (nitrogen and potassium) as both are dependent on the humus content in soil (Mishra et al., 2017).
A sturdy relationship was found between floristic diversity associated with P. acinosa and soil parameters across different sites in Kashmir Himalaya. Our results corroborate previous investigations of edaphic factors (Figueiredo et al., 2018; Jucker et al., 2018; Hofhansl et al., 2020), which found that vegetation attributes changed in relation to the regional variation of soil physico-chemical properties across different areas. Plant species composition and distribution is influenced by the soil characteristics (Yang et al., 2018). Across different sites soil properties such as, Ph, OC, OM among others show significant differences, that eventually impact the plant species composition (Rahman et al., 2022). The community composition and species distribution pattern is also regulated by the soil organic matter. Water holding capacity and colloidal nature of soil subsequently increased in plant communities with increase in OM content (Mumshad et al., 2021; Wani Z. A. et al., 2022). Higher values of phytosociological parameters across the study sites can be attributed to the favorable soil chemical properties.
The highest degree of similarity (71.39%) was recorded between SVII and SVIII (Figure 5), as both sites had almost similar soil characteristics and relatively similar altitudes. SI (fertile forest soil) and SIII (rocky soil) exhibited least similarity (37.01%) which can be ascribed to the variation in habitat characteristics, altitude and soil properties between the two sites, the vegetation composition changed in relation to the regional variation of soil properties and elevation across different areas (Ismail and ELawad, 2015). The presence of entirely random association with other plants (Figure 4) revealed that P. acinosa inhabit distinct habitats and co-associates in a natural community within a specific geographical area (Gulzar et al., 2022).
Our study has revealed that the change in soil properties leads to subsequent changes in plant communities, especially in terms of species diversity, richness and cooccurrence patterns. Since the plant species respond individually to changing climatic and soil conditions, plant community composition is thus likely to be modified (Lafleur et al., 2010). Environmental factors like soil moisture, temperature and elevation governs the vegetation patterns and soil physico-chemical properties to a greater extent (Solon et al., 2007; Hamid et al., 2021). This interpretation is supported by differentiation in community composition and structural features such as number of species, co-occurrence patterns, and diversity indices. The species richness varied in relation to the combined pattern of edaphic factors like OC, OM, N, and pH, with the number of species being substantially higher where these soil properties except pH were high, and vice-versa (Solon et al., 2007). The present study also reflected that SVII (Daksum) has highest species richness (83), OC (12.39%) and OM (21.36%) content while SX (Khag) had lowest species richness (27), OC (1.16%) and OM (2.00%). Floristic composition and soil fertility of a particular site is largely affected by the anthropogenic activities. The sites with undisturbed and fertile soils have quite rich species diversity and the sites with poor and disturbed soils support minimum plant diversity. The sites characterized by fertile soils, higher floristic diversity and productivity act as potential areas of carbon sequestration (Mishra et al., 2017). Therefore, proper management of such areas will not only help in mitigating the climate change, but also allow the sustainable provisioning of ecosystem services and conservation of floristic diversity. Besides, the sites with the low soil fertility and species diversity need better management strategies for the restoration and plant species conservation.
Since community composition and distribution of plant species is partly under the control of climatic variables like average precipitation and temperature, the projected global climate change is likely to alter the composition and distribution pattern of vegetation (Chen et al., 2011; Brown and Vellend, 2014). Moreover, as soil processes and properties are partially governed by climatic variables, the climate change is also likely to modify soil properties (Gelybo et al., 2018). Floristic composition is influenced by soil physicochemical properties, and any modification in soil factors due to the changing climate leads to the variation in vegetation responses (Lafleur et al., 2010). Hence, relationship between climate, vegetation and soil properties are complex, multi-scale, and multi-aspect in nature. The study of plant-soil systems has become important in understanding plant community composition, population dynamics, and ecosystems functioning. Soil microclimate and other environmental factors that contribute to plant–soil system effect ecosystem composition, species diversity and possibly play a role in the evolution of species (van der Putten et al., 2016). Therefore, a better understanding of ecological and evolutionary repercussions of soil-vegetation interactions might assist in projecting and mitigating the effects of human-induced global climate change, and land use change for the better sustainable future.
Conclusion
The present study indicates that Phytolacca acinosa displays a broad range of habitats reflecting its diverse phytosociological amplitude and tolerance to existing environmental conditions. The phytosociological characteristics reveals that in P. acinosa communities; Poa angustifolia, P. pratensis, and Cynadon dactylon were the dominant species. The findings of present study emphasis on the significance of relationship between soil parameters, vegetation characteristics and plant associations for better understanding of communities’ functioning. The lower IVI values of P. acinosa than associated plant species divulge its lesser ecological importance and perpetuation in the assemblages, hence needs conservation strategies. Moreover, the soil paraments plays a vital role in the establishment of plant communities with response to environmental conditions. The good soil properties and phytosociological attributes at some sites reflects the relatively stable nature of these sites and vice versa. All these parameters must be taken into consideration while studying species distribution pattern, community characteristics and association in a particular region. From our study it can be concluded that the knowledge of both species diversity and soil properties in different ecosystems can improve conservation efforts and ensure better management strategies for the restoration of degraded habitats over a period of time. In future, similar studies will help in understanding the community ecology their conservation strategies and restoration efforts, to ensure prolonged supply of ecosystem functioning and processes.
Data availability statement
The original contributions presented in this study are included in the article/Supplementary material, further inquiries can be directed to the corresponding author.
Author contributions
JM: methodology, data collection, and writing - original draft. BW: data collection. TI: curation and analysis, software, and visualization. AG: conceptualization and supervision, methodology, validation, and writing - original draft. IN: conceptualization, supervision, investigation, validation, and writing - review. All authors contributed to the article and approved the submitted version.
Funding
JM and BW acknowledged the Maulana Azad National Fellowship (MANF) for funding under MANF-201920-JKO416200268 and MANF-2018-19-JAM-100111, respectively and also TI acknowledged the University Grants Commission (UGC) under 924/(CSIRNET JUNE-2019) for providing financial assistance as Junior Research Fellowship.
Acknowledgments
The authors are thankful to the Head, Department of Botany, University of Kashmir. Colleagues at PRBGPR Laboratory, University of Kashmir are acknowledged for their kind support during the present study. We also thank to the Sajad A. Wani for giving valuable suggestion regarding the manuscript.
Conflict of interest
The authors declare that the research was conducted in the absence of any commercial or financial relationships that could be construed as a potential conflict of interest.
Publisher’s note
All claims expressed in this article are solely those of the authors and do not necessarily represent those of their affiliated organizations, or those of the publisher, the editors and the reviewers. Any product that may be evaluated in this article, or claim that may be made by its manufacturer, is not guaranteed or endorsed by the publisher.
Supplementary material
The Supplementary Material for this article can be found online at: https://www.frontiersin.org/articles/10.3389/ffgc.2022.976902/full#supplementary-material
References
Abekura, F., Park, J., Kwak, C. H., Ha, S. H., Cho, S. H., Chang, Y. C., et al. (2019). Esculentoside B inhibits inflammatory response through JNK & downstream NF-κB signaling pathway in LPS-triggered murine macrophage RAW 264.7 cells. Int. Immunopharmacol. 68, 156–163. doi: 10.1016/j.intimp.2019.01.003
Abinaya, G., and Paulsamy, S. (2015). Phytosociological analysis of the medicinal plant species, Thalictrum javanicum Blume in thottabetta, nilgiris, the western ghats. Kongunadu Res. J. 2, 56–62. doi: 10.26524/krj68
Agren, G. I., Hyvönen, R., Berglund, S. L., and Hobbie, S. E. (2013). Estimating the critical N: c from litter decomposition data and its relation to soil organic matter stoichiometry. Soil Biol. Biochem. 67, 312–318. doi: 10.1016/j.soilbio.2013.09.010
Ahmed, M. J., Murtaza, G., Shaheen, H., and Habib, T. (2020). Distribution pattern & associated flora of jurinea dolomiaea in the western Himalayan highlands of Kashmir: an indicator endemic plant of alpine phytodiversity. Ecol. Indic. 116:106461. doi: 10.1016/j.ecolind.2020.106461
Altaf, A., Haq, S. M., Shabnum, N., and Jan, H. A. (2021). Comparative assessment of PHYTO diversity in tangmarg forest division in Kashmir Himalaya, India. Acta. Ecol. Sin. *VP, doi: 10.1016/j.chnaes.2021.04.009
Aryal, K. P., Poudel, S., Chaudhary, R. P., Chettri, N., Chaudhary, P., Ning, W., et al. (2018). Diversity and use of wild and non-cultivated edible plants in the Western Himalaya. J. Ethnobiol. Ethnomed. 14:10. doi: 10.1186/s13002-018-0211-1
Basnet, A., and Kalauni, S. K. (2020). Phytochemical screening and biological activity analysis of some selected medicinal plants of Ilam district of Nepal. Curr. Perspect. Med. Aromat. Plants 3, 61–73. doi: 10.38093/cupmap.765409
Basumatary, A., and Bordoloi, P. K. (1992). Forms of potassium in some soils of Assam in relation to soil properties. J. Indian Soc. Soil Sci. 40, 443–446.
Brown, C. D., and Vellend, M. (2014). Non-climatic constraints on upper elevational plant range expansion under climate change. Proc. Biol. Sci. 281:20141779. doi: 10.1098/rspb.2014.1779
Chao, A., Chazdon, R. L., Colwell, R. K., and Shen, T. J. (2005). A new statistical approach for assessing similarity of species composition with incidence & abundance data. Ecol. Lett. 8, 148–159. doi: 10.1111/j.1461-0248.2004.00707.x
Chawla, A., Yadav, P. K., Uniyal, S. K., Kumar, A., Vats, S. K., Kumar, S., et al. (2012). Long-term ecological and biodiversity monitoring in the western Himalaya using satellite remote sensing. Curr. Sci. 102, 1143–1156.
Chen, I. C., Hill, J. K., Ohlemüller, R., Roy, D. B., and Thomas, C. D. (2011). Rapid range shifts of species associated with high levels of climate warming. Science 333, 1024–1026. doi: 10.1126/science.1206432
Chin, E. Y., and Kupfer, J. A. (2020). Identification of environmental drivers in urban greenway communities. Urban For. Urban Green. 47:126549. doi: 10.1016/j.ufug.2019.126549
Collins, S. L., Chung, Y. A., Baur, L. E., Hallmark, A., Ohlert, T. J., and Rudgers, J. A. (2020). Press–pulse interactions and long-term community dynamics in a Chihuahuan Desert grassland. J. Veg. Sci. 31, 722–732. doi: 10.1111/jvs.12881
Curtis, J. T., and McIntosh, R. P. (1950). The interrelations of certain analytic and synthetic phytosociological characters. Ecology 31, 434–455. doi: 10.2307/1931497
Davies, B. E. (1974). Loss-on-ignition as an estimate of soil organic matter. Soil Sci. Soc. Am. J. 38, 150–151. doi: 10.2136/sssaj1974.03615995003800010046x
Dengler, J. (2016). “Phytosociology,” in International Encyclopedia of Geography: People, the Earth, Environment & Technology: People, the Earth, Environment & Technology, eds N. Castree, M. F. Goodchild, A. Kobayashi, W. Liu, and R. A. Marston (Hoboken, NY: John Wiley & Sons), 1–6. *. doi: 10.1002/9781118786352.wbieg0136
Figueiredo, F. O., Zuquim, G., Tuomisto, H., Moulatlet, G. M., Balslev, H., and Costa, F. R. (2018). Beyond climate control on species range: the importance of soil data to predict distribution of Amazonian plant species. J. Biogeogr. 45, 190–200. doi: 10.1111/jbi.13104
Fujii, K., Shibata, M., Kitajima, K., Ichie, T., Kitayama, K., and Turner, B. L. (2018). Plant–soil interactions maintain biodiversity and functions of tropical forest ecosystems. Ecol. Res. 33, 149–160. doi: 10.1007/s11284-017-1511-y
Gairola, S., Sharma, C. M., Ghildiyal, S. K., and Suyal, S. (2012). Chemical properties of soils in relation to forest composition in moist temperate valley slopes of Garhwal Himalaya, India. Environmentalist 32, 512–523. doi: 10.1007/s10669-012-9420-7
Ganie, A. H., Tali, B. A., Khuroo, A. A., Reshi, Z. A., and Nawchoo, I. A. (2019). Impact assessment of anthropogenic threats to high-valued medicinal plants of Kashmir Himalaya, India. J. Nat. Conserv. 50:125715. doi: 10.1016/j.jnc.2019.125715
Ge, X. J., Zhang, L. B., Yuan, Y. M., Hao, G., and Chiang, T. Y. (2005). Strong genetic differentiation of the East-Himalayan Megacodon stylophorus (Gentianaceae) detected by inter-simple sequence repeats (ISSR). Biodivers. Conserv. 14, 849–861. doi: 10.1007/s10531-004-0655-6
Gelybo, G., Tóth, E., Farkas, C., Horel, A., Kasa, I., and Bakacsi, Z. (2018). Potential impacts of climate change on soil properties. Agrokémia és Talajtan 67, 121–141. doi: 10.1556/0088.2018.67.1.9
Griffith, D. M., Veech, J. A., and Marsh, C. J. (2016). Cooccur: probabilistic species co-occurrence analysis in R. J. Stat. Softw. 69, 1–17. doi: 10.18637/jss.v069.c02
Gulzar, R., Banday, F. A., Rather, Z. A., Rashid, I., and Khuroo, A. A. (2022). Naturalisation of ranunculus repens in Kashmir Himalaya: floristic and ecological aspects. Plant Biosyst. 1–7. doi: 10.1080/11263504.2022.2036847
Gupta, M. K., and Sharma, S. D. (2009). Effect of tree plantation on soil properties, profile morphology & productivity index-II. Poplar in Yamunanagar district of Haryana. Ann. For. 17, 43–70.
Hamid, M., Khuroo, A. A., Malik, A. H., Ahmad, R., and Singh, C. P. (2021). Elevation & aspect determine the differences in soil properties and plant species diversity on Himalayan mountain summits. Ecol. Res. 36, 340–352. doi: 10.1111/1440-1703.12202
Haq, S. M., Calixto, E. S., Rashid, I., and Khuroo, A. A. (2021). Human-driven disturbances change the vegetation characteristics of temperate forest stands: a case study from Pir Panchal mountain range in Kashmir Himalaya. Trees For. People 6:100134. doi: 10.1016/j.tfp.2021.100134
He, Y. W., Pan, Y. F., Wang, P., and Guo, J. S. (2004). The effects of Poke weed antiviral protein on replication of HCV. Chin. Hepatol. 7, 233–236.
Hedley, M. J., Stewart, J. W. B., and Chauhan, B. (1982). Changes in inorganic and organic soil phosphorus fractions induced by cultivation practices and by laboratory incubations. Soil Sci. Soc. Am. J. 46, 970–976. doi: 10.2136/sssaj1982.03615995004600050017x
Hofhansl, F., Chacon-Madrigal, E., Fuchslueger, L., Jenking, D., Morera-Beita, A., Plutzar, C., et al. (2020). Climatic and edaphic controls over tropical forest diversity and vegetation carbon storage. Sci. Rep. 10, 1–11. doi: 10.1038/s41598-020-61868-5
Iqbal, M., Khan, S. M., Ahmad, Z., Hussain, M., Nasar, S., Shah, S. K., et al. (2021). Vegetation classification of the margalla foothills, islamabad under the influence of edaphic factors & anthropogenic activities using modern ecological tools. Pakistan J. Bot. 53, 10–30848. doi: 10.30848/PJB2021-5(22)
Ismail, I. M., and ELawad, A. A. (2015). Phytosociological analysis and species diversity of herbaceous layer in rashad and Alabassia Localities, South Kordofan State, Sudan. Jordan J. Biol. Sci. 147, 1–7. doi: 10.12816/0027562
Jha, M. N., Rathore, R. K., and P&e, P. (1984). Soil factor affecting the natural regeneration of silver fir & spruce in Himachal Pradesh. Indian For. 110, 293–298.
Jiao, S., Li, J., Li, Y., Jia, J., and Xu, Z. (2019). Soil C, N, & P distribution as affected by plant communities in the yellow river delta, China. PLoS One 14:e0226887. doi: 10.1371/journal.pone.0226887
Jucker, T., Bongalov, B., Burslem, D. F., Nilus, R., Dalponte, M., Lewis, S. L., et al. (2018). Topography shapes the structure, composition and function of tropical forest landscapes. Ecol. Lett. 21, 989–1000. doi: 10.1111/ele.12964
Kamran, S., Khan, S. M., Ahmad, Z., Ur Rahman, A., Iqbal, M., Manan, F., et al. (2020). The role of graveyards in species conservation and beta diversity: a vegetation appraisal of sacred habitats from Bannu, Pakistan. J. For. Res. 31, 1147–1158. doi: 10.1007/s11676-019-00893-1
Khan, M., Khan, S. M., Ilyas, M., Alqarawi, A. A., Ahmad, Z., and Abd_Allah, E. F. (2017). Plant species and communities assessment in interaction with edaphic & topographic factors; an ecological study of the mount Eelum district Swat, Pakistan. Saudi J. Biol. Sci. 24, 778–786. doi: 10.1016/j.sjbs.2016.11.018
Khan, S. M., Page, S., Ahmad, H., Shaheen, H., and Harper, D. M. (2012). Vegetation dynamics in the Western Himalayas, diversity indices and climate change. Sci. Technol. Dev. J. 31, 232–243.
Khan, W., Ahmad, H., Haq, F., Islam, M., and Bibi, F. (2012). Present status of moist temperate vegetation of Thandiani forests district Abbottabad Pakistan. Int. J. Biosci. 10, 80–88.
Lafleur, B., Pare, D., Munson, A. D., and Bergeron, Y. (2010). Response of northeastern North American forests to climate change: will soil conditions constrain tree species migration? Environ. Rev. 18, 279–289. doi: 10.1139/A10-013
Lin, D. U., Wang, J. X., Wang, F., Zhou, H. Y., and Wei, D. U. (2018). Triterpenoid saponins from roots of Phytolacca acinosa. China J. Chin. Materia Med. 43, 2552–2556.
Ma, X. P., Lou, H. Y., Zhang, W. F., Song, J. R., Li, Y., and Pan, W. D. (2019). Triterpenoids from Phytolacca acinosa. Chem. Nat. Compd. 55, 292–295. doi: 10.1007/s10600-019-02670-2
Ma, X. P., Zhang, W. F., Yi, P., Lan, J. J., Xia, B., Jiang, S., et al. (2017). Novel flavones from the root of Phytolacca acinosa Roxb. Chem. Biodivers. 14:e1700361. doi: 10.1002/cbdv.201700361
Malfasi, F., and Cannone, N. (2020). Climate warming persistence triggered tree ingression after shrub encroachment in a high alpine tundra. Ecosystems 23, 1657–1675. doi: 10.1007/s10021-020-00495-7
Manoj, K., Nandakumar, M. K., Remya, M. P., Shinila, K., and Lakshmi, P. D. (2012). Phytosociological analysis of riparian tree species of Alakyam stream; Pariyaram, Kerala, India. Int. J. Environ. Sci. 2, 1895–1903.
Manzoor, N., Wani, I., Singh, K. P., and Pal, A. (2017). In-vitro antimicrobial activity phytochemical screening of Phytolacca acinosa in Kashmir Valley. Int. J. Sci. Res. 6, 102–106.
Mishra, G., Das, P. K., Borah, R., and Dutta, A. (2017). Investigation of phytosociological parameters & physico-chemical properties of soil in tropical semi-evergreen forests of Eastern Himalaya. J. For. Res. 28, 513–520. doi: 10.1007/s11676-016-0330-2
Mishra, G., Giri, K., and Pandey, S. (2018). Role of Alnus nepalensis in restoring soil fertility: a case study in Mokokchung, Nagaland. Nat. Acad. Sci. Lett. 41, 265–268. doi: 10.1007/s40009-018-0668-4
Mumshad, M., Ahmad, I., Khan, S. M., Rehman, K., Islam, M., Sakhi, S., et al. (2021). Phyto-ecological studies and distribution pattern of plant species and communities of Dhirkot, Azad Jammu and Kashmir, Pakistan. PLoS One 16:e0257493. doi: 10.1371/journal.pone.0257493
Nafeesa, Z., Haq, S. M., Bashir, F., Gaus, G., Mazher, M., Anjum, M., et al. (2021). Observations on the floristic, life-form, leaf-size spectra and habitat diversity of vegetation in the Bhimber hills of Kashmir Himalayas. Acta Ecol. Sin. 41, 228–234. doi: 10.1016/j.chnaes.2021.03.003
Noreen, I., Khan, S. M., Ahmad, Z., Rahman, I. U., Tabassum, A. B., and Abd_Allah, E. F. (2019). Response of different plant species to pollution emitted from oil and gas plant with special reference to heavy metals accumulation. Pakistan J. Bot. 51, 1231–1240. doi: 10.30848/PJB2019-4(39)
Ocon, J. P., Ibanez, T., Franklin, J., Pau, S., Keppel, G., Rivas-Torres, G., et al. (2021). Global tropical dry forest extent and cover: a comparative study of bioclimatic definitions using two climatic data sets. PLoS One 16:e0252063. doi: 10.1371/journal.pone.0252063
Olsen, S. R., Watanabe, F. S., Cosper, H. R., Larson, W. E., and Nelson, L. B. (1954). Residual phosphorus availability in long-time rotations on calcareous soils. Soil Sci. 78, 141–152. doi: 10.1097/00010694-195408000-00008
Paudel, P. K., Sipos, J., and Brodie, J. F. (2018). Threatened species richness along a Himalayan elevational gradient: quantifying the influences of human population density, range size, and geometric constraints. BMC Ecology 18:6. doi: 10.1186/s12898-018-0162-3
Phillips, E. A. (1959). Methods of vegetation study. New York, NY: Holt, Rhinehart and Winston, 107.
Powers, J. S., and Schlesinger, W. H. (2002). Relationships among soil carbon distributions and biophysical factors at nested spatial scales in rain forests of northeastern Costa Rica. Geoderma 109, 165–190. doi: 10.1016/S0016-7061(02)00147-7
POWO. (2022). Plants of the World Online. Available from: http://www.plantsoftheworldonline.org/ (accessed 13 March 2022)
R Core Team (2020). R: A Language and Environment for Statistical Computing. Vienna: R Foundation for Statistical Computing.
Rahman, I. U., Hart, R. E., Ijaz, F., Afzal, A., Iqbal, Z., Calixto, E. S., et al. (2022). Environmental variables drive plant species composition & distribution in the moist temperate forests of Northwestern Himalaya, Pakistan. PloS One 17:e0260687. doi: 10.1371/journal.pone.0260687
Rana, S. K., Rana, H. K., Luo, D., and Sun, H. (2021). Estimating climate-induced ‘Nowhere to go’range shifts of the Himalayan Incarvillea Juss. using multi-model median ensemble species distribution models. Ecol. Indic. 121:107127. doi: 10.1016/j.ecolind.2020.107127
Rawal, R. S., Rawal, R., Rawat, B., Negi, V. S., and Pathak, R. (2018). Plant species diversity & rarity patterns along altitude range covering treeline ecotone in Uttarakhand: conservation implications. Trop. Ecol. 59, 225–239.
Ray, J. G., and George, J. (2009). Phytosociology of roadside communities to identify ecological potentials of tolerant species. J. Ecol. Nat. Environ. 1, 184–190.
Romshoo, S. A., Bashir, J., and Rashid, I. (2020). Twenty-first century-end climate scenario of Jammu & Kashmir Himalaya. India, using ensemble climate models. Clim. Change 162, 1473–1491. doi: 10.1007/s10584-020-02787-2
Shaheen, H., Shinwari, Z. K., Qureshi, R. A., and Ullah, Z. (2012). Indigenous plant resources & their utilization practices in village populations of Kashmir himalayas. Pakistan J. Bot. 44, 739–745.
Shannon, C. E., and Weaver, W. W. (1963). The Mathematical Theory of Communication. Urbana: University of Illinois Press, 117.
Sofi, I. I., Zargar, S. A., Ganie, A. H., and Shah, M. A. (2022). Distribution dynamics of Arnebia Euchroma (Royle) IM Johnst. and associated plant communities in Trans-Himalayan Ladakh region in relation to local livelihoods under climate change. Trees For. People 7:100213. doi: 10.1016/j.tfp.2022.100213
Solon, J., Degorski, M., and Roo-Zielinska, E. (2007). Vegetation response to a topographical-soil gradient. Catena 71, 309–320. doi: 10.1016/j.catena.2007.01.006
Subbiah, B. V., and Asija, C. L. (1956). A rapid procedure for the estimation of available nitrogen in soils. Curr. Sci. 25, 256–260.
Tali, B. A., Ganie, A. H., Nawchoo, I. A., Wani, A. A., and Reshi, Z. A. (2015). Assessment of threat status of selected endemic medicinal plants using IUCN regional guidelines: a case study from Kashmir Himalaya. J. Nat. Conserv. 23, 80–89. doi: 10.1016/j.jnc.2014.06.004
Tali, B. A., Khuroo, A. A., Ganie, A. H., and Nawchoo, I. A. (2019). Diversity, distribution & traditional uses of medicinal plants in Jammu & Kashmir (J&K) state of Indian Himalayas. J. Herb. Med. 17:100280. doi: 10.1016/j.hermed.2019.100280
Tao, Y., Jiang, E., and Cai, B. (2020). Development of an ultra-high-performance liquid chromatography coupled with triple quadrupole mass spectrometry method for comparative pharmacokinetics of six triterpenoids in rat plasma and application to different forms of Phytolacca acinosa. J. Sep. Sci. 43, 1248–1255. doi: 10.1002/jssc.201901140
van der Putten, W. H., Bradford, M. A., Pernilla Brinkman, E., van de Voorde, T. F., and Veen, G. F. (2016). Where, when and how plant–soil feedback matters in a changing world. Funct. Ecol. 30, 1109–1121. doi: 10.1111/1365-2435.12657
Walentowski, H., Aas, G., Göllner, A., Ahl, L., and Feulner, M. (2018). Phytosociological studies of Malus sylvestris in North Hesse and upper Franconia. Germany. Tuexenia 38, 97–110.
Walkley, A., and Black, I. A. (1934). An examination of the degtjareff method for determining soil organic matter, and a proposed modification of the chromic acid titration method. Soil Sci. 37, 29–38. doi: 10.1097/00010694-193401000-00003
Wani, I. A., Verma, S., Gupta, R., Ganaie, M. M., Nigam, G., Shafik, H. M., (2022). Nutrient analysis and species diversity of alpine grasslands: a comparative analysis of less studied biodiversity hotspots. Sustainability 14:887. doi: 10.3390/su14020887
Wani, Z. A., Islam, T., Satish, K. V., Ahmad, K., Dhyani, S., and Pant, S. (2022). Cultural value and vegetation structure of Buxus wallichiana Bail. in Rajouri-Poonch region of Indian Himalayan region (VSI: Mountainous regions). Trees, For. People 7:100182. doi: 10.1016/j.tfp.2021.100182
Yadav, R. R., Negi, P. S., and Singh, J. (2021). Climate change and plant biodiversity in Himalaya. India. Proc. Indian Natl Sci. Acad. 87, 234–259. doi: 10.1007/s43538-021-00034-5
Yang, Y. S., Zhang, L., Li, H. Q., He, H. D., Wei, Y. X., Luo, J., et al. (2018). Soil physicochemical properties and vegetation structure along an elevation gradient and implications for the response of alpine plant development to climate change on the northern slopes of the Qilian Mountains. J. Mt. Sci. 15, 1006–1019. doi: 10.1007/s11629-017-4637-z
Zaz, S. N., Romshoo, S. A., Krishnamoorthy, R. T., and Viswanadhapalli, Y. (2019). Analyses of temperature and precipitation in the Indian Jammu and Kashmir region for the 1980–2016 period: implications for remote influence and extreme events. Atmos. Chem. Phys. 19, 15–37. doi: 10.5194/acp-19-15-2019
Keywords: climate change, community composition, Kashmir Himalaya, soil chemistry, species diversity
Citation: Magray JA, Wani BA, Islam T, Ganie AH and Nawchoo IA (2022) Phyto-ecological analysis of Phytolacca acinosa Roxb. assemblages in Kashmir Himalaya, India. Front. For. Glob. Change 5:976902. doi: 10.3389/ffgc.2022.976902
Received: 23 June 2022; Accepted: 13 July 2022;
Published: 04 August 2022.
Edited by:
Zishan Ahmad Wani, Baba Ghulam Shah Badshah University, IndiaReviewed by:
Ishfaq Ahmad Wani, Baba Ghulam Shah Badshah University, IndiaZeeshan Ahmad, Quaid-i-Azam University, Pakistan
Copyright © 2022 Magray, Wani, Islam, Ganie and Nawchoo. This is an open-access article distributed under the terms of the Creative Commons Attribution License (CC BY). The use, distribution or reproduction in other forums is permitted, provided the original author(s) and the copyright owner(s) are credited and that the original publication in this journal is cited, in accordance with accepted academic practice. No use, distribution or reproduction is permitted which does not comply with these terms.
*Correspondence: Junaid A. Magray, anVuYWlkbWFncmF5Nzg2QGdtYWlsLmNvbQ==