- 1Department of Forestry and Environmental Resources, North Carolina State University, Research Triangle Park, NC, United States
- 2Eastern Forest Environmental Threat Assessment Center, Southern Research Station, United States Department of Agriculture Forest Service, Research Triangle Park, NC, United States
Non-native (introduced, exotic, and alien) species alter forest ecosystem processes, cause landscape change, interfere with services provided by native trees, and contribute to biotic homogenization. To quantify the degree of invasion by non-native trees in the United States, we combined two broad-scale datasets: (1) tree species occurrence data from the national Forest Inventory and Analysis (FIA) plot network and (2) ecoregions characterized by relatively homogeneous environmental conditions. Using the FIA statistical design, we created an indicator of non-native tree regeneration success by estimating the proportion of small trees (seedlings and saplings) relative to all trees for non-native species in the conterminous United States and southeast Alaska, Hawaii, and Puerto Rico. Species with at least 75 percent of their stems consisting of smaller stems were classified as highly invasive while those with 60 percent to 75 percent of smaller stems were classified as moderately invasive. Above these thresholds, non-native species are of ecological concern because they are established and reproducing successfully and therefore likely to continue to spread in the future. For the conterminous United States and southeast Alaska, we identified 16 highly invasive and four moderately invasive non-native tree species. Widespread highly invasive and relatively well-established species included Ailanthus altissima, Triadica sebifera, and Ulmus pumila. The richness of highly invasive species was the highest in parts of the Midwest and Mid-Atlantic States, followed by much of the Southeast. In Hawaii, we identified seven highly invasive and three moderately invasive non-native tree species. The most widespread highly invasive and well-established tree species in the archipelago were Psidium cattleyanum, Psidium guajava, Ardisia elliptica, and Syzygium cumini. The largest numbers of highly invasive species were inventoried in the lowland/leeward dry and mesic forests of O’ahu and the lowland wet and mesic forests of Hawai’i Island. Puerto Rico had 17 highly invasive and two moderately invasive tree species. The most widespread and well-established non-native species were Leucaena leucocephala, Spathodea campanulata, Coffea arabica, Syzygium jambos, and Melicoccus bijugatus. The results of this assessment offer insights into which species are most likely to alter forest ecosystems and which forests may be effectively managed to control invasive trees.
Introduction
Non-native (introduced, exotic, alien) invasive species represent one of the most significant drivers of change to natural ecosystems globally (Brondizio et al., 2019) and are major indicators of global biodiversity decline (Butchart et al., 2010). The extent of international transport and commerce has resulted in few parts of the world being sheltered from the introduction of non-native species (Mack et al., 2000). The impacts of invasive non-native species on terrestrial ecosystems include alteration of the functions and services they provide (Pejchar and Mooney, 2009; Liebhold et al., 2017), reduction of native species abundance and diversity (Vila et al., 2011; Pyšek et al., 2012), and the homogenization of animal and plant communities (La Sorte and McKinney, 2007; McKinney and La Sorte, 2007). The ecosystem and biodiversity impacts of non-native invasive species have accelerated in recent decades (Brondizio et al., 2019; Pyšek et al., 2020) and are expected to increase in the future with the continued expansion of global commerce and other human activities (Lodge et al., 2006).
Contrary to some conventional thinking, even relatively undisturbed forests are affected by non-native invasive species (Martin et al., 2009). Not all non-native species are problematic, however, in the sense that they are not able to naturalize and spread (Richardson et al., 2000) or do not have adverse impacts on the environment or on human well-being (Tollington et al., 2017). A key challenge is to determine which species are likely to pose these risks (Bartz and Kowarik, 2019). Thousands of non-native tree species are planted around the world to provide an extensive list of ecosystem services, including wood and fiber production, erosion control, and aesthetic and amenity benefits (Richardson and Rejmánek, 2011; Brundu et al., 2020). In fact, non-native tree plantations encompass approximately 58 million ha globally, or about 44 percent of all planted forests, with higher proportions in South America, Africa, and Europe than in North America and Asia (FAO, 2020). Some non-native trees, however, have negative effects on ecosystems, such as the reduction of biodiversity and alteration of ecosystem functions, and on human well-being, such as damage to infrastructure and harm to human health (Shackleton et al., 2014; Castro-Díez et al., 2019). If left unchecked, some non-native trees have the potential to replace ecologically and commercially important native species (Webster et al., 2006). Such problems are most intense when non-native trees have naturalized and become invasive in areas beyond where they were planted (Richardson and Rejmánek, 2011; Brundu and Richardson, 2016). Globally, more than 400 tree species are recognized as naturalized (that is, consistently reproducing) and invasive (that is, spreading) in areas outside their native ranges (Rejmánek and Richardson, 2013).
In the United States, a national standardized network of forest plots (Bechtold and Patterson, 2005) provides an unbiased statistical view of plant invasions across broad areas and over time (Oswalt et al., 2015). Recent analyses using this Forest Inventory and Analysis (FIA) dataset have found that large areas of forest in the United States contain non-native invasive plant species (Oswalt et al., 2015; Riitters and Potter, 2019) and that forests of the eastern United States are more highly invaded than those of the West by multiple measures, including the richness of invasive species, the proportion of plots that are invaded, and the diversity of plant growth forms (Iannone et al., 2015, 2016). The probability of invasion is highest in eastern forests that are relatively productive and located in fragmented landscapes that contain developed or agricultural land cover (Riitters et al., 2017). These analyses focus on understory invasive plants rather than on non-native trees, which have been the emphasis of fewer analyses using the FIA data. These data, however, have been used to quantify the proportion of plots invaded by non-native tree species within ecoregions of the conterminous United States (the 48 States excluding Alaska and Hawaii), with exotic non-native trees found to be most prevalent along the Gulf Coast and within the Mid-Atlantic and Midwestern States (Potter and Simth, 2012). More recently, Lugo et al. (2022) documented that non-native tree species are undergoing a large-scale, low-intensity ecological process of expansion in the conterminous United States. These analyses, however, do not identify which non-native tree species are the most invasive, or invasive at all, based on the understanding that plant species are invasive when they are able to produce reproductive offspring in areas distant from their introduction sites (Richardson et al., 2000). Such information would be useful not only for the management of the forests of the conterminous States, but also for those of Hawaii and Puerto Rico, tropical island ecosystems where non-native trees account for nearly 40 percent of live aboveground tree carbon (Lugo et al., 2022).
To improve management efficacy and to better allocate limited resources, we need to distinguish species with a high potential for causing major ecosystem impacts from those with a more limited impact (Hulme et al., 2013; Kumschick et al., 2015). To evaluate the degree to which non-native tree species in the United States are or may become invasive, we developed a quantitative approach that combined two broad-scale datasets: (1) tree species occurrence data from the national Forest Inventory and Analysis (FIA) plot network and (2) ecoregions characterized by relatively homogeneous environmental conditions. Using the FIA statistical design, we created an indicator of non-native tree regeneration success by estimating the proportion of small trees (seedlings and saplings) relative to all trees for each non-native tree species inventoried by the FIA program in the 50 United States and Puerto Rico. This approach is similar to our recent publication that determined whether native trees were at risk of genetic degradation depending on whether the number of small trees in each species and within-species region was below an unsustainable reproduction threshold (Potter and Riitters, 2022). The threshold between sustainable and unsustainable proportions of small trees reflected the expectation of age-class balance at the landscape scale. Here, we used the same framework for non-native trees, but identified species across broad scales (within the conterminous United States and southeast Alaska, Hawaii, and Puerto Rico, respectively) and within ecoregions above a regeneration threshold as those of ecological concern because successful current regeneration by the non-native species may indicate continued spread in the future. We also calculated, as an indicator of establishment, the mean frequency of plot-level occurrences for each non-native species across the ecoregions in which it occurs.
Materials and methods
Data
The foundation of our analyses was the Forest Inventory and Analysis (FIA) equal-probability sample network of plots across the 50 States and Puerto Rico. The FIA program, administered by the United States Department of Agriculture Forest Service, is the primary source of information about condition, extent and trends of U.S. forest resources across both private and public ownership and management (Smith, 2002). It is the most comprehensive forest database currently available in the United States (Tinkham et al., 2018). The central feature of the FIA program is its extensive network of plots, spatially balanced through the use of a national hexagonal sampling framework (Bechtold and Patterson, 2005), with a random plot location within each 2,428 ha hexagon visited by field crews if it is determined by remotely sensed data to be in forest land use (Burrill et al., 2018). FIA plots are divided into spatially balanced panels of plots by state or territory, with one panel of plots measured every year on a rotating basis and with all panels together representing a compete measurement cycle. Measurement cycles in the eastern United States encompass five to seven panels, while those in the western United States encompass 10 panels. Forested plots are therefore typically visited every 5–7 years in the eastern United States (including Puerto Rico) and every 10 years in the West (including Hawaii). Our analyses used a recent cycle of plots for each state or territory (Figure 1), inventoried by field crews between 2004 and 2018. Because each plot is measured only once during a state or territory’s measurement cycle, pseudo-replication is not an issue.
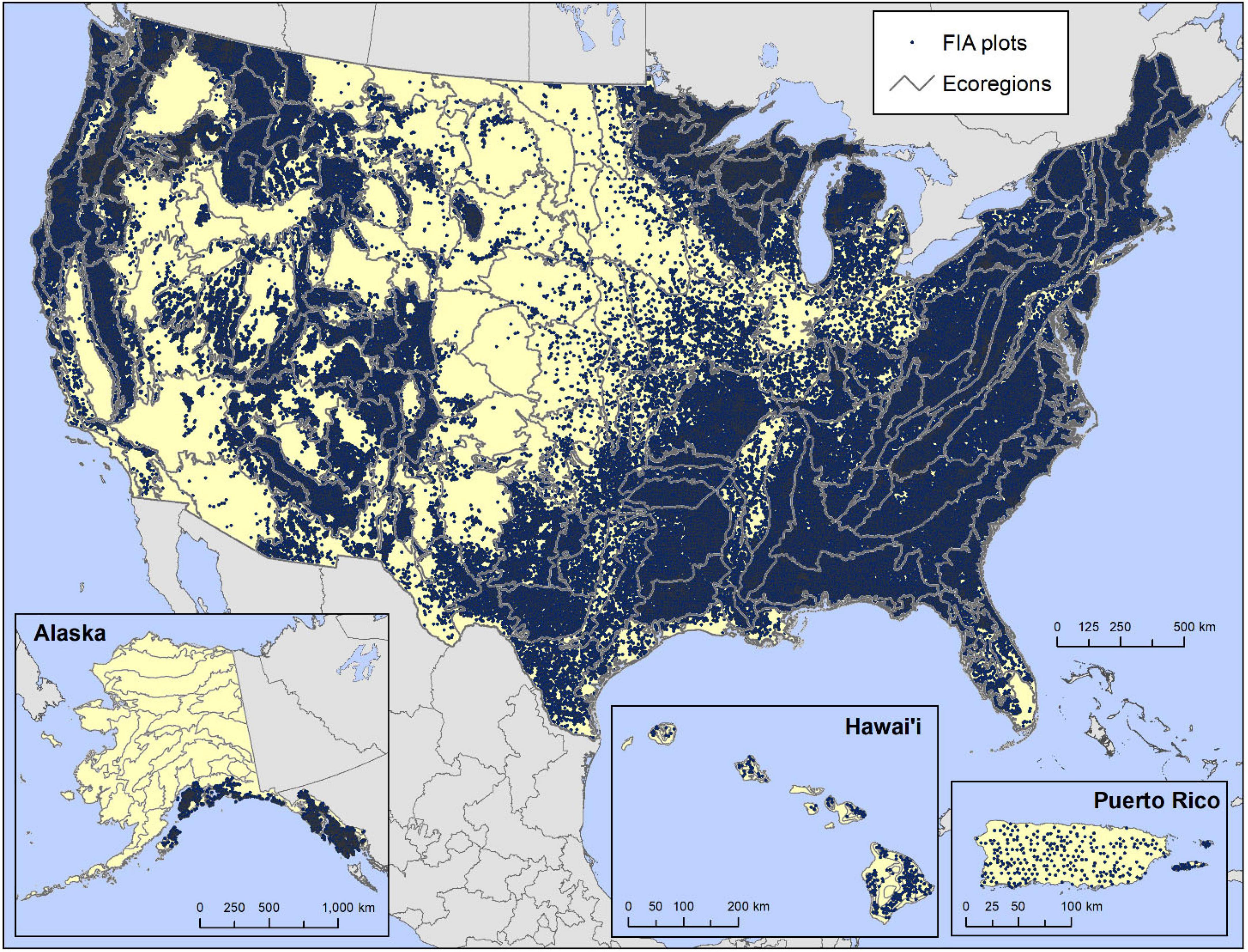
Figure 1. Tree species occurrence data from 140,026 USDA Forest Service Forest Inventory and Analysis (FIA) plots in the conterminous United States and Alaska, 238 in Hawaii, and 294 in Puerto Rico.
The FIA plots encompass four 7.31-m radius subplots arranged in a triangle, with one subplot in the center; each plot covers a total area of 0.067 hectares (Burrill et al., 2018). Data-collection crews measure many tree attributes, including the species, diameter, and height of each live tree with a DBH of ≥12.7 cm on the subplot. The crews measure sapling-sized trees and seedlings on a 2.07 m radius microplot offset from the center of each subplot. Saplings are stems with a DBH ≥ 2.54 cm and <12.7 cm, while seedlings are those with a DBH < 2.54 cm and height ≥ 30.48 cm if a hardwood, or a height of ≥15.24 cm if a conifer (Burrill et al., 2018). The plots included in the current analyses (140,026 in the conterminous United States and southeastern Alaska [sampling in interior Alaska is ongoing but not complete], 238 in Hawaii, and 294 in Puerto Rico). Plots are inventoried by field crews if the canopies of live trees of any size cover at least 10% of the plot area, or if there is evidence that this amount of live tree canopy cover existed in the past based on evidence such as stumps and snags, and if the tree canopy area is or was at least 0.4 ha in size and 37 m wide (Burrill et al., 2018). Tree-covered areas in agricultural production, such as orchards, or in urban parks are not considered forest land use and are therefore not inventoried.
Through the use of statistical “expansion factors” assigned to each plot, the FIA program’s systematic sampling design enables the calculation of population-level estimates of the number of trees in a sampled region (Bechtold and Patterson, 2005; Pugh et al., 2018). The expansion factor of a plot is calculated as the area within the stratum of interest (e.g., the forestland within a state) divided by the number of plots within the area of that stratum. To estimate the total number of trees of interest within a region (e.g., trees of a given species in an ecoregion in the northeastern United States or on the island of Puerto Rico), the expansion factor is first multiplied by the number of trees per hectare represented by each tree of interest on each plot (14.87 for trees with diameter at breast height [DBH] of ≥12.7 cm and 185.24 for trees <12.7 cm DBH) and then multiplied by an adjustment factor accounting for whether the tree was measured on a subplot or microplot (Burrill et al., 2018). Summing these estimates across all the plots within a region results in an estimate of the total number of stems of that species in the region.
Plots in our analyses were assigned to ecoregion sections within the conterminous United States (Cleland et al., 2007) and Alaska (Spencer et al., 2002) (Figure 1). These sections encompass regions similar in geology, climate, soils, potential natural vegetation, and potential natural communities (Cleland et al., 1997). Hawaiian plots were assigned to ecoregions developed for that State based on moisture and elevational/temperature characteristics within islands (Potter, 2020). No similar ecoregion map exists for Puerto Rico.
We determined the nativity of tree species in the conterminous United States, Alaska, and Puerto Rico from the USDA Plants database (U.S. Department of Agriculture Natural Resource Conservation Service, 2022). Our analyses differentiated between native and non-native tree species in Hawaii based on Imada (2012, 2019); to be consistent with the conterminous United States and Puerto Rico, species were considered non-native if they had been introduced to Hawaii following European arrival in the 18th century and subsequently had become naturalized. Our analyses were based on the separate lists of tree species inventoried by FIA within the conterminous United States and Alaska, Hawaii and other Pacific Islands, and the United States Caribbean islands. The lists for the Pacific and Caribbean, compared to the list for the mainland United States, tend to include several species that are sometimes more shrub-like than tree-like (habitat-dependent). For consistency and completeness, however, we include all species the FIA program inventories in these places rather than attempting to differentiate those that are always tree-like. Additionally, some inventoried non-native tree species may occur as remnants of abandoned agriculture or plantations, or in areas of active agroforestry, and may not be invasive. These are included to provide a complete comparison of all non-native species regardless of expected invasiveness. Finally, not all possible non-native tree species are included on the FIA regional lists, especially less common and recently introduced species. The emphasis of this assessment, however, is not to provide a comprehensive assessment of all non-native trees, but rather those that are common enough to be included in the FIA lists.
Analyses
Our indicators of non-native tree species invasion are based on the premise, described by Richardson et al. (2000), that a plant species is invasive if it is successfully producing reproductive offspring in areas distant from the site of its initial introduction. While we do not have information about the introduction locations of each non-native tree species, we assume that a species is successfully reproducing far from its introduction site if it does so across a broad geographic area, such as several ecoregions (in the conterminous United States or Hawaii) or many plots in Puerto Rico. Thus, the term “invasive” is used without any connection to ecological or economic impacts (Richardson et al., 2000). By our definition, successful regeneration is occurring if the non-native species includes a considerably larger proportion of small trees (seedlings and saplings) compared to the number of mature trees (60 percent for moderately invasive and 75 percent for highly invasive). This concept is similar to, but essentially the reverse of, a previous assessment of native tree vulnerability to genetic variation loss due to insufficient regeneration (Potter and Riitters, 2022). In that assessment, native trees were determined to be vulnerable if the number of seedlings and saplings did not exceed a threshold of 75 percent of all the species’ stems, either across its distribution or within ecologically similar parts of it.
The first step in our current assessment was to delineate the diameter at which to divide large trees from small trees (seedlings and saplings) for each species. The FIA program defines a diameter of 12.7 cm to be the threshold between a sapling and a mature tree (see above), but this does not account for difference in diameter size distribution among tree species. Some species, such as Eucalyptus robusta Sm. in Hawaii and Puerto Rico and Salix alba L. in the conterminous States, have broad size distributions, while others, such as Triadica sebifera (L.) Small in the conterminous States and Psidium cattleyanum Sabine in Hawaii, have much narrower distributions. To compare the relative regeneration success across species with different diameter distributions, we used a standardized method to separate small trees from large trees. To do this, we defined a cutoff within each species that was 20 percent of the 99.9th percentile maximum diameter estimate of all trees (including seedlings and saplings) separately within the 48 conterminous States and Alaska, Hawaii, and Puerto Rico. We calculated the estimated number of trees by species within each area using the FIA expansion factors described above. When a species had a 20th percentile greater than 12.7 cm (the FIA threshold between sapling and tree), we set our cutoff at 12.7 cm to be consistent with the FIA methodology (Hoover and Smith, 2020). Species having a 99th percentile maximum diameter estimate that was less than 2.54 cm constituted a special case, because diameters of seedlings less than 2.54 cm are not recorded on FIA plots. In other words, these species consisted only of stems in the seedling stage, and presumably are relatively early in the establishment phase of invasion. Such species may or may not become ecologically and economically problematic in the future depending on whether individuals are able to grow into the larger size classes and subsequently reproduce. Because each of these species occurred only on one or two plots, we classified the invasiveness of these species as undetermined.
In our second step, we used the FIA sample design to determine the percent of trees within each species that exceeds the small-tree cutoff. We applied the FIA expansion factors to estimate the total number of stems within size classes, within strata defined as the conterminous United States and Alaska, Hawaii, and Puerto Rico, and for ecoregion strata (described above) for the States (but not for Puerto Rico).
Third, we compared these percentages with thresholds at which we considered a non-native tree species to be successfully regenerating and thus to be invasive. Species with at least 75 percent of their stems consisting of smaller trees (seedlings and saplings) were classified as highly invasive while those with 60 percent to 75 percent of their stems consisting of smaller trees were classified as moderately invasive. Such species inventoried on fewer than five plots were classified as having “potentially high” or “potentially moderate” invasiveness because of the smaller sample size. Below the 60 percent threshold, species were considered to have low invasiveness. We calculated the number of species within each category within each region (conterminous United States and Alaska, Hawaii, and Puerto Rico) and within ecoregions. These thresholds were selected for consistency with the earlier assessment of native tree regeneration deficit, for which percentages of small tree stems below 75 percent were considered unsustainable and made a species vulnerable to losing genetic variation (Potter and Riitters, 2022). In this case, we are concerned about the likelihood of a non-native species to persist and continue to spread, so those with more than 75 percent small stems are highly likely to persist and spread and those with 60–75 percent are somewhat likely to persist and spread. These thresholds are somewhat arbitrary but are informed by the negative exponential or rotated sigmoid diameter distributions that are expected in balanced uneven-aged forests at the stand level (Rubin et al., 2006; Janowiak et al., 2008). We applied the idea of stand-level balance to stands aggregated at the landscape or statistical population level.
Fourth, we calculated the number and percent of non-native species that exceeded the regeneration thresholds across our three areas of interest (conterminous United States and Alaska, Hawaii, and Puerto Rico) and within the ecoregions of the States. The ecoregion results were mapped using ArcGIS 10.7.1 (ESRI, 2019). We also mapped the ecoregion-level invasiveness ratings (high, moderate, or low) for some widespread non-native tree species.
As a separate indicator of non-native species regional establishment, we determined the frequency of plot-level occurrences for each non-native species across the ecoregions in which it occurs (and for the entirety of Puerto Rico). For each species, we then calculated the mean proportion of these frequencies across the continental United States and Hawaiian ecoregions in which the species was inventoried. (Ecoregions containing fewer than five FIA plots were dropped from this analysis). For Puerto Rico, we reported the overall plot-level frequency of each tree species for the commonwealth.
Results
Species-level assessments of non-native tree invasion
Thirty non-native tree species were inventoried on FIA plots within the conterminous United States and southeast Alaska (Table 1). The most common of these were Triadica sebifera, detected on 1,302 plots, and Ailanthus altissima (Mill.) Swingle, found on 1,017. These represent, respectively, 0.9 percent and 0.7 percent of the 140,026 forested plots in the region. Using the FIA statistical design, we estimated the existence of approximately 470 million large stems and 2.7 billion small stems of T. sebifera and 132 million large stems and 1.2 billion small stems of A. altissima. Of the 30 non-native species, 16 were considered highly invasive (occurring on at least five FIA plots and exceeding the 75 percent threshold of all stems being small), four were potentially highly invasive (exceeding the 75 percent threshold but occurring on fewer than five plots), and four were moderately invasive (60–75 percent small stems). The highly invasive species that were the most geographically widespread – i.e., occurring in the most ecoregions – were A. altissima, Morus alba L., Ulmus pumila L., Acer platanoides L., and Picea abies (L.) H. Karst. Of the widespread species, T. sebifera was classified as highly invasive in every one of the 20 ecoregions in which it occurred. A. altissima was also categorized as highly invasive in nearly all the ecoregions where it was detected (39 of 44, 88.6 percent). Five species were classified as having low invasiveness, four of which encompassed no small stems.
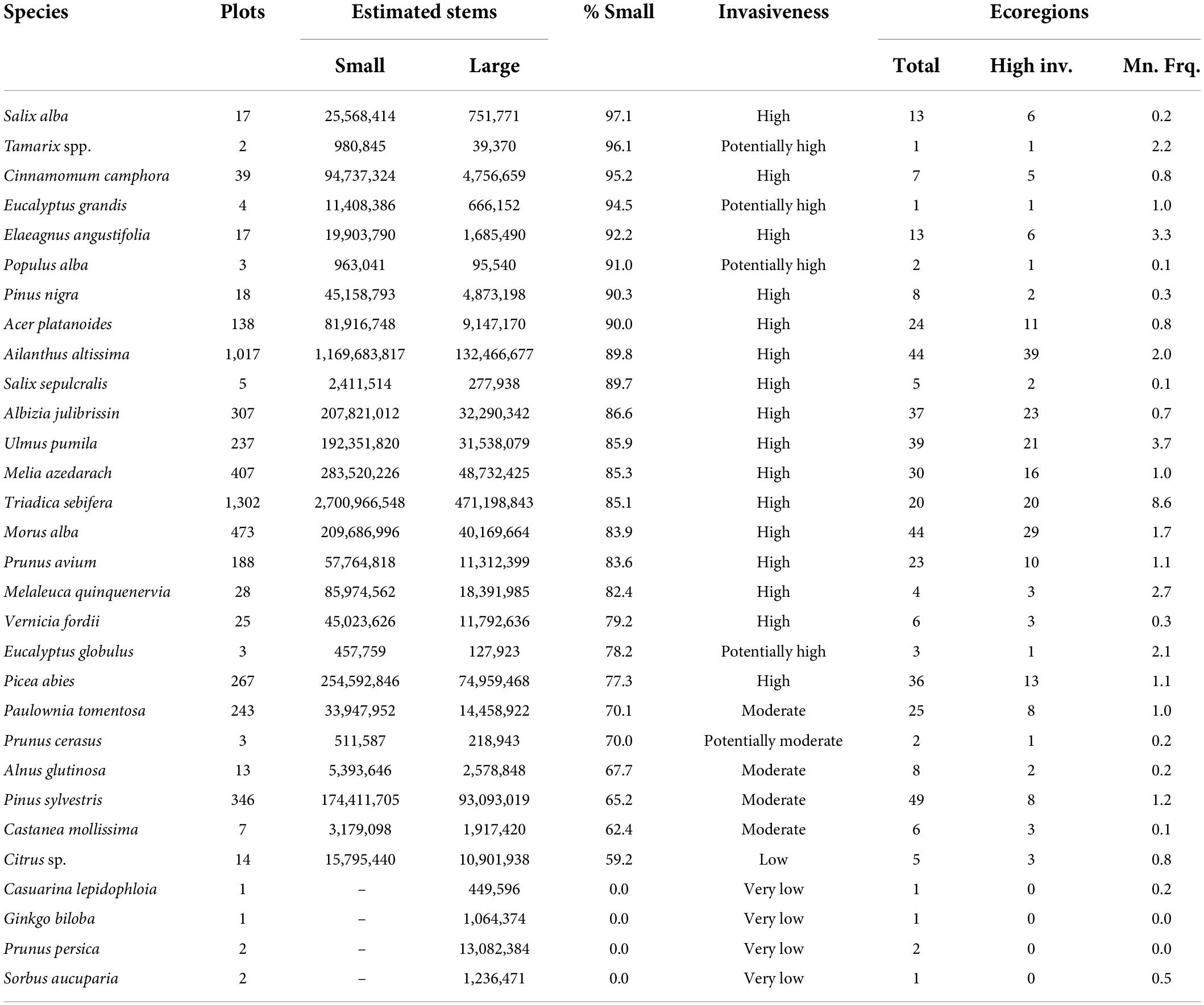
Table 1. Thirty non-native tree species detected by the Forest Inventory and Analysis (FIA) program in the conterminous United States and Alaska, along with the number of plots on which each was inventoried; the estimated number of small and large stems based on the FIA sample design; the percent of all trees within the small tree size class; the overall potential invasiveness assigned to the species based on its proportion of smaller trees, and the total number of ecoregion sections on which each species was detected, the number of ecoregion sections where each species is highly invasive, and the mean frequency (percent of plots) on which the species occurs across the ecoregions in which it is present.
More than twice as many non-native tree species (62) were inventoried in Hawaii than in the conterminous United States and Alaska (Table 2) despite its much smaller land area. Psidium cattleyanum was the most commonly detected, inventoried on 88 of the State’s 238 forested plots (40 percent). We estimated 270 million large stems and 1.2 billion small stems of this species. Field crews identified the closely related Psidium guajava L. on 32 plots and Schinus terebinthifolius G. Raddi on 28. We estimated that both Leucaena leucocephala Lam. (de Wit) and Ardisia elliptica Thunb. had more stems in Hawaii than P. guajava and S. terebinthifolius despite occurring on fewer plots (14 and 11, respectively) because their stem density was higher on the plots on which they occurred. Of the 62 non-native tree species on Hawaiian FIA plots, seven were classified as highly invasive, nine as potentially highly invasive, three as moderately invasive, and seven as potentially moderately invasive. An additional 11 species consisted only of small stems on one or two plots, so it was not possible to assess their invasiveness. Further, 18 species occurred only as large stems, and were therefore not invasive. At least some of these (including Eucalyptus deglupta Blume, Eucalyptus saligna Sm., and Pinus patula Schiede ex Schltdl. & Cham.) may occur in current or abandoned tree plantations. Several of the widespread species were highly invasive in the large majority of Hawaiian ecoregions in which they occurred, including P. cattleyanum, P. guajava, A. elliptica, and Prosopis pallida (Humb. & Bonpl. ex Willd.) Kunth.
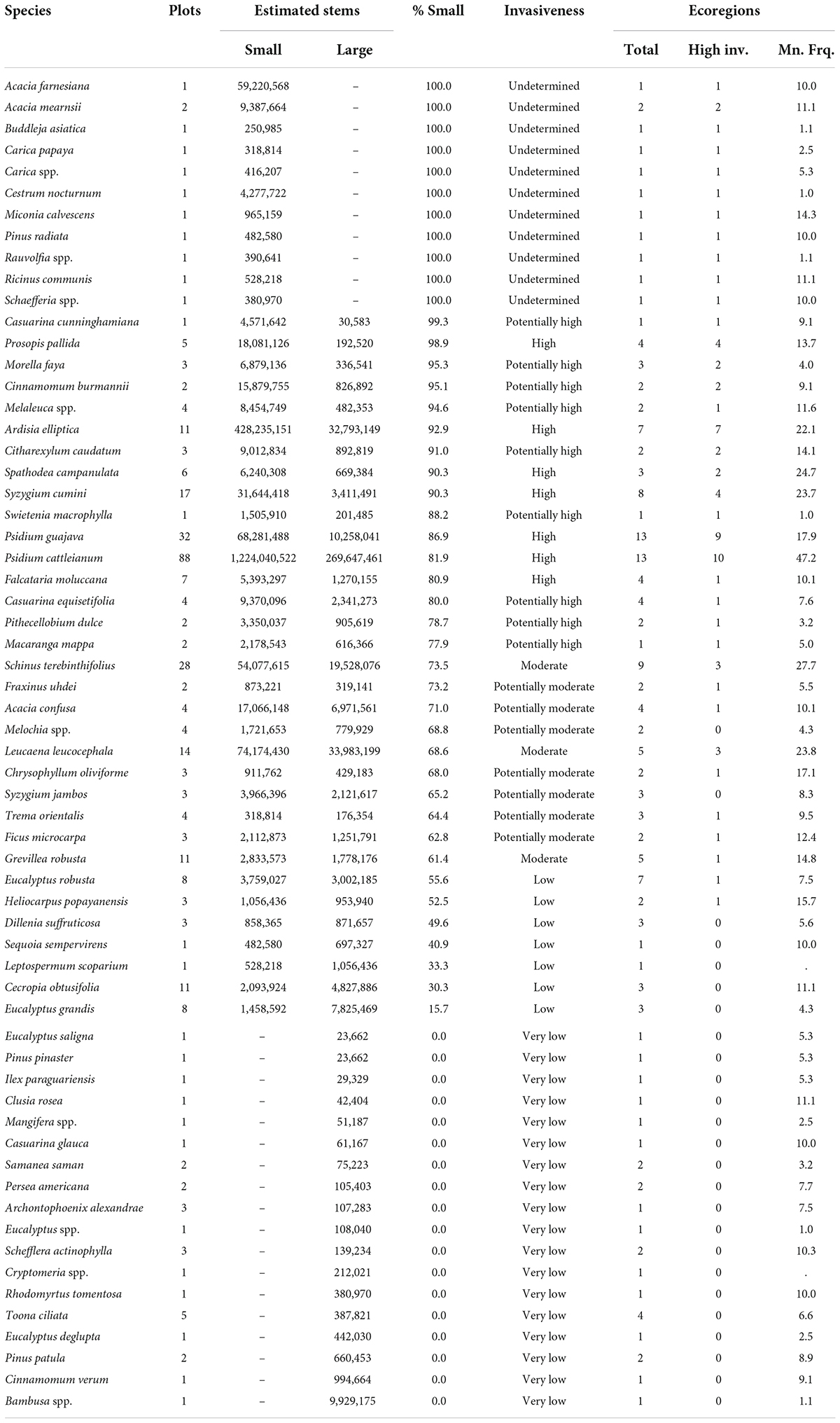
Table 2. Sixty-two non-native tree species detected by the Forest Inventory and Analysis (FIA) program in Hawaii, along with the number of plots on which each was inventoried, the estimated number of small and large stems based on the FIA sample design; the percent of all trees within the small tree size class; the overall invasiveness assigned to the species based on its proportion of smaller trees; and the total number of ecoregions on which each species was detected, the number of ecoregions where each species is highly invasive, and the mean frequency (percent of plots) on which the species occurs across the ecoregions in which it is present.
Fifty-seven non-native tree species were inventoried in Puerto Rico (Table 3), which has approximately half the land area of Hawaii. The most widespread and common tree species was Leucaena leucocephala, found on 37 of 294 forested plots (12.6 percent) and encompassing an estimated 90 million large stems and 247 million small stems. Other common species included Prosopis pallida (10.9 percent of FIA plots), Coffea arabica L. (6.1 percent), and Spathodea campanulata P. Beauv. (6.1 percent). Seventeen of the non-native tree species in Puerto Rico were categorized as highly invasive and 16 as potentially highly invasive, while only two were moderately invasive. Eight species consisted only of small stems on one or two plots, which thus had undetermined invasiveness, and eight species also consisted only of large stems, which thus were not invasive.
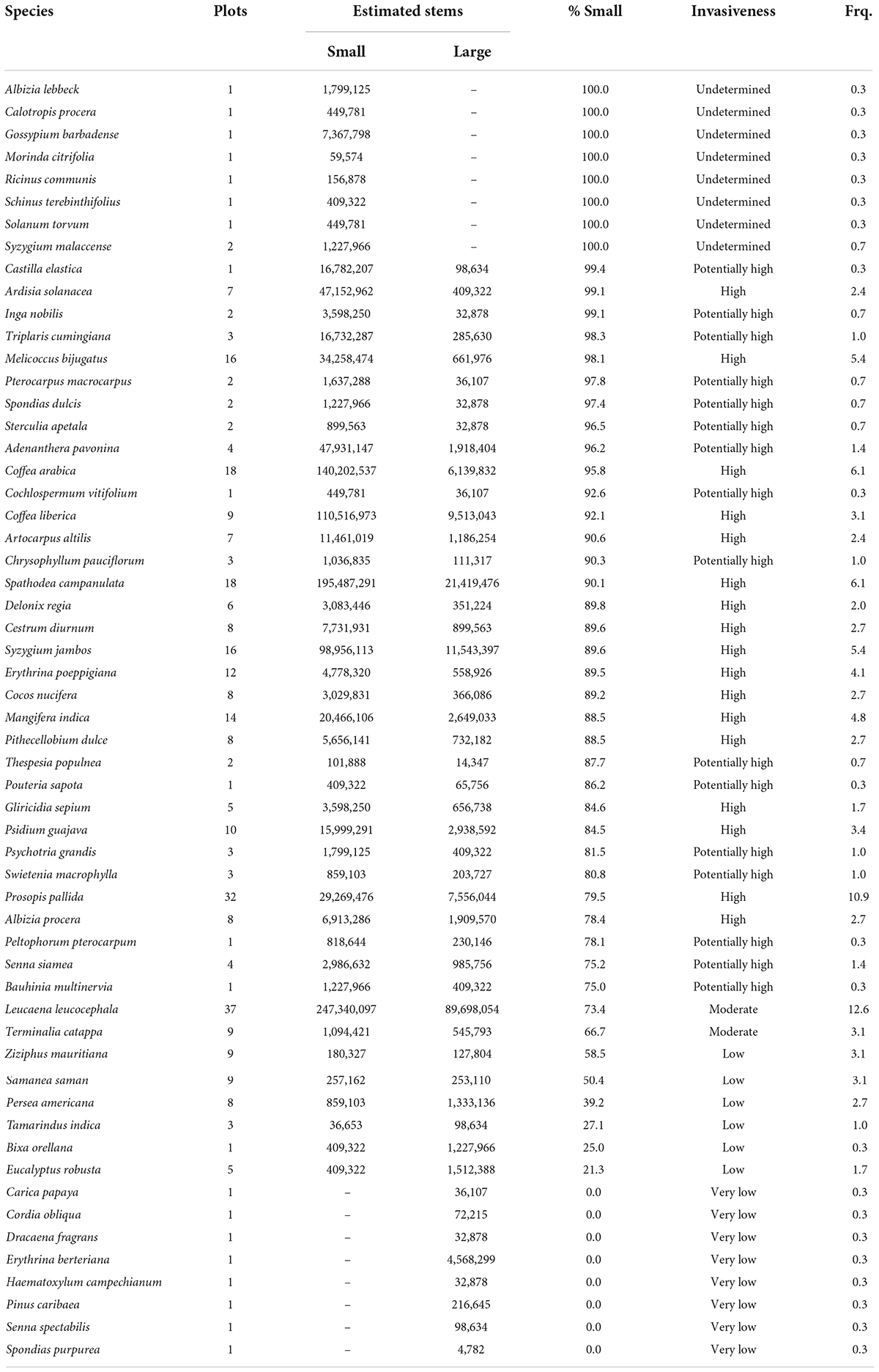
Table 3. Fifty-seven non-native tree species detected by the Forest Inventory and Analysis (FIA) program in Puerto Rico, along with the number of plots on which each was inventoried, the estimated number of small and large stems based on the FIA sample design, the percent of all trees within the small tree size class, the overall invasiveness assigned to the species based on its proportion of smaller trees, and the frequency (percent of plots in Puerto Rico) on which the species occurs.
Geographic patterns of non-native tree invasion
In the conterminous United States and Alaska, the ecoregions with the highest non-native tree richness are in the Mid-Atlantic and Great Lakes States, encompassing as many as 13 species (Figure 2). Broadly, the forests of the eastern States have more non-native species than do western forests. Non-native trees are almost entirely absent from the Rocky Mountain States and Alaska, while ecoregions along the Pacific Coast from California to Washington contain one or two species each. Highly invasive non-native species were most diverse in ecoregions of the Mid-Atlantic States and the Southeast, with seven to eight species each (Figure 3).
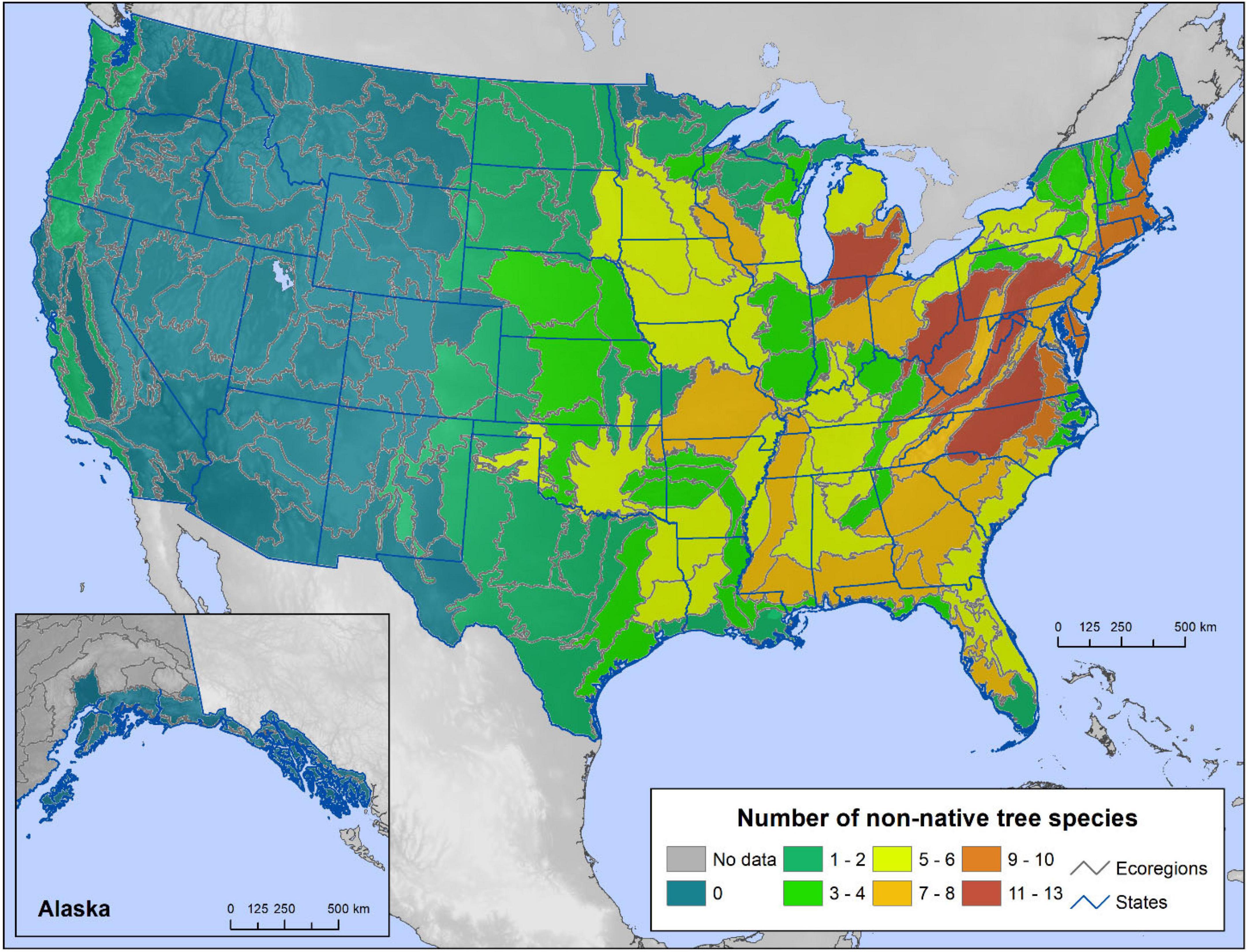
Figure 2. The number of non-native tree species present in each ecoregion section of the conterminous United States and coastal southeastern Alaska using Forest Inventory and Analysis (FIA) tree occurrence data.
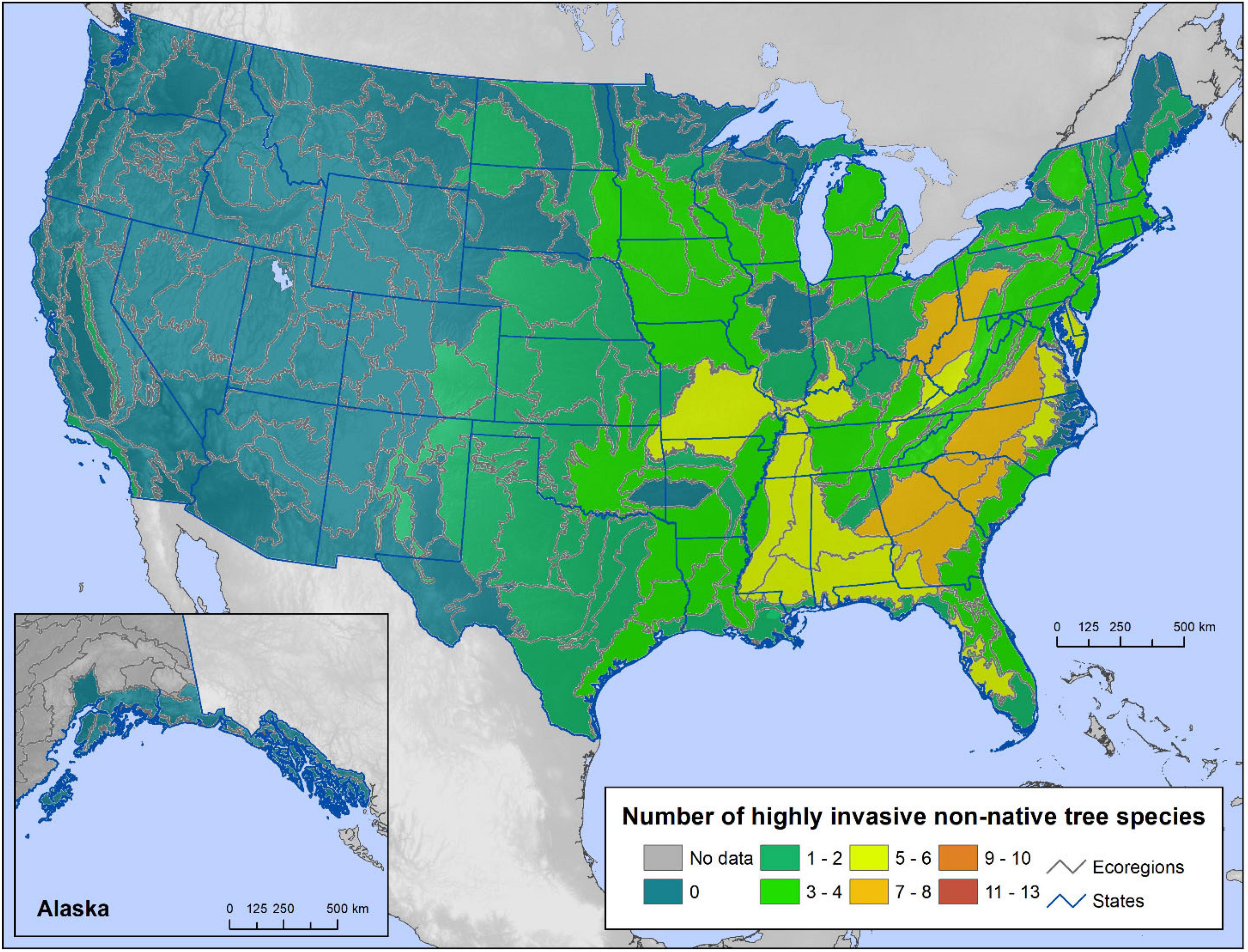
Figure 3. The number of highly invasive non-native tree species present in each ecoregion section of the conterminous United States and coastal southeastern Alaska using Forest Inventory and Analysis (FIA) tree occurrence data.
Two highly invasive species, A. altissima and Morus alba, were widespread across Eastern ecoregions, with A. altissima characterized as highly invasive in nearly all the ecoregions in which it occurred, while M. alba had lower levels of regeneration (i.e., less invasiveness) on about a third of its ecoregions (Supplementary Figures 1A,B). Among widespread non-native species, only T. sebifera was classified as highly invasive everywhere it occurred, which was across much of the Southeastern United States (Supplementary Figure 1C). Picea abies, meanwhile, was mainly limited to the Northeast and was highly invasive in only a third of its ecoregions (Supplementary Figure 1D).
The mean plot frequency of occurrence across ecoregions (an indicator of regional establishment) was less than 2 percent for most non-native tree species in the conterminous United States and Alaska (Table 1). Some highly invasive non-natives exceeded this number, however. Triadica sebifera, for example, was inventoried on an average of 8.6 percent of the plots across the 20 ecoregions in which it occurred. Ulmus pumila was detected on an average of 3.7 plots across 39 ecoregions, Elaeagnus angustifolia L. on 3.3 percent of plots in 13 ecoregions, Melaleuca quinquenervia (Cav.) S.T. Blake on 2.7 percent of plots in four ecoregions, and A. altissima on 2.0 percent of plots in 44 ecoregions.
In Hawaii, the Mesic ecoregion on O‘ahu and the Lowland Wet ecoregion on Hawai‘i Island had the greatest non-native tree richness, with 22 and 21 species, respectively (Figure 4). Also relatively high were the Mesic ecoregions on Maui (20) and Hawai‘i Island (17), and the Lowland/Leeward Dry ecoregion on O‘ahu (17). Non-native diversity was lower on Kaua‘i, the Lowland Wet ecoregions on O‘ahu and Maui, the Lowland/Leeward Dry ecoregions on Maui and Lāna‘i, the Montane Wet ecoregion on Maui, and the Subalpine ecoregion on Hawai‘i Island. The number of plots in some of these areas was relatively small, however. The diversity of highly invasive non-native trees was highest the Mesic ecoregions of O‘ahu (9) and Maui (10), and the Lowland/Leeward Dry ecoregion of O‘ahu (9) (Figure 5). In general, the richness of non-native tree species was higher in lower-elevation ecoregions across the archipelago.
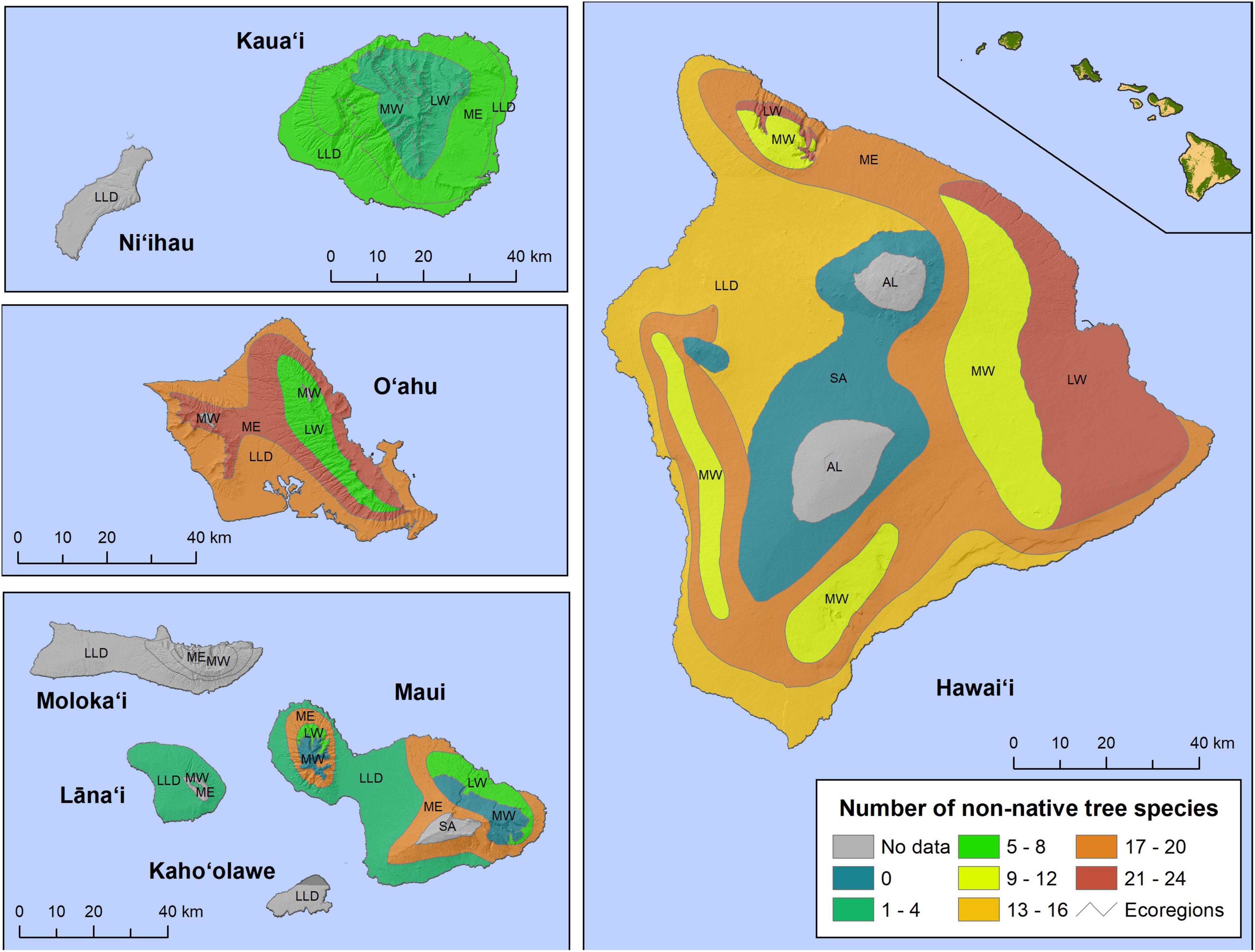
Figure 4. The number of non-native tree species present in each Hawaiian ecoregion, by island, using Forest Inventory and Analysis (FIA) tree occurrence data. (Note the binning of species richness differs from Figure 2). AL, alpine; LLD, lowland/leeward dry; LW, lowland wet; ME, mesic; MW, montane wet; SA, subalpline.
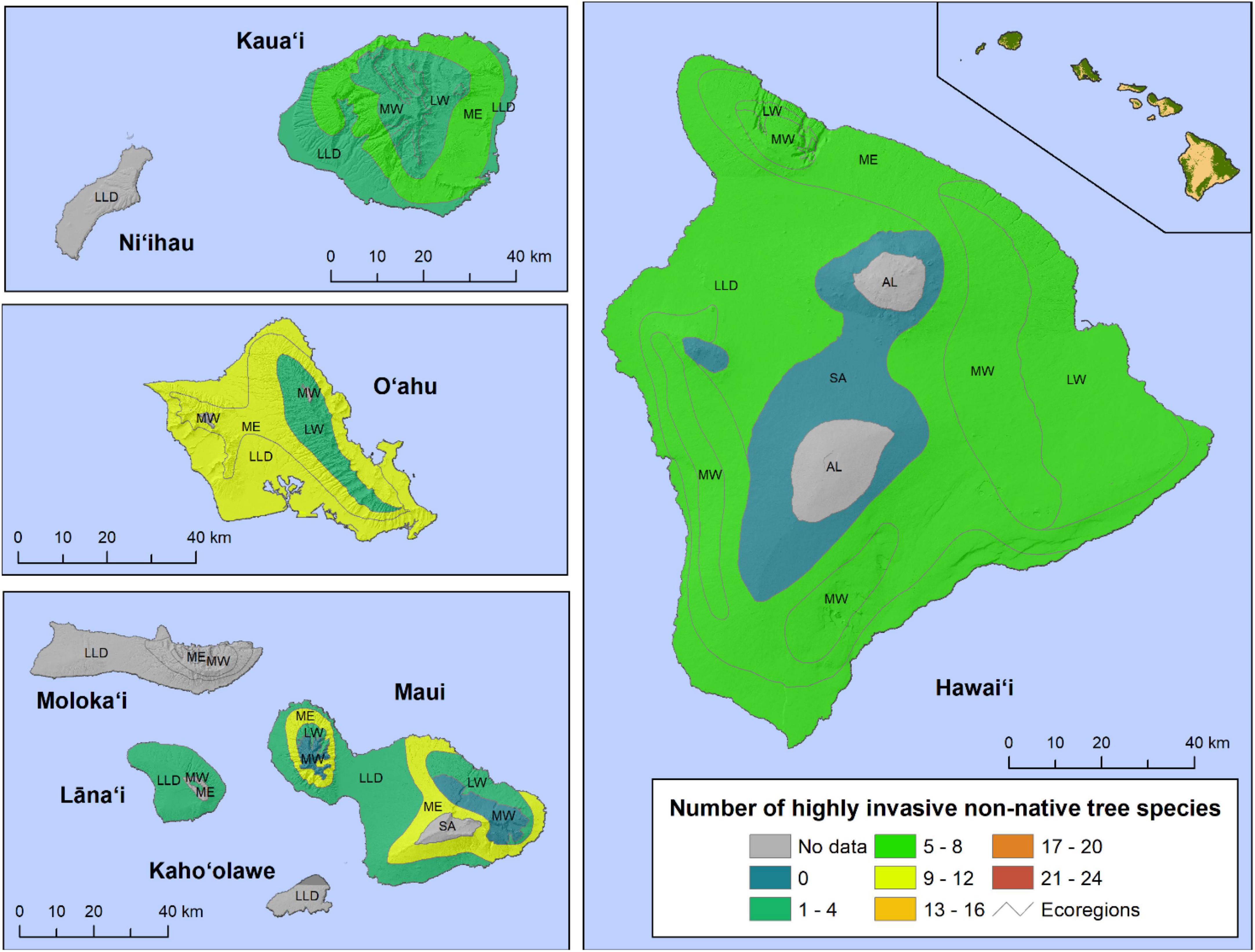
Figure 5. The number of highly invasive non-native tree species present in each Hawaiian ecoregion, by island, using Forest Inventory and Analysis (FIA) tree occurrence data. (Note the binning of species richness differs from Figure 3). AL, alpine; LLD, lowland/leeward dry; LW, lowland wet; ME, mesic; MW, montane wet; SA, subalpline.
The most common non-native tree in Hawaii, Psidium cattleyanum, was highly invasive in at least one ecoregion on each of the major surveyed islands (Supplementary Figure 2A). Syzygium cumini (L.) Skeels. was highly invasive in the lower-elevation ecoregions of Kaua‘i, the Mesic ecoregion of Maui, and the Lowland Wet ecoregion of Hawai‘i Island (Supplementary Figure 2B). Meanwhile, Ardisia elliptica was highly invasive and widespread on Kaua‘i, O‘ahu, and Maui, but was not yet present on Hawai‘i Island (Supplementary Figure 2C). Finally, Schinus terebinthifolius was highly invasive in one ecoregion each of O‘ahu (Lowland Wet), Maui (Lowland/Leeward Dry), and Hawai‘i Island (Mesic) (Supplementary Figure 2D).
Reflecting the highly invaded nature of Hawaiian forests (Dawson et al., 2017; Cordell, 2021), non-native species in the Pacific archipelago in general had much higher mean plot occurrence frequencies across ecoregions than in the continental United States. Psidium cattleyanum, for example, was present on an extraordinary 47.2 percent of plots on average across the 13 ecoregions in which it occurred. Other highly established non-native species included S. terebinthifolius (mean 27.7 plots, nine ecoregions), Spathodea campanulata (mean 24.7 plots, three ecoregions), L. leucocephala (mean 23.8 percent, five ecoregions), S. cumini (mean 23.7 percent, eight ecoregions), and A. elliptica (mean 22.1 percent, seven ecoregions).
Discussion
The threats posed by non-native trees vary among species and within their introduced ranges (Webster et al., 2006). To assess the relative invasiveness of non-native tree species across and within their distributions in the United States, we created an indicator of non-native tree regeneration success that employs a national systematic forest inventory to estimate the proportion of small trees (seedlings and saplings) relative to all trees. Species across their distributions and within ecoregions were determined to be most invasive when the number of small trees exceeded a threshold of ecological concern—those with 60–75% of stems exceeding the threshold were moderately invasive, while those with >75% were highly invasive. Such a quantification of the invasiveness of non-native tree species would be difficult in the absence of a large-scale inventory like the one provided by FIA (Lugo et al., 2022). We also calculated an indicator of regional establishment, as the mean frequency of plot-level occurrences for each non-native species across the ecoregions in which it occurs.
For the conterminous United States and Alaska, we identified 17 highly invasive non-native tree species and five moderately invasive species. We identified seven highly invasive and 10 moderately invasive non-native tree species in Hawaii and 17 highly invasive and two moderately invasive tree species in Puerto Rico. The relative richness of invasive species in Hawaii and Puerto Rico is quite high when considering that the tree canopy cover area (Coulston et al., 2012) of the two jurisdictions together sums to less than 0.5 percent that of the conterminous United States. This is not surprising given that islands on average globally have a relative richness of non-native plant species that is six times and a relative richness of invasive plant species that is more than three times that of mainland regions (Essl et al., 2019). Tropical islands are especially vulnerable to the naturalization of non-native species because their native species often compete poorly with the large number of continental species that have been introduced historically (Loope et al., 1988; Denslow, 2003). Hawaii, in particular, is a global hotspot of non-native species richness (Dawson et al., 2017; Cordell, 2021), with naturalized non-native plant taxa constituting approximately half its flora (Imada, 2012).
Across the Caribbean archipelago of which Puerto Rico is a part, 17 percent of plant species are not native (Acevedo-Rodriguez and Strong, 2008). Introduced tree species are very common across the island of Puerto Rico so that two-thirds of its forests comprise novel tree assemblages (Martinuzzi et al., 2013). This is the result, at least partially, of the relatively recent wide-scale abandonment and regrowth of agricultural land following the near complete clearing of forests across the island in its early colonial period (Lugo and Helmer, 2004; Brandeis et al., 2009). While non-native understory plants are problematic in parts of the conterminous United States (Oswalt et al., 2015; Riitters and Potter, 2019), non-native trees are comparatively uncommon (Potter and Simth, 2012; Lugo et al., 2022). They are also much less widely established, as indicated here by the frequency of plot occurrences across mainland ecoregions compared to Hawaiian ecoregions and to Puerto Rico in its entirety. Only seven non-native tree species in the conterminous United States and southeast Alaska occurred on at least 2 percent of FIA plots across the ecoregions in which they were inventoried. Meanwhile, 21 non-native tree species occurred on at least 2 percent of Puerto Rico’s plots. A remarkable 56 of 62 Hawaiian non-natives were inventoried on at least 2 percent of plots across Hawaii ecoregions on average, with 24 of these present on at least 10 percent of plots on average.
These results identify the non-native tree species that are successfully reproducing after reaching the canopy and therefore may be more likely to alter forest ecosystem functions and services. This is a different approach than comparing the invasiveness of non-native species based on the relative area they occupy in a region (Colautti and MacIsaac, 2004; Pereyra, 2016), which is an assessment only of how invasive those species have been to the present. Our approach additionally evaluates whether non-native species may continue to spread, with those classified as highly invasive being most likely to do so. In other words, this invasiveness indicator is a tool designed to identify which non-native tree species, across broad scales, are most likely to be problematic ecologically and economically into the future versus those that are present but less likely to cause problems. This information can be used to tailor management approaches to individual species. Efforts to manage the problems associated with non-native invasive trees span local-scale efforts to control invasions and mitigate their efforts to systematic national-scale strategies aiming to both reduce current impacts and reduce the risk of future problems (Richardson and Rejmánek, 2011; Shackleton et al., 2017; Iverson et al., 2019). Specific management approaches for invasive woody plants include public education, mechanical treatments, herbicide applications, and the development of effective biological control agents (Webster et al., 2006).
Additional indicators for each species, beyond its overall degree of invasiveness, quantify the geographic extent of its invasiveness (the number of ecoregions on which it occurs and the percent of these in which it is highly invasive) and the extent of its establishment (the mean proportion of plot occurrences in the ecoregions where it is inventoried). These indicators offer a glimpse of the areas where a species is likely to be problematic currently and where it may become so in the future without management intervention. In ecoregions where a species is highly invasive, complete elimination of a non-native tree species may not be possible except in high-value protected forests or on public land where invasive plants tend to be less widespread (Riitters et al., 2018). Areas of low invasiveness and establishment for a given species are places where eradication efforts are most likely to be effective because that species is not regenerating well on its own and spreading. Control efforts in ecoregions of moderate invasiveness and establishment may or may not be successful but are more likely to be so than places where a species is highly invasive. Examples in the conterminous United States and Alaska include Picea abies, Pinus sylvestris, and Paulownia tomentosa across much of their introduced distributions. Each of these species, incidentally, was inventoried on about 1 percent of plots across the ecoregions where it occurred, indicating that it may be a better candidate for broad-scale management than non-natives that are more widely established like T. sebifera, U. pumila, and A. altissima.
In the conterminous United States, A. altissima is uniquely problematic because it is highly invasive throughout its distribution (89.8 percent small trees) and across many ecoregions (39 of the 44 on which it occurs), where it is relatively well-established on average. This species has a long history in North America, introduced in the late 1700s and widely planted in urban areas of the Northeast during the 1800s because of its tolerance of air pollution (Miller, 1990). Invasion of A. altissima in forests increased in the late 1900s, generally following forest disturbances such as harvesting and outbreaks of defoliator insects (Kasson et al., 2013). In some cases, it has invaded mature forests using a “gap-obligate” strategy of forest recruitment, through which it reaches gaps in the canopy of mature forest following rapid growth during a single period of release (Knapp and Canham, 2000). Several of its life history characteristics undergird its invasive success, such as rapid growth, early reproductive maturity, the production of many wind-disseminated seeds each year, and the capacity for abundant vegetative reproduction (Miller, 1990). T. sebifera, which is highly invasive in every ecoregion where it occurs as well as being broadly well-established, has similar traits that make it a successful invader, including prolific production of seeds that are widely dispersed by birds and water (Renne et al., 2000; Vogt et al., 2021) and the ability to vegetatively sprout from stumps and roots (Bruce et al., 1997). It has a well-documented ability to invade both disturbed and undisturbed habitats in the Southeastern United States (Vogt et al., 2021). While T. sebifera and A. altissima are highly invasive across broad areas, Melaleuca quinquenervia appears to be highly invasive (inventoried on 2.7 percent of the plots in the ecoregions in which it occurs) in a limited geographic area in southern Florida. In these areas, M. quinquenervia has replaced native forest habitat and decreased biodiversity while threatening the ecological integrity of the Everglades ecosystem (Dray et al., 2006).
In Hawaii, Psidium cattleyanum is the most invasive non-native tree, occurring as a highly invasive species in most of the ecoregions where it occurs throughout the archipelago. It is also extremely well-established, generally present on almost half of the FIA plots in ecoregions where it occurs. Aggressive naturalized species like P. cattleyanum and Ardisia elliptica dominate the lowland wet forests of Hawaii, which are likely to eventually consist almost entirely of non-native trees in the absence of extensive control efforts (Cordell et al., 2009). The invasive success of P. cattleyanum is explained in part by its abilities to colonize undisturbed sites, to produce abundant seeds that germinate under a wide range of conditions, and to aggressively propagate clonally (Huenneke and Vitousek, 1990). Not surprisingly, the closely related P. guajava, which similarly exhibits extensive clonal reproduction, fast growth, and high fruiting rates (Urquia et al., 2019), is also highly invasive and well-established across much of Hawaii, although not as common as P. cattleyanum. P. guajava is one of a handful of species, along with Leucaena leucocephala and Spathodea campanulata, that are at least moderately invasive in both Hawaii (in the Pacific Ocean) and Puerto Rico (in the Caribbean Sea), offering evidence of the global transport and establishment of some tree species in tropical island ecosystems. Interestingly, Hawaii and Puerto Rico also share two species that we found to have low levels of invasiveness, Persea americana Mill. and Samanea saman (Jacq.) Merr., as well as one of currently undetermined invasiveness (because it exists only as small stems): Ricinus communis L. Non-native species that occurred only as small stems were limited to Hawaii and Puerto Rico; we found no such species in the conterminous United States and Alaska. This may suggest either that Puerto Rico and Hawaii contain more species that are in the early stages of naturalization or that the tree inventory lists for these places are more encompassing of newly introduced and/or shrubby species than the tree inventory list for the mainland (or a combination of the two). Regardless, these species may represent a better opportunity than others for suppression and eradication since presumably few trees have reached reproductive maturity yet. One leading candidate for management in Puerto Rico is Schinus terebinthifolius, since it is already shown itself to be moderately invasive in Hawaii but apparently has not moved much yet into the canopy of the Caribbean commonwealth.
Some of the Puerto Rican tree species that we found to be moderately to highly invasive (Table 3) are abundant enough to be considered well-suited as sources of timber and non-timber forest products, including S. campanulata, L. leucocephala, Syzgium jambos L. (Alston), and Mangifera indica L. (Forero-Montana et al., 2019). The land-use history of current forests in Puerto Rico is particularly relevant in understanding the distribution and importance of such non-native tree species. Specifically, recent land use has as much influence on the species composition of secondary Puerto Rican forests as biophysical factors such as climate and substrate (Chinea and Helmer, 2003). For example, S. campanulata, which commonly colonizes abandoned pastures (Rivera and Aide, 1998), has been the most abundant tree species in mainland Puerto Rico since the 1990s (Franco et al., 1997; Brandeis et al., 2007; Marcano-Vega, 2017). Because it is shade intolerant, it does not recruit in its own understory and therefore may decline while effectively providing habitat appropriate for the colonization of native tree species (Aide et al., 2000). Meanwhile, S. jambos, a shade-tolerant species that can form dense, monotypic stands under closed canopies (Brown et al., 2006), has become widely established on both former agricultural lands and abandoned coffee plantations (Brandeis et al., 2009). Because it can recruit under its own canopy, it may be able to remain indefinitely in the forests it inhabits unless it is managed via human intervention (Aide et al., 2000). The abundance of Coffea arabica and Coffea liberica Hiern is not surprising given that half of the FIA plots in Puerto Rican subtropical wet forest were in coffee cultivation in 1951 but were all abandoned by 1991 (Brandeis et al., 2009). Finally, the prevalence of fruit trees like M. indica in secondary forests reflects the past importance of the region’s agricultural economy (Marcano-Vega, 2017).
Many factors may impact the regeneration success of non-native tree species, especially at the stand level. For example, the succession of dry forest stands dominated by L. leucocephala and Prosopis pallida in Puerto Rico are sometimes arrested by chronic disturbance (Brandeis et al., 2009), while forests with highly eroded soils may persist in a degraded state (Flores et al., 2020) in which tree species are unable to grow to larger diameters. The focus of this project, however, was the development of relatively straightforward indicators that use empirical and spatially balanced data to report whether non-native species are likely to persist and possibly expand across broad regions of the United States. To do this, we aggregated plot-level observations to the landscape scale (the statistical population of our analyses) to determine whether the balance of small to large trees exceeds that expected for species that are successfully regenerating. Successful reproduction is a key factor in determining whether a species will be invasive and – at least at the landscape scale – we expect that it reflects a suite of factors relevant to plant invasion such as surrounding propagule pressure, time since invasion, and ability of a species to adapt to different environments. We see potential for developing a more comprehensive species-level invasive index that incorporates factors such as time since introduction, the likelihood of multiple introductions, and the ability of a species to adapt to different environments (e.g., Osunkoya et al., 2019), but such a project is beyond this scope of the current assessment.
We were able to combine information across tree species to determine which areas of the United States host the most non-native tree species and, among those, the most highly invasive tree species. In the conterminous States, the richness of all non-native species was highest in parts of the Midwest and Mid-Atlantic States, followed by much of the Southeast. The diversity of highly invasive species, however, was highest in parts of the Southeast and the Midwest. Meanwhile, the largest numbers of non-native tree species of Hawaii were inventoried in the lowland/leeward dry and mesic forests of O’ahu and the lowland wet and mesic forests of Hawai’i Island. Lower-elevation ecoregions across the Hawaiian archipelago had generally higher non-native species richness than higher elevation ecoregions, consistent with the findings of Ibanez et al. (2019) that elevation was negatively correlated with the richness of non-native plant species on Pacific tropical islands. Most of the ecoregions of Hawaii, especially on O’ahu and Hawai’i Island, had higher non-native richness than even the most highly invaded ecoregions in the conterminous United States. Parts of O’ahu and Maui had the most highly invasive tree species. Such information from broad-scale and systematic forest inventories could be useful in criteria and indicator (C&I) systems used by many nations to monitor forest sustainability status and trends relating to non-native tree species. For example, the Montréal Process Criteria and Indicators for the Conservation and Sustainable Management of Temperate and Boreal Forests, encompassing 12 countries and 49% of the world’s forests, tracks the area and percent of forest affected by biotic processes and agents (including invasive species) beyond reference conditions (Montréal Process Liaison Office, 2015). The Forests Europe sustainable management C&Is, meanwhile, include an indicator that specifically quantifies the amount of forest area covered by introduced tree species (Forest Europe, 2015).
We note some limitations of using the FIA dataset for the assessment of invasiveness in tree species. First, we were constrained by the lists of tally tree species the FIA program uses for each of three major regions: the conterminous United States and southeastern Alaska, the Pacific Islands including Hawaii, and the Caribbean islands including Puerto Rico. These lists are not entirely consistent in their incorporation of species groups (for example, bamboo is recorded on plots in Hawaii but not in the mainland United States or Hawaii) or in their inclusion of species that are sometimes shrub-like rather than consistently tree-like (such as Ricinus communis in both Puerto Rico and Hawaii). Relatedly, some inventoried non-native tree species may occur as remnants of abandoned agriculture or plantations, or in areas of active agroforestry, and may not be invasive. (This is often reflected in the results, which showed, for example, that eucalypt and pine species in Hawaii and Puerto Rico had low potential invasiveness). Second, we were unable to gauge the invasiveness of species that occur as small trees on only one or two plots. These species may be in the early stages of widespread invasion, or they may never be able to reproduce and spread their offspring after maturing into large trees. This point underscores the fact that FIA’s spatially unbiased sampling structure is not designed to detect newly introduced species that are early in the invasion process. Third, the results could be affected by the fact that the FIA program incorporates plots into its inventory based on forest land use rather than land cover. In other words, field crews inventory the trees at a plot location if it contains at least 10% canopy cover by live trees of any size or if it has had this amount of live tree canopy cover in the past based on evidence such as stumps and snags (Burrill et al., 2018). (Tree-covered areas in agricultural production such as fruit orchards or in urban parks are not considered forest land, however). As a result, areas of forest land use in some places, including those lacking much or any current forest cover because of a recent disturbance such as a storm or harvest, may be more susceptible to invasion by non-native trees than areas of relatively dense existing forest cover. These limitations may suggest less confidence in the results from Puerto Rico and Hawaii than in the conterminous States, but this does not negate the validity of this approach in these places. The continued collection of FIA data over time, meanwhile, will allow researchers to track changes in the indicators we describe here, to assess whether non-native tree species are successfully reproducing and spreading. This will be particularly useful for the species occurring on only a handful of plots, which may move out of the early stages of establishment into more widespread invasion.
Understanding spatial variation in the effects of invasive plant species is a key to better prioritizing management of these species, taking into account environmental variation and the invasion process (Hulme et al., 2013). Managers, policymakers, and scientists need effective and functional metrics to improve their understanding and management of biological invasions; such metrics would assist in assessing management progress, increase compatibility across borders, and facilitate comparisons among invasions (Wilson et al., 2014). The indicators we described here, based on a systematic national forest inventory, provide information across broad scales that can help guide the allocation of resources for the management of non-native tree species.
Data availability statement
Publicly available datasets were analyzed in this study. This data can be found here: https://apps.fs.usda.gov/fia/datamart/datamart.html.
Author contributions
KP conceived the ideas and wrote most of the manuscript. KP, KR, and QG developed the methodology. All authors participated equally in review and revision. All authors have read and agreed to the published version of the manuscript.
Funding
This work was supported in part through Cost Share Agreement 18-CS-11330110-033 between the United States Department of Agriculture, Forest Service, Southern Research Station, and North Carolina State University.
Acknowledgments
The authors thank the efforts of the Forest Inventory and Analysis (FIA) field crew members who collected the data used in this study. They also appreciate the constructive comments from two reviewers, TB and CH.
Conflict of interest
The authors declare that the research was conducted in the absence of any commercial or financial relationships that could be construed as a potential conflict of interest.
Publisher’s note
All claims expressed in this article are solely those of the authors and do not necessarily represent those of their affiliated organizations, or those of the publisher, the editors and the reviewers. Any product that may be evaluated in this article, or claim that may be made by its manufacturer, is not guaranteed or endorsed by the publisher.
Author’s disclaimer
Any use of trade, firm, or product names does not imply endorsement by the U.S. Government.
Supplementary material
The Supplementary Material for this article can be found online at: https://www.frontiersin.org/articles/10.3389/ffgc.2022.966407/full#supplementary-material
References
Acevedo-Rodriguez, P., and Strong, M. T. (2008). Floristic richness and affinities in the West Indies. Bot. Rev. 74, 5–36. doi: 10.1007/s12229-008-9000-1
Aide, T. M., Zimmerman, J. K., Pascarella, J. B., Rivera, L., and Marcano-Vega, H. (2000). Forest regeneration in a chronosequence of tropical abandoned pastures: Implications for restoration ecology. Restor. Ecol. 8, 328–338. doi: 10.1046/j.1526-100x.2000.80048.x
Bartz, R., and Kowarik, I. (2019). Assessing the environmental impacts of invasive alien plants: A review of assessment approaches. Neobiota 43, 69–99. doi: 10.3897/neobiota.43.30122
Bechtold, W. A., and Patterson, P. L. (2005). The enhanced forest inventory and analysis program: National sampling design and estimation procedures. Asheville, NC: USDA Forest Service, Southern Research Station.
Brandeis, T. J., Helmer, E. H., Marcano-Vega, H., and Lugo, A. E. (2009). Climate shapes the novel plant communities that form after deforestation in Puerto Rico and the US Virgin Islands. For. Ecol. Manage. 258, 1704–1718. doi: 10.1016/j.foreco.2009.07.030
Brandeis, T. J., Helmer, E. H., and Oswalt, S. N. (2007). The status of Puerto Rico’s forests, 2003. Asheville, NC: U.S. Department of Agriculture, Forest Service, Southern Research Station.
Brondizio, E. S., Settele, J., Diaz, S., and Ngo, H. T. (eds) (2019). Global assessment report on biodiversity and ecosystem services of the intergovernmental science-policy platform on biodiversity and ecosystem services. Bonn: IPBES Secretariat.
Brown, K. A., Scatena, F. N., and Gurevitch, J. (2006). Effects of an invasive tree on community structure and diversity in a tropical forest in Puerto Rico. For. Ecol. Manage. 226, 145–152. doi: 10.1016/j.foreco.2006.01.031
Bruce, K. A., Cameron, G. N., Harcombe, P. A., and Jubinsky, G. (1997). Introduction, impact on native habitats, and management of a woody invader, the Chinese tallow tree, Sapium sebiferum (L.) Roxb. Nat. Areas J. 17, 255–260.
Brundu, G., Pauchard, A., Pyšek, P., Pergl, J., Bindewald, A. M., Brunori, A., et al. (2020). Global guidelines for the sustainable use of non-native trees to prevent tree invasions and mitigate their negative impacts. Neobiota 61, 65–116. doi: 10.3897/neobiota.61.58380
Brundu, G., and Richardson, D. M. (2016). Planted forests and invasive alien trees in Europe: A code for managing existing and future plantings to mitigate the risk of negative impacts from invasions. Neobiota 30, 5–47. doi: 10.3897/neobiota.30.7015
Burrill, E. A., Wilson, A. M., Turner, J. A., Pugh, S. A., Menlove, J., Christiansen, G., et al. (2018). The forest inventory and analysis database: Database description and user guide version 8.0 for Phase 2 [Online]. Washington, DC: United States Department of Agriculture, Forest Service.
Butchart, S. H. M., Walpole, M., Collen, B., van Strien, A., Scharlemann, J. P. W., Almond, R. E. A., et al. (2010). Global biodiversity: Indicators of recent declines. Science 328, 1164–1168. doi: 10.1126/science.1187512
Castro-Díez, P., Vaz, A. S., Silva, J. S., Loo, M., Alonso, A., Aponte, C., et al. (2019). Global effects of non-native tree species on multiple ecosystem services. Biol. Rev. 94, 1477–1501. doi: 10.1111/brv.12511
Chinea, J. D., and Helmer, E. H. (2003). Diversity and composition of tropical secondary forests recovering from large-scale clearing: Results from the 1990 inventory in Puerto Rico. For. Ecol. Manage. 180, 227–240. doi: 10.1016/S0378-1127(02)00565-0
Cleland, D. T., Avers, P. E., McNab, W. H., Jensen, M. E., Bailey, R. G., King, T., et al. (1997). “National hierarchical framework of ecological units,” in Ecosystem management: Applications for sustainable forest and wildlife resources, eds M. S. Boyce and A. Haney (New Haven, CT: Yale University Press), 181–200.
Cleland, D. T., Freeouf, J. A., Keys, J. E., Nowacki, G. J., Carpenter, C. A., and McNab, W. H. (2007). Ecological subregions: Sections and subsections for the conterminous United States. Washington, DC: U.S. Department of Agriculture, Forest Service. doi: 10.2737/WO-GTR-76D
Colautti, R. I., and MacIsaac, H. J. (2004). A neutral terminology to define ‘invasive’ species. Divers. Distrib. 10, 135–141. doi: 10.1111/j.1366-9516.2004.00061.x
Cordell, S. (2021). “Regional summaries: Hawaii and U.S.-affiliated Pacific islands,” in Invasive species in forests and rangelands of the United States: A comprehensive science synthesis for the United States forest sector, eds T. M. Poland, T. Patel-Weynand, D. M. Finch, C. Ford Miniat, D. C. Hayes, and V. M. Lopez (Heidelberg: Springer International Publishing), 343–351.
Cordell, S., Ostertag, R., Rowe, B., Sweinhart, L., Vasquez-Radonic, L., Michaud, J., et al. (2009). Evaluating barriers to native seedling establishment in an invaded Hawaiian lowland wet forest. Biol. Conserv. 142, 2997–3004. doi: 10.1016/j.biocon.2009.07.033
Coulston, J. W., Moisen, G. G., Wilson, B. T., Finco, M. V., Cohen, W. B., and Brewer, C. K. (2012). Modeling percent tree canopy cover: A pilot study. Photogramm. Eng. Remote Sens. 78, 715–727. doi: 10.14358/PERS.78.7.715
Dawson, W., Moser, D., van Kleunen, M., Kreft, H., Pergl, J., Pyšek, P., et al. (2017). Global hotspots and correlates of alien species richness across taxonomic groups. Nat. Ecol. Evol. 1:186. doi: 10.1038/s41559-017-0186
Denslow, J. S. (2003). Weeds in paradise: Thoughts on the invasibility of tropical islands. Ann. Mo. Bot. Gard. 90, 119–127. doi: 10.2307/3298531
Dray, F. A., Bennett, B. C., and Center, T. D. (2006). Invasion history of Melaleuca quinquenervia (Cav.) S.T. Blake in Florida. Castanea 71, 210–225. doi: 10.2179/05-27.1
Essl, F., Dawson, W., Kreft, H., Pergl, J., Pyšek, P., van Kleunen, M., et al. (2019). Drivers of the relative richness of naturalized and invasive plant species on Earth. Aob Plants 11:plz051. doi: 10.1093/aobpla/plz051
FAO (2020). Global forest resources assessment 2020: Key findings. Rome: Food and Agriculture Organization (FAO).
Flores, B. M., Staal, A., Jakovac, C. C., Hirota, M., Holmgren, M., and Oliveira, R. S. (2020). Soil erosion as a resilience drain in disturbed tropical forests. Plant Soil 450, 11–25. doi: 10.1007/s11104-019-04097-8
Forero-Montana, J., Marcano-Vega, H., Zimmerman, J. K., and Brandeis, T. J. (2019). Potential of second-growth neotropical forests for forestry: The example of Puerto Rico. For. Trees Livelihoods 28, 126–141. doi: 10.1080/14728028.2019.1579673
Forest Europe (2015). State of Europe’s forests 2015. Madrid: Ministerial Conference on the Protection of Forests in Europe.
Franco, P. A., Weaver, P. L., and Eggen-McIntosh, S. (1997). Forest resources of Puerto Rico, 1990. Asheville, NC: Forest Service Resource Bulletin. doi: 10.2737/SRS-RB-22
Hoover, C. M., and Smith, J. E. (2020). Selecting minimum diameter for forest biomass and carbon estimation: How low should you go? Madison, WI: United States Department of Agriculture, Forest Service, Northern Research Station. doi: 10.2737/NRS-GTR-196
Huenneke, L. F., and Vitousek, P. M. (1990). Seedling and clonal recruitment of the invasive tree Psidium cattleianum: Implications for managment of native Hawaiian forests. Biol. Conserv. 53, 199–211. doi: 10.1016/0006-3207(90)90086-5
Hulme, P. E., Pyšek, P., Jarosik, V., Pergl, J., Schaffner, U., and Vila, M. (2013). Bias and error in understanding plant invasion impacts. Trends Ecol. Evol. 28, 212–218. doi: 10.1016/j.tree.2012.10.010
Iannone, B. V., Oswalt, C. M., Liebhold, A. M., Guo, Q., Potter, K. M., Nunez-Mir, G. C., et al. (2015). Region-specific patterns and drivers of macroscale forest plant invasions. Divers. Distrib. 21, 1181–1192. doi: 10.1111/ddi.12354
Iannone, B. V., Potter, K. M., Guo, Q. F., Liebhold, A. M., Pijanowski, B. C., Oswalt, C. M., et al. (2016). Biological invasion hotspots: A trait-based perspective reveals new sub-continental patterns. Ecography 39, 961–969. doi: 10.1111/ecog.01973
Ibanez, T., Hart, P., Ainsworth, A., Gross, J., and Monello, R. (2019). Factors associated with alien plant richness, cover and composition differ in tropical island forests. Divers. Distrib. 25, 1910–1923. doi: 10.1111/ddi.12989
C. T. Imada (ed.) (2012). Hawaiian native and naturalized vascular plants checklist. Honolulu, HI: Hawaii Biological Survey, Bishop Museum.
C. T. Imada (ed.) (2019). Hawaiian naturalized vascular plants checklist. Honolulu, HI: Hawaii Biological Survey, Bishop Museum.
Iverson, L. R., Rebbeck, J., Peters, M. P., Hutchinson, T., and Fox, T. (2019). Predicting Ailanthus altissima presence across a managed forest landscape in southeast Ohio. For. Ecosyst. 6:41. doi: 10.1186/s40663-019-0198-7
Janowiak, M. K., Nagel, L. M., and Webster, C. R. (2008). Spatial scale and stand structure in northern hardwood forests: Implications for quantifying diameter distributions. For. Sci. 54, 497–506.
Kasson, M. T., Davis, M. D., and Davis, D. D. (2013). The invasive Ailanthus altissima in Pennsylvania: A case study elucidating species introduction, migration, invasion, and growth patterns in the Northeastern U.S. Northeast. Nat. 20, 1–60.
Knapp, L. B., and Canham, C. D. (2000). Invasion of an old-growth forest in New York by Ailanthus altissima: Sapling growth and recruitment in canopy gaps. J. Torrey Bot. Soc. 127, 307–315. doi: 10.2307/3088649
Kumschick, S., Bacher, S., Evans, T., Markova, Z., Pergl, J., Pyšek, P., et al. (2015). Comparing impacts of alien plants and animals in Europe using a standard scoring system. J. Appl. Ecol. 52, 552–561. doi: 10.1111/1365-2664.12427
La Sorte, F. A., and McKinney, M. L. (2007). Compositional changes over space and time along an occurrence-abundance continuum: Anthropogenic homogenization of the North American avifauna. J. Biogeogr. 34, 2159–2167. doi: 10.1111/j.1365-2699.2007.01761.x
Liebhold, A., Brockerhoff, E. G., Kalisz, S., Nunez, M. A., Wardle, D. A., and Wingfield, M. J. (2017). Biological invasions in forest ecosystems. Biol. Invasions 19, 3437–3458. doi: 10.1007/s10530-017-1458-5
Lodge, D. M., Williams, S., MacIsaac, H. J., Hayes, K. R., Leung, B., Reichard, S., et al. (2006). Biological invasions: Recommendations for U.S. policy and management. Ecol. Appl. 16, 2035–2054. doi: 10.1890/1051-0761(2006)016[2035:BIRFUP]2.0.CO;2
Loope, L. L., Hamann, O., and Stone, C. P. (1988). Comparative conservation biology of oceanic archipelagoes: Hawaii and the Galapagos. Bioscience 38, 272–282. doi: 10.2307/1310851
Lugo, A. E., and Helmer, E. (2004). Emerging forests on abandoned land: Puerto Rico’s new forests. For. Ecol. Manage. 190, 145–161. doi: 10.1016/j.foreco.2003.09.012
Lugo, A. E., Smith, J. E., Potter, K. M., Marcano-Vega, H., and Kurtz, C. M. (2022). The contribution of non-native tree species to the structure and composition of forests in the conterminous United States in comparison with tropical islands in the Pacific and Caribbean. Río Piedras, PR: United States Department of Agriculture, Forest Service, International Institute of Tropical Forestry. doi: 10.2737/IITF-GTR-54
Mack, R. N., Simberloff, D., Lonsdale, W. M., Evans, H., Clout, M., and Bazzaz, F. A. (2000). Biotic invasions: Causes, epidemiology, global consequences, and control. Ecol. Appl. 10, 689–710. doi: 10.1890/1051-0761(2000)010[0689:BICEGC]2.0.CO;2
Marcano-Vega, H. (2017). Forests of Puerto Rico, 2014. Asheville, NC: US Department of Agriculture Forest Service, Southern Research Station. doi: 10.2737/FS-RU-121
Martin, P. H., Canham, C. D., and Marks, P. L. (2009). Why forests appear resistant to exotic plant invasions: Intentional introductions, stand dynamics, and the role of shade tolerance. Front. Ecol. Environ. 7, 142–149. doi: 10.1890/070096
Martinuzzi, S., Lugo, A. E., Brandeis, T. J., and Helmer, E. H. (2013). “Case study: Geographic distribution and level of novelty of Puerto Rican forests,” in Novel ecosystems: Intervening in the new ecological world order, 1st Edn, eds R. J. Hobbs, E. S. Higgs, and C. M. Hall (Oxford: Wiley-Blackwell), 81–87. doi: 10.1002/9781118354186.ch9
McKinney, M. L., and La Sorte, F. A. (2007). Invasiveness and homogenization: Synergism of wide dispersal and high local abundance. Glob. Ecol. Biogeogr. 16, 394–400. doi: 10.1111/j.1466-8238.2007.00296.x
Miller, J. H. (1990). “Ailanthus altissima (Mill.) Swingle,” in Silvics of North America: 2. Hardwoods, eds R. M. Burns and B. H. Honkala (Washington, DC: U.S. Department of Agriculture, Forest Service), 461–465.
Montréal Process Liaison Office (2015). The Montréal process criteria and indicators for the conservation and sustainable management of temperate and boreal forests, 5th Edn. Available online at: https://www.montreal-process.org/documents/publications/techreports/MontrealProcessSeptember2015.pdf (accessed June 10, 2022).
Osunkoya, O. O., Froese, J. G., Nicol, S., Perrett, C., Moore, K., Callander, J., et al. (2019). A risk-based inventory of invasive plant species of Queensland, Australia: Regional, ecological and floristic insights. Aust. Ecol. 44, 1123–1138. doi: 10.1111/aec.12776
Oswalt, C. M., Fei, S., Guo, Q., Iannone, B. V., Oswalt, S., Pijanowski, B., et al. (2015). A subcontinental view of forest plant invasions using national inventory data. Neobiota 24, 49–54. doi: 10.3897/neobiota.24.4526
Pejchar, L., and Mooney, H. A. (2009). Invasive species, ecosystem services and human well-being. Trends Ecol. Evol. 24, 497–504. doi: 10.1016/j.tree.2009.03.016
Pereyra, P. J. (2016). Revisiting the use of the invasive species concept: An empirical approach. Aust. Ecol. 41, 519–528. doi: 10.1111/aec.12340
Potter, K. M. (2020). “Introduction,” in Forest health monitoring: National status, trends, and analysis 2019, eds K. M. Potter and B. L. Conkling (Asheville, NC: U.S. Department of Agriculture Forest Service, Southern Research Station), 5–24. doi: 10.2737/SRS-GTR-250
Potter, K. M., and Riitters, K. (2022). A national multi-scale assessment of regeneration deficit as an indicator of potential risk of forest genetic variation loss. Forests 13:19. doi: 10.3390/f13010019
Potter, K. M., and Simth, W. D. (2012). “Large-scale assessment of invasiveness and potential for ecological impact by non-native tree species,” in Forest health monitoring 2009 National Technical Report, eds K. M. Potter and B. L. Conkling (Asheville, NC: U.S. Department of Agriculture, Forest Service, Southern Research Station), 79–94. doi: 10.2737/SRS-GTR-167
Pugh, S. A., Turner, J. A., Burrill, E. A., and David, W. (2018). The forest inventory and analysis database: Population estimation user guide [Online]. Available online at: http://www.fia.fs.fed.us/library/database-documentation (accessed November 16, 2021).
Pyšek, P., Hulme, P. E., Simberloff, D., Bacher, S., Blackburn, T. M., Carlton, J. T., et al. (2020). Scientists’ warning on invasive alien species. Biol. Rev. 95, 1511–1534. doi: 10.1111/brv.12627
Pyšek, P., Jarosik, V., Hulme, P. E., Pergl, J., Hejda, M., Schaffner, U., et al. (2012). A global assessment of invasive plant impacts on resident species, communities and ecosystems: The interaction of impact measures, invading species’ traits and environment. Glob. Change Biol. 18, 1725–1737. doi: 10.1111/j.1365-2486.2011.02636.x
Rejmánek, M., and Richardson, D. M. (2013). Trees and shrubs as invasive alien species-2013 update of the global database. Divers. Distrib. 19, 1093–1094. doi: 10.1111/ddi.12075
Renne, I. J., Gauthreaux, S. A., and Gresham, C. A. (2000). Seed dispersal of the Chinese tallow tree (Sapium sebiferum (L.) Roxb.) by birds in coastal South Carolina. Am. Midl. Nat. 144, 202–215. doi: 10.1674/0003-0031(2000)144[0202:SDOTCT]2.0.CO;2
Richardson, D. M., Pyšek, P., Rejmánek, M., Barbour, M. G., Panetta, F. D., and West, C. J. (2000). Naturalization and invasion of alien plants: Concepts and definitions. Divers. Distrib. 6, 93–107. doi: 10.1046/j.1472-4642.2000.00083.x
Richardson, D. M., and Rejmánek, M. (2011). Trees and shrubs as invasive alien species: A global review. Divers. Distrib. 17, 788–809. doi: 10.1111/j.1472-4642.2011.00782.x
Riitters, K., Potter, K., Iannone, B. V., Oswalt, C., Fei, S. L., and Guo, Q. F. (2017). Landscape correlates of forest plant invasions: A high-resolution analysis across the eastern United States. Divers. Distrib. 24, 274–284. doi: 10.1111/ddi.12680
Riitters, K., Potter, K. M., Iannone, B. V., Oswalt, C., Guo, Q. F., and Fei, S. L. (2018). Exposure of protected and unprotected forest to plant invasions in the eastern United States. Forests 9:12. doi: 10.3390/f9110723
Riitters, K. H., and Potter, K. M. (2019). “The invasibility and invadedness of eastern U.S. forest types,” in Forest health monitoring: National status, trends and analysis 2018, eds K. M. Potter and B. L. Conkling (Asheville, NC: United States Department of Agriculture, Forest Service, Southern Research Station), 115–124.
Rivera, L. W., and Aide, T. M. (1998). Forest recovery in the karst region of Puerto Rico. For. Ecol. Manage. 108, 63–75. doi: 10.1016/S0378-1127(97)00349-6
Rubin, B. D., Manion, P. D., and Faber-Langendoen, D. (2006). Diameter distributions and structural sustainability in forests. For. Ecol. Manage. 222, 427–438. doi: 10.1016/j.foreco.2005.10.049
Shackleton, R. T., Le Maitre, D. C., Pasiecznik, N. M., and Richardson, D. M. (2014). Prosopis: A global assessment of the biogeography, benefits, impacts and management of one of the world’s worst woody invasive plant taxa. AoB Plants 6:plu027. doi: 10.1093/aobpla/plu027
Shackleton, R. T., Le Maitre, D. C., van Wilgen, B. W., and Richardson, D. M. (2017). Towards a national strategy to optimise the management of a widespread invasive tree (Prosopis species; mesquite) in South Africa. Ecosyst. Serv. 27, 242–252. doi: 10.1016/j.ecoser.2016.11.022
Smith, W. B. (2002). Forest inventory and analysis: A national inventory and monitoring program. Environ. Pollut. 116, S233–S242. doi: 10.1016/S0269-7491(01)00255-X
Spencer, P., Nowacki, G., Fleming, M., Brock, T., and Jorgenson, T. (2002). Home is where the habitat is: An ecosystem foundation for wildlife distribution and behavior. Arct. Res. U.S.A. 16, 6–17.
Tinkham, W. T., Mahoney, P. R., Hudak, A. T., Domke, G. M., Falkowski, M. J., Woodall, C. W., et al. (2018). Applications of the United States forest inventory and analysis dataset: A review and future directions. Can. J. For. Res. 48, 1251–1268. doi: 10.1139/cjfr-2018-0196
Tollington, S., Turbe, A., Rabitsch, W., Groombridge, J. J., Scalera, R., Essl, F., et al. (2017). Making the EU legislation on invasive species a conservation success. Conserv. Lett. 10, 112–120. doi: 10.1111/conl.12214
U.S. Department of Agriculture Natural Resource Conservation Service (2022). The plants database [Online]. Greensboro, NC: National Plant Data Team.
Urquia, D., Gutierrez, B., Pozo, G., Pozo, M. J., Espin, A., and Torres, M. D. (2019). Psidium guajava in the Galapagos Islands: Population genetics and history of an invasive species. PLoS One 14:e0203737. doi: 10.1371/journal.pone.0203737
Vila, M., Espinar, J. L., Hejda, M., Hulme, P. E., Jarosik, V., Maron, J. L., et al. (2011). Ecological impacts of invasive alien plants: A meta-analysis of their effects on species, communities and ecosystems. Ecol. Lett. 14, 702–708. doi: 10.1111/j.1461-0248.2011.01628.x
Vogt, J. T., Olatinwo, R., Ulyshen, M. D., Lucardi, R. D., Saenz, D., and McKenney, J. L. (2021). An overview of Triadica sebifera (Chinese Tallowtree) in the Southern United States, emphasizing pollinator impacts and classical biological control. Southeast. Nat. 20, 536–559. doi: 10.1656/058.020.0403
Webster, C. R., Jenkins, M. A., and Jose, S. (2006). Woody invaders and the challenges they pose to forest ecosystems in the eastern United States. J. For. 104, 366–374.
Keywords: invasive species, forest sustainability, forest inventory, forest succession, United States, Hawaii, Puerto Rico
Citation: Potter KM, Riitters KH and Guo Q (2022) Non-native tree regeneration indicates regional and national risks from current invasions. Front. For. Glob. Change 5:966407. doi: 10.3389/ffgc.2022.966407
Received: 10 June 2022; Accepted: 11 August 2022;
Published: 31 August 2022.
Edited by:
Randall Morin, USDA Forest Service, United StatesReviewed by:
Thomas Brandeis, USDA Forest Service, United StatesCynthia Huebner, USDA Forest Service, United States
Copyright © 2022 Potter, Riitters and Guo. This is an open-access article distributed under the terms of the Creative Commons Attribution License (CC BY). The use, distribution or reproduction in other forums is permitted, provided the original author(s) and the copyright owner(s) are credited and that the original publication in this journal is cited, in accordance with accepted academic practice. No use, distribution or reproduction is permitted which does not comply with these terms.
*Correspondence: Kevin M. Potter, kevin.potter@usda.gov