- 1Bulkley Valley Research Centre, Smithers, BC, Canada
- 2Department of Earth and Ocean Sciences, University of Victoria, Victoria, BC, Canada
- 3Veridian Ecological Consulting, Nelson, BC, Canada
British Columbia’s (BC) diverse forest ecosystems include highly productive old growth with global importance for carbon storage and biodiversity. Current estimates of the remaining amount of “big-treed” old growth vary 10-fold, creating uncertainty that challenges provincial attempts to shift management policy toward ecological integrity. This uncertainty arises from using different remotely sensed indicators and definitions of tree size. No ideal indicator exists. We attempt to improve clarity by evaluating the reliability of candidate indicators, calibrating selected indicators to improve consistency, and generating multiple estimates of the amount of big-treed old growth using calibrated indicators. To evaluate reliability, we compared inventory estimates of tree size and site productivity with measured tree size in 1,945 ground plots. To assess the amount of big-treed old growth, we determined an equivalent “big” size threshold for each indicator and calculated the area of old growth above the size threshold. Stand volume, tree density, basal area, and diameter estimates performed poorly; we selected tree height and two measures of site productivity for further analysis. Estimated tree height best indicated the current old growth size, followed by inventory-based site index and finally ecosystem-based site index. The calibrated indicators agreed that very little remaining old growth supports large trees (1.5–3.3% for the biggest trees; 6–13% including medium-sized trees that represent the largest growing trees in lower productivity interior ecosystems). Tree height cannot be used to compare the remaining area of big-treed old growth to the area expected naturally, an important input for ecological risk assessment and conservation planning because height data are lost from the inventory after harvest. The two calibrated site productivity indicators agreed that the amount remaining is less than 30% of the expected historical amount, posing a high risk to biodiversity and resilience. We recommend using estimated height to identify the biggest remaining old-growth stands for regional planning and calibrated inventory-based site index for risk assessment until a detailed ecosystem mapping has been verified to represent old-growth variability. To reduce uncertainty, we suggest that planning groups compare several indicators and analysis approaches, adjusted to ensure equivalence, and use precaution to avoid any unknowingly increasing risks.
1. Introduction
British Columbia’s (BC) diverse forested ecosystems include highly productive old growth that supports massive trees with global importance for carbon storage, resilience, and biodiversity (Lutz et al., 2012; Law et al., 2018; Watson et al., 2018; Buotte et al., 2020). Because there is no agreed-upon definition of big-treed old growth, considerable uncertainty exists about how much remains, with estimates for BC varying from 3 to 30% of total old growth, suggesting either very high or relatively low risk to biodiversity and ecological functions (Brown, 2021; Price et al., 2021). This uncertainty challenges new provincial government attempts to shift old-growth policy toward ecological integrity (Gorley and Merkel, 2020; BC Government, 2021a) and has fueled public and professional divisiveness (Canadian Forest Industries [COFI], 2021; CBC News, 2021; Gelowitz, 2021; Muir, 2021; Osborne and Cecco, 2021). Existing indicators of tree size and ecosystem productivity focus on the growth of young trees and rely on remotely sensed data (Bourgeois et al., 2018); they have not been assessed for reliability in describing tree size in old growth. We fill this gap by using existing field data to evaluate how well remotely sampled indicators represent current old-growth tree size. To reduce uncertainty about how much big-treed old growth remains, we provide multiple lines of evidence; we select several reliable indicators, calibrate them to improve equivalence among indicators, and use each of them to ask how much big-treed old growth remains in BC. While our analyses focus on BC, the challenges inherent in adapting indicators designed for different purposes and opportunities to reduce uncertainty apply universally.
1.1. Big-treed old growth
Old-growth ecosystems are primary forests that have developed a complex structure over centuries (Spies and Franklin, 1996; Franklin et al., 2002). Old-growth character varies considerably with climate and natural disturbance regime: Where wildfire is rare, forests can be much older than the oldest trees, with a heterogeneous structure created by gap dynamics (Lertzman, 1992; Daniels and Gray, 2006), and where wildfire is more frequent, tree age is more uniform, with large trees remaining as legacies from previous disturbances (Burton et al., 2008). Old growth characteristics also vary with site features such as moisture and nutrient availability: higher productivity sites generally support forests with bigger trees, more dead wood, increased heterogeneity, and higher biodiversity (Spetich et al., 1999; Chase, 2010; Hämäläinen et al., 2018). These forests often include large old trees that form keystone ecological structures which are at risk globally (Lindenmayer et al., 2012, 2014; Lutz et al., 2012; Wardell-Johnson et al., 2018). Big-treed ecosystems have exceptionally high value as biodiversity hubs, carbon banks, and climate refugia (Holt and Hadfield, 2007; Ashcroft, 2010; Hauer et al., 2016).
Intact old-growth forests are vanishing worldwide (FRA, 2020) with dire implications for biodiversity, carbon storage, and ecosystem resilience (Foley et al., 2005; Luyssaert et al., 2008; Bradley et al., 2016; Frey et al., 2016; Law et al., 2018; Watson et al., 2018). Big-treed forests face the highest risk because they are targeted by timber harvesting and under-represented in conservation areas that typically avoid areas of high land-use conflict (Venter et al., 2014; Polak et al., 2016; Benner and Lertzman, 2022). Developing appropriate indicators of big-treed old growth is critical to understanding the status of these ecologically important at-risk ecosystems as a basis for improving management (Gorley and Merkel, 2020; Price et al., 2021).
1.2. BC’s old-growth forests
British Columbia has over 50 million hectares of publicly managed forests. Due to a varied climate and complex physiography, a long coastline, steep mountains, and plateaus, these ecosystems vary tremendously. Coastal and inland temperate rainforests, with their cool and moist climate, support forests that have developed complex structures over centuries and millennia, including massive western redcedars, Sitka spruces, and western hemlock trees (Gavin et al., 2003; Coxson et al., 2020). Interior forests include rolling sub-boreal conifer-deciduous mosaics in the north and open grasslands dotted with widely spaced conifers in the south (Biogeoclimatic Ecosystem Classification Program of British Columbia, 2012). Over the entire province, valley bottoms typically grow bigger trees quickly, while slopes support trees that become more stunted with elevation (Biogeoclimatic Ecosystem Classification Program of British Columbia, 2012; Benner and Lertzman, 2022).
About one-fifth of BC’s forests (11 million hectares) are classified as old growth using provincial age-based criteria (BC Government, 2022a). Because harvesting targets high-productivity, big-treed, forests, these remaining areas of old growth are biased towards smaller-statured forests that grow at high elevation, in low-productivity coastal bogs, and in remote northern regions (Price et al., 2021; Benner and Lertzman, 2022). Although large areas of low-productivity old growth remain, some of the most productive big-treed old-growth ecosystems, including Coastal Douglas-fir and inland temperate rainforest are on the verge of extirpation (DellaSala et al., 2021).
1.3. BC’s forest policy
Forest policy in BC over the past 25 years has driven this biased pattern in old-growth retention by ignoring tree size and site productivity in retention targets (BC Government, 1999). Policy developed in the 1990s recommended stratifying retention by fine-scaled ecosystem units that would represent site productivity (BC Government, 1995; Fenger, 1996). However, subsequent assessment of potential impacts on timber supply led to an explicit rejection of this approach, allowing for continued harvest of big-treed forests while meeting retention targets in smaller statured stands with a lower economic value (BC Government, 1999).
Public interest in big-treed old growth has recently resurged as the area of these ecosystems has declined. Demonstrations and over 1,000 arrests in Canada’s biggest act of civil disobedience have garnered the international spotlight (e.g., Lavoie, 2021; Osborne and Cecco, 2021). At the same time, collaborative First Nation, provincial government planning efforts have been initiated to address concerns about the cumulative effects of resource management on indigenous values within First Nations’ territories, including those associated with old growth, (BC Government, 2014, 2021b; Artelle et al., 2019; Yahey v. British Columbia, 2021).
In response to these emerging concerns, the BC government commissioned an independent old-growth review (Gorley and Merkel, 2020). During extensive consultation, the panel heard a consensus about the need for a paradigm shift in old-growth management from timber to ecological integrity as a priority. The provincial government committed to implementing all of the panel’s recommendations (BC Government, 2021a), some of which required assessing indicators and using them to map at-risk big-treed old growth.
1.4. Indicators of big-treed old growth
Indicators available in the BC forest inventory with the potential to assess big-treed old growth include several metrics of tree size (tree height, diameter, volume, basal area, and stem density) and two estimates of stand productivity (“inventory-based” site index and “ecosystem-based” site index). We examine productivity indicators because, while tree size metrics are direct, they cannot answer all questions. For example, comparing the current area of big-treed old growth to the area expected under historic disturbance regimes is used to assess the risk to biodiversity and resilience and is an important input into conservation planning (Lindenmayer et al., 2006; Keane et al., 2009; Seidl et al., 2016). Such analysis requires an indicator, like site productivity, that does not change as stands grow. Estimates of tree size, though potentially ideal for mapping the biggest remaining old growth, cannot answer questions about the historic distribution of big-treed old growth because the tree size data of old growth are lost after harvest, resulting in a sliding baseline (Pauly, 1995).
For most of BC, forest inventory data are derived from remote imagery with limited field sampling (Bourgeois et al., 2018). Although considerable work has examined potential young forest growth rates for input into timber supply projections (BC Government, 2010), very few studies have considered how well inventory data represent the current condition of old-growth forests (except Nussbaum, 1998), posing challenges for old growth management and conservation. We attempt to fill this gap by using existing field data to evaluate how well remotely sampled indicators of tree size and site productivity represent current old growth tree size. We then apply the most reliable indicators to the entire province and ask how much big-treed old growth remains.
2. Materials and methods
We evaluated the reliability of existing indicators of big-treed old growth by comparing inventory data to field samples. Both datasets are publicly available: the provincial forest inventory, based on remote imagery, covers over 50 million hectares of BC’s forest (BC Government, 2020); and field sampling is available for nearly 7,000 ground plots scattered across the province (BC Government, 2020). We evaluated direct indicators of tree size, as well as two indicators of site productivity. We then used three indicators to ask how much big-treed old growth remains in BC.
2.1. Definitions
2.1.1. Old growth age
While functional definitions of old growth, based on multiple stand attributes, are ecologically preferable (Spies and Franklin, 1988; Marcot et al., 1991; Pojar et al., 1992), they are complex and rarely used at large scales; instead, forest managers generally define old growth by simple and somewhat arbitrary forest age criteria (Hilbert and Wiensczyk, 2007). Functional definitions are not available for BC. The provincial methodology defines the seral stage by age criteria that vary based on estimates of historical disturbance regime: in general, wetter ecosystems are considered old above 250 years, and drier ecosystems above 140 years (BC Government, 1995). In BC, large-scale industrial harvest started circa 1900; hence forests older than 140 years can be classified as primary forests. In the evaluation phase, we used 140 years as the minimum age threshold to expand the field plot sample size for wetter ecosystems (BC Government, 2022b); while not all were old growth, this sample represents mature and old growth primary forests. In the assessment phase, we used provincial thresholds for the old seral stage (140 or 250 years), which we considered equivalent to old growth.
2.1.2. Forest type
At the broadest scale, BC’s ecosystems are classified by biogeoclimatic zones that describe coastal and inland temperate rainforests, dry forests of the southern interior, sub-boreal and boreal forests of the central and northern interior, and high-elevation mountain forests (Biogeoclimatic Ecosystem Classification Program of British Columbia, 2012). Within each zone, the predominant tree species vary with site conditions. Because ecosystems and tree growth patterns vary by species, we created “forest types” by dividing BC’s forests based on the biogeoclimatic zone and predominant tree species. Insufficient ground plots exist to stratify by tree species within finer biogeographic units (e.g., by biogeoclimatic variant; Biogeoclimatic Ecosystem Classification Program of British Columbia, 2012).
2.1.3. Indicators
We evaluated several direct indicators of tree size including tree height (defined as the height of healthy co-dominant trees of the predominant species within a plot), tree diameter (defined as quadratic mean diameter measured at 1.3 m above the ground within a plot, a measurement biased toward larger diameters), total above-ground tree volume/ha (based on height, diameter, species composition, and stem taper curves, including live and standing dead trees), basal area/ha (defined as tree cross-sectional area multiplied by stems/ha), and tree density (stems/ha, live and dead; BC Government, 2022c). We excluded trees smaller than 12.5 cm in diameter from all measures to remove the effects of young and/or suppressed trees.
We evaluated two indicators of site productivity: inventory-based site index (from Provincial Vegetation Resources Inventory; BC Government, 2020) and ecosystem-based site index (from the Provincial Site Productivity Layer; BC Government, 2021c). Although both indices estimate tree height in meters at age 50, they record different values for a given old growth forest. Inventory-based site index begins with current forest conditions interpreted from air photos and limited-field validation. From the age and height of the predominant tree species, it then applies growth curves to estimate height at age 50 (Mah and Nigh, 2015). Ecosystem-based site index attempts to reflect underlying ecosystem processes rather than measuring current conditions (BC Government, 2021c). In the portion of the province where site series (the finest division within the biogeoclimatic classification system; Biogeoclimatic Ecosystem Classification Program of British Columbia, 2012) are mapped (based on field assessment or predicted from landscape features), ecosystem-based site index is based on estimates for the predominant tree species within a site series; elsewhere, it uses a biophysical model (Nigh and de Jong, 2015).
2.2. Data processing
2.2.1. Ground plot data
Forest attributes have been measured based on provincial government standards within 6,978 ground plots across BC to support forest inventory and models of stand growth and change (Supplementary Figure 1; BC Government, 2020). Ground samples are managed by federal and provincial governments in collaboration with industry; the provincial government provided us with the most recent records for each, collected between 1995 and 2020. We extracted data for forests with measured age over 140 years and removed ecosystems classified as alpine (N = 10) as well as harvested stands (age not yet updated, but attributes updated; N = 65), leaving 1,945 ground plots of forest >140 years old. We retained naturally disturbed stands, assuming that in these stands, necromass replaced biomass. For analyses comparing productivity indices, we removed over 400 additional plots with missing ecosystem-based site index data and only used forest types with >10 samples, leaving 1,487 plots.
2.2.2. Inventory data
We extracted data from a recent publicly available forest inventory for BC Government (2020). Because inventory is not updated to address recent disturbances, we updated forest age to account for newer harvesting, mountain pine beetles (where percent dead >70%), and wildfire (in moderate and high severity portions of recent fires; Han et al., 2021). We excluded private and federally managed land; Tree Farm Licenses without public data; non-forested ecosystems including alpine, subalpine parkland, grassland, and shrub-dominated ecosystems; very low productivity forests (inventory-based site index of <5 m at 50 years, as used by the province to define the forested land base; BC Government, 2022c); and areas lacking forest age information. We extracted all records for forests classified as older than 140 years.
We used SELES (Fall and Fall, 2001) to overlay and summarize 1-ha raster grids of data layers and exported resultant files for analysis.
2.3. Evaluating indicator reliability
We overlaid the inventory and ground plot datasets and, at each old growth field plot location, compared inventory values to ground measurements (i.e., inventory tree height vs. measured tree height) within forest type. For productivity indices, because definitions are based on 50-year stands and hence cannot be measured in old growth, we examined the relationship between each index and measured tree height.
We explored the data initially by correlating measured and inventory attributes in forest types with more than 30 plots (N = 1,945 plots) using Pearson’s R-value to estimate relationship strength. The smallest group included 43 plots, meaning that any R-value of >0.3 was statistically significant at α = 0.05. Given an interest in indicators with consistency over the province, we looked for patterns across different forest types.
After eliminating attributes with poor correlations between measured and estimated data, explored the remaining attributes more formally in R (R Core Team, 2021), using a hierarchical Bayesian model on all forest types with more than ten plots (Gelman et al., 1995). As well as simplifying interpretation, Bayesian analysis explicitly models the probability of each parameter in the posterior distribution, allowing for richer inference possibilities and more complex models (Hamaker and Klugkist, 2011). Hierarchical models are a common Bayesian approach for nested data, allowing for information pooling and multiple scales of variability within one statistical model. We modeled ground plot data as a linear function of the inventory within two levels of hierarchy: biogeoclimatic zone and leading species within a zone. We chose weakly informative priors based on suggestions from Stan Development Team (2019) and modeled biogeoclimatic zones and tree species level parameters as normally distributed (detailed model specification in Supplementary material).
We specified and fit the model using the STAN modeling language (Gelman et al., 2015), and we used the rStan package (Stan Development Team, 2022) as an interface to R for further analysis. Initial model runs showed divergent MCMC iterations due to excess correlation between levels, so we used a non-centered parameterization for the hierarchical parameters, drawing them from a standard normal distribution initially and then scaling them. This is a common reparameterization for hierarchical models to address convergence issues in MCMC chains (Ogle and Barber, 2020). Posterior predictive checks confirmed model quality (Supplementary Figure 2). We also ran the frequentist equivalent mixed-effects model to validate the results from the Bayesian analysis.
We used the same model setup to test inventory height and diameter against measured values and to test site indices against attributes. For comparing model fit, we used the loo package (Vehtari et al., 2022) which uses Leave-One-Out Cross Validation, to calculate the expected log pointwise predictive densities (ELPD), a measure of how well the posterior distribution models the data (Vehtari et al., 2017). We compared ELPD estimates between models to determine model quality. For most model parameters, we present 90% credible intervals, symmetric around the median value.
Using a Bayesian framework allowed us to investigate estimates separately for each level of the hierarchy and compare full posterior distributions, including model fit. In frequentist statistics, R2 measures data variation explained by the model. Bayesian models do not have a direct R2 value, thus, we implemented a pseudo R2 calculation (Gelman et al., 2019). This statistic can be interpreted similarly but is applicable to the Bayesian paradigm. Furthermore, it provides a posterior R2 distribution instead of a single estimate, allowing for easier testing and interpretation. We calculated the pseudo R2 distributions for each model. To test for significant differences between two R2 posteriors (e.g., x and y, which are quasi-independent distributions), we calculated the probability of x given y for each MCMC iteration and averaged over iterations. That is: , where N is the number of iterations (after the burn-in period).
To assess the reliability of site productivity indices across the entire province, we used the frequentist equivalent mixed-effects model (R package “lme4” with biogeoclimatic zone and leading species as random effects) to compare the two site indices with estimated tree height in the full inventory database (N = 52,265,165 1-ha2 total; 18,221,436 ha >140 years old). Database size and computation power precluded the use of the hierarchical Bayesian model for inventory data. This analysis should give similar median estimates, but it lacks other information testing abilities available with the Bayesian analysis.
To model differences between inventory-based- and ecosystem-based site indices, we used the multilevel equivalent of a paired t-test, a mixed-effects model with the site as the random effect.
2.4. Using selected indicators to assess BC’s big-treed old growth
We used each indicator selected in the evaluation phase (tree height, inventory- based-, and ecosystem-based site index) to investigate two questions critical to BC’s land-use planning across the entire provincial inventory database: (1) what proportion of remaining old-growth forest supports big trees; and (2) how does the remaining amount compare to the expected historic amount. The first question addresses the current status without considering the harvest of previous big-treed old growth; the second attempts to reduce the shifting baseline effect, enabling ecological risk assessment based on the assumption that risk increases as ecosystems move further from their natural state (Keane et al., 2009). We examined forests defined as old growth (aged >250 years in less frequently disturbed and >140 years in more frequently disturbed ecosystems; BC Government, 1995). We used recently updated disturbance return intervals to determine the expected historic old forest area in each zone and productivity class (BC Government, unpublished data). We explored several approaches to investigate the uncertainty associated with using indicators designed for different purposes.
2.4.1. How much of remaining old growth is big-treed?
We used two approaches to address the current status. First, we determined equivalent “big-tree” size thresholds for three selected indicators (tree height, inventory-based site index, and ecosystem-based site index) and estimated the amount of old growth (>140 years old or >250 years old depending on the ecosystem) above the threshold for each. To allow comparisons with previously published estimates, we used an inventory-based site index threshold of >20 m as the baseline (Price et al., 2021). We estimated an equivalent height threshold based on a linear model of inventory-based site index and tree height. We estimated an equivalent ecosystem-based site index threshold by comparing values estimated for the two site indices in each ground plot and applying the difference for each ecosystem, taken from the mixed effects model with the site as the random effect.
Any choice of a threshold for a “big” tree is arbitrary and varies by ecosystem (i.e., a relatively big tree will be smaller in a less productive ecosystem). To address this challenge broadly, we accounted for differences in productivity between coastal and interior ecosystems by using a threshold of >20 m for coastal ecosystems and >15 m for the less productive interior ecosystems and used the estimated equivalent indices for each.
Our second approach focused solely on tree height. We divided the province’s old growth into five equal height classes (full height range defined as 1st–99th percentile within each ecosystem to remove outliers; small, small-medium, medium, medium-big, big). We summed the area in each height class and looked at the area of the biggest group.
2.4.2. How does the remaining amount compare to the expected historic amount?
The expected natural area of old growth provides a benchmark for risk assessment. To assess risk to biodiversity and forest resilience, we compared the area of big-treed old growth in each biogeoclimatic zone with the area expected under historic disturbance regimes (Lindenmayer et al., 2006; Keane et al., 2009; Seidl et al., 2016), assuming a random distribution of disturbance. We used provincial government estimates of disturbance regime to estimate the area of old growth expected for each zone based on a negative exponential disturbance model (BC Government, 1995; BC Government, 2016). We considered biodiversity and resilience to be at high risk in areas with less than 30% of the expected area of old growth (Andrén, 1994; Betts et al., 2007; Price et al., 2009) while recognizing that many species cross habitat thresholds at amounts higher than 30% (e.g., Zuckerberg and Porter, 2010; Gutzwiller et al., 2015).
These analyses, by necessity, focused on the two indices of ecosystem productivity; stand attributes, such as tree height, cannot answer questions about risk because they change as trees grow. Risk assessment requires comparing the current amount of an ecosystem to the expected historical amount and, hence, requires using an indicator that not only represents variability but also remains constant over time. Problematically, after harvest, provincial policy changes productivity index records from inventory-based to ecosystem-based value, meaning that the inventory-based indicator is not constant over time. Because previous values are deleted, it is not possible to check for historic records to determine the historic extent before 2002 (the oldest available version of the provincial inventory database; BC Government, 2020). We compared the 2002 and 2020 databases to determine the magnitude of the post-harvest shift in indicator value. Because shift magnitude varied with site productivity, we recalibrated the site index in harvested stands to previous values by applying a transition matrix based on the proportion of each pre-harvest site index class found in each post-harvest site index class within each biogeoclimatic zone in an attempt to create a more consistent denominator (Supplementary Table 1).
Using similar methods for current state analyses, we calibrated the ecosystem-based site index based on analyses of ground plot data to attempt to correct for the difference between the two indices and accounted for differences in coastal and interior ecosystems by using an inventory-based site index >20 m for coastal ecosystems and >15 m for interior ecosystems.
3. Results
3.1. Evaluating indicator reliability
3.1.1. Estimated vs. measured tree-size indicators
Estimated tree height in BC’s provincial forest inventory correlated moderately well with the height measured in 1,945 ground plots of forest >140 years old. In all 13 forest types (predominant species within the biogeoclimatic zone) with sufficient data (N > 30 ground plots), correlations met the threshold for at least a weak to moderate statistically significant relationship (R > 0.3; P < 0.05); for six groups, correlations surpassed R > 0.5, signifying a moderate to a strong relationship (Supplementary Table 2).
Correlations between measured and estimated values for remaining indicators were generally weaker and varied by forest type. For some forest types, height was the only correlation reaching the threshold of R > 0.3, while in others, measured and estimated diameter (7 of 13 forest types), measured and estimated total volume (5 of 13 forest types), and measured and estimated basal area (7 of 13 forest types) also correlated at this level. Measured stem density correlated very poorly with inventory estimates (meeting the R > 0.3 thresholds in only 2 of 13 forest types). This poor relationship decreased confidence in volume and basal area comparisons because these estimates are related in part to stem density. We selected tree height and diameter for further evaluation.
Inventory height predicted measured height better than inventory diameter predicted measured diameter [height: slope = 0.51 (0.45–0.56); R2 = 0.51 (0.48–0.53); diameter: slope = 0.39 (0.31–0.49); R2 = 0.41 (0.38–0.44); median (90% credible interval); ELPD difference = −853 ± 64; hierarchical Bayesian assessment of forest types with N > 10 ground plots; the difference between out-of-sample predictive accuracy]. Adding diameter to a model that included height did not improve, and slightly and non-significantly worsened, predictive power overall (ELPD difference = −0.7 ± 2.3; the difference between out-of-sample predictive accuracy), and slightly and non-significantly improved the model for western redcedar and Douglas-fir within the Coastal Western Hemlock zone. Given the lack of model improvement with diameter, we selected height as the most reliable indicator. The relationship between measured and inventory tree height varied somewhat amongst forest types, from a median slope of 0.37 in yellow-cedar-leading stands in the Coastal Western Hemlock biogeoclimatic zone to a median of 0.61 in amabilis-fir-leading stands in the same zone. The overall median slope of 0.52 reflects in part an overestimate of the height of short trees and an underestimate of tall trees.
3.1.2. Site productivity vs. measured tree height
Across all forest types, the inventory-based site index was significantly more strongly related to measured tree height than the ecosystem-based site index, with a steeper slope and better model fit [inventory-based site index: slope = 0.73 (0.46–1.07); R2 = 0.44 (0.41–0.47); ecosystem-based site index: slope = 0.55 (0.35–0.86); R2 = 0.40 (0.36–0.42); median and 90% credible intervals with N > 10 ground plots; significance test for difference in R2 P = 0.03; predictive accuracy: ELPD difference = −57.7 ± 17.6].
Patterns varied by forest type (Table 1 and Figure 1). For most forest types, stands with taller trees had higher site index estimates, although data for the Interior Cedar–Hemlock biogeoclimatic zone performed strangely. In this biogeoclimatic zone, all five leading species had median slopes near zero, and included negative slopes within the credible interval, suggesting that stands classified as more productive (by either index) could have shorter trees [Interior Cedar–Hemlock inventory-based site index: slope = 0.11 (−0.12–0.35); median R2 = 0.11; ecosystem-based site index: slope = 0.07 (−0.26–0.48); median R2 = 0.11]. There was no obvious reason for this anomaly. Removing this zone from the model tightened the credible interval for slopes but did not change conclusions.
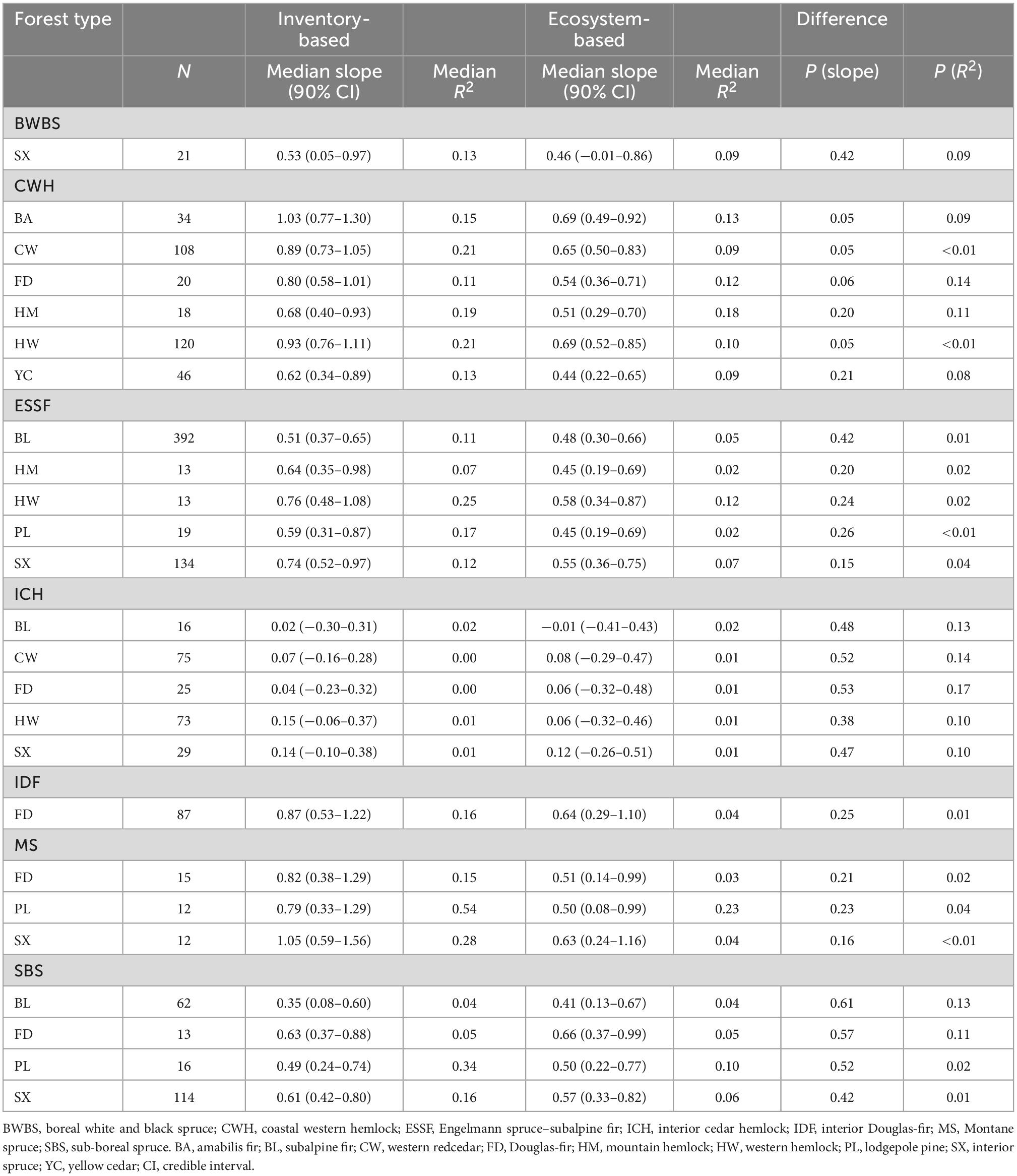
Table 1. Relationship between site index and measured tree height by forest type (predominant species within the biogeoclimatic zone; groups with N > 10 ground plots).
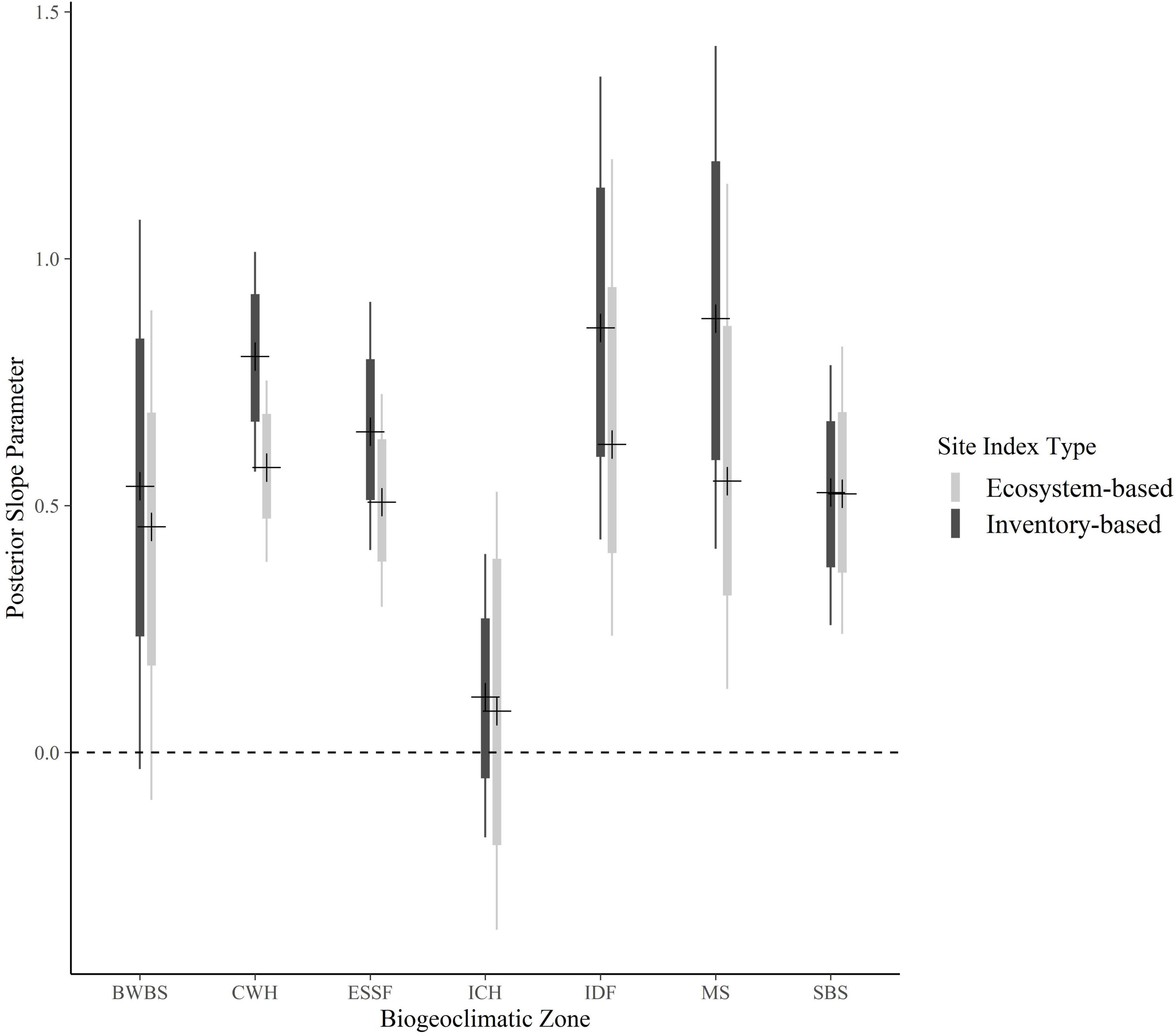
Figure 1. Relationship between site index and measured tree height by biogeoclimatic zone in 1,497 ground plots across BC. Crosses represent the median slope and bars show quartiles and 90% credible intervals. BWBS P = 0.3; CWH P = 0.006; ESSF P = 0.12; ICH P = 0.59; IDF P = 0.15; MS P = 0.11; SBS P = 0.26. Biogeoclimatic zones: BWBS, boreal white and black spruce; CWH, coastal western hemlock; ESSF, Engelmann spruce–subalpine fir; ICH, interior cedar hemlock; IDF, interior Douglas-fir; MS, Montane spruce; SBS, sub-boreal spruce.
The inventory-based site index tended to have stronger relationships with height than the ecosystem-based site index within the biogeoclimatic zone, although only Coastal Western Hemlock forests were significantly different (P = 0.006; Figure 1). Excluding the anomalous Interior Cedar–Hemlock zone, the median slope was higher for inventory-based site index in forest types except for three species in the Sub-Boreal Spruce zone (Table 1).
Comparing the three indicators (inventory tree height, inventory-based site index, and ecosystem-based site index) in the ground plot sites, inventory height was the most reliable indicator of measured tree height, with a significantly better model fit than inventory-based site index (P = 0.002); ecosystem-based site index was the least reliable.
3.1.3. Site productivity vs. inventory tree height
Expanding analyses to inventory data across the entire province, the inventory-based site index was more strongly related to inventory height than was the ecosystem-based site index, with a significantly steeper slope (inventory-based site index: slope = 1.40; ecosystem-based site index: slope = 0.51; P < 0.01; linear mixed model with site index type as predictor) and a higher model fit (inventory-based site index: R2 = 0.53; ecosystem-based site index: R2 = 0.08; Pseudo R2 for generalized linear mixed models). These patterns are consistent across biogeoclimatic zones (inventory-based site index slopes range from 1.22 to 1.55; ecosystem-based site index slopes range from 0.21 to 0.69; Supplementary Table 3).
3.1.4. Inventory-based vs. ecosystem-based site index
In old growth sample plots (>140 years old), inventory-based and ecosystem-based site indices were positively correlated with each other (rp = 0.52), with the ecosystem-based site index values consistently higher than the inventory site index (mean difference = 6.2 ± 0.1 m; SE; t = −58; df = 1 650; P < 0.01; paired t-test). The difference was higher in wetter temperate rainforest and high-elevation zones (Coastal Western Hemlock, Interior Cedar Hemlock, Engelman Spruce—Subalpine Fir) and lower in the dry ecosystems of the southern interior (Interior Douglas-fir; Table 2).
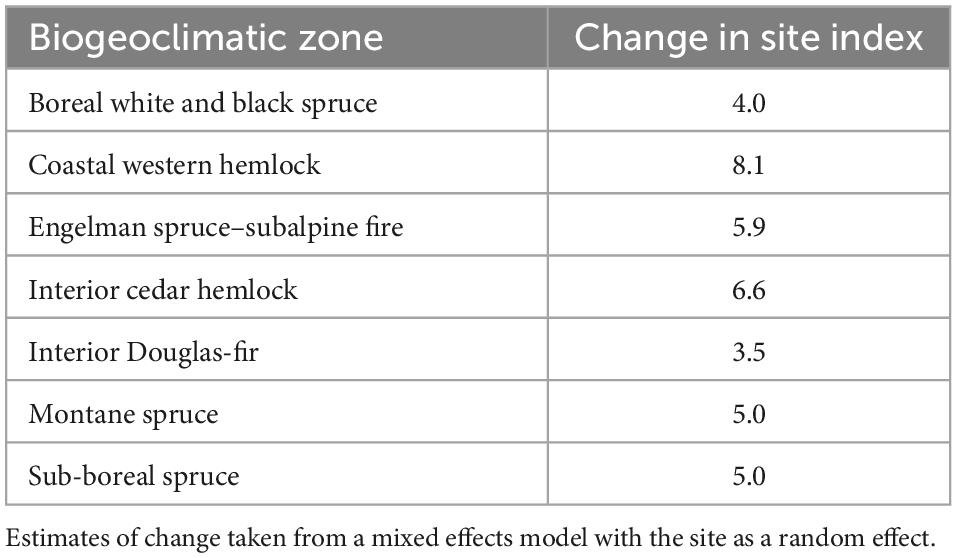
Table 2. Difference between site indices (ecosystem-based site index minus inventory-based site index) by biogeoclimatic zone in ground plots.
Consistent with the pattern in sites with ground plots, the ecosystem-based site index assigned a higher value to given stands than the inventory site index across the province (median 11 vs. 17.5; Figure 2).
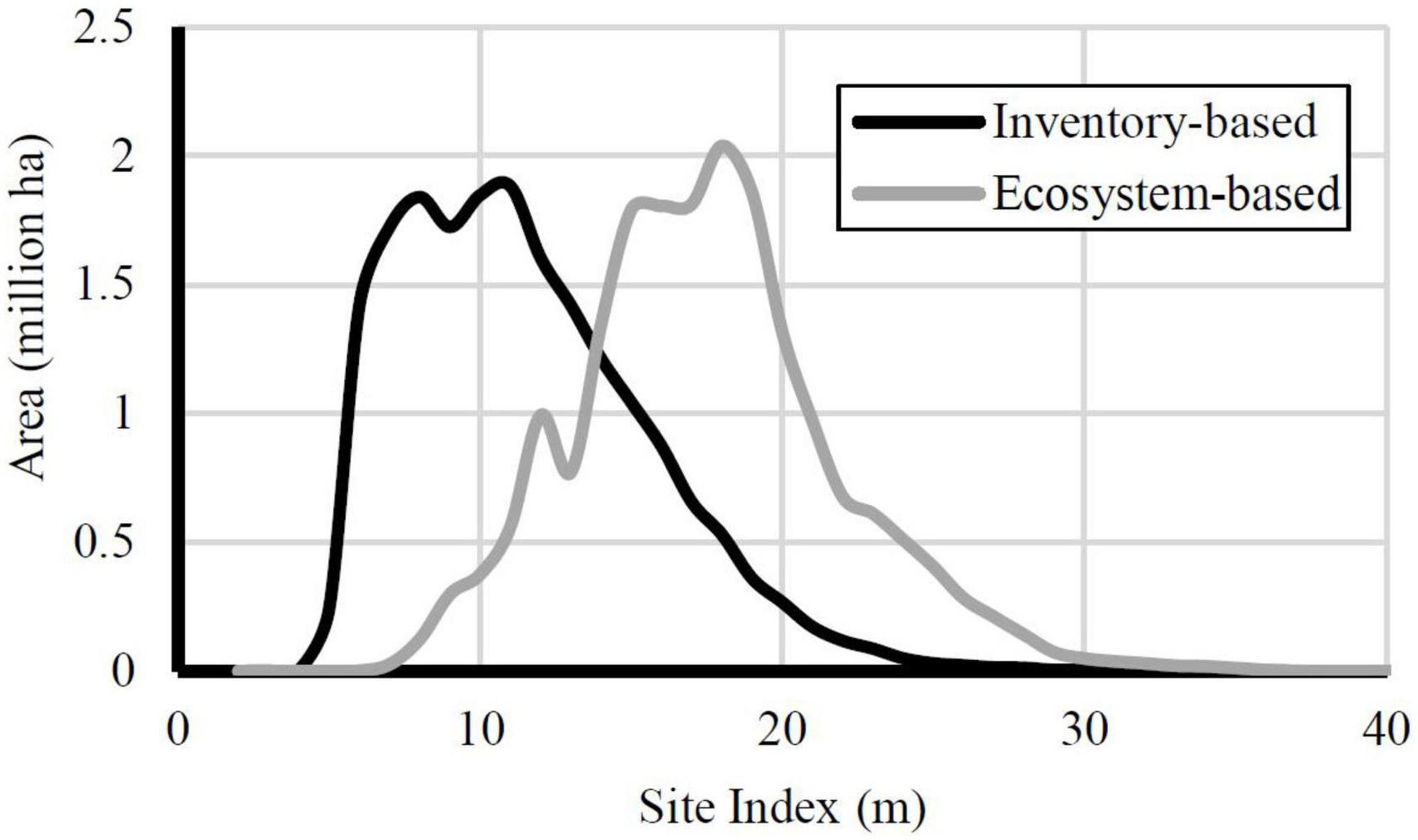
Figure 2. Distribution of area by inventory-based and ecosystem-based site index for BC’s forests >140 years.
3.2. Using selected indicators to assess BC’s big-treed old growth
We selected estimated tree height and both productivity indices to assess the current state of BC’s big-treed old growth from two perspectives, asking how much of BC’s current old growth supports big trees and how many of BC’s potentially big-treed ecosystems are currently old.
3.2.1. How much of remaining old growth is big-treed?
Given the differences among the three indicators, we first attempted to determine equivalent thresholds for each. We used the values in Table 2 to control for the difference between inventory-based and ecosystem-based site indexes by biogeoclimatic zone. Based on a linear model, we estimated an equivalent height threshold of 43 m for an inventory-based site index value of >20 m and 32 m for >15 m.
Given thresholds approximately equivalent to an inventory-based site index of >20 m, each indicator estimated a similar area of big-treed old growth varying from 1.5 to 3.3% (Table 3). Variability was higher when the definition of “big” was expanded to include relatively “mid-sized” trees in the lower productivity ecosystems in the interior of the province, with estimates ranging from 5.5 to 13.2%.
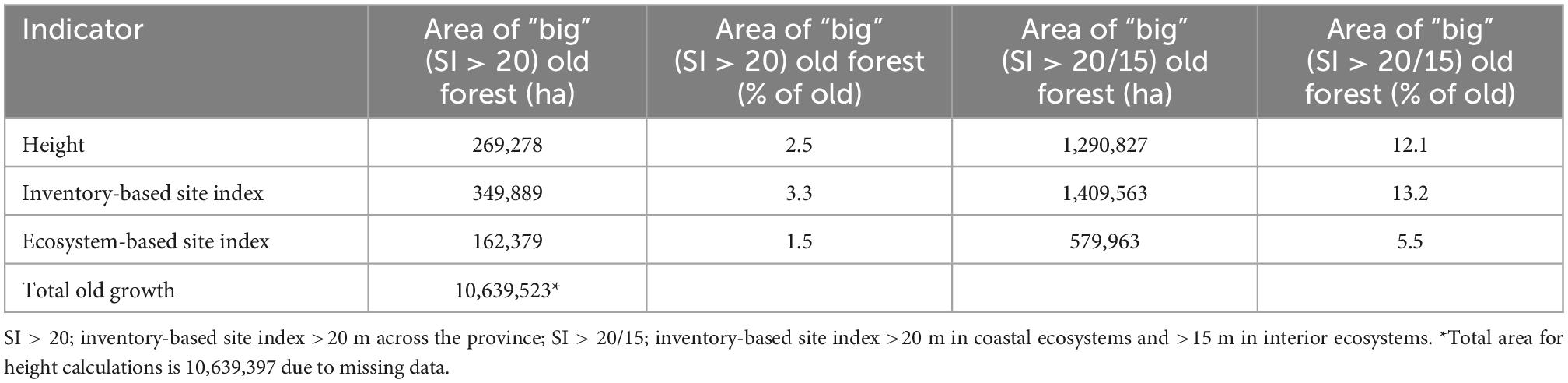
Table 3. Area of “big-treed” old growth in BC using equivalent thresholds for three indicators calibrated to improve consistency.
The second approach, focusing on height as the best indicator of old growth tree size, found that the largest of five equal-height classes, representing the biggest trees in the province (>40 m tall), covered 485,148 ha, representing 4.6% of BC’s old growth.
3.2.2. How does the remaining amount compare to the expected historic amount?
Using an unaltered inventory-based site index as the reference point, 6% of highly productive stands (defined by inventory-based site index >20 m) were classified as old growth. Recalibrating harvested stands to their pre-harvest site index values to improve consistency over time decreased the denominator (i.e., the area of forest with site index defined as >20 m) and estimated that 8% of the highly productive stands were old (Table 4). Using an adjusted ecosystem-based site index, 15% of high-productivity ecosystems were classified as old. When lower productivity forests in BC’s interior were included, the proportion of old forests increased slightly to 9% for the inventory-based site index and decreased to 11% for the ecosystem-based site index (Table 4).
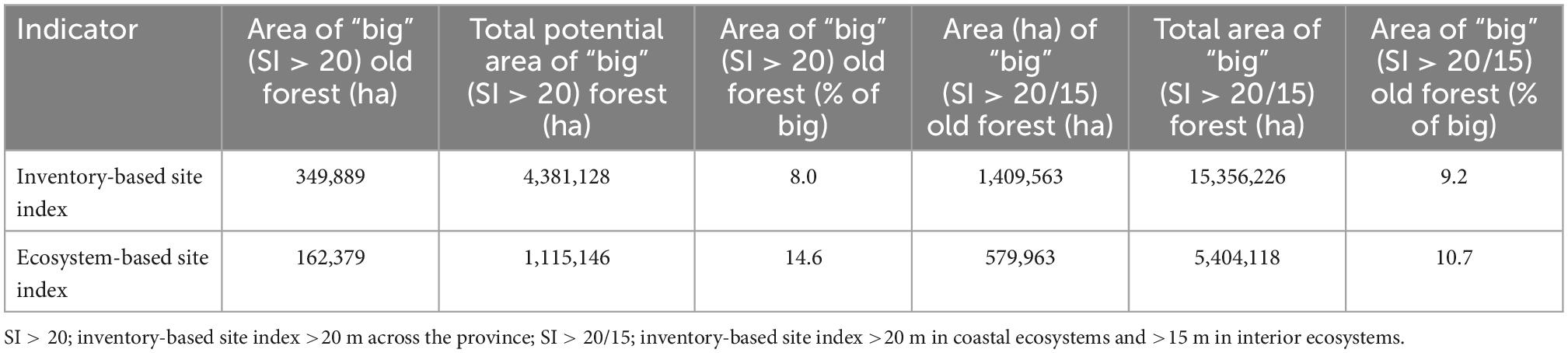
Table 4. The proportion of high-productivity forests classified as old growth estimated using two indicators calibrated to improve consistency.
The two methods agree well on the estimated risk to biodiversity and ecological function for the province as a whole (high risk; <30% of expected) and in most biogeoclimatic zones (Table 5). Analyses suggest that risk is relatively low (>50% of the expected historic amount of highly productive old growth) in the Boreal White and Black Spruce, high (<30% of expected) in the Coastal Western Hemlock, Engelman Spruce—Subalpine Fir, Interior Cedar—Hemlock, and Sub-Boreal Spruce zones, and very high (<10% of expected) in the Coastal Douglas-fir, Interior Douglas-fir, and Ponderosa Pine zones. The methods differ in their assessment of risk in the Mountain Hemlock and Sub-Boreal Pine—Spruce, where the ecosystem-based site index suggests that the risk is relatively low and the inventory-based site index suggests that the risk is high.
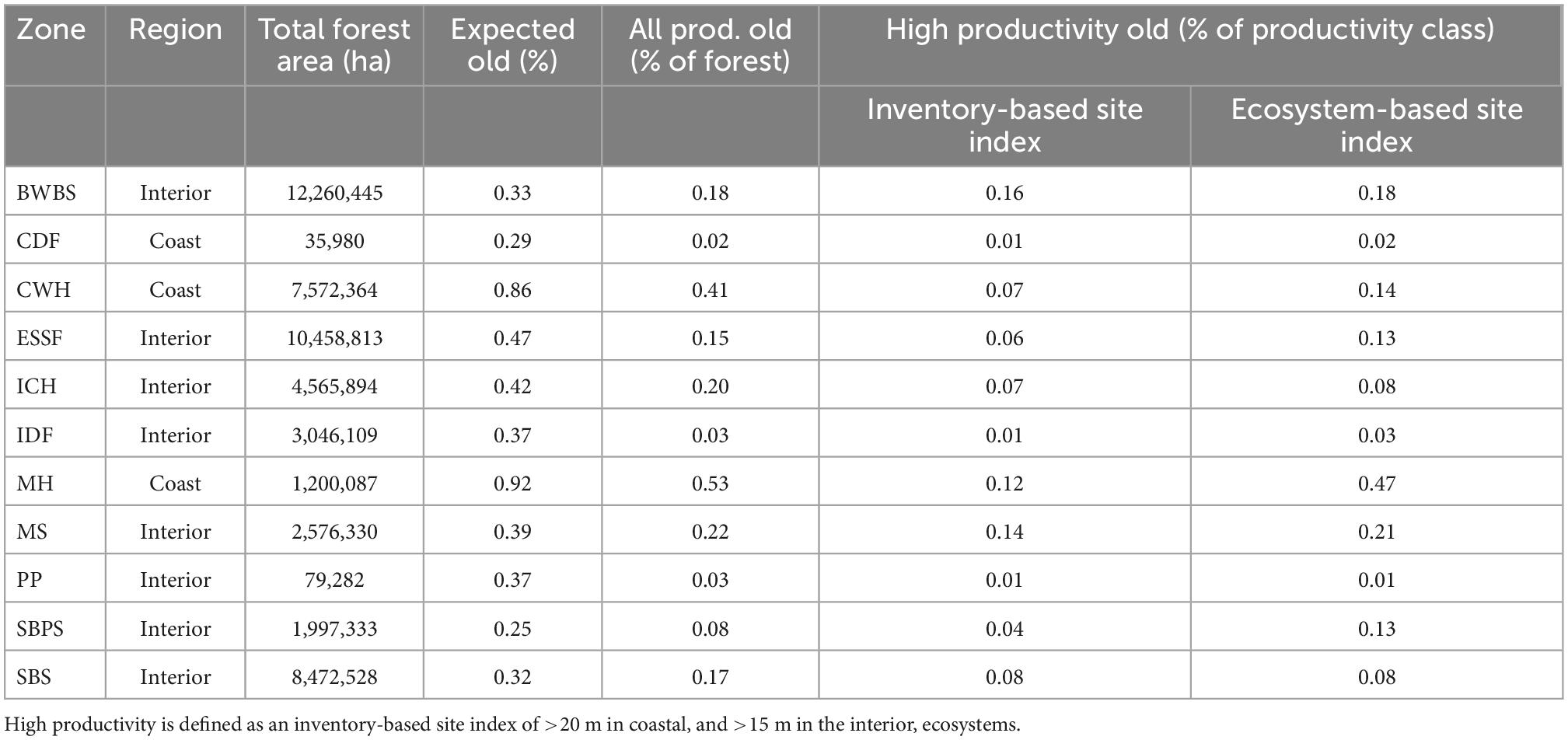
Table 5. Percent of total forest (all productivities) and high productivity forest that is currently old by biogeoclimatic zone based on two indicators calibrated to improve consistency.
4. Discussion
Our evaluation of potential indicators of BC’s big-treed old growth found that inventory tree height was the most reliable remotely sensed indicator of measured tree size in old growth, followed by inventory-based site productivity. No indicator is ideal to assess the amount of big-treed old growth because no indicator was designed for this purpose. Post-harvest data loss and change within the inventory database create shifting baselines, and the lack of fine-scaled ecosystem mapping limits the ability to differentiate among old growth ecosystems. These complexities compelled us to use multiple lines of evidence with indicators calibrated to improve consistency. Our assessment of old growth using calibrated indicators agreed that the amount of big-treed old growth in BC accounts for about 3% of all old growth. The indicators agreed that the amount of big-treed old growth remaining is less than 30% of the expected amount, posing a high risk to biodiversity and resilience.
4.1. Evaluating indicator reliability
Because tree size and site productivity both influence old growth structure and function (Spetich et al., 1999; Chase, 2010; Lindenmayer et al., 2012, 2014; Lutz et al., 2012), we compared existing indicators of tree size and site productivity against measured stand attributes to determine the most appropriate indicators used to assess the state of “big-treed” old forest in BC. We found that estimated tree height best indicates the current old growth tree size, followed by the inventory-based site index and finally the ecosystem-based site index.
Because trees grow differently depending on life history strategy (Hara et al., 1991; Messier et al., 1999; Omari et al., 2021), height does not capture all the elements of a big-treed forest (e.g., some of BC’s massively wide western redcedars are relatively short); hence, we investigated secondary attributes, including diameter and volume metrics, to build a more nuanced model of old forest structure (Burrascano et al., 2013). Available attributes, however, were poorly related to measured values for a given stand, and adding diameter did not improve the relationship between estimated and measured tree size. Finer-scaled analyses (e.g., within the biogeoclimatic variant) may find that diameter improves the model, particularly in temperate rainforests, but we were unable to detect patterns within provincial data.
Inventory-based site index was better related to measured height than was the ecosystem-based site index across the province, particularly in the coastal temperate rainforest. The finding is unsurprising. The inventory-based site index bases conclusions about productivity on current old growth stand height, while the ecosystem-based site index bases productivity estimates on growth rates in young trees (Mah and Nigh, 2003). While early growth is relevant to projections of site productivity, it does not necessarily predict old growth conditions. For example, although western redcedar grows slowly and has a low ecosystem-based site index, it can continue growing for centuries to become massive in the coastal and inland temperate rainforests (Antos et al., 2016); conversely, lodgepole pine grows rapidly and hence has a high ecosystem-based site index (Mah and Nigh, 2015), but this growth rate does not translate into massive old growth stands. A previous study found that younger stands (20–120 years old) also have a poor relationship between the modeled ecosystem-based site index and stand height (Nigh and de Jong, 2015). Where good ecosystem mapping exists, relationships may be improved, although this assumption remains to be tested.
Provincial ground plot data suggest that both inventory-based and ecosystem-based site indices in the Interior Cedar—Hemlock biogeoclimatic zone may be in error. In this zone, stands with taller trees had similar (or sometimes lower) productivity measures compared to stands with shorter trees. Inspection revealed no obvious causes. This anomalous pattern requires further investigation.
4.2. How much big-treed old growth remains in BC?
We explored the potential of the three selected indicators to address two questions important to conservation and land-use planning. First, we investigated the current state, asking how much of BC’s remaining old growth supports big trees. This assessment, useful for identifying stands for conservation or special management, requires an indicator that captures old growth tree size. Second, we assessed ecological risk by comparing the remaining amount of big-treed old growth to the amount expected under historic disturbance regimes (BC Government, 2000; Keane et al., 2009; Seidl et al., 2016). This assessment additionally requires an indicator that is consistent as stands age.
Determining how much remaining old growth supports big trees requires selecting a threshold for “big.” As a baseline for comparing the three indicators at the provincial scale, we used a single threshold of inventory-based site index >20 m, consistent with Price et al. (2021). We determined an equivalent threshold of 43 m for the tree height indicator and rescaled the ecosystem-based site index to improve consistency (i.e., by aligning median productivity values by biogeoclimatic zone). At the provincial scale, the indicators estimate a similar area of old growth above consistent size thresholds, representing 1.5–3.3% of remaining old growth, matching a previous estimate (Price et al., 2021). This result contrasts with a previous estimate that 30% of BC’s old growth is highly productive (Brown, 2021) based on the ecosystem-based site index. Our results suggest that the 10-fold increase in the amount found in that study is primarily due to overlooking the increased values estimated by the ecosystem-based site index relative to the inventory-based site index for a given site. In essence, the approach selects a different tree-size threshold by including trees defined as “medium” by Price et al. (2021).
Appropriate size thresholds vary with the ecosystem and ecological value of interest. For example, planning for carbon storage at the provincial (or larger) scale may focus on identifying the biggest trees in the province, mostly growing in the coastal and inland temperate rainforests, where stored carbon values are among the highest in the world (Keith et al., 2009; DellaSala et al., 2022). Conversely, planning for biodiversity would benefit from selecting thresholds that vary among ecosystems to identify the biggest trees remaining in a region. Our simple stratification into highly productive coastal (inventory-based site index of >20 m) and less productive interior regions (inventory-based site index of >15 m, equivalent to >32 m tall in our analyses) considerably increased the estimate of the productive proportion of remaining old growth (6–13% of remaining old growth depending on the indicator). A more nuanced approach would separate the productive inland temperate rainforest from the less productive interior ecosystems (DellaSala et al., 2022).
Comparing remaining big-treed old growth to the baseline amount expected historically requires an indicator that captures variation in tree size and remains constant over time, thus excluding height. In theory, fine-scaled ecosystem mapping to biogeoclimatic site series (BC Government, 1998; Biogeoclimatic Ecosystem Classification Program of British Columbia, 2012) should be capable of reflecting old growth size and structure and be stable over time; unfortunately, fine-scale field-based mapping was not completed in BC, limiting current potential indicators to estimates of site productivity. Both existing productivity indices face challenges: the ecosystem-based site index remains consistent over time, but represents tree size diversity poorly in old growth; and the inventory-based site index better represents tree size but changes over time. After harvest, provincial policy updates the site index from inventory-based to ecosystem-based values (Mah and Nigh, 2003), adding an average of 6 m to the site index.
We improved consistency over time by updating the inventory-based site index in harvested stands to account for the discrepancy by using a transition matrix based on a comparison of the 2002 and 2020 inventory. This approach led to an estimate that 8% of highly productive stands (with a site index threshold of >20 m) are currently old. Using uncalibrated data, we estimate that 6% of highly productive stands are old, 2% lower than Price et al. (2021), due to recent harvesting and updated inventory. Calibrated ecosystem-based site index estimates that 15% of highly productive stands are old. The indices agree that ecological risk in BC, expressed as the amount remaining as a proportion of the expected amount, is high (<30% of the expected amount of 57%). At a finer scale, the indices agree on risk levels for most biogeoclimatic zones. Unfortunately, the two zones where the indices reach different conclusions about risk in big-treed old growth, Mountain Hemlock and Sub-Boreal Pine Spruce, had insufficient data for analysis in the ground plot sample, challenging further investigation.
4.3. Reducing uncertainty
No ideal indicator exists for big-treed old growth in British Columbia, leading to highly publicized uncertainty about how much remains, with estimates ranging from 3 to 30% (Brown, 2021; Price et al., 2021). Formal strategies exist for making management decisions under uncertainty (Possingham et al., 2001; Burgman, 2005; Marcot, 2020). At the broadest conceptual level, strategies that apply to assessing old growth include increasing precaution, using multiple lines of evidence, and decreasing uncertainty via monitoring and increased ground-truthing (Walters, 1986; Burgman, 2005). Our analyses compare multiple approaches, with indicators calibrated to improve equivalence.
The provincial scale of our analysis increases uncertainty at smaller scales. Analyses based on provincial-scale data covering over 50 million hectares of diverse forest, while designed for strategic purposes, including timber supply analyses and high-level land-use planning (BC Government, 2020), cannot capture BC’s forest diversity. While we attempted to capture coarse variability by analyzing patterns by biogeoclimatic zone and predominant tree species and completed sensitivity analyses of site index thresholds by selecting a lower value for interior than coastal ecosystems, we stress that regional planning will need finer-scale analyses supported by local expertise and ground-truthing.
The complexity of using indicators designed for different purposes to assess the current amount of big-treed old growth highlights opportunities to improve inventory. Retaining historic data after harvesting (either tree height or site productivity) would avoid shifting baselines, well recognized as a challenge in fisheries research but less emphasized in forestry (Pauly, 1995); stable estimates of site attributes are a pre-requisite to risk assessment. The one indicator with a stable estimate over time, ecosystem-based site productivity, performed least well in predicting measured tree size, pointing to the need to know how well remotely sensed data reflect actual conditions. We suggest that keeping data to allow trend analysis is critical.
Complete implementation of BC’s ecosystem classification system (Biogeoclimatic Ecosystem Classification Program of British Columbia, 2012) could provide a stable, reliable indicator. While tree size varies somewhat predictably by biogeoclimatic zone, old growth attributes can vary as much within these classes, driven by differences in soil moisture and nutrient availability (Meidinger and Pojar, 1991; MacKinnon et al., 1992), captured by biogeoclimatic site series. For example, within the coastal temperate rainforest, adjacent forest ecosystems can vary from highly productive valley-bottom gallery forests with massive Sitka spruce to bog forests dominated by tiny shore pine. Biogeoclimatic site series are, potentially, sufficiently fine units to capture big-treed forests but are not mapped for most of the province. Where detailed ecosystem mapping exists, formal analyses have not yet investigated whether this potential for reliability is achieved. The assessment suggests that challenges remain: in the Great Bear Rainforest, circum-music site series are sometimes grouped, meaning that variation within a stratum can be high enough to allow preferential harvest of the biggest stands (Holt and Daust, unpublished data). We recommend that future study evaluates the ability of detailed ecosystem mapping to improve the assessment of big-treed old growth.
Recent provincial efforts are building LiDAR data inventory, potentially improving the stratification of old growth (Mora et al., 2013; BC Government, 2022d). LiDAR is generally linearly related to inventory tree height (Tompalski et al., 2021) with height variability within inventory polygons (unpublished results). With the ability to identify small patches of big trees, LiDAR may be useful in designing conservation networks as regional planning continues.
4.4. Policy implications
Forest harvest targets big-treed stand (Martin et al., 2020; Price et al., 2021; Benner and Lertzman, 2022). BC is currently updating regional land-use planning without an agreed-upon metric of big-treed old-growth forests. Choosing an indicator is a technical issue if parties share a vision. However, although Gorley and Merkel (2020) heard consensus about the need for an old-growth forest paradigm shift, and although the provincial government has committed to a shift (BC Government, 2021a), there is no shared vision for implementing the shift. In this situation, indicator choice and transparency become crucial.
British Columbia’s policy over the past 30 years demonstrates how legally enshrined pressure to maintain timber supply (Forest Planning and Practices Regulation, 2004), with a cap of 4% timber impact for biodiversity conservation (BC Government, 1999), has led to strategies that meet legal targets, yet miss conservation intent, focusing on implementation while overlooking effectiveness (Failing and Gregory, 2003; Price and Daust, 2009). Misrepresenting the current old growth state by combining forest types is easy: current BC policy combines big-treed and small-treed forests within biogeoclimatic units (BC Government, 1999), obscuring patterns in big-treed old growth (Price et al., 2021). Using appropriate big-treed old growth indicators would improve transparency, which is a prerequisite for a paradigm shift (Gorley and Merkel, 2020).
Our analyses suggest that the selection of a big-treed old growth indicator depends upon the question. To assess the current condition, we suggest that inventory tree size is a reasonable indicator for initial planning, allowing identification of the largest remaining stands. At the provincial scale, maps are available of BC’s biggest-treed stands stratified by biogeoclimatic variant (BC Government, 2022a). We recommend that regional planning efforts improve the initial estimate by assessing and ground-truthing the remaining old growth area in each height class.
Assessing ecological risk is more challenging. Current provincial policy recommends using ecosystem-based rather than inventory-based site index to determine productivity in old growth (Mah and Nigh, 2015; Nigh and de Jong, 2015). In old growth, inventory-based site index typically underestimates site productivity compared with similar harvested stands (Nussbaum, 1998; Mah and Nigh, 2003), likely due to several factors, including different methodology, changed growth rate over time, and damaged tree tops (Stearns-Smith, 2001). The policy is based on studies that focused on improving estimates of absolute site productivity (Nussbaum, 1998) rather than on capturing variation among stands (Mah and Nigh, 2003). Our analyses, focusing on relative tree size in old growth, demonstrate that the ecosystem-based site index performs poorly at discriminating tree size. While it may reflect average growth potential, it cannot be used to divide old growth into size classes, and hence should not be used to estimate the remaining amount of highly productive big-treed old growth. We suggest that until detailed ecosystem mapping has been completed and verified to represent the full diversity of existing old growth stands, inventory-based site index be used for risk assessment, with harvested stands adjusted to account for the post-harvest shift. Until uncertainty about risk is reduced, precaution dictates erring on the side of conservation.
Available indicators, calibrated to improve consistency, agree that BC’s big-treed old growth ecosystems are at high risk. Current management policy will result in further threats from timber harvesting (Price et al., 2021; Benner and Lertzman, 2022). The provincial government has committed to changing its old growth management paradigm (BC Government, 2021a) to address carbon storage, climate adaptation, biodiversity conservation, and reconciliation with indigenous people. Agreed-upon definitions and transparent analyses of remaining big-treed old growth status are prerequisites to success.
Data availability statement
Publicly available datasets were analyzed in this study. This data can be found here: https://catalogue.data.gov.bc.ca.
Author contributions
KP, DD, and RH contributed to the conception and design of the study. KP coordinated analyses and wrote the first draft of the manuscript. DD organized the database and completed spatial analyses. KD designed and performed the statistical analyses and wrote a section of the manuscript. All authors contributed to manuscript revision, read and approved the submitted version.
Acknowledgments
We thank the many unidentified people who collected and managed provincial databases, provincial government staff for providing data and advice, Peter Ott for statistical assistance including verifying Bayesian models, and David Lindenmayer and Peter Quinby for helpful comments.
Conflict of interest
The authors declare that the research was conducted in the absence of any commercial or financial relationships that could be construed as a potential conflict of interest.
Publisher’s note
All claims expressed in this article are solely those of the authors and do not necessarily represent those of their affiliated organizations, or those of the publisher, the editors and the reviewers. Any product that may be evaluated in this article, or claim that may be made by its manufacturer, is not guaranteed or endorsed by the publisher.
Supplementary material
The Supplementary Material for this article can be found online at: https://www.frontiersin.org/articles/10.3389/ffgc.2022.958719/full#supplementary-material
References
Andrén, H. (1994). Effects of habitat fragmentation on birds and mammals with different proportions of suitable habitat: a review. Oikos 71, 355–366. doi: 10.2307/3545823
Antos, J. A., Filipescu, C. N., and Negrave, R. W. (2016). Ecology of western redcedar (Thuja plicata): implications for management of a high-value multiple-use resource. For. Ecol. Manag. 375, 211–222. doi: 10.1016/j.foreco.2016.05.043
Artelle, K. A., Zurba, M., Bhattacharyya, J., Chan, D. E., Brown, K., Housty, J., et al. (2019). Supporting resurgent Indigenous-led governance: a nascent mechanism for just and effective conservation. Biol. Conserv. 240:108284. doi: 10.1016/j.biocon.2019.108284
BC Government (1995). Biodiversity Guidebook. Forest Practices Code of British Columbia. Available online at: https://www.for.gov.bc.ca/hfd/library/documents/bib19715.pdf. (accessed October 7, 2020).
BC Government, (1998). Standard for Terrestrial Ecosystem Mapping in British Columbia. Available online at: https://www2.gov.bc.ca/assets/gov/environment/natural-resource-stewardship/nr-laws-policy/risc/tem_man.pdf (accessed October 24, 2022).
BC Government (1999). Landscape Unit Planning Guide. Forest Practices Code of British Columbia. Available online at: https://www2.gov.bc.ca/assets/gov/farming-natural-resources-and-industry/natural-resource-use/land-water-use/crown-land/land-use-plans-and-objectives/policies-guides/lup_guide.pdf (accessed October 7, 2020).
BC Government (2000). Environmental Risk Assessment (ERA): An Approach for Assessing and Reporting Environmental Conditions. Habitat Branch Technical Bulletin 1. Available online at: https://www.env.gov.bc.ca/wld/documents/era.pdf (accessed October 7, 2022).
BC Government (2014). Environmental Stewardship Initiative. Available online at: https://www2.gov.bc.ca/gov/content/environment/natural-resource-stewardship/consulting-with-first-nations/collaborative-stewardship-bc/environmental-stewardship-initiative (accessed April 6, 2022).
BC Government (2016). Great Bear Rainforest Order. Available from Available online at: https://www2.gov.bc.ca/assets/gov/farming-natural-resources-and-industry/natural-resourceuse/land-water-use/crown-land/land-use-plans-and-objectives/westcoast-region/great-bear-rainforest/gbr_land_use_order.pdf (accessed October 7, 2020).
BC Government (2020). Datasets include VRI 2020: Forest vegetation composite rank 1 layer (R1), VRI – Historical vegetation resource inventory (2002–2019), Provincial site productivity layer, generalized forest cover ownership, fire burn severity - Historical, 2018 same year burn severity, BEC Map (version 12). Available online at: https://catalogue.data.gov.bc.ca/ (accessed April 7, 2022).
BC Government (2010). TASS: Predicting British Columbia’ Future Forests: Forest Science Program, Victoria, B.C. Available online at: www.for.gov.bc.ca/hfd/pubs/Docs/Bro/Bro90.htm (accessed October 24, 2022).
BC Government (2021a). Old Growth Forests. Available online at: https://www2.gov.bc.ca/gov/content/industry/forestry/managing-our-forest-resources/old-growth-forests#approach (accessed April 6, 2022).
BC Government (2021b). B.C. Blueberry River First Nations Reach Agreement on Existing Permits, Restoration Funding. News Release. Available online at: https://news.gov.bc.ca/releases/2021IRR0063-001940 (accessed April 6, 2022).
BC Government (2021c). Provincial Site Productivity Layer. Available online at: https://www2.gov.bc.ca/gov/content/industry/forestry/managing-our-forest-resources/forest-inventory/site-productivity/provincial-site-productivity-layer (accessed April 6, 2022).
BC Government (2022a). Old Growth Information and Analysis. Available online at: https://www2.gov.bc.ca/gov/content/industry/forestry/managing-our-forest-resources/old-growth-forests/information-and-analysis (accessed April 6, 2022).
BC Government (2022b). Old Growth Information and Analysis. Available online at: https://www2.gov.bc.ca/assets/gov/farming-natural-resources-and-industry/forestry/stewardship/old-growth-forests/og_tap_background_and_technical_appendices.pdf (accessed April 6, 2022).
BC Government (2022c). Vegetation Resources Inventory Photo Interpretation Procedures Version 3.8. Available online at: https://www2.gov.bc.ca/gov/content/industry/forestry/managing-our-forest-resources/forest-inventory/forest-cover-inventories/photo-interpretation/standards (accessed November 3, 2022).
BC Government (2022d). LiDAR BC. Available online at: https://www2.gov.bc.ca/gov/content/data/geographic-data-services/lidarbc (accessed May 29, 2022).
Benner, J., and Lertzman, K. (2022). Policy interventions and competing management paradigms shape the long-term distribution of forest harvesting across the landscape. Proc. Natl. Acad. Sci. U.S.A. 119:e2208360119. doi: 10.1073/pnas.2208360119
Betts, M. G., Forbes, G. J., and Diamond, A. W. (2007). Thresholds in songbird occurrence in relation to landscape structure. Conserv. Biol. 21, 1046–1058. doi: 10.1111/j.1523-1739.2007.00723.x
Biogeoclimatic Ecosystem Classification Program of British Columbia (2012). “BECdb: biogeoclimatic ecosystem classification codes and names; version 8 [MSAccess 2007 format],” in British columbia ministry of forests, lands, and natural resource operations, ed. W. H. MacKenzie (Victoria, BC: Biogeoclimatic Ecosystem Classification Program of British Columbia).
Bourgeois, W., Binkley, C., LeMay, V., Moss, I., and Reynolds, N. (2018). British Columbia Forest Inventory Review Panel Technical Background Report. Prepared for the Office of the Chief Forester Division, British Columbia Ministry of Forests, Lands. Natural Resource Operations and Rural Development. Available online at: https://www2.gov.bc.ca/gov/content/industry/forestry/managing-our-forest-resources/forest-inventory (accessed November 3, 2020).
Bradley, C. M., Hanson, C. T., and DellaSala, D. A. (2016). Does increased forest protection correspond to higher fire severity in frequent-fire forests of the western United States? Ecosphere 7:e01492. doi: 10.1002/ecs2.1492
Buotte, P. C., Law, B. E., Ripple, W. J., and Berner, L. T. (2020). Carbon sequestration and biodiversity co-benefits of preserving forests in the western United States. Ecol. Appl. 30:e02039. doi: 10.1002/eap.2039
Burgman, M. (2005). Risks and Decisions for Conservation and Environmental Management. Cambridge, MA: Cambridge University Press.
Burrascano, S., Keeton, W. S., Sabatini, F. M., and Blasi, C. (2013). Commonality and variability in the structural attributes of moist temperate old-growth forests: a global review. For. Ecol. Manag. 291, 458–479.
Burton, P. J., Parisien, M. A., Hicke, J. A., Hall, R. J., and Freeburn, J. T. (2008). Large fires as agents of ecological diversity in the North American boreal forest. Int. J. Wildland Fire 17, 754–767. doi: 10.1071/WF07149
Canadian Forest Industries [COFI] (2021). COFI-Commissioned Study: Productive Old Growth in BC is 30% not 3%. Available online at: https://www.woodbusiness.ca/cofi-commissioned-study-productive-old-growth-in-bc-is-30-not-3
CBC News (2021). How B.C.’s Newest War in the Woods Shows the Complex Web of Environmental Politics. Available online at: https://www.cbc.ca/news/canada/british-columbia/fairy-creek-blockade-election-1.6161196 (accessed April 7, 2022).
Chase, J. M. (2010). Stochastic community assembly causes higher biodiversity in more productive environments. Science 328, 1388–1391. doi: 10.1126/science.1187820
Coxson, D., Goward, T., and Werner, J. R. (2020). “The inland temperate rainforest and interior wetbelt biomes of western North America,” in Encyclopedia of the world’s biomes, eds M. I. Goldstein, and D. A. DellaSala (Amsterdam: Elsevier).
Daniels, L. D., and Gray, R. W. (2006). Disturbance regimes in coastal British Columbia. J. Ecosyst. Manag. 7, 44–56.
DellaSala, D. A., Keith, H., Sheehan, T., Strittholt, J., Mackey, B., Connolly, M., et al. (2022). Estimating carbon stocks and stock changes in interior wetbelt forests of British Columbia. Canada. Ecosphere 13:e4020.
DellaSala, D. A., Strittholt, J. R., Degagne, R., Mackey, B., Werner, J. R., Connolly, M., et al. (2021). Red-listed ecosystem status of interior wetbelt and inland temperate rainforest of British Columbia. Canada. Land 10:775.
Failing, L., and Gregory, R. (2003). Ten common mistakes in designing biodiversity indicators for forest policy. J. Environ. Manag. 68, 121–132. doi: 10.1016/s0301-4797(03)00014-8
Fall, A., and Fall, J. (2001). A domain-specific language for models of landscape dynamics. Ecol. Mode. 141, 1–18.
Fenger, M. (1996). Implementing biodiversity conservation through the British Columbia forest practices code. For. Ecol. Manag. 85, 67–77.
Foley, J. A., DeFries, R., Asner, G. P., Barford, C., Bonan, G., Carpenter, S. R., et al. (2005). Global consequences of land use. Science 309, 570–574.
Forest Planning and Practices Regulation (2004). BC Reg. 14/2004. British Columbia Canada. Available online at: https://www.bclaws.gov.bc.ca/civix/document/id/complete/statreg/14_2004 (accessed May 30, 2022).
FRA (2020). Global Forest Resources Assessment 2020. Available online at: http://www.fao.org/forest-resources-assessment/2020 (accessed 1 September 2020).
Franklin, J. F., Spies, T. A., Van Pelt, R., Carey, A. B., Thornburgh, D. A., Berg, D. R., et al. (2002). Disturbances and structural development of natural forest ecosystems with silvicultural implications, using douglas-fir forests as an example. For. Ecol. Manag. 155, 399–423. doi: 10.1016/S0378-1127(01)00575-8
Frey, S. J., Hadley, A. S., Johnson, S. L., Schulze, M., Jones, J. A., and Betts, M. G. (2016). Spatial models reveal the microclimatic buffering capacity of old-growth forests. Sci. Adv. 2:e1501392. doi: 10.1126/sciadv.1501392
Gavin, D. G., Brubaker, L. B., and Lertzman, K. P. (2003). Holocene fire history of a coastal temperate rain forest based on soil charcoal radiocarbon dates. Ecology 84, 186–201.
Gelman, A., Carlin, J. B., Stern, H. S., and Rubin, D. B. (1995). Bayesian Data Analysis. London: Chapman and Hall.
Gelman, A., Goodrich, B., Gabry, J., and Vehtari, A. (2019). R-squared for bayesian regression models. Am. Stat. 73, 307–309. doi: 10.1080/00031305.2018.1549100
Gelman, A., Lee, D., and Guo, J. (2015). Stan: a probabilistic programming language for bayesian inference and optimization. J. Educ. Behav. Stat. 40, 530–543.
Gelowitz, C. (2021). Let’s take a deep breath about the old growth technical panel. BC For. Profess. 7.
Gorley, A., and Merkel, G. (2020). A New Future for Old Forests: A Strategic Review of How British Columbia Manages for Old Forests Within Its Ancient Ecosystems. Available online at: https://www2.gov.bc.ca/assets/gov/farming-natural-resources-and-industry/forestry/stewardship/old-growth-forests/strategic-review-20200430.pdf (accessed April 7, 2022).
Gutzwiller, K. J., Riffel, S. K., and Flather, C. H. (2015). Avian abundance thresholds, human-altered landscapes, and the challenge of assemblage-level conservation. Landsc. Ecol. 30, 2095–2110. doi: 10.1007/s10980-015-0233-1
Hamaker, E. L., and Klugkist, I. (2011). “Bayesian estimation of multilevel models,” in Handbook of Advanced Multilevel Analysis, eds J. Hox and J. K. Roberts (London: Psychology Press), 137–162.
Hämäläinen, A., Strengbom, J., and Ranius, T. (2018). Conservation value of low-productivity forests measured as the amount and diversity of dead wood and saproxylic beetles. Ecol. Appl. 28, 1011–1019. doi: 10.1111/1365-2664.13509
Han, D., Di, X., Yang, G., Sun, L., and Weng, Y. (2021). Quantifying fire severity: a brief review and recommendations for improvement. Ecosyst. Health Sust. 7:1973346.
Hara, T., Kimura, M., and Kikuzawa, K. (1991). Growth patterns of tree height and stem diameter in populations of Abies veitchii, A. mariesii and Betula ermanii. J. Ecol. 79, 1085–1098.
Hauer, F. R., Locke, H., Dreitz, V. J., Hebblewhite, M., Lowe, W. H., Muhlfeld, C. C., et al. (2016). Gravel-bed river floodplains are the ecological nexus of glaciated mountain landscapes. Sci. Adv. 2:e1600026. doi: 10.1126/sciadv.1600026
Hilbert, J., and Wiensczyk, A. (2007). Old-growth definitions and management: a literature review. J. Ecosyst. Manag. 8, 15–31.
Holt, R., and Hadfield, T. (2007). Key Elements of Biodiversity in BC: Some Examples From Freshwater and Aquatic Realms. Technical Subcommittee Component Report for the Report on the Status of Biodiversity in BC. Available online at: http://www.biodiversitybc.org/ (accessed October 7, 2020).
Keane, R. E., Hessburg, P. F., Landres, P. B., and Swanson, F. J. (2009). The use of historical range and variability (HRV) in landscape management. For. Ecol. Manag. 258, 1025–1037. doi: 10.1016/j.foreco.2009.05.035
Keith, H., Mackey, B. G., and Lindenmayer, D. B. (2009). Re-evaluation of forest biomass carbon stocks and lessons from the world’s most carbon-dense forests. Proc. Natl. Acad. Sci. U.S.A. 106, 11635–11640. doi: 10.1073/pnas.0901970106
Lavoie, J. (2021). Inside the Fight for Old-Growth Forests at British Columbia’s Fairy Creek. Vogue. Available online at: https://www.vogue.com/article/inside-the-fight-for-old-growth-forests-at-fairy-creek-british-columbia (accessed April 6, 2022).
Law, B. E., Hudiburg, T. W., Berner, L. T., Kent, J. J., Buotte, P. C., and Harmon, M. E. (2018). Land use strategies to mitigate climate change in carbon dense temperate forests. Proc. Natl. Acad, Sci. U.S.A. 115, 3663–3668. doi: 10.1073/pnas.1720064115
Lertzman, K. P. (1992). Patterns of gap-phase replacement in a subalpine, old-growth forest. Ecology 73, 657–669.
Lindenmayer, D. B., Franklin, J. F., and Fischer, J. (2006). General management principles and a checklist of strategies to guide forest biodiversity conservation. Biol. Conserv. 131, 433–445.
Lindenmayer, D. B., Laurance, W. F., and Franklin, J. F. (2012). Global decline in large old trees. Science 338, 1305–1306.
Lindenmayer, D. B., Laurance, W. F., Franklin, J. F., Likens, G. E., Banks, S. C., Blanchard, W., et al. (2014). New policies for old trees: averting a global crisis in a keystone ecological structure. Conserv. Lett. 7, 61–69. doi: 10.1111/conl.12013
Lutz, J. A., Larson, A. J., Swanson, M. E., and Freund, J. A. (2012). Ecological importance of large-diameter trees in a temperate mixed-conifer forest. PLoS One 7:e36131. doi: 10.1371/journal.pone.0036131
Luyssaert, S., Schulze, E.-D., Börner, A., Knohl, A., Hessenmöller, D., Law, B. E., et al. (2008). Old-growth forests as global carbon sinks. Nature 455, 213–215. doi: 10.1038/nature07276
MacKinnon, A., Meidinger, D., and Klinka, K. (1992). Use of the biogeoclimatic ecosystem classification system in British Columbia. For. Chron. 68, 100–120.
Mah, S., and Nigh, G. D. (2003). SIBEC Site Index Estimates in Support of Forest Management in British Columbia. BC Technical Report 004. Research Branch, BC Ministry of Forests, Victoria BC. Available online at: www.for.gov.bc.ca/hfd/pubs/Docs/Tr/Tr004.htm (accessed April 7, 2022).
Mah, S., and Nigh, G. D. (2015). Site Index Estimates by Site Series (SIBEC): First and Second Generation-type Approximation Estimates For Tree Species In British Columbia. Available online at: https://www2.gov.bc.ca/assets/gov/environment/plants-animals-andecosystems/ecosystems/sibecbackgrounder_jan2015.pdf (accessed April 7, 2022).
Marcot, B. G. (2020). The Science and Management of Uncertainty: Dealing With Doubt in Natural Resource Management, 1st Edn. Boca Raton, FL: CRC Press.
Marcot, B. G., Holthausen, R. S., Teply, J., and Carrier, W. D. (1991). “Wildlife and vegetation of unmanaged douglas-fir forests,” in General Technical Report PNW-285, eds L. F. Ruggiero, K. B. Aubry, A. B. Carey, and M. H. Huff (Portland, OR: USDA, Forest Service).
Martin, M., Boucher, Y., Fenton, N. J., Marchand, P., and Morin, H. (2020). Forest management has reduced the structural diversity of residual boreal old-growth forest landscapes in Eastern Canada. For. Ecol. Manag. 458:17765.
Meidinger, D., and Pojar, J. (1991). Ecosystems of British Columbia. BC Ministry of Forests, Research Branch. Special Report 6. Available online at: https://www.for.gov.bc.ca/hfd/pubs/docs/srs/Srs06/front.pdf (accessed May 30, 2022).
Messier, C., Doucet, R., Ruel, J. C., Claveau, Y., Kelly, C., and Lechowicz, M. J. (1999). Functional ecology of advance regeneration in relation to light in boreal forests. Can. J. For. Res. 29, 812–823.
Mora, B., Wulder, M. A., Hobart, G. W., White, J. C., Bater, C. W., Gougeon, F. A., et al. (2013). Forest inventory stand height estimates from very high spatial resolution satellite imagery calibrated with lidar plots. Int. J. Remote Sens. 34, 4406–4424.
Muir, S. (2021). Modernization, meet paradigm shift. Paradigm shift, meet chaos. For. Chron. 97, 226–231.
Nigh, G. D., and de Jong, J. (2015). Validating the Site Productivity Layer for British Columbia With Equivalence Testing. Available online at: www.for.gov.bc.ca/hfd/pubs/Docs/Tr/Tr085.htm (accessed April 7, 2022).
Nussbaum, A. F. (1998). Site Index Adjustments for Old-Growth Stands Based on Paired Plots. BC Ministry of Forests. Research Branch. Working Paper 37. Available online at: https://www.for.gov.bc.ca/hfd/pubs/docs/wp/wp37.htm (accessed April 7, 2022).
Ogle, K., and Barber, J. J. (2020). Ensuring identifiability in hierarchical mixed effects Bayesian models. Ecol. Appl. 30, e02159. doi: 10.1002/eap.2159
Omari, K., Kranabetter, J. M., and de Montigny, L. (2021). Productivity of coastal douglas-fir and western redcedar in response to species mixture, planting density, and soil carbon: nitrogen ratio. Can. J. For. Res. 51, 668–674.
Osborne, J., and Cecco, L. (2021). Anti -Logging Protest Becomes Canada’s Biggest Ever Act of Civil Disobedience. The Guardian. Available online at: https://www.theguardian.com/environment/2021/sep/08/canada-logging-protest-vancouver-island (accessed April 6, 2022).
Pauly, D. (1995). Anecdotes and the shifting baseline syndrome of fisheries. Trends Ecol. Evol. 10:430.
Pojar, J., Hamilton, E., Meidinger, D., and Nicholson, A. (1992). “Old growth forests and biological diversity in British Columbia,” in Landscape Approaches to Wildlife and Ecosystem Management. Proceedings of the 2nd Symposium Canadian Society for Landscape Ecology and Management, eds G. B. Ingram and M. R. Moss (Morin Heights, ON: Polyscience Publications).
Polak, T., Watson, J. E. M., Bennett, J. R., Possingham, H. P., Fuller, R. A., and Carwardine, J. (2016). Balancing ecosystem and threatened species representation in protected areas and implications for nations achieving global conservation goals. Conserv. Lett. 9, 438–445. doi: 10.1111/conl.12268
Possingham, H. P., Andelman, S. J., Noon, B. R., Trombulak, S., and Pulliam, H. R. (2001). “Making smart conservation decisions,” in Conservation Biology: Research Priorities for the Next Decade, Vol. 23, eds M. E. Soule and G. H. Orians (Washington, DC: Island Press), 225–244.
Price, K., and Daust, D. (2009). Making monitoring manageable: a framework to guide learning. Can. J. For. Res. 39, 1881–1892.
Price, K., Holt, R. F., and Daust, D. (2021). Conflicting portrayals of remaining old growth: the British Columbia case. Can. J. For. Res. 51, 742–752.
Price, K., Roburn, A., and MacKinnon, A. (2009). Ecosystem-based management in the Great Bear Rainforest. For. Ecol. Manag. 258, 495–503. doi: 10.1016/j.foreco.2008.10.010
R Core Team (2021). R: A Language and Environment for Statistical Computing. Vienna: R Foundation for Statistical Computing.
Seidl, R., Spies, T. A., Peterson, D. L., Stephens, S. L., and Hicke, J. A. (2016). Searching for resilience: addressing the impacts of changing disturbance regimes on forest ecosystem services. J. Appl. Ecol. 53, 120–129. doi: 10.1111/1365-2664.12511
Spetich, M. A., Shifley, S. R., and Parker, G. R. (1999). Regional distribution and dynamics of coarse woody debris in midwestern old-growth forests. For. Sci. 45, 302–313. doi: 10.1093/forestscience/45.2.302
Spies, T. A., and Franklin, J. F. (1988). Old-growth and forest dynamics in douglas-fir region of Western Oregon and Washington. Nat. Areas J. 8, 190–201.
Spies, T. A., and Franklin, J. F. (1996). “The diversity and maintenance of old-growth forests,” in Biodiversity in Managed Landscapes, eds R. C. Szaro and D. W. Johnston (New York, NY: Oxford University Press), 296–314.
Stan Development Team (2019). Stan Modeling Language Users Guide and Reference Manual, Version 2.29. Available online at: https://mc-stan.org (accessed May 15, 2022).
Stan Development Team (2022). RStan: the R interface to Stan. R package version 2.21.5. Available online at: https://mc-stan.org/ (accessed May 4, 2022).
Stearns-Smith, S. (2001). Making sense of site index estimates in British Columbia: a quick look at the big picture. J. Ecosyst. Manag. 1:art6.
Tompalski, P., White, J. C., Coops, N. C., Wulder, M. A., Leboeuf, A., Sinclair, I., et al. (2021). Quantifying the precision of forest stand height and canopy cover estimates derived from air photo interpretation. Forestry 94, 611–629.
Vehtari, A., Gabry, J., Magnusson, M., Yao, Y., Bürkner, P., Paananen, T., et al. (2022). loo: Efficient Leave-One-Out Cross-Validation and WAIC for Bayesian Models. R package version 2.5.1. Available online at: https://mc-stan.org/loo/ (accessed May 15, 2022).
Vehtari, A., Gelman, A., and Gabry, J. (2017). Practical Bayesian model evaluation using leave-one-out cross-validation and WAIC. Stat. Comput. 27, 1413–1432. doi: 10.1007/s11222-016-9696-4
Venter, O., Fuller, R. A., Segan, D. B., Carwardine, J., Brooks, T., Butchart, S. H. M., et al. (2014). Targeting global protected area expansion for imperiled biodiversity. PLoS Biol. 12:e1001891. doi: 10.1371/jour-nal.pbio.1001891
Walters, C. J. (1986). Adaptive Management of Renewable Resources. New York, NY: Macmillan Publishers Ltd.
Wardell-Johnson, G., Wardell-Johnson, A., Schultz, B., Dortch, J., Robinson, T., Collard, L., et al. (2018). The contest for the tall forests of south-western Australia and the discourses of advocates. Pacific Conserv. Biol. 25, 50–71.
Watson, J. E. M., Evans, T., Venter, O., Williams, B., Tulloch, A., Stewart, C., et al. (2018). The exceptional value of intact forest ecosystems. Nat. Ecol. Evol. 2, 599–610. doi: 10.1038/s41559-018-0490-x
Yahey v. British Columbia (2021). BC Supreme Court 1287. Available online at: https://www.bccourts.ca/jdb-txt/sc/21/12/2021BCSC1287.htm (accessed October 7, 2022).
Keywords: old growth, indicator, tree size, productivity, British Columbia, Bayesian analysis
Citation: Price K, Daust D, Daust K and Holt R (2023) Estimating the amount of British Columbia’s “big-treed” old growth: Navigating messy indicators. Front. For. Glob. Change 5:958719. doi: 10.3389/ffgc.2022.958719
Received: 31 May 2022; Accepted: 07 December 2022;
Published: 09 January 2023.
Edited by:
Maxence Martin, Université du Québec en Abitibi-Témiscamingue, CanadaReviewed by:
David Lindenmayer, The Australian National University, AustraliaPeter Quinby, Ancient Forest Exploration & Research, Canada
Copyright © 2023 Price, Daust, Daust and Holt. This is an open-access article distributed under the terms of the Creative Commons Attribution License (CC BY). The use, distribution or reproduction in other forums is permitted, provided the original author(s) and the copyright owner(s) are credited and that the original publication in this journal is cited, in accordance with accepted academic practice. No use, distribution or reproduction is permitted which does not comply with these terms.
*Correspondence: Karen Price, pricedau@telus.net