- 1State Key Laboratory of Subtropical Silviculture, Zhejiang A&F University, Lin'an, China
- 2Key Laboratory of Carbon Cycling in Forest Ecosystems and Carbon Sequestration of Zhejiang Province, Zhejiang A&F University, Lin'an, China
- 3School of Environmental and Resources Science, Zhejiang A&F University, Lin'an, China
- 4Jiyang College of Zhejiang A&F University, Zhuji, China
Newly sprouted culm (culm_new) of Moso bamboo can complete its height growth within 1 or 2 months without growing any leaf. This explosive growth without enough leaf photosynthesis may rely heavily on external carbon input, such as carbon storage in the rhizomes or culms from the nearby mother culms (culm_mother). However, the existing studies have not explored the role of new photosynthesized carbon by the nearby culm_mother and the corresponding water dynamics. Therefore, this study monitored non-structural carbohydrates in the culm_mother and water transfer between the culm_new and their attached culm_mother in a 3-month experiment. Nine pairs of newly sprouted and attached culm_mother were categorized into three groups with three different treatments, that is, (1) control without any treatment, (2) cutting off rhizomes between the culm_new and culm_mother (Treatment I), and (3) removing all leaves of culm_mother based on the Treatment I (Treatment II). The differences between non-structural carbohydrates of the culm_mother in the control and treatment I were defined as transferred carbohydrates transferred outward from culm_mother. The difference between treatments I and II was defined as newly photosynthesized carbohydrates of the culm_mother. Before the culm_new leafing, there were significant transferred carbohydrates and newly photosynthesized carbohydrates from the culm_mother. In contrast, the carbon transfer became fewer after leafing. At the same time, the sap flow direction in the rhizomes indicated water flows from culm_new to culm_mother during the day and vice versa at night of the culm_new at the pre-leafing stage. These findings may suggest that the explosive growth of the culm_new relies on both previous carbon storage and newly photosynthesized carbohydrates, and the carbon transfer may be coordinated with water transportation between the culms. Further study may pay attention to the potential support from the overall network of the bamboo stand or groves.
Introduction
In the growing season, newly sprouted bamboo culms can maintain a high growth rate of 10 to 80 cm per day and can achieve a height of 12 to 15 m in a short period of about 4 weeks (Zhou, 2006; Zhou et al., 2010; Liese and Köhl, 2015). Under suitable conditions, some species can grow as fast as 120 cm day−1 (Durst et al., 1994). This rapid growth is often referred to as “explosive growth” (Song et al., 2016). Explosive growth is unique in that the newly sprouted culms produce no leaves and only a few new roots before completing the height development. Leaves are the most essential photosynthetic and transpiration organs of plants. Without leaves, newly sprouted culms cannot provide themselves with the carbon needed for growth during the explosive growth period.
In terms of the carbon source of newly sprouted culms, existing studies have mainly focused on two aspects: the support of carbon storage in the underground rhizome system (Li et al., 1998) and the mother culms (Li et al., 1998; Song et al., 2016). These studies were based primarily on the physiological integration found in an increasing number of related plants (Lau and Young, 1988; Chapman et al., 1992; Dong, 1996; Hutchings and Wijesinghe, 1997; Adonsou et al., 2016). Plants with connected roots or rhizomes have the possibility to share resources directly. Resource exchange among connected individuals is referred to as “physiological integration” (Dong, 1996). As an underground passway connecting the aboveground culms, rhizomes are thought to have the dual functions of storing and transporting nutrients simultaneously (Zhou and Fu, 2004; Huang et al., 2020). Newly sprouted culms without leaves may rely more on physiological integration to obtain resources, especially carbohydrates, from other established culms (Song et al., 2016). Therefore, external carbon input should be the primary carbon source of newly sprouted culms during the explosive growth period. It is generally believed that this kind of source may include the rhizomes and mother culms directly connected with the newly sprouted culms (Li et al., 1998; Song et al., 2016), and probably (but not yet proven) other culms in the same bamboo forests or bamboo groves.
Except for carbon sources of the newly sprouted culms, the mechanism that drives carbon into the newly sprouted culms was unclear and might also obey the Münch theory (Münch, 1927). The theory supposed that carbohydrates produced in leaves were driven from organs of the carbon sources to the carbon sinks by hydrostatic pressure. Such hydrostatic pressure is produced by a high concentration of carbohydrates in leaf phloem and the process of absorbing water from nearby xylem. The theory is more logistic in explaining carbohydrate movement from leaf downward to other organs than from mother bamboo to newly sprouted bamboos. Because the pathway of such movement is in U-shape and has to overcome gravity, the time for transporting carbohydrates is tricky for newly sprouted bamboos. Our recent studies have observed water transfer from mother culms to fast-growing newly sprouted culms using deuterium isotope tracers and thermal dissipation probe (Mei, 2017; Fang et al., 2019; Mei et al., 2019). At the same time, by monitoring the sap flow on the rhizomes, we found that the mother culms may provide 48% of daily water use of the newly sprouted culms which have not yet grown leaves and the newly sprouted culms use water at night, mainly before leafing (Fang et al., 2019). Furthermore, water transfer between mother and newly sprouted culms was observed in a bamboo forest treated with a manipulated summer drought (Tong et al., 2021). The thermal dissipation probe may partly explain the carbohydrate movement in newly sprouted culms because such a method cannot distinguish the solution movement in the xylem and phloem. Due to lack of leaves and hence transpiration of newly sprouted culms before leafing, water flux reflected by thermal dissipation probes might be more related to the movement of carbohydrates solution in the phloem. Therefore, former observations of water transfer between mother and newly sprouted culms may have essential roles in coordinating carbon transport during specific periods (e.g., rapid growth and interference pressure).
This study tested the following hypotheses relating to Moso bamboo's carbon and water dynamics during the explosive growth period. (1) The carbon sources of newly sprouted culms are not only limited to the stem-stored carbon but also newly photosynthetic fixed carbon of mother culms; and (2) Carbon input may have a synergistic relationship with water transfer between the mother and newly sprouted culms.
Materials and methods
Study site and treatment on experimental sample culms
The study site locates in the Pingshan experimental base of the Zhejiang A&F University, Lin an, Zhejiang Province of China. Zhejiang Province is located in southeast China and belongs to a typical subtropical monsoon climate zone with an average temperature of 17.6 ± 0.4°C and an average annual rainfall of 1,579.7 ± 263.5 mm from 2008 to 2017 (data obtained from the National Meteorological Information Center; http://data.cma.cn/site/index.html) (Mei et al., 2020; Tong et al., 2021).
At the beginning of the growing season of Moso bamboo in 2021 (bamboo shoots were observed emerging from the ground around April 1), bamboo shoots (being referred to as newly sprouted culms in this study) with about 20 to 50 cm above the ground were selected as experimental objects (Table 1). The selected newly sprouted culms should have healthy (thick and plump) appearances with bright green tips that have guttation in the morning. Additionally, there were no degrading trends. We dug around them after selecting newly sprouted culms and found the nearest mother culms following the rhizomes. Mother culms should have no apparent diseases, insect pests, surface damage, or complete crown. After the mother culm was identified, we cut off rhizomes around them but only kept those connected to the selected newly sprouted culms. In this way, each pair of mother and newly sprouted culms were isolated, eliminating carbon and water interference from other standing culms in the system as much as possible. After determining the mother and newly sprouted culms, the exposed rhizomes were backfilled with soil in time to reduce the damage.
With the above criteria, nine pairs of newly sprouted and attached mother culms were categorized into three groups (three pairs per group) with three different treatments (Figure 1), that is, (1) control without any treatment, (2) cutting off rhizomes between the newly sprouted and mother culms (Treatment I), and (3) removing all leaves of mother culms based on the Treatment I (Treatment II). The difference between non-structural carbohydrates of the mother culms in the control and treatment I was defined as the outward transferred carbohydrates of mother culms (NSC_transferred). The difference between treatments I and II was defined as the newly produced carbohydrates of the mother culms (NSC_leaf_produced).
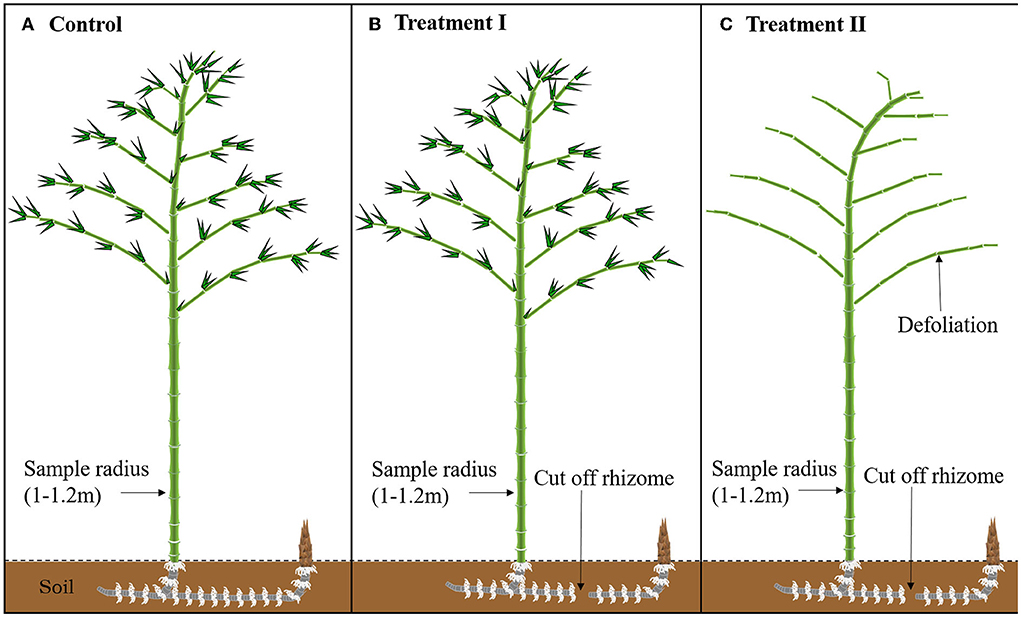
Figure 1. Experimental design of the three treatments. (A) The control without any treatment (Control), (B) the cutting off rhizomes between the newly sprouted and mother culms (Treatment I), and (C) the removing all leaves of mother culms based on the Treatment I (Treatment II).
Detection of the non-structural carbohydrate content in the stem of mother culms
During the explosive growth period, stems of mother culms were sampled six times on 19 April, 26 April, 10 May, 25 May, 23 June, and 11 July 2021. At 7:00 h, 10 g of stems were drilled from mother culms at 1 to 1.2 m height, wrapped with aluminum foil paper, kept in self-sealing bags, and immediately put into a liquid nitrogen tank for storage. After sampling, the samples were taken back to the laboratory and stored in the freezer at minus 40°C before further treatment. Before testing non-structural carbohydrates, the samples were first dried for half an hour in a 105°C oven to inactivate enzymes and then dried to a constant weight at 65°C. Next, the dried samples were then ground by a grinder, passed through a 100-mesh screen, and sealed into a self-sealing bag for testing. Non-structural carbohydrate content (starch and solute sugars) was tested with an improved anthrone colorimetric method (Jan and Roel, 1993; Yu et al., 2011; Song et al., 2016; Li et al., 2022). A dried sample with a weight of 0.100 g was transferred to a 15-ml centrifuge tube with 10 ml distilled water. Then, the centrifuge tube sat in a 70°C water bath for 30 min. After cooling, samples were centrifuged at 3,000 r for 7 min, and the supernatant solution was collected. The processes of centrifugation and collection of supernatant solution were repeated twice and combined into a 50-ml flask for constant volume. After that, 1 ml of the supernatant solution was collected, transferred into a colorimetric tube, and mixed with 5 ml anthrone reagent (0.5 g anthrone dissolved in 500 ml of 80% sulfuric acid). The solution was then sat in boiling water for 15 min and cooling to room temperature afterward. Finally, the soluble sugar contents were determined in a UV-visible spectrophotometer at 620 nm. The residue left in the tube was used to determine the starch content. About 3 ml of distilled water was added to each sample tube, boiled for 15 min, and then cooled down. After cooling, 2 ml of 74% HClO4 were added to the tube and mixed eventually with the shaking table. The supernatant was collected twice and combined into a 50-ml flask for constant volume. Starch content was determined in a UV-visible spectrophotometer at 620 nm.
Sap flow monitoring of bamboo culms and rhizomes
This study monitored sap flow with an improved self-build thermal dissipation probe (TDP+) based on the classical Granier type (TDP; Granier, 1985). The classical TDP includes a pair of heating and reference sensors, which are separately installed on stems to measure sap flow according to the temperature differences between the two sensors. However, TDP is unable to detect the direction of sap flow. Our proposed solution to this problem is to add another two reference sensors around the heating sensor (Fang et al., 2019; Tong et al., 2021). When installing TDP+ on the stems/rhizomes, the two new reference sensors were installed in the upstream and downstream positions 5 mm from the heating sensor, respectively. As the heat will be brought by sap flow downstream, the temperature at the downstream position should be higher than that of the upstream position. Therefore, by comparing the temperatures measured by the two additional reference sensors, we can determine the sap flow directions in the stems or rhizomes of Moso bamboo.
The 10-mm-length TDP+ were installed on 1.2 m height stems of both mother and newly sprouted culms, while 5-mm-length TDP+ were on rhizomes between the mother and newly sprouted culms. The heating probes were provided with a constant current of 120 mA, generating about 0.1 and 0.05 W of heat for a 10- and 5-mm probe, respectively. The probes were covered with PVC plastic boxes and reflective and rainproof film to prevent external damage, solar radiation, and rain. They were also sealed with waterproof tape and rubber straps on the upper end of the stem to avoid stem flow on rainy days. The probe signals were collected by data loggers (CR1000 & AM32/64, Campbell, USA), which scanned the signals every 30 s and averaged and recorded them every 10 min.
Judging sap flow direction based on TDP+ Probe
In the field, we simultaneously recorded the temperature difference between the heating sensor and each of the two additional reference sensors (ΔTup and ΔTdown). Ideally, when the sap flow rate is zero, ΔTup = ΔTdown; when the sap flow is from the upstream to the downstream, ΔTup < ΔTdown, and on the contrary, ΔTup > ΔTdown. However, due to the potential displacement of the installation position, the difference between the absolute values of ΔTup and ΔTdown may not fully reflect the direction of the flow. Therefore, to eliminate this potential error, we first standardized ΔTup and ΔTdown separately on a daily scale to a range of 0 to 1. Then, we compared the standardized values (|ΔTup| and |ΔTdown|) to judge the direction of sap flow (Formula 1).
When θ is positive, it indicates that the sap flow direction is from the upstream to the downstream. When θ is negative, the sap flow direction is from the downstream to the upstream.
Statistical analysis
Considering the limited sampling amounts, we applied a non-parametric test (Wilcoxon rank-sum test) to examine whether there was a significant difference in non-structural carbohydrates among the three treatment groups (Control, Treatment I, and Treatment II) on each sampling day. The same examination was also conducted on the difference between carbon transferred from mother to newly sprouted culms and newly produced carbon by leaves of mother culms.
To compare the dynamics of sap flow directions in rhizomes and stems of the monitored culms, we plotted averaged hourly θ (difference between standardized ΔTup and ΔTdown, see formula 1) before and after leafing of the newly sprouted culms. Further, to observe at which hour the sap flow changed its direction, we plotted histograms and density plots of hours when there was a change in the sap flow direction in rhizomes, stems of both mother and newly sprouted culms before and after leafing of newly sprouted culms.
Data processing, statistical analysis, and plotting were performed in SAS 9.4 (SAS Institute Inc., Cary, NC, USA).
Results
Sources of non-structural carbohydrates in newly sprouted culms
The study period was divided into two stages, that is, before and after 25 May 2021 (namely, pre-leafing and leafing stages of the newly sprouted culms, respectively). On 19 April 2021, when the newly sprouted culms had no leaves and did not receive any treatment, we conducted a pre-treatment measurement and found no significant difference in the NSC content of the mother culms among the three treatment groups (P > 0.05; Figure 2), indicating that the conditions before treatment were the same in each group.
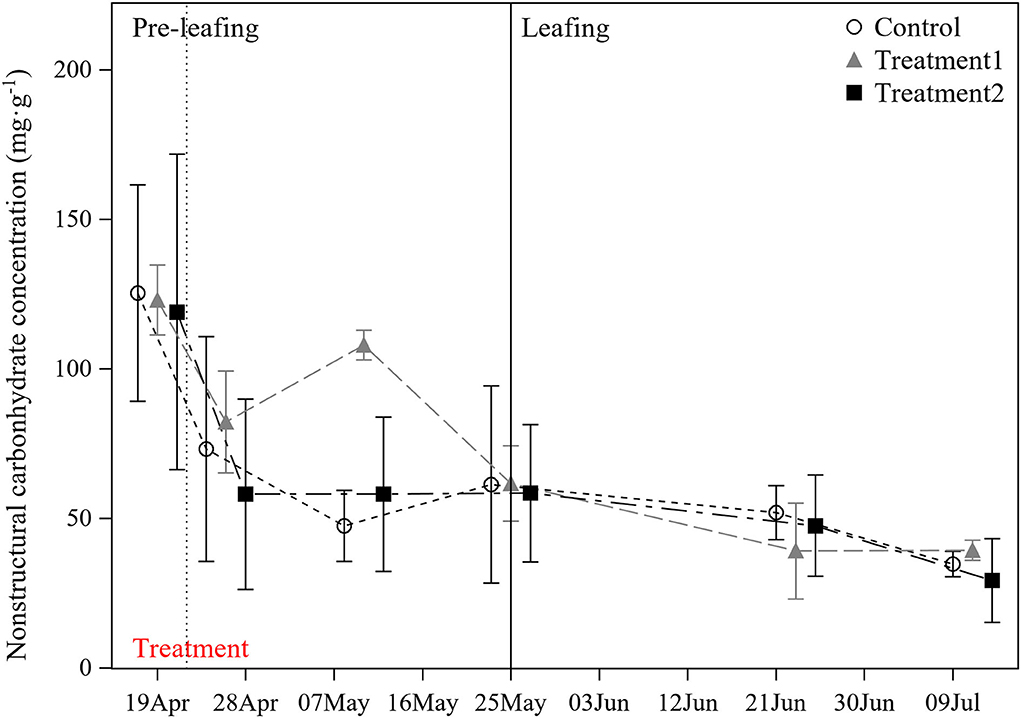
Figure 2. Dynamics of non-structural carbohydrates in stems of mother culms over the experimental period.
After treatment and in the pre-leafing stage, the NSC content of mother culms in the Control group (no rhizome cut or leaves removed) decreased continuously before 10 May 2021. It then increased slightly at the end of the pre-leafing stage. A similar decreasing trend happened in the treatment II group (rhizome cut and leaves removed), but NSC content was more stable from 26 April to 25 May 2021 (Figure 2). In contrast, the NSC content in the treatment I group (rhizome cut) decreased more slowly than in the other two groups during the first week after treatment. Particularly on 10 May 2021, the NSC content in the treatment I group was significantly higher than in both the control and treatment II groups (P < 0.05; Figure 2). We found that the increase in NSC in treatment I was mainly due to an increase in soluble sugars. Soluble sugar accounted for about 80% of NSC, while starch accounted for about 20%. Compared with the increase of NSC on 26 April 2021, the increase of soluble sugar was 3.22 times that of starch on 10 May 2021. However, in the leafing stage, the NSC content in the three groups showed a similar continuous decreasing trend and reached the lowest value at the end of the stage. Additionally, there was no significant difference among the three groups on each sampling day (P > 0.05; Figure 2).
From observed NSC differences between different treatments, NSC_transferred and NSC_leaf_produced were derived to demonstrate possible carbon transfer patterns between mother culms and newly sprouted culms during the explosive growth period (Figure 3). The result may indicate a significant NSC transfer (P < 0.05) from the mother culm to the newly sprouted culm in the pre-leafing stage, which was different from the leafing stage (Figure 3A). Similarly, newly produced NSC was also increased in the pre-leafing stage compared to leafing stage (Figure 3A). Soluble sugar contributes significantly to the newly produced NSC and the transferred NSC in the pre-leafing stage. However, it makes no significant contribution in the leafing stage (Figure 3B). At the same time, starch dynamics showed a different pattern without a significant difference between the pre-leafing and leafing stages (Figure 3C).
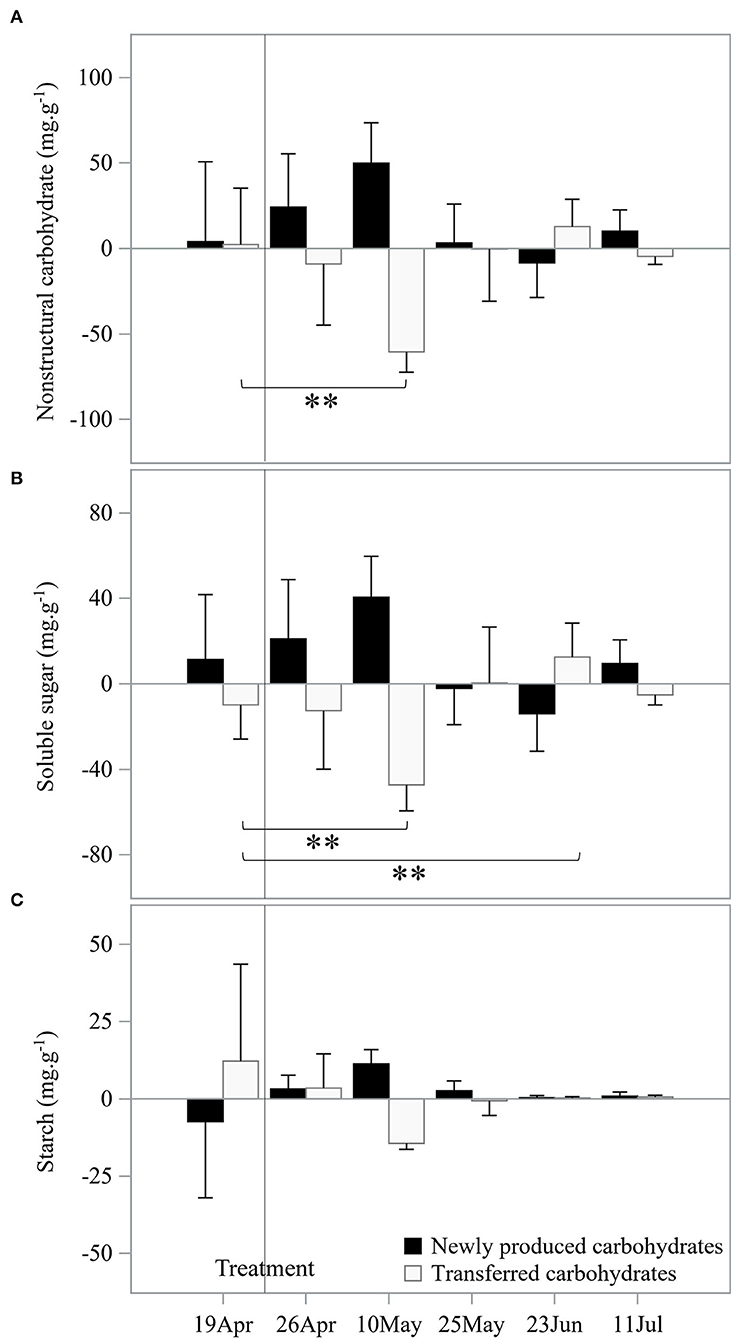
Figure 3. Dynamics of transferred carbohydrates from stems of mother culms to newly sprouted culms and newly produced carbohydrates from leaves of mother culms over the experimental period. (A) The dynamics of non-structural carbohydrates, (B) the dynamics of soluble sugar, and (C) the dynamics of starch. **Indicated a significant difference (P < 0.01) between two data sets at the two ends of the bracket.
Characteristics of water transfer in the bamboo forest during the explosive growth period
In the pre-leafing stage, the diurnal sap flow of mother culms showed a typical pattern of “upstream in the daytime and downstream at the nighttime” with peak values in the mid-day (Figure 4). On the contrary, the sap flow of newly sprouted culms showed a reverse pattern of “upstream at the nighttime and downstream in the daytime” with peak values at night (Figure 4). Corresponding to these sap flow dynamics in the culms, the sap flow direction in rhizomes was from newly sprouted culms to mother culms during the day and vice versa at night (Figure 4). However, in the leafing stage, the daily sap flow of both mother and newly sprouted culms showed the same pattern of “UDDN pattern,” but the sap flow direction in rhizomes was the same as the newly sprouted culms at the pre-leafing stage.
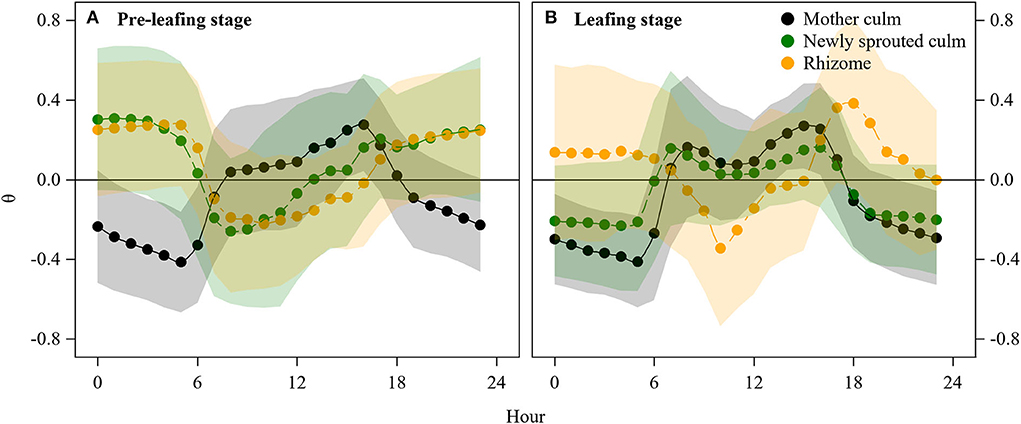
Figure 4. Diurnal hourly sap flow direction shifts before (A) and after (B) leafing of the newly sprouted culms. For the mother and newly sprouted culms, positive and negative θ indicated upward and downward sap flow, respectively. For the rhizomes, positive and negative θ indicated sap flowing to newly sprouted and mother culms, respectively.
To further analyze when the water transfer direction changed before and after leafing, we plotted the hours at which the water flow direction changed (Figure 5). In the pre-leafing stage, the maximum probability (22%) of mother culms' sap flow shifts from downward to upward at 6:00 to 8:00 h and upward to downward at 18:00 to 20:00 h (23%). In contrast, the maximum probability (17%) of newly sprouted culms' sap flow shifts from downward to upward at 14:00 to 16:00 h and upward to downward at 6:00 to 8:00 h (32%). Corresponding to the sap flow shift of the culms, the maximum probability of the rhizome's sap flow shift from newly sprouted culms to mother culms at 6:00 to 8:00 h (29%) and mother culms to newly sprouted culms at 16:00 to 18:00 h (18%).
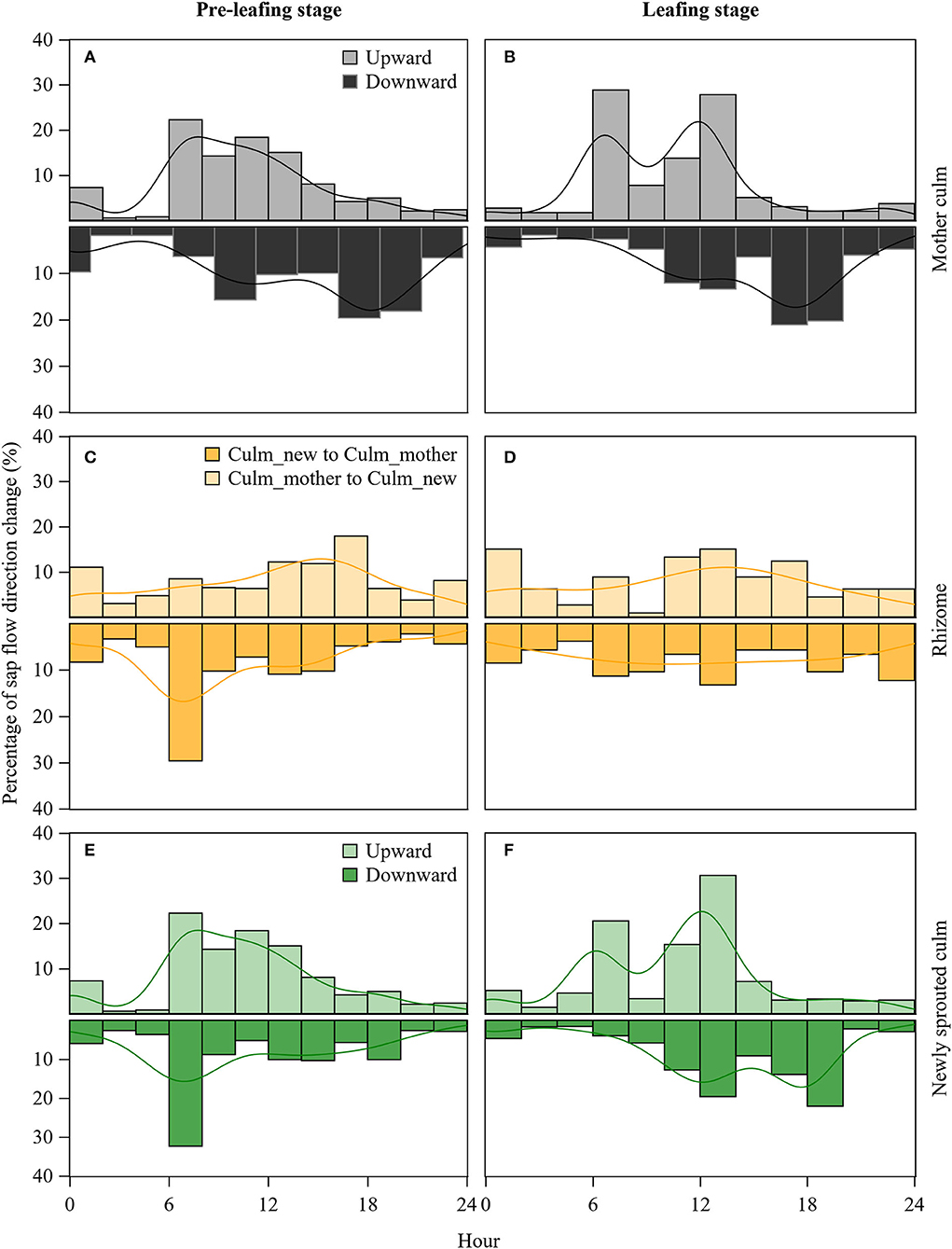
Figure 5. Probabilities at which hours when there was a change in the sap flow direction in rhizomes (C,D), stems of both mother (A,B), and newly sprouted (E,F) culms at the pre-leafing and leafing stages of newly sprouted culms.
In the leafing stage, the maximum probability of mother culms' sap flow shifting from downward to upward appeared at 6:00 to 8:00 h (22%) and from upward to downward at 16:00 to 20:00 h (39%). In contrast, the maximum probability (17%) of newly sprouted culms' sap flow shift from downward to upward appeared at 6:00 to 8:00 h and 10:00 to 14:00 h (20% and 30%) and upward to downward at 18:00 to 20:00 h (22%). However, the sap flow transition in rhizomes was not obvious, and the probability of occurrence was similar at all times of the day. Corresponding to the sap flow shift of the culms, the maximum probability of the rhizome's sap flow shift from newly sprouted culms to mother culms appeared at 12:00 to 14:00 h (15%) and mother culms to newly sprouted culms also at 12:00 to 14:00 h (13%). Therefore, it may indicate a tight competition for water between the mother and newly sprouted culms in the leafing stage.
Discussion
The contribution of stored carbohydrates and newly photosynthesized carbohydrates in mother culms to the growth of newly sprouted culms
In the treatment I group of this experiment, the rhizomes between the mother and newly sprouted culms were cut off to prevent the non-structural carbohydrates from being transferred between the culms. In the treatment II group, the rhizomes were cut off, and all the leaves of mother culms were removed. The difference between non-structural carbohydrates of the culm_mother in the control and treatment I was defined as transferred carbohydrates transferred outward from culm_mother (NSC_transferred). The difference between treatments I and II was defined as newly photosynthesized carbohydrates of the culm_mother (NSC_leaf_produced).
Compared with the Control group, the NSC content in stems of mother culms in the treatment I group was significantly increased after cutting off the rhizomes (Figure 2), implying that the new carbohydrates generated by mother culm leaves may be accumulated in stems. It may further suggest that the carbon needed for the growth of newly sprouted culm may come from both stored carbon of mother culm and newly generated carbon of mother culm leaves. At the end of height growth of newly sprouted culm, NSC in the control group was slightly increased. This may be due to the decreased carbon demand of newly sprouted culm. In contrast, leaves of the mother culms may maintain the original productivity level due to less radiation and temperature fluctuation over the observation period (The average temperatures in April, May, and June of 2021 are 11°C to 20°C, 18°C to 26°C, and 20°C to 29°C, respectively).
Previous studies suggested that the carbon required for explosive growth mainly comes from the non-structural carbohydrate stored in the stems and rhizomes of mother culms (Li et al., 1998; Song et al., 2016). Similarly, this study implied possible support of NSC stored in mother culms to newly sprouted culm for its explosive growth. However, the traditional view emphasized the contribution of carbon storage too much and neglected the role of new carbon fixation in mother bamboo leaves during explosive growth. This study showed that the production of new carbon also increased significantly during the same period when carbon transfer took place (Figure 3). Our study indicated that the contribution of newly produced carbon by mother bamboo to the total transferred carbon to freshly sprouted bamboos should not be ignored.
Potential negative feedback regulation of stem non-structural carbohydrates on leaf photosynthesized carbohydrates of mother culms
Contrary to the ascending trend of mother culm in the Control group in the pre-leafing stage, the NSC of mother culm in the treatment I group was significantly decreased at the same time, which was inconsistent with our expectation. This may be caused by the regulatory feedback generated by the increase of NSC content in the rhizome-cut mother culm stem. This regulatory feedback path may include (1) upward regulation of carbon sources, that is, inhibition of leaf production of new NSC. Compared with leaves that mainly contain newly fixed NSC, stems have larger quantities of stored NSC. Over-saturation of NSC in culms, for example, in treatment I where culms with continuous leaf photosynthesis but lack of output due to rhizome cutting may impair fixation of carbohydrates in leaves (Asao and Ryan, 2015); and (2) downward movement to the underground root system due to sink-control (Friend et al., 2019; Cabon et al., 2022), for example, repairing wound tissues due to cutting off rhizomes in treatment I.
When the rhizomes of the mother culms were cut off, NSC was no longer transferred to newly sprouted culms. In this case, the stem accumulated a certain amount of NSC, becoming the carbon source compared with the rhizomes. The NSC stored in the stem was transferred from the source to the reservoir of rhizomes. Studies have pointed out that the source-sink relationship of different functional organs will change at various stages of plants, and the distribution pattern of carbon resources will also change (Wang et al., 2004; Ji et al., 2016; Savage et al., 2016). Therefore, it is speculated that the change of stem NSC content of the mother culms may lead to the shift in source-sink relation. And the NSC concentration of the mother culm can be restored to the same level of the same period by the feedback. This study was expected to have different levels of NSC concentration after different treatments while observing only a short period of NSC change after treatments. Bamboos seem to have resilience in stabilizing NSC concentrations to the disturbances, that is, treatments in this study. Such resilience may be due to the carbon source-sink feedback, although its mechanism is not fully understood.
Possible rhythm of carbon and water supply of mother to newly sprouted culms
Previous studies found that the stem sap flow pattern of newly sprouted culms was opposite to that of their mother culms at the same time before completing their height growth (Fang et al., 2019; Mei et al., 2019). The maximum value of sap flow occurred at night and daytime for newly sprouted culms and mother culms, respectively, indicating that they were using water resources of bamboo forest at alternate peaks. Such water use characteristics may be related to how carbon enters newly sprouted culms. Munch's theoretical framework explains how carbon produced by leaves is transported from leaves to other organs through the phloem using pressure generated by water potential differences (Münch, 1927, 1931). However, bamboo lacks leaves before completing its height growth, and the carbon required for its growth comes from mother culms (Li et al., 1998; Song et al., 2016). The carbohydrates transportation from the mother to newly sprouted culms involved additional pathways from rhizomes upward to the newly sprouted culms, which creates an additional gravitational resistance along the transport path of unstructured carbon.
Bamboo's gravity-defying carbohydrate transport may require strong forces to overcome, and the power generated at the source and sink may not be enough to overcome gravity. The nighttime root pressure of bamboo may act as an external force against gravity, thus guaranteeing successful transportation of carbohydrates. Root pressure is the pressure formed by the water potential difference between the inside and outside of the cell membrane (Cao et al., 2012). We hypothesized that the mother culms transported carbohydrates to the rhizomes neighboring the newly sprouted culms, producing a lower water potential, which encouraged the roots to absorb water and promote the sap flow in rhizomes. During the day, due to the mother culms' vigorous transpiration, the water absorbed by the rhizomes is more supplied to the mother culms. Instead, as the mother's transpiration decreases at night, intense root pressure sends water to newly sprouted culms. Along with the water, non-structural carbohydrates are transported to newly sprouted culms. This study supports the coordinated transfer of carbon and water at night to provide carbohydrates for the growth of newly sprouted culms. Studies have shown that NSC is involved in regulating xylem water transport (Adams et al., 2017; Tomasella et al., 2019, 2020) and indirectly supported the idea of carbon-water collaborative transport.
Experimental deficiencies and future research prospects
The NSC content of the mother culm in the Treatment II group remained stable after treatment but decreased significantly after the leafing of newly sprouted culms (starting from 25 May). We conducted the difference analysis of NSC of mother culms stems in the Treatment II group before treatment (19 April) and after leafing stage (25 May, 23 June, and 11 July) (p > 0.05, p > 0.05, p < 0.05). Significantly higher air temperature after leafing (11°C to 20°C in April vs. 18°C to 26°C in May) may lead to increased respiration of the mother culms in the treatment II group. Before reaching the optimal temperature, plant respiration rate increases with temperature (Smith and Dukes, 2012). In addition, the carbon input for the stems was zero due to the removal of leaves, while the carbon stored in the stems was consumed by continuous respiration, which significantly reduced the NSC content. Due to the lack of a sustainable carbon source, two of the three mother culms that were treated with rhizome removal died at the end of July and August, respectively. Studies have shown that too low NSC content may lead to plant death (Tomasella et al., 2019, 2020). However, as stem respiration was not monitored synchronically in this study, there was a lack of data support to explain the decline in NSC content of the mother culms in the Treatment II group. Follow-up studies could monitor related indicators.
Due to the lack of defoliation treatment (without rhizome cut) in this study, the contribution of new carbon produced by the leaf of mother culms to the growth of newly sprouted culm cannot be determined. Therefore, the amount of new carbon (Treatment I and Treatment II) obtained in this study only indicates the amount of new non-structural carbohydrates generated by mother culms during the experiment. Whether this NSC is wholly or partially involved in the growth of Newly sprouted culm needs to be further studied.
Conclusion
In this study, we hypothesized that carbohydrates supporting the growth of newly sprouted culms were from both stored and newly produced carbohydrates of mother leaves, and carbohydrates were reallocated to the newly sprouted culms along with the water use rhythm of the “UNDD pattern.” This study found a significant amount of newly produced carbohydrates from mother leaves during the explosive growth period. Furthermore, the study demonstrated that the stored carbohydrates could contribute to newly sprouted culms. The above results supported the multi-source carbohydrates supporting the growth of newly sprouted culms. Furthermore, the mother-rhizome-new bamboo system showed specific water use characteristics linked to the explosive growth of newly sprouted culm, that is, “UNDD pattern.” Such water use rhythm may be related to the transportation of carbohydrates from mother culms to their related newly sprouted culms.
Data availability statement
The datasets presented in this article are not readily available because access to the data is available with agreement of corresponding author and could be used for publication only the corresponding author is listed as a co-author. Requests to access the datasets should be directed to ttmei@zafu.edu.cn.
Author contributions
TM, XL, and CY contributed to the experimental design, field installations, data analysis, figure-making, and wrote and revised the manuscript. DF and GZ contributed to the experimental design and revision of the manuscript. QZ contributed to the field installations. YC and HD contributed to the revision of the manuscript. All authors contributed to the article and approved the submitted version.
Funding
The research was funded by the National Nature Science Foundation of China (Grant Number: 32001102), the Zhejiang Provincial Natural Science Foundation (Grant Number: Q19C160022), the Scientific Research Foundation of Zhejiang A&F University (Grant Number: 2020FR050), the Scientific Research Foundation of Jiyang College of Zhejiang A&F University (Grant Number: 05251700038), the Overseas Expertise Introduction Project for Discipline Innovation (111 Project D18008), and the Key Research and Development Program of Zhejiang Province (2021C02005).
Acknowledgments
The authors acknowledge the administrator of the Pingshan Experimental Base, Zhejiang A&F University. We also thank other students in ZAFU who helped in both the fieldwork and laboratory work; they are Shan Zhou, Lulu Lin, Xinyi Chen, and Kai Pu.
Conflict of interest
The authors declare that the research was conducted in the absence of any commercial or financial relationships that could be construed as a potential conflict of interest.
Publisher's note
All claims expressed in this article are solely those of the authors and do not necessarily represent those of their affiliated organizations, or those of the publisher, the editors and the reviewers. Any product that may be evaluated in this article, or claim that may be made by its manufacturer, is not guaranteed or endorsed by the publisher.
References
Adams, H. D., Zeppel, M., Anderegg, W., Hartmann, H., and Mcdowell, N. G. (2017). A multi-species synthesis of physiological mechanisms in drought-induced tree mortality. Nat. Ecol. Evol. 1, 1285–1291. doi: 10.1038/s41559-017-0248-x
Adonsou, K. E., Annie, D. R., and Francine, T. (2016). Physiological integration of connected balsam poplar ramets. Tree Physiol. 36, 797–806. doi: 10.1093/treephys/tpv142
Asao, S., and Ryan, M. G. (2015). Carbohydrate regulation of photosynthesis and respiration from branch girdling in four species of wet tropical rain forest trees. Tree Physiol. 35, 608–620. doi: 10.1093/treephys/tpv025
Cabon, A., Kannenberg, S. A., Arain, A., Babst, F., Baldocchi, D., Belmecheri, S., et al. (2022). Cross-biome synthesis of source versus sink limits to tree growth. Science, 376, 758–761. doi: 10.1126/science.abm4875
Cao, K. F., Yang, S. J., Zhang, Y. J., and Brodribb, T. J. (2012). The maximum height of grasses is determined by roots. Ecol. Lett. 15, 666–672. doi: 10.1111/j.1461-0248.2012.01783.x
Chapman, D. F., Robson, M. J., and Snaydon, R. W. (1992). Physiological integration in the clonal perennial herb trifolium repens L. Oecologia, 89, 338–347. doi: 10.1007/BF00317411
Dong, M. (1996). Clonal growth in plant in relation to resource heterogeneity : foraging behavior. Acta Bot. Sin. 38, 8.
Durst, P. B., Ulrich, W., Kashio, M., and Pacific, F. (1994). Non-wood forest products in Asia. J. Chromatogr. Sci. 48, 262.
Fang, D., Mei, T., Alexander, R., and Dirk, H.lscher. (2019). Water transfer between bamboo culms in the period of sprouting. Front. Plant Sci. 10, 786–786. doi: 10.3389/fpls.2019.00786
Friend, A. D., Eckes-Shephard, A. H., Fonti, P., Rademacher, T. T., Rathgeber, C. B. K., Richardson, A. D., et al. (2019). On the need to consider wood formation processes in global vegetation models and a suggested approach. Ann. For. Sci. 76, 49. doi: 10.1007/s13595-019-0819-x
Granier, A. (1985). Une nouvelle méthode pour la mesure du flux de sève brute dans le tronc des arbres. Ann.for.sci. 42, 193–200. doi: 10.1051/forest:19850204
Huang, W., Olson, E., Wang, S., and Shi, P. (2020). The growth and mortality of Pleioblastus pygmaeus under different light availability. Glob. Ecol. Conserv. 24:e01262. doi: 10.1016/j.gecco.2020.e01262
Hutchings, M. J., and Wijesinghe, D. K. (1997). Patchy habitats, division of labour and growth dividends in clonal plants. Trends Ecol. Evol. 12, 390–394. doi: 10.1016/S0169-5347(97)87382-X
Jan, B., and Roel, M. (1993). An improved colorimetric method to quantify sugar content of plant tissue. J. Exp. Bot. 44, 1627–1629. doi: 10.1093/jxb/44.10.1627
Ji, L. K., Xie, J. Z., Zhang, W., Lu, P., and Zhang, L. (2016). Organic carbon allocation pattern and changes regulation in various organs of Phyllostachys violascens clone system in shooting period. Acta Ecol. Sin. 36, 7624–7634. doi: 10.5846/stxb201512142488
Lau, R. R., and Young, D. R. (1988). Influence of physiological integration on survivorship and water relations in a clonal herb. Ecology, 69, 215–219. doi: 10.2307/1943177
Li, L., Li, J., Wang, X., Wang, W., Leung, F., Liu, X., et al. (2022). Growth reduction and alteration of non-structural carbohydrate (NSC) allocation in a sympodial bamboo (Indocalamus decorus) under atmospheric O3 enrichment. Sci. Total Environ. 826:154096. doi: 10.1016/j.scitotenv.2022.154096
Li, R., Werger, M. J. A., During, H. J., and Zhong, Z. C. (1998). Carbon and nutrient dynamics in relation to growth rhythm in the giant bamboo Phyllostachys pubescens. Plant Soil. 201, 113–123. doi: 10.1023/A:1004322812651
Liese, W., and Köhl, M. (2015). Bamboo: The Plant and Its Uses. Cham: Springer. doi: 10.1007/978-3-319-14133-6
Mei, T. (2017). Measurements of Tropical Bamboo Water Use. Available online at: https://ediss.uni-goettingen.de/handle/11858/00-1735-0000-0023-3E1A-8 (accessed February 23, 2018).
Mei, T., Fang, D., Rll, A., and Hlscher, D. (2019). Bamboo water transport assessed with deuterium tracing. Forests, 10, 623. doi: 10.3390/f10080623
Mei, T., Liu, X., Fang, D., Zhou, G., Ye, C., Li, P., et al. (2020). Spring leafing phenology favors younger culms of Moso bamboo: aspects from water use relations. Front. Plant Sci. 11, 550. doi: 10.3389/fpls.2020.00550
Savage, J. A., Clearwater, M. J., Haines, D. F., Klein, T., Mencuccini, M., Sevanto, S., et al. (2016). Allocation, stress tolerance and carbon transport in plants: how does phloem physiology affect plant ecology? Plant Cell Env. 39, 709–725. doi: 10.1111/pce.12602
Smith, N. G., and Dukes, J. S. (2012). Plant respiration and photosynthesis in global-scale models: Incorporating acclimation to temperature and CO2. Glob. Change Biol. 19, 45–63. doi: 10.1111/j.1365-2486.2012.02797.x
Song, X., Peng, C., Zhou, G., Gu, H., Li, Q., and Zhang, C. (2016). Dynamic allocation and transfer of non-structural carbohydrates, a possible mechanism for the explosive growth of Moso bamboo (Phyllostachys heterocycla). Sci. Rep. 6:25908. doi: 10.1038/srep25908
Tomasella, M., Casolo, V., Aichner, N., Petruzzellis, F., Savi, T., Trifil,ò, P., et al. (2019). Non-structural carbohydrate and hydraulic dynamics during drought and recovery in Fraxinus ornus and Ostrya carpinifolia saplings. Plant Physiol. Biochem. 145, 1–9. doi: 10.1016/j.plaphy.2019.10.024
Tomasella, M., Petrussa, E., Petruzzellis, F., Nardini, A., and Casolo, V. (2020). The possible role of Non-structural carbohydrates in the regulation of tree hydraulics. Int. J. Mol. Sci. 21, 144. doi: 10.3390/ijms21010144
Tong, C., Zhang, X., Xie, J., Mei, T., Fang, D., and Li, Y. (2021). Water use strategies of different aged moso bamboo culms under summer drought. For. Ecol. Manag. 498, 119567. doi: 10.1016/j.foreco.2021.119567
Wang, Y., Hong, R., Huang, D., Teng, X., Li, Y., Shiyomi, M., et al. (2004). The translocation of photosynthate between clonal ramets of Leymus chinensis population. Acta Ecol. Sin. 24, 900–907.
Yu, L., Wang, C., and Wang, X. (2011). Allocation of non-structural carbohydrates for three temperate tree species in Northeast China. Chin. J. Plant Ecol. 35, 1245–1255. doi: 10.3724/SP.J.1258.2011.01245
Zhou, B. Z., and Fu, M. Y. (2004). Review on bamboo's under ground rhizome-root system research. For. Res. 17, 8.
Zhou, G. (2006). Carbon storage, fixation and distribution in Moso bamboo (Phyllostachys pubescens) stands ecosystem. Zhejiang university.
Keywords: explosive growth, water transfer, Moso bamboo, carbon transfer, sap flow
Citation: Li X, Ye C, Fang D, Zeng Q, Cai Y, Du H, Mei T and Zhou G (2022) Non-structural carbohydrate and water dynamics of Moso bamboo during its explosive growth period. Front. For. Glob. Change 5:938941. doi: 10.3389/ffgc.2022.938941
Received: 08 May 2022; Accepted: 04 July 2022;
Published: 27 July 2022.
Edited by:
Nobuo Imai, Tokyo University of Agriculture, JapanReviewed by:
Weiwei Huang, Nanjing Forestry University, ChinaDavid Herrera, Max Planck Institute for Biogeochemistry, Germany
Copyright © 2022 Li, Ye, Fang, Zeng, Cai, Du, Mei and Zhou. This is an open-access article distributed under the terms of the Creative Commons Attribution License (CC BY). The use, distribution or reproduction in other forums is permitted, provided the original author(s) and the copyright owner(s) are credited and that the original publication in this journal is cited, in accordance with accepted academic practice. No use, distribution or reproduction is permitted which does not comply with these terms.
*Correspondence: Tingting Mei, ttmei@zafu.edu.cn
†These authors have contributed equally to this work and share first authorship