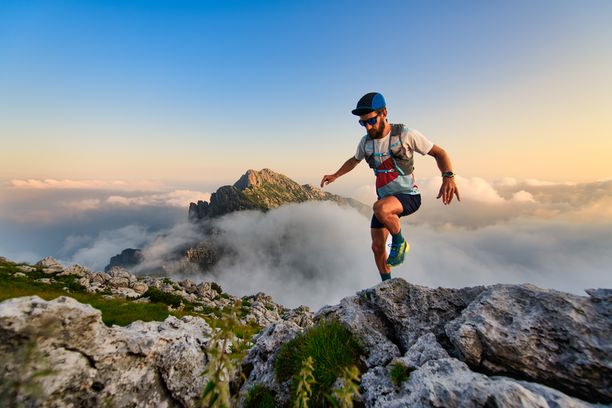
95% of researchers rate our articles as excellent or good
Learn more about the work of our research integrity team to safeguard the quality of each article we publish.
Find out more
ORIGINAL RESEARCH article
Front. For. Glob. Change, 12 August 2022
Sec. Forest Management
Volume 5 - 2022 | https://doi.org/10.3389/ffgc.2022.933020
This article is part of the Research TopicForest Carbon Credits as a Nature-Based Solution to Climate Change?View all 10 articles
The costs and technical expertise associated with forest carbon offset projects can be significant, while decades-long time commitments can discourage participation from the outset. Considering these challenges, several new approaches have emerged in the United States under the auspices of both long-standing and recently-established programs, attempting to leverage increased carbon mitigation. What several of these approaches have in common is reduced emphasis on long-term storage, what we refer to as a traditional perspective of permanence. Instead, each considers shorter periods of time—up to and including single year harvest deferrals—as eligible project commitments. Here, we provide a brief discussion of the historical permanence and accounting literature, with an emphasis on contradictory views and how these perspectives have evolved over time. Next, we quantitatively assess the long-term influence of different permanence requirements as envisioned in several new and existing forest carbon programs, estimating net mitigation across a variety of forest types and project configurations. We conclude with a presentation of our quantitative findings in the context of the existing literature, while also highlighting unmet research needs on these so-called new offsets, those emerging novel approaches for forest carbon mitigation that challenge the research and practice status quo.
Global climatechange mitigation continues to be a critical concern, necessitating abatement from all sectors of the economy to reduce long-term effects of warming (de Coninck et al., 2018). Declarations from the most recent United Nations Framework Convention on Climate Change (UNFCCC) Conference of the Parties (COP) reaffirm the importance of forest carbon storage as a mitigation strategy (Conference of Parties 26 [COP26], 2021). Current estimates suggest that the global land use systems can provide 20–30% of mitigation needed to hit long-term climate stabilization targets (IPCC, 2022). Recent United States-focused projections suggest that the forest sector could play a key role in the land-based mitigation portfolio, with substantial contributions coming from temporary carbon sequestration strategies such as extended rotations and management intensification (Austin et al., 2020; Wade et al., 2022). Despite growing model-based evidence that forest management interventions can provide additional and low-cost climate mitigation, mobilizing mitigation in forests has proved challenging. Implementation of individual offset projects can be an arduous process (Galik and Cooley, 2012). The costs and technical expertise associated with offset project establishment can be significant, while decades-long time commitments can discourage participation from the outset (Galik et al., 2012; Ruseva et al., 2020). As a result, traditional offset projects have generally been undertaken by larger landowners (Bigsby, 2009), and market growth has been limited. Further, concerns have been raised about the integrity of individual offset projects, specifically that they do not represent new, additional mitigation (Haya et al., 2020) or that on-site mitigation could be undercut by activities encouraged elsewhere, i.e., leakage (Murray et al., 2004).
Despite these challenges, a re-emergence of interests in forest carbon offsets has resulted in new approaches to offset crediting in the United States to improve participation rates among forest landowners and land managers that would not otherwise participate in carbon markets. These approaches could be more appealing to forest landowners by both reducing the commitment period and eliminating most of the landowner-borne costs associated with measurement, monitoring, and verification (MMV). A defining characteristic of these new market offerings is short (as low as 1 year) time commitments required for participation and the leveraging of remote sensing to minimize the costs of individual participation.
As reflected in a recent online commentary on the subject, however, “[s]urprisingly, there is not a clear intellectual framework for thinking about the climate benefits of temporary carbon storage” (Chay et al., 2022). While true in an applied sense, there is also a deep literature and decades of implementation experience to draw upon to develop such a framework. The discussion of the potential for type-I and type-II errors in forest carbon crediting by Chomitz (1998) implicitly captures the tension in current offset markets, where concerns of over-crediting still meet concerns of under-supply (e.g., Ruseva et al., 2020). The potential for long-term commitments to discourage participation from both particular sizes of landowner (Bigsby, 2009) and particular types of management activities (Chomitz, 1998) has likewise been long articulated.
The emergence of new market models could therefore be seen as relitigating previously identified concerns over offset quality vs. quantity of mitigation supplied. At present, there exists little research on the specifics of these market models, as applied, and how they specifically relate back to the expansive body of research on forest carbon sequestration. Here, we address this crucial data gap by first providing a brief discussion of permanence accounting in the existing literature, recalling past debates over the adequacy and appropriateness of ton-year accounting, project commitment period, and discounting. Next, we quantitatively assess the long-term influence of different permanence requirements as envisioned in several new and existing forest carbon offset programs, estimating net carbon mitigation across a variety of United States forest types, permanence requirements, accounting assumptions, and project configurations. The literature is replete with examples of similar protocol road tests, making use of both empirical field data (e.g., Pearson et al., 2008; Galik et al., 2009) and stand-based allometric equations (e.g., Foley et al., 2009; Galik et al., 2012), but the accounting assumptions under which many road tests have been performed are now potentially dated. To this end, we frame our conclusions about permanence in the context of continuing research needs on so-called new offsets, or emerging novel approaches for forest carbon mitigation that challenge the research and practice status quo of recent decades.
The value of forest carbon as a GHG mitigation strategy has been recognized for decades (Marland, 1988; Sedjo, 1989), but properly accounting for carbon sequestration has been the source of continuous debate and deliberation. In many instances, these debates and deliberations have played out in the literature itself, in which individual papers directly built onto or refuted previous works. Presented below is a brief discussion of contradictory views, and how these perspectives have evolved over time.
Early papers on forest carbon accounting emphasized uncertainty and the timing of emissions reductions (e.g., Marland et al., 1997; van Kooten et al., 1997). In their analysis of temporary storage, Marland et al. (1997) specifically argued that the timing of emissions reductions was important to observe—not just the end-point value—utilizing a so-called carbon discount factor in their assessment of different sequestration patterns over time. Carbon discounting was similarly applied in an analysis of lowland forest management by Boscolo et al. (1997), while Fearnside (1997), van Kooten et al. (1997), and Fearnside et al. (2000) each discussed the appropriateness of discounting and the choice of a proper rate. Though these and other examples from the literature also review the practice of discounting of physical quantities, subsequent contributions have raised concerns with the approach (e.g., Marshall and Kelly, 2010). As O’Hare et al. (2009) later cautioned, “discounting is correctly applied only to economic rather than physical quantities, so before such economic analysis can be meaningfully pursued the relationship between physical and economic quantities must be established” (4).
Continued discussion of the timing and permanence of forest carbon sequestration stretched into the early 2000s, featuring seminal papers by Fearnside et al. (2000) and Moura-Costa and Wilson (2000). In calculating the value of a single ton of CO2 removed for a single year, Moura-Costa and Wilson (2000), argued that the resulting ton-year approach “removes uncertainty related to the long-term permanence of forests, because crediting for forestry activities can be calculated in proportion to the project timeframe on a “pay-as-you-go” basis” (58). Fearnside et al. (2000) continued the development of the ton-year concept, describing in detail what have come to be defined as the “Moura-Costa” and “Lashof” methods for temporary accounting. While the former evaluates the benefits associated with carbon temporarily sequestered in a forest stand by comparing it to the total amount of carbon that would have been retained in the atmosphere over a given period following a release (usually 100 years), the latter is equivalent to the carbon retained in the atmosphere as a result of the delayed emissions associated with the project activity after the same given period of time (again usually 100 years).
The choice of an equivalence period, defined as the period of time in which atmospheric warming from a one unit emission of CO2 is counteracted by additional sequestration (Moura-Costa and Wilson, 2000), was seen as a critical aspect of temporary crediting, particularly a ton-year approach. As equivalence is assessed over a finite period, the choice of that period is by necessity a policy determination and not a purely scientific one (Dutschke, 2002; Fearnside, 2002). And while the selection of the relevant timescale is subjective, the choice of that timescale was noted to strongly influence the relative climate benefits yielded by temporary storage (Levasseur et al., 2012a). As evidenced by early discussions of temporary accounting (e.g., Fearnside et al., 2000; Moura-Costa and Wilson, 2000), a 100-year time horizon was initially seen as a relevant timescale over which to evaluate the contributions of temporary sequestration. Though arguments have been made that the default choice of 100 years “appears hard to justify” in terms of long-term climate benefits (Jørgensen and Hauschild, 2013; p. 747), recent analysis has underscored the consistency of a 100-year time horizon with discount rates used in broader societal measures of climate impact (Sarofim and Giordano, 2018; Mallapragada and Mignone, 2020).
While some have presented arguments speaking to the benefits of temporary storage, others have presented counter arguments stating that the contributions of temporary storage are inherently limited (Korhonen et al., 2002; Jørgensen and Hauschild, 2013). These points were underscored in a pair of works by Kirschbaum (2003, 2006) in which the author reviewed the value of temporary storage through the lens of three separate climate change indicators (instantaneous effect of increased temperature, rate of temperature increase, and cumulative effect of increased temperature). While noting that temporary storage could be useful—but only if it corresponds to times of peak concentrations or greatest rates of change—Kirschbaum (2003) concluded that “[t]he simplistic notion that trees can “buy time” is rarely justified, and a more specific analysis needs to be carried out to define exactly whether, or under what conditions, trees can, indeed, play a useful role in minimizing climate-change impacts” (69). These conditions were further hypothesized by Kirschbaum (2006) to include “a rolling progression of different carbon-sink projects on different parcels of land so that, put together, the total of all individual stands more closely approaches the situation of permanently increased carbon storage” (1159).
This idea that incentives for carbon could yield permanent aggregate increases in carbon storage—despite the temporary nature of individual projects—was earlier articulated by Marland et al. (2001). Drawing conceptually from this earlier work, Dornburg and Marland (2008) provided a rebuttal to Kirschbaum (2006) in which they again argued for the value provided by temporary storage, while also agreeing that a ton-year approach might not be a preferred mechanism to credit that storage. An additional rebuttal to Kirschbaum (2006) was provided by Fearnside (2008), in which the latter focused less on the conceptual benefits of temporary storage and instead challenged the relevance of metrics used as the basis for the former’s conclusions.
At the same time, research elsewhere was attempting to assess the role of different accounting approaches on project configuration and individual management decisions. Fearnside et al. (2000) detailed the role of timing and discounting in encouraging different types of projects, suggesting that greater time preference (high discount rate or shorter equivalence period) tended to encourage temporary sequestration and those that generate quick benefits (e.g., avoided deforestation). van Kooten (2009) meanwhile suggested that high transaction costs associated with project monitoring and verification could lead to rent-seeking behavior by project proponents, contributing to the existence of so-called dubious projects.
An increased variety of project and accounting assumptions have been assessed in recent years, providing additional insight into the influence of accounting on management decision-making. Lintunen et al.’s (2016) in-depth analysis of a carbon rental approach suggested that outright carbon purchase was most advantageous for quickly growing forests, while carbon rental was most advantageous for standing, well-stocked forests. Juutinen et al. (2018) meanwhile explored the influence of short-term (1-year) carbon payments on forest management, carbon storage, and timber yield, finding that carbon payment resulted in rotation extension across a variety of forest types and conditions, but that higher prices did not always result in further lengthening of rotations. In agreement with Lintunen et al. (2016); Juutinen et al. (2018) further concluded that short-term payments were perhaps best suited for landowners with mature stands, allowing capture of carbon storage benefits by extending rotation length.
The extant literature on temporary carbon storage can perhaps be best summed up in a single passage by Levasseur et al. (2012b), who flatly stated that “the ability of a temporary sequestration and storage project to compensate for a certain GHG emission highly depends on the chosen assessment method, and on the related assumptions” (772). Brandão et al. (2013) similarly concluded that the observed benefits of temporary storage would “rely on policy-based accounting choices,” and accordingly called for making such accounting decisions both “explicit and transparent” (238). With perspectives on the value of temporary storage ranging from negative (e.g., Kirschbaum, 2006) to positive (e.g., Dornburg and Marland, 2008), the question ultimately becomes whether to allow for temporary sequestration in forest carbon offset projects, and if so, how best to account for it.
One challenge with evaluating alternative crediting schemes is the choice of methodology—new offset markets can induce system-wide effects that impact both forest management decisions and product markets, but it is difficult to represent these nascent markets in systems models. Forest sector economic models simulate forest management change in response to market and policy drivers while accounting for heterogeneity in age class structure, land productivity and relative cost structures over time (Latta et al., 2013). Modeling approaches typically quantify mitigation outcomes relative to a baseline scenario, which has the benefit of only rewarding additional mitigation and netting out leakage if emissions changes are summed across all modeled regions. While these models provide insight into forest management and market dynamics in the presence of broadly applied carbon policy incentives, they do not capture the nuance or implementation challenges of voluntary forest carbon crediting schemes (e.g., Latta et al., 2011). For example, models have traditionally not distinguished between temporary vs. long-term storage credits, and often introduce mandatory incentive structures (e.g., carbon pricing) that are applied broadly across the forest landscape and sector.
Given the wide variety of accounting considerations—and contradictions—featured in the literature above, stand-level analysis can fill critical knowledge gaps on the relative performance of crediting schemes as-applied. Therefore, our methodology here compares the relative performance of different temporary crediting approaches at a stand level in order to complement systems perspectives on forest mitigation opportunities. We do not, however, capture the complex suite of decisions a forest manager must make at a given point in time (including whether or not to harvest or defer and sell carbon credits) or the interactions between carbon incentives and traditional markets for stumpage. Despite these limitations, a combination of model-based simulations and stand-level analysis of temporary carbon crediting can provide key insight to decision-makers on economic opportunities for mitigation investments and tradeoffs among incentive structure design choices.
In this analysis, our emphasis is again on permanence, particularly in the context of short duration projects. A variety of approaches to account for project permanence have been adopted by registries and programs in recent years. Among these include assumed permanence periods of 100 years, 20-year commitment periods with additional 20-year holding periods, 10-year commitment periods, and, emerging in only the last few years, single-year harvest deferral project periods. The section below provides a brief review of these approaches, citing examples with a focus on their application in existing Improved Forest Management (IFM) programs or practices. Immediately following this description is an overview of the quantitative analysis or “road test” exercise we undertake to assess the relative performance of each accounting approach across a variety of forest and project conditions. All data and calculations are available in an Excel spreadsheet located in the Supplementary Data Sheet 1.
The largest IFM participation in the United States uses the California’s 2006 Global Warming Solutions Act (AB32) IFM offset methodology which is linked with the Quebec and Ontario cap-and-trade programs and has been adopted for use in the Regional Greenhouse Gas Initiative in the northeast. This program requires a 100-year maintenance of stocks following the last issuance of credits over the 25-year crediting period. The Climate Action Reserve (CAR) which shares a lineage with the AB32 program also requires a 100-year commitment to maintaining carbon stocks at or above the levels at final credit issuance.
The Voluntary Carbon Standard (VCS) requires a typical cutting cycle as the basis for permanence in their forest-based IFM and Afforestation, Reforestation, and Revegetation (ARR) methodologies. The minimum of 30 years or the maximum of 100 years have been applied in the five IFM projects that have earned VCS credits as of the writing of this paper while the four ARR projects range from 32 to 70 years in length.
The American Carbon Registry (ACR) IFM methodology is the second most utilized in the United States and it requires a 20-year crediting period, which can be followed by another 20-year crediting period. In either case a 20-year maintenance of stocks period is required setting the total project permanence requirement to be 40 or 60 years. The Small Non-Industrial Private Forestlands methodology approved by ACR in the fall of 2021 is one recent example of this framework approach as targeted to smaller landowners (American Carbon Registry [ACR], 2021).
One recent methodology currently under review at VCS, but also being implemented outside of the three primary United States registries is the Family Forest Carbon Program (FFCP). The FFCP is a collaborative effort between the American Forest Foundation and the Nature Conservancy. The pilot program currently targeting family-owned forests in the northeastern United States utilizing two potential mitigation practices: growing mature forests and enhancing future forests. The growing mature forests options requires a 20-year commitment to defer harvest and the enhancing future forests option requires a 10-year commitment. To date most participation has fallen under the growing future forests option.
An approach utilizing single-year deferral harvests is currently offered by Natural Capital Exchange (NCX). Though an underlying ton-year methodology was recently tabled for consideration by the accreditation entity Verra (2022), the single-year deferral model has attracted a great deal of interest and thus warrants consideration here. With an emphasis on harvest deferral activities, commitment periods for participating landowners under the NCX model are defined in 1-year increments, implemented across quarterly “fulfillment events” with the option to re-enroll (Parisa et al., 2020; Parisa, 2021). The value of a 1-year deferral is itself calculated assuming a discounted 48-year equivalence period (Parisa et al., 2020). The use of discounting further reduces the equivalence period to 17 years by more heavily weighting near-term emission avoidance. This approach is justified using recent literature on the consistency of 100-year global warming potentials with set discount rates (Sarofim and Giordano, 2018; Mallapragada and Mignone, 2020).
To estimate the influence of accounting on the net mitigation yielded by hypothetical projects operating under a variety of approaches drawn from the above examples, this analysis uses stand-level allometric equations as detailed in Galik et al. (2012) to assess the live tree carbon associated on a hypothetical harvest deferral project under multiple stand growth, accounting, and re-enrollment conditions, with an emphasis on Southeast (SE) loblolly-shortleaf pine, Northern lake state (NLS) oak-hickory, and Pacific Northwest-west (PNWW) high management Douglas fir stands (see Figure 1; Supplementary Data Sheet 1). For all project configurations indicated in Table 1, we assume the stand would have been harvested in year one of the baseline scenario, chosen to reflect the approximate average rotation age in each stand type for stands still accumulating carbon (“Increasing”) or to assess the influence of accounting decisions on older stands that have achieved steady-state carbon flux (“Stable”).
For harvest and regrowth projects, the stand is assumed to be replanted after harvest and allowed to regrow indefinitely from year one. In harvest and conversion projects, we assume that the forest is not replanted after harvest but is converted to some other non-forest use (e.g., urban development), with post-harvest stand carbon thus maintained at zero. For decay and discounted decay scenarios, both baseline and project stand emissions are adjusted by the amount of carbon that would remain in the atmosphere following a pulse of removals, using annual decay factors as specified in Parisa et al. (2021) (Sheet B, Rows 240-241, Supplementary Data Sheet 1). This implicitly assumes that all removals are instantaneously emitted to the atmosphere. For discounted decay scenarios, annual emissions and sequestration are discounted by an additional 3.3% per Parisa et al. (2021) to account for a preference for near-term climate mitigation. In ton-year scenarios, we ascribe an annual benefit equal to 0.021 to foregone removals in the baseline, reflecting an equivalence period of approximately 48 ton-years over a 100-year time horizon per Parisa et al. (2020).
Net sequestration is calculated by comparing carbon flux in the baseline to the project in each of our 144 total scenarios. In 1-, 2-, 10-, and 100-year projects, this is simply the sum of the baseline over these time periods compared to the project over these same time periods. For the “1 + 1” scenario, we assume consecutive 1-year projects for a total of two straight years of program enrollment. For the “20 + 20” scenario, additional carbon storage is only credited for the initial 20 years of the carbon project, but no harvest is allowed until year 40. In the “decay” and “discounted decay” scenarios, this implies that credit is still earned for displaced harvest for the entire 40-year project, but not for additional sequestration in the stand in years 21–40. In the consecutive 1-year scenarios, the project scenario is compared to a year-zero baseline in the first year but a year-one baseline in the second to assess the effects of renewing a short-term project. Note that in all scenarios we account for stand growth in both the baseline and project. Full calculations are available in the spreadsheet associated with this analysis (Supplementary Data Sheet 1).
Summary results from the quantitative accounting exercise above can be seen in Figure 2. Across all project lengths, forest types, and project baseline scenarios, we find the greatest variability of average annual sequestration in decay-based accounting approaches (undiscounted and discounted decay). These two approaches likewise tend to produce more favorable estimates of carbon sequestration, as indicated by the lower negative means and larger negative ranges. Ton-year accounting yields a comparatively reduced interquartile range that still falls largely below zero, with a net negative mean of annual average sequestration values.
Figure 2. Comparison of accounting approaches across project scenarios, showing average annual net sequestration by accounting approach, including all forest types, project lengths, and project baseline scenarios (e.g., reforestation vs. conversion). Ton-year results reflect a 100-year time horizon. Negative values represent net sequestration relative to a baseline, immediate-harvest scenario.
Breaking out the results indicated in Figure 2 by forest type allows for increased emphasis on the influence of accounting and project assumptions on estimated net sequestration (Figure 3). Interestingly, the patterns are roughly similar to those presented in Figure 2, while also demonstrating the influence of Douglas fir on the range of estimated sequestration (seen in both the increased negative and positive emissions associated with projects in that forest type). As in Figure 2, the tendency for decay and discounted decay scenarios to yield net reductions is apparent in all forest types. Ton-year generally shows a smaller range, with the exception of increasing growth loblolly stands; depending on the age of the stand, regrowth in the baseline counterfactual can outpace continued growth in the project scenario.
Figure 3. Comparison of accounting approaches across project scenarios, showing average annual net sequestration by forest type and accounting approach, including all project lengths and project baseline scenarios (e.g., reforestation vs. conversion). “Increasing” and “Stable” reflect stand carbon accumulation at the time of harvest. Ton-year results reflect a 100-year time horizon. Negative values represent net sequestration relative to a baseline, immediate-harvest scenario.
Figures 2, 3 are indicative of the range of results, but greater insight is necessary as to the particular drivers of aggregate results. Expressing results as a scatterplot can help to discern results otherwise masked. For example, in harvest and regrowth forest offset projects (Figure 4), aforementioned post-harvest stand growth in the baseline creates conditions reducing net project sequestration in many cases, particularly for projects beginning at later stand ages. In projects assuming post-harvest stand conversion (Figure 5), there is both a greater proportion of negative-flux project configurations and greater magnitudes of negative flux achieved.
Figure 4. Average annual net flux (tC/ha) by project length for deferred harvest and regrowth projects. Accounting approach and forest type for extreme values are indicated. Ton-year results reflect a 100-year time horizon. Negative values represent net sequestration relative to a baseline, immediate-harvest scenario.
Figure 5. Net flux (tC/ha) by project length for deferred harvest and conversion projects. Accounting approach and forest type for extreme values are indicated. Ton-year results reflect a 100-year time horizon. Negative values represent net sequestration relative to a baseline, immediate-harvest scenario.
Across project configurations in both Figures 4, 5, assuming decay of foregone emissions and the discounting of decayed foregone emissions tends to result in the largest net reductions in the early years of the project. In harvest and regrowth projects (Figure 4), the advantage associated with undiscounted atmospheric decay is quickly displaced by new stand growth in the baseline, eventually leading to net positive emissions associated with the project by year 40, while discounted decayed emissions continue to generate negative project fluxes across all years of the scenario. Both decay and discounted decay approaches lead to negative fluxes in projects across all years in the harvest and conversion scenarios. Alternatively, ton-year accounting is more consistent across project lengths and configurations. In harvest and conversion scenarios, net flux tends to scale proportionally with time, decreasing as project length increases. In harvest and regrowth projects, the pattern is less discernable, but clusters around zero. Finally, the array of forest types is itself interesting. In both Figures 4, 5, Douglas fir tends to be seen more often at the extremes of estimated flux because of its higher stand carbon stocking.
If we vary the time horizon for ton-year accounting, or the discount rate for discounted decay accounting, changes in net flux again vary by project length, forest type, and project scenario (Table 2). The trend is as we might expect; longer time horizons result in lesser short-term harvest deferral benefit, again largely due to the presence of a quickly regrowing stand in the baseline. This dynamic results in the interesting finding of widely diverging flux totals, particularly for 20 + 20 and 100-year projects, depending only on the time horizon selected.
Table 2. Sensitivity analysis for ton-year accounting, showing values of average annual net carbon flux (tC/ha) varying by time horizon.
Revisiting our assumed discount rates, the selection of alternative discount rates again generates some degree of variation between estimated flux, but the patterns between estimates are more consistent (Table 3). Here, the pattern is one of increasing carbon flux for longer project lengths and a generally higher discount rate, though the dynamics of stand sequestration do influence the arrangement of the latter in longer harvest and conversion projects. The results are largely as would be expected, however, with increased preference for near-term mitigation.
Table 3. Sensitivity analysis for discounted decay accounting, showing values of average annual net carbon flux (tC/ha) varying by assumed discount rate.
Collectively, the results above demonstrate how, particularly for shorter projects, assumed atmospheric decay of baseline-emitted carbon or discounting of those decayed emissions can increase the perceived benefits of the project. The project configurations assessed here, generally mature and slow-growing, also contribute to a situation in which the carbon benefits associated with the rapid regrowth of a harvested stand in the baseline can reduce the net mitigation associated with a number of project configurations and accounting approaches. The project configurations and accounting approaches yielding the greatest negative flux, however, are less consistent. The analysis indicates that discounted decay consistently generates the largest estimated reductions in the harvest and regrowth scenario but varies by time in harvest and conversion projects.
Forest offset markets are in a time of transition, and there are unresolved questions about how new market models align with existing, decades-old research on forest carbon accounting. New offerings like those from NCX, ACR, the Family Forest Carbon Program, and others represent a new approach to encourage forest carbon sequestration, potentially addressing what have long been seen as structural challenges to offset market participation (e.g., Chomitz, 1998). But even as a novel approach in practice, the concept of shorter project periods recalls several conceptual arguments previously advanced in the scientific literature. The purported aggregate landscape benefits of individual temporary storage projects in the presence of carbon incentives recalls arguments put forth decades ago (e.g., Marland et al., 2001). The use of a 100-year time horizon is likewise consistent with previous reviews of temporary carbon accounting (e.g., Moura-Costa and Wilson, 2000).
Elsewhere, the reliance on temporary mitigation, particularly projects with short commitment periods (i.e., 1 year), has been suggested to potentially encourage “dubious projects” and free riders (Dutschke, 2002; van Kooten, 2009) while failing to fundamentally address climate change over the long run (Maréchal and Hecq, 2006). The literature has featured wide-ranging values for temporary storage equivalence periods over the last few decades, as well—Marland et al. (2001) alone identifies estimates ranging from 42 to 150 years—underscoring the uncertainty surrounding selection of this influential metric. As reinforced by one reviewer of this manuscript, the discounting of physical quantities of carbon mitigation is itself controversial, with O’Hare et al. (2009) cautioning that “discounting is correctly applied only to economic rather than physical quantities” (4). More recently, Groom and Venmans (2022) derive a social value of offsets (SVO) as a function of the social cost of carbon to better account for offset impermanence and non-additionality. Cullenward et al. (2022) meanwhile raise concerns about both the use of discounting in NCX accounting, as well as the use of a net discount rate. As a reviewer likewise pointed out, the choice of project horizon can help screen for disproportionate losses in the early years of an offset project—the logic being that there are insufficient opportunities to recapture emitted carbon—making the choice of that horizon an important consideration independent of any discounting decision.
The analysis here, comparing both new and long-standing models for temporary carbon accounting, demonstrates the importance of resolving the above uncertainties and of developing a clear and consistent framework for articulating the value of temporary storage (Chay et al., 2022). The analysis, limited as it is, also provides some guidance in how we might derive such a framework. We show that the use of discounting and decay functions can increase the perceived value of temporarily avoiding emissions, reducing the cost of bringing eventual tons to market. These findings are corroborated by Parisa et al. (2020), in which a hypothetical short-term harvest deferral using both discount and decay accounting achieves forest carbon mitigation at a far lower cost on a per ton-year basis than alternative project designs operating under other offset market models. Ton-year tends to be more consistent across project and forest types.
More generally, our analysis highlights the variability introduced by project design and operation itself. The type of project, particularly what is expected to happen after the foregone harvest activity, has a strong influence on estimated net flux that is observable in the difference between patterns observed in Figures 4, 5. The influence of these baseline assumptions, and indeed all assumptions about project operation over the life of the project, introduce uncertainty into offset impermanence accounting that increased precision on the units used to track sequestration necessarily fail to overcome. This is particularly important given the improved performance (i.e., negative mitigation values) seen in the conversion projects (Figure 5) relative to the regrowth projects (Figure 4), highlighting the incentives that might exist to misrepresent anticipated outcomes.
If nothing else, our analysis demonstrates the influence of project accounting on the estimated stream of project credits, indicating that an academic debate over the appropriateness of discounting, equivalence period, time horizon, and baseline each have potential real-world consequences. Within our analysis, it appears as though ton-year, and possibly undiscounted decay metric in harvest and conversion projects, are the most consistent accounting approaches across project timelines, forest type, and project configuration. These approaches are consistent across the array of variables assessed here, which is an important consideration in development of ways to address project impermanence, particularly in light of recent attention to short-term deferrals like those assessed in this paper.
Our analysis is necessarily limited, relying on off-the-shelf forest carbon data and considering only a small subset of all possible forest types and project configurations. Further analysis should consider a wider array of project configurations, project conditions (e.g., influence of probabilistic disturbance), forest types, and project portfolios. As our analysis here shows, the choice of accounting approach will influence the estimated flux generated by each project. By extension, the choice of accounting approach by a market or registry will encourage a certain type or configuration of project, and it is helpful to understand the types of activities, forest types, or management regimes that will be either encouraged or impeded by these choices. For example, the greater annual average mitigation estimates calculated for short-term projects under decay and discounted decay approaches suggests that use of these metrics could in-turn implicitly encourage shorter projects as well as projects that could be more susceptible to free ridership and non-additionality (Dutschke, 2002; van Kooten, 2009).
While the presence of incentives to manage for carbon storage may increase the aggregate carbon storage across a given landscape relative to what it might have been otherwise (Marland et al., 2001), attributing this aggregate increase to the actions of individual landowners remains a complicated undertaking. As one reviewer points out, the sale of carbon adds to the already-complex management landscape being considered by forest landowners. Stand-level analyses like the one conducted here are incapable of capturing these broader landscape- or market-level dynamics, questions that are better suited for forest sector economic models (e.g., Latta et al., 2011). What can be said, however, is that the increased offering of shorter commitment periods has the potential to attract new entrants into the carbon market, introducing or prioritizing active management considerations on additional tracts (Markowski-Lindsay et al., 2011; Galik et al., 2013) and further complicating efforts to project the net individual and aggregate mitigation they achieve.
In this way, we acknowledge that our analysis does not comprehensively review the full accounting approach of new market models like those being offered by NCX, ACR, the Family Forest Carbon Program and others, and necessarily only focuses on a narrow set of permanence considerations and calculations. There may be feedback between project permanence considerations and leakage rates, for example (e.g., Fearnside, 2009), or between project distribution and reversal risk (e.g., Cooley et al., 2012). The ability of the remotely sensed data to assist to calculate additional carbon mitigation at the landscape level may depend on a certain level of market penetration given the potential for free-ridership (e.g., Dutschke, 2002). Further research should attempt such integrated analysis of these new offset market models at both individual and landscape scales to assess the potential for real, immediate—and necessary—forest carbon storage.
The original contributions presented in this study are included in the article/Supplementary material, further inquiries can be directed to the corresponding author.
CG performed the analysis. CG drafted the initial version of the manuscript and all authors collectively revised. All authors conceived of the analysis, interpreted the results, and approved the submitted version.
This study was partially funded by the Forest Carbon Quantification Consortium.
The authors declare that the research was conducted in the absence of any commercial or financial relationships that could be construed as a potential conflict of interest.
All claims expressed in this article are solely those of the authors and do not necessarily represent those of their affiliated organizations, or those of the publisher, the editors and the reviewers. Any product that may be evaluated in this article, or claim that may be made by its manufacturer, is not guaranteed or endorsed by the publisher.
The Supplementary Material for this article can be found online at: https://www.frontiersin.org/articles/10.3389/ffgc.2022.933020/full#supplementary-material
American Carbon Registry [ACR] (2021). Methodology for the quantification, monitoring, reporting and verification of greenhouse gas emissions reductions and removals from small non-industrial private forestlands. Version 1.0. Arlington, VA: American Carbon Registry.
Austin, K., Baker, J. S., Sohngen, B. L., Wade, C., Ragnauth, S., and Ohrel, S. (2020). The economic costs of planting, preserving, and managing the world’s forests for climate change mitigation. Nat. Commun. 11:5946. doi: 10.1038/s41467-020-19578-z
Bigsby, H. (2009). Carbon banking: Creating flexibility for forest owners. For. Ecol. Manage. 257, 378–383. doi: 10.1016/j.foreco.2008.09.018
Boscolo, M., Buongiorno, J., and Panayotou, T. (1997). Simulating options for carbon sequestration through improved management of a lowland tropical forest. Environ. Dev. Econ. 2, 241–263. doi: 10.1017/S1355770X97000028
Brandão, M., Levasseur, A., Kirschbaum, M. U., Weidema, B. P., Cowie, A. L., Jørgensen, S. V., et al. (2013). Key issues and options in accounting for carbon sequestration and temporary storage in life cycle assessment and carbon footprinting. Int. J. Life Cycle Assess. 18, 230–240. doi: 10.1007/s11367-012-0451-6
Chay, F., Badgley, G., Martin, K., Freeman, J., Hamman, J., and Cullenward, D. (2022). Unpacking ton-year accounting. Available online at: https://carbonplan.org/research/ton-year-explainer (accessed March 18, 2022).
Chomitz, K. M. (1998). Baselines for greenhouse gas reductions: Problems, precedents, solutions. Washington, DC: Development research Group, World Bank.
Conference of Parties 26 [COP26] (2021). Glasgow leaders’ declaration on forests and land use. Available online at: https://ukcop26.org/glasgow-leaders-declaration-on-forests-and-land-use/ (accessed November 20, 2021).
Cooley, D. M., Galik, C. S., Holmes, T. P., Kousky, C., and Cooke, R. M. (2012). Managing dependencies in forest offset projects: Toward a more complete evaluation of reversal risk. Mitig. Adapt. Strateg. Glob. Change 17, 17–24. doi: 10.1007/s11027-011-9306-x
Cullenward, D., Chay, F., and Badgley, G. (2022). A critique of NCX’s carbon accounting methods. Available online at: https://carbonplan.org/blog/ton-year-ncx (last accessed March 18, 2022).
de Coninck, H., Revi, A., Babiker, M., Bertoldi, P., Buckeridge, M., Cartwright, A., et al. (2018). “Strengthening and implementing the global response,” in Global warming of 1.5°C. An IPCC special report on the impacts of global warming of 1.5°C above pre-industrial levels and related global greenhouse gas emission pathways, in the context of strengthening the global response to the threat of climate change, sustainable development, and efforts to eradicate poverty, eds V. Masson-Delmotte, P. Zhai, H. O. Pörtner, D. Roberts, J. Skea, P. R. Shukla, et al. (Cambridge: Cambridge University Press). 313–443.
Dornburg, V., and Marland, G. (2008). Temporary storage of carbon in the biosphere does have value for climate change mitigation: A response to the paper by Miko Kirschbaum. Mitig. Adapt. Strateg. Glob. Change 13, 211–217. doi: 10.1007/s11027-007-9113-6
Dutschke, M. (2002). Fractions of permanence–squaring the cycle of sink carbon accounting. Mitig. Adapt. Strateg. Glob. Change 7, 381–402. doi: 10.1023/A:1024775814723
Fearnside, P. M. (1997). Monitoring needs to transform Amazonian forest maintenance into a global-warming mitigation option. Mitig. Adapt. Strateg. Glob. Change 2, 285–302. doi: 10.1007/BF02437209
Fearnside, P. M. (2002). Why a 100-year time horizon should be used for global warming mitigation calculations. Mitig. Adapt. Strateg. Glob. Change 7, 19–30. doi: 10.1023/A:1015885027530
Fearnside, P. M. (2008). On the value of temporary carbon: A comment on Kirschbaum. Mitig. Adapt. Strateg. Glob. Change 13, 207–210. doi: 10.1007/s11027-007-9112-7
Fearnside, P. M. (2009). Carbon benefits from Amazonian forest reserves: Leakage accounting and the value of time. Mitig. Adapt. Strateg. Glob. Change 14, 557–567. doi: 10.1007/s11027-009-9174-9
Fearnside, P. M., Lashof, D. A., and Moura-Costa, P. (2000). Accounting for time in mitigating global warming through land-use change and forestry. Mitig. Adapt. Strateg. Glob. Change 5, 239–270. doi: 10.1023/A:1009625122628
Foley, T. G., Richter, D. D., and Galik, C. S. (2009). Extending rotation age for carbon sequestration: A cross-protocol comparison of North American forest offsets. For. Ecol. Manage. 259, 201–209. doi: 10.1016/j.foreco.2009.10.014
Galik, C. S., and Cooley, D. M. (2012). What makes carbon work? A sensitivity analysis of factors affecting forest offset viability. For. Sci. 58, 540–548. doi: 10.5849/forsci.11-046
Galik, C. S., Cooley, D. M., and Baker, J. S. (2012). Analysis of the production and transaction costs of forest carbon offset projects in the USA. J. Environ. Manage. 112, 128–136. doi: 10.1016/j.jenvman.2012.06.045
Galik, C. S., Mobley, M. L., and Richter, D. D. (2009). A virtual “field test” of forest management carbon offset protocols: The influence of accounting. Mitig. Adapt. Strateg. Glob. Change 14, 677–690. doi: 10.1007/s11027-009-9190-9
Galik, C. S., Murray, B. C., and Mercer, D. E. (2013). Where is the carbon? Carbon sequestration potential from private forestland in the Southern United States. J. For. 111, 17–25. doi: 10.5849/jof.12-055
Groom, B., and Venmans, F. (2022). The social value of offsets. Res. Square. [preprint]. doi: 10.21203/rs.3.rs-1515075/v1
Haya, B., Cullenward, D., Strong, A. L., Grubert, E., Heilmayr, R., Sivas, D. A., et al. (2020). Managing uncertainty in carbon offsets: Insights from California’s standardized approach. Clim. Policy 20, 1112–1126. doi: 10.1080/14693062.2020.1781035
IPCC (2022). “Climate change 2022: mitigation of climate change,” inContribution of Working Group III to the Sixth Assessment Report of the Intergovernmental Panel on Climate Change, eds P. R. Shukla, J. Skea, R. Slade, A. Al Khourdajie, R. van Diemen, D. McCollum, et al. (Cambridge: Cambridge University Press).
Jørgensen, S. V., and Hauschild, M. Z. (2013). Need for relevant timescales when crediting temporary carbon storage. Int. J. Life Cycle Assess. 18, 747–754. doi: 10.1007/s11367-012-0527-3
Juutinen, A., Ahtikoski, A., Lehtonen, M., Mäkipää, R., and Ollikainen, M. (2018). The impact of a short-term carbon payment scheme on forest management. For. Policy Econ. 90, 115–127. doi: 10.1016/j.forpol.2018.02.005
Kirschbaum, M. U. F. (2003). Can trees buy time? An assessment of the role of vegetation sinks as part of the global carbon cycle. Clim. Change 58, 47–71. doi: 10.1023/A:1023447504860
Kirschbaum, M. U. F. (2006). Temporary carbon sequestration cannot prevent climate change. Mitig. Adapt. Strateg. Glob. Change 11, 1151–1164. doi: 10.1007/s11027-006-9027-8
Korhonen, R., Pingoud, K., Savolainen, I., and Matthews, R. (2002). The role of carbon sequestration and the tonne-year approach in fulfilling the objective of climate convention. Environ. Sci. Policy 5, 429–441. doi: 10.1016/S1462-9011(02)00091-6
Latta, G., Adams, D. M., Alig, R. J., and White, E. (2011). Simulated effects of mandatory versus voluntary participation in private forest carbon offset markets in the United States. J. For. Econ. 17, 127–141. doi: 10.1016/j.jfe.2011.02.006
Latta, G. S., Sjølie, H. K., and Solberg, B. (2013). A review of recent developments and applications of partial equilibrium models of the forest sector. J. For. Econ. 19, 350–360. doi: 10.1016/j.jfe.2013.06.006
Levasseur, A., Brandão, M., Lesage, P., Margni, M., Pennington, D., Clift, R., et al. (2012a). Valuing temporary carbon storage. Nat. Clim. Change 2, 6–8. doi: 10.1038/nclimate1335
Levasseur, A., Lesage, P., Margni, M., Brandão, M., and Samson, R. (2012b). Assessing temporary carbon sequestration and storage projects through land use, land-use change and forestry: Comparison of dynamic life cycle assessment with ton-year approaches. Clim. Change 115, 759–776. doi: 10.1007/s10584-012-0473-x
Lintunen, J., Laturi, J., and Uusivuori, J. (2016). How should a forest carbon rent policy be implemented? For. Policy Econ. 69, 31–39. doi: 10.1098/rstb.2012.0160
Mallapragada, D. S., and Mignone, B. K. (2020). A theoretical basis for the equivalence between physical and economic climate metrics and implications for the choice of Global Warming Potential time horizon. Clim. Change 158, 107–124. doi: 10.1007/s10584-019-02486-7
Maréchal, K., and Hecq, W. (2006). Temporary credits: A solution to the potential non-permanence of carbon sequestration in forests? Ecol. Econ. 58, 699–716. doi: 10.1016/j.ecolecon.2005.08.017
Markowski-Lindsay, M., Stevens, T., Kittredge, D. B., Butler, B. J., Catanzaro, P., and Dickinson, B. J. (2011). Barriers to Massachusetts forest landowner participation in carbon markets. Ecol. Econ. 71, 180–190. doi: 10.1016/j.ecolecon.2011.08.027
Marland, G. (1988). The prospect of solving the CO2 problem through global reforestation. Springfield, VA: U.S. Department of Energy, Office of Energy Research, Carbon Dioxide Research Division.
Marland, G., Fruit, K., and Sedjo, R. (2001). Accounting for sequestered carbon: The question of permanence. Environ. Sci. Policy 4, 259–268. doi: 10.1016/S1462-9011(01)00038-7
Marland, G., Schlamadinger, B., and Leiby, P. (1997). Forest/biomass mitigation strategies: Does the timing of carbon reductions matter? Crit. Rev. Environ. Sci. Technol. 27, S213–S226. doi: 10.1080/10643389709388521
Marshall, L., and Kelly, A. (2010). The time value of carbon and carbon storage: Clarifying the terms and the policy implications of the debate. WRI Working Paper. Washington, DC: World Resources Institute, 23. doi: 10.2139/ssrn.1722345
Moura-Costa, P., and Wilson, C. (2000). An equivalence factor between CO2 avoided emissions and sequestration—descriptions and applications in forestry. Mitig. Adapt. Strateg. Glob. Change 5, 51–60. doi: 10.1023/A:1009697625521
Murray, B. C., McCarl, B. A., and Lee, H. C. (2004). Estimating leakage from forest carbon sequestration programs. Land Econ. 80, 109–124. doi: 10.2307/3147147
O’Hare, M., Plevin, R. J., Martin, J. I., Jones, A. D., Kendall, A., and Hopson, E. (2009). Proper accounting for time increases crop-based biofuels’ greenhouse gas deficit versus petroleum. Environ. Res. Lett. 4:024001. doi: 10.1088/1748-9326/4/2/024001
Parisa, Z. (2021). Explaining business as usual in carbon credits. Available online at: https://blog.ncx.com/explaining-business-as-usual-in-carbon-credits/ (accessed September 26, 2021).
Parisa, Z., Marland, E., Sohngen, E., Marland, G., and Jenkins, J. (2021). The time value of carbon storage. Available online at: https://www.researchsquare.com/article/rs-966946/v1 (accessed November 20, 2021). doi: 10.21203/rs.3.rs-966946/v1
Parisa, Z., Nova, M., and Vermeer, G. (2020). Forests and carbon: A guide for buyers and policymakers. Version 0.4.
Pearson, T. R., Brown, S., and Andrasko, K. (2008). Comparison of registry methodologies for reporting carbon benefits for afforestation projects in the United States. Environ. Sci. Policy 11, 490–504. doi: 10.1016/j.envsci.2008.06.004
Ruseva, T., Hedrick, J., Marland, G., Tovar, H., Sabou, C., and Besombes, E. (2020). Rethinking standards of permanence for terrestrial and coastal carbon: Implications for governance and sustainability. Curr. Opin. Environ. Sustain. 45, 69–77. doi: 10.1016/j.cosust.2020.09.009
Sarofim, M. C., and Giordano, M. R. (2018). A quantitative approach to evaluating the GWP timescale through implicit discount rates. Earth Syst. Dyn. 9, 1013–1024. doi: 10.5194/esd-2018-6
Sedjo, R. A. (1989). Forests: A tool to moderate global warming? Environment 31, 14–20. doi: 10.1080/00139157.1989.9929929
van Kooten, G. C. (2009). Biological carbon sequestration and carbon trading re-visted. Clim. Change 95, 1–2. doi: 10.1007/s10584-009-9572-8
van Kooten, G. C., Grainger, A., Ley, E., Marland, G., and Solberg, B. (1997). Conceptual issues related to carbon sequestration: Uncertainty and time. Crit. Rev. Environ. Sci. Technol. 27, S65–S82. doi: 10.1080/10643389709388510
Verra (2022). Verra defers updates to the VCS program. Available online at: https://verra.org/verra-defers-updates-to-the-vcs-program/ (accessed July 19, 2022).
Keywords: offsets, permanence, climate change mitigation, carbon sequestration, ton-year
Citation: Galik CS, Baker JS, Daigneault A and Latta G (2022) Crediting temporary forest carbon: Retrospective and empirical perspectives on accounting options. Front. For. Glob. Change 5:933020. doi: 10.3389/ffgc.2022.933020
Received: 30 April 2022; Accepted: 25 July 2022;
Published: 12 August 2022.
Edited by:
Gary Bull, University of British Columbia, CanadaReviewed by:
Patrick O’Connor, The University of Adelaide, AustraliaCopyright © 2022 Galik, Baker, Daigneault and Latta. This is an open-access article distributed under the terms of the Creative Commons Attribution License (CC BY). The use, distribution or reproduction in other forums is permitted, provided the original author(s) and the copyright owner(s) are credited and that the original publication in this journal is cited, in accordance with accepted academic practice. No use, distribution or reproduction is permitted which does not comply with these terms.
*Correspondence: Christopher S. Galik, Y3NnYWxpa0BuY3N1LmVkdQ==
Disclaimer: All claims expressed in this article are solely those of the authors and do not necessarily represent those of their affiliated organizations, or those of the publisher, the editors and the reviewers. Any product that may be evaluated in this article or claim that may be made by its manufacturer is not guaranteed or endorsed by the publisher.
Research integrity at Frontiers
Learn more about the work of our research integrity team to safeguard the quality of each article we publish.