- 1Department of Ecosystem and Conservation Sciences, University of Montana, Missoula, MT, United States
- 2Smithsonian Tropical Research Institute, Panama City, Panama
- 3Smithsonian Institution Forest Global Earth Observatory, Washington, DC, United States
Large areas of the Panama Canal Watershed have been converted to monocultures of teak (Tectona grandis), a non-native timber species that is generally not providing hoped-for economic and ecological benefits of Forest Landscape Restoration. Enrichment planting offers a potential strategy for revitalizing these underperforming plantations through the addition of high-value, native species to the understory, but more information is needed to guide implementation and management in this region and other tropical areas. We assessed the performance of six promising native species (Byrsonima crassifolia, Dalbergia retusa, Dipteryx oleifera, Hyeronima alchorneoides, Platymiscium pinnatum, Terminalia amazonia) as an enrichment planting in teak plantations, and specifically considered how light availability, crowding pressure and annual fertilization affected seedling performance, we measured survival and growth for the first 30 months post-planting for ∼3,000 seedlings; half received annual fertilization and half did not. We found that growth rate did not significantly affect survival among- or within-species, except for a positive relationship for D. oleifera. Overall seedling survival was high (83%), and, while species varied widely, there was not a strong effect of light, crowding or fertilization on survival. In contrast, overall growth of species was significantly affected by these factors. Across all species growth was negatively related to crowding and positively related to light availability and fertilization. There were among-species differences; while all but one species (D. oleifera) were negatively affected by crowding, only half responded positively to light availability (D. retusa, P. pinnatum, and B. crassifolia) and fertilization (D. retusa, P. pinnatum, and T. amazonia). Our findings suggest that all study species except for B. crassifolia, which suffered unacceptably high mortality, have high potential for use in enrichment planting in Panama teak plantations. Among-species differences in response to fertilization and growing environment highlight the need for continued studies to establish specific silvicultural guidelines for species in the enrichment planting context.
Introduction
Planted forests are a major component of ongoing forest landscape restoration (FLR) initiatives that seek to strategically increase tree cover in degraded or deforested landscapes (e.g., Lamb et al., 2012; Dave et al., 2019; Brancalion and Holl, 2020; Korhonen et al., 2020). Plantation forestry has been heralded as a “win-win” that both provides opportunity for rural landowners to generate income and also preserves the ecosystem services of natural forest cover (FSC, 2012; Kissinger et al., 2012), but these potential benefits are not always realized. Factors that limit the socio-economic benefits of plantations for low-resource producers include insecure land rights and long return times on initial financial investments (Brown et al., 2008; Brancalion et al., 2012; Sinacore et al., 2022), as well lack of technical support and access to markets (Shyamsundar et al., 2018). Ecologically, plantations can contribute to a heterogeneous landscape (Barlow et al., 2007) and increase per-area productivity to facilitate conservation and minimize over-harvesting of natural forests in other areas (Parrotta et al., 1997; Paquette and Messier, 2010), as well as enhancing benefits of natural forests by buffering edges and increasing connectivity amongst forest fragments (Brockerhoff et al., 2008). However, plantation establishment and management practices are critical factors in determining ecological benefits (Aubin et al., 2008; Paquette and Messier, 2010), and active management of plantation understory may be necessary to encourage a future forest trajectory that is similar to reference plant communities in natural forest (Parrotta et al., 1997).
Incorporating a diversity of native tree species can increase the ecological and social value of planted forests in comparison to exotic monocultures that are still widespread, throughout the tropics (Payn et al., 2015; Messier et al., 2021). Enrichment planting is an evidence-based, low-risk strategy for incorporating native species and increasing both ecological and economic value of timber plantations (Ashton et al., 1997; Paquette et al., 2006; Millet et al., 2012; Ouédraogo et al., 2014). In enrichment planting, valuable species are planted into the understory of already-established plantations or natural forests (Paquette et al., 2009). Potential ecological benefits of enrichment plantings include increasing structural diversity and facilitating natural regeneration (Lamb et al., 2005; Griscom and Ashton, 2011). Economically, land managers can benefit through staggered harvest rotations: combining early seral species, which are a quicker return on investment due to fast growth rates, with late successional species, which have longer harvest rotation, allow for several commercial harvests instead of just one and thereby provide a more sustained income with lower economic risks (Piotto et al., 2004; Lamb et al., 2005). Additionally, enrichment planting in already-established plantations lowers startup costs and requires less additional maintenance labor (Keefe et al., 2012).
The widespread adoption of enrichment planting and integration of native species into FLR are limited by critical knowledge gaps (Paquette et al., 2006; Hall et al., 2011a; Schneider et al., 2014). There is a lack of information on species-specific performance in plantation or enrichment planting settings (Wishnie et al., 2007; Schweizer and Brancalion, 2020), as well as best practices for overstory management and fertilization. More specifically there is a gap in knowledge regarding the extent to which the performance of individual planted trees depends on the neighborhood environment in which it is growing. Interactions among pre-established vegetation and newly-planted seedlings can range from facilitation, through amelioration of harsh growing conditions and increased nutrient availability, to suppression through competition for above- and below-ground resources (Kelty and Cameron, 1995; Forrester et al., 2006; Rappaport and Montagnini, 2014). Overstory density has been shown to negatively affect enrichment planting growth, but species differ in their response to levels of crowding (Ramos and del Amo, 1992; Ashton et al., 1997; Forrester et al., 2005). While the partially shaded conditions of an enrichment planting setting can improve seedling survival of later-succession species when compared with full-sun conditions (Ashton et al., 1997; Cole et al., 2011), these same shaded conditions may inhibit the growth of more heliotropic species (Hooper et al., 2002). This variation among species, or niche differentiation, can allow for more effective utilization of available light, water and nutrients in a mixed species plantation than in a homogenous monocultures (Kelty and Cameron, 1995), but improper species and site combinations can also suppress growth of desired species (Loreau and Hector, 2001; Redondo-Brenes and Montagnini, 2006). Quantifying the effects of crowding from neighboring trees on species targeted for enrichment plantings can improve understanding of appropriate planting densities in mixed plantations, and additional assessment of light availability can help disentangle shade responses from below-ground resource competition (Canham et al., 2004).
There is also limited information on post-establishment fertility requirements of native trees in plantations. A one-time, localized application of inorganic Nitrogen- Phosphorus-Potassium (N-P-K) fertilizer during or shortly-after planting is a common practice (Smethurst, 2010) that has been shown to positively affect establishment and initial growth for a variety of tree species across different regions and plantation systems, including timber production in the tropics (Chamshama and Hall, 1987; Cochran et al., 1991; Wang et al., 2008; Oskarsson and Brynleifsdóttir, 2009; Cicek et al., 2010). In addition to the initial fertilizer treatment, some managers use regular application of inorganic N-P-K in the years following planting; the efficacy of these regular applications has not been well studied and results have been mixed. While continued fertilization has been shown to benefit some widely commercialized timber species (Giardina et al., 2003; Albaugh et al., 2004; Coyle et al., 2016), response to increased nutrient availability differs widely and unpredictably among species and depending on site conditions (McDonald et al., 2003; Carpenter et al., 2004; Stape et al., 2008; Smethurst, 2010). Given the cost of fertilization and low profit margins for plantation forests, information on how this practice impacts the growth of native tree species is critical. Furthermore, excessive N fertilization of plantations can result in potentially negative ecological consequences such as leaching (Georgiadis et al., 2017), acidification (Wright et al., 2011; Santiago et al., 2012), and decreased soil microbial activity (Treseder, 2008).
The Panama Canal Watershed (PCW) - a nexus of social, economic, cultural and ecological interest - is an important focus area for improved plantation management and, therefore, an ideal case study in FLR. The key hydrologic functions of forests (van Noordwijk et al., 2017; Zhang et al., 2017; Adamowicz et al., 2019; Peña-Arancibia et al., 2019) are especially crucial in the PCW, where seasonal high- and low-flows routinely threaten Canal operations (Ibáñez et al., 2002). Furthermore, natural forests of the PCW have a “sponge-effect” allowing for water infiltration, which regulates both base flow during the dry season and maximum runoff during storms (Ogden et al., 2013). In addition to hydrological function, PCW forests provide other key ecosystem services including wildlife habitat and carbon sequestration (Ibáñez et al., 2002; Hall et al., 2022).
In the 1990s a number of laws incentivizing forestry in Panama (Hall et al., 2015) resulted in a flurry of commercial plantation establishment (Dale et al., 2003). From these early days to present, the vast majority of PCW plantations are monocultures of teak (Tectona grandis) - an exotic timber species that is highly valuable but ill-suited to the area’s infertile, poor-drainage clay soils (Stefanski et al., 2015). Similar to other types of exotic monoculture plantations worldwide, there is evidence that PCW teak plantations are not achieving the hoped-for economic and ecological benefits (Kraenzel et al., 2003; Cernusak et al., 2007; Fernández-Moya et al., 2014; Stefanski et al., 2015).
Studies in Central America (e.g., Piotto et al., 2010; Griess and Knoke, 2011; Sinacore et al., 2022) suggest that using native species in PCW FLR efforts can provide greater economic and ecological benefits, but more information is needed to guide implementation and management. Toward this end, this study contributes to a small but growing body of literature (e.g., Piotto et al., 2010; Hall et al., 2011a; Plath et al., 2011; Schneider et al., 2014) that seeks to evaluate the survivorship and growth performance of valuable native species in plantations. It builds on preliminary research (Marshall et al., 2020) by including a broader range of species with varying life history strategies, as well as a greater variety of teak plantation conditions. Specifically, we studied variability in the performance of six native species (Byrsonima crassifolia, Dalbergia retusa, Dipteryx oleifera, Hyeronima alchorneoides, Platymiscium pinnatum, Terminalia amazonia) valued for timber and fruit, and the factors that are predictive of these species’ performance as an enrichment planting in Panama Canal Watershed teak plantations. Our research addresses the performance of these species in an enrichment planting setting and, specifically, the following questions:
1) To what extent do seedling survival and growth (basal diameter, height, total biomass, relative growth rate) vary by species and is growth predictive of survival?
2) To what extent is seedling survival or growth (basal diameter) related to crowding, light availability, or other site conditions, and do these relationships vary by species?
3) To what extent does annual application of chemical N-P-K fertilizer affect seedling survival or growth and do results vary by species?
Materials and methods
Study site
This study was conducted in the province of Colon, Panama on the Caribbean (Eastern) side of the central Canal basin within an approximately 8-km radius (Longitude: 9°11’55”N to 9°17’02”N and Latitude: 79°41’01”W to 79°47’07”W). Six enrichment planting areas were established in existing monoculture teak (Tectona grandis) plantations (Figure 1). Two of the planting areas are privately-owned near the towns of El Limon and El Giral. The remaining four are within the 30-ha teak plantation in the Smithsonian Tropical Research Institute’s Agua Salud research site (Stallard et al., 2010), an approximately 15-km2 area near the town of Frijolito. To complement enrichment plantings and represent traditional open-grown plantation establishment conditions, an additional planting area was established in an Agua Salud pasture, and cattle were henceforth excluded from the site. Given the lack of replication, this site was not included for statistical analysis but rather as anecdotal comparison.
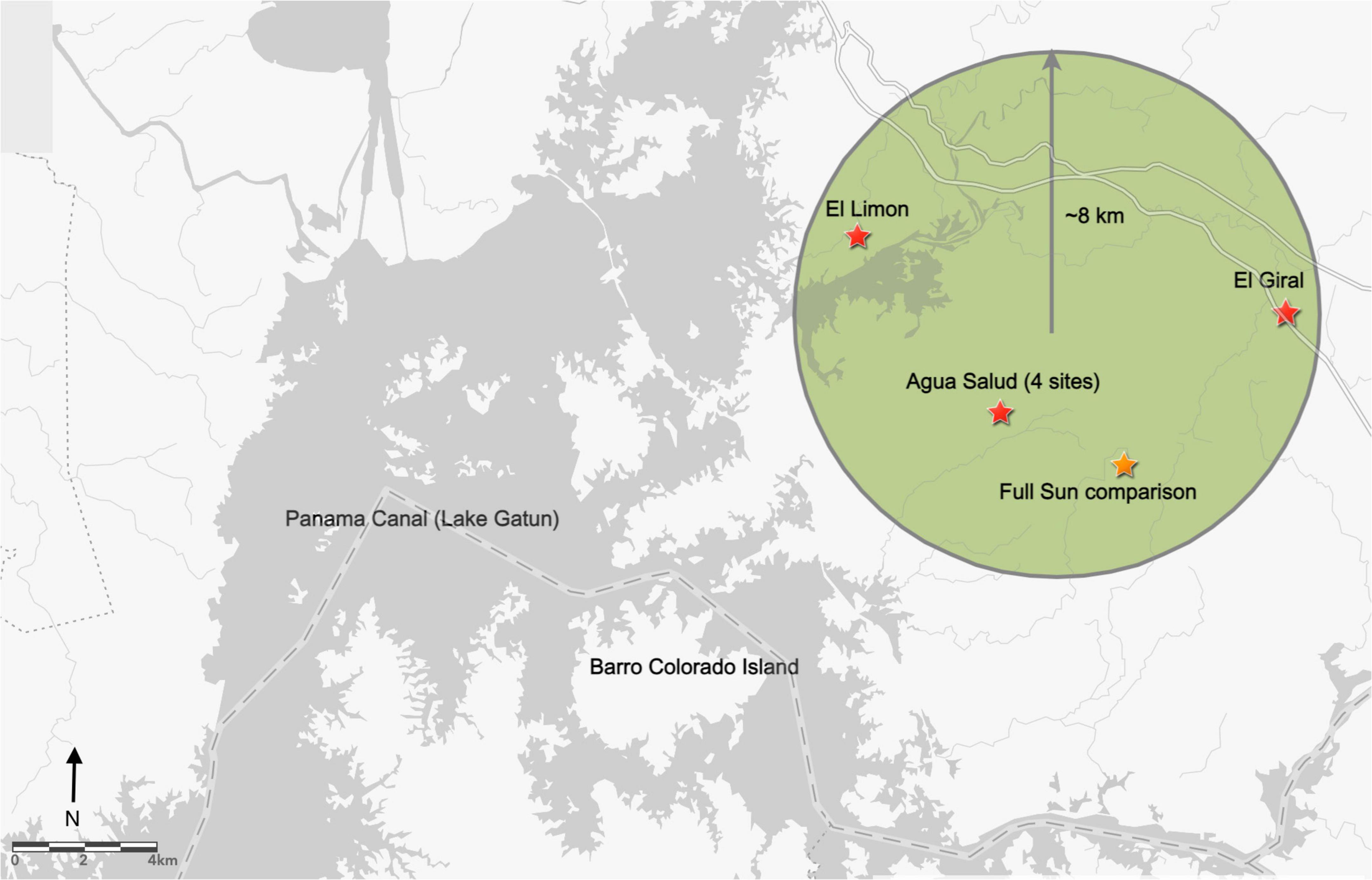
Figure 1. Study area locations (stars) in the Eastern Panama Canal watershed, within an approximately 8 km radius (Longitude: 9°11′55″N to 9°17′02″N and Latitude: 79°41′01″W to 79°47′07″W). Two planting areas are in privately owned teak plantations near the towns of El Limon and El Giral. The remaining four areas are located within the 30-ha teak plantation in the Agua Salud research site.
Climate is seasonal humid tropical and similar across planting areas. Daytime high temperature averages 32°C year-round, and annual rainfall averages 2,700 mm and typically is received almost exclusively during the wet season from May to mid-December (Ogden et al., 2013). The initial planting year of this study followed an historic El Niño drought, one of the three driest periods of the last century in the PCW (Sinacore et al., 2019). After 2016, precipitation remained within normal range for the duration of the study (Supplementary Figure 1). Planting area soils are acidic, highly-weathered Oxisol and Inceptisol clays typical of the surrounding PCW. Initial soil sampling, conducted in 2016 (prior to fertilizer treatment application), confirmed that levels and variation in mineral and macronutrient content among Agua Salud planting areas in this study was similar to variation among private plantations and that the sites are representative of low-fertility sites in the PCW (Table 1, detailed sampling protocol and methods available in Supplementary materials). Findings of extensive prior soil testing of the Agua Salud plantation are further described in publications including (Hassler et al., 2011; Mayoral et al., 2018; van Breugel et al., 2019).
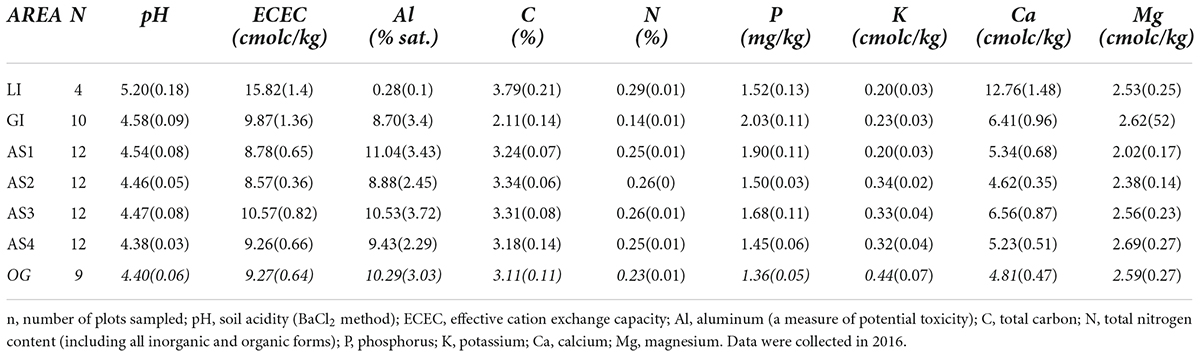
Table 1. Mean (SE) soil characteristics for all study planting areas: two located in private teak plantations (LI, Limon; GI, Giral), four located within the Agua Salud research site (AS 1–4); and the open-grown pasture comparison area (OG, italics).
Plantation characteristics
The age of teak plantations ranged from 11 to 25 years at the time of measurement in January 2019. Although teak were initially planted at a regular 3-m spacing, thinning treatments as well as mortality created variability in tree spacing in all planting areas. One year after enrichment planting stem density averaged 64% of the original planting density across all planting areas (Hall, unpublished data). The growth of teak in the Agua Salud plantations is classified as well below average, based on site index curves established for Central American teak (Keogh, 1982; Hall, 2013), and observations from the more fertile soils of the Panama Este and Darien provinces (Fennica et al., 2020). Given low growth rates, teak at Aqua Salud are not projected to be financially profitable within a 20-year rotation (Hall, 2013; Sinacore et al., 2022). In the private plantations, teak growth is similarly poor, though trees are older and thus, on-average, slightly larger (Table 2).
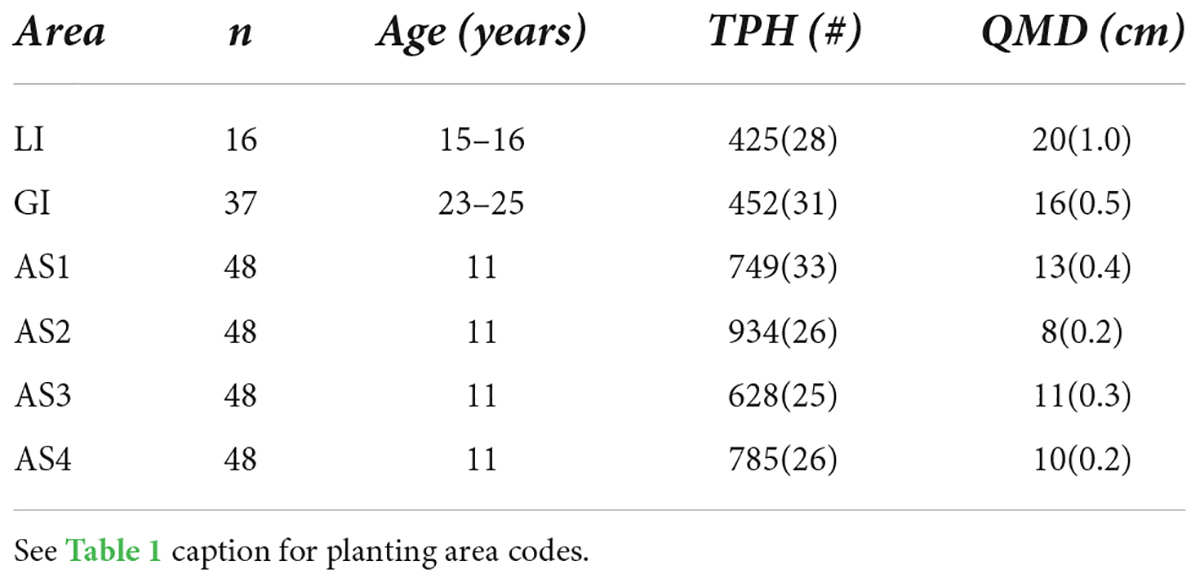
Table 2. Number of 12-seedling experimental plots (n), plantation age (Age, years after initial teak planting), mean (SE) number of teak trees per hectare (TPH) and quadratic mean diameter (QMD) of teak at the last measurement period (30-months after enrichment planting; January 2019) for all study planting areas.
Study species
The six study species were selected from a list of species with high potential or broader interest for commercial production based on existing literature and author communications with land managers (van Breugel et al., 2011; also see below) (Table 3). Species had shown high survivorship and good growth on low fertility, moist sites across a rainfall-soil fertility matrix (Hall and Ashton, 2016).
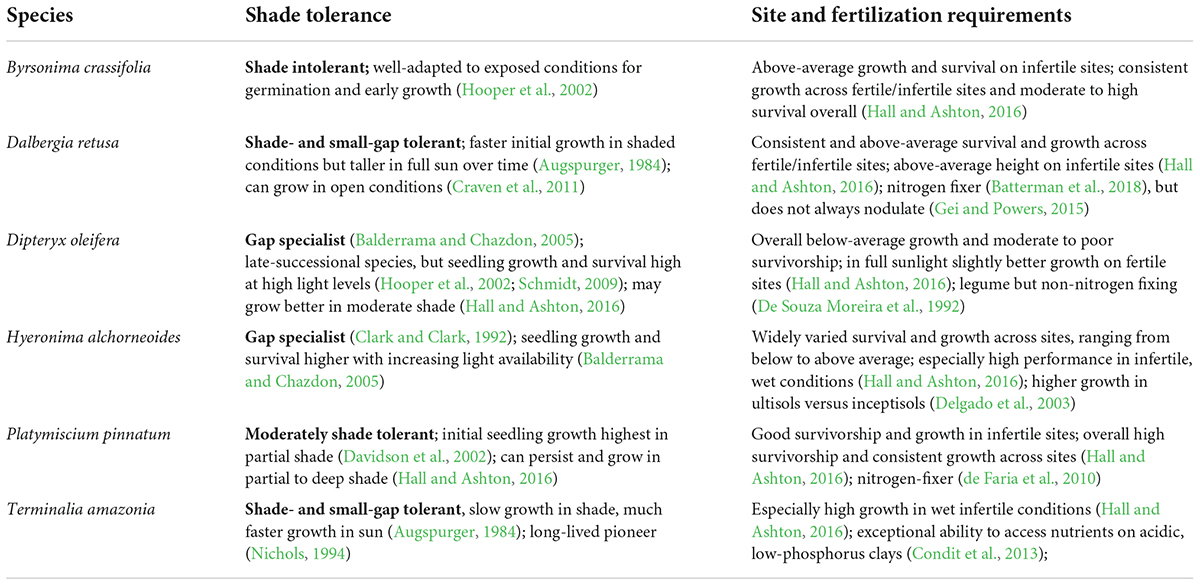
Table 3. Shade tolerance and site and fertilization requirements for each of the six study species, based on the pre-existing literature.
Byrsonima crassifolia (Malpighiaceae)
Byrsonima crassifolia, commonly known in Panama as “nance,” is a highly valued, multiuse species across its broad neotropical range, from central Mexico to central South America (Correa, 2010). There is archeological evidence of human use of the species in Panama over 4,000 years ago (Cooke and Ranere, 1992). Compounds in the bark, roots and leaves are used medicinally, and fruits are sold commercially for culinary use in desserts and drinks (Correa, 2010). The dense wood is highly valued in rural areas for firewood and small-scale construction (Love and Spaner, 2005; Garen et al., 2011), and when forests are cleared for agriculture B. crassifolia is commonly protected and left standing (Aguilar and Condit, 2001). The primary source of B. crassifolia timber and fruit is natural regeneration, although there is some planted commercial production on the Pacific coast of Mexico (Duarte, 2011). B. crassifolia is considered to be “incipiently domesticated,” as cultivated populations don’t differ genetically from wild populations (Croft, 2012). It is typically found in savanna and open pastures (Correa, 2010) and is considered to be highly shade intolerant (Hooper et al., 2002). B. crassifolia has a deep, radical root system, providing access to deeper, more consistent moisture sources and allowing the species to maintain fruit production under drought conditions (Torres et al., 2018) and also on infertile soils (van Breugel et al., 2011).
Dalbergia retusa (Fabaceae)
Of the species tested in this study, D. retusa produces the highest-value wood, and is most commonly referred to by the tradename ‘rosewood’ in reference to the distinctive scent (UNEPWCMC, 2015) and locally known as “cocobolo.” D. retusa has long been used in indigenous artisanry throughout the species’ Central American range, and is now one of the most prized woods worldwide for fine artisan work, especially as tonewood for musical instruments (Vardeman and Runk, 2020). Illegal logging has greatly decreased its abundance in natural dry tropical primary and secondary forests, prompting a CITES restriction on all Dalbergia species and listing as a vulnerable species on the IUCN Red List; it accounted for 35% of the total value of worldwide plant and animal seizures from 2005 to 2014 (UNODC, 2020). In Panama, the Emberá and Wounaan indigenous groups use the dense and rot-resistant wood for carvings and household tools, which they sell commercially, but historically avoided overharvesting by utilizing fallen limbs or roots. A surge in illegal logging, fueled by international demand and a developing high-volume trading market, led to rampant poaching from indigenous-managed forests in Eastern Panama which peaked in 2014–2015 but is ongoing despite national and international protections (Vardeman and Runk, 2020). Increasing plantation production is therefore a high priority to mitigate continued threat to natural populations. Given the high value and cultural importance of this species, Panamanian farmers have expressed especially strong interest in cultivation of D. retusa (Garen et al., 2011; Hall et al., 2011a). In a study comparing plantations of native timber species to teak in the PCW, D. retusa was far more productive than teak by all measures (Sinacore, 2018; Sinacore et al., 2022). The species consistently achieves high growth and survival across a range of environmental conditions including dry and infertile sites (Craven et al., 2013; Hall and Ashton, 2016; Soderlund, 2016), but in full-sun conditions D. retusa tends to have a wide-spreading, multi-stemmed growth form that is not ideal for timber; identifying strategies for improving growth form is a management priority (Mayoral et al., 2017). In addition to its cultural and economic importance, D. retusa is ecologically valuable as a leguminous tree species and exceptionally strong nitrogen fixer through associations with rhizobia bacteria (Batterman et al., 2018). High-nutrient leaf litter can enhance soil fertility in the surrounding forest floor (Paul et al., 2012; Gei and Powers, 2013; but see also Quesada-Ávila et al., 2021). D. retusa is also very drought tolerant (Sinacore et al., 2019); the species has high water use efficiency (Cernusak et al., 2007) and avoids drought by losing its leaves during the dry season (Sinacore et al., 2019).
Dipteryx oleifera (Fabaceae)
Dipteryx oleifera (pseudonym D. panamensis) is most commonly known as “Almendro,” Spanish for almond, in reference to its large, nutrient-dense, abundant seeds. These seeds are an important food source for many birds and mammals, and for this critical ecological role D. oleifera is considered a “keystone species” of tropical wet forests throughout Central America (Schmidt, 2009). D. oleifera is also highly valued for its beauty and high-quality, dense timber (Aguilar and Condit, 2001), which is used for construction (Tenorio et al., 2016). D. oleifera is not widely grown in plantations, and commercial harvesting from natural forests has decreased its abundance and prompted its listing as a CITES threatened species (Schmidt, 2009). Growth and survival were relatively low in previous growing trials, but in some cases high-quality compensated for low-quantity wood production in overall value estimation (Tilkia and Fisherb, 1998; Redondo-Brenes and Montagnini, 2006). D. oleifera has been found to have higher survival and growth under nurse trees versus in open-grown plantations (van Breugel et al., 2011). Despite being large seeded and considered shade tolerant, D. oleifera has displayed a strong, positive growth response to increased light and also has been observed to grow faster on high fertility sites (De Steven, 1988; Delgado et al., 2003; Balderrama and Chazdon, 2005). Though a member of the legume family, this species does not appear to form root nodules or to fix nitrogen (Montagnini, 2000).
Hyeronima alchorneoides (Euphorbiaceae)
Hyeronima alchorneoides (spelling variant Hieronyma) is one of the most widely used native species in Costa Rican commercial timber plantations (De Los Santos-Posadas et al., 2011), and is increasingly planted throughout its Central and South American range. It is a tall, canopy tree that primarily grows in low-elevation, humid forests (Flores, 1993). In Panama, the species is commonly known as “zapatero” or “pilon” and is valued in rural communities for construction and firewood (Aguilar and Condit, 2001). Its wood has high commercial value for its excellent density, workability and rot resistance (Tenorio et al., 2016), though one study found plantation-grown wood was inferior to natural sources (Roque and Leandro, 2009). While this species was the top performer in a 13-year study of native timber species in Costa Rican plantations (Ewel et al., 2015), another long-term Costa Rican plantation study found growth was below average compared to other study species (Fonseca et al., 2012).
Platymiscium pinnatum (Fabaceae)
Platymiscium pinnatum is culturally valued and a traditional timber tree in Panama, where it is commonly known as “quira” (Klitgaard, 2005). While its dense, attractive wood is commercially valuable, there is very little plantation cultivation of P. pinnatum (Fournier, 1993). Despite strong interest among Panamanian farmers in planting this species (Hall et al., 2011a), there is very little published research on its growth and survival in plantations, and the results that are available show contrasting trends: in Costa Rican plantations, P. pinnatum had relatively high survival but low growth after 5 years (Leopold et al., 2001), whereas in Ecuador it was one of the top performing species after 2 years (Villacís et al., 2016). This species is a strong candidate for enrichment planting trials due to its shade tolerance: a greenhouse study identified P. pinnatum as a later-successional species that performs best under shaded conditions rather than full light (Davidson et al., 2002). P. pinnatum has high water-use efficiency, comparable to a C4 grass (Cernusak et al., 2007). The same study also observed nodulation, suggesting nitrogen fixation, though it was less pronounced than it was on D. retusa, the other N-fixing species included in our study.
Terminalia amazonia (Combretaceae)
Terminalia amazonia has the longest legacy and most widespread current use in commercial timber plantations of the six study species (Nichols, 1994). Natural regeneration occurs in both pastures and forests, and typically in wetter sites (Hall and Ashton, 2016). This species may be less well-known outside of the regions in which it is common: in a survey of farmers in Rio Hato and Los Santos, both drier areas of Panama, the majority didn’t recognize or express interest in this species (Hall et al., 2011a), while in another study in the Las Pavas area of the PCW, a wetter climate, participants reported preferentially protecting T. amazonia during field clearing and burning (Aguilar and Condit, 2001). The straight bole and narrow crown typical of T. amazonia is excellent for timber production. The common name “Amarillo” refers to the light, yellow-colored wood that is high quality (Flores, 2009), though quality of plantation wood may be inferior to natural sources due to reduced heartwood content (Roque and Leandro, 2009). Numerous studies across Latin America have noted the exceptionally high performance of T. amazonia across a variety of site conditions (Cusack and Montagnini, 2004; Piotto, 2007), and height index curves have been established for Costa Rica (Montero et al., 2003; De Los Santos-Posadas et al., 2006). Early growth of this species is notably slower relative to other native species, but after the first couple of years T. amazonia seems to “take off” (Craven et al., 2011; Sinacore et al., 2019). In a study comparing growth in mixtures versus monocultures, T. amazonia had the highest growth of any study species and performed better in mixtures than monocultures due to lower intra-specific competition (Mayoral et al., 2017). T. amazonia’s improved performance when surrounded by smaller neighboring trees (of different species) suggests this species was sensitive to the effects of crowding. Along the same lines, T. amazonia survival was positively related to plantation spacing when grown in pure plantations (Montero et al., 2003).
Experimental design
Plot layout
Each of the four Agua Salud planting areas were subdivided into 48 contiguous, variably sized plots, with eight plots for each of the six species. Each plot was randomly assigned one of the six study species and had 12 seedlings of this species (Supplementary Figure 2), planted at least 6 m apart (to avoid among-seedling interactions) and at least 1 m away from teak trees. Where possible, plots were planted in four rows of three to form ∼18 × 24 m (ca. 432 m2) rectangular plots, but plot shape and size frequently deviated to maintain a 6-m buffer from plantation edges. Due to space constraints and landowner preference, the two planting areas that are privately owned have fewer plots and do not include all study species: the Giral plantation contains 41 plots and 5 of the 6 study species (B. crassifolia was not planted); and the Limon plantation contains 16 plots and 4 of the 6 study species (P. pinnatum and H. alchorneoides were not planted).
Planting media and protocol
All seedlings were grown in tubettes at the Futuro Forestal1 nursery in Las Lajas, in the Chiriquí province of Panama (see also Roman et al., 2012). Exact seedling age at planting time varied depending on species-specific phenology of seed production and germination requirements, but all seedlings were less than 1-year-old when outplanted. Pre-planting seedling size differed significantly among species for all measures (p-values < 0.001) (Supplementary Table 2). Immediately prior to planting, seedlings across all species had mean stem length of 34.6 ± 1.2 cm, mean root collar of 4.1 ± 0.18 mm and mean dry biomass of 2.8 ± 0.23 g.
One to two months prior to planting, understory vegetation was cut with machetes and, immediately prior to planting, a 1-m diameter circle was cleared to bare dirt around each seedling planting site. Similar clearing was repeated 2–3 times annually for the duration of the study. At the time of planting, each seedling received 90 g of 12-24-12 NPK dry, granular chemical fertilizer (10.8g N, 21.6g P, 10.8g K) and one handful of organic material (composted cow manure and forest duff), incorporated with loose dirt at the bottom of the planting hole. Planting occurred in both 2016 and 2017 due to planting stock availability. B. crassifolia, T. amazonia, D. retusa, and D. oleifera were planted in August 2016, and H. alchorneoides and P. pinnatum, for which stock was initially unavailable, were planted in July-August 2017. Only seedlings with good vigor and without evidence of foliar disease were planted. A timeline of all sampling and planting activities is provided in Supplementary Table 1.
Fertilizer treatment
For each species, half of plots were chosen to receive annual fertilization (treatment group); the remaining half received no additional fertilization following planting (control group). Assignment to fertilizer treatment or control was done by subjectively stratifying treatments across topographic conditions to ensure their even distribution throughout each planting area; in other words, we ensured that both treatment and control plots were spread across upper to lower hill positions for each species. For the fertilization treatment plots, 100 g of 12-24-12 N-P-K fertilizer was applied once annually at the start of the rainy season, beginning the first year after planting. For each planted seedling in treated plots, the fertilizer was split evenly between two ∼15 cm-deep holes dug 15–20 cm uphill from the base of the seedling and covered with a layer of soil. Given the applied nature of this study, we selected the most widely-available and commonly used fertilizer in Panama. The amount of fertilizer and method of application were determined based on personal communication with plantation managers, with the intention of testing a management strategy that could realistically be implemented given economic and labor constraints faced by land managers.
Sampling
Survival and growth of native seedlings
In order to assess survival and growth of enrichment planting seedlings, seedling status (alive or dead), height (m), and basal diameter (BD, cm) were recorded 6 months post planting and then annually during the dry season (January) from 2017 to 2020 for 2,976 seedlings in all planting areas (n = 6 species × 2 levels of fertilizer × 216 to 264 seedlings/planting area). Height was measured from the soil surface vertically to the highest point of main photosynthetic tissue, excluding leaves (Pérez-Harguindeguy et al., 2013). For each seedling with BD ≤ 5 cm, 2 BD measurements were made with digital calipers from opposite sides of the stem (to account for irregularly shaped stems) and averaged; for larger seedlings, a single measurement was made with a diameter tape. For trees with multiple stems at or below 5 cm above the root collar, the tallest stem was measured and BD was recorded below the branching point. Final measurements were taken ∼42 months post-planting (after 3 full growing season) for species planted in 2016 and ∼30 months post-planting (following 2 full growing seasons) for species planted in 2017. Analyses were done on the 30 months post-planting data only, since 42 months data were only available for a subset of species (Supplementary Table 3).
Basal diameter relative growth rate (RGR), a common and widely-used metric for growth comparison, was calculated for the time between initial and final post-planting measurements using the standard equation (Fisher, 1921):
where M1 and M2 are initial (6 months) and final (30 months) BDs, respectively, and t2-t1 is the number of years (2) between measurements.
Total biomass (TB, kg), which reflects both growth and wood density for each species, was estimated using an equation developed through direct measurement of native tree species growing in PCW plantations (Sinacore et al., 2017):
where BD is the individual BD measurement (cm) of a given seedling and WSG is the wood-specific gravity of the species as approximated based on compiled results of prior studies in the region (Zanne et al., 2009; ICRAF, 2020). This equation was developed with 6-year-old trees and its reliability for younger seedlings is unknown, but it is the best measure available for among-species comparison of same-aged seedlings.
Crowding pressure
Crowding pressure from teak overstory trees was assessed for one centrally located target seedling per plot using a 6-m fixed-radius subplot (Berger et al., 2008). Target seedlings were located in the center of the plot, so that the subplot would fall within the larger plot boundaries. For each teak within the subplot, DBH (cm) and distance (m) were measured from the target seedling. Distance was measured two ways: ground distance and slope-corrected distance. Measurements proved highly correlated (Adj R2 = 0.97) and only ground distance was used in the final analysis. Because seedlings were planted at 6-m spacing, and understory vegetation was removed, only overstory teak trees (and not other seedlings) fell within the subplot radius.
Total crowding pressure for each target seedling was calculated as
where i = 1….n neighboring teak, DBH = diameter (cm) of main teak bole at 1.35 m above soil surface, and Dist = ground distance (m) from base of target seedling to base of teak trunk closest to seedling. Crowding pressure increases with increasing DBH of each teak neighbor and decreases with increasing distance from the target seedling, and the combined effects of all neighbors provide an estimate of both below-ground and above-ground interactions (Fichtner et al., 2015). The summed effects of all neighbors was divided by 100 to keep values within the same order of magnitude of other variables for more easily-interpretable coefficient estimates.
Light availability
In order to partition the above-ground effects of crowding from below-ground effects such as competition for nutrients and water, light availability was measured for each seedling using hemispherical photography. Photos were taken with a CI-110 Plant Canopy Imager, positioned directly above seedlings at a height of 1 m, in early morning, late afternoon or during overcast weather to avoid direct sunlight. These data were collected in January 2018, when seedlings were still too short to affect light availability of one another and teak overstory had not begun to drop leaves as occurs later in the dry season on some sites. In this region of Panama teak retain their leaves for the majority of the year, and in some wetter years or locations are not deciduous, so measuring light availability with full overstory foliage was the best representation of typical conditions. CID™ Plant Canopy Image Analysis software was used to calculate the transmission coefficient for the ray penetrations (or the fraction of visible sky) used to represent PAR (Mailly, 2017). Software assessment of each photo was checked for accuracy, and incorrect classification was revised when possible or else excluded from the final analysis.
Statistical analysis
Survival and growth
Survival and growth were compared among species (Q1) using ANOVA models, with separate models for each response variable (BD, BD RGR, height and TB). ANOVA assumptions confirmed with standard diagnostic plots. Significant differences were further explored using Tukey’s Honest Significant Differences method. Survival was calculated at the plot level as the % of the 12 planted seedlings still living after 30 months. Growth was assessed as plot-level mean ± SE of each response variable at 30 months. For these analyses only seedlings in control plots (no post-planting fertilization) were used because there were significant differences in BD between fertilizer treatments for all species combined (p-value = 0.04) and for some, but not all, individual species.
The relationship between survivorship and growth was assessed using linear regression, with a model for all species as well as separate models for each species. For these and subsequent models, BD was selected as the primary response variable representing growth because it was strongly related to height (Adj R2 = 0.72, p-value < 0.001), and the height data had more error due to factors such as machete or herbivore damage, seedling position, and observer bias. RGR and TB were considered but not used as the primary response variable due to the applied nature of this study; actual, achieved growth and variation may be the most useful measure for land managers and others interested in assessing the performance of these particular species. Additionally, most seedlings were not measured at planting so RGR does not account for growth prior to the first measurement at 6 months post-planting; BD is the only direct measurement (TB was calculated with equations based on BD) that accounts for the full study period.
Effect of growing environment and fertilizer
The effects of growing environment (Q2) and fertilizer (Q3) on enrichment planting survival and growth were assessed using linear mixed effects models (LMM), with separate models for each response variable (survival and BD). LMMs for all species combined and for each species individually were constructed. For these LMMs, fertilizer treatment (two levels) and species (six levels; only in the all-species models) were included as categorical fixed effects and either crowding pressure or transmission coefficient was included as a continuous fixed effect. We did not use both crowding and transmission coefficient in the same LMMs because, due to the collinearity of these variables, neither was significant when included together in the same model (P > 0.7) (Supplementary Figure 3). Planting area (six levels) was included as a random intercept to account for the hierarchical data structure and potential among-area variation in planting conditions not captured by crowding or light measures. The importance of among-area variance was confirmed by using the likelihood-ratio test to compare LMMs with the null model containing only the fixed effects (Harrison et al., 2018). Treating planting area as a random effect allows for broader inference from these data, which aligns with this study’s goal of informing management of poorly performing teak plantations throughout the PCW. Distribution of data was examined both visually and using descriptive statistics produced with the fitdistrplus R package (Delignette-Muller, 2014) (Supplementary Figure 4).
Linear mixed effects models initially included all possible interactions among fixed effects, as other studies have found among-species differences in response to fertilizer application (i.e., Lawrence, 2003; Smethurst, 2010) and light availability (i.e., Hooper et al., 2002; Rappaport and Montagnini, 2014; Falster et al., 2018), and soil nutrient availability may affect light or crowding responses (i.e., Coates et al., 2013). Insignificant interactions were successively eliminated based on the Likelihood Ratio Test (LRT), which uses the Chi square distribution to test the significance of fixed effects and interactions. The Akaike’s information criterion (AIC) was compared for these nested models. This approach was appropriate for testing hypotheses related to the importance and potential interaction of specific fixed effects (rather than maximizing global predictive power of the entire model), given that the random effect was the same across all potential models and there were relatively few parameters (Grueber et al., 2011).
Model interpretation and validation
The explanatory power (effect size) of fixed effects within the all-species LMM and each single-species LMM was assessed by comparing the marginal R2 of models both with and without each fixed effect. In linear regression, R2 values are a standard and intuitive way of assessing the amount of variance explained by study variables, but in LMM it is important to differentiate between the Conditional R2, the overall variance explained by both fixed and mixed effects, and the Marginal R2, the variance explained by only fixed factors (Harrison et al., 2018). Said values were calculated using methods established by Nakagawa and Schielzeth (2013; see also Nakagawa et al., 2017), and the effects of each variable were portioned by comparing marginal R2 of nested models both with and without each fixed effect using the piecewise SEM R package (Lefcheck, 2016). Standard model diagnostics were conducted using the sjPlot R Package (Lüdecke, 2020) to confirm assumptions of data distribution and error structure. Final models were cross-validated using a K-fold method of resampling that accounted for hierarchical data structure: 10 mutually exclusive subsets were created using pooled data from all planting areas and including observations from each area within each subset or “fold.” Each of the 10-folds was then used as a “testing set” for models developed based on the nine remaining “training sets” (Yang and Huang, 2014).
Results
Survival and growth (Q1)
Overall mean ± SE survival at 30 months post-planting was 83 ± 2% across all control plots, all species and all planting areas. Survival varied significantly among species (p-value < 0.001), ranging from a mean of 98 ± 1% for P. pinnatum to 47 ± 3% for B. crassifolia (Table 4). With B. crassifolia excluded from analysis, the overall survival of all other enrichment planting seedlings was above 90% after 30 months. D. oleifera had the second lowest survival (72 ± 5%) of any other species. Rates of survival among the remaining 4 species were not significantly different (p-values = 0.07–0.99).
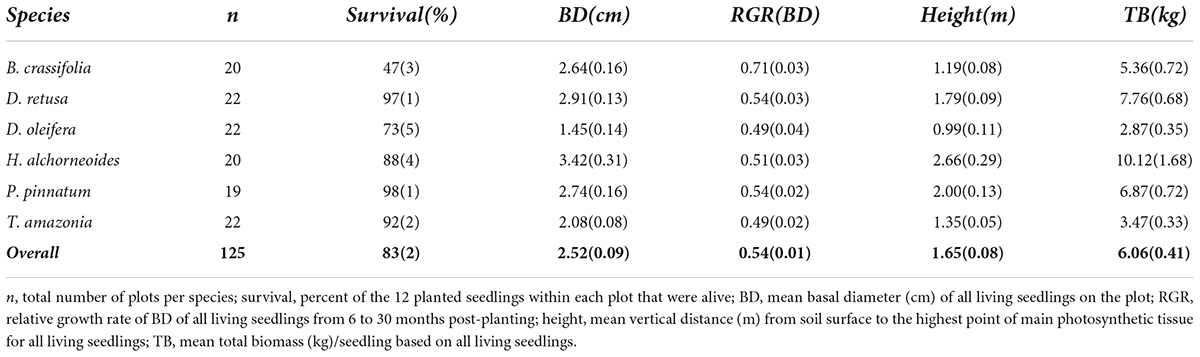
Table 4. Mean (SE) survival and growth for the six study species (and all species overall, bold) across all control (i.e., no annual fertilization) plots within all planting areas at 30 months post-planting.
Across all species and planting areas, mean ± SE BD in control plots at 30 months was 2.52 ± 0.09 cm, mean BD RGR was 0.54 ± 0.01 per year, mean height was 1.65 ± 0.08 m, and mean seedling TB was 6.06 ± 0.41 kg. There were significant among-species differences for all measures of growth (p-values all < 0.001). H. alchorneoides had the largest BD (3.42 ± 0.31 cm), height (2.66 ± 0.29 m) and seedling TB (10.12 ± 1.68 kg); D. oleifera had the smallest values of all three measures (1.45 ± 0.14 cm BD, 0.99 ± 0.11 m height, 2.87 ± 0.35 kg TB). BD RGR was significantly higher for B. crassifolia (0.71 ± 0.03 per year, p-value < 0.001) than for any other species, but did not differ significantly among the other five species (p-values = 0.16–0.99) (Figure 2).
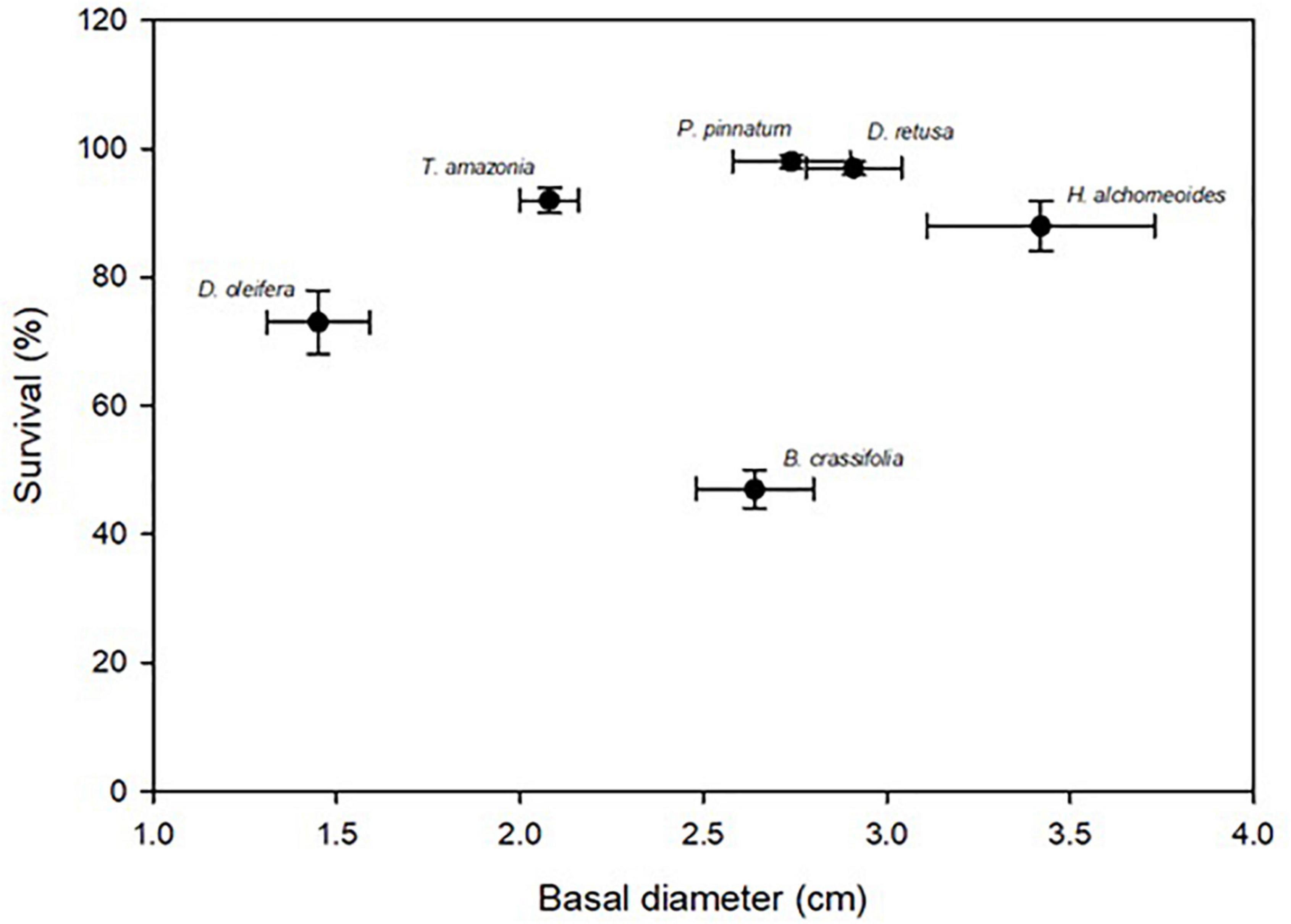
Figure 2. Mean (SE) survival (%) and growth (30-month basal diameter, cm) for the six enrichment planting species across all control (i.e., no annual fertilization) plots within all planting areas. Species names are above corresponding points. There was not a significant relationship between survival and growth across all species.
For all species combined, there was not a significant relationship between survival and growth (p-value = 0.65, Figure 2). Within species, there was a positive relationship between survival and growth for D. oleifera: plots with seedlings with greater BD also had higher survival (Adj. R2 = 0.42, p-value < 0.01). For the other five species, however, survival and growth were not significantly related (p-values = 0.09–0.25).
Effect of growing environment (Q2)
Across all planting areas, crowding pressure on individual target seedlings ranged from 0.03 to 0.61, averaging 0.26 (Supplementary Figure 5A). Crowding pressure was not predictive of survival for all species combined (p-value = 0.12) nor for any individual species (p-values = 0.06–0.86).
Across all planting areas, transmission coefficients (light availability) for individual seedlings spanned the spectrum from full sun (1.0) to highly shaded (0.03); plot-level mean transmission coefficients ranged from 0.28 to 0.83, averaging 0.51 (Supplementary Figure 5B). For all species combined, light availability was not predictive of survival (p-value = 0.88). In single-species models light availability was significantly and negatively related to survival for D. oleifera and H. alchorneoides (p-values < 0.001) but was not significantly related to survival for the remaining four species (p-values = 0.39–0.83).
Both crowding pressure and light availability explained a significant amount of variation in growth (30-month basal diameter) for all species combined and for some, but not all, species when considered separately. Crowding pressure was significantly negatively correlated with growth of all species together, but explained < 2% of variability (p-value = 0.009) (Supplementary Table 4). There was not a significant crowding x species interaction in the multispecies growth model (p = 0.1). However, in single-species models, crowding had a significant effect on growth of 5 of 6 species (D. oleifera was the exception; p-value = 0.85) (Table 5A). B. crassifolia was the most negatively impacted (p-value = 0.008): crowding explained 22% of variation in growth (after accounting for the effects of fertilizer and planting area, see below). For the remaining four species, crowding accounted for a significant but smaller percent of variation in growth: 7% for P. pinnatum, 9% for D. retusa, 10% for H. alchorneoides, and 11% for T. amazonia (p-values < 0.05) (Figure 3).
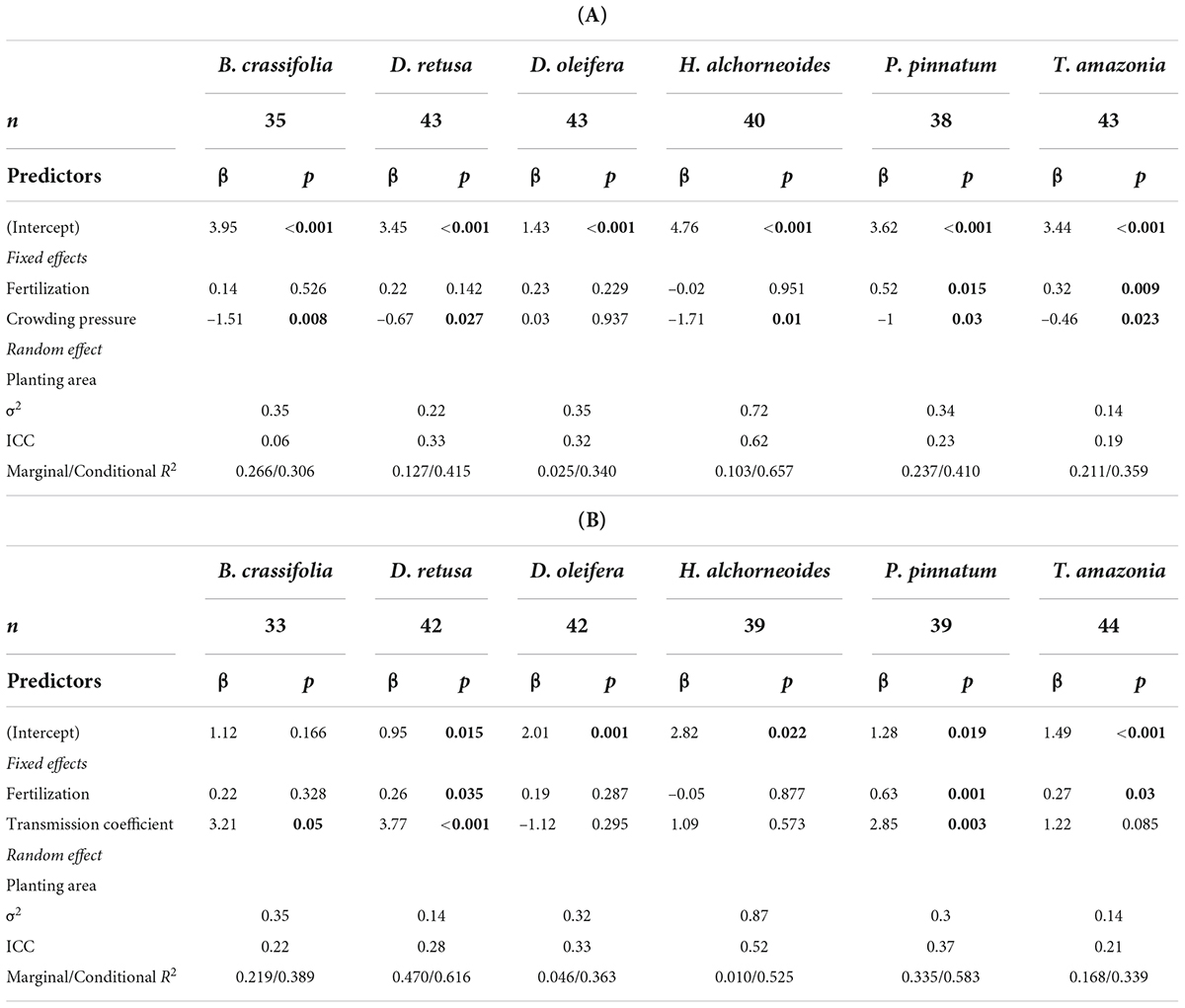
Table 5. Summary statistics for the effect of growing environment and fertilizer on BD, with separate tables for crowding pressure (A) and light availability (B). n, number of observations (plots); β, coefficient estimates; p, p-values (<0.05 bolded); σ2, random effect variance (the variance explained by planting area); ICC, intra-class correlation coefficient (the proportion of the total variance explained by the random effect); Marginal R2 is the proportion of variance in BD explained by fixed effects only and conditional R2 is the proportion of variation explained by the full model (fixed and random effects). Confidence intervals for coefficient estimates available in Supplementary Tables 5A,B.
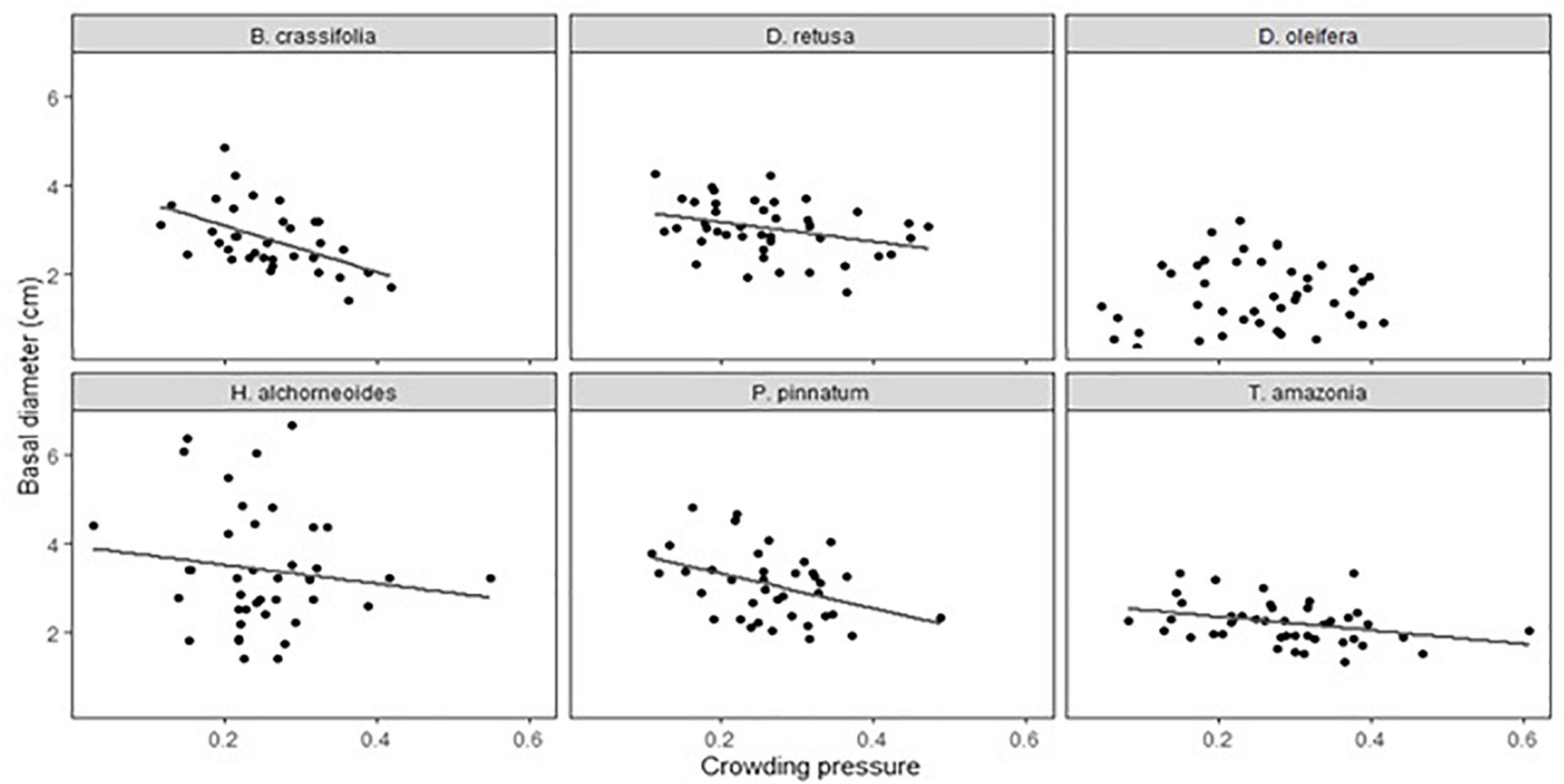
Figure 3. Effect of crowding pressure from teak on seedling growth (30-month basal diameter, cm) for each of the six enrichment planting species. Basal diameter values are plot-level means (n = 12 seedlings). Crowding pressure values are for one target seedling per plot. There were significant, negative relationships (P < 0.05) for all species except for D. oleifera.
Light availability was significantly positively correlated with growth of all species together and explained ∼2.7% of variability in growth (p-value-0.003) (Supplementary Table 4). There was a significant light availability × species interaction in the multispecies growth model (p < 0.001). For three of six species (D. oleifera, H. alchorneoides, and T. amazonia) light did not significantly affect growth (p > 0.05) (Table 5B). Light availability explained 43% of variation in growth for D. retusa, 21% for P. pinnatum, and 20% for B. crassifolia (p-values < 0.03) (Figure 4).
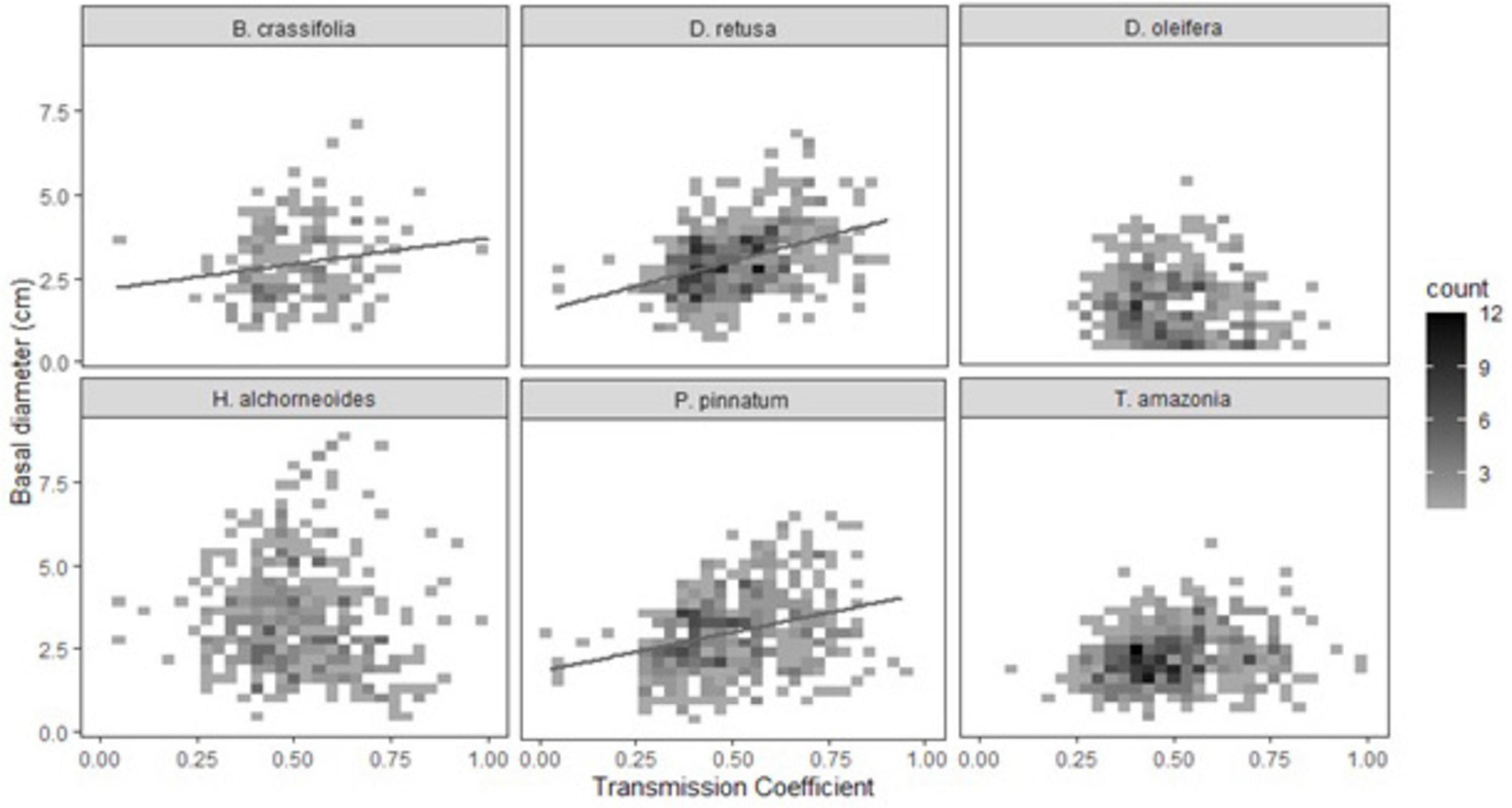
Figure 4. Effect of light availability (transmission coefficient) on seedling growth (30-month basal diameter, cm). Due to a large number of observations, data are presented as binned counts: graph area was divided into x,y grid cells (30 × 30 rectangles). Shading of each grid cell represents the number of individual seedlings (n = 1–12) with values of light availability and growth that fall within that cell; lighter shading denotes fewer seedlings and dark shading denotes a higher number of seedlings. Light availability was significantly related to growth of B. crassifolia, D. retusa, and P. pinnatum but not the other three species.
There were significant effects of planting area on enrichment planting growth not accounted for by crowding or light. For all species combined, planting area had a significant effect on growth (p-values < 0.01), but explained only a small percent of variation in the multi-species model (7 and 9% for the crowding and light models respectively) (Supplementary Table 4). The effect size of planting area varied among individual species. In individual species models, planting area was significant for five of six species when crowding was included (the exception was B. crassifolia, p-value = 0.19) (Table 5A) and for all models that included light (Table 5B). Different planting areas affected species differently: no area was the most or least productive across all species. Planting area had the largest effect for H. alchorneoides, explaining over 50% of variation in growth in both crowding and light models (p-value < 0.05). For other species, the effect was smaller, explaining between 15 and 33% of variation in growth in both the crowding and light models (p-value < 0.05). In contrast with growth, enrichment planting survival was not significantly different among planting areas after accounting for the effects of crowding and light (p-values = 0.06–0.52).
Effect of fertilization (Q3)
There was no difference in survival between fertilized and control (unfertilized) plots for all species together or for any species individually (p-values = 0.11–0.96), with the exception of B. crassifolia in the model that also included crowding (p-value = 0.023). However, fertilizer had a significant effect on growth: for all species combined, BD was 11% higher for fertilized plots versus control plots (p-value = 0.04). Growth response to fertilization differed among species (Figure 5). In individual species models, fertilization significantly affected mean BD of P. pinnatum and T. amazonia in both the models that included light and those that included crowding (p-values = 0.001–0.03) and D. retusa in the crowding model only (p-value = 0.035), but did not have a significant on mean BD of the other species (p-values = 0.14–0.95) (Table 4). BD of P. pinnatum fertilized seedlings was 22% larger on-average than control seedlings, 13% larger for T. amazonia and 8% larger for D. retusa. In the multispecies model there was a significant interaction between fertilization and light availability (p-value = 0.011).
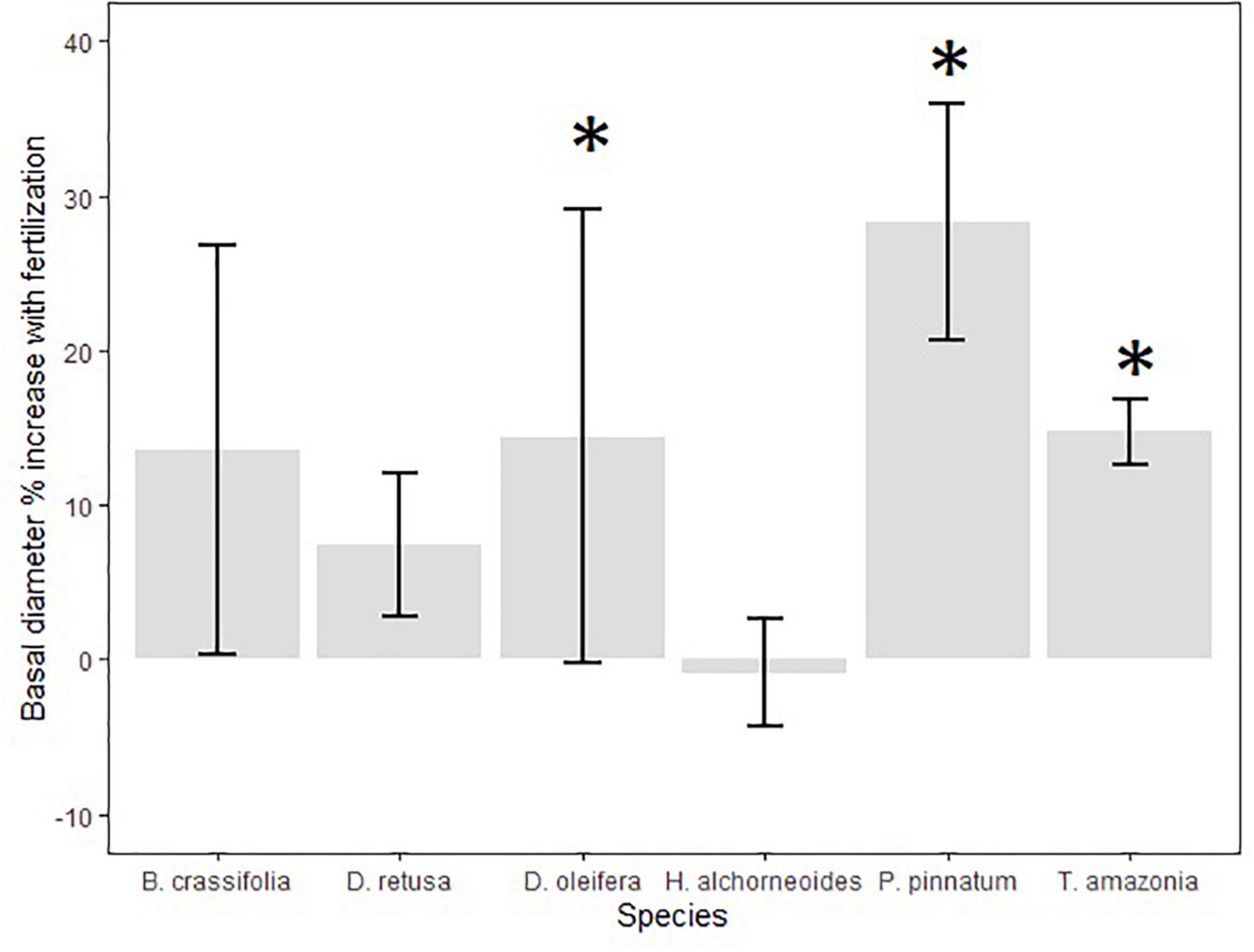
Figure 5. Mean (SE) % increase in seedling growth (30-month basal diameter, cm) between fertilizer and control treatments (calculated as [((treatment/control)-1)*100]) among all planting areas. Fertilizer treatment seedlings received applications of chemical N-P-K fertilizer once annually; control seedlings were not fertilized after planting. Annual application of chemical fertilizer significantly increased basal diameter for D. retusa, T. amazonia, and P. pinnatum (indicated with *) but not the other three species.
Discussion
Many native neotropical species have long been utilized by rural communities of Panama practicing sustained-use forestry and stewardship of natural forests (Aguilar and Condit, 2001). These species remain culturally valued today (Garen et al., 2009; Paquette and Messier, 2010; Hall et al., 2011b) and have high potential for financial profitability (Griess and Knoke, 2011; Sinacore et al., 2022), yet have been underutilized in larger-scale FLR projects in the PCW and other regions. In order to better integrate native species into FLR efforts, there is a need for information on the species and management approaches that will be financially and ecologically appropriate. Specifically, land managers need evidence on whether enrichment planting can offer a potential path forward to recoup sunk costs of already-established and underperforming teak plantations. Our results indicate that native timber species are capable of establishing and growing in an enrichment planting setting within teak plantations, aligning with more recent research (Wolfe et al., 2015) that challenge longstanding dogma that teak plantations inhibit understory growth (Healey and Gara, 2003). Among-species differences in responses to crowding, light availability and fertilization highlight the continued need for species-specific silvicultural information to inform enrichment planting projects.
Survival and growth (Q1)
All of the study species were selected for their relatively high survival in growth trials and other previous studies (e.g., Park et al., 2010; van Breugel et al., 2011; Hall and Ashton, 2016), and overall they achieved similarly high survival in the enrichment planting context (with the exception of B. crassifolia and, to a lesser extent, D. oleifera). Variation in conditions across sites and planting years makes growth comparison to previous studies difficult, but overall the early results are promising. While average growth of enrichment planting species is far below, for example, projected 3-year growth for teak in Panama on fertile sites (Ross, 2013), the wood of these species is similar to far greater in financial value (Schmidt, 2009; Tenorio et al., 2016; Vardeman and Runk, 2020). Furthermore, adding in these trees as an enrichment planting to an already-established plantation minimized startup costs and labor (Keefe et al., 2012). The additional ongoing maintenance of these trees, clearing understory plants around the base, was likely lower than in the traditional open-grown establishment conditions due to shading from teak overstory (Hooper et al., 2002). A full financial assessment is beyond the scope of this study, but early results suggest that the addition of high-value native species could change the profitability of underperforming teak plantations such as the Agua Salud plantation, which was not projected to financially break even prior to enrichment planting (Hall, 2013; Sinacore et al., 2022).
In the same planting year, an open-grown site (previously a cattle pasture) was established as a comparison planting (Supplementary Table 6). Though, due to lack of replication, we were unable to test for differences in growth between the enrichment plantings and this open-grown planting area, we anecdotally noted that survival was 8% lower, but 30-month BD was 24% higher in the open grown site compared to the enrichment planting areas. This trade-off in growth versus survival has been widely documented in enrichment planting (i.e., Forrester et al., 2005; Paquette et al., 2006; Redondo-Brenes and Montagnini, 2006), and future research should include a replicated open-grown comparison site to investigate present observations.
In the restoration context, growth is often used as a proxy for fitness and the fastest-growing species and individuals are often assumed to have the highest survival (Craven et al., 2009; Charles et al., 2018). However, we did not find a significant relationship between survival and growth among species. The species that achieved the highest BD growth after 30 months, H. alchorneoides, ranked 4th in survival while the smallest species in absolute growth, D. oleifera, ranked 5th. The species with the lowest survival (B. crassifolia) had the highest RGR but the two species that tied for 2nd highest RGR (P. pinnatum and D. retusa) had the 1st and 2nd highest survival, respectively. And within individual species, the only significant relationship between survival and growth was a positive correlation for D. oleifera seedlings; growth did not predict survival for individuals of any other species. This suggests that growth may not be the best predictor of fitness and practitioners should be careful to avoid overreliance on growth as a measure of plant performance. Though some level of mortality is acceptable given that pre-commercial thinning is typically necessary in plantations to avoid over-crowding. One caveat to comparing and integrating our research with existing literature relating survival and growth is that seedlings were transplanted, so these results do not reflect germination or initial establishment.
Effect of growing environment (Q2)
While crowding had a fairly consistent negative effect on growth for all but one species (D. oleifera), species responses to light availability ranged widely. Crowding reflects below-ground competition for water and nutrients in addition to light, while light availability is a measure of a strictly aboveground resource. Differences in species responses to crowding versus light suggests that these below-ground interactions may be important and that seedlings are not exclusively light limited. This aligns with a preliminary enrichment planting trial in Agua Salud teak plantations (Marshall et al., 2020) which found that crowding explained more variation in enrichment planting growth than light alone, though among-species differences in response to growing environment in the present study caution against over-generalizing. If the negative effects of crowding were driven by nutrient competition, we would expect that the species that responded positively to fertilizer would also have responded negatively to crowding, and this was not always the case. The finding that crowding effects were not explained by either light or nutrients (fertilization) points to the complexity of resource competition among forest trees.
Shade tolerance based on previous studies and conventional wisdom was not a good predictor of species responses to light availability. The three species that did not have a significant positive growth response to light spanned the range of shade tolerance from low (T. amazonia) to moderate (H. alchorneoides) to high (D. oleifera). Interestingly, across all species, trees with the highest growth were in partial shade (0.5–0.75 transmission coefficient). This aligns with previous findings that partial shade rather than fully open grown conditions can be optimal for mid- and later-successional species (Ashton, 1995; Marshall et al., 2020).
Interestingly, the effect of planting area on all species together explained less variation in growth than for most species individually, indicating that there was no universal “best” or “worst” planting area for all species. This finding speaks to the complex relationship between site conditions and the unique requirements of different species. H. alchorneoides growth was by far the most affected by planting area, whereas B. crassifolia was the least-affected, which is consistent with prior findings that this species grows well even on very harsh sites where few other tree species are found (Jimenez Madrigal, 2002). While the effect of aspect on light and moisture availability in the tropics is less important than in the Northern hemisphere (Méndez-Toribio et al., 2017), slope position and gradient can be an important factors (Mayoral et al., 2018). These factors, as well as soil nutrient status, were not assessed in our study but are valuable future research directions.
Effect of fertilization (Q3)
The observed growth increase associated with annual fertilization for some of the study species is interesting from both a management and ecological perspective. Among-species differences highlight that growth benefits from application of supplemental fertilization cannot be generalized (Xia and Wan, 2008) and should be experimentally evaluated for individual species in field conditions similar to those of management interest (Smethurst, 2010).
Strong early growth can give tree seedlings a greater competitive advantage against understory weeds (Craven et al., 2009), thus if fertilizer increases seedling growth it could potentially reduce management effort. In addition, if the positive growth response to fertilizer of D. retusa, P. pinnatum, and T. amazonia continue over time, trees that receive fertilization could be significantly larger at harvest, thereby increasing profits of these high value of these species. Furthermore, trees could reach merchantable harvest size more quickly, benefiting land managers by shortening rotation times for a faster return on investment. We intentionally tested the most affordable and widely-available chemical fertilizer (12-24-12 NPK) in order to assess whether this low-cost option was a worthwhile management investment. At the application rate of 100 g annually, the per-tree cost of fertilizing for the first 10 years is 1 kg fertilizer plus labor. Given the high-value wood of study species, very minimal growth increases would be required to break even financially on the investment.
The lack of growth increase from fertilization for half of the species in this study is a notable finding, especially given the low-fertility soils of planting areas in this study. This contrasts with the strong response to fertilizer of many exotic timber species such as teak, even in sites with more productive soils (Kumar, 2005; Smethurst, 2010; Jerez and de Andrade Coutinho, 2017). There was also no significant difference in variability in growth between seedlings in fertilized and unfertilized plots. This was surprising given that there were differences in nutrient availability among plots even within the same planting areas. If plot nutrient status was a major driver of differences in growth, one might expect to see lower variability in growth (i.e., more consistent growth) with fertilization. However, we did not find that to be the case.
The small to insignificant effect size of fertilization for study species not only provides useful and important information for the management of these particular native species, but also ties into recent research on soil nutrient limitations in tropical forests. Of the three macronutrients supplied by the chemical fertilizer, phosphorus is of particular interest given that this is generally considered to be the most limiting nutrient in tropical soils (Hedin et al., 2009) and soil testing confirmed low levels of phosphorus in this study’s planting areas. The phosphorus affinity, or growth response to phosphorus availability, of tree species has been identified locally as one of the strongest predictors of tropical forest community composition (Condit et al., 2013; Zalamea et al., 2016). Conveniently, a previous assessment of growth responses to phosphorus availability in lowland tropical forest species in Panama included five of the six study species (all except D. retusa) (Condit et al., 2013). All three species that didn’t have a significant growth response to fertilization in the present study (B. crassifolia, D. oleifera, and H. alchorneoides) were also found by Condit et al. (2013) to have below-average affinity to phosphorus. Furthermore, P. pinnatum, which had by far the largest positive response to fertilizer in this study, was found by Condit et al. (2013) to have an above-average phosphorus affinity. The positive fertilizer response of both nitrogen-fixing species, P. pinnatum and D. retusa, adds support to the focus on phosphorus (rather than nitrogen) limitation and aligns with previous findings that low levels of available phosphorous have been found to limit the growth of nitrogen-fixing trees (Batterman et al., 2013).
The significant effect of fertilizer on T. amazonia growth complicates this tidy story. According to Condit et al. (2013), this species has an especially low phosphorus affinity (i.e., grows well on sites low in phosphorus), and for other pioneer species in Panama, though not T. amazonia specifically, distribution across a natural phosphorus gradient was predictive of growth response to phosphorus (Zalamea et al., 2016). Yet in our study, T. amazonia had the second-largest positive response to fertilization. A previous study of T. amazonia response to fertilization in Costa Rica (Carpenter et al., 2004) found that, similar to this study, fertilized T. amazonia was significantly larger after 2 years. After 8 years, Carpenter et al. (2004) found there was no longer a significant difference between fertilized and unfertilized trees, though there were important differences in soils of the study site and experimental design: different from our study, Carpenter et al. (2004) only applied the fertilizer treatment for the initial 2 years post-planting. The same Costa Rica study also tested interplanting T. amazonia with nitrogen-fixing species Inga edulis and found this increased T. amazonia growth and foliar nitrogen content (Nichols and Carpenter, 2006). These findings suggest that nitrogen can be a limiting factor in T. amazonia growth, so it’s possible that the positive response to fertilization in the present study is due to additional nitrogen availability rather than to phosphorous.
A meta-analysis of growth response to fertilization in tropical seedlings (Lawrence, 2003) found that light-demanding species were more likely to respond positively to fertilization compared with shade-tolerant species. In our study, shade tolerance (based on previous studies) was not strongly predictive of fertilizer response: whereas the light-demanding species B. crassifolia had no response to fertilization, the more shade-tolerant species P. pinnatum had the greatest growth response. Regardless of a given species’ shade tolerance, the significant light availability × fertilization interaction in the multispecies model suggests that seedlings are better able to utilize additional nutrients at higher light levels. In their review of interacting above- and below-ground resource constraints, Coomes and Grubb (2000) note that the minimum light availability at which seedlings respond to increased nutrient availability varies among species and is related to both shade tolerance and response to nutrient supply. The light levels in their reviewed studies (2–45% of full daylight) fall within the spectrum of light availability tested in our study.
Performance by species
Byrsonima crassifolia
Byrsonima crassifolia had by far the lowest survival; this is different than the full-sun PRORENA growth trials, where the species achieved moderate to high survivorship (van Breugel et al., 2011). Growth of surviving individuals was strong, however: though it wasn’t the largest in absolute measures after 30 months, B. crassifolia had the highest RGR of any study species. While the cause of mortality for these seedlings was undetermined, significant foliar damage was observed suggesting that pest and disease may have played a role. Pre-planting seedlings were small and with less woody growth than other species at planting, which could help explain high early mortality but not continued high mortality throughout the study. Additionally, researchers noted prior to planting some trays and individuals showed signs of what appeared to be post-emergence damping-off; seedlings with obvious above-ground signs of disease were avoided but it’s possible that below-ground damage was undetected. When collecting root samples from second-year seedlings researchers noted large, unusual galls on B. crassifolia roots, further suggesting some type of pest. In addition, and to the great inconvenience of sampling crews, defoliation by the highly-venomous caterpillar Megalopyge opercularis was observed more frequently on B. crassifolia than any other species. Another possible explanation could be seed source: in Panama cultivated B. crassifolia is genetically indistinguishable from native populations, likely because there has been little to no artificial selection (Croft, 2012). Given this high genetic diversity and phenotypic variability of the species (Correa, 2010), it’s possible that seedlings in this study came from a population that is less vigorous than the species overall.
Byrsonima crassifolia is considered to be shade intolerant (Hooper et al., 2002) and has a lower wood density than our other study species; these characteristics are associated with a syndrome of lower survival but higher growth rate (Ruger et al., 2012), which aligns with what we observed. Given the low shade tolerance of B. crassifolia, the relatively weak response to light availability was surprising. In contrast, this species had the highest relative growth response to crowding: Reducing average crowding pressure by half would increase this species’ average 30-month BD by an estimated 23% based on coefficient estimates for single-species models. The relatively high proportion of growth variation explained by crowding pressure suggests that a resource other than light may be limiting growth of B. crassifolia, which is contrary to expectations given that this species is thought to be highly drought-tolerant (Torres et al., 2018) and performs best in infertile soils (Hall and Ashton, 2016).
Dalbergia retusa
The strong growth of D. retusa in this study (second highest BD and TB after 30 months) aligns with robust performance in a preliminary enrichment planting trial at the Agua Salud research site (Marshall et al., 2020) and other past observations (i.e., Mayoral et al., 2017); in another study comparing D. retusa and T. amazonia growth under similar full-sun, plantation conditions, D. retusa initially outperformed T. amazonia but growth slowed considerably after the first two years. This slowdown was not observed in this study: D. retusa was the tallest and had the largest TB and second-largest BD after 42-months (H. alchorneoides and P. pinnatum not included for this final measurement).
Light explained more variation in growth for D. retusa (43%) than any other potential predictor for any other species, while crowding explained far less (9%). D. retusa performed well despite low fertility and drought conditions in other studies (i.e., Craven et al., 2013; Hall and Ashton, 2016; Soderlund, 2016), which aligns with our findings that crowding pressure, which reflects competition for belowground resources including water and nutrients, had a relatively small effect. The large response to light availability when separated from other effects of crowding is harder to explain. This contrasts with findings from a preliminary enrichment planting study that D. retusa achieved highest growth at intermediate light levels (Marshall et al., 2020). One potential consideration is the branching tendency of this species and the way BD was measured: if there were multiple stems at or below 5 cm height, a single BD measurement was taken below the lowest branching point. This could explain why mean BD was lower for single-stemmed versus multi-stemmed seedlings (p-value = 0.001). We hoped that partial shade would improve growth form and indeed found that mean light availability was lower for single-stemmed trees than trees with multiple stems (i-value < 0.001), so it’s possible that the increased branching with increased light could explain some of the strong positive correlation between light and BD. Height was not related to light availability (p-value = 0.13), though interestingly multi-stemmed trees were taller on-average than single-stemmed trees (p-value < 0.001).
Dipteryx oleifera
Dipteryx oleifera performed poorly relative to other species in this study: survival was the second-lowest (after B. crassifolia) and growth was far lower than other species. Interestingly there was a positive correlation between growth and survival across plots for D. oleifera seedlings (Adj. R2 = 0.42, p-value < 0.01) but not for any other species (p-values = 0.09–0.25); it’s possible that both growth and survival were affected by a factor that was not identified in this study, such as a below-ground disease or pest. While D. oleifera was by far the smallest after 30 months, in later intervals (18–30 months and 30–42 months) RGR was above-average compared with other species. Given the slow-but-steady growth pattern of this species (Schmidt, 2009), low early survival and growth may not be indicative of longer-term performance. This species also has exceptionally high wood density (Tenorio et al., 2016), which is often associated with slower growth (Ruger et al., 2012).
The negative relationship between D. oleifera survival and light availability contrasts with previous findings that this species achieves consistent survival across varying light levels (Balderrama and Chazdon, 2005). D. oleifera was the only species that did not show a significant growth response to either light or crowding, and this contrasts with previous findings. D. oleifera is naturally occurring across a range of light conditions (De Steven, 1988; Clark and Clark, 1992; Butterfield and Mariano, 1995; Schmidt, 2009), but a previous study found that growth is maximized at higher light availability (Balderrama and Chazdon, 2005). D. oleifera responded positively to thinning treatments in single-species plantation trials (Schmidt, 2009), suggesting some sensitivity to crowding. It’s possible that the growth of D. oleifera was strongly affected by an unidentified or unmeasured factor such as disease or moisture availability, and therefore the effects of the predictors considered in this study were less evident.
Hyeronima alchorneoides
This species achieved by far the highest growth in all final values at 30 months of growth, though did not have the highest RGR. This aligns with strong performance in previous studies and, more generally, the rapid early growth strategy of emergent species (Butterfield and Mariano, 1995; Balderrama and Chazdon, 2005; Hall and Ashton, 2016). Additionally, H. alchorneoides seedlings were the largest on-average at planting, which is known to strongly benefit early establishment and growth (Riikonen and Luoranen, 2018). While H. alchorneoides is considered to have dense wood (Roque and Leandro, 2009), it was on the lower end of these study species (all selected for especially high-value timber) and the relatively higher initial growth aligns with this ranking given that denser wood is often associated with slower growth (Ruger et al., 2012).
The negative relationship between light availability and survival for H. alchorneoides contrasts with findings from a previous study in Costa Rica (Balderrama and Chazdon, 2005) that survival for this species increased at higher light levels. In another Costa Rican study, H. alchorneoides achieved consistently high survival across a spectrum of shade conditions including mature forest (Piotto, 2007). H. alchorneoides was the most affected by crowding: this explained 10% of BD growth variation and reducing average crowding pressure by half would increase average 30-month BD by an estimated 20%. In contrast, the effect of light availability was not significant. Lack of light response is consistent with a previous study in Costa Rica in which H. alchorneoides was the only species capable of sustaining growth as an enrichment planting in mature forest conditions and achieved consistently high growth in pasture, secondary and mature forest (Piotto, 2007). However, a different Costa Rican study found the opposite: H. alchorneoides growth was strongly affected by light availability (Balderrama and Chazdon, 2005).
Platymiscium pinnatum
Platymiscium pinnatum achieved the highest survival of any species and slightly above-average growth. High survival aligns with previous findings (Davidson et al., 2002; Hall and Ashton, 2016). The exceptionally dense wood of this species (Klitgaard, 2005) means that biomass accumulation is higher than for similarly-sized trees of other species. Given that high wood density is associated with lower intrinsic growth rate (Ruger et al., 2012), it’s impressive that this species achieved higher-than-average growth.
Platymiscium pinnatum growth was more strongly related to light availability than crowding, which was not entirely expected given this species is considered shade-tolerant (Davidson et al., 2002; Klitgaard, 2005). Interestingly, in the full-sun comparison site, five of the six species had lower survival but higher growth while P. pinnatum was the exception in both cases: survival was 2% higher and growth was the same for full-sun versus enrichment planting seedlings.
Terminalia amazonia
The relatively unremarkable performance of T. amazonia is in itself remarkable given the standout performance of this species in prior studies. Survival was relatively high but T. amazonia growth was below-average for all measures compared to other study species. Importantly, though, other studies have noted a rapid increase in growth rates after the initial 2 years (Craven et al., 2011; Sinacore et al., 2019). Even 42-months post-planting, however, T. amazonia growth was still below average for all species in our study.
The lack of a significant relationship between T. amazonia growth and light availability was especially surprising given that the leaf traits and natural distribution of this species are typical of a more light-demanding species (Craven et al., 2011). This is also different than preliminary enrichment planting results from Marshall et al. (2020) that show a positive relationship between light availability and T. amazonia growth. Previous research suggests that this species, while a strong competitor, is also sensitive to competitive pressure (Montero et al., 2003; Craven et al., 2009; Mayoral et al., 2017), but our results don’t support this: The negative effects of crowding on T. amazonia growth were slight, similar to P. pinnatum, D. retusa, and H. alchorneoides. Given that early growth is not indicative of longer-term trends for this species, it’s possible that the effects of light and crowding are not yet apparent for this species but will become more pronounced over time.
Conclusion
Results from this study provide critical information for land managers in need of an alternative to the largely unprofitable teak plantations that dominate the PCW (Stefanski et al., 2015) and other areas of the globe. Our hope is that these findings support increased integration of culturally, ecologically, and in some cases even economically valuable native species into PCW FLR efforts, thereby encouraging much-needed community participation (Oestreicher et al., 2009). Findings are broadly relevant, even outside the region, for informing the increasingly popular but under-researched strategy of enrichment planting and the use of native species in FLR efforts (Paquette et al., 2006; Redondo-Brenes and Montagnini, 2006; Hall et al., 2011a; Kammesheidt, 2011; Messier et al., 2021). Specifically, understanding enrichment planting dynamics in the early growth period is especially important given that teak (as is often the case with overstory species) are faster-growing and will be harvested prior to the maturation of enrichment planting species. However, while our findings are valuable for the management of early enrichment planting projects, longer-term studies are needed to assess how older, larger trees may respond to growing conditions and fertilizer, and how responses and overall performance vary among-species differences in performance. Additional studies are also necessary to understand the effect of planting year and pre-planting growing conditions. Each species in our study was assessed for a single planting and from planting stock grown by one nursery (though from varied genetic sources); year-to-year weather variation and nursery conditions are important factors that may affect performance. These studies are critical for silvicultural guidelines for species with diverse values, such as those selected for this study, and should continue to be a high priority for researchers seeking to inform FLR management in the PCW and elsewhere.
Additionally, this research provides insight into more basic ecological questions related to biotic interactions among forest trees. For example, effects of crowding pressure were not captured by aboveground dynamics (variation in light) alone: some species responded more strongly to just the aboveground effect of light availability, whereas others were most affected by the combined above- and belowground effects captured in our estimate of crowding pressure. Our study did not disentangle belowground competition for water and soil nutrients, and exploring these dynamics is a valuable area of future research for informing enrichment planting management.
The finding that higher phosphorus affinity was generally predictive of positive fertilizer response for our species (except for T. amazonia) aligns with the idea that phosphorus is the most limiting nutrient in tropical soils (Hedin et al., 2009) and a major shaping force of these forest communities (Condit et al., 2013; Zalamea et al., 2016). It will be interesting to see whether the early benefits of fertilization continue as trees mature, though even the initial positive response is an important finding for successful management of FLR projects (Craven et al., 2009; Charles et al., 2018). Longer-term measurements will give a better picture of how enrichment planting affects the financial profitability of teak plantations, as well as ecological effects of the resulting increased biodiversity.
Data availability statement
The original contributions presented in the study are included in the article/Supplementary material, further inquiries can be directed to the corresponding author/s.
Author contributions
JH conceived of the original research idea. JH and AM designed and directed the research, in consultation with CN. AM analyzed the data and wrote the manuscript. CN and JH revised and edited the manuscript. All authors contributed to the article and approved the submitted version.
Funding
This research was supported by the Hoch Family, Frank and Kristin Erickson Levinson, Stanley Motta, and the Lloyds Tercentenary Trust Research Foundation (LTRF), with additional support from ForestGEO. Additional funding was provided by scholarships from the University of Montana’s W.A. Franke College of Forestry and Conservation and Associated Students of University of Montana. The Hoch Family, Frank Levinson and Kristin Erickson Levinson, and Stanley Motta were not involved in the study design, collection, analysis, interpretation of data, the writing of this article or the decision to submit it for publication.
Acknowledgments
This work is a contribution of Smart Reforestation® and the Agua Salud Project, a collaboration between the Smithsonian Tropical Research Institute (STRI), the Panama Canal Authority (ACP) and the Ministry of the Environment of Panama (MiAmbiente). Agua Salud is part of the Smithsonian Institution Forest Global Earth Observatory (ForestGEO) and the Working Land and Seascapes program of the Smithsonian Institution Conservation Commons. We thank Dr. Anna Sala and Dr. Steve Siebert (University of Montana) for guidance on the study design and comments on a previous version of the manuscript, and Dr. David Affleck (University of Montana) for statistics consultation. We also thank the many Agua Salud field technicians, interns and crew members that assisted in the planting, monitoring, and ongoing maintenance of the study plantations and seedlings.
Conflict of interest
The authors declare that the research was conducted in the absence of any commercial or financial relationships that could be construed as a potential conflict of interest.
Publisher’s note
All claims expressed in this article are solely those of the authors and do not necessarily represent those of their affiliated organizations, or those of the publisher, the editors and the reviewers. Any product that may be evaluated in this article, or claim that may be made by its manufacturer, is not guaranteed or endorsed by the publisher.
Supplementary material
The Supplementary Material for this article can be found online at: https://www.frontiersin.org/articles/10.3389/ffgc.2022.925877/full#supplementary-material
Footnotes
References
Adamowicz, W., Calderon-Etter, L., Entem, A., and Stallard, R. (2019). Assessing ecological infrastructure investments. Proc. Natl. Acad. Sci. U.S.A. 116, 5254–5261. doi: 10.1073/pnas.1802883116
Aguilar, S., and Condit, R. (2001). Use of native tree species by an hispanic community in Panama. Econ. Bot. 55, 223–235. doi: 10.1007/BF02864560
Albaugh, T. J., Allen, H. L., Dougherty, P. M., and Johnsen, K. H. (2004). Long term growth responses of loblolly pine to optimal nutrient and water resource availability. For. Ecol. Manage. 192, 3–19. doi: 10.1016/j.foreco.2004.01.002
Ashton, M. S. (1995). Seedling growth of co-occurring Shorea species in the simulated light environments of a rain forest. For. Ecol. Manage. 72, 1–12. doi: 10.1016/0378-1127(94)03452-3
Ashton, P. M. S., Gamage, S., Gunatilleke, I. A. U. N., and Gunatilleke, C. V. S. (1997). Restoration of a sri lankan rainforest: using caribbean pine pinus caribaea as a nurse for establishing late-successional tree species. J. Appl. Ecol. 34, 915–925. doi: 10.2307/2405282
Aubin, I., Messier, C., and Bouchard, A. (2008). Can plantations develop understory biological and physical attributes of naturally regenerated forests? Biol. Conserv. 141, 2461–2476. doi: 10.1016/j.biocon.2008.07.007
Augspurger, C. K. (1984). Light requirements of neotropical tree seedlings: a comparative study of growth and survival. J. Ecol. 72, 777–795. doi: 10.2307/2259531
Balderrama, S. I. V., and Chazdon, R. L. (2005). Light-dependent seedling survival and growth of four tree species in Costa Rican second-growth rain forests. J. Trop. Ecol. 21, 383–395. doi: 10.1017/S026646740500235X
Barlow, J., Gardner, T. A., Araujo, I. S., and Peres, C. A. (2007). Quantifying the biodiversity value of tropical primary, secondary, and plantation forests. Proc. Natl. Acad. Sci. U.S.A. 104, 18555–18560. doi: 10.1073/pnas.0703333104
Batterman, S. A., Hall, J. S., Turner, B. L., Hedin, L. O., Walter, J. K. L., Sheldon, P., et al. (2018). Phosphatase activity and nitrogen fixation reflect species differences, not nutrient trading or nutrient balance, across tropical rainforest trees. Ecol. Lett. 21, 1486–1495. doi: 10.1111/ele.13129
Batterman, S. A., Wurzburger, N., and Hedin, L. O. (2013). Nitrogen and phosphorus interact to control tropical symbiotic N 2 fixation?: a test in Inga punctata. J. Ecol. 101, 1400–1408. doi: 10.1111/1365-2745.12138
Berger, U., Piou, C., Schiffers, K., and Grimm, V. (2008). Competition among plants: Concepts, individual-based modelling approaches, and a proposal for a future research strategy. Perspect. Plant Ecol. Evol. Syst. 9, 121–135. doi: 10.1016/j.ppees.2007.11.002
Brancalion, P. H. S., and Holl, K. D. (2020). Guidance for successful tree planting initiatives. J. Appl. Ecol. 57, 2349–2361. doi: 10.1111/1365-2664.13725
Brancalion, P. H. S., Viani, R. A. G., Strassburg, B. B. N., and Rodrigues, R. R. (2012). Finding the money for tropical forest restoration. Unasylva 63:239.
Brockerhoff, E. G., Jactel, H., Parrotta, J. A., Quine, C. P., and Sayer, J. (2008). Plantation forests and biodiversity: oxymoron or opportunity? Biodivers. Conserv. 17, 925–951. doi: 10.1007/s10531-008-9380-x
Brown, D., Seymour, F., and Peskett, L. (2008). “How do we achieve Redd co-benefits and avoid doing harm?,” in Moving Ahead With REDD: Issues, Options And Implications, ed. A. Angelsen (Bogor: Center for International Forestry Research (CIFOR))
Butterfield, R. P., and Mariano, E. C. (1995). Screening trial of 14 tropical hardwoods with an emphasis on species native to Costa Rica: fourth year results. New For. 9, 135–145.
Canham, C. D., LePage, P. T., and Coates, K. D. (2004). A neighborhood analysis of canopy tree competition: effects of shading versus crowding. Can. J. For. Res. 34, 778–787. doi: 10.1139/x03-232
Carpenter, F. L., Nichols, J. D., Pratt, R. T., and Young, K. C. (2004). Methods of facilitating reforestation of tropical degraded land with the native timber tree, Terminalia amazonia. For. Ecol. Manage. 202, 281–291.
Cernusak, L. A., Aranda, J., Marshall, J. D., and Winter, K. (2007). Large variation in whole-plant water-use efficiency among tropical tree species. http://www.newphytologist.orgwww.newphytologist.org. New Phytol. 173, 294–305. doi: 10.1111/j.1469-8137.2006.01913.x
Chamshama, S. A. O., and Hall, J. B. (1987). Effects of site preparation and fertilizer application at planting on Eucalyptus tereticornis at Morogoro, Tanzania. For. Ecol. Manage. 18, 103–112. doi: 10.1016/0378-1127(87)90137-X
Charles, L. S., Dwyer, J. M., Smith, T. J., Connors, S., Marschner, P., and Mayfield, M. M. (2018). Seedling growth responses to species-, neighborhood-, and landscape-scale effects during tropical forest restoration. Ecosphere 9:e02386. doi: 10.1002/ecs2.2386
Cicek, E., Yilmaz, F., and Yilmaz, M. (2010). Effect of N and NPK fertilizers on early field performance of narrow-leaved ash, Fraxinus angustifolia. Environ. Biol. 31, 109–114. doi: 10.1590/0001-3765201820150519
Clark, D. A., and Clark, D. B. (1992). Life history diversity of canopy and emergent trees in a neotropical rain forest. Ecol. Monogr. 62, 315–344. doi: 10.2307/2937114
Coates, K. D., Lilles, E. B., and Astrup, R. (2013). Competitive interactions across a soil fertility gradient in a multispecies forest. J. Ecol. 101, 806–818. doi: 10.1111/1365-2745.12072
Cochran, P. H., Newman, R. P., and Barrett, J. W. (1991). Fertilization And Spacing Effects On Growth Of Planted Ponderosa Pine. Washington, DC: US Dept. of Agriculture.
Cole, R. J. J., Holl, K. D. D., Keene, C. L. L., and Zahawi, R. A. A. (2011). Direct seeding of late-successional trees to restore tropical montane forest. For. Ecol. Manage. 261, 1590–1597. doi: 10.1016/j.foreco.2010.06.038
Condit, R., Engelbrecht, B. M. J., Pino, D., and Turner, B. L. (2013). Species distributions in response to individual soil nutrients and seasonal drought across a community of tropical trees. Proc. Natl. Acad. Sci. U.S.A. 110, 5064–5068. doi: 10.1073/pnas.1218042110
Cooke, R., and Ranere, A. J. (1992). Prehistoric human adaptations to the seasonally dry forests of panama. World Archaeol. 24, 114–133.
Coomes, D. A., and Grubb, P. J. (2000). Impacts of root competition in forests and woodlands: a theoretical framework and review of experiments. Ecol. Monogr. 70, 171–207.
Correa, A. M. D. (2010). “Byrsonima crassifolia (L.) Kunth,” in Tropical Tree Seed Manual, ed. J. A. Vozzo (Washington, DC: USDA Forest Service), 342–345.
Coyle, D. R., Aubrey, D. P., and Coleman, M. D. (2016). Growth responses of narrow or broad site adapted tree species to a range of resource availability treatments after a full harvest rotation. For. Ecol. Manage. 362, 107–119. doi: 10.1016/J.FORECO.2015.11.047
Craven, D., Cedeño, N., Mariscal, E., Deago, J., Wishnie, M., and Hall, J. S. (2011). “Amelioration of growing conditions in mixed species plantation of terminalia amazonia and nitrogen-fixing dalbergia retusa,” in Restoring Degraded Landscapes With Native Species In Latin America, eds F. Montagnini and C. Finney (New York, NY: Nova Science Publishers), 63–79.
Craven, D., Hall, J. S., and Verjans, J.-M. (2009). Impacts of herbicide application and mechanical cleanings on growth and mortality of two timber species in saccharum spontaneum grasslands of the panama canal watershed. Restor. Ecol. 17, 751–761. doi: 10.1111/j.1526-100X.2008.00408.x
Craven, D., Hall, J. S., Ashton, M. S., and Berlyn, G. P. (2013). Water-use efficiency and whole-plant performance of nine tropical tree species at two sites with contrasting water availability in Panama. Trees 27, 639–653. doi: 10.1007/s00468-012-0818-0
Croft, G. K. M. (2012). Evolution And Ecology During Domestication In The Neotropical Fruit Tree, Byrsonima Crassifolia (Malpighiaceae). St. Louis, MO: Washington University.
Cusack, D., and Montagnini, F. (2004). The role of native species plantations in recovery of understory woody diversity in degraded pasturelands of Costa Rica. For. Ecol. Manage. 188, 1–15. doi: 10.1016/S0378-1127(03)00302-5
Dale, V. H., Brown, S., Calderón, M. O., Montoya, A. S., and Martínez, R. E. (2003). Estimating baseline carbon emissions for the eastern Panama Canal watershed. Mitig. Adapt. Strateg. Glob. Chang. 8, 323–348. doi: 10.1023/B:MITI.0000005613.85127.3f
Dave, R., Saint-Laurent, C., Murray, L., Daldegan, G. A., Brouwer, R., De Mattos, S., et al. (2019). Second Bonn Challenge Progress Report. Gland: IUCN.
Davidson, R., Mauffette, Y., and Gagnon, D. (2002). Light requirements of seedlings: a method for selecting tropical trees for plantation forestry. Basic Appl. Ecol. 3, 209–220.
de Faria, S. M., Diedhiou, A. G., de Lima, H. C., Ribeiro, R. D., Galiana, A., Castilho, A. F., et al. (2010). Evaluating the nodulation status of leguminous species from the Amazonian forest of Brazil. J. Exp. Bot. 61, 3119–3127. doi: 10.1093/jxb/erq142
De Los Santos-Posadas, H. M., Montero-Mata, M., and Kanninen, M. (2006). Dynamic dominant height growth curves for Terminalia amazonia (Gmel.) excell in costa rica. Agrociencia 40, 521–532.
De Los Santos-Posadas, H. M., Montero-Mata, M., Valdez-Lazalde, J. R., and Kanninen, M. (2011). “Productivity, growth, and timber yield of hyeronima alchorneoides allemao (pilón) plantations in costa rica,” in Restoring Degraded Landscapes With Native Species In Latin America, eds F. Montagnini and C. Finney (New York, NY: Nova Science Publishers), 97–112.
De Souza Moreira, F. M., da Silva, M. F., and De Earia, S. M. (1992). Occurrence of nodulation in legume species in the Amazon region of Brazil. New Phytol. 121, 563–570. doi: 10.1111/j.1469-8137.1992.tb01126.x
De Steven, D. (1988). Light gaps and long-term seedling performance of a Neotropical canopy tree (Dipteryx panamensis, Leguminosae). J. Trop. Ecol. 4, 407–411. doi: 10.1017/S0266467400003084
Delgado, A., Montero, M., Murillo, O., and Castillo, M. (2003). Crecimiento de especies forestales nativas en la zona norte de Costa Rica. Agron. Costarric. 27, 63–78.
Delignette-Muller, M. L. (2014). Fitdistrplus: an r package for fitting distributions. J. Stat. Softw. 64, 1–34.
Duarte, O. (2011). “Nance (byrsonima crassifolia (L.) kunth),” in Postharvest Biology and Technology of Tropical and Subtropical Fruits, Volume 4 – Mangosteen to White Sapote, ed. E. M. Yahia (Sawston: Woodhead Publishing Limited), 44–50. doi: 10.1016/j.foodres.2018.05.063
Ewel, J. J., Celis, G., and Schreeg, L. (2015). Steeply increasing growth differential between mixture and monocultures of tropical trees. Biotropica 47, 162–171. doi: 10.1111/btp.12190
Falster, D. S., Duursma, R. A., and FitzJohn, R. G. (2018). How functional traits influence plant growth and shade tolerance across the life cycle. Proc. Natl. Acad. Sci. U.S.A. 115, E6789–E6798. doi: 10.1073/pnas.1714044115
Fennica, S., Seppänen, P., and Mäkinen, A. (2020). Comprehensive yield model for plantation teak in Panama. Silva Fenn. 54:25. doi: 10.14214/sf.10309
Fernández-Moya, J., Alvarado, A., Forsythe, W., Ramírez, L., Algeet-Abarquero, N., and Marchamalo-Sacristán, M. (2014). Soil erosion under teak (Tectona grandis L.f.) plantations: general patterns, assumptions and controversies. Catena 123, 236–242. doi: 10.1016/j.catena.2014.08.010
Fichtner, A., Forrester, D. I., Härdtle, W., Sturm, K., and von Oheimb, G. (2015). Facilitative-competitive interactions in an old-growth forest: the importance of large-diameter trees as benefactors and stimulators for forest community assembly. PLoS One 10:e0120335. doi: 10.1371/journal.pone.0120335
Fisher, R. A. (1921). Some remarks on the methods formulated in a recent article on “the quantitative analysis of plant growth.”. Ann. Appl. Biol. 7, 367–372. doi: 10.1111/j.1744-7348.1921.tb05524.x
Flores, E. M. (1993). “Hyeronima alchorneoides Allemão,” in Arboles y semillas del neotropico = Trees and seeds from the neotropics (San Jose: Museo Nacional de Costa Rica), 514–517.
Flores, E. M. (2009). “Terminalia amazonia,” in Arboles y semillas del neotropico = Trees and seeds from the neotropics (San Jose: Museo Nacional de Costa Rica), 748–751.
Fonseca, W., Alice, F. E., and Rey-Benayas, J. M. (2012). Carbon accumulation in aboveground and belowground biomass and soil of different age native forest plantations in the humid tropical lowlands of Costa Rica. New For. 43, 197–211. doi: 10.1007/s11056-011-9273-9
Forrester, D. I., Bauhus, J., and Cowie, A. L. (2005). On the success and failure of mixed-species tree plantations: lessons learned from a model system of Eucalyptus globulus and Acacia mearnsii. For. Ecol. Manage. 209, 147–155. doi: 10.1016/J.FORECO.2005.01.012
Forrester, D. I., Bauhus, J., Cowie, A. L., and Vanclay, J. K. (2006). Mixed-species plantations of eucalyptus with nitrogen-fixing trees: a review. For. Ecol. Manage. 233, 211–230. doi: 10.1016/j.foreco.2006.05.012
Fournier, L. A. (1993). “Platymiscium pinnatum (Jacq.) dugand,” in Arboles y semillas del neotropico = Trees and seeds from the neotropics (San Jose: Museo Nacional de Costa Rica), 643–644. doi: 10.1093/treephys/tpp052
Garen, E. J., Saltonstall, K., Ashton, M. S., Slusser, J. L., Mathias, S., and Hall, J. S. (2011). The tree planting and protecting culture of cattle ranchers and small-scale agriculturalists in rural panama: opportunities for reforestation and land restoration. For. Ecol. Manage. 261, 1684–1695. doi: 10.1016/j.foreco.2010.10.011
Garen, E. J., Saltonstall, K., Slusser, J. L., Mathias, S., Ashton, M. S., and Hall, J. S. (2009). An evaluation of farmers’ experiences planting native trees in rural Panama: implications for reforestation with native species in agricultural landscapes. Agrofor. Syst. 76, 219–236. doi: 10.1007/s10457-009-9203-4
Gei, M. G., and Powers, J. S. (2013). Do legumes and non-legumes tree species affect soil properties in unmanaged forests and plantations in Costa Rican dry forests? Soil Biol. Biochem. 57, 264–272. doi: 10.1016/j.soilbio.2012.09.013
Gei, M. G., and Powers, J. S. (2015). The influence of seasonality and species effects on surface fine roots and nodulation in tropical legume tree plantations. Plant Soil 388, 187–196. doi: 10.1007/s11104-014-2324-1
Georgiadis, P., Taeroe, A., Stupak, I., Kepfer-Rojas, S., Zhang, W., Bastos, R. P., et al. (2017). Fertilization effects on biomass production, nutrient leaching and budgets in four stand development stages of short rotation forest poplar. For. Ecol. Manage. 397, 18–26. doi: 10.1016/J.FORECO.2017.04.020
Giardina, C., Ryan, M., Binkley, D., and Fownes, J. (2003). Primary production and carbon allocation in relation to nutrient supply in a tropical experimental forest. Glob. Chang Biol. 9, 1438–1450.
Griess, V. C., and Knoke, T. (2011). Can native tree species plantations in panama compete with teak plantations? An economic estimation. New For. 41, 13–39. doi: 10.1007/s11056-010-9207-y
Griscom, H. P., and Ashton, M. S. (2011). Restoration of dry tropical forests in central america: a review of pattern and process. For. Ecol. Manage. 261, 1564–1579. doi: 10.1016/j.foreco.2010.08.027
Grueber, C. E., Nakagawa, S., Laws, R. J., and Jamieson, I. G. (2011). Multimodel inference in ecology and evolution: challenges and solutions. J. Evol. Biol. 24, 699–711. doi: 10.1111/j.1420-9101.2010.02210.x
Hall, J. S. (2013). Growth and development of the Agua Salud teak (Tectona grandis) plantation four growing seasons post-establishment. Panamá: Smithsonian Tropical Research Institute.
Hall, J. S., and Ashton, M. S. (2016). Guide to Early Growth and Survival in Plantations of 64 Tree Species Native to Panama and the Neotropics. Panamá city: Smithsonian Tropical Research Institute.
Hall, J. S., Ashton, M. S., Garen, E. J., and Jose, S. (2011a). The ecology and ecosystem services of native trees: Implications for reforestation and land restoration in Mesoamerica. For. Ecol. Manage. 261, 1553–1557. doi: 10.1016/j.foreco.2010.12.011
Hall, J. S., Cerezo, A., and Entem, A. (2015). “Managing the Panama canal watershed,” in Managing watersheds for ecosystem services in the steepland neotropics. Inter-American development bank monograph, Vol. 340, eds J. S. Hall, V. Kirn, and E. Yanguas-Fernandez (Panama City: Inter-American Development Bank), 92–106.
Hall, J. S., Love, B. E., Slusser, J. L., et al. (2011b). Tree plantations on farms: evaluating growth and potential for success. For. Ecol. Manage. 261, 1675–1683. doi: 10.1016/J.FORECO.2010.09.042
Hall, J. S., Plisinski, J. S., Mladinich, S. K., van Breugel, M., Lai, H. R., Asner, G. P., et al. (2022). Deforestation scenarios show the importance of secondary forest for meeting Panama’s carbon goals. Landsc. Ecol. 37, 673–694. doi: 10.1007/s10980-021-01379-4
Harrison, X. A., Donaldson, L., Correa-Cano, M. E., Evans, J., Fisher, D. N., Goodwin, C. E. D., et al. (2018). A brief introduction to mixed effects modelling and multi-model inference in ecology. PeerJ 6:e4794. doi: 10.7717/peerj.4794
Hassler, S. K., Zimmermann, B., van Breugel, M., Hall, J. S., and Elsenbeer, H. (2011). Recovery of saturated hydraulic conductivity under secondary succession on former pasture in the humid tropics. For. Ecol. Manage. 261, 1634–1642. doi: 10.1016/j.foreco.2010.06.031
Healey, S. P., and Gara, R. I. (2003). The effect of a teak (Tectona grandis) plantation on the establishment of native species in an abandoned pasture in Costa Rica. For. Ecol. Manage. 176, 497–507.
Hedin, L. O., Brookshire, E. N. N. J. N. J., Menge, D. N. L. L., and Barron, A. R. (2009). The nitrogen paradox in tropical forest ecosystems. Annu. Rev. Ecol. Evol. Syst. 40, 613–635. doi: 10.1146/annurev.ecolsys.37.091305.110246
Hooper, E., Condit, R., and Legendre, P. (2002). Responses of 20 native tree species to reforestation strategies for abandoned farmland in Panama. Ecol. Appl. 12, 1626–1641. doi: 10.2307/3099927
Ibáñez, R., Condit, R., Angehr, G., Aguilar, S., García, T., Martínez, R., et al. (2002). An ecosystem report on the panama canal: monitoring the status of the forest communities and the watershed. Environ. Monit. Assess. 80, 65–95. doi: 10.1023/A:1020378926399
Jerez, M., and de Andrade Coutinho, S. (2017). “Establishment and management of planted teak forests,” in The Global Teak Study. Analysis, Evaluation and Future Potential of Teak Resources, eds W. Kollert and M. Kleine (Vienna: International Union of Forest Research Organizations), 49–65. doi: 10.1094/PDIS-03-20-0641-PDN
Jimenez Madrigal, Q. (2002). Arboles Maderables De Costa Rica : Ecologia Y Silvicultura = Timber Trees Of Costa Rica?: Ecology And Silviculture. Santo Domingo: InBio, Instituto Nacional de Biodiversidad.
Kammesheidt, L. (2011). Guest editorial planting native quality timber trees in South-East Asia: Pipedream or lucrative business? J. Trop. For. Sci. 23, 355–357.
Keefe, K., Alavalapati, J. A. A., and Pinheiro, C. (2012). Is enrichment planting worth its costs? A financial cost–benefit analysis. For. Policy Econ. 23, 10–16. doi: 10.1016/j.forpol.2012.07.004
Kelty, M., and Cameron, I. R. (1995). Plot designs for the analysis of species interactions in mixed stands. Commonw. For. Rev. 322–332.
Keogh, R. M. (1982). Teak (Tectona grandis Linn. f.) provisional site classification chart for the Caribbean, Central America, Venezuela and Colombia. For. Ecol. Manage. 4, 143–153. doi: 10.1016/0378-1127(82)90011-1
Kissinger, G., Herold, M., and De Sy, V. (2012). Drivers of deforestation and forest degradation: A Synthesis Report for REDD+ Policymakers. Vancouver, BC: Lexeme Consulting.
Klitgaard, B. B. (2005). Ptatymiscium (Leguminosae: Dalbergieae): biogeography systematics, morphology, taxonomy and uses. Kew Bull. 60, 321–400.
Korhonen, J., Nepal, P., Prestemon, J. P., and Cubbage, F. W. (2020). Projecting global and regional outlooks for planted forests under the shared socio-economic pathways. New For. 52, 197–216. doi: 10.1007/s11056-020-09789-z
Kraenzel, M., Castillo, A., Moore, T., and Potvin, C. (2003). Carbon storage of harvest-age teak (Tectona grandis) plantations Panama. For. Ecol. Manage. 173, 213–225. doi: 10.1016/S0378-1127(02)00002-6
Kumar, B. M. (2005). “Sustainable teak plantations in the tropics: the question of nutrient management,” in Proceedings of the International Conference: Quality Timber Products Of Teak From Sustainable Forest Management, eds K. M. Bhat, K. K. N. Nair, K. V. Bhat, E. M. Muralidharan, and J. K. Sharma (Peechi: Kerala Forest Research Institute), 179–187.
Lamb, D., Erskine, P. D., and Parrotta, J. A. (2005). Restoration of degraded tropical forest landscapes. Science 310, 1628–1632.
Lamb, D., Stanturf, J., and Madsen, P. (2012). “What is forest landscape restoration?,” in BT – Forest Landscape Restoration: Integrating Natural and Social Sciences, eds J. Stanturf, D. Lamb, and P. Madsen (Dordrecht: Springer Netherlands), 3–23.
Lawrence, D. (2003). The response of tropical tree seedlings to nutrient supply: meta-analysis for understanding a changing tropical landscape. J. Trop. Ecol. 19, 239–250. doi: 10.1017/S0266467403003274
Lefcheck, J. S. (2016). PiecewiseSEM: piecewise structural equation modelling in R for ecology, evolution, and systematics. Methods Ecol. Evol. 7, 573–579. doi: 10.1111/2041-210X.12512
Leopold, A. C., Andrus, R., Finkeldey, A., and Knowles, D. (2001). Attempting restoration of wet tropical forests in Costa Rica. For. Ecol. Manage. 142, 243–249. doi: 10.1016/S0378-1127(00)00354-6
Loreau, M., and Hector, A. (2001). Partitioning selection and complementarity in biodiversity experiments. Nature 412, 72–76. doi: 10.1038/35083573
Love, B., and Spaner, D. (2005). A survey of small-scale farmers using trees in pastures in herrera province, panama. J. Sustain. For. 20, 37–65. doi: 10.1300/J091v20n03_03
Lüdecke, D. (2020). Data Visualization for Statistics in Social Science [R Package Sjplot Version 2.8.6]. Available online at: http://CRAN.R-Project.org/package=sjPlot
Mailly, D. (2017). “Hemispherical photography in support of forest inventory and silviculture,” in BT – Hemispherical Photography in Forest Science: Theory, Methods, Applications, eds R. A. Fournier and R. J. Hall (Dordrecht: Springer Netherlands), 227–252. doi: 10.1002/eap.1691
Marshall, A., McLaughlin, B. P., Zerr, C., Yanguas-Fernández, E., and Hall, J. S. (2020). Early indications of success rehabilitating an underperforming teak (Tectona grandis) plantation in Panama through enrichment planting. New For. 52, 377–395. doi: 10.1007/s11056-020-09801-6
Mayoral, C., van Breugel, M., Cerezo, A., and Hall, J. S. (2017). Survival and growth of five Neotropical timber species in monocultures and mixtures. For. Ecol. Manage. 403, 1–11. doi: 10.1016/j.foreco.2017.08.002
Mayoral, C., van Breugel, M., Turner, B., Asner, G. P., Vaughn, N. R., and Hall, J. S. (2018). Effect of microsite quality and species composition on tree growth: a semi empirical modeling approach. For. Ecol. Manage. 432, 534–545. doi: 10.1016/j.foreco.2018.09.047
McDonald, M. A., Hofny-Collins, A., Healey, J. R., and Goodland, T. C. R. (2003). Evaluation of trees indigenous to the montane forest of the Blue Mountains, Jamaica for reforestation and agroforestry. For. Ecol. Manage. 175, 379–401.
Méndez-Toribio, M., Ibarra-Manríquez, G., Navarrete-Segueda, A., and Paz, H. (2017). Topographic position, but not slope aspect, drives the dominance of functional strategies of tropical dry forest trees. Environ. Res. Lett. 12:085002.
Messier, C., Bauhus, J., Sousa-Silva, R., Auge, H., Baete, L., Barsoum, N., et al. (2021). For the sake of resilience and multifunctionality, let’s diversify planted forests! Conserv. Lett. 15:e12829. doi: 10.1111/conl.12829
Millet, J., Tran, N., Vien Ngoc, N., Tran Thi, T., and Prat, D. (2012). Enrichment planting of native species for biodiversity conservation in a logged tree plantation in Vietnam. New For. 44, 369–383. doi: 10.1007/s11056-012-9344-6
Montagnini, F. (2000). Accumulation in above-ground biomass and soil storage of mineral nutrients in pure and mixed plantations in a humid tropical lowland. For. Ecol. Manage. 134, 257–270. doi: 10.1016/S0378-1127(99)00262-5
Montero, M., Kanninen, M., Montero, M., and Kanninen, M. (2003). Índice de sitio para terminalia amazonia en Costa Rica. Agron. Costarric. 27, 29–35.
Nakagawa, S., and Schielzeth, H. (2013). A general and simple method for obtaining R2 from generalized linear mixed-effects models. Methods Ecol. Evol. 4, 133–142. doi: 10.1111/j.2041-210x.2012.00261.x
Nakagawa, S., Johnson, P. C. D., and Schielzeth, H. (2017). The coefficient of determination R2 and intra-class correlation coefficient from generalized linear mixed-effects models revisited and expanded. J. R. Soc. Interface 14:20170213. doi: 10.1098/rsif.2017.0213
Nichols, J. D. (1994). Terminalia amazonia (Gmel.) exell: development of a native species for reforestation and agroforestry. Commonw For Rev 73, 9–13.
Nichols, J. D., and Carpenter, F. L. (2006). Interplanting Inga edulis yields nitrogen benefits to Terminalia amazonia. For. Ecol. Manage. 233, 344–351. doi: 10.1016/J.FORECO.2006.05.031
Oestreicher, J. S., Benessaiah, K., Ruiz-Jaen, M. C., Sloan, S., Turner, K., Pelletier, J., et al. (2009). Avoiding deforestation in Panamanian protected areas: an analysis of protection effectiveness and implications for reducing emissions from deforestation and forest degradation. Glob. Environ. Chang. 19, 279–291. doi: 10.1016/j.gloenvcha.2009.01.003
Ogden, F. L., Crouch, T. D., Stallard, R. F., and Hall, J. S. (2013). Effect of land cover and use on dry season river runoff, runoff efficiency, and peak storm runoff in the seasonal tropics of Central Panama. Water Resour. Res. 49, 8443–8462. doi: 10.1002/2013WR013956
Oskarsson, H., and Brynleifsdóttir, S. J. (2009). The interaction of fertilization in nursery and field on survival, growth and the frost heaving of birch and spruce. Icel. Agric. Sci. 22, 59–68.
Ouédraogo, D.-Y., Fayolle, A., Daïnou, K., Demart, C., Bourland, N., Lagoute, P., et al. (2014). Enrichment of logging gaps with a high conservation value species (Pericopsis elata) in a Central African Moist Forest. Forests 5, 3031–3047. doi: 10.3390/f5123031
Paquette, A., and Messier, C. (2010). The role of plantations in managing the world’s forests in the Anthropocene. Front. Ecol. Environ. 8:27–34. doi: 10.1890/080116
Paquette, A., Bouchard, A., and Cogliastro, A. (2006). Survival and growth of under-planted trees: a meta-analysis across four biomes. Ecol. Appl. 16, 1575–1589. doi: 10.1890/1051-0761(2006)016[1575:sagout]2.0.co;2
Paquette, A., Hawryshyn, J., Senikas, A., and Potvin, C. (2009). Enrichment planting in secondary forests: a promising clean development mechanism to increase terrestrial carbon sinks. Ecol. Soc. 14:31. doi: 10.5751/ES-02781-140131
Park, A., van Breugel, M., Ashton, M. S., Wishnie, M., Mariscal, E., Deago, J., et al. (2010). Local and regional environmental variation influences the growth of tropical trees in selection trials in the Republic of Panama. For. Ecol. Manage. 260, 12–21. doi: 10.1016/j.foreco.2010.03.021
Parrotta, J. A., Turnbull, J. W., and Jones, N. (1997). Catalyzing native forest regeneration on degraded tropical lands. For. Ecol. Manage. 99, 1–7. doi: 10.1016/S0378-1127(97)00190-4
Paul, G. S., Montagnini, F., Berlyn, G. P., Craven, D. J., van Breugel, M., and Hall, J. S. (2012). Foliar herbivory and leaf traits of five native tree species in a young plantation of Central Panama. New For. Dordr. 43, 69–87.
Payn, T., Carnus, J.-M., Freer-Smith, P., Kimberley, M., Kollert, W., Liu, S., et al. (2015). Changes in planted forests and future global implications. For. Ecol. Manage. 352, 57–67. doi: 10.1016/J.FORECO.2015.06.021
Peña-Arancibia, J. L., Bruijnzeel, L. A., Mulligan, M., and van Dijk, A. I. J. M. (2019). Forests as ‘sponges’ and ‘pumps’: assessing the impact of deforestation on dry-season flows across the tropics. J. Hydrol. 574, 946–963. doi: 10.1016/j.jhydrol.2019.04.064
Pérez-Harguindeguy, N., Díaz, S., Garnier, E., Lavorel, S., Poorter, H., Jaureguiberry, P., et al. (2013). New handbook for standardised measurement of plant functional traits worldwide. Aust. J. Bot. 61, 167–234. doi: 10.1071/BT12225
Piotto, D. (2007). Growth of native tree species planted in open pasture, young secondary forest and mature forest in humid tropical Costa Rica. J. Trop. For. Sci. 19, 92–102.
Piotto, D., Craven, D., Montagnini, F., and Alice, F. (2010). Silvicultural and economic aspects of pure and mixed native tree species plantations on degraded pasturelands in humid Costa Rica. New For. 39, 369–385. doi: 10.1007/s11056-009-9177-0
Piotto, D., Vıìquez, E., Montagnini, F., and Kanninen, M. (2004). Pure and mixed forest plantations with native species of the dry tropics of Costa Rica: a comparison of growth and productivity. For. Ecol. Manage. 190, 359–372. doi: 10.1016/j.foreco.2003.11.005
Plath, M., Mody, K., Potvin, C., and Dorn, S. (2011). Establishment of native tropical timber trees in monoculture and mixed-species plantations: small-scale effects on tree performance and insect herbivory. For. Ecol. Manage. 261, 741–750. doi: 10.1016/j.foreco.2010.12.004
Quesada-Ávila, G., Turner, B., and Hall, J. (2021). No evidence that the valuable timber species, Dalbergia retusa, enhances nutrient cycling and uptake by neighbouring timber species. J. Sustain. For. 40, 1–12. doi: 10.1080/10549811.2021.1989700
Ramos, J., and del Amo, S. (1992). Enrichment planting in a tropical secondary forest in Veracruz, Mexico. For. Ecol. Manage. 54, 289–304. doi: 10.1016/0378-1127(92)90018-5
Rappaport, D., and Montagnini, F. (2014). Tree species growth under a rubber (Hevea brasiliensis) plantation: native restoration via enrichment planting in southern Bahia, Brazil. New For. 45, 715–732. doi: 10.1007/s11056-014-9433-9
Redondo-Brenes, A., and Montagnini, F. (2006). Growth, productivity, aboveground biomass, and carbon sequestration of pure and mixed native tree plantations in the Caribbean lowlands of Costa Rica. For. Ecol. Manage. 232, 168–178. doi: 10.1016/J.FORECO.2006.05.067
Riikonen, J., and Luoranen, J. (2018). Seedling production and the field performance of seedlings. Forests 9:740. doi: 10.3390/f9120740
Roman, F., De Liones, R., Sautu, A., Deago, J., and Hall, J. S. (2012). Guía para La Propagación de 120 Especies de Árboles Nativos de Panamá y el Neotropico. New Haven, CT: ELTI.
Roque, R., and Leandro, L. (2009). Wood characteristics of Terminalia amazonia, Vochysia guatemalensis and Hyeronima alchorneoides planted in Costa Rica. Bosque 30, 78–87.
Ross, L. (2013). Plan De Reforestacion Y Manjeo Forestal De 30 Hectareas Con Tectona grandis. Panama: Frijolito Colon.
Ruger, N. R., Wirth, C., Wright, S. J., and Condit, R. (2012). Functional traits explain light and size response of growth rates in tropical tree species. Ecology 93, 2626–2636. doi: 10.2307/41739620
Santiago, L. S., Wright, S. J., Harms, K. E., Yavitt, J. B., Korine, C., Garcia, M., et al. (2012). Tropical tree seedling growth responses to nitrogen, phosphorus and potassium addition. J. Ecol. 100, 309–316. doi: 10.1111/j.1365-2745.2011.01904.x
Schmidt, F. (2009). The effect of Site Selection On The Growth Of Dipteryx Panamensis In Timber Plantations In Costa Rica And Panama Master Of Science In Tropical Forestry And Management. Dresden: University of Technology.
Schneider, T., Ashton, M. S., Montagnini, F., and Milan, P. P. (2014). Growth performance of sixty tree species in smallholder reforestation trials on Leyte, Philippines. New For. 45, 83–96. doi: 10.1007/s11056-013-9393-5
Schweizer, D., and Brancalion, P. H. S. (2020). Rescue tree monocultures! A phylogenetic ecology approach to guide the choice of seedlings for enrichment planting in tropical monoculture plantations. Restor. Ecol. 28, 166–172. doi: 10.1111/rec.13064
Shyamsundar, P., Ahlroth, S., Kristjanson, P., and Onder, S. (2018). Understanding Forests’ Contribution to Poverty Alleviation Policy Research Working Paper No. 8462. Washington, DC: World Bank.
Sinacore, K. (2018). Variation In Plant Water Use, Growth, And Water-Use Efficiency Of Planted Mixtures And Monocultures: Toward Proper Species Selection For Reforestation Efforts In The Seasonally Dry Tropics. Durham: University of New Hampshire.
Sinacore, K., Asbjornsen, H., Hernández-Santana, V., and Hall, J. S. (2019). Drought differentially affects growth, transpiration, and water use efficiency of mixed and monospecific planted forests. Forests 10:153. doi: 10.3390/f10020153
Sinacore, K., García, E. H., Howard, T., van Breugel, M., Lopez, O., Finkral, A. J., et al. (2022). Towards effective reforestation: Growth and commercial value of four commonly planted tropical timber species on infertile soils in Panama. New For. doi: 10.1007/s11056-022-09906-0 [Epub ahead of print].
Sinacore, K., Hall, J. S., Potvin, C., et al. (2017). Unearthing the hidden world of roots: Root biomass and architecture differ among species within the same guild. PLoS One 12:e0185934. doi: 10.1371/journal.pone.0185934
Smethurst, P. J. (2010). Forest fertilization: Trends in knowledge and practice compared to agriculture. Plant Soil 335, 83–100. doi: 10.1007/s11104-010-0316-3
Soderlund, S. (2016). Inter- And Intra-Specific Variability In Functional Traits Of Seedlings Planted In Successional Tropical Dry Forest. Uppsala: Swedish University of Agricultural Sciences.
Stallard, R. F., Ogden, F., Elsenbeer, H., and Hall, J. S. (2010). Panama canal watershed experiment: agua salud project. Water Resour. Impact 12, 17–20.
Stape, J. L., Binkley, D., and Ryan, M. G. (2008). Production and carbon allocation in a clonal Eucalyptus plantation with water and nutrient manipulations. For. Ecol. Manage. 255, 920–930. doi: 10.1016/j.foreco.2007.09.085
Stefanski, S. F., Shi, X., Hall, J. S., Hernandez, A., and Fenichel, E. P. (2015). Teak–cattle production tradeoffs for panama canal watershed small scale producers. For. Policy Econ. 56, 48–56. doi: 10.1016/j.forpol.2015.04.001
Tenorio, C., Moya, R., Salas, C., and Berrocal, A. (2016). Evaluation of wood properties from six native species of forest plantations in Costa Rica. Bosque 37, 71–84. doi: 10.4067/S0717-92002016000100008
Tilkia, F., and Fisherb, R. F. (1998). Tropical leguminous species for acid soils: studies on plant form and growth in Costa Rica. For. Ecol. Manage. 108, 175–192. doi: 10.1016/S0378-1127(98)00225-4
Torres, R., Salazar-Garcia, S., and Ibarra-Estrada, M. (2018). Seasonal variation of transpiration of Nance (Byrsonima crassifolia L.) HBK selections under subtropical conditions climate: basic study. Kriter. Rev. Filos. 5:320. doi: 10.15741/revbio.05.01.08
Treseder, K. K. (2008). Nitrogen additions and microbial biomass: a meta-analysis of ecosystem studies. Ecol. Lett. 11, 1111–1120. doi: 10.1111/j.1461-0248.2008.01230.x
UNEPWCMC (2015). Overview of Dalbergia spp. from South and Central America- a basic Review. Cambridge: UNEPWCMC.
van Breugel, M., Craven, D., Lai, H. R., Bailon, M., Turner, B. L., and Hall, J. S. (2019). Soil nutrients and dispersal limitation shape compositional variation in secondary tropical forests across multiple scales. J. Ecol. 107, 566–581. doi: 10.1111/1365-2745.13126
van Breugel, M., Hall, J. S., Craven, D. J., Gregoire, T. G., Park, A., Dent, D. H., et al. (2011). Early growth and survival of 49 tropical tree species across sites differing in soil fertility and rainfall in Panama. For. Ecol. Manage. 261, 1580–1589. doi: 10.1016/j.foreco.2010.08.019
van Noordwijk, M., Tanika, L., and Lusiana, B. (2017). Flood risk reduction and flow buffering as ecosystem services–part 1: theory on flow persistence, flashiness and base flow. Hydrol. Earth Syst. Sci. 21, 2321–2340.
Vardeman, E., and Runk, J. V. (2020). Panama’s illegal rosewood logging boom from Dalbergia retusa. Glob. Ecol. Conserv. 23:e01098. doi: 10.1016/j.gecco.2020.e01098
Villacís, J., Casanoves, F., Hang, S., Keesstra, S., and Armas, C. (2016). Selection of forest species for the rehabilitation of disturbed soils in oil fields in the ecuadorian amazon. Sci. Total Environ. 566–567, 761–770. doi: 10.1016/j.scitotenv.2016.05.102
Wang, Q. K., Wang, S. L., and Liu, Y. X. (2008). Responses to N and P fertilization in a young Eucalyptus dunnii plantation: Microbial properties, enzyme activities and dissolved organic matter. Appl. Soil Ecol. 40, 484–490. doi: 10.1016/j.apsoil.2008.07.003
Wishnie, M. H., Dent, D. H., Mariscal, E., Deago, J., Cedeño, N., Ibarra, D., et al. (2007). Initial performance and reforestation potential of 24 tropical tree species planted across a precipitation gradient in the Republic of Panama. For. Ecol. Manage. 243, 39–49. doi: 10.1016/j.foreco.2007.02.001
Wolfe, B. T., Dent, D. H., Deago, J., and Wishnie, M. H. (2015). Forest regeneration under Tectona grandis and Terminalia amazonia plantation stands managed for biodiversity conservation in western Panama. New For. 46, 157–165. doi: 10.1007/s11056-014-9448-2
Wright, S. J., Yavitt, J. B., Wurzburger, N., Turner, B. L., Tanner, E. V. J., Sayer, E. J., et al. (2011). Potassium, phosphorus, or nitrogen limit root allocation, tree growth, or litter production in a lowland tropical forest. Ecology 92, 1616–1625. doi: 10.1890/10-1558.1
Xia, J., and Wan, S. (2008). Global response patterns of terrestrial plant species to nitrogen addition. New Phytol. 179, 428–439. doi: 10.1111/j.1469-8137.2008.02488.x
Yang, Y., and Huang, S. (2014). Suitability of five cross validation methods for performance evaluation of nonlinear mixed-effects forest models - a case study. Forestry 87, 654–662. doi: 10.1093/forestry/cpu025
Zalamea, P.-C., Turner, B. L., Winter, K., Jones, F. A., Sarmiento, C., and Dalling, J. W. (2016). Seedling growth responses to phosphorus reflect adult distribution patterns of tropical trees. New Phytol. 212, 400–408. doi: 10.1111/nph.14045
Zanne, A. E., Lopez-Gonzalez, G., Coomes, D. A., Ilic, J., Jansen, S., Lewis, S. L., et al. (2009). Global Wood Density Database, Dryad Digital Repository. doi: 10.5061/dryad.234
Keywords: forest restoration, Dalbergia retusa, Terminalia amazonia, Dipteryx oleifera, Hyeronima alchorneoides, Platymiscium pinnatum, Byrsonima crassifolia, Tectona grandis (teak)
Citation: Marshall A, Nelson CR and Hall JS (2022) Species selection and plantation management in enrichment planting with native timber species in the Panama Canal watershed. Front. For. Glob. Change 5:925877. doi: 10.3389/ffgc.2022.925877
Received: 22 April 2022; Accepted: 01 July 2022;
Published: 05 August 2022.
Edited by:
Arshad Ali, Hebei University, ChinaReviewed by:
Cinthia Pereira De Oliveira, Universidade Estadual do Amapá, BrazilVictor Santos, Universidade do Estado do Amazonas, Brazil
Copyright © 2022 Marshall, Nelson and Hall. This is an open-access article distributed under the terms of the Creative Commons Attribution License (CC BY). The use, distribution or reproduction in other forums is permitted, provided the original author(s) and the copyright owner(s) are credited and that the original publication in this journal is cited, in accordance with accepted academic practice. No use, distribution or reproduction is permitted which does not comply with these terms.
*Correspondence: Jefferson S. Hall, aGFsbGplQHNpLmVkdQ==