- 1CEFE, Univ Montpellier, CNRS, EPHE, IRD, Montpellier, France
- 2Department of Renewable Natural Resources and Environment, Faculty of Agricultural, Aleppo University, Aleppo, Syria
Belowground responses are critical in understanding how plants cope with the predicted increase in drought intensity and frequency in the Mediterranean region, because plant root systems compete with each other and interact with soil microbial communities under limiting water availability. However, the combined responses of plant roots and soil microorganisms to drought are not well understood. Using an experimental approach, we addressed the question of how recurrent drought and different combinations of two individuals from three common Mediterranean plant species (Quercus coccifera, Cistus albidus, and Brachypodium retusum) affect root characteristics and soil microbial activity. We hypothesized that drought effects on root characteristics and associated soil microbial communities are stronger when plant individuals of the same species compete for limiting water compared to inter-specific competition. Root morphological traits were more affected by the species identity of the neighbor individual than by recurrent severe drought, particularly in B. retusum, which may in part explain the higher survival previously observed for this species. On the other hand, drought and plant composition had little effect on root-associated soil microbes’ ability to use a wide range of different carbon substrates. Overall, our results did not confirm stronger effects of intra- compared to interspecific competition on the root and microbial responses, but showed strong species identity effects.
Introduction
Global climate models predict changes in precipitation regimes – an increase in the frequency and severity of drought in the Mediterranean region (Field et al., 2014; Forster et al., 2018) that will likely affect the plant and the soil microbial communities simultaneously and interactively (Wardle et al., 2004; Bardgett et al., 2014). To date, most of research on understanding drought effects has focused on aboveground plant traits, especially those related to leaf physiology and phenology (Garnier et al., 2001; Reich et al., 2003; Wright et al., 2005; Díaz et al., 2016). Roots have a major role in water acquisition and direct implications for growth and survival in water-limited environments, but the response of root traits to changes in soil water availability received less attention in Mediterranean species (but see Hernández et al., 2010; Larson and Funk, 2016), in part because roots are more difficult to study. A number of recent reviews, synthesis and conceptual developments, however, highlighted the importance of root traits and root trait economic spectrum in understanding plant responses to environmental changes and competition (McCormack et al., 2015; Freschet et al., 2017; Ma et al., 2018; Bergmann et al., 2020).
The plants respond by showing physiological and phenological adjustments of aboveground parts (Ferrio et al., 2003; Volaire et al., 2009). The belowground adjustments involve increasing biomass allocation to the root system (Markesteijn and Poorter, 2009), root length density (Padilla et al., 2015), and overall length of fine roots (Hertel et al., 2013). A plastic and rapidly growing root system (i.e., with high elongation rate) allowing rapid exploration of deep soil layers with higher moisture levels (Lloret et al., 1999; Paula and Pausas, 2011) is a particularly efficient way to face drought. Drought influences as well the structure and growth of plant roots, with species adapted to dry climates generally having higher root-to-shoot ratios, deeper root systems and investing more biomass into long-lasting root biomass (see Brunner et al., 2015 for review). Root functional traits such as rooting depth, root mass fraction (that is the ratio between root dry biomass and whole plant dry biomass), root diameter, specific root length (SRL; the root length achieved per unit of root biomass invested), and root tissue density (RTD, i.e., the ratio of root biomass/root volume) are expected to respond particularly strongly to drought, because they play a critical role in water acquisition (Hernández et al., 2010; Pérez-Ramos et al., 2013). The SRL, which is generally negatively correlated to root diameter and/or tissue density, is particularly relevant in characterizing a plant species’ potential for soil exploration and resource uptake (Wright and Westoby, 1999; Nicotra et al., 2002; McCormack et al., 2015). A high SRL implies a high surface:volume ratio maximizing the root-soil interface and hence the root absorption potential (Larcher, 2003). Moreover, thin roots with high SRL offer less resistance to the radial flow of water, thus increasing radial conductivity (Huang and Eissenstat, 2000). Plants with high SRL also tend to show higher root hydraulic conductance per unit leaf surface area (Peman et al., 2006) or per stem cross sectional area (Hernández et al., 2010). Consequently, plants with high SRL show high uptake rates of water and nutrients (Comas et al., 2002; Valverde-Barrantes et al., 2020).
Root growth patterns and traits may differ among different soil layers for a given plant species (Schenk, 2008; Prieto et al., 2015). In surface soil layers, comparatively higher nutrient concentrations (Jobbágy and Jackson, 2001; Arndt et al., 2004) and higher water availability during short precipitation events may favor the proliferation of roots with higher SRL, and thus a higher capacity of resource uptake. In contrast, roots in deeper soil layers ensure plant anchorage and provide often a safety investment for water uptake during dry spells because of a generally higher and longer term water availability in deeper layers (Laclau et al., 2013). Differences in abiotic conditions, such as soil moisture, temperature, and pH, between shallow and deep soil layers may lead to contrasting suites of traits with depth since root traits are highly plastic and respond to heterogeneous resource distributions (Hodge, 2004; Ostonen et al., 2007; Wang et al., 2020).
Plant species vary strongly in their root traits (e.g., Bergmann et al., 2020) leading to temporarily and spatially complementary resource use across the soil profile that may result in less competition for a given amount of water resource between individuals of different species than between individuals of the same species (Turnbull et al., 2013). Drought effects may therefore be more accentuated when individuals of the same species grow together compared to species rich plant communities (Isbell et al., 2015; Wagg et al., 2017).
Through their roots, but also through the modification of microclimatic conditions and the production of organic matter deposited on and in the soil through rhizodeposition and litter input, plants affect soil microorganisms and the processes they drive (Grayston et al., 1998; Eviner and Chapin, 2003; Bardgett et al., 2014). Altered plant physiology and carbon allocation in response to drought may affect soil microorganisms (Schimel, 2018). Changes in root traits also should influence soil microbial community composition and functioning (Bardgett et al., 2014; Legay et al., 2014; Gillespie et al., 2021, but see De Long et al., 2019). In turn, soil microorganisms may affect plant responses to drought by different mechanisms, including alteration of C allocation to the roots (Song et al., 2012; Brunner et al., 2015; Hasibeder et al., 2015; Kannenberg and Phillips, 2017), production of exopolysaccharides that protect plant roots from desiccation and improve water use efficiency (e.g., Naseem et al., 2018; Chai and Schachtman, 2022), changes in stomatal behavior, photosynthetic rates, leaf water potential, and xylem tension (Friesen et al., 2011; Rapparini and Peñuelas, 2014; Fan et al., 2015). The multiple mechanisms involved in these complex interactions are currently not well identified (Williams and de Vries, 2019; Aslam et al., 2022), and they may vary as a function of the identity and diversity of involved plant species.
In this study, we compared three typical co-existing perennial plant species that dominate in many shrublands of the northern part of the Mediterranean basin. Two of them are woody shrub species (Quercus coccifera and Cistus albidus), both with the strategy of dehydration avoidance during drought. The third species is the perennial grass Brachypodium retusum with a dehydration tolerance strategy. We aimed to quantify the effect of recurrent drought events on plant root traits and associated soil microbial communities in shallow and deep soil, and for plants either competing intra- or inter-specifically. Based on model predictions of more frequent and more severe drought events in the Mediterranean (Hertig and Tramblay, 2017), we simulated three repetitive drought events compared to a single drought event typical for the Mediterranean summer. Two plant individuals, either of the same species or of two different species in all three possible combinations, were grown in custom-made rhizotrons. We evaluated root traits and their responses to recurrent drought events and the identity of the neighbor individual. We previously showed that recurrent drought increased the mortality of woody species, but not that of B. retusum, and decreased the root and shoot relative growth rates of B. retusum, but not that of woody species, and that these responses were strongly dependent on the identity of the neighbor plant species (Shihan et al., 2020). Based on these previous results, we expected that recurrent drought affects root morphological traits of the grass species more than those of the woody species, and that this effect further depends on the identity of the neighbor individual. Because different plant species with contrasted root traits should use limiting water in a more complementary way than individuals of the same species (niche complementarity, Leibold, 1995), we hypothesized that drought effects on root traits would be stronger under intra- compared to interspecific competition for water. We further hypothesized that changes in root traits would be tracked by the soil microbial community showing different catabolic capabilities, with again stronger effects in the grass species and under interspecific competition.
Materials and methods
Plant material and rhizotron experiment
The rhizotron experiment was previously described in Shihan et al. (2020). Briefly, we used three Mediterranean plant species that are abundant and locally often dominant in garrigue-type Mediterranean shrublands in the west of southern Europe: the evergreen woody shrub Q. coccifera, the semi-deciduous woody shrub C. albidus, and the rhizomatous perennial grass B. retusum. The woody shrub species are dehydration avoiders (Damesin et al., 1998; Galmés et al., 2007), while B. retusum, as most native perennial grasses in Mediterranean areas, is regarded as dehydration tolerant (Pérez-Ramos et al., 2013). C. albidus and Q. coccifera individuals were grown from seeds and acorns, respectively, and B. retusum were obtained from ramets (collected from different patches in a garrigue near Marseille), all in 1 L-pots using a soil-sand mix (see below) with regular watering. At time of planting into the rhizotrons, C. albidus seedlings were 1-year-old with a mean total seedling biomass of 1.6 ± 0.1 g (dry matter, n = 10), and Q. coccifera seedlings were 5 months old with an average total seedling biomass of 1.2 ± 0.08 g (dry matter, n = 10). For B. retusum, individual ramets consisted of a 3–5 cm long rhizome with roots and one single shoot with a total average biomass of 0.12 ± 0.02 g (dry matter, n = 10). The custom-made 85 × 34 × 2.3 cm rhizotrons (described in Shihan et al., 2020) contained a calcareous soil-sand mix (in a 50:50 volume ratio), with field capacity at 23 ± 0.1% and the permanent wilting point at 5.1 ± 0.12% of gravimetric soil water content (determined at soil water potential = −0.063 and −15 MPa, respectively, Supplementary Figure 1). This substrate was sterilized at 120°C for 48 h in order to suppress the seed bank and the soil microbial community inherited from previous vegetation. Physico-chemical properties of the soil-sand mix are shown in Supplementary Table 1.
Plant growth condition and drought treatment
Two seedlings or ramets were planted in rhizotrons, with either two individuals of the same species (referred as BB, CC, and QQ) or of two different species in all possible combinations (BC, BQ, and CQ), yielding a total of six different plant combinations that were replicated 12 times for a total of 72 rhizotrons installed in six blocks (Supplementary Figure 2). After installation, the plants were allowed to establish over 12 months while keeping the substrate at field capacity, until the drought experiment started. At the end of this establishment phase, B. retusum, C. albidus, and Q. coccifera roots reached a soil depth of 81.6 ± 0.6, 80.3 ± 2.5, and 77 ± 5.8 cm, respectively, i.e., they all basically reached the bottom of the rhizotrons.
Most previous experimental studies applied drought treatments on the basis of a fixed time span, which means that individual plants may experience vastly different soil water conditions because of differences in the size and growth rate of root systems and different response dynamics to changing soil water content. Here we chose to simulate an increased frequency of drought events and to control the drought intensity at the level of individual rhizotrons in order to expose each individual plant to the same drought intensity. With this approach we were able to compare the root biomasses and traits of different species and individual plants of different sizes at the same drought exposure, while we had to accept a rhizotron-specific duration of the experiment. At the start of the drought experiment (3 December 2014), half of the rhizotrons were randomly assigned to the recurrent drought treatment, while the other half were used for the one drought treatment. Rhizotrons were kept under a transparent plastic rain shelter. Air temperature, relative humidity under the rain shelter and the daily air vapor pressure deficit VPD (kPa) are shown Supplementary Figures 3A,B (for more details see Shihan et al., 2020). In order to avoid the unnatural situation of constantly high soil water availability, the plants subjected to one drought treatment were allowed, to dry moderately down to 75 ± 3% of field capacity before they were re-watered to reach field capacity (Supplementary Figure 4A). For the soil-sand mix, this value corresponds to the gravimetric water content of 17.5 ± 0.5 g of water per 100 g of dry soil (i.e., 17.5 ± 0.5%, soil water potential = −0.141 MPa, Supplementary Figure 1). For the recurrent drought treatment, two drying cycles were applied during which the gravimetric soil water content reached 11 ± 0.4% (Supplementary Figure 4B). This threshold was defined as the soil water content at which all leaves of B. retusum entirely senesced and the gravimetric soil water content did not further decrease during two additional weeks. Each individual rhizotron was weighed once every week to determine gravimetric soil water content and the exact date of re-watering. Watering was resumed as soon as a particular individual rhizotron reached 17.5% (one drought) or 11% (recurrent drought treatment) of gravimetric soil water content (Supplementary Figure 4). Each watering event was adjusted to reach field capacity. After that, we applied a final drying cycle (reaching 11% of soil water content) to rhizotrons of both one drought and recurrent drought treatments (Supplementary Figure 4), i.e., three drying cycles for the recurrent drought treatment compared to one drying cycle for the one drought treatment. We did this because in our study system, summer drought is naturally occurring every year, and we wanted to explicitly test the impact of repetitive drought. After this final drying cycle when all rhizotrons reached the threshold of 11% of soil water content, they were again watered to reach field capacity. The rhizotrons were kept at soil water contents higher than 17.5% for 3 weeks, so that microbial and root parameters could stabilize following re-watering and be measured under similar soil water content conditions; all plants were then harvested.
One rhizotron for each plant composition and drought treatment was equipped with a soil humidity sensor (WATERSCOUT SM 100, SDEC, France) installed horizontally at two depths (10 and 50 cm from the soil surface) to measure volumetric soil moisture once per hour (Supplementary Table 2).
At the beginning of the drought treatment and at the end of every drying cycle, root systems were recorded by successive drawings through the glass pane at two different soil depths (17.5 and 57.5 cm, corresponding to the soil depths of final root harvest) in 15 × 15 cm within the center zone of the rhizotron (Supplementary Figure 5). Root elongation rate (RE), was calculated as follows:
, where RE is the daily root elongation rate (mm d–1), L1 and L2 are the recorded root lengths at t1 and t2, respectively.
Additionally, five individuals of C. albidus and of B. retusum and three of Q. coccifera were cultivated following the same protocol as for the other plants for the establishment period, but with one single individual per rhizotron, i.e., without intra- or interspecific competition. These individuals were harvested at the end of establishment period and before the start of drought treatment and used to determine the root morphological traits.
Plant and soil harvest
By the end of the experimentation and at final harvest, a total of 14 C. albidus individuals, 27 Q. coccifera individuals and 40 B. retusum individuals survived, corresponding to average survival rates of 30, 58, and 80% across all treatments, respectively (see Shihan et al., 2020). All rhizotrons were harvested 3 weeks exactly after the end of the last drought cycle that was common for all recurrent drought and one drought rhizotrons (see above). The rhizotrons were placed horizontally, and the glass pane was removed. Three adjacent soil cores (4.4 cm in diameter for 34.97 cm3 of volume) were yielded in the thickness of the soil at 17.5 and at 57.5 cm depth below the soil surface within the center zone of the rhizotron (Supplementary Figure 5). Roots were immediately recovered from these three soil cores, washed to remove adhering soil particles, and, for the two-species rhizotrons, sorted by species since they are visually distinctive. For two of the cores (not the centered one), fine roots (<0.55 mm in diameter; i.e., corresponding to absorptive roots for the woody species) were selected and put in water for morphological analysis. The remaining roots (>0.55 mm in diameter and roots from the third centered core) were kept for total biomass determination (see below).
The soil recovered from all three soil cores was pooled for each depth and homogenized to yield representative samples for microbial analyses. The soil samples were air-dried in the dark at 25°C, sieved at 2 mm and stored in a dry and dark room until analysis.
Root morphological traits
Before root morphology could be assessed, B. retusum fine roots were stained with methylene blue (1 g L–1) for 5 min to increase the contrast during the scanning. The darker roots of C. albidus and Q. coccifera did not require staining. The fine roots were rinsed in distilled water, spread out on a fine mesh while immerged in water to avoid overlapping roots, and transferred to a transparent acetate sheet (Roumet et al., 2008; Birouste et al., 2012). Samples were scanned as grayscale images at a resolution of 600 dpi using a scanner equipped with a transmitting light source that avoids shadows (EPSON Expression 1680). All roots were then recovered from the acetate sheet, oven-dried at 65°C for 48 h and weighed to determine fine root dry biomass (RB, g). A digital image analyzing software (Winrhizo, version 2003b, Regent Instrument, Québec, Canada) was used to determine root length (L, cm), root mean diameter (Diam, mm), and root volume (V, cm3) from the sum of all volumes calculated on the basis of 11 diameter classes (from 0 to 0.55 mm, with a class width of 0.05 mm). From these measurements we calculated SRL, (m g–1, as the ratio L/RB), RTD (g cm–3, as the ratio of RB/V), root thickness (RTh, cm3 m–1, as the ratio V/L), and finally, root density (RD, root cm. soil cm–3, as the ratio L/soil volume) (Roumet et al., 2008; Birouste et al., 2012).
The rhizotron-weighted mean traits RWM (SRLRWM, RTDRWM, RThRWM, RDRWM, and DiamRWM) and the functional dissimilarity FD for fine roots (SRLFD, RTDFD, RThFD, RDFD, DiamFD, and the global trait dissimilarity AllFD) were calculated in rhizotrons where both individuals were still alive by the end of treatments (n = 26). RWM were calculated as the average trait values of morphological traits following (Garnier et al., 2004): traitRWM = RBi. traiti + RBj.traitj, where RBi and RBj are the percentage of root mass and traiti and traitj are the trait value for species i and j, respectively. The functional dissimilarity of traits in rhizotrons with two species was calculated according to Rao’s quadratic entropy (Botta-Dukát, 2005) for each rhizotron using dbFD function of FD package (Laliberte and Legendre, 2010) as: TraitFD = RBi.RBj. dij, where dij is the Euclidian distance between species i and j for the trait considered (TraitFD values are 0 in mono-specific rhizotrons). Because the measured traits differ in their numerical value ranges, standard normal deviates (so as to get an expected value of zero and a variance of one for traits values) were used to calculate functional dissimilarity.
Community level physiological profiles of the soil microbial community
We used the MicroResp™ profiling (Macaulay Scientific Consulting, Aberdeen, UK) to characterize the catabolic capabilities of the soil microbial community as previously described (Shihan et al., 2017). Community level physiological profiles (CLPP) were characterized at the rhizotron level because the distinction between the influence of the two plant individuals was not possible within a common soil volume. Briefly, about 0.49 g of soil were incubated in triplicate with 1.5 mg C g–1 soil (except for the low-solubility phenolic acids and cellulose for which 0.75 mg C g–1 soil were added) of 15 different carbon substrates (D-glucose, xylan, cellulose, N-acetyl-glucosamine, L-asparagine, L-glutamine, L-lysine, L-serine, L-glycine, malic acid, oxalic acid, uric acid, caffeic acid, syringic acid, and vanillic acid), plus one control with deionized water, so as to reach 80% of the field capacity in 96-deepwell microplates. After an initial 2-h pre-incubation at 25°C in the dark, each deepwell microplate was covered with a Cresol red detection plate using a silicone gasket (MicroResp™, Aberdeen, UK), and the assembly was secured with a clamp, and incubated for four additional hours. Optical Density at 590 nm (OD590) was measured for each detection well before and after incubation using a Victor 1420 Multilabel Counter (Perkin Elmer, Massachusetts, USA). Final OD590 were normalized using pre-incubation OD590, and converted as substrate induced respiration rates expressed in μg C-CO2 respired per g of soil per hour. The respiration rates for the different C substrates were summed across all 15 substrates (sum15) as a proxy of the global catabolic activity, and standardized rate for each substrate was computed as the rate measured for this substrate divided by sum15, as proposed by Leff et al. (2012). For each plot, a Shannon catabolic diversity index was computed as: H′ , and a Simpson catabolic dominance index was computed as: D2, where (pi) is the standardized respiration rate for substrate (i).
Data analysis
Plant parameters were analyzed for each species separately for the effects of drought (one drought vs. recurrent drought event), neighbor plant identity, soil depth sampling (top vs. deep soil layer) and their interactions. Soil microbial parameters were analyzed at the rhizotron level because they could not be associated to a particular plant species or individual. We used mixed linear models [lme function of R package “nlme” (Bates and Pinheiro, 1998) and r.squaredGLMM function of R package “MuMIn” (Kamil, 2016) to calculate R square values] to assess whether RB, RE, root morphological traits (SRL, RTD, Rth, RD, and Diam) and soil microbial parameters (sum15, H′, and D) differed according to the drought treatment (one drought vs. recurrent drought) and neighbor plant identity (or plant species composition for soil microbial parameters) and their interactions. We also included soil depth (top, 17.5 cm and deep, 57.5 cm) as an additional factor in the model: soil depth was nested within rhizotron identity and fitted as random variable. As a result of mortality during the experiment (see Shihan et al., 2020) the period of presence of individuals that died during the experiment was integrated as the percentage of total presence period of living individual, and used in model fits as a co-variable. Likewise, the time during which each rhizotron was kept in the experiment (from plantation to harvest, depending on the duration of the drying cycles) was also fitted as a co-variable in the models. Differences between drought treatments, identity of the neighbor or composition and soil depth were tested using Tukey HSD post hoc comparison tests [lsmeans function of the eponymous R package (Lenth, 2016)]. In the rhizotrons where both individuals were alive by the end of the experiment (n = 26 rhizotrons), the relationship between soil microbial catabolic parameters (sum15, H′, D, and standardized respiration rates for individual substrate) and root traits [either community rhizotron-weighed mean traits (TraitRWM) or functional dissimilarity (TraitFD)] were explored using Pearson’s correlations. Heteroscedastic variables were transformed to meet the assumption of normal distribution of residuals. Statistical analyses were performed with the R software (version 3.6.3, R Core Team, 2020) with significance levels indicated as ′P < 0.10, *P < 0.05, **P < 0.01, and ***P < 0.001. Data are presented as means ± SEM.
Results
Root traits and biomass
After the 1-year establishment phase of the plants and before the drought treatment started, B. retusum and Q. coccifera had the highest and lowest SRL, and the lowest and highest RTD, RTh, and root diameter (Diam), respectively. The root traits in C. albidus were not significantly different from the other two species (Supplementary Table 3A). At the end of the drought experiment, and irrespective of the drought treatment, the species-specific differences remained mostly the same (Supplementary Table 3B). Compared to the root traits measured before the drought treatment started, the RTD was higher, and Diam and RTh were lower in all three species (Supplementary Table 3). We were not able to measure the hydric behavior of all the rhizotrons, but measurements in only one rhizotron per plant combination and per drought treatment showed that the soil water content varied across plant composition, drought treatment and soil depth (Supplementary Table 2). Because some plant combinations did not survive to the first (BC) or to the third drought event (BB and BQ), it was difficult to conclude about the role of plant combination on the hydric behavior in the rhizotrons. Root parameters measured on B. retusum, C. albidus, and Q. coccifera in the two drought treatments and soil depth and according to the identity of the neighbor species are shown in Supplementary Table 4.
Root biomass differed in the two soil layers in C. albidus and Q. coccifera, with a lower biomass in the deep compared to top soil layer. RE was higher in B. retusum only in the deep compared to top soil layer. Overall, soil depth influenced several root traits, especially in B. retusum. In B. retusum, SRL, RD, and Diam were higher and RTD was lower in the subsoil compared to the topsoil layer (Table 1). The differences between the two soil layers were modified by drought and the identity of the neighbor individuals (see below).
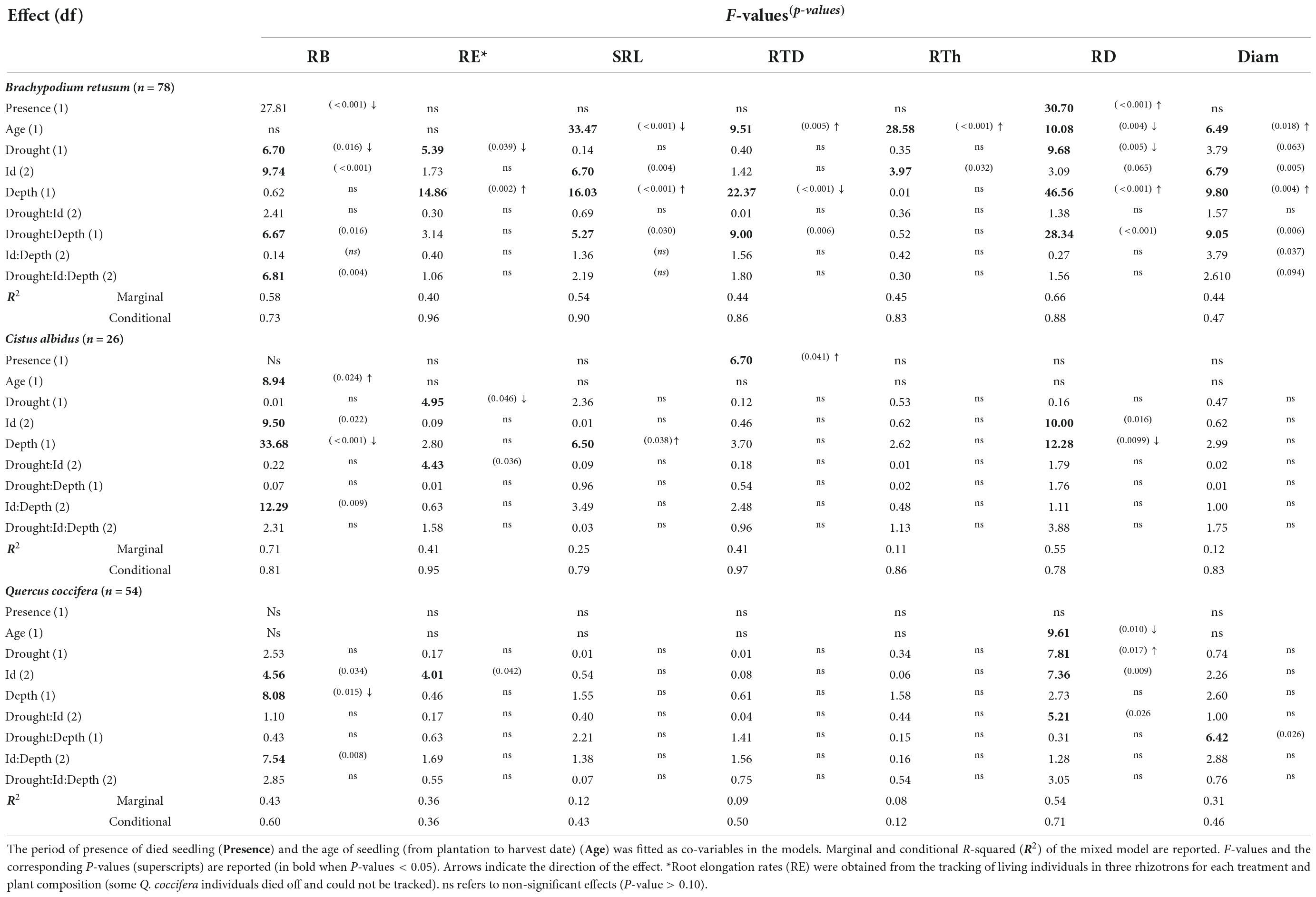
Table 1. Output of multiple linear mixed models testing for the effects of drought treatment (Drought), community composition (Id), horizon sampling (Depth) and their interactions (Drought:Id and Drought:Id:Depth) on root dry mass in soil cores (RB) specific root length (SRL), root tissue density (RTD), root thickness (RTh), root length per soil volume (RD) and average root diameter (Diam).
Root biomass and traits differed in response to recurrent drought cycles compared to the one drought treatment (Table 1 and Figures 1A–F). In B. retusum, RB and RE were lower in response to recurrent compared to one single drought (Table 1), with stronger effects on RB in deep compared to top soil (Table 1 and Figure 1A). B. retusum also showed a lower RD in response to recurrent drought, especially in the deep soil (Figure 1E). Root diameter responded differently to recurrent drought depending on soil depth, with larger and smaller diameters in the top and the deep soil layer, respectively, compared to one drought (Table 1 and Figure 1F). SRL and RTD in B. retusum were lower and higher, respectively, under recurrent drought in the deep soil layer (Table 1 and Figures 1B,C), but not significantly different between the two drought treatments in the top soil layer (significant Drought × Depth interactions, Table 1). The diameter of Q. coccifera roots was distinctly affected by recurrent drought depending on the soil layer, with smaller diameters only in the top, but not in the deep, soil layer (significant Drought × Depth interaction, Table 1). C. albidus showed only lower RE rates with recurrent drought compared to the one drought treatment, but there were no other drought effects on other root traits or biomass (Table 1).
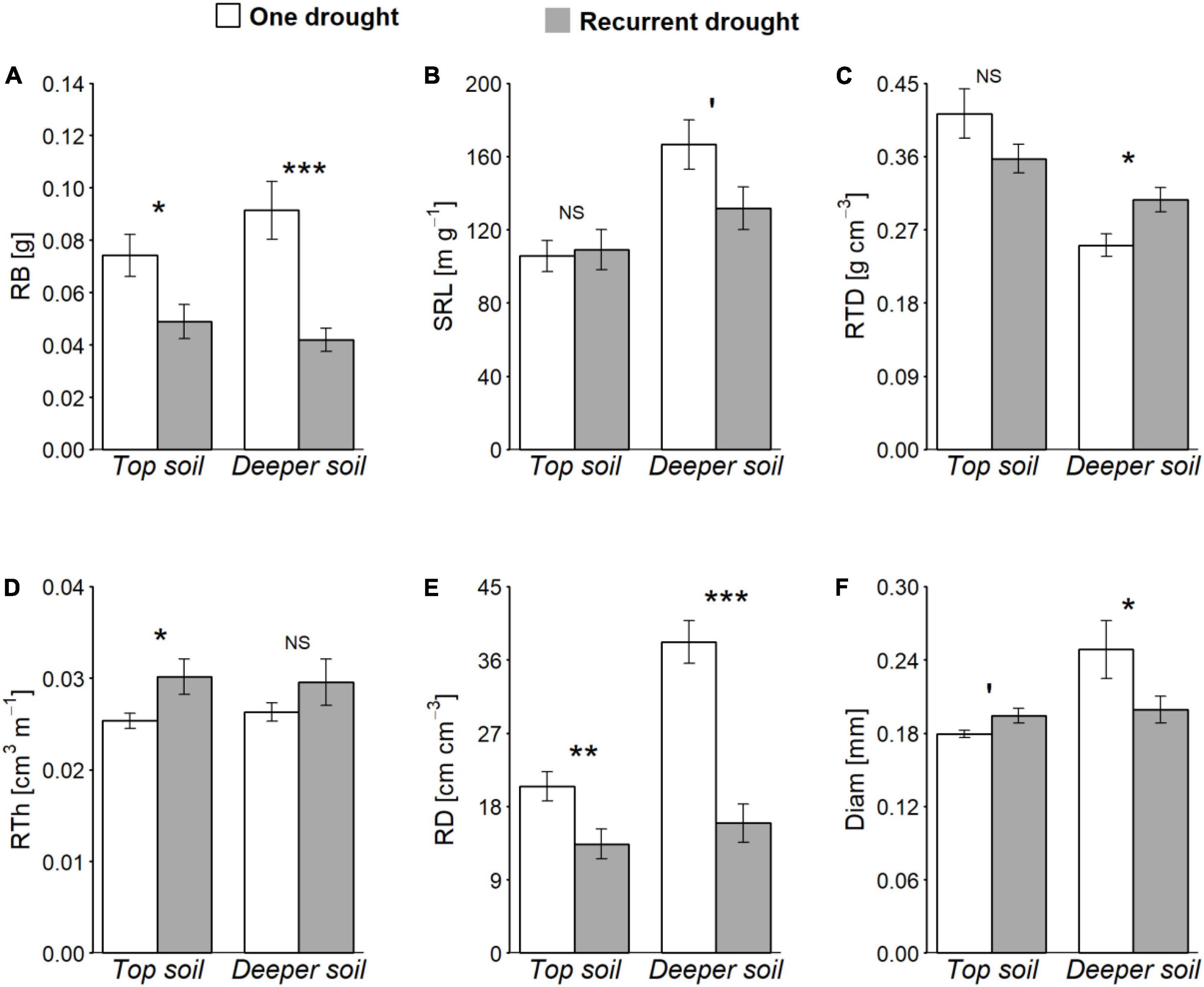
Figure 1. Root biomass (RB) and morphological traits [specific root length (SRL), root tissue density (RTD), root thickness (RTh), root length per soil volume (RD) and mean root diameter (Diam)] of B. retusum (A–F, respectively) as a function of soil depth (top soil = 17.5 cm and deeper soil = 57.5 cm), in one drought (white bars) and recurrent drought (gray bars) conditions (across all neighbor species identity treatments). Mean values (±SE) are shown. Asterisks indicate significant statistical differences among treatments (NSnot significant, ′P < 0.10, *P < 0.05, **P < 0.01, and ***P < 0.001, Tukey HSD test).
Overall, the identity of the neighbor plant species impacted a larger number of root parameters than drought (Table 1 and Figures 2A–F). Root biomass of B. retusum was higher when it grew with a conspecific as neighbor compared to when it grew with C. albidus or Q. coccifera, regardless of the drought treatment (Table 1 and Figure 2A). SRL tended to be higher when B. retusum grew together with an individual of C. albidus or of Q. coccifera than when it grew with a conspecific (Figure 2B). Conversely, RTh and Diam were lower when B. retusum grew with an individual of C. albidus than with a conspecific (Table 1 and Figures 2D,F). For C. albidus individuals (Table 1) (which did not survive until the end of the experiment when growing with B. retusum), RB was higher when it grew together with a conspecific individual than when it grew together with Q. coccifera, especially in the top soil layer. RD was also higher when it grew with a conspecific individual than when it grew together with an individual of Q. coccifera. Similarly, RE was higher in C. albidus when growing with a conspecific compared to when growing with a Q. coccifera individual, but only under recurrent drought (Table 1). In contrast to B. retusum, RB of Q. coccifera in the top soil was lower in individuals growing with a conspecific compared to those growing with C. albidus (Table 1). RE and RD in Q. coccifera plants were lower when they grew with a conspecific compared to when they grew with C. albidus, but there were no differences when it grew with B. retusum. RD in Q. coccifera when growing together with C. albidus decreased strongly under recurrent drought (significant Id × Drought interaction, Table 1).
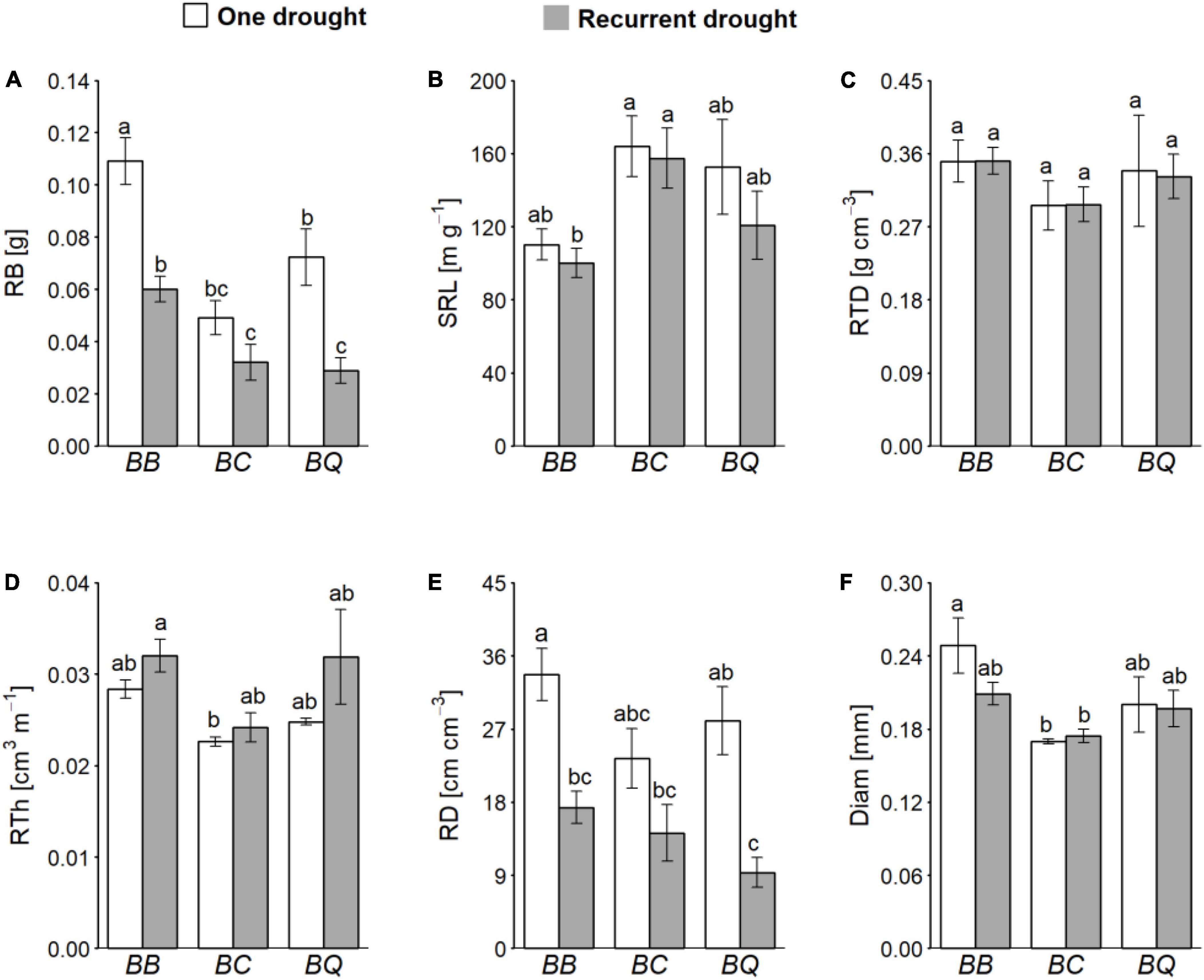
Figure 2. Root biomass (RB) and morphological traits [root dry mass (RB), specific root length (SRL), root tissue density (RTD), root thickness (RTh), root length per soil volume (RD), and mean root diameter (Diam)] of B. retusum (A–F, respectively) as a function of neighbor species identity (B for B. retusum, C for C. albidus, and Q for Q. coccifera) in one drought (white bars) and recurrent drought (gray bars) conditions. Mean values (±SE) are shown. Different small letters indicate significant differences among treatments (Tukey HSD test, P-values < 0.05).
Finally, because we respected the exact same intensity of drought within each of the two drought treatments across all plant species combinations, individual rhizotrons were harvested at different times as soon as they completed the full cycle of drought events (Supplementary Figure 4). Consequently, individual plants remained for different periods of time in the experiment and thus, varied somewhat in age at harvest. In addition, individual plants were experiencing competition from the neighbor plant for different durations depending on mortality. We accounted for these differences in our statistical models (see section “Data analysis” and Table 1). Age of seedling and presence of the neighbor had some effects on response variables of roots. The age of individual plants (due to differences in harvest dates) did not influence RB but had significant effects on all root traits in B. retusum (SRL and RD were lower, and RTD, RTh, and Diam higher in older compared to younger B. retusum plants). The age of seedling was positively related to RB in C. albidus, and was negatively related to RD in Q. coccifera (with lower RD in older compared to younger plants). The duration of the presence of neighbor individual plants within the rhizotrons had generally few effects on root traits, except of a small number of traits in either B. retusum or C. albidus. B. retusum had a somewhat lower RB and a higher RD with increasing duration of the presence of a neighbor individual, irrespective of its species identity. C. albidus showed a higher RTD with increasing duration of the presence of a neighbor individual, again irrespective of its species identity.
Community level physiological profiles of soil microbial communities
The global catabolic activity (sum15), the Shannon catabolic diversity index (H′) and the Simpson catabolic dominance index (D) were characterized at the rhizotron level because the distinction between two plant individuals was not possible within a common soil volume. The average values for these parameters for the six plant species combinations, in top and deep soil layers are shown in (Table 2). Soil depth had the largest influence on the soil microbial metabolic properties, with higher global metabolic activity (sum15) and microbial metabolic dominance (D) and lower microbial metabolic diversity (H′) in deep compared to top soil layers across all plant species composition and drought treatments (Table 3). Notably, relative substrate induced respiration rates rSIR were higher for cellulose, but lower for asparagine, lysine, glutamic, and caffeic acids in deep compared to top soil (Table 3). rSIR rates for xylan and caffeic acid were lower in deep compared to top soil when Q. coccifera grew with B. retusum or with C. albidus (for xylan), and when B. retusum grew with Q. coccifera or with a conspecific species (significant Composition × Depth interaction, Table 3).
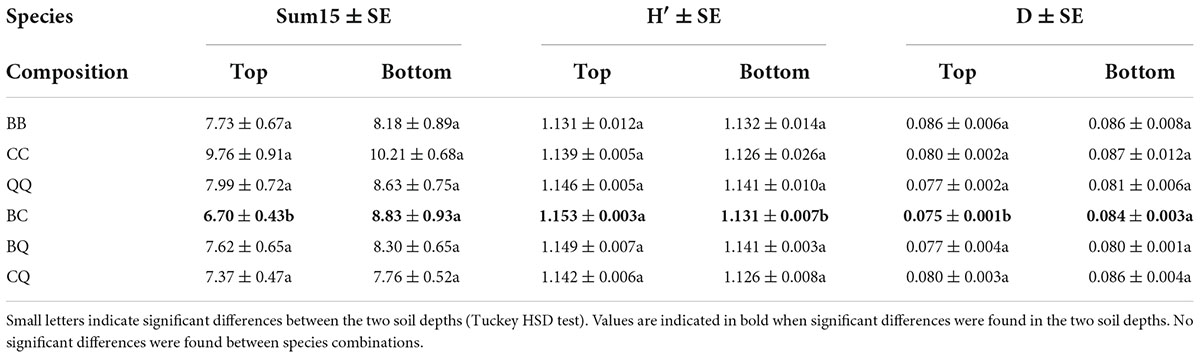
Table 2. Means ± SE (pooled across one and recurrent drought treatments) of global metabolic activity (sum15) expressed in μg C-CO2 g–1 soil h–1, Shannon microbial metabolic diversity index (H′) and Simpson microbial metabolic dominance index (D) at two soil depths (top = 17.5 cm and bottom = 52.5 cm) for each of the six different species combinations (B for B. retusum, C for C. albidus, and Q for Q. coccifera).
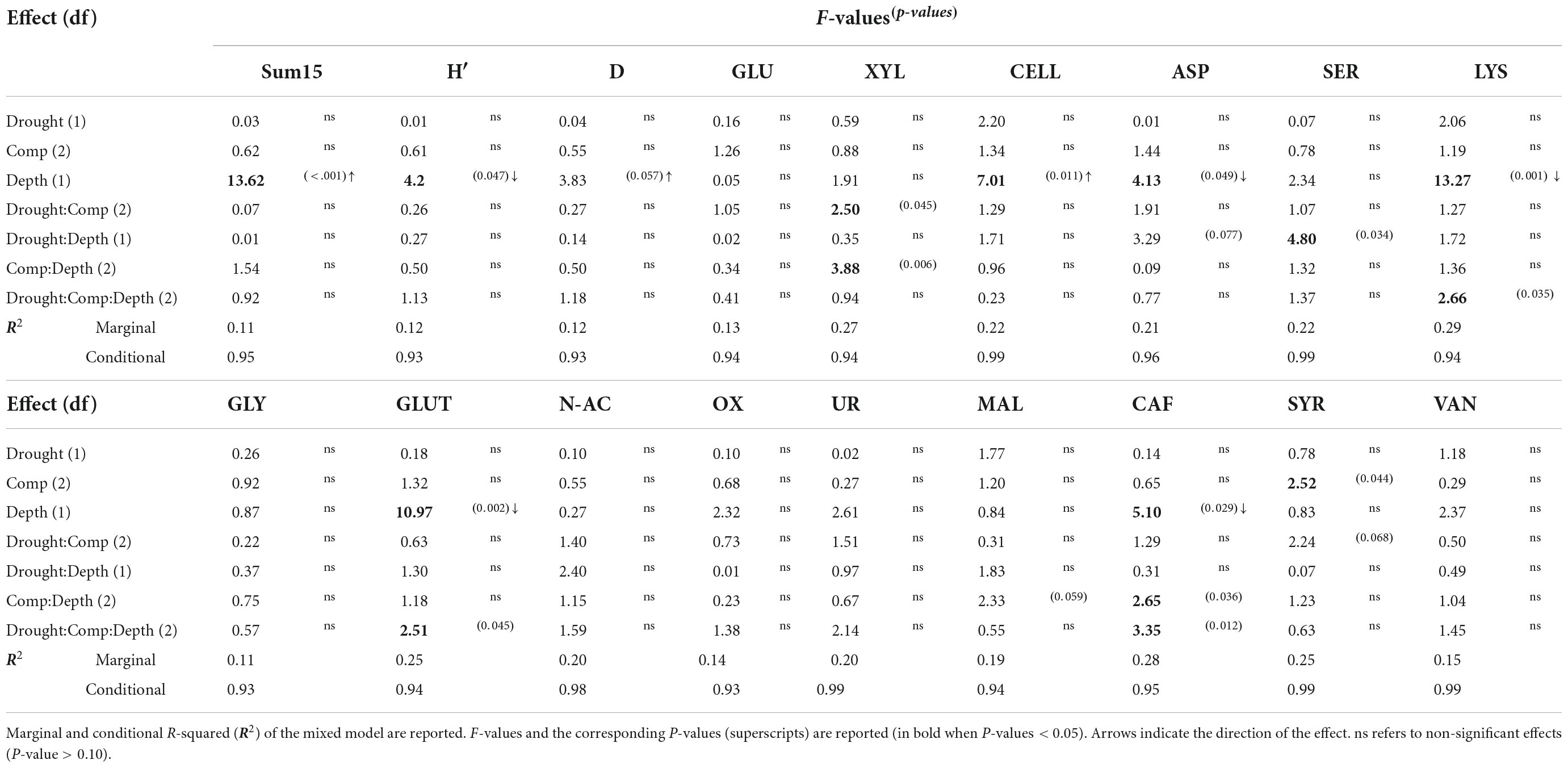
Table 3. Output of multiple linear mixed models testing for the effects of drought (Drought), plant species composition (Comp), soil depth (Depth) and their interactions on soil microbial parameters [global metabolic activity (sum15), microbial metabolic diversity (H′), and microbial metabolic dominance (D)] and on the community level respiration rates of 15 carbon substrates.
Recurrent drought had a few effects on CLPP parameters in interaction with other experimental factors. For instance, rSIR of xylan was higher in the treatment with recurrent drought compared to the one drought treatment when B. retusum grew with a conspecific species but lower when it grew with C. albidus (significant Drought × Composition interaction, Table 3).
Finally, unlike the overall strong effect of plant composition on root traits reported above, CLPP were only weakly affected by plant composition (Table 3). The sole exception was observed for syringic acid rSIR that was higher in soil when C. albidus was the only plant species compared to soil from rhizotrons in which C. albidus grew together with Q. coccifera or when Q. coccifera grew together with B. retusum.
Relationship between root traits and soil microbial community level physiological profiles
We evaluated correlations between soil microbial properties and mean root traits (TraitRWM) or functional dissimilarity (TraitFD). There were no significant correlations between sum15 and mean root traits, but H′ increased and D decreased with increasing DiamRWM (Table 4). Sum15, but not H′ nor D, correlated negatively with functional dissimilarity of root traits (except for DiamFD). On the other hand, there were some significant correlations between rSIR of individual substrates and TraitRWM, but not for TraitFD (Table 4). The ability of the soil microbial community to respire on lysine increased with increasing RTDRWM and RThRWM and with decreasing SRLRWM and RDRWM. The relative use of cellulose and syringic acid increased and that of oxalic acid decreased with increasing DiamRWM, and the relative use of oxalic acid decreased with increasing RThRWM.
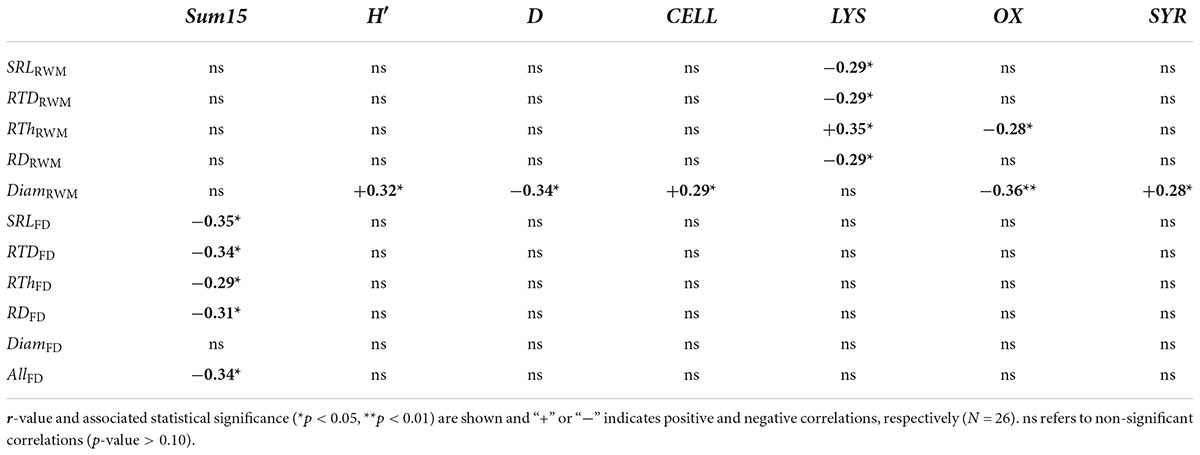
Table 4. Pearson’s correlation matrix between mean fine root traits (SRLRWM, RTDRWM, RThRWM, RDRWM, and DRWM) or trait dissimilarity (SRLFD, RTDFD, RThFD, RDFD, DiamFD, and AllFD) and soil microbial parameters [total metabolic activity (sum15), microbial metabolic diversity (H′), and microbial metabolic dominance (D)], and the community level respiration rates of 15 carbon substrates (only those that correlated significantly with at least one trait are mentioned).
Discussion
In a previous study using the same experiment, we showed that recurrent drought treatment reduced the relative growth rates for shoot and root in B. retusum but not in the two woody species C. albidus and Q. coccifera. Conversely, recurrent drought increased the mortality of the woody species, but not that of B. retusum, as compared to the plants in the control treatment with only one drought cycle (Shihan et al., 2020). Here, we report how these three Mediterranean plant species responded to recurrent drought in terms of root biomass (RB), RE, and various root morphological traits and associated soil microbial community in two different soil depths as a function of the identity of the neighbor plant species.
Drought stress generally develops from the top down as the soil surface dries, while adequate soil moisture can remain deep in the soil profile after surface soils have dried (Skinner and Comas, 2010). Here, soil water content was generally (two- to threefold) higher in the deep compared to top soil layer for every drought period (except for the BQ combination at the end of the drought event for the one drought treatment) (Supplementary Table 2). Indeed, several root and microbial parameters differed between top and deep soil layer, and with frequent interactive effects between soil depth and the drought treatment. Under severe water deficit, limited root growth may occur because of low soil water availability and high soil resistance (Cornish et al., 1984; Comas et al., 2005). Accordingly, root proliferation in deep soil, where moisture remains favorable over a longer period of time, would result in a higher proportion of deep to shallow roots, as shown by Skinner et al. (1998) and Skinner (2008). However, we found here effects of recurrent drought on root parameters in B. retusum in the deep soil layer (Figure 1A,B). Also, biomass allocation to the root faction was decreased under recurrent compared to one drought treatment in Q. coccifera only (Shihan et al., 2020). These data suggest that parameters other than root biomass and morphological traits, such as higher soil water content in the deep soil layer, or altered root exudation following recurrent drought (Williams and de Vries, 2019) may be important to explain soil microbial catabolic capabilities.
Because of distinct water use, mortality rates, and plant sizes, the dry-out dynamics, and thus, the total duration of the dry periods, varied among plant combinations and drought treatments. These differences may have influenced root and microbial responses to drought to some degree. However, our previous results about plant survival during the same rhizotron experiment suggest that soil water availability was the key driver for plant survival regardless of the conditions or the duration of drying phases (Shihan et al., 2020). Indeed, like most Mediterranean species, the studied species are adapted to long periods of atmospheric drought.
In line with our initial hypothesis of a stronger drought effect on the drought-tolerant grass species compared to drought-avoiding woody species, we found that B. retusum generally showed strong responses, while the two woody species showed very few adjustments in root trait expression in response to recurrent drought. Under moderate drought, Mediterranean grass species may delay dehydration by increasing water uptake (Volaire et al., 2009), which may in part be achieved by physiological and morphological adjustments of roots. The observed higher SRL, RD and Diam, and lower RTD in the deep soil in B. retusum under recurrent drought is in line with such improved soil water acquisition with fine roots of high SRL or RD, as previously documented in herbaceous species (Freschet et al., 2015; Prieto et al., 2015).
By varying SRL, plants can adjust their capacity to explore the soil and acquire resources (Eissenstat, 1992; Larcher, 2003; Chapman et al., 2012), and thus, can have an important role in how plants respond to drought (Comas et al., 2013). Indeed, a high SRL is correlated with a high surface:volume ratio for the same carbon investment, which is maximizing the root–soil interface and hence the root absorption potential (Larcher, 2003). The high SRL in B. retusum compared to that in the woody species, and the response of SRL to recurrent drought may contribute to the persistence of this species during severe and/or recurrent drought in addition to maintaining only the leaf meristems as an important above-ground drought survival strategy of B. retusum. By increasing SRL when grown together with C. albidus compared to a conspecific neighbor, B. retusum may have increased belowground interspecific competition for limiting water, which may further improve the survival of B. retusum at the expense of a competing neighbor of another species (see Shihan et al., 2020). Increasing SRL may be particularly effective in deeper soil layers, where soil water content diminishes more slowly than in top soil with proceeding drought. Indeed, SRL was higher in B. retusum and C. albidus in deep soil compared to the top soil layer (Table 1 and Figure 2B), however, this soil depth effect was more pronounced in the control treatment opposite to the expected stronger impact with increasing drought frequency. Perhaps greater physical resistance of soils with repetitive drought even in deep soil layers may have led to somewhat denser root tissues as suggested elsewhere (Whitmore and Whalley, 2009; Bengough et al., 2011). In addition to its importance for the penetration of dense or dry (and hard) soils, RTD also reflects anatomical differences among species (Ryser, 1998; Wahl and Ryser, 2000). High RTD implies high biomass investment per unit length of roots, which is opposite to the strategy of high spatial spread of roots. From allometric relationships, RTD is expected to be negatively correlated with SRL (Ryser and Lambers, 1995), as shown in our experiment with a negative relationship between RTD and SRL (Supplementary Figure 6). Other studies did not always observe this expected relationships in either woody (Comas et al., 2002; Comas and Eissenstat, 2004, 2009) or grass species (Picon-Cochard et al., 2012).
Both, smaller root diameters or lower root tissue densities can lead to higher SRL. Accordingly, we observed negative correlations between SRL and root diameter in the two woody species, but not in B. retusum. Although the variability in root diameter did not correlate with that in SRL and was not affected by recurrent drought in B. retusum, roots got thinner when B. retusum grew together with C. albidus, indicating some plasticity in B. retusum root diameter in response to interspecific competition, but not to recurrent drought.
Also, small root diameters together with high SRL, i.e., high surface area in contact with soil water, would translate into an increased hydraulic conductance by decreasing the apoplastic barrier of water entering the xylem (Eissenstat and Achor, 1999; Hernández et al., 2010; Comas et al., 2012). Indeed, a decrease in root diameter has been proposed as an efficient strategy to improve plant acquisition of water and productivity under drought condition (Wasson et al., 2012). Both woody and herbaceous plants adapted to dry conditions were found to have smaller fine root diameter and greater SRL as compared to plants that were not drought-adapted (Hernández et al., 2010; Henry et al., 2012). Our study confirms these responses for Q. coccifera in the top (drier) soil layer, suggesting that the Q. coccifera is more competitive for soil water resources under recurrent drought than C. albidus. This later result is in line with (Rodriguez-Ramirez et al., 2018) showing that Q. coccifera was more tolerant to aggravated drought than C. albidus in a Mediterranean garrigue. Finally, we could not conclude about more pronounced effects of recurrent drought under intra- compared to inter-specific competition (our second hypothesis), probably in part because of the small numbers of individuals that survived (especially in C. albidus). Our data rather suggest that the identity of both the target individual and the neighbor individual, and their strategy facing drought, are important to understand the species-specific response to drought.
While several root traits (essentially in B. retusum) were affected by drought and by the identity of the neighbor species, and sometimes depended on soil depth, the CLPP parameters (sum15, H′, and D) responded neither to plant species composition nor to recurrent drought. Among the 15 different substrates provided to soil microbial communities, only xylan and serine showed drought effects on microbial respiration rates in interaction with plant species composition and soil depth, respectively. Because plant resource acquisition strategies generally influence the soil microbial communities and their functioning (Orwin et al., 2010; de Vries et al., 2012; Legay et al., 2014), the discrepancy between the responses of root traits and those of microbial communities was rather surprising. One possible explanation could be related to the fact that we could not neatly distinguish between plant-species specific soil influence zones in the rhizotrons with two plant species and intermingled root systems, from which we took the soil cores for microbial analyses. In a previous field study with shrub species in different combinations, the experimentally applied moderate rain-exclusion did slightly impact soil CLPP while the shrub species composition overall had a stronger impact (Fromin et al., 2020). Under drought condition, soil microbial respiration generally decreases (Schimel et al., 2007; Talmon et al., 2011; Bérard et al., 2015 for review). Such a drought effect was not observed here, although the global soil microbial activity was higher in the deep, wetter soil layer. This could be explained by the overall wetter deep soil layer, but may be explained by root parameters that change in the deep compared to top soil layer. Also, the weak effect of recurrent drought could be explained by the 3 week-period under non-stressing soil humidity after the last drought event, during which soil microbial communities could have recovered from drought more rapidly than root systems.
Regardless of the drought treatment and plant species combinations, we observed clear differences between the two soil layers. Total microbial activity was higher (although root biomass decreased in the woody species), but the diversity of substrates used was lower in the deep soil layer. Because several parameters changed concomitantly in the two soil layers, such as higher soil humidity in deep compared to top soil (Supplementary Table 2) or differences in root traits (Table 2 and Supplementary Table 4), it is difficult to decipher causality for these differences between soil layers. The lower microbial metabolic diversity in deep soil may be rather a consequence of changes in the impact of roots and their traits than that of moister soil conditions. Indeed, previous studies reported changes in the microbial community structure, such as lower microbial diversity and abundance or increased dominance of drought-resistant taxa in response to drier conditions (Wilkinson et al., 2002; Schimel et al., 2007; Bérard et al., 2011). On the other hand, microbial metabolic parameters were poorly correlated to root functional identity (TraitRWM) and dissimilarity (TraitFD). Four of the five TraitRWM were weakly but significantly correlated with rSIR for lysine as the sole substrate, although we miss arguments to explain the ecological relevance of this substrate. Only a few studies addressed the issue of how resource acquisition/conservation strategies of plants are related to soil microbial functioning (Grigulis et al., 2013, Legay et al., 2014; De Long et al., 2019), but this was rarely addressed in a context of water shortage and for root traits. For example, Lõhmus et al., 2006a,b showed that the specific root area of short roots was positively correlated with the functional diversity and activity of microbial communities in the soil. In our study, we found that the global microbial activity, but not the functional diversity, was nicely explained by the dissimilarity in several root traits. In a previous field study (Shihan et al., 2017), we reported significant effects of functional dissimilarity in traits of litter mixture decomposing at the soil surface on soil microbial parameters. Here, RTD and root diameter [that control the length and surface area of root systems for a given biomass allocated to the roots (Fitter, 2002)] reflect the amount of surface directly interacting with soil, such as the root surface colonized by mycorrhizal fungi (Smith and Read, 2002). Also, roots with higher mean root traits (in our study DiamRWM) promote higher soil metabolic diversity and roots with contrasting functional traits may produce exudates of contrasted chemical composition, favoring different microbial groups that differ in their metabolic capabilities.
Collectively, our results showed that both drought treatment and plant species composition affected the response of root morphological traits in the studied three plant species. In line with our first hypothesis, we measured stronger responses in the dehydration tolerant grass species to drought and to changing species identity of the neighbor plant compared to the two woody species. Accordingly, with the three species studies here, there was no clear stronger effect of intraspecific competition on the root and microbial responses than that of interspecific competition. Rather, species identity effects driven mostly by the contrast between grass and woody species were the main driver of the observed responses to recurrent drought and neighbor plant identity. This may point towards shifts in the dominance of growth forms as the primary consequences in these drought-prone Mediterranean ecosystems. However, an extended pool of studied species may yield different results for root responses in future studies, but not necessarily for soil microbial substrate utilization patterns that differed mostly between deep and top soil layers, but were not directly affected by drought or species composition.
Data availability statement
The original contributions presented in this study are publicly available. These data can be found here: https://data.indores.fr/dataset.xhtml?persistentId=doi:10.48579/PRO/UZGBKB.
Author contributions
AS and SH conceived and designed the experiment. AS planned and conducted the experiment with assistance of SH and NF, and led data acquisition, analysis, and manuscript redaction. AS, SH, and NF edited the manuscript. All authors contributed to the article and approved the submitted version.
Funding
This study was funded by the French National Research Agency (ANR) as part of the CLIMED research project (contract “ANR-09-CEP-007”) and through a grant of Aleppo University, Syria to AS.
Acknowledgments
We are grateful to David Degueldre, Jeremy Devaux, Pauline Durbin, and Thierry Mathieu from the platform “Terrain d’expérimentations” [supported by the LabEx CeMEB and ANR “Investissements d’avenir” program (ANR-10-LABX-04-01)] for their continued support and help in the planning and maintenance of the experiment and help during plant harvest. We also thank Catherine Roumet and Pascal Chapon for their advice and technical support and Florence Volaire for helpful comments during writing.
Conflict of interest
The authors declare that the research was conducted in the absence of any commercial or financial relationships that could be construed as a potential conflict of interest.
Publisher’s note
All claims expressed in this article are solely those of the authors and do not necessarily represent those of their affiliated organizations, or those of the publisher, the editors and the reviewers. Any product that may be evaluated in this article, or claim that may be made by its manufacturer, is not guaranteed or endorsed by the publisher.
Supplementary material
The Supplementary Material for this article can be found online at: https://www.frontiersin.org/articles/10.3389/ffgc.2022.921191/full#supplementary-material
References
Arndt, S. K., Arampatsis, C., Foetzki, A., Li, X., Zeng, F., and Zhang, X. (2004). Contrasting patterns of leaf solute accumulation and salt adaptation in four phreatophytic desert plants in a hyperarid desert with saline groundwater. J. Arid Environ. 59, 259–270. doi: 10.1016/j.jaridenv.2004.01.017
Aslam, M. M., Okal, E. J., Idris, L., Qian, Z., Xu, W., Karanja, J. K., et al. (2022). Rhizosphere microbiomes can regulate plant drought tolerance. Pedosphere 32, 61–74. doi: 10.1016/S1002-0160(21)60061-9
Bardgett, R. D., Mommer, L., and De Vries, F. T. (2014). Going underground: Root traits as drivers of ecosystem processes. Trends Ecol. Evol. 29, 692–699. doi: 10.1016/j.tree.2014.10.006
Bates, D. M., and Pinheiro, J. C. (1998). Linear and nonlinear mixed-effects models. Conf. Appl. Stat. Agric. 1998:1273. doi: 10.4148/2475-7772.1273
Bengough, A. G., McKenzie, B. M., Hallett, P. D., and Valentine, T. A. (2011). Root elongation, water stress, and mechanical impedance: A review of limiting stresses and beneficial root tip traits. J. Exp. Bot. 62, 59–68. doi: 10.1093/jxb/erq350
Bérard, A., Ben Sassi, M., Kaisermann, A., and Renault, P. (2015). Soil microbial community responses to heat wave components: drought and high temperature. Clim. Res. 66, 243–264. doi: 10.3354/cr01343
Bérard, A., Bouchet, T., Sévenier, G., Pablo, A., and Gros, R. (2011). Resilience of soil microbial communities impacted by severe drought and high temperature in the context of Mediterranean heat waves. Eur. J. Soil Biol. 47, 333–342. doi: 10.1016/j.ejsobi.2011.08.004
Bergmann, J., Weigelt, A., van der Plas, F., Laughlin, D. C., Kuyper, T. W., Guerrero-Ramirez, N., et al. (2020). The fungal collaboration gradient dominates the root economic space in plants. Sci. Adv. 6:3756. doi: 10.1126/sciadv.aba3756
Birouste, M., Kazakou, E., Blanchard, A., and Roumet, C. (2012). Plant traits and decomposition: Are the relationships for roots comparable to those for leaves? Ann. Bot. 109, 463–472. doi: 10.1093/aob/mcr297
Botta-Dukát, Z. (2005). Rao’s quadratic entropy as a measure of functional diversity based on multiple traits. J. Veg. Sci. 16, 533–540. doi: 10.1111/j.1654-1103.2005.tb02393.x
Brunner, I., Herzog, C., Dawes, M. A., Arend, M., and Sperisen, C. (2015). How tree roots respond to drought. Front. Plant. Sci. 6:547. doi: 10.3389/fpls.2015.00547
Chai, Y. N., and Schachtman, D. P. (2022). Root exudates impact plant performance under abiotic stress. Trends Plant Sci. 27, 80–91. doi: 10.1016/j.tplants.2021.08.003
Chapman, N., Miller, A. J., Lindsey, K., and Whalley, W. R. (2012). Roots, water, and nutrient acquisition: Let’s get physical. Trends Plant Sci. 17, 701–710. doi: 10.1016/j.tplants.2012.08.001
Comas, L. H., Anderson, L. J., Dunst, R. M., Lakso, A. N., and Eissenstat, D. M. (2005). Canopy and environmental control of root dynamics in a long-term study of concord grape. New Phytol. 167, 829–840. doi: 10.1111/j.1469-8137.2005.01456.x
Comas, L. H., Becker, S. R., Cruz, V. M. V., Byrne, P. F., and Dierig, D. A. (2013). Root traits contributing to plant productivity under drought. Front. Plant Sci. 4:442. doi: 10.3389/fpls.2013.00442
Comas, L. H., Bouma, T. J., and Eissenstat, D. M. (2002). Linking root traits to potential growth rate in six temperate tree species. Oecologia 132, 34–43. doi: 10.1007/s00442-002-0922-8
Comas, L. H., and Eissenstat, D. M. (2004). Linking fine root traits to maximum potential growth rate among 11 mature temperate tree species. Funct. Ecol. 18, 388–397. doi: 10.1111/j.0269-8463.2004.00835.x
Comas, L. H., and Eissenstat, D. M. (2009). Patterns in root trait variation among 25 co-existing North American forest species. New Phytol. 182, 919–928. doi: 10.1111/j.1469-8137.2009.02799.x
Comas, L. H., Mueller, K. E., Taylor, L. L., Midford, P. E., Callahan, H. S., and Beerling, D. J. (2012). Evolutionary patterns and biogeochemical significance of angiosperm root traits. Int. J. Plant Sci. 173, 584–595. doi: 10.1086/665823
Cornish, P. S., So, H. B., and McWilliam, J. R. (1984). Effects of soil bulk density and water regimen on root growth and uptake of phosphorus by ryegrass. Aust. J. Agric. Res. 35, 631–644. doi: 10.1071/AR9840631
Damesin, C., Rambal, S., and Joffre, R. (1998). Co-occurrence of trees with different leaf habit: A functional approach on Mediterranean oaks. Acta Oecol. 19, 195–204. doi: 10.1016/S1146-609X(98)80024-6
De Long, J. R., Jackjson, B. G., Wilkinson, A., Pritchard, W. J., Oakley, S., Mason, K. E., et al. (2019). Relationships between plant traits, soil properties and carbon fluxes differ between monocultures and mixed communities in temperate grassland. J. Ecol. 107, 1704–1719. doi: 10.1111/1365-2745.13160
de Vries, F. T., Manning, P., Tallowin, J. R. B., Mortimer, S. R., Pilgrim, E. S., Harrison, K. A., et al. (2012). Abiotic drivers and plant traits explain landscape-scale patterns in soil microbial communities. Ecol. Lett. 15, 1230–1239. doi: 10.1111/j.1461-0248.2012.01844.x
Díaz, S., Kattge, J., Cornelissen, J. H., Wright, I. J., Lavorel, S., Dray, S., et al. (2016). The global spectrum of plant form and function. Nature 14, 167–171. doi: 10.1038/nature16489
Eissenstat, D. (1992). Costs and benefits of constructing roots of small diameter. J. Plant Nutr. 15, 763–782. doi: 10.1080/01904169209364361
Eissenstat, D. M., and Achor, D. S. (1999). Anatomical characteristics of roots of citrus rootstocks that vary in specific root length. New Phytol. 141, 309–321. doi: 10.1046/j.1469-8137.1999.00342.x
Eviner, V. T., and Chapin, F. S. (2003). “Biogeochemical interactions and biodiversity,” in Interactions of the Major Biogeochemical Cycles: Global Change and Human Impacts, eds J. M. Melillo, C. B. Field, and B. Moldan (Washington, DC: Island Press).
Fan, X., Hu, H., Huang, G., Huang, F., and Palta, J. (2015). Soil inoculation with Burkholderia sp. LD-11 has positive effect on water-use efficiency in inbred lines of maize. Plant Soil 390, 337–349. doi: 10.1007/s11104-015-2410-z
Ferrio, J. P., Florit, A., Vega, A., Serrano, L., and Voltas, J. (2003). Δ13C and tree-ring width reflect different drought responses in Quercus ilex and Pinus halepensis. Oecologia 137, 512–518. doi: 10.1007/s00442-003-1372-7
Field, C. B., Barros, V. R., Mach, K. J., Mastrandrea, M. D., et al. (2014). “Technical summary,” in Climate Change 2014: Impacts, Adaptation, and Vulnerability. Part A: Global and Sectoral Aspects. Contribution of Working Group II to the Fifth Assessment Report of the Intergovernmental Panel on Climate Change, eds C. B. Field, V. R. Barros, D. J. Dokken, K. J. Mach, M. D. Mastrandrea, and T. E. Bilir (Cambridge: Cambridge University Press), 35–94.
Forster, P., Huppmann, D., Kriegler, E., Mundaca, L., Smith, C., Rogelj, J., et al. (2018). “Mitigation pathways compatible with 1.5°c in the context of sustainable development supplementary material,” in Global Warming of 1.5°C. An IPCC Special Report on the Impacts of Global Warming of 1.5°C Above Pre-Industrial Levels and Related Global Greenhouse Gas Emission Pathways, in the Context of Strengthening the Global Response to the Threat of Climate Change, Sustainable Development, and Efforts to Eradicate Poverty, eds V. Masson-Delmotte, P. Zhai, H.-O. Pörtner, D. Roberts, and J. Skea.
Fitter, A. (2002). “Characteristics and functions of root systems,” in Plant Roots, the Hidden Half, eds Y. Waisel, A. Eshel, and U. Kafkafi (CRC Press), 21–50.
Freschet, G. T., Swart, E. M., and Cornelissen, J. H. C. (2015). Integrated plant phenotypic responses to contrasting above- and below-ground resources: Key roles of specific leaf area and root mass fraction. New Phytol. 206, 1247–1260. doi: 10.1111/nph.13352
Freschet, G. T., Valverde-Barrantes, O. J., Tucker, C. M., Craine, J. M., McCormak, M. L., Violle, C., et al. (2017). Climate, soil and plant functional types as drivers of global fin-root trait variation. J. Ecol. 105, 1181–1196. doi: 10.1111/1365-2745.12769
Friesen, M. L., Porter, S. S., Stark, S. C., von Wettberg, E. J., Sachs, J. L., and Martinez-Romero, E. (2011). Microbially mediated plant functional traits. Annu. Rev. Ecol. Evol. Syst. 2011:145039. doi: 10.1146/annurev-ecolsys-102710-145039
Fromin, N., Shihan, A., Santonja, M., Baldy, V., and Hättenschwiler, S. (2020). Soil microbial activity in a Mediterranean garrigue responds more to changing shrub community than to reduced rainfall. Plant Soil. 449, 405–421. doi: 10.1007/s11104-020-04501-8
Galmés, J., Medrano, H., and Flexas, J. (2007). Photosynthetic limitations in response to water stress and recovery in Mediterranean plants with different growth forms. New Phytol. 175, 81–93. doi: 10.1111/j.1469-8137.2007.02087.x
Garnier, E., Cortez, J., Billès, G., Navas, M.-L., Roumet, C., Debussche, M., et al. (2004). Plant functional markers capture ecosystem properties during secondary succession. Ecology 85, 2630–2637. doi: 10.1890/03-0799
Garnier, E., Laurent, G., Bellmann, A., Debain, S., Berthelier, P., Ducout, B., et al. (2001). Consistency of species ranking based on functional leaf traits. New Phytol. 152, 69–83. doi: 10.1046/j.0028-646X.2001.00239.x
Gillespie, L., Hättenschwiler, S., Milcu, A., Wambsganss, J., Shihan, A., and Fromin, N. (2021). Tree species mixing affects soil microbial functioning indirectly via root and litter traits and soil parameters in European forests. Funct. Ecol. 35, 2190–2204. doi: 10.1111/1365-2435.13877
Grayston, S. J., Wang, S., Campbell, C. D., and Edwards, A. C. (1998). Selective influence of plant species on microbial diversity in the rhizosphere. Soil Biol. Biochem. 30, 369–378. doi: 10.1016/S0038-0717(97)00124-7
Grigulis, K., Lavorel, S., Krainer, U., Legay, N., Baxendale, C., Dumont, M., et al. (2013). Relative contributions of plant traits and soil microbial properties to mountain grassland ecosystem services. J. Ecol. 101, 47–57. doi: 10.1111/1365-2745.12014
Hasibeder, R., Fuchslueger, L., Richter, A., and Bahn, M. (2015). Summer drought alters carbon allocation to roots and root respiration in mountain grassland. New Phytol. 205, 1117–1127. doi: 10.1111/nph.13146
Henry, A., Cal, A. J., Batoto, T. C., Torres, R. O., and Serraj, R. (2012). Root attributes affecting water uptake of rice (Oryza sativa) under drought. J. Exp. Bot. 63, 4751–4763. doi: 10.1093/jxb/ers150
Hernández, E. I., Vilagrosa, A., Pausas, J. G., and Bellot, J. (2010). Morphological traits and water use strategies in seedlings of Mediterranean coexisting species. Plant Ecol. 207, 233–244. doi: 10.1007/s11258-009-9668-2
Hertel, D., Strecker, T., Müller-Haubold, H., and Leuschner, C. (2013). Fine root biomass and dynamics in beech forests across a precipitation gradient – is optimal resource partitioning theory applicable to water-limited mature trees? J. Ecol. 101, 1183–1200. doi: 10.1111/1365-2745.12124
Hertig, E., and Tramblay, Y. (2017). Regional downscaling of Mediterranean droughts under past and future climatic conditions. Glob. Planet. Change 151, 36–48. doi: 10.1016/j.gloplacha.2016.10.015
Hodge, A. (2004). The plastic plant: Root responses to heterogeneous supplies of nutrients. New Phytol. 162, 9–24. doi: 10.1111/j.1469-8137.2004.01015.x
Huang, B., and Eissenstat, D. M. (2000). Linking hydraulic conductivity to anatomy in plants that vary in specific root length. J. Am. Soc. Hortic. Sci. 125, 260–264. doi: 10.21273/JASHS.125.2.260
Isbell, F., Craven, D., Connolly, J., Loreau, M., Schmid, B., Beierkuhnlein, C., et al. (2015). Biodiversity increases the resistance of ecosystem productivity to climate extremes. Nature 526, 574–577. doi: 10.1038/nature15374
Jobbágy, E. G., and Jackson, R. B. (2001). The distribution of soil nutrients with depth: Global patterns and the imprint of plants. Biogeochemistry 53, 51–77. doi: 10.1023/A:1010760720215
Kannenberg, S. A., and Phillips, R. P. (2017). Soil microbial communities buffer physiological responses to drought stress in three hardwood species. Oecologia 183, 631–641. doi: 10.1007/s00442-016-3783-2
Laclau, J.-P., da Silva, E. A., Rodrigues Lambais, G., Bernoux, M., Le Maire, G., Stape, J. L., et al. (2013). Dynamics of soil exploration by fine roots down to a depth of 10 m throughout the entire rotation in Eucalyptus grandis plantations. Front. Plant Sci. 4:243. doi: 10.3389/fpls.2013.00243
Laliberte, E., and Legendre, P. (2010). A distance-based framework for measuring functional diversity from multiple traits. Ecology 91, 299–305. doi: 10.1890/08-2244.1
Larcher, W. (2003). Physiological Plant Ecology: Ecophysiology and Stress Physiology of Functional Groups. Berlin: Springer-Verlag Berlin and Heidelberg GmbH & Co.
Larson, J. E., and Funk, J. L. (2016). Seedling root responses to soil moisture and the identification of a belowground trait spectrum across three growth forms. New Phytol. 210, 827–838. doi: 10.1111/nph.13829
Leff, J. W., Nemergut, D. R., Grandy, A. S., O’Neill, S. P., Wickings, K., Townsend, A. R., et al. (2012). The effects of soil bacterial community structure on decomposition in a tropical rain forest. Ecosystems 15, 284–298. doi: 10.1007/s10021-011-9510-2
Legay, N., Baxendale, C., Grigulis, K., Krainer, U., Kastl, E., Schloter, M., et al. (2014). Contribution of above- and below-ground plant traits to the structure and function of grassland soil microbial communities. Ann. Bot. 114, 1011–1021. doi: 10.1093/aob/mcu169
Leibold, M. A. (1995). The niche concept revisited: Mechanistic models and community context. Ecology 76, 1371–1382. doi: 10.2307/1938141
Lenth, R. V. (2016). Least-squares means: The R package lsmeans. J. Stat. Softw 2016:e01. doi: 10.18637/jss.v069.i01
Lloret, F., Casanovas, C., and Peñuelas, J. (1999). Seedling survival of Mediterranean shrubland species in relation to root: Shoot ratio, seed size and water and nitrogen use. Funct. Ecol. 13, 210–216. doi: 10.1046/j.1365-2435.1999.00309.x
Lõhmus, K., Truu, J., Truu, M., Kaar, E., Ostonen, I., Alama, S., et al. (2006a). Black alder as a promising deciduous species for the reclaiming of oil shale mining areas. WIT Trans. Ecol. Environ. 94, 87–97. doi: 10.2495/BF060091
Lõhmus, K., Truu, M., Truu, J., Ostenen, I., Kaar, E., Vares, A., et al. (2006b). Functional diversity of culturable bacterial communities in the rhizosphere in relation to fine-root and soil parameters in alder stands on forest, abandoned agricultural, and oil-shale mining areas. Plant Soil 283, 1–10. doi: 10.1007/s11104-005-2509-8
Ma, Z., Guo, D., Xu, X., Lu, M., Bardgett, R., Eissenstat, D. M., et al. (2018). Evolutionary history resolves global organization of root functional traits. Nature 555, 94–97. doi: 10.1038/nature25783
Markesteijn, L., and Poorter, L. (2009). Seedling root morphology and biomass allocation of 62 tropical tree species in relation to drought- and shade-tolerance. J. Ecol. 97, 311–325. doi: 10.1111/j.1365-2745.2008.01466.x
McCormack, M. L., Dickie, I. A., Eissenstat, D. M., Fahey, T. J., Fernandez, C. W., Guo, D., et al. (2015). Redefining fine roots improves understanding of below-ground contributions to terrestrial biosphere processes. New Phytol. 207, 505–518. doi: 10.1111/nph.13363
Naseem, H., Ahsan, M., Shahid, M. A., and Khan, N. (2018). Exopolysaccharides producing rhizobacteria and their role in plant growth and drought tolerance. J. Basic Microbiol. 58, 1009–1022. doi: 10.1002/jobm.201800309/
Nicotra, A., Babicka, N., and Westoby, M. (2002). Seedling root anatomy and morphology: An examination of ecological differentiation with rainfall using phylogenetically independent contrasts. Oecologia 130, 136–145. doi: 10.1007/s004420100788
Orwin, K. H., Buckland, S., Johnson, D., Turner, B. L., Smart, S., Oakley, S., et al. (2010). Linkages of plant traits to soil properties and the functioning of temperate grassland. J. Ecol. 98, 1074–1083. doi: 10.1111/j.1365-2745.2010.01679.x
Ostonen, I., Lõhmus, K., Helmisaari, H.-S., Truu, J., and Meel, S. (2007). Fine root morphological adaptations in scots pine, norway spruce and silver birch along a latitudinal gradient in boreal forests. Tree Physiol. 27, 1627–1634. doi: 10.1093/treephys/27.11.1627
Padilla, F. M., Miranda, J., Armas, C., and Pugnaire, F. I. (2015). Effects of changes in rainfall amount and pattern on root dynamics in an arid shrubland. J. Arid Environ. 114, 49–53. doi: 10.1016/j.jaridenv.2014.11.005
Paula, S., and Pausas, J. G. (2011). Root traits explain different foraging strategies between resprouting life histories. Oecologia 165, 321–331. doi: 10.1007/s00442-010-1806-y
Peman, J., Voltas, J., and Gil-Pelegrin, E. (2006). Morphological and functional variability in the root system of Quercus ilex L. subject to confinement: Consequences for afforestation. Ann. For. Sci. 63, 425–430. doi: 10.1051/forest:2006022
Pérez-Ramos, I. M., Volaire, F., Fattet, M., Blanchard, A., and Roumet, C. (2013). Tradeoffs between functional strategies for resource-use and drought-survival in Mediterranean rangeland species. Environ. Exp. Bot. 87, 126–136. doi: 10.1016/j.envexpbot.2012.09.004
Picon-Cochard, C., Pilon, R., Tarroux, E., Pagès, L., Robertson, J., and Dawson, L. (2012). Effect of species, root branching order and season on the root traits of 13 perennial grass species. Plant Soil 353, 47–57. doi: 10.1007/s11104-011-1007-4
Prieto, I., Roumet, C., Cardinael, R., Dupraz, C., Jourdan, C., Kim, J. H., et al. (2015). Root functional parameters along a land-use gradient: Evidence of a community-level economics spectrum. J. Ecol. 103, 361–373. doi: 10.1111/1365-2745.12351
Rapparini, F., and Peñuelas, J. (2014). “Mycorrhizal fungi to alleviate drought stress on plant growth,” in Use of Microbes for the Alleviation of Soil Stresses, ed. M. Miransari (New York: Springer Science+Business Media), doi: 10.1007/978-1-4614-9466-9_2
R Core Team (2020). R: A Language and Environment for Statistical Computing. Vienna: R Foundation for Statistical Computing. Available online at: https://www.R-project.org/
Reich, P. B., Wright, I. J., Cavender-Bares, J., Craine, J. M., Oleksyn, J., Westoby, M., et al. (2003). The evolution of plant functional variation: traits, spectra, and strategies. Int. J. Plant Sci. Int. J. Plant Sci. 1643, 143–164. doi: 10.1086/374368
Rodriguez-Ramirez, N., Santonja, M., Baldy, V., Ballini, C., and Montes, N. (2018). Shrub species richness decreases negative impacts of drought in a Mediterranean ecosystem. J. Veg. Sci. 28, 985–996. doi: 10.1111/jvs.12558
Roumet, C., Lafont, F., Sari, M., and Warembourg, F. (2008). Root traits and taxonomic affiliation of nine herbaceous species grown in glasshouse conditions. Plant Soil 312, 69–83. doi: 10.1007/s11104-008-9635-z
Ryser, P. (1998). Intra- and Interspecific Variation in Root Length, Root Turnover and the Underlying Parameters. Leiden: Backhuys Publishers.
Ryser, P., and Lambers, H. (1995). Root and leaf attributes accounting for the performance of fast- and slow-growing grasses at different nutrient supply. Plant Soil 170, 251–265. doi: 10.1007/BF00010478
Schenk, H. J. (2008). Soil depth, plant rooting strategies and species’ niches. New Phytol. 178, 223–225. doi: 10.1111/j.1469-8137.2008.02427.x
Schimel, J. P., Balser, T. C., and Wallenstein, M. (2007). Microbial stress-response physiology and its implications for ecosystem function. Ecology 88, 1386–1394. doi: 10.1890/06-0219
Schimel, J. P. (2018). Life in dry soils: Effects of drought on soil microbial communities and processes. Ann. Rev. Ecol. Evol. Syst. 49, 409–432.
Shihan, A., Hättenschwiler, S., Milcu, A., Joly, F.-X., Santonja, M., and Fromin, N. (2017). Changes in soil microbial substrate utilization in response to altered litter diversity and precipitation in a Mediterranean shrubland. Biol. Fertil. Soils 53, 171–185. doi: 10.1007/s00374-016-1166-9
Shihan, A., Volaire, F., and Hättenschwiler, S. (2020). Neighbor identity affects growth and survival of Mediterranean plants under recurrent drought. Oecologia 194, 555–569. doi: 10.1007/s00442-020-04739-0
Skinner, H. R., and Comas, L. H. (2010). Root distribution of temperate forage species subjected to water and nitrogen stress. Crop Sci. 50, 2178–2185. doi: 10.2135/cropsci2009.08.0461
Skinner, R. H. (2008). Yield, root growth, and soil water content in drought-stressed pasture mixtures containing chicory. Crop Sci. 48, 380–388. doi: 10.2135/cropsci2007.04.0201
Skinner, R. H., Hanson, J. D., and Benjamin, J. G. (1998). Root distribution following spatial separation of water and nitrogen supply in furrow irrigated corn. Plant Soil 199, 187–194. doi: 10.1023/A:1004369227455
Song, F., Han, X., Zhu, X., and Herbert, S. J. (2012). Response to water stress of soil enzymes and root exudates from drought and non-drought tolerant corn hybrids at different growth stages. Can. J. Soil Sci. 92, 501–507. doi: 10.4141/CJSS2010-057
Talmon, Y., Sternberg, M., and Grünzweig, J. M. (2011). Impact of rainfall manipulations and biotic controls on soil respiration in Mediterranean and desert ecosystems along an aridity gradient. Glob. Change Biol. 17, 1108–1118. doi: 10.1111/j.1365-2486.2010.02285.x
Turnbull, L. A., Levine, J. M., Loreau, M., and Hector, A. (2013). Coexistence, niches and biodiversity effects on ecosystem functioning. Ecol. Lett. 16, 116–127. doi: 10.1111/ele.12056
Valverde-Barrantes, O. J., Maherali, H., Baroloto, C., and Blackwood, C. B. (2020). Independent evolutionary changes in fine-root traits among main clades during the diversification of seed plants. New Phytol. 228, 541–553. doi: 10.1111/nph.16729
Volaire, F., Norton, M. R., and Lelièvre, F. (2009). Summer drought survival strategies and sustainability of perennial temperate forage grasses in Mediterranean areas. Crop Sci. 4, 2386–2392. doi: 10.2135/cropsci2009.06.0317
Wagg, C., O’Brien, M. J., Vogel, A., Scherer-Lorenzen, M., Eisenhauer, M., Schmid, B., et al. (2017). Plant diversity maintains long-term ecosystem productivity under frequent drought by increasing short-term variation. Ecology 98, 2952–2961. doi: 10.1002/ecy.2003
Wahl, S., and Ryser, P. (2000). Root tissue structure is linked to ecological strategies of grasses. New Phytol. 148, 459–471. doi: 10.1046/j.1469-8137.2000.00775.x
Wang, Y., Li, Z., Wang, Z., and Gu, J. (2020). Functional trait plasticity but not coordination differs in absorptive and transport fine roots in response to soil depth. Forests 11:42. doi: 10.3390/f11010042
Wardle, D. A., Bardgett, R. D., Klironomos, J. N., Setälä, H., van der Putten, W., and Wall, D. H. (2004). Ecological linkages between aboveground and belowground biota. Science 304, 1629–1633. doi: 10.1126/science.1094875
Wasson, A. P., Richards, R. A., Chatrath, R., Misra, S. C., Sai Prasad, S. V., Rebetzke, G. J., et al. (2012). Traits and selection strategies to improve root systems and water uptake in water-limited wheat crops. J. Exp. Bot. 63, 3485–3498. doi: 10.1093/jxb/ers111
Whitmore, A. P., and Whalley, W. R. (2009). Physical effects of soil drying on roots and crop growth. J. Exp. Bot. 60, 2845–2857. doi: 10.1093/jxb/erp200
Wilkinson, S. C., Anderson, J. M., Scardelis, S. P., Tisiafouli, M., Taylor, A., and Wolters, V. (2002). PLFA profiles of microbial communities in decomposing conifer litters subject to moisture stress. Soil Biol. Biochem. 34, 189–200. doi: 10.1016/S0038-0717(01)00168-7
Williams, A., and de Vries, F. T. (2019). Plant root exudation under drought: implications for ecosystem functioning. New Phytol. 225, 1899–1905. doi: 10.1111/nph.16223
Wright, I. J., Reich, P. B., Cornelissen, J. H. C., Falster, D. S., Garnier, E., Hikosaka, K., et al. (2005). Assessing the generality of global leaf trait relationships. New Phytol. 166, 485–496. doi: 10.1111/j.1469-8137.2005.01349.x
Keywords: root functional traits, soil microbial activity, drought, inter- vs. intra-specific competition, belowground response, shrubland
Citation: Shihan A, Hättenschwiler S and Fromin N (2022) Root traits rather than functional diversity of soil microorganisms respond to drought and plant species composition in Mediterranean shrubland species. Front. For. Glob. Change 5:921191. doi: 10.3389/ffgc.2022.921191
Received: 15 April 2022; Accepted: 07 September 2022;
Published: 29 September 2022.
Edited by:
Yolima Carrillo, Western Sydney University, AustraliaReviewed by:
Jörg Luster, Swiss Federal Institute for Forest, Snow and Landscape Research (WSL), SwitzerlandManjunatha Chandregowda, Western Sydney University, Australia
Copyright © 2022 Shihan, Hättenschwiler and Fromin. This is an open-access article distributed under the terms of the Creative Commons Attribution License (CC BY). The use, distribution or reproduction in other forums is permitted, provided the original author(s) and the copyright owner(s) are credited and that the original publication in this journal is cited, in accordance with accepted academic practice. No use, distribution or reproduction is permitted which does not comply with these terms.
*Correspondence: Nathalie Fromin, nathalie.fromin@cefe.cnrs.fr
†Present address: Nathalie Fromin, CNRS, Odeillo, France