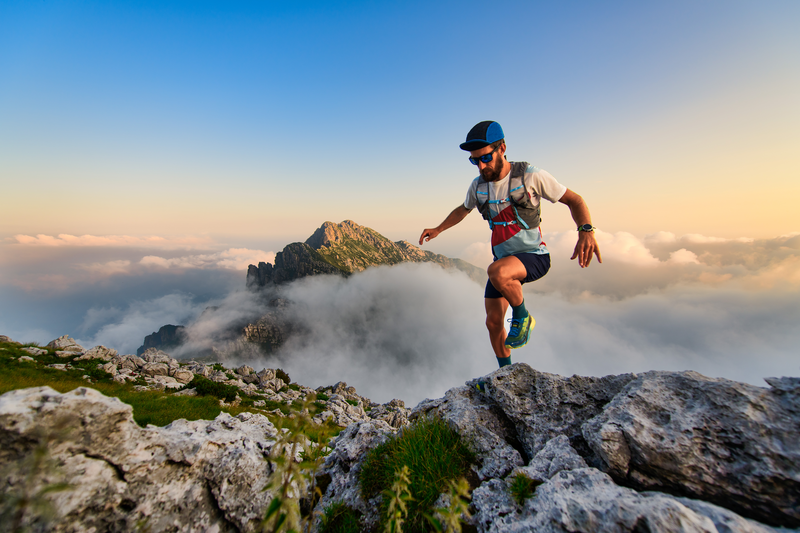
95% of researchers rate our articles as excellent or good
Learn more about the work of our research integrity team to safeguard the quality of each article we publish.
Find out more
ORIGINAL RESEARCH article
Front. For. Glob. Change , 07 July 2022
Sec. Tropical Forests
Volume 5 - 2022 | https://doi.org/10.3389/ffgc.2022.913426
This article is part of the Research Topic Monitoring and Predicting Bamboo Forest Dynamics Under a Changing Climate View all 4 articles
Moso bamboo (Phyllostachys pubescens) forests are utilized for food, building materials, and carbon fixation in East Asia. Hence, understanding the factors that influence productivity is important. Long-term records of managed Moso bamboo forests have provided evidence for 2-year cycles of new shoot production. A widely accepted explanatory hypothesis is that the 2-year leaf life span and unequal proportions of newer and older leaves in bamboo stands are the cause of the 2-year shoot production cycle. However, 2-year cycles are not observed in all circumstances. If the 2-year leaf life span causes the biennial production cycle, why are the 2-year cycles of new shoot production not observed in some periods? By constructing an age-structured population growth model that considered the Moso bamboo leaf life span, this study aimed to clarify the possible mechanisms that could suppress the 2-year cycle of new shoot production. The simulation demonstrated that the 2-year cycle may readily disappear because of the contribution of considerable carbohydrates originating from photosynthesis in old leaves and in new leaves of zero-year-old culms, and from belowground carbon storage in roots and rhizomes. The results suggested that the contribution of photosynthesis in old leaves and in new leaves of zero-year-old culms may be overlooked at the population scale, and that belowground carbon storage in Moso bamboo rhizome systems might act as buffer to stabilize the year-to-year variations in new shoot production.
Bamboo is the common term for a group of woody grasses belonging to the family Poaceae. Bamboo species are distributed widely, growing naturally in tropical and subtropical regions on all continents except Europe and Antarctica, and from sea level to 4,300 m elevation (Clark et al., 2015). Moso bamboo (Phyllostachys pubescens) is a monopodial species that dominates a major forest type in East Asia. The species is native to southern China and was introduced to neighboring regions for practical utilization for food and materials (Benton, 2015). Unlike tree species, new shoots of Moso bamboo sprout in early spring and complete their growth within 2 months (Song et al., 2016). Subsequently, no further annual height and radial growth occurs; thus, new shoot emergence in spring determines the annual aboveground productivity in Moso bamboo forest. Recently, Moso bamboo forest has been considered to be an important carbon sink and increasing attention has focused on its ecological benefits, such as for climate change mitigation (Shinohara et al., 2014; Lin et al., 2017; Xu et al., 2018; Zhou et al., 2019). Hence, an understanding of new shoot productivity is important to formulate appropriate management practices in bamboo forests.
Two-year cycles in shoot production have been observed in many Moso bamboo stands: a high number of new shoots are produced in a “good” year, followed by a “poor” year with development of a low number of new shoots (Li et al., 1998a; Kleinhenz and Midmore, 2001; Song et al., 2016). Two-year cycles in shoot production have also been observed in many species of fruit trees, such as apple and pear (Monselise and Goldschmidt, 1982). Recent studies suggest that at the individual scale, after a good year, a fruit tree takes time to recover from depletion of carbohydrate reserves; at the stand level, abnormal weather may act as a trigger to synchronize the fruiting cycles of individual trees (Silvertown and Charlesworth, 2009). Moso bamboo is well known for extremely rapid vegetative growth rate, and its fastest growth rate could reach 1-m day–1 (Xu et al., 2011; Li et al., 2018). Unlike fruit trees, which take time to recover from the reproductive costs, new shoots of Moso bamboo elongate rapidly and then initiate photosynthesis, thereby contributing newly synthesized carbohydrates to the bamboo stand (Wang et al., 2020). Thus, competition between shoot production and vegetative carbon sinks is unlikely, suggesting that the mechanism responsible for the periodic population dynamic of Moso bamboo forests may differ from that of fruit trees.
Long-term records in East Asian countries support the distinctive 2-year cycle of new shoot production by Moso bamboo. One explanatory hypothesis has been widely accepted: the 2-year leaf life span combined with the imbalance between “even” and “odd” culm-age structure at the population scale leads to the 2-year production cycle (Li et al., 1998a). The life span of Moso bamboo leaves is mainly 2 years; that is, the life-span of leaves of zero-year-old culms is 1 year, whereas the life-span of all leaves of ≥1-year-old culms is 2 years. Furthermore, all leaves of an individual are of an identical age, and all culms of a specific age bear leaves of the same age. In this manner, the leaves of zero-year-old culms and odd-age culms are new leaves (i.e., 1st year leaves). In contrast, the leaves of even-aged culms are old leaves (i.e., 2nd year leaves) (Li et al., 1998a). The photosynthesis rate of new leaves is much higher than that of old leaves (Huang et al., 1989), leading to more substantial contribution of carbohydrate from new leaves than from old leaves to new shoot production. Thus, the number of new shoots is positively correlated with the proportion of bamboo culms with new leaves in a stand. If for any reason (e.g., disturbance or management practices) the ratio between the number of mature culms with new leaves and those with old leaves deviates from 1:1, the bamboo stand will include a higher proportion of new (or old) leaves in a given year and a higher proportion of old (or new) leaves in the following year. This mechanism was interpreted by Li et al. (1998a) and has been widely accepted to explain the marked biennial alternation in Moso bamboo new shoot production.
Although the 2-year cycle of new shoot production in managed forests has been widely observed, 2-decade observation of the productivity of Moso bamboo (Li et al., 1998a) revealed that the 2-year cycle was not obvious in certain periods. Why is the 2-year cycle of new shoot production not obvious in some periods if the 2-year leaf life span causes the biennial cycle in Moso bamboo forest? Possible reasons for suppression of the 2-year cycle may involve (1) photosynthesis in the old leaves of even-aged culms, (2) photosynthesis in the new leaves of zero-year-old culms, and (3) belowground carbon allocation and storage. First, the contribution of old leaves (with a lower photosynthesis rate) to total carbon assimilation might not be negligible at the population scale and may be sufficient to suppress the year-to-year variation in new shoot production. Second, the leaf life span of zero-year-old culms is an exception–such culms renew their leaves after 1 year (Kleinhenz and Midmore, 2001). Given that their leaves are new and productive, the carbohydrate assimilated by this age class is presumed to be high in every year, probably providing a large amount of carbohydrate for new shoot production in the following spring, and moderating the reduction in new shoot production in the following year (although the following year is potentially a poor year). Third, the large belowground carbon storage, probably supported by a high root:shoot ratio (Li et al., 1999; Mokany et al., 2006; Yuen et al., 2017), acts as a buffer to stabilize new shoot production. A similar phenomenon has been noted in the seed bank of plant species, in which the belowground seed bank can buffer plant population dynamics against environmental perturbations (MacDonald and Watkinson, 1981; Eager et al., 2014).
To understand new shoot productivity in Moso bamboo forest, we aimed to clarify the possible mechanisms that may suppress the 2-year cycle of new shoot production by constructing a new age-structured population growth model. We first established an age-structured population growth model that considered the specific leaf life span and belowground carbon storage in Moso bamboo forest. Using this model, we examined the impact of differential photosynthesis rates of new and old leaves on new shoot production at the population scale. Specifically, we examined the effects of photosynthesis rate of (1) new leaves of zero-year-old culms and (2) old leaves of even-aged culms on the periodicity of new shoot production, respectively. Subsequently, we examined, and (3) the impact of belowground carbon storage on the periodicity of new shoot production by changing the allocation of carbohydrate from the leaves.
We considered the age structure of the bamboo population (Figure 1). The density of bamboo culms at age i (i = 0, 1, 2,…, 6) in a stand (Ni) changes year by year. The density of new shoots depends on the leaf photosynthesis rate and carbohydrate storage of the whole stand in accordance with Moso bamboo carbon allocation (e.g., Isagi et al., 1997; Li et al., 1998b; Song et al., 2016). An individual culm of age i enters the next age class (i + 1) with the constant survival rate si before age 6 (si = 0.95 for i = 0, 1, 2, …, 5). These assumptions give the following equation,
where Ni(t) is evaluated at the beginning of year t. We assumed an individual that grows up to age 7 is harvested and removed from the stand, so its survival rate is assumed to be zero (s6 = 0).
Figure 1. Schematic representation of (A) a Moso bamboo forest showing clonal new shoot reproduction with a rhizome system and (B) the Moso bamboo age-structured model. In (A), black arrows represent flows of carbohydrates from surrounding adult culms to a new shoot via rhizomes. In (B), Ni represents the density of bamboo culms of age i. PH(t) and ST(t) represent net annual carbohydrate in leaves and belowground carbon storage at year (t), which is determined by proportions a and b and carbon loss fraction r. The orange and brown arrows represent flows of carbohydrates; the brighter and darker green horizontal arrows represent transitions to the next culm age classes with the survival rate si of 0.95 and 0, respectively. Leaf conditions in culm age classes are also shown using green color bars; length of the bars represent leaf life span, and brighter and darker green colors correspond to newer (first year) and older (second year) leaves, respectively.
PH(t), which represents the net annual production of carbohydrate from leaf photosynthesis evaluated at the end of year t, decreases by the total population density () with the density dependence strength d as a consequence of self-shading. This relationship is given by the following formula,
To simulate the 2-year leaf life span, we distinguished three types of leaves. New shoots of N0 have new leaves of 1-year life span; age one, three and five (N1, N3, and N5) have new leaves with a 2-year life span; and age two, four, and six (N2, N4, and N6) have old leaves with a 2-year life span (see Figure 1B). The net photosynthesis rate of each leaf type (i.e., gross carbon assimilation minus aboveground autotrophic respiration) is controlled by the parameter Pk (k = new_N0, new, or old), where Pnew_N0 is the net photosynthesis rate of new leaves of zero-year-old culms, Pnew is the net photosynthesis rate of new leaves, and Pold is the net photosynthesis rate of old leaves. At the beginning of the following year t + 1, a proportion a of PH(t) contributes to new shoot production, whereas the remaining proportion (1 - a) is transferred to belowground carbon storage (ST; Figure 1B).
The model also considered carbon storage loss during the growing season as a result of belowground autotrophic respiration, including fine and coarse roots, and rhizomes (Hsieh et al., 2016), with the fraction r. It follows that the carbon storage remaining at the end of year t is represented as (1−r)⋅ST(t). A proportion (1 - b) of the remaining carbon storage contributes to new shoot production at the beginning of year t + 1, whereas the remaining proportion b is stored. Therefore, the density of bamboo culms at age 0 at the beginning of year t + 1 is given by,
Similarly, the carbon storage ST(t + 1) evaluated at the beginning of year t + 1 is given by,
The iteration of this age-structured model (Eqs 1–4) simulates the interannual dynamics of bamboo density with belowground carbon storage. Details of the parameters included in this study are presented in Table 1.
This study focused on the dynamics of production of new shoots (N0) as an indicator of bamboo forest productivity. We focused on the final state of the model bamboo population (i.e., the equilibrium, periodic fluctuations, or chaotic fluctuations), but not on the transient states that are highly dependent on the initial conditions. For this purpose, the model was run from year 0 to 108 (t = 0–108) and recorded the population size in the final 500 years for further analyses, which represents the final state (i.e., attractor) of the population dynamics.
To clarify the impact of Moso bamboo-specific leaf photosynthesis on the number of new shoots, we examined the relationship between the parameter Pk (Pnew_N0, Pnew, or Pold) and the final state patterns of N0 using the coefficient of variation (CV). The CV, which is defined as the ratio of the standard deviation to the mean, is a standardized measure of temporal fluctuation. If the final state is at equilibrium (i.e., constant N0 values), the CV will be equal to 0. If the final state shows a 2-year cycle, the CV will be equal to , where N0_max and N0_min represent the number of new shoots in a good year and poor year, respectively). When the final state shows more than a 2-year cycle, the CV will be larger than zero and the exact value will depend on the dynamic of the N0 population. When calculating the CV, two of the parameters Pnew_N0, Pnew, and Pold were systematically changed from zero to 10 with a step of 0.05, whereas the other parameter was fixed. To confirm that the three types of final state did not appear simultaneously in narrow ranges of the parameters, we examined the asymptotic behavior of N0 (equilibrium, periodic fluctuations, or chaotic fluctuations) using a bifurcation diagram, which is a visual summary of the shifts as a function of the parameters (Murray, 2002). The range of the parameters Pnew_N0, Pnew, and Pold was extended to 50 in the bifurcation diagram (see Supplementary Figure 1).
To clarify the impact of carbon storage on new shoot production, we examined the relationship between the belowground carbon allocation parameters a, b, and r and the final state patterns (i.e., CV) using representative parameter sets of Pnew_N0, Pnew, and Pold. The parameters a, b, and r were changed from 0 to 1.0 with a step of 0.1. In this study, we conducted all numerical calculations using the C language.
The final states of the model were classified into three types: 2-year cycles, stable equilibrium, and others (i.e., more than 2-year cycles or chaotic fluctuations) (Figure 2). In the case of 2-year cycles (Figure 2B), the population size (the number of annual new shoots) alternated regularly between two values in the final state, resulting in CV = (note that N0_max and N0_min depend on the combination of the parameter Pk). In the stable equilibrium type (Figure 2A), population sizes were constant in the final state (CV = 0). In the other type (Figure 2C), population size alternated between more than two values, resulting in positive CV values. Although 2-year and other cycles apparently showed positive CV values, we confirmed that the two types appeared in different ranges of the parameter values using bifurcation diagrams (Supplementary Figure 1). The other type only appeared when Pnew_N0 was considerably larger than Pnew and Pold (Supplementary Figures 2, 3).
Figure 2. Example of the three types of final states in the Moso bamboo age-structured model. (A) Stable type with the parameter sets Pnew_N0 = 1.2, Pnew = 1.2, Pold = 0.4, (B) 2-year cycle type with the parameter set Pnew_N0 = 0.5, Pnew = 2.5, Pold = 0.2, and (C) more than 2-year cycle with the parameter set Pnew_N0 = 5.0, Pnew = 0.2, and Pold = 0.2. Note that Pnew_N0, Pnew, and Pold represent photosynthesis rates of zero-year-old culms’ leaves, new leaves, and old leaves, respectively.
The regions with CV = 0 (i.e., stable type) were broadened with increase in Pold, indicating that the contribution of carbohydrate from old leaves to new shoot production at the population scale weakened the 2-year cycle (Figures 3A–C). When Pold was small (0), the stable, 2-year cycle, and more than 2-year cycle types covered approximately 57, 36, and 7% of the total area, respectively, in the range 0 ≤ Pnew ≤ 10 and 0 ≤ Pnew_N0 ≤ 10 (Figure 3A). The 2-year cycle appeared when Pnew > Pnew_N0, whereas the more than 2-year cycle type appeared when Pnew_N0 > Pnew (Figure 3A). When Pold was relatively large (2.5), most parameter sets produced stable conditions, and the stable type covered approximately 97% of the total area in the range 0 ≤ Pnew ≤ 10 and 0 le Pnew_N0 ≤ 10 (Figure 3C).
Figure 3. Effect of net photosynthesis rate in old leaves on new shoot production. The figures show the final state coefficient of variation (CV) values from a series of Pold values of (A) 0.0, (B) 1.0, and (C) 2.5. The color gradation bar represents the CV. Parameter settings of the upper-left blue region generate the more than 2-year cycles or chaotic condition of production. Parameter settings of the central white region generate stable production. Parameter settings of the lower-right red region generate the 2-year cycles.
The stable type regions with CV = 0 were also broadened with Pnew_N0, indicating that the presence of leaves of zero-year-old culms weakened the 2-year cycle (Figures 4A–C). When Pnew_N0 was small (0), the stable, 2-year cycle, and more than 2-year cycle types covered approximately 85, 15, and 0% of the total area, respectively, in the range 0 ≤ Pnew ≤ 10 and 0 ≤ Pold ≤ 10 (Figure 4A). The 2-year cycle appeared when Pnew > Pold (Figure 4A). When Pnew_N0 was relatively large (10), most parameter sets produced stable conditions, and the stable type covered approximately 98% of the total area in the range 0 ≤ Pnew ≤ 10 and 0 ≤ Pold ≤ 10 (Figure 4C).
Figure 4. Effect of net photosynthesis rate in new leaves of zero-year-old culms on new shoot production. The figures show the final state coefficient of variation (CV) values from a series of Pnew_N0 values of (A) 0.0, (B) 5.0, and (C) 10.0. The color gradation bar represents the CV. Parameter settings of the lower-right red region generate the 2-year cycles of production. Parameter settings of the central white region generate stable production. Parameter settings of the lower-left blue region generate more than 2-year cycles or chaotic conditions.
With decrease in parameter a (which determines the proportion of carbohydrate from leaves allocated to new shoot production and hence belowground storage), the regions with CV = 0 (i.e., stable type) were distinctly broadened (Figures 5A,B,E), indicating that increase in belowground storage stabilized interannual variation in new shoot production. When a = 1.0, the stable type covered approximately 57% of the total area in the range 0 ≤ Pnew ≤ 10 and 0 ≤ Pnew_N0 ≤ 10 (Figure 5A). When a = 0.8, the stable type covered approximately 92% of the total area (Figure 5E). Accordingly, the percentage of the 2-year cycle region in the total area decreased from 36 to 0% (Figures 5A,E).
Figure 5. Effect of belowground carbon storage on new shoot production. The figures show the final state coefficient of variation (CV) values. The parameter settings of upper left (A) are identical to those of Figure 3A. The other figures show the final state CV values from six sets of parameters a and b. The parameter a ranged from 1.0 [upper panel; (A)], 0.9 [middle panels; (B–D)], to 0.8 [lower panels; (E–G)]. The parameter b ranged from 0.0 [left column; (A,B,E)], 0.3 [central columns; (C,F)], to 0.6 [right columns; (D,G)]. The color gradation bars represent the CV.
Increase in the parameters b and r (which determine the proportion of carbohydrate in belowground storage utilized to produce new shoots, and the carbon loss from roots and rhizomes, respectively) increased the probability of the 2-year cycle, although the final state conditions were less sensitive to parameters b and r compared with a (Figures 5, 6). When b = 0, the stable, 2-year cycle, and more than 2-year cycle types covered approximately 82, 11, and 7% of the total area, respectively, in the range 0 ≤ Pnew ≤ 10 and 0 ≤ Pnew_N0 ≤ 10 (Figure 5B). When b = 0.6, the stable, 2-year cycle, and more than 2-year cycle types covered approximately 74, 21, and 5% of the total area, respectively (Figure 5D). In addition, increase in r led to decrease in the stable type and to increase in the 2-year cycle type (Figure 6). When r increased from 0 to 0.6, the regions of the stable and 2-year cycle types changed from 81 to 68% and from 12 to 25%, respectively (Figures 6A,C).
Figure 6. Effect of loss of belowground carbon storage on new shoot production. The figures show the final state coefficient of variation (CV) value from a series of r values of (A) 0.0, (B) 0.2, and (C) 0.6. The color gradation bars represent the CV.
In this study, the increase in net photosynthesis rate of old leaves stabilized new shoot production (Figure 3). In addition, the increase in net photosynthesis rate of new leaves of zero-year-old culms led to the stable state or more than 2-year periodic cycles (Figure 4). In contrast, the increase in net photosynthesis rate in new leaves promoted the 2-year cycle of new shoot production (Figures 3, 4). Only when the photosynthesis rates of new leaves were much higher than those of old leaves and of new leaves of zero-year culms, was the 2-year cycle imposed. The simulation supported the hypothesis that the contribution of carbohydrate from old leaves might not be negligible at the population scale and that the carbohydrate from old leaves may readily cancel the 2-year cycle of new shoot production. The simulation also supported the conclusion that the new leaves of zero-year-old culms can eliminate the 2-year cycle, as the presence of the new leaves with high net photosynthesis rates on zero-year-old culms provided a large carbohydrate input for new shoot production in a poor year following a good year.
The present simulation raised a question mark regarding the general applicability of the traditional hypothesis proposed by Li et al. (1998a), that is, the biennial cycle of new shoot production results from interannual variation in the number of adult shoots carrying new leaves. Previous field measurements showed that photosynthesis activity is lower in old leaves than in new leaves; the photosynthesis rates of old leaves can be one-third of those of new leaves and the photosynthesis rates of new leaves of zero-year-old culms is almost identical to those of new leaves (Huang et al., 1989). Surprisingly, the parameter settings that satisfy the Huang et al. (1989)’s conditions (i.e., Pold = 1/3 Pnew or Pnew = Pnew_N0) did not generate the 2-year cycle of new shoot production in Moso bamboo forest (Figures 3–5). Therefore, we concluded that the 2-year leaf life span alone cannot be the causal factor of the 2-year cycle of new shoot production. It is possible that the biennial cycle of new shoot production in Moso bamboo forest is a transitional phenomenon induced by artificial disturbances, e.g., harvesting of old culms (Katanoda, 2007), or natural disturbances, e.g., drought in early spring (Xu et al., 2016; Tong et al., 2021) and foraging of shoots by animals (Kobayashi, 2020).
Our understanding of leaf photosynthesis rates in Moso bamboo forest remains limited compared with that of tree species (Kattge et al., 2011). Recently, field measurements showed that culm aging can impact on physiological traits, such as hydraulic conductivity and stomatal conductance (Tsuruta et al., 2016; Zhao et al., 2017; Gu et al., 2019; Wu et al., 2019). Thus, the amount of assimilated carbon in new and old leaves might change with culm aging. We strongly recommend measuring leaf photosynthesis rates in accordance with culm-age classes with consideration of seasonal variation or interannual monitoring of aging-related decline in leaf photosynthesis rates in certain individual culms. Such data should clarify (1) whether photosynthesis rates in old leaves can be less than one-third of those of new leaves regardless of culm age and (2) whether photosynthesis rates in new leaves of zero-year-old culms can be less than those of new leaves in other age-class culms.
The present study showed that the increases in carbon allocation from annual photosynthesis to belowground storage (smaller a) and the increase in contribution of belowground carbon to new shoot production (smaller b and r) canceled the 2-year cycles of new shoot production (Figures 5, 6). The simulation supported the hypothesis that enhanced belowground carbon storage would act as a buffer to stabilize interannual variation in new shoot production. The highly developed rhizome systems in Moso bamboo forest, including fine and coarse roots and rhizomes, can be a major carbon stock in Moso bamboo forest (Lin et al., 2017; Yuen et al., 2017). The belowground carbon stocks are almost identical to those aboveground in Moso bamboo forest (Lin et al., 2017; Yuen et al., 2017). This is in contrast to other forest types, such as temperate coniferous and broadleaved forests, in which belowground carbon stocks are approximately 60–70% smaller than those aboveground (Qi et al., 2019).
Elucidating belowground carbon cycling, including carbon stocks and flows, is important to understand the mechanism of the 2-year cycle of new shoot production. Moso bamboo has a unique growth pattern [new shoots complete their growth (in height and diameter) within 2 months after new shoot emergence in spring], suggesting that the rapid new shoot growth requires massive resources within a short period. A previous study indicated that the neighboring mature culms can transfer via the rhizomes almost all non-structural carbohydrates (NSCs) required to complete new shoot growth (Song et al., 2016; Wang et al., 2020). Culms with old leaves and new leaves of zero-year-old culms shed their leaves and produce new leaves during the new shoot emergence period (Li et al., 1998a; Zhou et al., 2019). It is possible that culms with new leaves transfer NSCs for new shoot growth, whereas culms with old leaves and zero-year-old culms mainly use NSCs to produce new leaves in the following year. In this case, NSCs originating from old leaves and new leaves of zero-year-old culms have little impact on new shoot production, and hence NSCs originating from new leaves mostly control new shoot production. However, our understanding of the carbon dynamics between new shoots and older culms during the new shoot emergence period is limited. The residence time of assimilated carbon belowground is also unknown. A stable carbon isotope pulse labeling technique (e.g., Dannoura et al., 2011; Epron et al., 2012) using a carbon balance approach (Li et al., 1998b; Song et al., 2016) would provide insight into the temporal changes in belowground carbon stocks and flows in Moso bamboo forest, and hence the possible mechanism of the periodicity of new shoot production.
The present simulation suggested that the 2-year cycle of new shoot production can be readily canceled by carbohydrate input from old leaves and new leaves of zero-year-old culms at the population scale, that may be a transitional phenomenon, enhanced by artificial and natural disturbances. Climate change might also enhance the 2-year cycle of new shoot production through changes in carbon allocation in Moso bamboo forest. The increase in temperature can cause exponential increase in respiration of plant organs (Reich et al., 2016; Crous et al., 2022), probably leading to increase in carbon loss and hence decrease in the belowground carbon stock (see Figures 5, 6). Indeed, high-temperature sensitivity of root respiration in a Moso bamboo forest has been suggested (Hsieh et al., 2016). The carbon loss induced by temperature increase might enhance the 2-year cycle of new shoot production, although the effects of temperature increase on carbon cycling are still uncertain (e.g., Dusenge et al., 2020; Zhang et al., 2021; Crous et al., 2022). In addition, a high frequency of drought during the shoot emergence period has been noted in some regions (e.g., Kao et al., 2013), which may also enhance the 2-year cycle. Supra-annual monitoring of new shoot production would be helpful to clarify the mechanism of new shoot production in Moso bamboo forest in the context of climate change. Furthermore, meta-analysis of interannual new shoot production cycles using data derived from a broad geographic area, covering the southern and northern limits of Moso bamboo distribution, would enable examination of the potential impacts of temperature increase on the periodicity of new shoot production, and determination of appropriate management practices for stable new shoot production in Moso bamboo forest under a changing environment. Such a meta-analysis will also act as the test of our model predictions regarding the presence/absence and frequently of the new shoot production cycles and their dependence on temperature related parameter values. If we find the gaps between the model predictions and the geographic patterns, it will motivate us to further develop a mathematical model for better understanding the long-term behavior of the Moso bamboo populations.
The original contributions presented in this study are included in the article/Supplementary Material, further inquiries can be directed to the corresponding author.
C-YL and TM designed the research protocol, developed the model, and performed numerical simulations. C-YL and TK surveyed the previous literature and drafted the manuscript. All authors approved the manuscript.
This study was supported by the MOST program (grant number: MOST 104-2621-B-002-005-MY3) and was partly supported by JSPS Grants-in-Aid for Scientific Research (grant number:19H02996).
The authors declare that the research was conducted in the absence of any commercial or financial relationships that could be construed as a potential conflict of interest.
All claims expressed in this article are solely those of the authors and do not necessarily represent those of their affiliated organizations, or those of the publisher, the editors and the reviewers. Any product that may be evaluated in this article, or claim that may be made by its manufacturer, is not guaranteed or endorsed by the publisher.
We thank W. L. Liang from National Taiwan University and Keito Kobayashi from the Forestry and Forest Products Research Institute in Japan for helpful advice. We would also like to thank Robert McKenzie, Ph.D., from Edanz (https://jp.edanz.com/ac) for editing a draft of this manuscript.
The Supplementary Material for this article can be found online at: https://www.frontiersin.org/articles/10.3389/ffgc.2022.913426/full#supplementary-material
Benton, A. (2015). “Priority species of bamboo,” in Bamboo: The Plant and its Uses, eds W. Liese and M. Köhl (Berlin: Springer International Publishing), 31–41.
Clark, L. G., Londoño, X., and Ruiz-Sanchez, E. (2015). “Bamboo taxonomy and habitat,” in Bamboo: The Plant and its Uses, eds W. Liese and M. Köhl (Berlin: Springer International Publishing), 1–30.
Crous, K. Y., Uddling, J., and De Kauwe, M. G. (2022). Temperature responses of photosynthesis and respiration in evergreen trees from boreal to tropical latitudes. New Phytol. 234, 353–374. doi: 10.1111/nph.17951
Dannoura, M., Maillard, P., Fresneau, C., Plain, C., Berveiller, D., Gerant, D., et al. (2011). In situ assessment of the velocity of carbon transfer by tracing 13C in trunk CO2 efflux after pulse labelling: variations among tree species and seasons. New Phytol. 190, 181–192. doi: 10.1111/j.1469-8137.2010.03599.x
Dusenge, M. E., Madhavji, S., and Way, D. A. (2020). Contrasting acclimation responses to elevated CO2 and warming between an evergreen and a deciduous boreal conifer. Glob. Change Biol. 26, 3639–3657. doi: 10.1111/gcb.15084
Eager, E. A., Rebarber, R., and Tenhumberg, B. (2014). Global asymptotic stability of plant-seed bank models. J. Math. Biol. 69, 1–37. doi: 10.1007/s00285-013-0689-z
Epron, D., Bahn, M., Derrien, D., Lattanzi, F. A., Pumpanen, J., Gessler, A., et al. (2012). Pulse-labelling trees to study carbon allocation dynamics: a review of methods, current knowledge and future prospects. Tree Physiol. 32, 776–798. doi: 10.1093/treephys/tps057
Gu, D. X., He, W., Huang, K. C., Otieno, D., Zhou, C. M., He, C. G., et al. (2019). Transpiration of Moso bamboo in southern China is influenced by ramet age, phenology, and drought. For. Ecol. Manag. 450:117526. doi: 10.1016/j.foreco.2019.117526
Hsieh, I. F., Kume, T., Lin, M. Y., Cheng, C. H., and Miki, T. (2016). Characteristics of soil CO2 efflux in an invasive species, Moso bamboo, in forests of central Taiwan. Trees 30, 1749–1759. doi: 10.1007/s00468-016-1405-6
Huang, Q. M., Yang, D. D., and Gao, A. X. (1989). A study on photosynthesis of bamboo. Sci. Silvae Sin. 25, 366–369.
Isagi, Y., Kawahara, T., Kamo, K., and Ito, H. (1997). Net production and carbon cycling in a bamboo Phyllostachys pubescens stand. Plant Ecol. 130, 41–52.
Kao, S. J., Kume, T., Komatsu, H., and Liang, W. L. (2013). Spatial and temporal variations in rainfall characteristics in mountainous and lowland areas in Taiwan. Hydrol. Proc. 27, 2651–2658. doi: 10.1002/hyp.9416
Katanoda, I. (2007). Calibration characteristics of reserve stool for bamboo-sprout cultivation. Kyushu J. For. Res. 60, 159–162. in Japanese,
Kattge, J., Díaz, S., Lavorel, S., Prentice, I. C., Leadley, P., Bönisch, G., et al. (2011). TRY – a global database of plant traits. Glob. Change Biol. 17, 2905–2935. doi: 10.1111/gcb.14869
Kobayashi, K. (2020). Growth failure and wild animal activity in a moso bamboo Phyllostachys edulis stand in Kyoto, western Japan. Landsc. Ecol. 25, 153–157. doi: 10.5738/jale.25.153
Li, L., Cheng, Z. C., Ma, Y. J., Bai, Q. S., Li, X. Y., Cao, Z. H., et al. (2018). The association of hormone signalling genes, transcription and changes in shoot anatomy during moso bamboo growth. Plant Biotechnol. J. 16, 72–85. doi: 10.1111/pbi.12750
Li, R., Werger, M. J. A., During, H. J., and Zhong, Z. C. (1998a). Biennial variation in production of new shoots in groves of the giant bamboo Phyllostachys pubescens in Sichuan, China. Plant Ecol. 135, 103–112.
Li, R., Werger, M. J. A., During, H. J., and Zhong, Z. C. (1998b). Carbon and nutrient dynamics in relation to growth rhythm in the giant bamboo Phyllostachys pubescens. Plant Soil 201, 113–123.
Li, R., Werger, M. J. A., During, H. J., and Zhong, Z. C. (1999). Biomass distribution in a grove of the giant bamboo Phyllostachys pubescens in Chongqing, China. Flora 194, 89–96.
Lin, M. Y., Hsieh, I. F., Lin, P. H., Laplace, S., Ohashi, M., Chen, T. H., et al. (2017). Moso bamboo (Phyllostachys pubescens) forests as a significant carbon sink? A case study based on 4-year measurements in central Taiwan. Ecol. Res. 32, 845–857. doi: 10.1007/s11284-017-1497-5
MacDonald, N., and Watkinson, A. R. (1981). Models of an annual plant population with a seedbank. J. Theoret. Biol. 93, 643–653.
Mokany, K., Raison, R. J., and Prokushkin, A. S. (2006). Critical analysis of root : shoot ratios in terrestrial biomes. Glob. Change Biol. 12, 84–96. doi: 10.1111/j.1365-2486.2005.001043.x
Monselise, S. P., and Goldschmidt, E. (1982). “Alternate bearing in fruit trees,” in Horticultural Reviews, Vol. 4, ed. J. Janick (Hoboken: NJ: Wiley), 128–173.
Qi, Y. L., Wei, W., Chen, C. G., and Chen, L. D. (2019). Plant root-shoot biomass allocation over diverse biomes: a global synthesis. Glob. Ecol. Conserv. 18:e00606. doi: 10.1016/j.gecco.2019.e00606
Reich, P. B., Sendall, K. M., Stefanski, A., Wei, X. R., Rich, R. L., and Montgomery, R. A. (2016). Boreal and temperate trees show strong acclimation of respiration to warming. Nature 531, 633–636. doi: 10.1038/nature17142
Silvertown, J., and Charlesworth, D. (2009). Introduction to Plant Population Biology. Hoboken, NJ: Wiley.
Shinohara, Y., Kume, T., Ichihashi, R., Komatsu, H., and Otsuki, K. (2014). Moso-bamboo forests in Japan: What are the effects of their area expansion on ecosystem services? J. Jpn. For. Soc. 96, 351–361. in Japanese, doi: 10.4005/jjfs.96.351
Song, X., Peng, C., Zhou, G., Gu, H., Li, Q., and Zhang, C. (2016). Dynamic allocation and transfer of non-structural carbohydrates, a possible mechanism for the explosive growth of Moso bamboo (Phyllostachys heterocycla). Sci. Rep. 6:25908. doi: 10.1038/srep25908
Tong, C. Z., Zhang, X., Xie, J. B., Mei, T. T., Fang, D. M., and Li, Y. (2021). Water use strategies of different aged moso bamboo culms under summer drought. For. Ecol. Manag. 498:119567. doi: 10.1016/j.foreco.2021.119567
Tsuruta, K., Okumura, M., Kume, T., Ichihashi, R., Shinohara, Y., and Kosugi, Y. (2016). Insignificant effects of culm age on transpiration in a managed Moso bamboo forest, Kyoto, Japan. Hydrol. Res. Lett. 10, 1–7. doi: 10.3178/hrl.10.1
Wang, S., Chen, T. H., Liu, E. U., and Liu, C. P. (2020). Accessing the nursing behaviour of Moso bamboo (Phyllostachys edilus) on carbohydrates dynamics and photosystems. Sci. Rep. 10:1015. doi: 10.1038/s41598-020-57643-1
Wu, X. P., Liu, S. R., Luan, J. W., Wang, Y., and Cai, C. J. (2019). Responses of water use in Moso bamboo (Phyllostachys heterocycla) culms of different developmental stages to manipulative drought. For. Ecosyst. 6:31. doi: 10.1186/s40663-019-0189-8
Xu, L., Shi, Y., Zhou, G., Xu, X., Liu, E., Zhou, Y., et al. (2018). Structural development and carbon dynamics of moso bamboo forests in Zhejiang province, China. For. Ecol. Manag. 409, 479–488. doi: 10.1016/j.foreco.2017.11.057
Xu, X. J., Du, H. Q., Zhou, G. M., Li, P. H., Shi, Y. J., and Zhou, Y. F. (2016). Eddy covariance analysis of the implications of drought on the carbon fluxes of Moso bamboo forest in southeastern China. Trees 30, 1807–1820. doi: 10.1007/s00468-016-1414-5
Xu, Y., Wong, M. H., Yang, J. L., Ye, Z. Q., Jiang, P. K., and Zheng, S. J. (2011). Dynamics of carbon accumulation during the fast growth period of bamboo plant. Bot. Rev. 77, 287–295. doi: 10.1007/s12229-011-9070-3
Yuen, J. Q., Fung, T., and Ziegler, A. D. (2017). Carbon stocks in bamboo ecosystems worldwide: estimates and uncertainties. For. Ecol. Manag. 393, 113–138. doi: 10.1016/j.foreco.2017.01.017
Zhang, S. B., Li, Y. F., Singh, B. P., Wang, H. L., Cai, X. Q., Chen, J. H., et al. (2021). Contrasting short-term responses of soil heterotrophic and autotrophic respiration to biochar-based and chemical fertilizers in a subtropical Moso bamboo plantation. Appl. Soil Ecol. 157:103758. doi: 10.1016/j.apsoil.2020.103758
Zhao, X. H., Zhao, P., Zhang, Z. Z., Zhu, L. W., Niu, J. F., Ni, G. Y., et al. (2017). Sap flow-based transpiration in Phyllostachys pubescens: applicability of the TDP methodology, age effect and rhizome role. Trees 31, 765–779. doi: 10.1007/s00468-016-1407-4
Keywords: Moso bamboo, production, two-year cycles, age-structured population growth model, leaf phenology, storage
Citation: Lin C-Y, Miki T and Kume T (2022) Potential Factors Canceling Interannual Cycles of Shoot Production in a Moso Bamboo (Phyllostachys pubescens) Stand. Front. For. Glob. Change 5:913426. doi: 10.3389/ffgc.2022.913426
Received: 05 April 2022; Accepted: 09 June 2022;
Published: 07 July 2022.
Edited by:
Ling Zhang, Jiangxi Agricultural University, ChinaReviewed by:
Qiang Wei, Nanjing Forestry University, ChinaCopyright © 2022 Lin, Miki and Kume. This is an open-access article distributed under the terms of the Creative Commons Attribution License (CC BY). The use, distribution or reproduction in other forums is permitted, provided the original author(s) and the copyright owner(s) are credited and that the original publication in this journal is cited, in accordance with accepted academic practice. No use, distribution or reproduction is permitted which does not comply with these terms.
*Correspondence: Tomonori Kume, a3VtZXR0QGdtYWlsLmNvbQ==
Disclaimer: All claims expressed in this article are solely those of the authors and do not necessarily represent those of their affiliated organizations, or those of the publisher, the editors and the reviewers. Any product that may be evaluated in this article or claim that may be made by its manufacturer is not guaranteed or endorsed by the publisher.
Research integrity at Frontiers
Learn more about the work of our research integrity team to safeguard the quality of each article we publish.