- 1Applied Ecology and Conservation Lab, Programa de Pós-graduação em Ecologia e Conservação da Biodiversidade, Universidade Estadual de Santa Cruz, Ilhéus, Brazil
- 2Instituto Juruá, Manaus, Brazil
- 3Programa de Pós-graduação em Ecologia, Instituto Nacional de Pesquisas da Amazônia, Manaus, Brazil
Forest loss is one of the most serious threats to biodiversity in the tropics and mainly occurs due to the conversion of native forests by the expansion of human activities. In addition, regional climate change is likely to adversely affect the remaining biota. These disturbances may have direct or indirect consequences on the demographic structure of plant species in human-modified landscapes. To test this hypothesis, and thus look for management practices aimed at enhancing the population viability, we used the palm species Euterpe edulis Mart. (Arecaceae) to assess the demographic structure of five ontogenetic stages prior (2014) and after (2017) a prolonged drought event (2015) that occurred in the northeastern Atlantic Forest of Brazil. We also investigated the influence of landscape forest cover on the ontogenetic demographic structure, given that forest remnants were embedded within landscapes ranging from 6 to 97% of forest amount. We revealed that forest cover was a key predictor explaining the abundance patterns of E. edulis, with all ontogenetic stages (except seedlings, immature, and adults) exhibiting lower abundance in forest remnants surrounded by lower amount of native forests. Conversely, the regional drought event unaffected the demographic structure of this palm species, which may suggest that populations of E. edulis were able to cope with an isolated, though severe, drought event. The impacts of forest loss on E. edulis demographic structure, particularly on juveniles, raises a concern about the future persistence of E. edulis populations, since the early stages represent the adult generation in the near future. Management measures, including species reintroduction, forest restoration, environmental education programmes and the enforcement of environmental laws must be encouraged to safeguard E. edulis populations in the Atlantic Forest.
Introduction
Habitat loss is one of the main threats to global biodiversity (Foley et al., 2005) and occurs mainly due to the conversion of continuous native forests by anthropogenic activities (Tabarelli et al., 2010). These anthropogenic impacts usually create fragments of varying sizes and degrees of isolation embedded within landscapes exhibiting different proportions of native forest (Fahrig, 2003). Tropical forests, while harboring more than three-quarters of the world’s biodiversity, have suffered the greatest rates of deforestation, with pervasive consequences for biodiversity persistence (Barlow et al., 2018). In fact, several studies have shown the negative effects of habitat loss on different taxonomic groups in tropical forests, such as birds (Morante-Filho et al., 2015; Melo et al., 2018), mammals (Bovendorp et al., 2019; Alves et al., 2020), and invertebrates (Souza et al., 2020). In addition, habitat loss severely decreases plant diversity, affecting both juvenile and adult tree assemblages (Rigueira et al., 2013; Benchimol et al., 2017), with drastic consequences for the their demographic structure (Leal et al., 2021).
Among the pervasive effects of forest loss is the widespread shifting of regional patterns of rainfall distribution and temperatures, thus potentially affecting species persistence within forest remnants (Lawrence and Vandecar, 2015; Spera et al., 2020). For instance, climatic factors are essential for the successful growth and development of plants. Abrupt climate events might lead to a decrease in plant density or local extinction of certain species (Allen et al., 2010). Moreover, intense or prolonged periods of drought can increase mortality, decrease plant growth and also modulate the distribution of sensitive species (Engelbrecht et al., 2007; Phillips et al., 2009), ultimately affecting forest recovery (Liebsch et al., 2008). Yet some plant species are able to acclimatize after abrupt climate event such a severe summer drought (Luo et al., 2016). Also, species response might be different according to the ontogenetic stage (classification of individuals based on the ontogeny or plant development stage) and the intensity and type of disturbance (Demirevska et al., 2009).
For several plant species, the transition from one ontogenetic stage to another is often highly sensitive to environmental variations (Jordano et al., 2004; Valiente-Banuet et al., 2015), resulting in demographic bottlenecks. Studies on the demographic structure may be key for assessing the species response after a disturbance event, or even anticipate the potential responses of species to the type of land use, its resources and management, although census data either in undisturbed areas or preferably prior to the disturbance would be required for comparisons (Hughes and Westoby, 1992; Chazdon, 2003). Additionally, demographic studies are also important to check which ontogenetic life stage(s) affect the population growth rate. Though, Condit et al. (1998) point out the complexity of using population structure as an indicator of future population trends. Indeed, population structure is only acceptable as a diagnostic tool for population health and viability when replicated in time and space. Therefore, long-term demographic observations are necessary to propose effective conservation practices and management actions (Morris et al., 2008). For instance, by assessing long-term population dynamics of the endangered Euterpe edulis plants in a Brazilian Atlantic Forest landscape, Portela and Dirzo (2020) revealed a substantial alteration in the palm stage distribution, with a decline in reproductive stages and a dominance of infants, mediated by severe defaunation. As management recommendations, the authors posit the importance of considering refaunation, especially of predators to overcome the current situation. However, few studies have monitored plant populations in tropical forest remnants, although this needs to be encouraged to enhance our understanding on the effects of disturbances on populations.
Among a wide diversity of tree families in the tropics, palms are among the main components of forest functioning (Terborgh, 1986) given the great production of fleshy fruits and seeds essential for frugivorous animals (Zona and Henderson, 1989; Galetti et al., 2013). However, the intense and rapid pace of deforestation observed in tropical forests is disrupting palm assemblages, with potential long-term consequences for the entire system. In the Brazilian Atlantic forest, for instance, palms exhibit contrasting patterns of responses to landscape-scale forest loss–both richness and abundance of “forest-interior” species were severely reduced in forest remnants surrounded by less than 40% of forest cover in the landscape, whereas “open-area” species proliferated in these areas (Benchimol et al., 2017). Landscape-scale deforestation might change the local conditions of forest remnants, such as increasing light availability, precipitation, and soil fertility. These local changes might negatively impact shade-tolerant species and shape the relative abundance of palm species in neotropical forests (Fauset et al., 2017; Muscarella et al., 2020). This is the case of the neotropical palm, E. edulis Mart. (Areacaceae), which grows preferentially under the closed and shady forest canopy, and tend to be absent in highly deforested (<25% forest cover) areas and large clearings (Gatti et al., 2011; Cerqueira et al., 2021; Leal et al., 2021). We argue that this species deserves special attention, given its underlying ecological role as an important food resource (especially in lean times) for several frugivorous vertebrate species, while representing a valuable economic asset for human consumption (Henderson et al., 1995). However, because harvesting of the heart from this single-stemmed palm leads to plant death, E. edulis has been overexploited, being locally extirpated from several forest remnants in the Atlantic forest (Galetti and Fernandez, 1998). Due to the combination of all these factors, E. edulis is classified as a vulnerable species, and thus, a conservation priority (Martinelli and Moraes, 2013).
Considering the high sensitivity of tree palms to climate conditions (Muscarella et al., 2020), coupled with the increasing drought events in tropical regions due to human-induced climate change, it is imperative to improve our understanding on how population structure is affected by extreme climatic events. In fact, plant responses to extreme weather events are still not completely understood. This mainly occurs because species responses are influenced by the intensity and frequency of events, and alternating periods of drought and rain in a given time scale can affect tree development (Lloret et al., 2012). Thus, we expect that abundance and occurrence patterns of the different ontogenetic stages of E. edulis will be affected by forest loss and local climatic conditions. Here, we used this endangered palm species to assess the demographic structure prior (2014) and after (2017) a prolonged drought event (2015) that occurred in the northeastern Atlantic Forest of Brazil. We also investigated the influence of landscape-scale forest cover on the ontogenetic demographic structure, given that forest remnants were embedded within landscapes ranging from 6 to 97% of forest amount. In particular, we hypothesized that early life stages (i.e., seedling and juveniles) will experience the greatest abundance decrease after the drought event, especially in more deforested landscapes, given their higher sensitivity to environmental changes (Larcher, 2000), such as deforestation and abiotic changes (Gatti et al., 2014; Leal et al., 2021).
Materials and methods
Study area
We conducted this study in ten forest remnants of the Atlantic Forest from southern Bahia state, Brazil (15°0′–16°0′ S and 39°0′–39°30′ W). Southern Bahia is considered a priority region for biodiversity conservation across the Atlantic forest hotspot, given its great number of forest species and endemisms (Martini et al., 2007). Deforestation has been occurring since the late 19th century, resulting in drastic changes in landscape composition. Currently, forest remnants are embedded within different agroecosystems, mostly composed of cattle pastures, rubber (Hevea brasiliensis), eucalyptus (Eucalyptus spp.), and cocoa agroforests (Theobroma cacao) (Benchimol et al., 2017). The vegetation is characterized as Tropical Wet Forest (Thomas, 2003), the climate type is Af following Köpen classification, being hot and humid, with annual average temperature and precipitation of 25°C and 1200 mm, respectively (Mori et al., 1983).
Forest fragments were selected using high-resolution satellite images from 2009 to 2011 RapidEye® (European space agency), QuickBird® (DigitalGlobe Inc. of Longmont, CO, USA), and WorldView® (Maxar Technologies Holdings Inc., in San Francisco, CA, USA), based on the percentage of native forest cover at the landscape-scale (Morante-Filho et al., 2015). In 2014, 10 forest fragments distributed in a contrasting gradient of landscape-scale forest cover were selected to perform demographic surveys of E. edulis (Oliveira, 2014)–six located in the municipalities of Mascote and Belmonte (“Southern” region, ranging from 6 to 59% of forest amount) and four located in the region of Una (“Northern” region, ranging from 46 to 97% of forest amount) (Figure 1). The two regions are 60 km apart, and present the same forest type, and exhibit similar soil composition, topography, and flora species (Thomas et al., 1998).
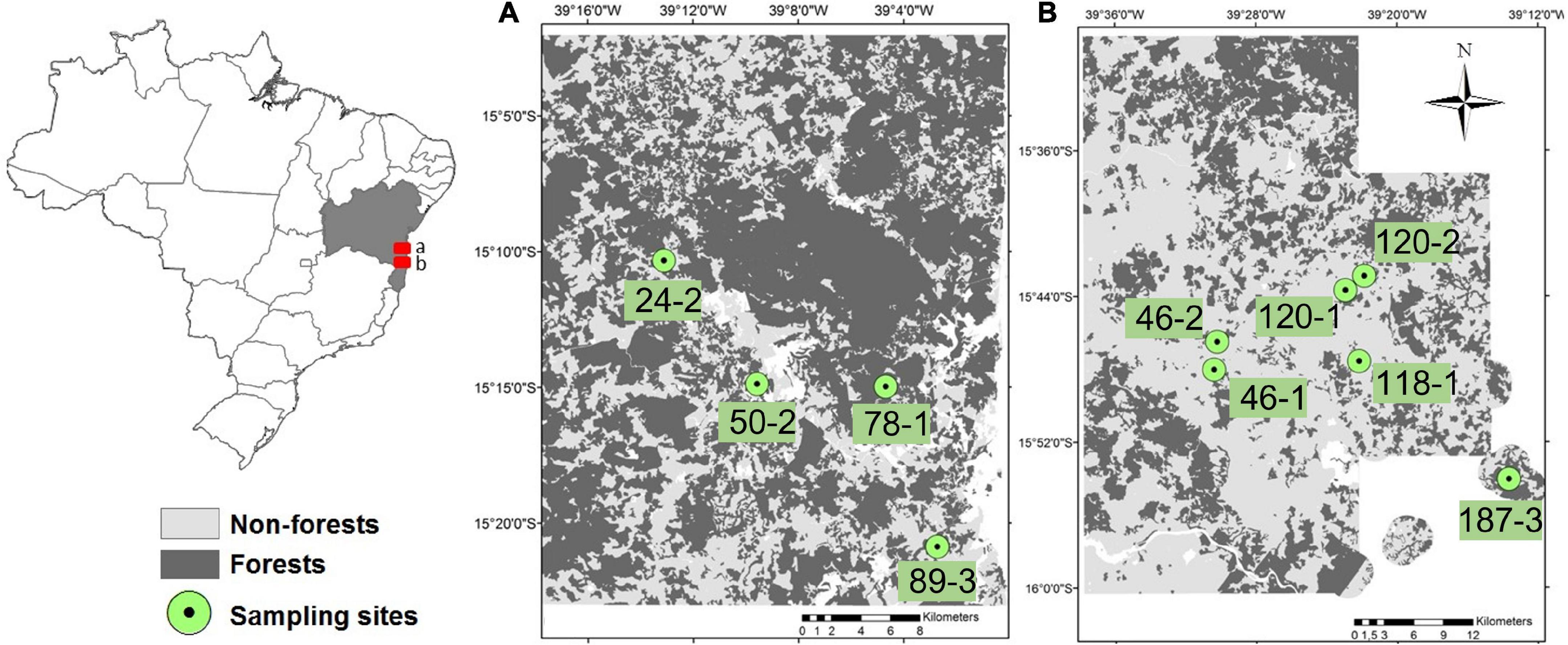
Figure 1. Distribution of the 10 sampled forest fragments located in the Atlantic Forest of southern Bahia, including (A) Una region (Northern) and (B) Belmonte region (Southern).
Until the 19th century, landscape changes occurred in low intensity in both regions. From 1890 on, forests were increasingly lost in both regions. In the Southern region, eucalyptus plantations and cattle pastures became the predominant agroecosystems, whereas rubber and cocoa agroforests comprised the main land use types in the Northern region (Benchimol et al., 2017). However, large tracts of continuous forests were preserved in the North region, by the establishment of the largest protected area in the southern Bahia (Una Biological Reserve, with ∼18.000 hectares).
Demographic structure of Euterpe edulis Mart.
In 2014, one 0.5 ha forest plot (100 × 50 m) was established in the center of each selected forest fragment (Oliveira, 2014), and all individuals of E. edulis were recorded at different ontogenetic stages, based on Silva et al. (2009)–(i) seedlings–one or two palmate leaves and an endosperm reserve; (ii) juvenile (divided into two size classes): juvenile I–pinnate leaves, although palmate leaves might still be present, height ≤0.15 m; juvenile II–pinnate leaves only, height ≤1 m; (iii) immature–stem height >1 m with no sign of reproductive events; (iv) adults–stems with past or present signs of reproductive events. Three years later (i.e., 2017), we re-visited the same forest plots and performed another demography sampling following the same procedures described above.
Drought impact
Beginning in late 2014 and extending into early 2016, a severe and prolonged drought affected the southern region of Bahia, due to an El-Niño Southern Oscilation (ENSO) event (for more details see Getirana, 2016; Gateau-rey et al., 2018). Thus, to characterize this event in our study regions, we extracted annual rainfall data from the 5 years that potentially influenced our data collection (Supplementary Figure 1). These data were extracted from the stations closest to our study regions by INMTE (Instituto Nacional De Meteorologia, 2022). Additionally, to evaluate the potential impact of the drought period on the demographic structure of E. edulis, we used precipitation measurements from two different periods: 2013, which corresponds to the previous year of the first survey, and 2015, which was the drought year (see Supplementary Figure 2). We extracted precipitation measurements from the Tropical Rainfall Measure Mission (TRMM) of NASA (US National Aeronautics and Space Administration) with 0.25°× 0.25° resolution. Precipitation of the driest month of each year were downloaded from the satellite TRMM 3B43 version 7 (TRMM 2011), and the number of dry days was calculated using daily measures of the TRMM 3B42 version 7 (GES DISC 2016).
Forest cover amount
Using the ARCGis 10.2 program (Environmental Systems Research Institute [ESRI], 2014), we obtained the amount of native forest surrounding each fragment by calculating the percentage of forest cover at different buffer size radii–200, 400, 600, 800, and 1000 m. Multi-scale analysis is widely recommended for assessing the scale of effect when previous knowledge is lacking on the spatial scale that the target biological group responds (Thompson and McGarigal, 2002). The scales were selected based on the dispersal capacity of toucans (Ramphastos vitellinus), the largest frugivorous bird species that consume E. edulis fruits in our study region (Holbrook, 2011). We avoided scales larger than 1000 m due to overlapping and potentially non-independence among landscapes. We thus used the values of R2, which measures the proportion of variation in Y (ontogenetic stage) explained by X (amount of forest cover in each spatial scale), selecting the model that obtains the highest value of R2 (Supplementary Table 1). The 800 m radius had the highest R2 value for 3 of the 5 ontogenetic stages; therefore, this was the radius size used in all subsequent analysis. Therefore, forest cover measurements at this scale ranged from 6 to 97% (Figure 1).
Data analysis
All analyses were conducted in R version 3.5.1 (R Core Team, 2018). We first controlled for high levels of inter-dependence for all of our continuous predictors: number of dry days, precipitation of the driest month, and forest cover; by performing a Pearson’s correlation matrix, retaining non-correlated variables (r < | 0.70|). The number of dry days and precipitation during the driest month were highly correlated (r = −0.97), therefore the driest month was used in our analysis. We thus performed Generalized Linear Mixed Models (GLMMs) using a Negative Binomial distribution for overdispersed count data (Hardin and Hilbe, 2007) to assess the influence of forest cover and the precipitation of the driest month on E. edulis abundance using their z-standardized values, and ontogenetic stage in interaction with all predictors. To deal with the repeated measures nature of the data we settled the year as a random part of the model.
All candidate models were built using Maximum Likelihood (ML) procedures with lme4 package (Bates et al., 2015). We used the dredge function of MuMIn (Bartón, 2018) to examine every possible first-order variable combination and selected the models based on the Akaike Information Criterion (AICc) corrected for small samples (Burnham and Anderson, 2004). We considered as parsimonious the model that presented the lowest AICc value. Conditional and marginal R2 was calculated following (Nakagawa et al., 2017).
Results
In 2014, a total of 2.183 individuals of E. edulis were recorded, representing all five ontogenetic stages across all sampled fragments. In 2017, we recorded a total of 2.359 individuals, which represents an increase of 8.06% compared to 2014 (Supplementary Table 2). Considering the different ontogenetic stages, in 2014 the largest percentage of individuals was juvenile II (N = 1.125; 51.53%), followed by juvenile I (N = 449; 20.57%), immature (N = 279; 12.78%), seedlings (N = 196; 8.98%), and adults (N = 134; 6.14%). We also recorded a similar pattern in 2017, in which the largest percentage of individuals was also juvenile II (N = 1.285; 54.57%), followed by juvenile I (N = 484; 20.52%), immature (N = 339; 14.37%), seedlings (N = 140; 5.93%), and adults (N = 111;4.71%) (Figure 2).
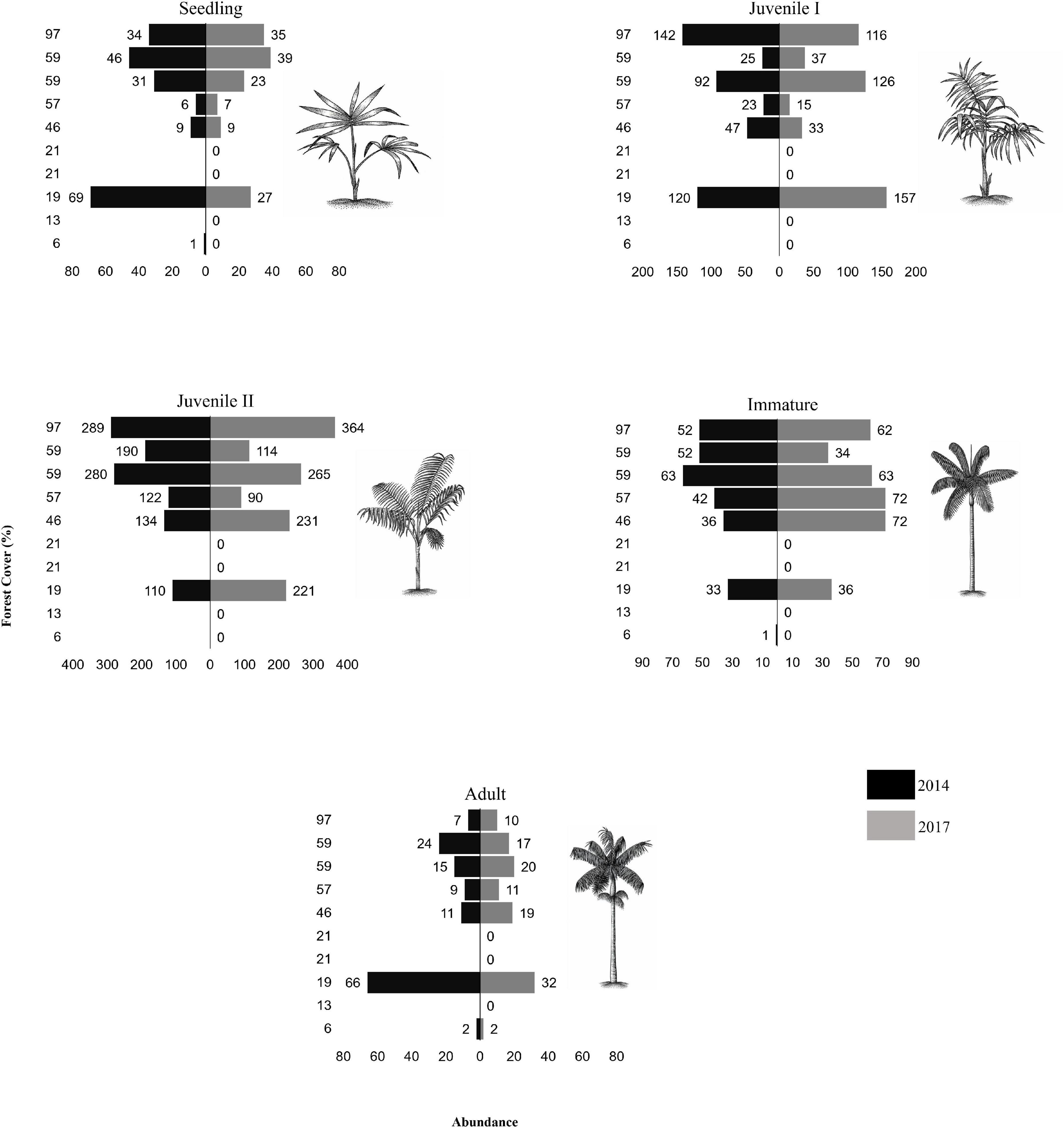
Figure 2. Abundance of Euterpe edulis in the different ontogenetic stages in the years 2014 and 2017 in a forest cover gradient in ten forest fragments sampled in the Atlantic Forest of Southern Bahia.
Our results showed that forest cover at the landscape scale, not precipitation of the driest month, was the most important predictor of E. edulis abundance (Figure 3), retaining a single best model with 70% of ɷAICc (Table 1), marginal R2 = 0.40, conditional R2 = 0,40. The ontogenetic stages also reflected this effect, being juvenile II the most sensitive to forest cover loss followed by juvenile I (Figure 3 and Table 2). We did not detect any effect of year explaining differences in E. edulis abundance (Table 2).
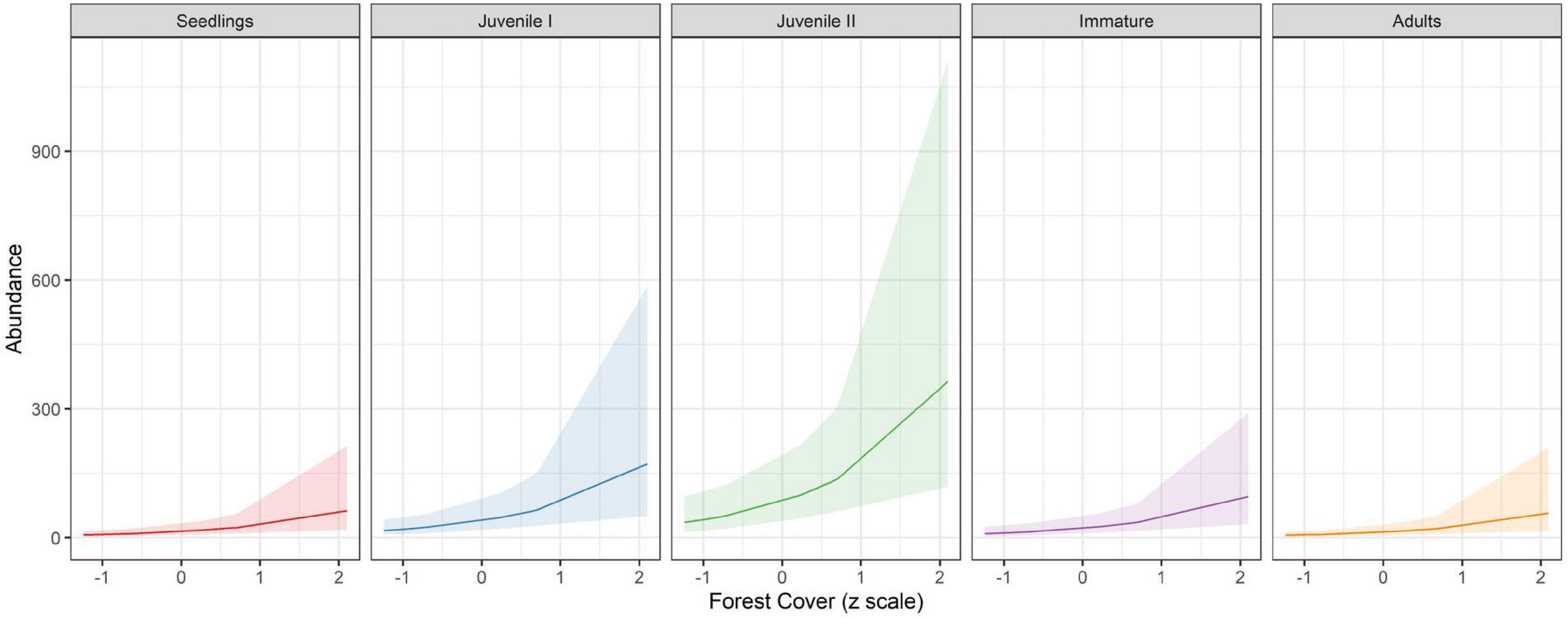
Figure 3. Relationship between forest cover (z scale) and E. edulis ontogenetic stages. The 95% confidence intervals around linear fit are shown in gray.
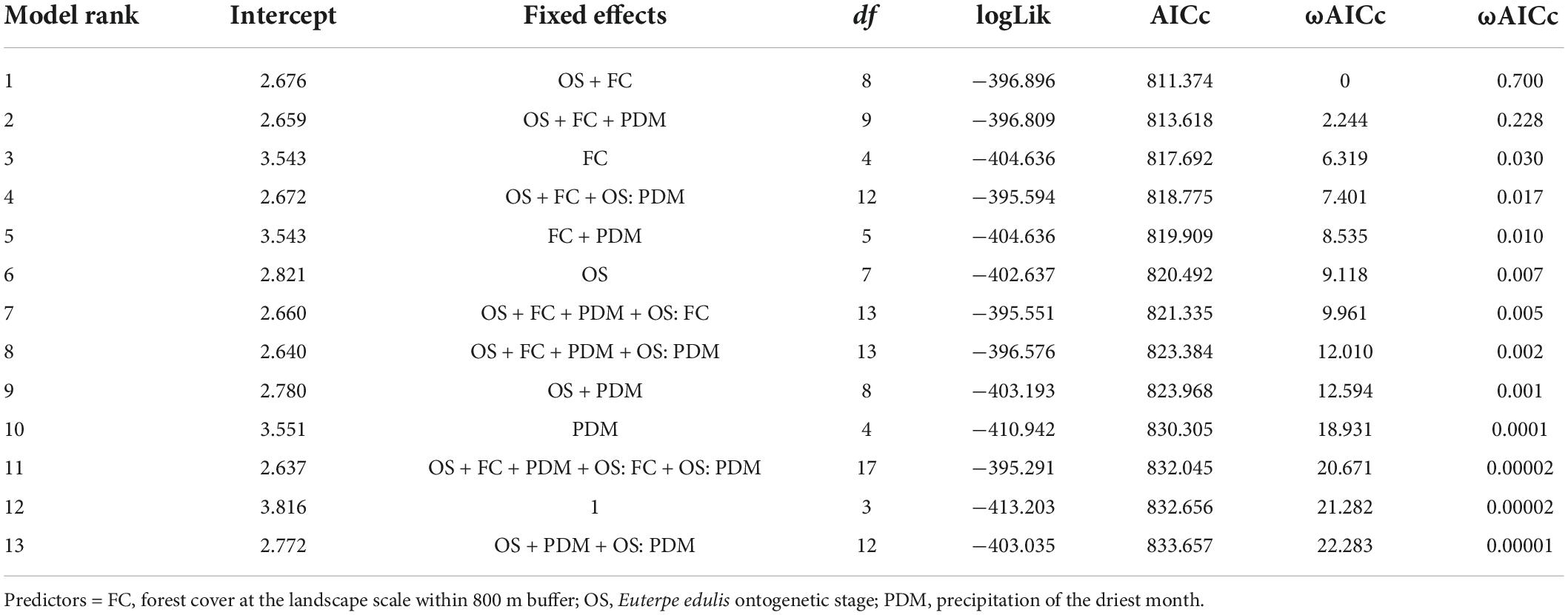
Table 1. Top-ranked candidate models, and their respective Akaike’s information criterion with small sample size correction (AICc), the difference between a given model and the best model (ΔAICc), and the Akaike weights (ωAICc).
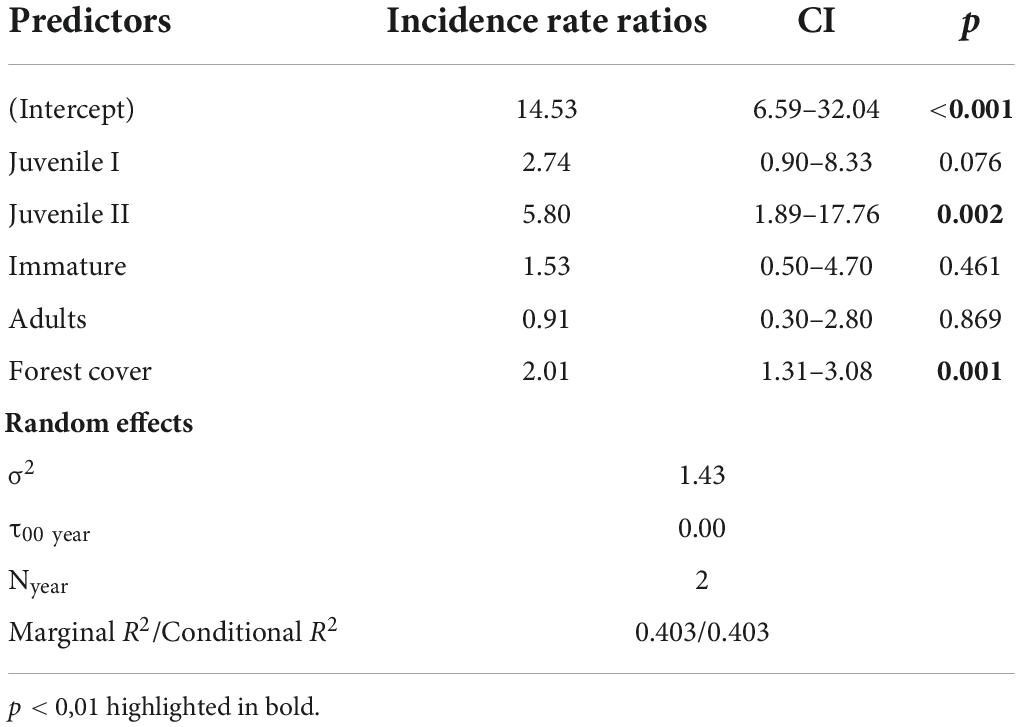
Table 2. Top-ranked Generalized Linear Mixed Model coefficients explaining the relationship between Euterpe edulis abundance, its ontogenetic stage and forest cover at landscape scale within 800 m radius buffer.
Discussion
Our results demonstrate the effects of landscape-scale forest loss on the demographic structure of E. edulis, a endangered species endemic to the Atlantic Forest. Forest loss affected juveniles I and II, strongly decreasing the number of individuals of both ontogenetic stages in highly deforested landscapes. Surprisingly, the regional drought event did not affect the abundance of E. edulis individuals, regardless of the ontogenetic stage, considering all sampling fragments. These results suggest that this threatened species is greatly affected by landscape deforestation but is presumably able to cope with oscillations in precipitation in terms of demography. Therefore, increasing forest cover is a top conservation priority to mitigate population depletion of E. edulis in fragmented forest landscapes in the threatened Brazilian Atlantic Forest.
Contrary to our prediction, our results showed that E. edulis can mostly tolerate a regional drought event in southern Bahia, by maintaining population density over 3 years. Yet a single fragment, exhibiting 19% of landscape forest cover, had experienced a greatly decay of adults after the drought, which can be associated to its specific local conditions. Indeed, this small fragment has a stream that turns the entire soil greatly wet along the year, which also potentially explains the highest local density of adults and comparable densities of seedlings and juveniles to those sites immersed in high-forested landscapes cover. Therefore, the severe drought might have pronounced affected the persistence of adults in this specific location, regardless other studied forest fragments. It is known that factors such as climate and hydrology are important predictors of palm species distribution (Eiserhardt et al., 2011). Physiological and morphological attributes of Arecaceae species, such as low drought-tolerance, have been suggested as one of the reasons explaining the limits of family distribution to the tropical and subtropical regions (Henderson et al., 1995). Particularly for E. edulis, a substantial decrease in seed water content across different populations was observed during an extremely dry year (Portela et al., 2020). This would be important considering the increasing severity of ENSO events projected due to climate change scenarios (Cai et al., 2014). These events in turn lead to several environmental changes such as the 2015 drought in North-eastern Brazil (Getirana, 2016; Gateau-rey et al., 2018), with potential detrimental effects on plant communities (Engelbrecht et al., 2007; Allen et al., 2010). However, Foden et al. (2019) state that different populations may respond to climate change differently along their general distribution. In fact, E. edulis showed high seed germination in contrasting conditions of light and water availability, being considered a species with a wide seed germination niche (Braz et al., 2014). In view of this, we emphasize the importance of further studies evaluating the influence of climate change, especially drought, not only on the abundance patterns of this species, but also the underlying processes structuring these patterns.
Landscape-scale forest loss was the determining factor explaining E. edulis abundance, according to previous findings that revealed the importance of forest cover in explaining the demographic patterns of this species considering all ontogenetic stages (Leal et al., 2021). In thus study, we unveil that juveniles were greatly affected by landscape deforestation. Generally, the number of seedlings in both years and in both regions was exceptionally low, exhibiting similar values to other studied areas impacted by harvesting (Portela, 2008) or under intense seed predation (Fadini et al., 2009). Additionally, studies suggest the presence of a “Bahia” ecotype that presented low fruit production (Bovi et al., 1987; Coelho, 2010). Previous research in the same fragments showed that 82% of all E. edulis seeds were predated by vertebrates and invertebrates, while only 2.8% of the dispersed seeds successfully recruited (Soares et al., 2015). Indeed, the local assemblage of seed predators is an important factor in the recruitment of new individuals. We suggest that the low abundance of recruiting seeds is the result of a combined effect of the intrinsic low fertility of regional ecotype, the high levels of seed predation, and overall changes in local conditions triggered by deforestation. Moreover, landscape deforestation causes not only substitution of specialist bird species by generalists and the overall loss of frugivorous bird species (Morante-Filho et al., 2015), but also reduces the number of interactions between frugivorous birds of the understory and species of fruit plants (Menezes Pinto et al., 2021). Considering that frugivorous birds consume a greater amount of fruit compared to species that belong to other food guilds, which consume fruit only sporadically (Garcia and Martinez, 2012; Sebastián-González, 2017), the loss of frugivorous birds and the reduction of interactions with plants may have consequences for the dispersal of E. edulis seeds. However, we were not able to detect this in this study, and the seedlings may not yet have reflected this event. Additionally, developing seedlings of E. edulis continue to consume seed reserves within approximately 6 months after germination, which might increase survival success even in adverse conditions, potentially explaining the maintenance of seedling abundance in deforested landscapes (Haig and Westoby, 1991; Andrade et al., 1996).
Our results also showed that immature and adult abundance did not change with the landscape forest loss. The number of individuals from this ontogenetic stage was also low, independent of the year evaluated. Nevertheless, when present, this species was among the most abundant adult palm species in our studied area (Benchimol et al., 2017), suggesting that young individuals are those experiencing the worse effects. Previous demographic studies on E. edulis in the southern Bahia region assessed the conservation status of the species (Melito et al., 2014), and indicated that population densities in this region were higher than those in regions stressed by harvesting or intense seed predation, but much smaller than those in areas protected for a longer time, that is, biological reserve with stricter supervision. To maintain viable E. edulis populations in forest remnants, at least 50 adults per hectare is recommended (Freckleton et al., 2003). According to this criterion, only one forest remnant assessed in our study presented a viable population, the exceptional wet one embedded within 19% FC. Thus, while forest loss unaffected the abundance of adults in different years, added to the fact of having little recruitment of seedlings, it is possible that those future adult populations will likely experience negative consequences given that previous ontogenetic stages are showing greater sensitivity to forest loss.
According to our expectations, forest loss had detrimental effects on E. edulis juveniles. These ontogenetic stages showed the highest abundance in accordance to previous demographic studies on E. edulis (Marcos and Matos, 2003; Milanesi, 2012; Portela and Dirzo, 2020). Together, these life stages represent more than 70% of the total abundance of individuals in the evaluated landscapes. These stages might be sensitive to current changes in landscape-scale forest loss due to the known effects of deforestation on forest structure (Rocha-Santos et al., 2016). In deforested landscapes the local forest structure shrinks, becoming similar to secondary forests, i.e., tree density and basal area are reduced, trees are thinner and smaller, leaves become more concentrated in lower forest strata and canopy openness increase (Rocha-Santos et al., 2016). The changes triggered by those disturbances might negatively affect the light regime and microclimate and hydrological forest cycles (Wright, 2010), ultimately affecting E. edulis juveniles.
Implications for conservation
Despite the reduced sample size, our results suggest that E. edulis populations in the southern Bahia were able to hold out during a drought event, but not forest loss at the landscape scale, especially the juvenile stage. This result raises a concern about the future persistence of E. edulis populations, given that the earlier stages will represent the succeeding adult generation in the near future. Given the high deforestation rates in the Atlantic Forest, especially in the Bahia state (Fundação SOS Mata Atlântica and INPE, 2021), we highlight that management actions is urgent to prevent further population decreases in the remaining forest fragments. In particular, we suggest a combination of conservation efforts to reverse the current situation, including: (i) species reintroduction, especially in those highly deforested landscapes; (ii) forest restoration initiatives, together with the maintenance of high amount of forest cover at the landscape scale; (iii) environmental education programs, and (iv) enforcement of the existing environmental laws to avoid the species harvest for human consumption. Safeguarding this endangered species will certainly contribute to the provision of fruit resource for frugivores. In fact, the maintenance of this complex and delicate network is pivotal to assure long-term conservation and resilience of the threatened Atlantic Forest. In the Atlantic Forest, landscape deforestation changes the structure of forest remnants, which tends to be potentiated with climate change. In addition, deforestation by itself affects the persistence of E. edulis, however, the species is also the target of high exploitation, mainly in smaller fragments, where people have easier access. Therefore, it is important to curb deforestation and restore forest remnants in order to mitigate the negative effects of deforestation and climate change.
Data availability statement
The original contributions presented in this study are included in the article/Supplementary material, further inquiries can be directed to the corresponding author.
Author contributions
AL contributed to the conception of the research idea, sample design, data collection, writing, and funding acquisition. MB and EC contributed to the conception of the research idea, sample design, writing, and funding acquisition. HC contributed to the analysis and interpretation of data and writing. DF contributed to the sample design, writing, and funding acquisition. All authors approved the final manuscript.
Funding
The authors disclosed receipt of the following financial support for the research of this article: By “Rede Sisbiota” project (publication number 45), funded by the CNPq (Brazilian Council for Research and Technology) (grant number: 563216/2010-7); UESC–PROPP (Santa Cruz State University–Dean of Research and Graduate Studies) (grant number: 00220.1100.1708); and financed in part by the Coordenação de Aperfeiçoamento de Pessoal de Nível Superior–Brasil (CAPES)–Finance Code 001. This study forms part of a masters research for which AL received a scholarship from FAPESB (Foundation for Research Support of the State of Bahia) (grant number: 409/2016) and EC was granted by CNPq (grant number: 311545/2021-1).
Acknowledgments
We thank the reviewers and editor for all the suggestions and improvements on the manuscript and Albérico Queiroz, Fábio Soares, Ícaro Menezes, Juliana Monteiro, Pollyanna Campos, and Thamyrys Souza for their help in the fieldwork; and local landowners for allowing us to work on their properties. We also thank Rita Portela and Carine Emer for insightful comments along the preparation of the manuscript.
Conflict of interest
The authors declare that the research was conducted in the absence of any commercial or financial relationships that could be construed as a potential conflict of interest.
Publisher’s note
All claims expressed in this article are solely those of the authors and do not necessarily represent those of their affiliated organizations, or those of the publisher, the editors and the reviewers. Any product that may be evaluated in this article, or claim that may be made by its manufacturer, is not guaranteed or endorsed by the publisher.
Supplementary material
The Supplementary Material for this article can be found online at: https://www.frontiersin.org/articles/10.3389/ffgc.2022.909901/full#supplementary-material
References
Allen, C. D., Macalady, A. K., Chenchouni, H., Bachelet, D., McDowell, N., Vennetier, M., et al. (2010). A global overview of drought and heat-induced tree mortality reveals emerging climate change risks for forests. For. Ecol. Manag. 259, 660–684. doi: 10.1016/j.foreco.2009.09.001
Alves, T. S., Alvarado, F., Arroyo-Rodríguez, V., and Santos, B. A. (2020). Landscape-scale patterns and drivers of novel mammal communities in a human-modified protected area. Landsc. Ecol. 35, 1619–1633. doi: 10.1007/s10980-020-01040-6
Andrade, A. D., Venturi, S., and Paulilo, M. T. S. (1996). Efeito do tamanho das sementes de Euterpe edulis Mart. sobre a emergência e crescimento inicial. Rev. Bras. Sementes 18, 225–231.
Barlow, J., França, F., Gardner, T. A., Hicks, C. C., Lennox, G. D., Berenguer, E., et al. (2018). The future of hyperdiverse tropical ecosystems. Nature 559, 517–526. doi: 10.1038/s41586-018-0301-1
Bates, D., Mächler, M., Bolker, B. M., and Walker, S. C. (2015). Fitting linear mixed-effects models using lme4. J. Stat. Softw. 67, 1–48. doi: 10.18637/jss.v067.i01
Benchimol, M., Talora, D. C., Mariano-Neto, E., Oliveira, T. L. S., Leal, A., Mielke, M. S., et al. (2017). Losing our palms: The influence of landscape-scale deforestation on arecaceae diversity in the Atlantic forest. For. Ecol. Manag. 384, 314–322. doi: 10.1016/j.foreco.2016.11.014
Bovendorp, R. S., Brum, F. T., McCleery, R. A., Baiser, B., Loyola, R., Cianciaruso, M. V., et al. (2019). Defaunation and fragmentation erode small mammal diversity dimensions in tropical forests. Ecography 42, 23–35. doi: 10.1111/ecog.03504
Bovi, M. L. A., Júnior, G. G., and Sáes, L. A. (1987). Pesquisas com o genero Euterpe e Bactris no Instituto Agronomico de Campinas. O Agron. 39, 129–174.
Braz, M. I. G., Portela, R., de, C. Q., Cosme, L. H. M., Marques, V. G. C., and de Mattos, E. A. (2014). Germination niche breadth differs in two co-occurring palms of the Atlantic Rainforest. Nat. Conserv. 12, 124–128. doi: 10.1016/j.ncon.2014.09.003
Burnham, K. P., and Anderson, D. R. (2004). “Basic use of the information – theoretic approach,” in Model selection and multimodel inference, eds K. P. Burnham and D. R. Anderson (New York, NY: Springer), 98–148.
Cai, W., Borlace, S., Lengaigne, M., Van Rensch, P., Collins, M., Vecchi, G., et al. (2014). Increasing frequency of extreme El Niño events due to greenhouse warming. Nat. Clim. Chang. 4, 111–116. doi: 10.1038/nclimate2100
Cerqueira, A. F., Rocha-Santos, L., Benchimol, M., and Mielke, M. S. (2021). Habitat loss and canopy openness mediate leaf trait plasticity of an endangered palm in the Brazilian Atlantic Forest. Oecologia 196, 619–663. doi: 10.1007/s00442-021-04879-x
Chazdon, R. L. (2003). Tropical forest recovery: Legacies of human impact and natural disturbances. Perspect. Plant Ecol. Evol. Syst. 6, 51–71. doi: 10.1078/1433-8319-00042
Coelho, G. M. (2010). Estrutura genética populacional em morfotipos de euterpe edulis. Ilhéus: Universidade Estadual de Santa Cruz.
Condit, R., Sukumar, R., Hubbell, S. P., and Foster, R. B. (1998). Predicting population trends from size distributions: A direct test in a tropical tree community. Am. Nat. 152, 495–509. doi: 10.1086/286186
Demirevska, K., Zasheva, D., Dimitrov, R., Simova-Stoilova, L., Stamenova, M., and Feller, U. (2009). Drought stress effects on Rubisco in wheat: Changes in the Rubisco large subunit. Acta Physiol. Plant. 31, 1129–1138. doi: 10.1007/s11738-009-0331-2
Environmental Systems Research Institute [ESRI] (2014). ArcGIS desktop help 10.2 geostatistical analyst.
Eiserhardt, W. L., Svenning, J. C., Kissling, W. D., and Balslev, H. (2011). Geographical ecology of the palms (Arecaceae): Determinants of diversity and distributions across spatial scales. Ann. Bot. 108, 1391–1416. doi: 10.1093/aob/mcr146
Engelbrecht, B. M. J., Comita, L. S., Condit, R., Kursar, T. A., Tyree, M. T., Turner, B. L., et al. (2007). Drought sensitivity shapes species distribution patterns in tropical forests. Nature 447, 80–82. doi: 10.1038/nature05747
Fadini, R. F., Fleury, M., Donatti, C. I., and Galetti, M. (2009). Effects of frugivore impoverishment and seed predators on the recruitment of a keystone palm. Acta Oecol. 35, 188–196. doi: 10.1016/j.actao.2008.10.001
Fahrig, L. (2003). Effects of habitat fragmentation on biodiversity. Annu. Rev. Ecol. Evol. Syst. 34, 487–515. doi: 10.1146/annurev.ecolsys.34.011802.132419
Fauset, S., Gloor, M. U., Aidar, M. P. M., Freitas, H. C., Fyllas, N. M., Marabesi, M. A., et al. (2017). Tropical forest light regimes in a human-modified landscape. Ecosphere 8:e02002. doi: 10.1002/ecs2.2002
Foden, W. B., Young, B. E., Akçakaya, H. R., Garcia, R. A., Hoffmann, A. A., Stein, B. A., et al. (2019). Climate change vulnerability assessment of species. Wiley Interdiscip. Rev. Clim. Change 10, 1–36. doi: 10.1002/wcc.551
Foley, J. A., DeFries, R., Asner, G. P., Barford, C., Bonan, G., Carpenter, S. R., et al. (2005). Global consequences of land use. Science 309, 570–574. doi: 10.1126/science.1111772
Freckleton, R. P., Silva Matos, D. M., Bovi, M. L. A., and Watkinson, A. R. (2003). Predicting the impacts of harvesting using structured population models: the importance of density-dependance and timing of harvest for a tropical palm tree. J. Appl. Ecol. 40, 846–858.
Fundação SOS Mata Atlântica and INPE (2021). Atlas dos remanescentes florestais da Mata Atlântica: Período 2019/2020, relatório técnico. São Paulo: Fundação SOS Mata Atlântica, 73.
Galetti, M., and Fernandez, J. C. (1998). Palm heart harvesting in the Brazilian atlantic forest: Changes in industry structure and the illegal trade. J. Appl. Ecol. 35, 294–301. doi: 10.1046/j.1365-2664.1998.00295.x
Galetti, M., Guevara, R., Cortes, M. C., Fadini, R., Von Matter, S., Leite, A. B., et al. (2013). Functional extinction of birds drives rapid evolutionary changes in seed size. Science 340, 1086–1090. doi: 10.1126/science.1233774
Garcia, D., and Martinez, D. (2012). Species richness matters for the quality of ecosystem services: a test using seed dispersal by frugivorous birds. Proc. R. Soc. B Biol. Sci. 279, 3106–3113. doi: 10.1098/rspb.2012.0175
Gateau-rey, L., Tanner, E. V. J., Rapidel, B., Marelli, P., and Royaert, S. (2018). Climate change could threaten cocoa production : Effects of 2015-16 El Ni ñ o-related drought on cocoa agroforests in Bahia, Brazil. PLoS One 13:e0200454. doi: 10.1371/journal.pone.0200454
Gatti, M. G., Campanello, P. I., and Goldstein, G. (2011). Growth and leaf production in the tropical palm Euterpe edulis: Light conditions versus developmental constraints. Flora Morphol. Distribut. Funct. Ecol. Plants 206, 742–748. doi: 10.1016/j.flora.2011.04.004
Gatti, M. G., Campanello, P. I., Villagra, M., Montti, L., and Goldstein, G. (2014). Hydraulic architecture and photoinhibition influence spatial distribution of the arborescent palm Euterpe edulis in subtropical forests. Tree Physiol. 34, 630–639. doi: 10.1093/treephys/tpu039
Getirana, A. (2016). Extreme water deficit in Brazil detected from space. J. Hydrometeorol. 17, 591–599. doi: 10.1175/JHM-D-15-0096.1
Haig, D., and Westoby, M. (1991). Seed size, pollination costs and angiosperm success. Evol. Ecol. 5, 231–247. doi: 10.1007/BF02214230
Hardin, J. W., and Hilbe, J. (2007). Generalized linear models and extensions. College Station, TX: Stata Press.
Henderson, A., Galeano, G., and Bernal, R. (1995). Field guide to the palms of the Americas. Princeton, NJ: Princeton University Press.
Holbrook, K. M. (2011). Home range and movement patterns of toucans : implications for seed dispersal. Biotropica 43, 357–364.
Hughes, L., and Westoby, M. (1992). Fate of seeds adapted for dispersal by ants in australian sclerophyll vegetation. Ecology 73, 1285–1299.
Instituto Nacional De Meteorologia (2022). INMET. Available online at: https://bdmep.inmet.gov.br/# (accessed August 29, 2022).
Jordano, P., Pulido, F., Arroyo, J., García-Castaño, J. L., and García-Fayos, P. (2004). “Procesos de limitación demográfica,” in Ecología del bosque mediterráneo en un mundo cambiante, ed. F. Valladares (Madrid: Ministerio de Medio Ambiente), 229–248.
Lawrence, D., and Vandecar, K. (2015). Erratum: Effects of tropical deforestation on climate and and agriculture. Nat. Clim. Chang. 5:174. doi: 10.1038/NCLIMATE2430
Leal, A., Benchimol, M., Faria, D., Dodonov, P., and Cazetta, E. (2021). Landscape-scale forest loss shapes demographic structure of the threatened tropical palm Euterpe edulis mart. (Arecaceae). For. Ecol. Manag. 502:119716.
Liebsch, D., Marques, M. C. M., and Goldenberg, R. (2008). How long does the Atlantic Rain Forest take to recover after a disturbance? Changes in species composition and ecological features during secondary succession. Biol. Conserv. 141, 1717–1725. doi: 10.1016/j.biocon.2008.04.013
Lloret, F., Escudero, A., Iriondo, J. M., Martínez-Vilalta, J., and Valladares, F. (2012). Extreme climatic events and vegetation: The role of stabilizing processes. Glob. Chang. Biol. 18, 797–805. doi: 10.1111/j.1365-2486.2011.02624.x
Luo, Z., Guan, H., Zhang, X., Zhang, C., Liu, N., and Li, G. (2016). Responses of plant water use to a severe summer drought for two subtropical tree species in the central southern China. J. Hydrol Reg. Stud. 8, 1–9. doi: 10.1016/j.ejrh.2016.08.001
Marcos, C. S., and Matos, D. M. S. (2003). Estrutura de populações de palmiteiro (Euterpe edulis Mart.) em áreas com diferentes graus de impactação na Floresta da Tijuca, RJ. Floresta E Ambiente 10, 27–37.
Martinelli, G., and Moraes, M. A. (2013). Livro vermelho da Flora do Brasil, 1st Edn. Rio de Janeiro: Instituto de Pesquisas Jardim Botânico do Rio de Janeiro.
Martini, A. M. Z., Fiaschi, P., Amorim, A. M., and Da Paixão, J. L. (2007). A hot-point within a hot-spot: A high diversity site in Brazil’s Atlantic Forest. Biodivers. Conserv. 16, 3111–3128. doi: 10.1007/s10531-007-9166-6
Melito, M. O., Faria, J. C., Amorim, A. M., and Cazetta, E. (2014). Demographic structure of a threatened palm (Euterpe edulis Mart.) in a fragmented landscape of Atlantic Forest in northeastern Brazil. Acta Bot. Bras. 28, 249–258. doi: 10.1590/S0102-33062014000200011
Melo, I., Ochoa-Quintero, J. M., de Oliveira Roque, F., and Dalsgaard, B. (2018). A review of threshold responses of birds to landscape changes across the world. J. Field Ornithol. 89, 303–314. doi: 10.1111/jofo.12272
Menezes Pinto, Í, Emer, C., Cazetta, E., and Morante-Filho, J. C. (2021). Deforestation simplifies understory bird seed-dispersal networks in human-modified landscapes. Front. Ecol. Evol. 9:640210. doi: 10.3389/fevo.2021.640210
Milanesi, L. (2012). Dinâmica de uso da paisagem e sua influência nas características populacionais de Euterpe edulis Martius. Available online at: http://repositorio.ufsc.br/handle/123456789/96285 (accessed March 01, 2022).
Morante-Filho, J. C., Faria, D., Mariano-Neto, E., and Rhodes, J. (2015). Birds in anthropogenic landscapes: The responses of ecological groups to forest loss in the Brazilian Atlantic forest. PLoS One 10:e0128923. doi: 10.1371/journal.pone.0128923
Mori, S. A., Boom, B. M., de Carvalho, A. M., and dos Santos, T. S. (1983). Southern Bahian moist forests. Bot. Rev. 49, 155–232. doi: 10.1007/BF02861011
Morris, W. F., Pfister, C. A., Tuljapurkar, S., Chirrakal, V., Haridas, C. L. B., Boyce, M. S., et al. (2008). Longevity can buffer plant and animal populations against changing climatic variability. Ecology 89, 19–25. doi: 10.1890/07-0774.1
Muscarella, R., Emilio, T., Phillips, O. L., Lewis, S. L., Slik, F., Baker, W. J., et al. (2020). The global abundance of tree palms. Glob. Ecol. Biogeogr. 29, 1495–1514. doi: 10.1111/geb.13123
Nakagawa, S., Johnson, P. C. D., and Schielzeth, H. (2017). The coefficient of determination R2 and intra-class correlation coefficient from generalized linear mixed-effects models revisited and expanded. J. R. Soc. Interf. 14:20170213. doi: 10.1098/rsif.2017.0213
Oliveira, L. C. (2014).Efeitos da perda de habitat na densidade de indivíduos e fenologia reprodutiva de Euterpe edulis na região sul da Bahia. Universidade Estadual de Santa Cruz: Ilhéus, BA.
Phillips, O. L., Aragão, L. E. O. C., Lewis, S. L., Fisher, J. B., Lloyd, J., López-González, G., et al. (2009). Drought sensitivity of the Amazon Rainforest. Science 323, 1344–1347. doi: 10.1126/science.1164033
Portela, R. C. Q. (2008). Ecologia populacional de tres especies de palmeiras em uma paisagem fragmentada no dominio da Mata Atlantica, RJ. Tese de Doutorado. Campinas: Universidade Estadual de Campinas.
Portela, R. D. C. Q., and Dirzo, R. (2020). Forest fragmentation and defaunation drive an unusual ecological cascade: Predation release, monkey population outburst and plant demographic collapse. Biol. Conserv. 252:108852. doi: 10.1016/j.biocon.2020.108852
Portela, R., de, C. Q., da Silva, V. M. F., Mendes, E. T. B., de Lima, T. M., Braz, M. I. G., et al. (2020). Differential phenological shifts in euterpe edulis mart. During an extremely dry year along an altitudinal gradient. Oecol. Australis 24, 389–405. doi: 10.4257/oeco.2020.2402.11
Rigueira, D. M. G., da Rocha, P. L. B., and Mariano-Neto, E. (2013). Forest cover, extinction thresholds and time lags in woody plants (Myrtaceae) in the Brazilian Atlantic Forest: Resources for conservation. Biodivers. Conserv. 22, 3141–3163. doi: 10.1007/s10531-013-0575-4
Rocha-Santos, L., Pessoa, M. S., Cassano, C. R., Talora, D. C., Orihuela, R. L. L., Mariano-Neto, E., et al. (2016). The shrinkage of a forest: Landscape-scale deforestation leading to overall changes in local forest structure. Biol. Conserv. 196, 1–9. doi: 10.1016/j.biocon.2016.01.028
Sebastián-González, E. (2017). Drivers of species role in avian seed-dispersal mutualistic networks. J. Anim. Ecol. 12, 3218–3221. doi: 10.1111/ijlh.12426
Silva, M. D. G. C. P. C., Martini, A. M. Z., and De Araújo, Q. R. (2009). Estrutura populacional de Euterpe edulis Mart. no Sul da Bahia. Brasil. Rev. Bras. Bot. 32, 393–403. doi: 10.1590/S0100-84042009000200017
Soares, L. A. S. S., Faria, D., Vélez-Garcia, F., Vieira, E. M., Talora, D. C., and Cazetta, E. (2015). Implications of habitat loss on seed predation and early recruitment of a keystone palm in anthropogenic landscapes in the Brazilian Atlantic Rainforest. PLoS One 10:e0133540. doi: 10.1371/journal.pone.0133540
Souza, T. B., França, F. M., Barlow, J., Dodonov, P., Santos, J. S., Faria, D., et al. (2020). The relative influence of different landscape attributes on dung beetle communities in the Brazilian Atlantic forest. Ecol. Indic. 117:106534. doi: 10.1016/j.ecolind.2020.106534
Spera, S. A., Winter, J. M., and Partridge, T. F. (2020). Brazilian maize yields negatively affected by climate after land clearing. Nat. Sustain. 3, 845–852. doi: 10.1038/s41893-020-0560-3
Tabarelli, M., Aguiar, A. V., Ribeiro, M. C., Metzger, J. P., and Peres, C. A. (2010). Prospects for biodiversity conservation in the Atlantic Forest: Lessons from aging human-modified landscapes. Biol. Conserv. 143, 2328–2340. doi: 10.1016/j.biocon.2010.02.005
Terborgh, J. (1986). “Keystone plant resources in the tropical forest,” in Conservation Biology, eds I. Soulé and E. Michael (Sunderland: Sinauer), 330–344.
Thomas, W. W. (2003). “Natural vegetation types in southern Bahia,” in Corredor de Biodiversidade da Mata Atlântica do Sul da Bahia, eds P. I. Prado, E. C. Landau, R. T. Moura, L. P. S. Pinto, G. A. B. Fonseca, and K. Alger (Ilhéus: Publicação em CD-ROM).
Thomas, W., Carvalho, A., Amorim, A., Garrison, J., and Arbeláez, A. (1998). Plant endemism in two forests in southern Bahia, Brazil. Biodivers. Conserv. 7, 311–322. doi: 10.1023/A:1008825627656
Thompson, C. M., and McGarigal, K. (2002). The influence of research scale on bald eagle habitat selection along the lower Hudson River, New York (USA). Landsc. Ecol. 17, 569–586. doi: 10.1023/A:1021501231182
Valiente-Banuet, A., Aizen, M. A., Alcántara, J. M., Arroyo, J., Cocucci, A., Galetti, M., et al. (2015). Beyond species loss: The extinction of ecological interactions in a changing world. Funct. Ecol. 29, 299–307. doi: 10.1111/1365-2435.12356
Wright, S. J. (2010). The future of tropical forests. Ann. N. Y. Acad. Sci. 1195, 1–27. doi: 10.1111/j.1749-6632.2010.05455.x
Keywords: Arecaceae, habitat loss, ontogenetic stages, regional drought, tropical palm
Citation: Leal A, Benchimol M, Costa HCM, Faria D and Cazetta E (2022) Impacts of landscape-scale forest loss and a dry event on the demographic structure of the endangered palm Euterpe edulis Mart. in the Atlantic Forest. Front. For. Glob. Change 5:909901. doi: 10.3389/ffgc.2022.909901
Received: 31 March 2022; Accepted: 01 September 2022;
Published: 20 September 2022.
Edited by:
Marion Pfeifer, Newcastle University, United KingdomReviewed by:
Iván Torres, University of Castilla-La Mancha, SpainEric J. Gustafson, Northern Research Station, United States Forest Service (USDA), United States
Copyright © 2022 Leal, Benchimol, Costa, Faria and Cazetta. This is an open-access article distributed under the terms of the Creative Commons Attribution License (CC BY). The use, distribution or reproduction in other forums is permitted, provided the original author(s) and the copyright owner(s) are credited and that the original publication in this journal is cited, in accordance with accepted academic practice. No use, distribution or reproduction is permitted which does not comply with these terms.
*Correspondence: Adrielle Leal, YWRyaWVsbGVfbGVhbEBob3RtYWlsLmNvbQ==