- 1Italian Academy of Forest Sciences, Florence, Italy
- 2Department of Agriculture, Food, Environment and Forestry, University of Florence, Florence, Italy
The aim of this study was to investigate the potential of the Biological Soil Quality index (QBS-ar index) for monitoring the impact of wildfires on Mediterranean pine forests soils. The QBS-ar is a simplified eco-morphological index, based on edaphic microarthropods. We analyzed the QBS-ar values of pine stands burned one or two times and at different time spans from fire (from 10 days to 11 years after fire), in comparison to the unburned control area. Effect of fire on the biological soil quality evaluated with QBS-ar index was analyzed using univariate and multivariate techniques. Our results showed a significant variation of QBS-ar values between burned areas and those never burned. The impact of fire on the QBS-ar index and the microarthropod community was higher in the areas burned twice. A relevant average dissimilarity of taxonomic groups was found between the control area and the area burned twice, but also between the control area and the one observed 3 months after the fire. The taxa that contributed more to the dissimilarity were Pseudoscorpiones and Diplopoda, which reduced their presence after fire. Our study shows that the QBS-ar index is a dependable method for monitoring the impact of fire on soil quality in forest ecosystems and indicates that edaphic microarthropods communities in these Mediterranean pine forests return to the before fire condition 11 years after fire. The QBS-ar index could therefore be a useful tool for monitoring forest ecosystem rehabilitation after fire.
Introduction
Wildfires are one of the major disturbances in Mediterranean forest ecosystems worldwide (Keeley et al., 2012), affecting their ability to provide ecosystem services (e.g., tourism, protection, biomass production, biodiversity conservation, climate mitigation) (Taboada et al., 2021; Nocentini et al., 2022).
Frequent wildfires can trigger soil degradation by reducing or temporarily eliminating the vegetation cover and exposing the soil to erosion agents such as water and wind, thus reducing soil quality as well as ecosystem resilience to consecutive fires (Baeza et al., 2007); therefore, assessing fire impacts on soil is critical to quantifying land degradation processes and to support post-fire restoration plans (Caon et al., 2014; Francos et al., 2020; Jiménez-Morillo et al., 2020; Fernández-García et al., 2021).
Soil biota is one of the first soil components to respond to fire exposure, as fire alters species composition and decreases zoocenotic density (Bezkorovainay et al., 2007).
Soil biological quality is defined as the capacity of a specific kind of soil to function within natural or managed ecosystem boundaries, to sustain plant and animal productivity, to maintain or enhance the air and water quality and support human health (Doran and Parkin, 1994; Karlen et al., 1997, 2008). Soil biological quality has also direct and indirect relationships with the ability of a soil to stock carbon (Luo et al., 2017), and is thus a critical parameter for ensuring environmental sustainability.
Soil biota and particularly edaphic microarthropods in addition to supporting soil carbon accrual (Soong et al., 2016), play an important role in regulating rates of decomposition (Wallwork, 1983; Seastedt, 1984) and nutrient cycling (Heneghan and Bolger, 1998; Yan et al., 2012; Lakshmi et al., 2020) through interactions with the microbial and fungal communities (Seastedt, 1984; Lussenhop, 1992), contributing to soil structure and humus formation (Wallwork, 1983; Striganova, 2000).
For all these reasons and for its connection to climate change (Lakshmi et al., 2020; Barreto and Lindo, 2022) the preservation of soil biodiversity should be considered an integral component of forest management practices (Marshall, 2000).
The methods proposed for biological soil quality assessment are either based on a general evaluation of edaphic microarthropods communities (Parisi, 2001, Parisi et al., 2005; Blasi et al., 2013; Galli et al., 2014; Pinto et al., 2017; Lazzaro et al., 2018), or consider a single taxon (Lobry de Bruyn, 1999; Paoletti and Hassal, 1999; Gulvik, 2007). In general, the use of biotic indices based on edaphic invertebrate communities is still limited, probably because of the difficulties in classifying these organisms and because they are highly sensitive to natural and anthropic disturbances.
A simplified eco-morphological index, the QBS-ar (Biological Soil Quality index, based on edaphic microarthropods, Parisi, 2001; Parisi et al., 2005) has been proposed as a means for biomonitoring soil quality. The QBS-ar index is based on the biological form approach (Sacchi and Testard, 1971) and does not require the classification of organisms at species level, but only at order/class level.
After 15 years of QBS-ar index applications at the global scale (Menta et al., 2018), this index has been recognized as a standard protocol for measuring soil fauna across Europe in the LTER sites ExpeErt Ecosystem Research Program (Firbank et al., 2017), and from 2010 it is reported also by the European Commission DG ENV (Turbé et al., 2010).
Edaphic microarthropods have been utilized for soil biomonitoring after forest fires (Saulnier and Athias-Binche, 1986; Broza et al., 1993; Sgardelis et al., 1995; Broza and Izhaki, 1997; García-Ruiz, 2001; Tajovský, 2002; Trucchi et al., 2009; Lisa et al., 2015; Certini et al., 2021), however, to our knowledge, the QBS-ar index has been tested only in a recent study for monitoring biological soil quality after wildfires in Mediterranean area and in different types of stands (Mantoni et al., 2020).
The aim of our work was to evaluate the effectiveness of the QBS-ar index in monitoring the impact of fire on soil in a Mediterranean pine forest. Specifically, our objectives were: (a) to check the index efficiency at three points in time after the fire: immediately, 2–3 and 10–11 years later and (b) to analyze the effect of fire recurrence.
We selected the QBS-ar index because it is based on the concept that the higher the soil quality the higher will be the number of the edaphic microarthropod groups, and above all, because it is relatively simple to use in practice (Parisi et al., 2005; Gardi et al., 2008).
Materials and Methods
Study Area
We applied the QBS-ar method in an area where we had already studied the effect of fire frequency on edaphic microarthropod abundance (Lisa et al., 2015). The study was carried out in Tuscany (Central Italy) in a hilly area 50 km east of Pisa (Figure 1).
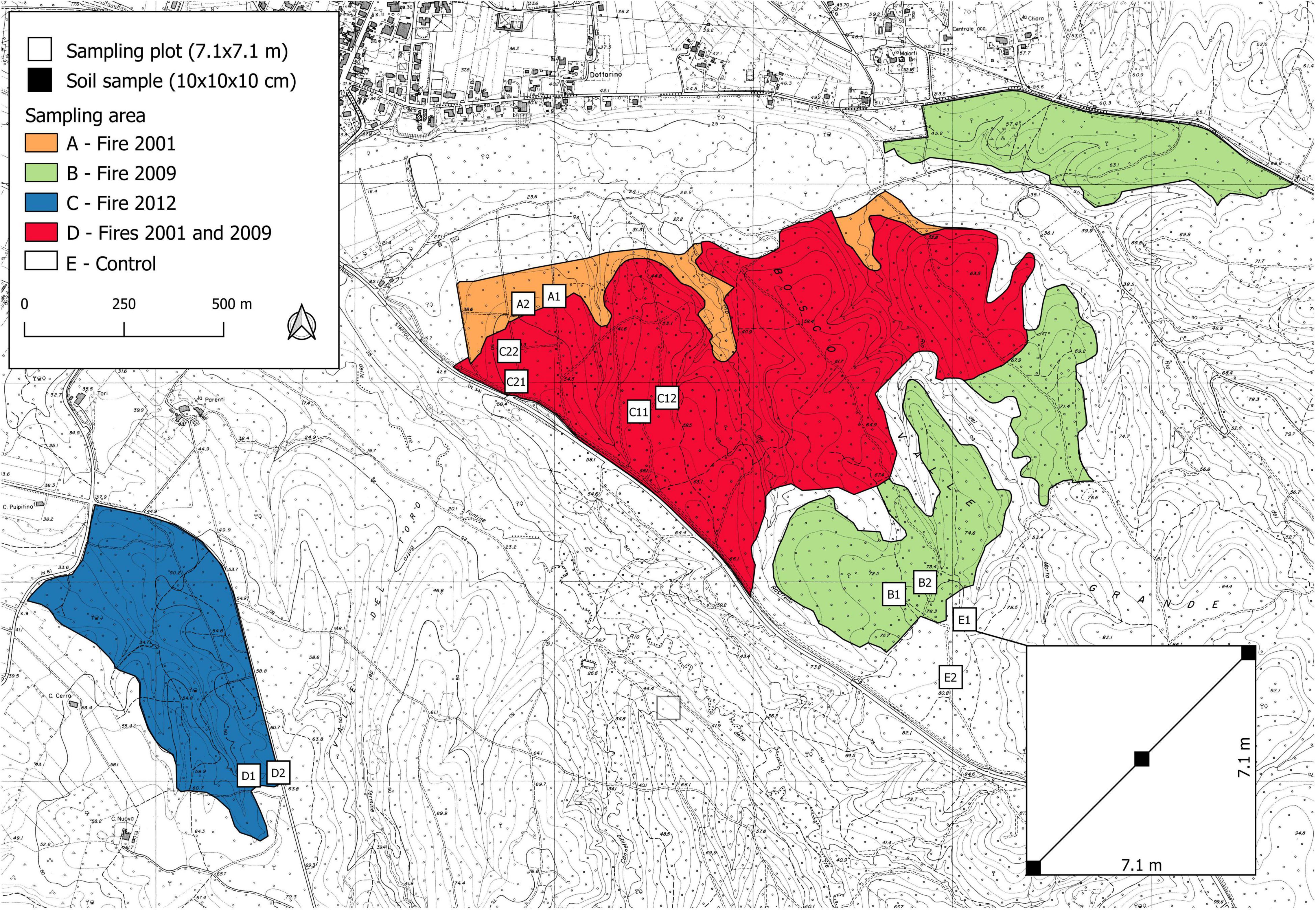
Figure 1. Sampling areas (A–E), distribution of the sampling plots, and scheme for soil sampling within the plot.
The area is located at an altitude between 45 and 114 m a.s.l. and it is characterized by a humid temperate climate (Köppen, 1936; Sbragia, 2004) with an average annual rainfall of 1,000–1,150 mm (concentrated in autumn and winter) and an average annual temperature of 14–15°C. The geological setting is a coastal and sandy conglomerate (Pliocene), the soils are loose, medium-deep, with a mainly acid reaction and variable in consistency, drainage and pore (Olivari, 2004).
The most common forest types are maritime pine (Pinus pinaster Aiton) and mixed oaks (Quercus cerris L. and Q. petraea Liebl.). Further details on area description and forest species composition can be found in Lisa et al. (2015).
The study area is classified as “high fire frequency area.” Between 2001 and 2012 the area had been hit by three wildfires, in August 2001, August 2009, and March 2012, with some areas burned twice, in 2001 and 2009. The information about these fires is showed in Table 1. In all cases, fire spread mainly as passive and active crown fires involving duff, litter, shrubs and the tree canopy. Burn severity was estimated at the time of fire by fire managers involved in fire suppression operations based on direct field observations; the severity of all fires ranged from “moderate or severe surface burn” to “deep burning or crown fire,” as reported in Keeley (2009). The “moderate or severe surface burn” fire severity category includes areas with “trees with some canopy cover killed, but needles not consumed, all understorey plants charred or consumed, fine dead twigs on soil surface consumed and logs charred.” The “deep burning or crown fire” severity category includes areas with “canopy trees killed and needles consumed, surface litter of all sizes and soil organic layer largely consumed, white ash deposition and charred organic matter to several cm depth,” The matrix reported by Keeley (2009) was proposed by Ryan and Noste (1985) and modified from Turner et al. (1994) and Ryan (2002).
In 2011–2012, at the moment of sample collection, natural pine regeneration (around 10 years old) had set in, together with many shrubs and ferns [Pteridium aquilinum (L.) Kuhn] in the area burned in 2001. In the area burned in 2009 there were burned pine snags and ferns. The areas burned both in 2001 and in 2009 were covered by nitrogen-fixing species such as brooms [Cytisus scoparius (L.) Link], cistus (Cistus spp.), heathers (Ericae spp.), and fire moss [Ceratodon purpureus (Hedw.) Brid]. In the area burned in 2012 the terrain was totally covered by gray and white ash and the undergrowth layer was completely destroyed by fire; most of the trees were highly fire damaged or dead (see Figure 2 in Lisa et al., 2015). In the area affected by fire in 2001 and 2009, i.e., high fire recurrence (two fires in 8 years), the natural pine regeneration was missing due to the lack of a viable seed bank.
Data Sampling
Monitoring of biological soil quality after wildfire was carried out in the years 2011 and 2012 in 5 sampling areas (Figure 1), including one control area (Lisa et al., 2015; Table 2):
- Area burned in August 2001 (A);
- Area burned in August 2009 (B);
- Area burned twice, in 2001 and again in 2009 (C);
- Area burned in March 2012 (D);
- Area not affected by fire at least in the preceding 40 years (Control E).
In area C, two sub-areas (C1 and C2) were selected to take into account the apparent higher soil variability due to the presence of areas covered by shrubs (C1) and areas covered by moss (C2), but also because area C is larger than other sampling areas (Figure 1).
In each area, field work was carried out in two sampling plots 7.1 × 7.1 m with homogeneous forest cover, slope, exposure and soil conditions. The geographic coordinates of all 12 sampling plots recorded with a GPS receiver with 2–5 m positional accuracy are reported in Table 2. For the identification of edaphic microarthropod communities, in each sampling plot three soil samples (10 × 10 × 10 cm) were collected along a 10-m transect placed along the plot diagonal (Figure 1), for a total of 36 sampling points.
In areas A, B, C, and in the Control E, soil samples were collected four times between 2011 and 2012: in Spring 2011, in Autumn 2011, in Spring 2012, in Autumn 2012. In area D, soil samples were collected three times in 2012 after the passage of fire: 10 days, 3 and 5 months after the fire (Table 2). Thus, we were able to perform soil monitoring at three points in time after the passage of fire: in the short-term interval (from 10 days to 5 months in area D), in the intermediate interval (2–3 years in area B), and in the long-term interval (10–11 years in area A) after the fire.
In each plot, the soil was analyzed for carbon content and pH by collecting three randomly selected samples with a metal cylinder (10-cm long with a 5-cm inner diameter) after litter removal. A moisture meter (HH2—Readout Unit; Delta-T Devices) was used to measure soil moisture and temperature at the depth of 10 cm.
Biological Soil Quality Evaluation
Edaphic microarthropods were extracted from soil samples using the procedure described in Lisa et al. (2015). Then a stereomicroscope with a magnification of 40 x was used to classify the soil fauna to the Class/Order taxonomic level.
The biological soil quality was evaluated using the QBS-ar index (Parisi et al., 2005). Soil organisms were separated into biological forms according to their soil morphological adaptation. Each of these forms was associated with a score, named eco-morphological index (EMI), which ranges from 1 to 20.
As a general rule, eu-edaphic forms (i.e., deep-soil living) get an EMI = 20, epi-edaphic forms (i.e., surface-living) receive a score equal to 1 and hemi-edaphic forms (intermediate) receive an intermediate score proportionate to their degree of specialization. Some orders of microarthropods have multiple scores (e.g., Chilopoda, Diplopoda, Collembola, and Coleoptera), whereas others (e.g., Protura, Symphila, and Diplura) receive a single score, because all species belonging to these groups show a similar level of adaptation. Scoring tables of Eco-morphologic indices (EMIs) was published by Parisi in 2001.
The QBS-ar index value was obtained from the sum of the EMI of all collected groups. If in a group, biological forms with different EMI scores were considered, only the higher value, which represents the highest degree of adaptation to edaphic life in the soil was selected to represent the group in the QBS-ar calculation (Testi et al., 2012). The higher biological soil quality corresponds to the higher value of QBS-ar ranging from 0 to >200.
The carbon content in the soil was assessed using the Walkley-Black method (Nelson and Sommers, 1996). A pH meter with a suspension of 10 g of soil and water, with a soil solution ratio of 1:5 was used to determinate the soil pH.
Statistical Analysis
Effect of fire on the biological soil quality, evaluated with the QBS-ar index, was analyzed using both univariate and multivariate techniques. Statistical analysis was performed using PAST Program ver. 2.17 (Hammer et al., 2001).
The non-parametric Kruskal-Wallis test (Kruskal and Wallis, 1952) was used to evaluate differences of QBS-ar values in plots with different time span from the last fire. In case of statistically significant differences (p ≤ 0.05), the Mann-Whitney post hoc comparison test was used, and the Bonferroni correction applied (Bonferroni, 1935, 1936).
To identify community gradients in the short- (5 months), intermediate-(2–3 years) and long-(10–11 years) term intervals, a principal component analysis (PCA) (Davis, 1986; Harper, 1999) was carried out on a rectangular matrix (samples plot × taxa) of EMI data. A bootstrap resampling technique with 1,000 replicates was employed to evaluate the number of informative axes (Jackson, 1993). The significance of eigenvector coefficients was evaluated by determining the 95% confidence limits. Eigenvectors were then considered informative if at least two variables were significant on that axis.
The Non-Parametric MANOVA (NPMANOVA), based on a Bray–Curtis distance applied on EMI data matrix was carried out to verify differences of microarthropod community structure among disturbed/undisturbed areas (burned in 2001, 2009, 2012, and burned twice).
The SIMPER analysis (cut off, ≈70%, Clarke, 1993) with Bray-Curtis similarity measure was used to quantify the contribution of each taxa to the dissimilarity among groups of samples. Then the groups of taxa which most contributed to dissimilarity were checked with the Kruskal-Wallis test to assess if fire influenced the presence or absence of specific microarthropod groups.
The relationships between QBS-ar values and physical (carbon content, moisture and temperature) and chemical (pH) soil characteristics were investigated by means of linear regression analysis.
Results
Effects of Fire on the Biological Soil Quality Index
Out of 129 soil samples collected from 2011 to 2012, a total of 23 microarthopod groups belonging to Chelicerarata and Mandibolata subphyla were identified. The Chelicerata subphylum is represented by Araneae, Pseudoscorpiones and Acari, while Isopoda (1 taxon) and Tracheata (19 taxa) represent the Mandibolata subphylum.
The average number of taxa and QBS-ar found during all periods of soil sampling (from Spring 2011 to Autumn 2012) is reported in Figure 2, which shows that areas E and A had the highest biodiversity levels in the term of number of taxa (>15), while area C had the lowest biodiversity level (<10). Figure 2 also shows a reduction in the number of taxa in the short-term period (area D) respect to the intermediate-(area B) and the long-term (area A) periods after fire; in particular, we noted the absence of Pseudoscorpions 3 months after the fire in area D.
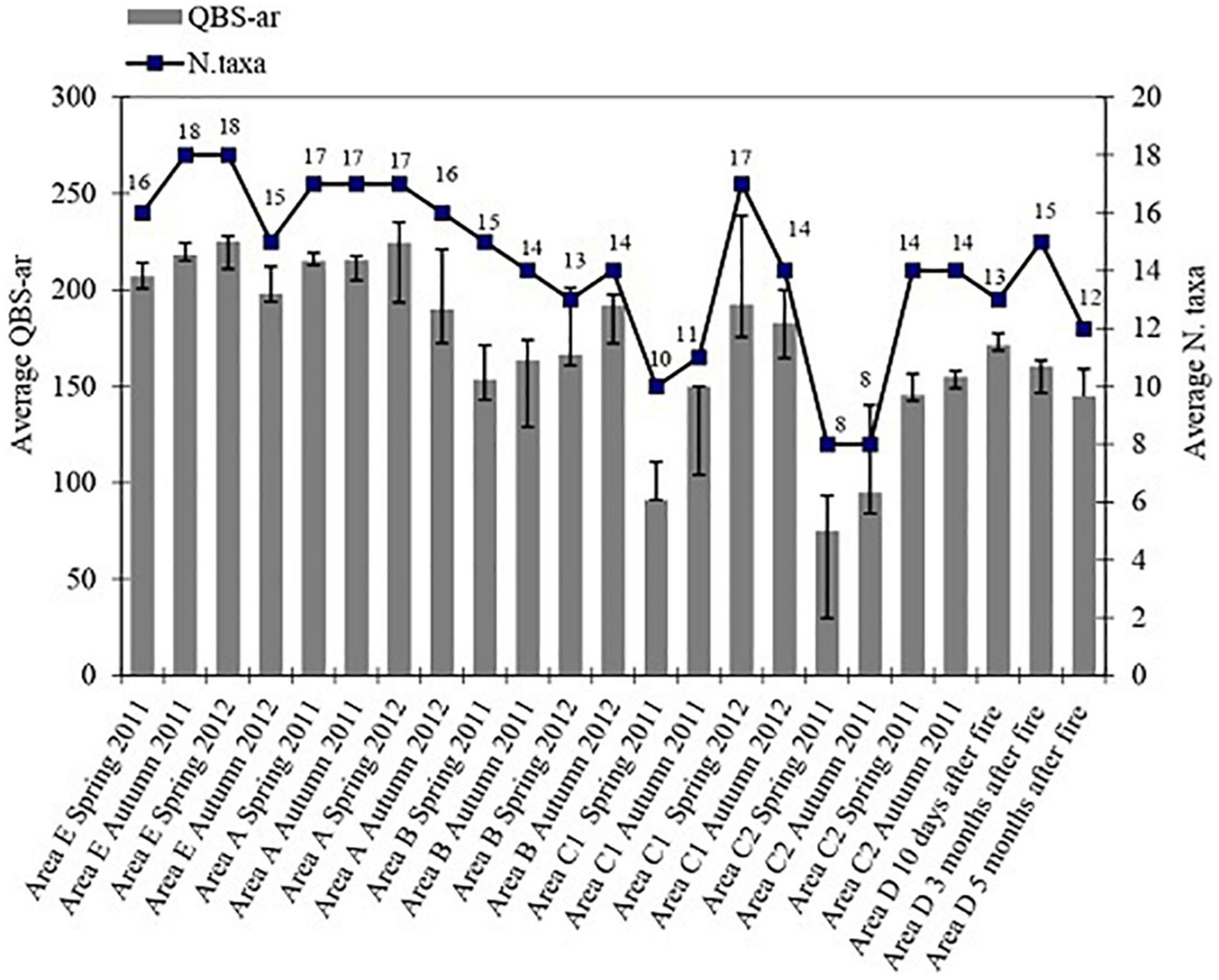
Figure 2. Average number of taxa and average values of QBS-ar in the sampling area from Spring 2011 to Autumn 2012.
The highest number of taxa (18) corresponds to the highest QBS-ar value (225) found in the area E in Spring 2012, while the lowest number of taxa (8) was observed in the two samples from the areas burned twice (C), with an average QBS-ar of 91 for area C1 (Spring 2011) and 75 for area C2 (Autumn 2011).
We found a positive relationship between QBS-ar values and the number of taxonomic groups with a coefficient of determination (R2) equal to 0.73 (p < 0.01). The same positive relationship was also observed by Blasi et al. (2013).
Table 3 shows the average QBS-ar values and the standard deviation obtained during all periods of soil sampling (from Spring 2011 to Autumn 2012), while the distribution of QBS-ar values is shown in Figure 3. Values >200 all came from areas E and A, while those <100 came exclusively from the area burned twice (C). The soil samples collected shortly after fire in area D showed QBS-ar values ranging between 181 and 135.
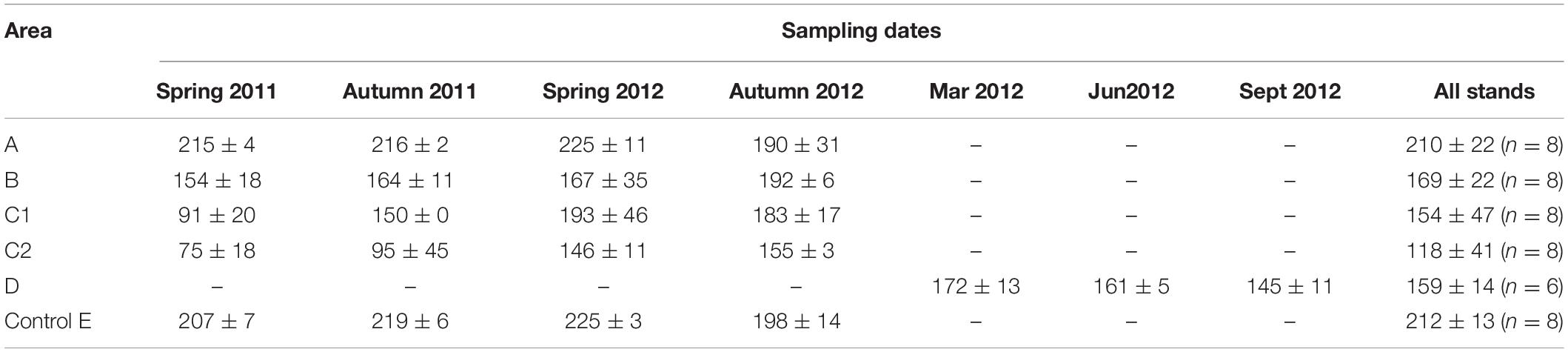
Table 3. Average QBS-values and standard deviation obtained during seasonally and annual monitoring (n = number of samples/year).
The effect of fire on biological soil quality, evaluated with QBS-ar, was assessed with the non-parametric Kruskal–Wallis test (Kruskal and Wallis, 1952) using all samples. The test was highly significant (p < 0.001), with Hc value equal to 29.79 (Table 4). A significant variation of QBS-ar was observed between Control (E) and all burned areas, except for the 2001 fire (A). In the sub-area C2 (burned twice), the effect of fire on the microarthropod community was more evident.
In the sampling plots no significant relationships were found between QBS-ar values and physical (moisture, temperature carbon content) and chemical (pH) properties of soil.
Multivariate Analysis
The Principal Component Analysis showed that the first two components explain overall 53% of the total variance (eigenvalues 2.5%) (Figure 4).
The PC1 (37% of variances) shows a significant direct correlation with QBS–ar values (Figure 5), which may be interpreted as a forest soil quality gradient. This significant direct correlation was also observed in a study of QBS-ar in Mediterranean forest ecosystems (Blasi et al., 2013).
The soil samples characterized by the highest soil quality, estimated by the QBS-ar index, are grouped in the positive half of the PC1 (right side in Figure 4) and most of them belong to Control (E) and the area burned in 2001 (A).
Soil samples characterized by the lowest soil quality are grouped together in the negative half-axis of PC1 (left side of Figure 4) and most of them belong to the samples collected in the area burned twice (C1 and C2) except for two soil samples collected in C2 during autumn 2012.
PC1 was mainly related to the presence of Pseudoscorpiones, Diplopoda, Symphyla, Chilopoda, Pauropoda, Protura and Euedaphic Collembola (EMI 20) (Figure 6). Protura, Simphyla and Pauropoda are typical groups of stable environments characterized by a high level of soil morphological adaptations which does not allow them to resist to adverse environmental conditions. PC2, which explains 16% of the total variance, was related to the central role of Pauropoda and Symphyla.
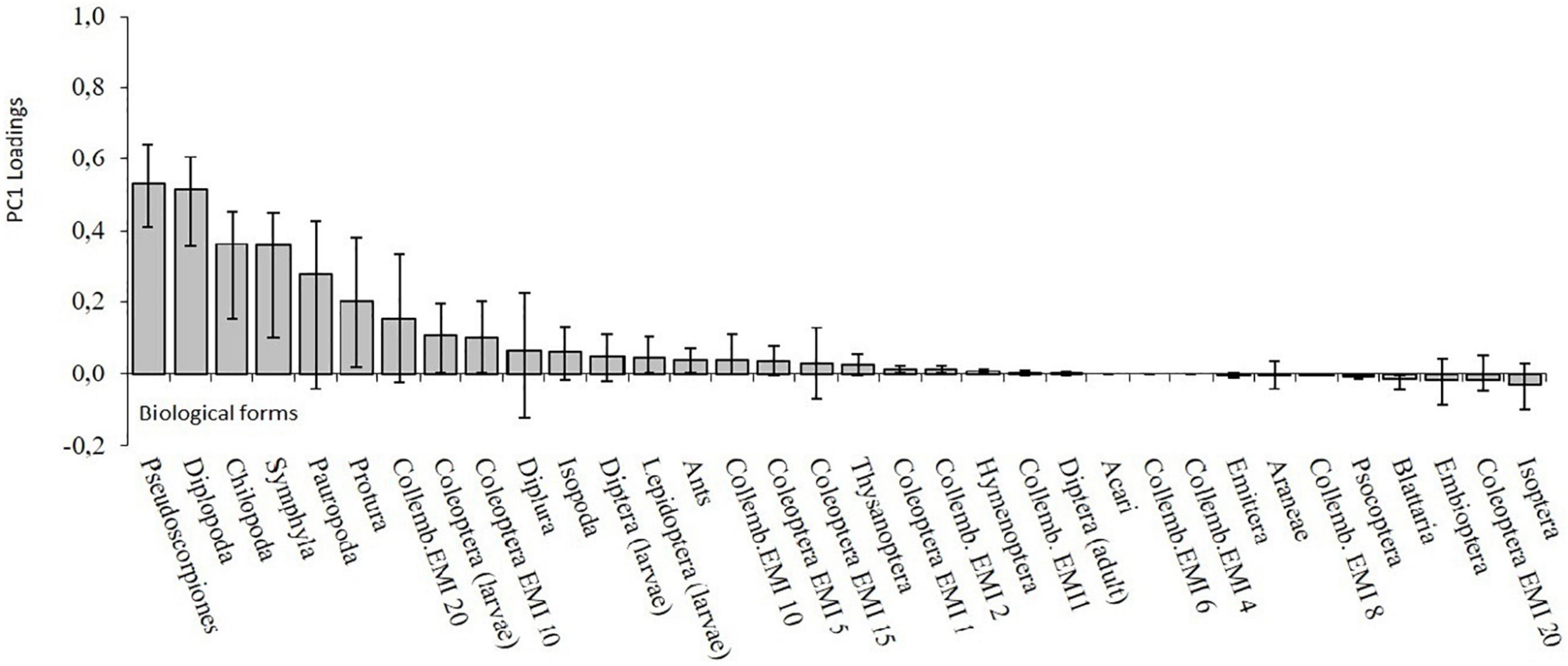
Figure 6. PC1 factor loadings. The line represents the 95% bootstrap confidential interval of coefficient loadings.
According to the NPMANOVA Test, the microarthropod community showed a clear difference between burned, especially for soils burned twice (C1 and C2), and never burned plots (F = 3.624, p < 0.001).
The statistical analysis on EMI data also revealed a change in edaphic soil quality among burned and never burned areas equal to 29.9% with a greatest difference in subarea C2 (32%). The SIMPER procedure showed that the edaphic groups that contribute most to this difference are Pseudoscorpions and Diplopods. In burned areas, there is a reduction in these two taxa which disappears in the twice-burned subarea C2 (Figure 7). This is in line with what we found in a previous study (Lisa et al., 2015) where the analysis of edaphic microarthropod abundance was used to monitor soil after fire.
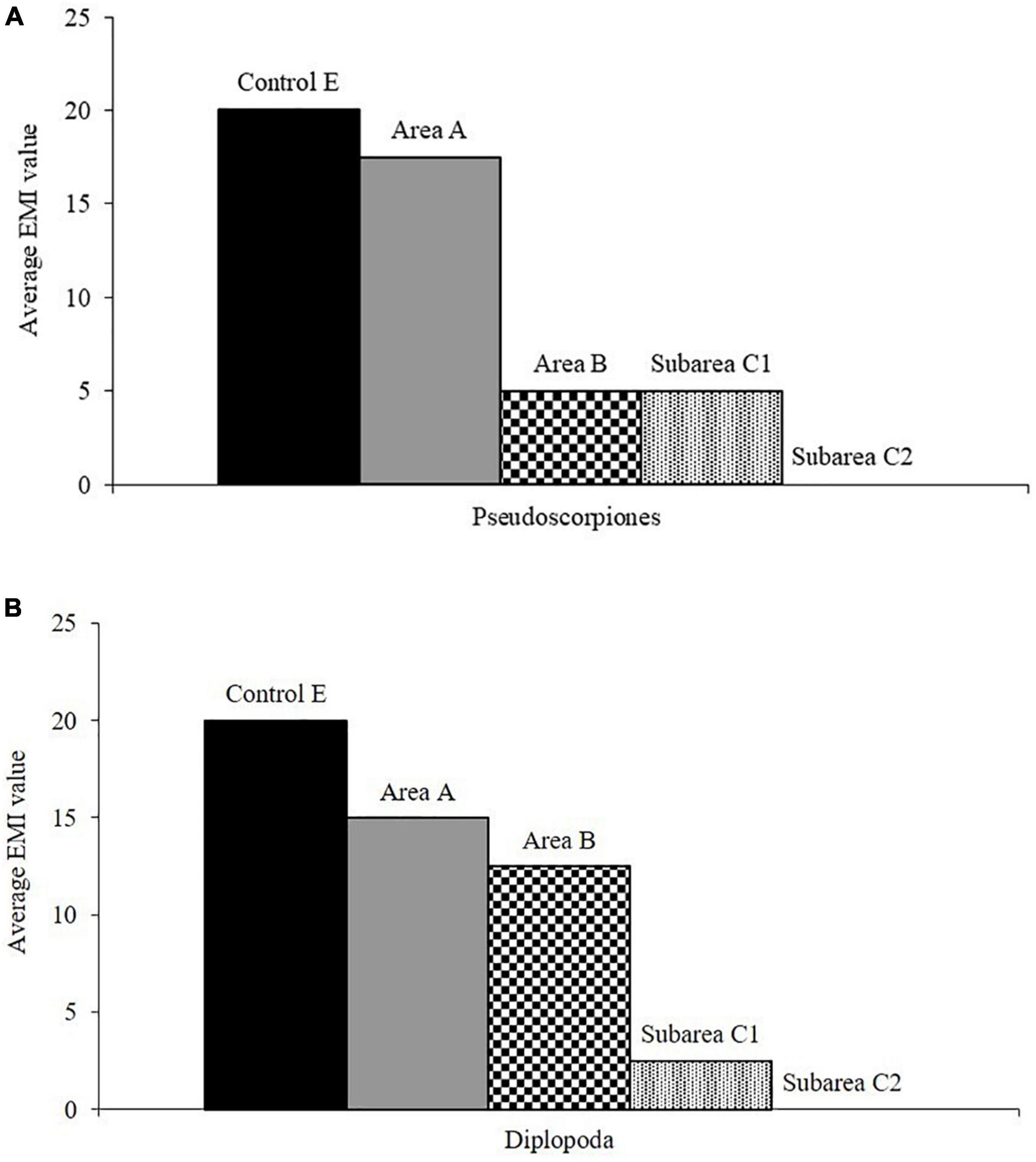
Figure 7. Average EMI values of taxonomic groups (A = Pseudoscorpiones; B = Diplopoda) with major contribution to dissimilarity for plot never burned (E) and areas burned (SIMPER procedure; cut off ≈ 70% EMI data).
In the short-term after fire (area D) the outcomes of multivariate PCA showed a clear separation between the soil biological quality groups before and after fires along PC1 (Figure 8), which explains 34% of total variance (eigenvalues 2.5%), whereas the PC2 explains a variance equal to 25% (total variances equal to 59%).
The soil samples taken in the Control area (E) are distributed in the positive axis of PC1 (right side, Figure 8), while the samples collected 3 and 5 months after the fire are in the negative axes of PC1. PC1 was related to the central role of Pseudoscorpiones and Diplopoda (Figure 9) while PC2 (25% of total variance) was mainly associated to the presence of euedaphic Collembola (EMI 20) and Symphyla.
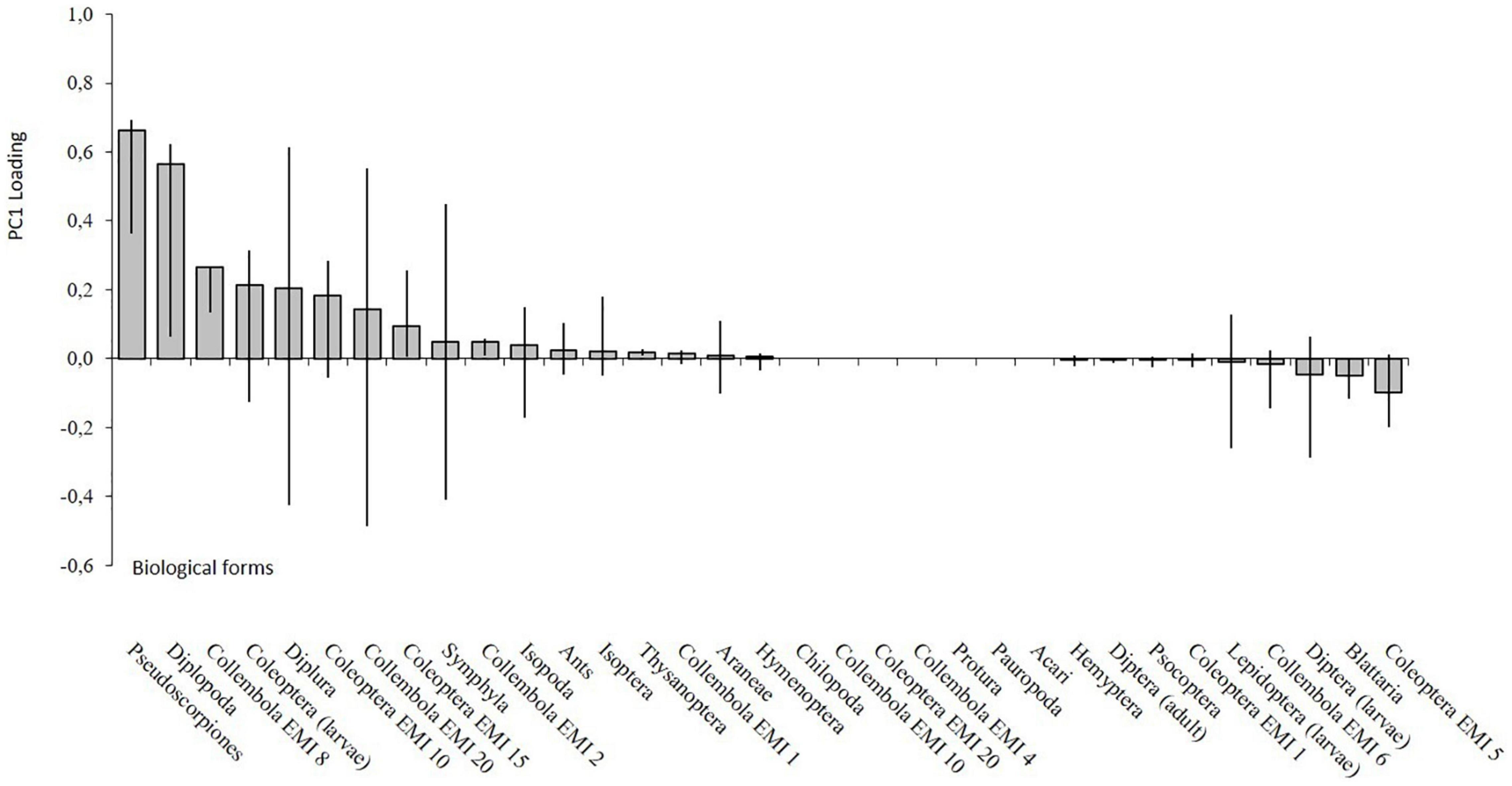
Figure 9. PC1 factor loadings. The line represents the 95% bootstrap confidential interval of coefficient loadings.
The NPMANOVA Test demonstrated significant differences (F = 10.9, p < 0.001) between the biological soil quality of the area never burned and the one examined in the different intervals of time after wildfire (area D). The SIMPER analysis showed that the highest average dissimilarity (25.32%) was obtained between the microarthropod edaphic communities in the Control area (E) and the one observed 3 months after the fire. The taxa that contributed more to this dissimilarity were Pseudoscorpiones, euedaphic Collembola, and Diplopoda. However, it is worth noting that the presence of euedaphic Collembola was observed in the soil 5 months after the fire, while Pseudoscorpiones and Diplopoda were still missing as also noted in the study of Lisa et al. (2015).
Discussion and Conclusion
Monitoring the effect of wildfire on forest ecosystems through biological indicators may provide critical information that can be difficult to obtain from experimental burns, since wildfires typically occur under more extreme conditions (Raymond and Peterson, 2005). Results of this type of investigation can be subject to various sources of uncertainty, due to the unpredictability of wildfire behavior, inaccurate or missing data, incomplete scientific understanding of the ecological response to fire (Thomson and Calkin, 2011) and the absence of replicas in other areas with similar fire conditions.
When more fires occur over time in the same forest area, soil biological activity, as well as the vegetation (Moreira et al., 2011), are affected more and there is also a mosaic effect (mosaic fire or patch-mosaic burning) (Brockett et al., 2001), with areas which are more damaged and others that are less impacted by direct and indirect effects of fire, as observed, e.g., in a study on carbon storage (Santana et al., 2016) or on forest bird communities richness (Wills et al., 2020).
Our previous work (Lisa et al., 2015) showed that repeated and frequent fires in the same area, i.e., two fires with an interval of 8 years, produced significant changes on abundance of some type of edaphic microarthropods. The QBS-ar index investigated in this paper shows a similar trend, the biological quality of the soil changes both in the medium-long-term and in the short-term after fire, with lower QBS-ar values found in the areas burned twice.
The PCA analysis revealed that two functional groups, Pseudoscorpiones and Diplopoda, reacted negatively to the passage of fire both in the short-term and 2 or 3 years after fire, and the absence of Pseudoscorpiones was more pronounced in the soil burned twice. From the viewpoint of biological diversity, Pseudoscorpiones and Diplopoda can be considered good bioindicators because their reduction is connected to soil deterioration (Blesic and Mitrovski, 2003) and these functional groups can be also considered valid bioindicators of burned soils because their reduction is strongly linked, directly or indirectly, to the passage of fire as reported in other studies (e.g., York, 1999, in Australian eucalypt forests; Gagan, 2002, in deciduous forests; Sileshi and Mafongoya, 2006, in miombo forests in Zambia; Gongalsky and Persson, 2013, in a Boreal forest; Lisa et al., 2015, in Mediterranean pine forests). Chilopoda, Simphyla, Pauropoda, and Protura, taxonomic groups typical of stable environments linked to undisturbed soil (Bedano et al., 2006; New, 2014) were more sensitive to fire, even if less relevant compared to the Pseudoscorpiones and Diplopoda.
As for the short-term after fire, the effect seems mainly direct and edaphic microarthropods were probably killed by fire and heat, rather than by indirect effects, as also observed by Malmström et al. (2008). In our case the absence of microarthropods like Pseudoscorpiones, euedaphic Collembola, and Diplopoda influenced biological soil quality.
Repeated and frequent fires in the same area (in this case, two fires over a period of 8 years) have produced significant changes in biological soil quality which is reflected in a significant reduction of the QBS-ar values as also observed in the study by Mantoni et al. (2020).
Our study also showed that Pseudoscorpiones and Diplopoda are the ecomorphological groups most sensitive to fire both in the medium to long term and in the short term after fire and could be considered valid bioindicators for future fire monitoring studies.
Results of our study showed that edaphic microarthropod communities return to the before fire situation only 11 years after the first fire, while in the short-term and 2 or 3 years after fire, the QBS-ar never reached values equal to 200. However, monitoring biological soil quality over time is necessary to confirm this trend.
Usually, soil quality monitoring is often inaccessible to land and forest managers because measurement systems are too complex, expensive or both (Herrick, 2000; Blasi et al., 2013). A widespread use of this type of monitoring would instead require “user friendly” indicators.
In this sense, the QBS-ar is a useful index, which in practice has proven more effective than monitoring only chemical or physical soil parameters or edaphic microarthropod abundances, which is usually more expensive and more time consuming. In the QBS-ar index estimation, scoring of Collembola and Coleoptera could be improved, but nevertheless, the QBS-ar index proved to be a dependable method to detect the effect of fire on soil quality in Mediterranean pine forests.
Biomonitoring and ecological indicators can quantify the magnitude of disturbance, the degree of exposure or ecological response to stresses (Dale and Beyeler, 2001), providing a simple and efficient method for the analysis of composition and function of complex ecological systems.
The QBS-ar index has shown valuable potential by providing useful information for management and rehabilitation of forest ecosystems impacted by fire, in an accessible, low cost and time efficient way. Its further development, combined with other innovative approaches (e.g., Fernández-García et al., 2021) could increase knowledge of post-fire forest dynamics in order to promote increasingly sustainable and informed management.
Data Availability Statement
The raw data supporting the conclusions of this article will be made available by the authors, without undue reservation.
Author Contributions
DT, SN, and EM contributed to conception and design of the study. DT, CL, and DP performed the field work. CL carried out the biological soil quality evaluation and wrote the first draft of the manuscript. CL and DP performed the statistical analysis. All authors contributed to manuscript revision, read, and approved the submitted version.
Conflict of Interest
The authors declare that the research was conducted in the absence of any commercial or financial relationships that could be construed as a potential conflict of interest.
Publisher’s Note
All claims expressed in this article are solely those of the authors and do not necessarily represent those of their affiliated organizations, or those of the publisher, the editors and the reviewers. Any product that may be evaluated in this article, or claim that may be made by its manufacturer, is not guaranteed or endorsed by the publisher.
Acknowledgments
We thank two reviewers and the editor for their valuable comments which helped us improve the earlier version of this manuscript.
References
Baeza, M. J., Valdecantos, A., Alloza, J. A., and Vallejo, V. R. (2007). Human disturbance and environmental factors as drivers of long-term post-fire regeneration patterns in Mediterranean forests. J. Veg. Sci. 18, 243–252. doi: 10.1111/j.1654-1103.2007.tb02535.x
Barreto, C., and Lindo, Z. (2022). Response of soil biodiversity to global change. Pedobiologia 90:150792. doi: 10.1016/j.pedobi.2022.150792
Bedano, J. C., Cantú, M. P., and Doucet, M. E. (2006). Soil Springtails (Hexapoda: Collembola), symphylans and pauropods (Arthropoda: Myriapoda) under different management systems in agroecosystems of the subhumid Pampa (Argentina). Eur. J. Soil Biol. 42, 107–119. doi: 10.1016/j.ejsobi.2005.11.004
Bezkorovainay, I., Krasnoshchekova, E., and Ivanova, G. (2007). Transformation of soil invertebrate complex after surface fires of different intensity. Biol. Bull. 34, 517–522. doi: 10.1134/S1062359007050159
Blasi, S., Menta, C., Balducci, L., Conti, F. D., Petrini, E., and Piovesan, G. (2013). Soil microarthropod communities from Mediterranean forest ecosystems in Central Italy under different disturbances. Environ. Monit. Assess. 185:16371655. doi: 10.1007/s10661-012-2657-2
Blesic, B., and Mitrovski, A. (2003). Investigation of soil arthropods in the Kragujeva Basin, Serbia. Acta Entomol. Serbica 8, 57–62.
Bonferroni, C. E. (1935). Il calcolo delle assicurazioni su gruppi di teste in Studi in onore del Professore Salvatore Ortu Carboni, (Bardi: Assistenza Pubblica), 13–60.
Bonferroni, C. E. (1936). Teoria statistica delle classi e calcolo delle probabilità” [Statistical theory of classes and probability]. Pubbl. R Ist. Sup. Sci. Econ. Commer. Fir. 8, 3–62.
Brockett, B., Biggs, H. C., and Van Wilgen, B. (2001). A patch mosaic burning system for conservation areas in southern African savannas. Int. J. Wildland Fire 10, 169–183. doi: 10.1071/WF01024
Broza, M., and Izhaki, I. (1997). Post-fire arthropod assemblages in Mediterranean forest soils in Israel. Int. J. Wildland Fire 7, 317–325. doi: 10.1071/WF9970317
Broza, M., Poliakov, D., Weber, S., and Izhaki, I. (1993). Soil microarthropods on post-fire pine forest on Mount Carmel, Israel. Water Sci. Technol. 27, 533–538. doi: 10.2166/wst.1993.0592
Caon, L., Vallejo, V. L., Coen, R. J., and Geissen, V. (2014). Effects of wildfire on soil nutrients in Mediterranean ecosystems. Earth-Sci. Rev. 139, 47–58. doi: 10.1016/j.earscirev.2014.09.001
Certini, G., Moya, D., Lucas-Borja, M. E., and Mastrolonardo, G. (2021). The impact of fire on soil-dwelling biota: a review. For. Ecol. Manag. 488:118989. doi: 10.1016/j.foreco.2021.118989
Clarke, K. R. (1993). Non-parametric multivariate analysis of changes in community structure. Aust. J. Ecol. 18, 117–143. doi: 10.1111/j.1442-9993.1993.tb00438.x
Dale, V. H., and Beyeler, S. C. (2001). Challenges in the development and use of ecological indicators. Ecol. Indicat. 1, 3–10. doi: 10.1016/S1470-160X(01)00003-6
Doran, J. W., and Parkin, T. B. (1994). “Defining and assessing soil quality,” in Defining soil quality for a sustainable environment, eds J. W. Doran, D. C. Coleman, D. F. Bezdicek, and B. A. Stewart (Madison: SSSA), 3–21. doi: 10.2136/sssaspecpub35.c1
Fernández-García, V., Marcos, E., Huerta, S., and Calvo, L. (2021). Soil-vegetation relationships in Mediterranean forests after fire. For. Ecosyst. 8:18. doi: 10.1186/s40663-021-00295-y
Firbank, L. G., Bertora, C., Blankman, D., Delle Vedove, G., Frenzel, M., et al. (2017). Towards the co-ordination of terrestrial ecosystem protocols across European research infrastructures. Ecol. Evol. 7, 3967–3975. doi: 10.1002/ece3.2997
Francos, M., Pereira, P., and Úbeda, X. (2020). Effect of pre- and post-wildfire management practices on plant recovery after a wildfire in Northeast Iberian Peninsula. J. For. Res. 31, 1647–1661. doi: 10.1007/s11676-019-00936-7
Gagan, A. B. (2002). The Effects of Prescribed Fire on Millipede and Salamander Populations in a Southern Appalachian Deciduous Forest. Electronic Theses and Dissertations. Nashville: Tennessee State University.
Galli, L., Capurro, M., Menta, C., and Rellini, I. (2014). Is the QBS-ar index a good tool to detect the soil quality in Mediterranean areas? A cork tree Quercus suber L. wood as a case of study. Italian J. Zool. 81, 126–135. doi: 10.1080/11250003.2013.875601
García-Ruiz, A. (2001). Estudio de los efectos de un incendio sobre las poblaciones de miriàpodos [Study of the effects of fire on populations of miriapodes]. Ecología 15, 269–273.
Gardi, C., Menta, C., and Leoni, A. (2008). Evaluation of environmental impact of agricultural management practices using soil microarthropods. Fresen. Environ. Bull. 17, 1165–1169. doi: 10.1016/j.scitotenv.2018.08.291
Gongalsky, K. B., and Persson, T. (2013). Recovery of soil macrofauna after wildfires in boreal forests. Soil Biol. Biochem. 57, 182–191. doi: 10.1016/j.soilbio.2012.07.005
Gulvik, M. E. (2007). Mites (Acari) as indicators of soil biodiversity and land use monitoring: a review. Polish J. Ecol. 55, 415–440.
Hammer, O., Harper, D. A. T., and Ryan, P. D. (2001). PAST: palaeontological statistics software package for education and data analysis. Palaeontologia Electronica 4, 1–9.
Heneghan, L., and Bolger, T. (1998). Soil microarthropod contribution to forest ecosystem processes: the importance of observational scale. Plant Soil 205, 113–124. doi: 10.1023/A:1004374912571
Herrick, J. E. (2000). Soil quality: an indicator of sustainable land management? Appl. Soil Ecol. 15, 73–83. doi: 10.1016/S0929-1393(00)00073-1
Jackson, D. A. (1993). Stopping rules in principal components analysis: a comparison of heuristical and statistical approaches. Ecology 74, 2204–2214. doi: 10.2307/1939574
Jiménez-Morillo, N. T., Almendros, G., DelaRosa, J. M., Jordán, A., Zavala, L. M., Granged, A. J. P., et al. (2020). Effect of a wildfire and of post-fire restoration actions in the organic matter structure in soil fractions. Sci. Total Environ. 728:138715. doi: 10.1016/j.scitotenv.2020.138715
Karlen, D. L., Andrews, S. S., Wienhold, B. J., and Zobeck, T. M. (2008). Soil quality assessment: past, present and future. Elect. J. Integrat. Biosci. 6, 3–14.
Karlen, D. L., Mausbach, M. J., Doran, J. W., Cline, R. G., Harris, R. F., and Schuman, G. E. (1997). Soil quality: a concept, definition, and framework for evaluation. Soil Sci. Soc. Am. J. 61, 4–10. doi: 10.2136/sssaj1997.03615995006100010001x
Keeley, J. E. (2009). Fire intensity, fire severity and burn severity: a brief review and suggested usage. Int. J. Wildland Fire 18, 116–126. doi: 10.1071/WF07049
Keeley, J. E., Bond, W. J., Bradstock, R. A., Pausas, J. G., and Rundel, W. (2012). Fire in Mediterranean Ecosystems. Cambridge: Cambridge University Press. doi: 10.1017/CBO9781139033091
Köppen, W. (1936). Das geographische System der Klimate, Handbuch der Klimatologie [The Geographical System of the Climate, Handbook of Climatology]. Berlin: Borntraeger.
Kruskal, W. H., and Wallis, W. A. (1952). Use of ranks in one-criterion variance analysis. J. Am. Stat. Assoc. 47, 583–621. doi: 10.1080/01621459.1952.10483441
Lakshmi, G., Okafor, B. N., and Visconti, D. (2020). “Soil microarthropods and nutrient cycling,” in Environment, climate, plant and vegetation growth, eds S. Fahad, M. Hasanuzzaman, M. Alam, H. Ullah, M. Saaed, I. Khan, et al. (Cham: Springer), 453–472. doi: 10.1007/978-3-030-49732-3_18
Lazzaro, L., Mazza, G., d’Errico, G., Fabiani, A., Giuliani, C., Inghilesi, A. F., et al. (2018). How ecosystems change following invasion by Robinia pseudoacacia: Insights from soil chemical properties and soil microbial, nematode, microarthropod and plant communities. Sci. Total Environ. 622, 1509–1518. doi: 10.1016/j.scitotenv.2017.10.017
Lisa, C., Paffetti, D., Nocentini, S., Marchi, E., Bottalico, F., Fiorentini, S., et al. (2015). Impact of wildfire on the edaphic microarthropod community in a Pinus pinaster forest in central Italy. iForest. Biogeosci. For. 8, 874–883. doi: 10.3832/ifor1404-008
Lobry de Bruyn, L. A. (1999). Ants as bioindicators of soil function in rural environments. Agricult. Ecosyst. Environ. 74, 425–441. doi: 10.1016/S0167-8809(99)00047-X
Luo, Z., Yiqi Luo, Y., Baldock, J., and Wang, E. (2017). Soil organic carbon dynamics jointly controlled by climate, carbon inputs, soil properties and soil carbon fractions. Glob. Chan. Biol. 23, 4430–4439. doi: 10.1111/gcb.13767
Lussenhop, J. (1992). Mechanism of microarthropods-microbial interaction in the soil. Adv. Ecol. Res. 23, 1–33. doi: 10.1016/S0065-2504(08)60145-2
Malmström, A., Persson, T., and Ahlström, K. (2008). Effects of fire intensity on survival and recovery of soil microarthropods after a clear-cut burning. Can. J. For. Res. 38, (9): 2465–2475. doi: 10.1139/X08-094
Mantoni, C., Di Musciano, M., and Fattorini, S. (2020). Use of microarthropods to evaluate the impact of fire on soil biological quality. J. Environ. Manag. 266:110624. doi: 10.1016/j.jenvman.2020.110624
Marshall, V. G. (2000). Impacts of forest harvesting on biological process in northern forest soils. For. Ecol. Manag. 133, 43–60. doi: 10.1016/S0378-1127(99)00297-2
Menta, C., Conti, F. D., Pinto, S., and Bodini, A. (2018). Soil biological quality index (QBS-ar): 15 years of application at global scale. Ecol. Indic. 85, 773–780. doi: 10.1016/j.ecolind.2017.11.030
Moreira, F., Viedma, O., Arianoutsou, M., Curt, T., Koutsias, N., Rigolot, E., et al. (2011). Landscape – wildfire interactions in southern Europe: implications for landscape management. J. Environ. Manag. 92, 2389–2402. doi: 10.1016/j.jenvman.2011.06.028
Nelson, D. W., and Sommers, L. E. (1996). “Total carbon, organic carbon, and organic matter,” in Methods of Soil Analysis: Part 3 - Chemical Methods, eds D. L. Sparks, A. L. Page, P. A. Helmke, R. H. Loeppert, P. N. Soltanpour, M. A. Tabatabai, et al. (Madison: Soil Science Society of America), 961–1010.
New, T. I. (2014). Insects, Fire and Conservation. Berlin: Springer International Publishing. doi: 10.1007/978-3-319-08096-3
Nocentini, S., Travaglini, D., and Muys, B. (2022). Managing mediterranean forests for multiple ecosystem services: research progress and knowledge gaps. Curr. For. Rep. 2022, 1–28. doi: 10.1007/s40725-022-00167-w
Olivari, S. (2004). “Morfologia, geologia e pedologia [Morphology, geology and soil science],” in La Riserva Naturale di Montefalcone: storia, ambiente e territorio, eds F. Cappelli, V. Cappelli, F. Fabbrizzi, S. Olivari, P. Piussi, M. Sbragia, et al. (Pisa: Tipografia La Grafica Pisana), 21–30.
Paoletti, M. G., and Hassal, M. (1999). Woodlice (Isopoda: Oniscidea): their potential for assessing sustainability and use as bioindicators. Agricult. Ecosyst. Environ. 74, 157–165. doi: 10.1016/s0167-8809(99)00035-3
Parisi, V. (2001). La qualità biologica del suolo. Un metodo basato sui microartropodi. Acta Naturalia de L’Ateneo Parmense 37, 97–106.
Parisi, V., Menta, C., Gardi, C., Jacomini, C., and Mozzanica, E. (2005). Microarthropod communities as a tool to assess soil quality and biodiversity: a new approach in Italy. Agricult. Ecosyst. Environ. 105, 323–333. doi: 10.1016/j.agee.2004.02.002
Pinto, S., Gatti, F., García-Montero, L. G., and Menta, C. (2017). Does soil fauna like truffles just as humans do? One-year study of biodiversity in natural brûlés of Tuber aestivum Vittad. Sci. Total Environ. 58, 1175–1184. doi: 10.1016/j.scitotenv.2017.01.181
Raymond, C. L., and Peterson, D. L. (2005). Fuel treatments alter the effects of wildfire in a mixed evergreen forest, Oregon, USA. Can. J. For. Res. 35, 2981–2995. doi: 10.1139/x05-206
Ryan, K. C. (2002). Dynamic interactions between forest structure and fire behavior in boreal ecosystems. Silva Fennica 36, 13–39. doi: 10.14214/sf.548
Ryan, K. C., and Noste, N. V. (1985). “Evaluating prescribed fires,” in ‘Proceedings, Symposium and Workshop on Wilderness Fire’, 15–18 November 1983, eds J. E. Lotan, B. M. Kilgore, Fischer, and R. W. Mutch (Missoula: USDA Forest Service), 230–238.
Santana, V. M., Gonzalez-Pelayo, O., Maia, P. A. A., Varela, T. M. E., Vallejo, V. R., et al. (2016). Effects of fire recurrence and different salvage logging techniques on carbon storage in Pinus pinaster forests from northern Portugal. Eur. J. For. Res. 135, 1107–1117. doi: 10.1007/s10342-016-0997-0
Saulnier, L., and Athias-Binche, F. (1986). Modalités de la cicatrisation des écosystème méditerranéens aprés incendie: cas de certains arthropodes du sol 2. Les myriapodes édaphiques [Methods of recovering of Mediterranean ecosystem after fire: the case of some soil arthropods 2. Soil Myriapods]. Vie Milieu 36, 191–204.
Sbragia, M. (2004). “Il clima,” in La Riserva Naturale di Montefalcone: storia, ambiente e territorio [The climate. In: The Montefalcone natural reserve: history, environment and territory], eds F. Cappelli, V. Cappelli, F. Fabbrizzi, S. Olivari, P. Piussi, M. Sbragia, et al. (Pisa: Tipografia La Grafica Pisana), 15–20. doi: 10.1371/journal.pone.0127277
Seastedt, T. R. (1984). The role of microarthropods in decomposition and mineralization process. Ann. Rev. Entomol. 29, 25–46. doi: 10.1146/annurev.en.29.010184.00032
Sgardelis, S. P., Pantis, J. D., Argyropoulou, M. D., and Stamou, G. P. (1995). Effects of fire on soil macroinvertebrates in a Mediterranean Phryganic ecosystem. Int. J. Wildland Fire 5, 113–121. doi: 10.1071/WF9950113
Sileshi, G., and Mafongoya, P. L. (2006). The Short-term Impact of Forest Fire on Soil Invertebrates in the Miombo. Biodivers. Conserv. 15, 3153–3160. doi: 10.1007/s10531-005-5411-z
Soong, J. L., Vandegehuchte, M. L., Horton, A. J., Nielsen, U. N., Denef, K. E., Shaw, A., et al. (2016). Soil microarthropods support ecosystem productivity and soil C accrual: Evidence from a litter decomposition study in the tallgrass prairie. Soil Biol. Biochem. 92, 230–238. doi: 10.1016/j.soilbio.2015.10.014
Striganova, B. R. (2000). Locomotory and trophic activity of invertebrates as a factor of soil structure formation. Eurasian Soil Sci. 33, 1094–1100.
Taboada, A., García-Llamas, P., Fernández-Guisuraga, J. M., and Calvo, L. (2021). Wildfires impact on ecosystem service delivery in fire-prone maritime pine-dominated forests. Ecosyst. Serv. 50:101334. doi: 10.1016/j.ecoser.2021.101334
Tajovský, K. (2002). “Soil macrofauna (Diplopoda, Chilopoda, Oniscidea) in a pine forest disturbed by wildfire,” in Proceedings of the 6th Central European Workshop on Soil Zoology: Studies on soil fauna in Central Europe, eds K. Tajovský, V. Balik, and V. Pižl (Ceské Budejovice), 227–232.
Testi, A., Fanelli, G., Crosti, R., Castigliani, V., and DAngeli, D. (2012). Characterizing river habitat quality using plant and animal bioindicators: a case study of tirino river (Abruzzo Region, Central Italy). Ecol. Indicat. 20, 24–33. doi: 10.1016/j.ecolind.2012.01.027
Thomson, M. P., and Calkin, D. E. (2011). Uncertainty and risk in wildland fire management: a review. J. Environ. Manag. 92, 1895–1909. doi: 10.1016/j.jenvman.2011.03.015
Trucchi, E., Pitzalis, M., Zapparoli, M., and Bologna, M. A. (2009). Short-term effects of canopy and surface fire on centipede (Chilopoda) communities in a semi natural Mediterranean forest. Entomol. Fennica 20, 129–138. doi: 10.33338/ef.84471
Turbé, A., DeToni, A., Benito, P., Lavelle, P., Ruiz, N., VanderPutten, W. H., et al. (2010). Soil biodiversity: functions, threats and tools for policy makers. Brussels: European Commission, DG Environment.
Turner, M. G., Hargrove, W. W., Gardner, R. H., and Romme, W. H. (1994). Effects of fire on landscape heterogeneity in Yellowstone National Park, Wyoming. J. Veg. Sci. 5, 731–742. doi: 10.2307/3235886
Wallwork, J. A. (1983). Oribatids in forest ecosystems. Ann. Rev. Entomol. 28, 109–130. doi: 10.1146/annurev.en.28.010183.000545
Wills, A. J., Liddelow, G., and Tunsell, V. (2020). Wildfire and fire mosaic effects on bird species richness and community composition in south-western Australia. Fire Ecol. 16:5. doi: 10.1186/s42408-019-0065-5
Yan, S., Singh, A. N., Fu, S., Liao, C., Wang, S., Li, Y., et al. (2012). A soil fauna index for assessing soil quality. Soil Biol. Biochem. 47, 158–165. doi: 10.1016/j.soilbio.2011.11.014
Keywords: microarthropod community, QBS-ar biological index, biomonitoring, forest fires, Pinus pinaster Aiton
Citation: Lisa C, Paffetti D, Marchi E, Nocentini S and Travaglini D (2022) Use of an Edaphic Microarthropod Index for Monitoring Wildfire Impact on Soil in Mediterranean Pine Forests. Front. For. Glob. Change 5:900247. doi: 10.3389/ffgc.2022.900247
Received: 20 March 2022; Accepted: 10 May 2022;
Published: 09 June 2022.
Edited by:
Leonor Calvo Galvan, Universidad de León, SpainReviewed by:
Cristina Fernández, Centro de Investigación Forestal de Lourizán, SpainDaniel Moya, University of Castilla-La Mancha, Spain
Copyright © 2022 Lisa, Paffetti, Marchi, Nocentini and Travaglini. This is an open-access article distributed under the terms of the Creative Commons Attribution License (CC BY). The use, distribution or reproduction in other forums is permitted, provided the original author(s) and the copyright owner(s) are credited and that the original publication in this journal is cited, in accordance with accepted academic practice. No use, distribution or reproduction is permitted which does not comply with these terms.
*Correspondence: Davide Travaglini, davide.travaglini@unifi.it