- Forest Insect Disturbance Ecology Laboratory, Department of Forest and Conservation Sciences, University of British Columbia, Vancouver, BC, Canada
Outbreaks of western spruce budworm (Choristoneura freemani Freeman) in Douglas-fir [Pseudotsuga menziesii (Mirb.) Franco] forests of western Canada have shifted northward in recent decades. Using forest health survey data collected during the previous century (1909 to 2011) to assess the pattern of range shifts, we found that outbreaks have rapidly shifted to higher latitudes and elevations while gradually retracting from the southern and western portions of its range. Given that the survival of western spruce budworm is affected by access to developing buds, we tested the prediction that this shift was associated with climate change-induced alterations in synchrony between budworm larval emergence and Douglas-fir bud development. We simulated the dates of larval emergence and host budburst annually from 1901 to 2011 using existing phenology models and long-term weather data. Over the last century, the timing of larval emergence in relation to budburst has converged toward optimal synchrony at higher latitudes and elevations, while diverging at lower latitudes and elevations. Shifting synchrony arises from differential responses to temperature by the insect and its host tree; both are reliant upon degree day accumulation, but Douglas-fir bud development is putatively dependent upon an additional photoperiodic threshold. Thus, the potential for warming to cause directional shifts in phenological matching between early season folivores and their host trees may be contingent upon the degree to which each depends on different components of their environments for their development.
Introduction
Herbivorous insects are highly sensitive to variations in climate (Bale et al., 2002; Deutsch et al., 2008; Pörtner and Farrell, 2008). Due to their short life cycles, reproductive potential, high mobility and physiological sensitivity to temperature, even modest changes in environmental conditions will result in rapid changes to their abundance and distribution. Climate change is expected to affect herbivorous insects through direct impacts to their development and survival, and/or indirect impacts mediated through trophic interactions and interactions with abiotic components of the environment (Ayres and Lombardero, 2000; Hughes, 2000; Weed et al., 2013; Simler-Williamson et al., 2019). Range shifts are arguably the most evident outcome of direct and indirect climate impacts to herbivorous insects, manifesting as both range expansions (e.g., Hickling et al., 2005; Safranyik et al., 2010; Jepsen et al., 2011) and range contractions (Wilson et al., 2005; Lombardo et al., 2022).
Range expansions by herbivorous insects can result in extensive impacts to ecosystem processes (Jepsen et al., 2013; Rasmann et al., 2014). This is particularly true for forest insects with irruptive dynamics characterized by episodic landscape-scale outbreaks (Jepsen et al., 2008; Safranyik et al., 2010; Howe et al., 2022). Climate change has facilitated range expansions by irruptive herbivores through amelioration of extreme cold events promoting overwinter survival (Jepsen et al., 2008; Safranyik et al., 2010), milder spring conditions that facilitate improved phenological matching (Miller-Rushing et al., 2010; Jepsen et al., 2011), warmer summers that enhance development, emergence and dispersal (Battisti et al., 2006; Sambaraju et al., 2019), and increased access to evolutionarily naïve host tree populations and species with reduced defensive capacities (Cudmore et al., 2010; Cullingham et al., 2011). Outbreaks by irruptive herbivores in newly invaded forests threaten a variety of values including wildlife habitat, water quantity and quality, timber supply (Safranyik et al., 2010), and in extreme cases may modify biome-scale biogeophysical processes such as carbon cycling and sequestration (Kurz et al., 2008).
The western spruce budworm (Choristoneura freemani Freeman) (Lepidoptera: Tortricidae) is an irruptive herbivore that feeds mainly on Douglas-fir [Pseudotsugae menziesii (Mirb.) Franco] (Volney, 1994; Nealis and Régnière, 2021). It is an early season folivore that preferentially feeds on the new foliage of its host trees. Budworms overwinter as second-instar larvae in hibernacula within tree crowns. They emerge in early May to late June and temporarily mine needles and buds until buds flush, after which they feed on the new needles of elongating shoots. Only after completely consuming new foliage will larvae feed on older needles (Shepherd, 1992; Nealis and Nault, 2005; Nealis and Régnière, 2009; Nealis, 2012). Severe or prolonged defoliation reduces tree growth and vigour and may result in the death of the top portion of the tree. Top-kill often leads to multiple leaders, stem deformation, predisposition to secondary insect attacks and wood-decaying fungi, or even death of the entire tree (Van Sickle et al., 1983; Alfaro and Maclauchlan, 1992).
The western spruce budworm is one of the most destructive and widely distributed defoliators in western North America, with periodic outbreaks occurring within Douglas-fir forests of Canada and the United States (Swetnam and Lynch, 1993; Volney, 1994; Campbell et al., 2006). In Canada, outbreaks historically occurred in southwestern British Columbia (BC), and in particular on southern Vancouver Island (Alfaro et al., 2018). However, since 1930, outbreaks have only occurred at higher latitudes and elevations in the Douglas-fir forests of the interior of BC (Campbell et al., 2006; Maclauchlan et al., 2006, 2018; Thomson and Benton, 2007), suggesting a shift in optimal conditions for budworm survival.
Thomson and Benton (2007) proposed that the absence of western spruce budworm outbreaks in the southwest portion of western Canada was due to a warming-induced loss of synchrony between the emergence of overwintering larvae and the initiation of budburst by Douglas-fir. However, Régnière and Nealis (2018) dismissed the role of variation in larval emergence-budburst synchrony as a significant source of mortality, claiming that any losses of early-emerging larvae would be offset by improved survival of later instars and greater fecundity of resultant adults. Instead, these authors (Nealis and Régnière, 2016; Régnière and Nealis, 2019) suggested that the lack of outbreaks in milder climates was a result of high rates of mortality among overwintering larvae due to resource exhaustion resulting from warmer temperatures during diapause, whereas the northward shift in outbreak populations had been favoured by improved phenological matching of later larval instars with new foliage prior to its lignification (Clancy et al., 1988; Nealis, 2012; Régnière and Nealis, 2018). By incorporating these relationships into a process-based model, Régnière and Nealis (2019) simulated changes in western spruce budworm population fitness through time. Contrary to the observed changes in the spatial pattern of outbreaks, they suggested that the fitness of western spruce budworm populations had actually increased in all regions of BC since 1900, and that it was low in the southwest early in the previous century when outbreaks were common there. This seeming contradiction suggests that the potential impact of variation in synchrony between budburst and larval emergence on the spatial changes in western spruce budworm outbreaks requires further investigation.
Synchrony between the emergence of feeding life stages and the onset of bud development by host trees is critical to the survival of many early season insect folivores, as leaves are easiest to eat and are at their peak nutritional value at this time (Quiring, 1992; Carroll, 1999; Visser and Holleman, 2001; Carroll and Quiring, 2003; van Asch and Visser, 2007), or buds have developed sufficiently to allow penetration by young larvae (Shepherd, 1992; Nealis and Régnière, 2009; Nealis, 2012). This is true for the western spruce budworm. Emergence by overwintering second-instar larvae approximately 18 days in advance of the onset of Douglas-fir budburst has been shown to be optimal for survival (Beckwith and Burnell, 1982; Thomson et al., 1984; Thomson and Benton, 2007). Although early emergence by young larvae necessitates feeding on potentially suboptimal resources (old needles and closed buds), it maximizes access to newly flushed foliage by later instars that are more dependent upon a high-quality diet to complete development (Lawrence et al., 1997; Nealis, 2012; Régnière and Nealis, 2018). The degree of synchrony, and therefore budworm survival, can vary from year to year due to differential responses by insects and host trees to variations in temperature and precipitation (Shepherd, 1992; Bylund, 1999; Campbell et al., 2006) or defoliation (Régnière and Nealis, 2018). Annual weather-related variations in the degree of synchrony suggest that the interaction between western spruce budworm and Douglas-fir may be very sensitive to climate change. Therefore, we predicted that the northward range shift of western spruce budworm defoliation is a consequence of climate change-induced shift in the regions with optimal synchrony between spring larval emergence and Douglas-fir budburst. If this prediction is correct, we would expect to observe a shift in the mean outbreak range of western spruce budworm toward higher latitudes and elevations, along with a similar shift in the optimal synchrony between larval emergence and Douglas-fir budburst.
Materials and Methods
An analysis of the distribution of western spruce budworm outbreaks was conducted to quantify patterns of range shift within BC during the past century. Our prediction of a northward shift of optimal synchrony was evaluated in a second analysis where the dates of budworm emergence and Douglas-fir budburst were simulated across BC for the same period using budworm and host phenology models combined with long-term daily weather data. The following sections describe the data sources, the methods used to extract the relevant data, and the statistical analyses that were employed.
Infestation Data
Forest health data for the province of BC have been collected since the beginning of the 20th century. The history of forest health surveys in BC were described in detail by Harris et al. (1985) and Van Sickle et al. (2001). Briefly, surveys were performed by the Canadian Forest Service from 1909 to 1995. Starting in 1998, assessments were conducted by the BC Ministry of Forests, Lands, Natural Resource Operations and Rural Development (BC FLNRORD). Before 1949, boundaries of forest insect infestations were estimated during outbreaks via ad hoc ground surveys. From 1949 to 1959, systematic annual ground surveys were conducted with occasional support from observers in aircraft. By the 1960s, annual surveys of the entire province were conducted by observers in aircraft followed by ground truthing to confirm species identification. These efforts have resulted in a nearly continuous dataset comprising the location, extent and severity of impacts by forest pests for over a century throughout BC.
Like most irruptive defoliators, western spruce budworm populations fluctuate between extreme levels. At high densities, many thousands of larvae occur on a single mature tree, whereas at sub-outbreak levels, there may be only a few individuals (e.g., Royama, 1984, 1992). Therefore, only outbreak populations cause sufficient defoliation to be detected and mapped. To assess patterns of range shift by the western spruce budworm within BC, we used the forest health survey data indicating the location of outbreak populations from 1909 to 2011 (BC Ministry of Forests Lands, Natural Resource Operations and Rural Development, 2022a).
Landscape Modeling of Budburst and Larval Emergence Phenology
For trees in seasonal environments, the timing of bud development has evolved as a trade-off between maximizing growth resources while minimizing the risk of damage by late frosts (Saxe et al., 2001). The physiological processes associated with bud development prior to flushing are difficult to observe and not well known, but they are assumed to be a function of temperature accumulation following a chilling requirement (reviewed by Linkosalo et al., 2006; Caffarra and Donnelly, 2011). The most common and widely used models to predict developmental phenology by trees in seasonal environments are based on temperature accumulation after a fixed starting date in late winter or early spring [i.e., thermal-time models (Kramer, 1994; Linkosalo et al., 2006)]. The fixed date to begin temperature accumulation is hypothesized to arise from the requirement for a critical signal from the light environment such as night or day length (i.e., photoperiod) to begin development (Linkosalo et al., 2006)]. Thomson and Moncrieff (1982) developed a simple thermal-time model for Douglas-fir bud burst (defined as the average date at which buds on all trees in a given stand first open to expose green needles) based on the accumulation of 318 degree-days above 2.78°C beginning on March 12. The model was validated against a long-term data set and shown to predict the average date of budburst to within 1 day in 80% of years (Thomson and Moncrieff, 1982).
Like most insects in seasonal environments (reviewed by Rebaudo and Rabhi, 2018), the timing of western spruce budworm larval emergence in the spring is a direct function of temperature accumulation. We used a degree-day model developed by McMorran (1973) and Thomson et al. (1984) where the initiation of second-instar larval emergence from overwintering hibernaculae begins after the accumulation of 116 degree-days above a threshold of 5.5°C. While linear degree-day models of developmental rate may ignore the physiological responses of insects to temperature extremes, simpler functions may provide better descriptions of development over relatively narrow thermal ranges (Quinn, 2017).
Given differences in the underlying response mechanisms that determine the phenology of each organism (Chen et al., 2003; Thomson and Benton, 2007; van Asch and Visser, 2007), we extended the finer scale analysis by Thomson and Benton (2007) to consider historical phenological synchrony between the western spruce budworm and its host across the range of Douglas-fir in western Canada. We combined both phenology models, along with long-term weather data, within BioSIM (Régnière, 1996; Régnière et al., 2013) to calculate an estimate of the date of initial budworm larval emergence and average Douglas-fir budburst each year from 1901 to 2011, as well as to calculate the relative differences between larval emergence and budburst dates across BC. BioSIM is a software package created for landscape-wide projections of temperature-driven simulation models in pest management (Régnière, 1996; Régnière et al., 2013). There are four important components to the BioSIM software package: (i) weather databases; (ii) models that allow for a large number of simulations to be run while model parameters may be systematically varied based on the user’s needs; (iii) analytical tools for creating graphs, summary tables, and fitted regression equations; and (iv) interpolation methods that use digital elevation models (DEMs) to generate maps of the modeled events, which then can be used for further geographical information systems (GIS) analyses (Régnière and Logan, 1996; Régnière et al., 2013).
We entered the degree-day and threshold information for the budworm and host into the Reverse Degree Day (Annual) model provided in BioSIM to calculate the Julian date that the summation of degree-days is reached each year for first larval emergence and host budburst based on the methods described by Thomson and Benton (2007). This method assumes that the daily temperature cycle takes the form of a sine curve using the maximum and minimum temperatures from each day, with the accumulation of degree-days occurring under the curve between the upper and lower thresholds. The simulation of the Reverse Degree Day (Annual) model was conducted with 3,000 randomly selected points throughout BC generated by BioSIM. When the simulation was executed, BioSIM selected the “best” sources of weather data (i.e., the eight nearest georeferenced weather stations) for each location, while adjusting for variation in elevation, latitude, and longitude.
The two outputs, one containing the yearly dates of larval emergence at each location and the other the host budburst dates, were merged into a single output of the absolute difference between the Julian date of Douglas-fir budburst and the Julian date of western spruce budworm larval emergence. In BioSIM, landscape-level maps of BC were created for every year from 1901 to 2011 that displayed a raster grid of pixels with associated absolute difference between the Julian dates of Douglas-fir budburst and budworm larval emergence. The maps were produced using the universal kriging method on the data associated with the 3,000 location points in the final merged output mentioned above, along with elevation as a drift variable and the BC30s DEM within BioSIM (Régnière et al., 2013). The maps were exported for use in GIS analyses.
GIS Analyses
Range Shifts of Western Spruce Budworm Outbreaks
To examine the pattern of range shifts by the western spruce budworm, the annual aerial overview surveys of outbreaks were imported into a GIS, ArcMap™ 10.1®. The infestation data in our study comprised vector geographical features in the form of polygons of affected areas recorded during surveys, with each polygon representing an infestation. Attribute information included with individual polygons contained location information, tree species affected, and the area of the polygon. The years 1996 through 1999 were excluded as these were years during which data for the entire province of BC were not available. The final data set included 99 layers of western spruce budworm infestation polygons [mean (± SE) polygon area = 5.55 ± 0.18 km2] one for each year from 1909 through 1995 and 2000 through 2011.
To facilitate assessment of changes in the annual location of infestations, centroids were calculated for each polygon. This was done by first determining the latitude and longitude of the centers of the polygons and adding them to the attribute tables using ArcMap’s Calculate Geometry tool. Centroids were then calculated with the Make XY Event Layer tool and added to the map as a new layer containing point features that symbolized the centers of infestation polygons each year. Hopkin’s Bioclimatic Law (e.g., Hopkins, 1919; Liang, 2016; Richardson et al., 2019) states that phenological events in temperate North America are altered by approximately 4 days for every 122 m gain in elevation due to atmospheric lapse rates. Thus, to equate centroids from equivalent latitudes, but different elevations, we calculated the “effective latitude” [Latitude + (Elevation/122 m)] of each centroid and added those values to the attribute tables. Elevation was obtained from a 30 arc-second DEM of North America (U.S. Geological Survey’s Center for Earth Resources Observation and Science, 2010). The attribute tables for each year containing effective latitude and other information for every polygon were then exported to a spreadsheet program. A total of 19,351 observations (centroids) were available for subsequent statistical analyses.
Changes in Synchrony of Larval Emergence and Host Budburst
To quantify temporal changes in the synchrony of larval emergence and Douglas-fir budburst across BC, the vector polygon data output by BioSIM were imported into ArcMap. The attributes of each of these polygons included the year and a value representing the absolute difference between Douglas-fir budburst date and budworm larval emergence date. Each layer, from 1901 through 2011, was clipped (i.e., restricted) to correspond to the approximate range of Douglas-fir as identified by the 2015 provincial forest inventory (BC Ministry of Forests Lands, Natural Resource Operations and Rural Development, 2022b), as the possibility of western spruce budworm emergence timing outside of the range of its host was not relevant to the study. The polygon centroids and the effective latitude of the centroids were calculated and added to the map using the methods described above.
Statistical Analyses
Two statistical analyses were conducted—one to assess the patterns of range shifts of western spruce budworm outbreaks, and another to test for annual changes in the synchrony of western spruce budworm larval emergence and Douglas-fir budburst from the BioSIM data. The R statistical software package (version 3.0.2) was used for all analyses (R Core Team, 2014).
Patterns of Range Shifts
When ecological constraints such as temperature accumulation requirements act on an organism, the effects of the constraints may not be best represented by changes in the mean of a response variable (Cade et al., 1999; Cade and Noon, 2003). Quantile regression (Koenker and Bassett, 1978) fits conditional quantiles of the response with a general linear model that assumes no parametric form for the conditional distribution of the response. It estimates multiple rates of change (slopes) from the minimum to maximum response, providing a more complete picture of the relationships between variables missed by other regression methods (Cade and Noon, 2003). To assess the potential impact of a warming environment on a shift in the distribution of western spruce budworm outbreaks to the north and toward more continental conditions, we considered latitude and longitude quantiles, respectively, in relation to year. We chose the 5th (τ = 0.05) and 95th (τ = 0.95) quantiles to represent the trailing and leading edges of the distribution, as well as the 50th quantile (i.e., the median, τ = 0.50) to assess any shift in the overall distribution of western spruce budworm outbreaks through time. Quantile regressions were performed using the Quantreg library for R (Koenker, 2006; R Core Team, 2014).
Larval Emergence and Host Budburst Synchrony
Annual changes in the synchrony of western spruce budworm larval emergence and Douglas-fir budburst were assessed by analyzing the difference in Julian date between predicted average budburst and predicted larval emergence at each point over time. Due to the large number of observations, trends could not be identified in the raw data. However, by grouping the data into categories based on effective latitude, the noise in the data could be reduced and trends made apparent. The dataset was divided into bin sizes of 5° effective latitude, which ranged from 48° to 82° [representing the distribution of Douglas-fir in BC; 5–2,410 m elevation and 48.4–53.2° latitude (533 km)]. Within each bin, the annual averages were calculated. The number of days above or below optimal synchrony (i.e., larval emergence 18 days ahead of mean budburst) was then computed for each of the data values. Linear regressions were used to determine changes in average annual larval emergence-budburst synchrony (avgDayDiff) through time for each of the effective latitude bins using the model: avgDayDiff = β0 + β1(Year) + εi.
Results
Patterns of Range Shifts
As suggested by others (Thomson and Benton, 2007; Maclauchlan et al., 2018), there has been an obvious shift in western spruce budworm outbreak areas through time in western Canada (Figure 1). Before 1960, outbreaks were restricted to the southwest portion of the province of BC, including large areas of infestation on southern Vancouver Island. After 1960, outbreaks have occurred exclusively in the interior of the province, with an apparent shift to the north and east during the two most recent outbreak periods.
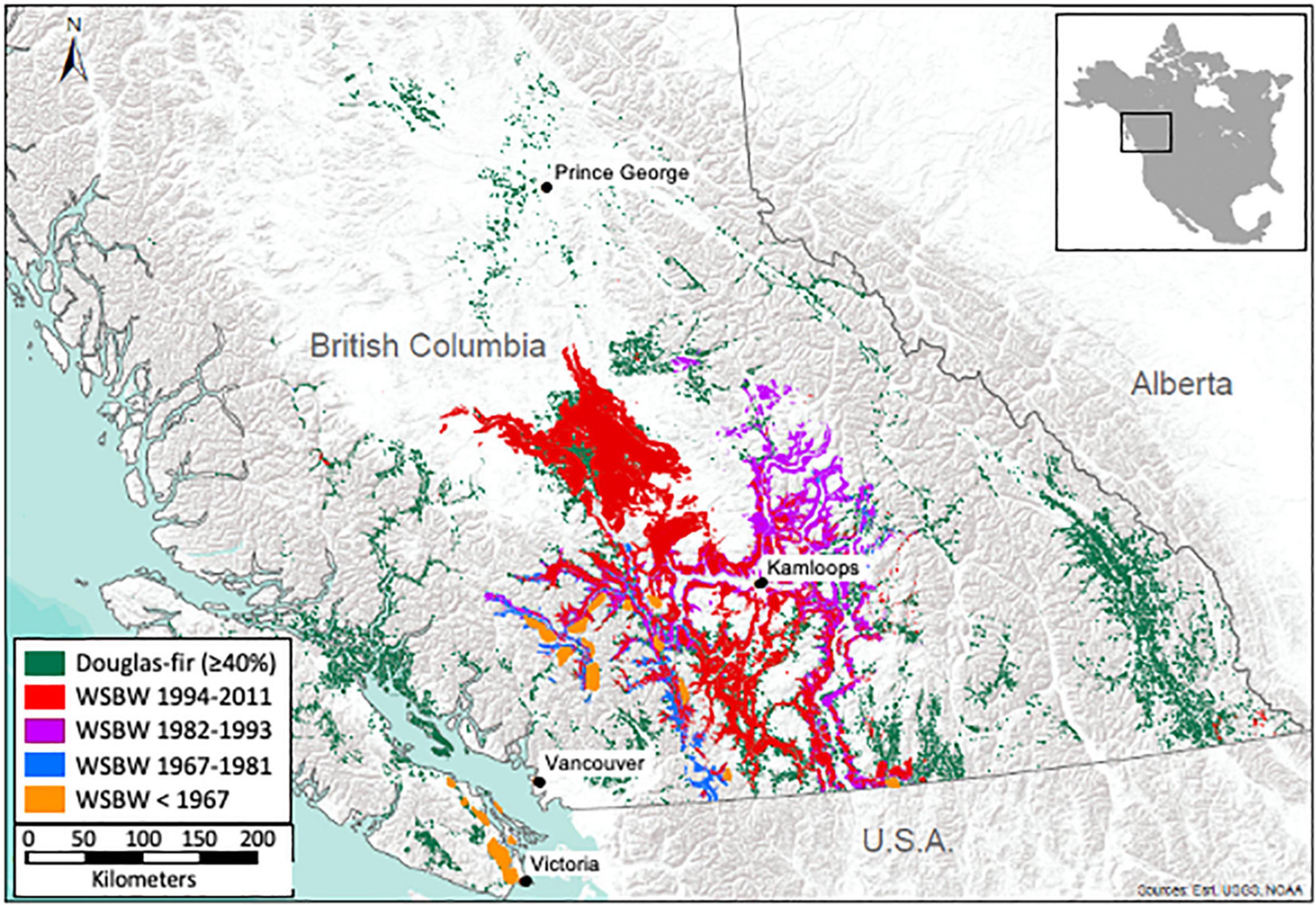
Figure 1. Distribution of western spruce budworm (WSBW) outbreaks from 1909 to 2011 in relation to its primary host, Douglas-fir, in British Columbia, Canada. Only polygons comprising ≥ 40% Douglas-fir are depicted. Western spruce budworm infestations are grouped into four periods to facilitate visualization of range shifts over time. The location of infestations was determined by ground observations from 1909 to 1960 and from aerial surveys thereafter. Data were provided by the BC Ministry of Forests, Lands, Natural Resource Operations and Rural Development.
Interestingly, the direction and rate of change in the distribution of western spruce budworm outbreaks was not consistent throughout the range of Douglas-fir. The median (0.50 quantile) outbreak distribution has steadily moved north accompanied by a retraction in the south at a similar rate as indicated by the 5th quantile (Figure 2A and Table 1). By contrast, the leading edge of the budworm’s distribution (95th quantile) has shifted at a high rate toward the north throughout the previous 100 years. Indeed, high rates of northern expansion have occurred among all quantiles greater than the median throughout the period of consideration (Figure 2B).
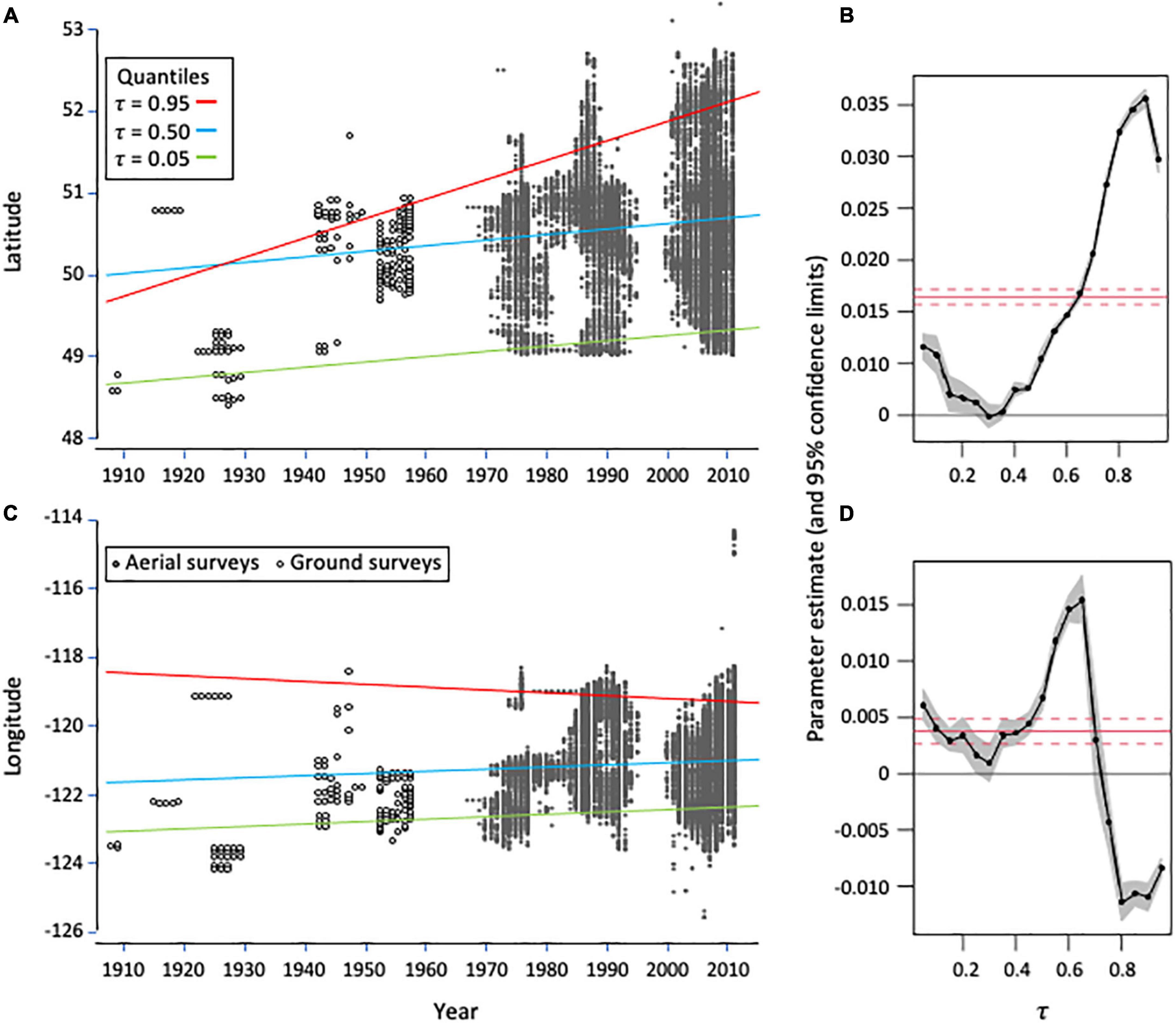
Figure 2. Quantile regression analyses examining the relationships between year and (A) latitude and (C) longitude of outbreak infestation centroids of the western spruce budworm, Choristoneura freemani, in British Columbia (BC), Canada from 1909 to 2011. Regressions were fit to the 5th (τ = 0.05), median (τ = 0.50) and 95th (τ = 0.95) quantiles. Estimates of the slope of the quantile linear regressions for latitude (B) and longitude (D) as a function of τ (black line, 95% confidence intervals in gray) are also presented. The ordinary least squares coefficients are shown in panels (B,D) as solid red lines with their confidence intervals as dotted lines. The location of infestations was determined by ground observations from 1909 to 1960 and from aerial surveys thereafter. Data were provided by the BC Ministry of Forests, Lands, Natural Resource Operations and Rural Development.
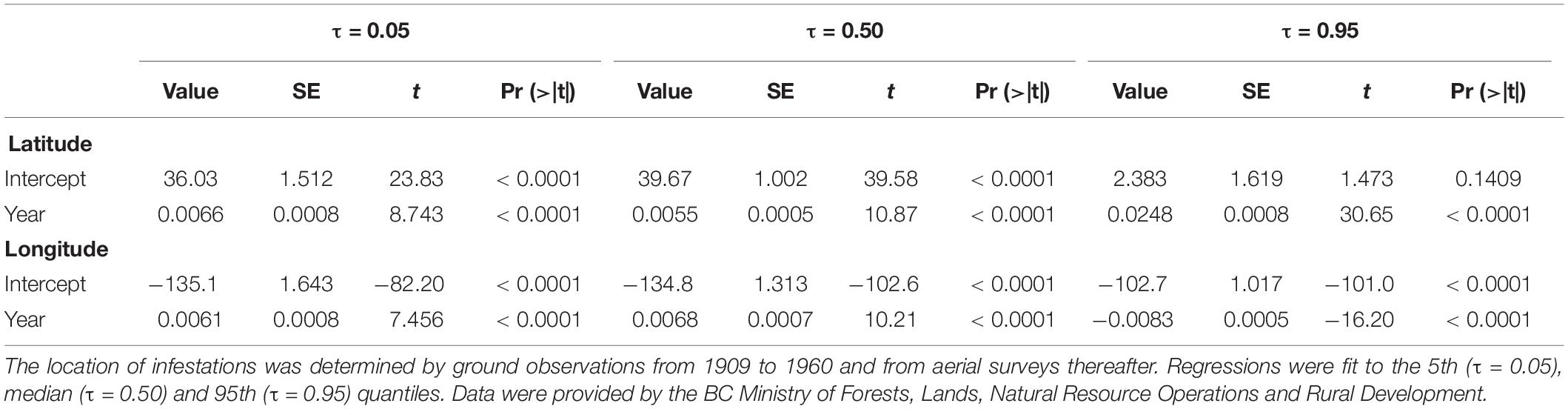
Table 1. Quantile regression analyses examining the relationships between year and the latitude and longitude of outbreak infestation centroids of the western spruce budworm, Choristoneura freemani, in British Columbia (BC), Canada.
Longitudinal changes in the distribution of western spruce budworm were less clear. Both the median and 5th quantiles tended to shift eastward (Figure 2C and Table 1). However, populations at the 95th quantile tended to shift back toward the west. This is likely a consequence of the constrained distribution of Douglas-fir in the northern portions of its range (Figure 1). The highest rates of eastern shifts occurred near the median, whereas relatively high rates of western shifts occurred among the quantiles ≥ 0.80 (Figure 2D).
Phenological Synchrony
Examination of the relationships between average annual larval emergence-budburst synchrony and time along an elevation/latitude gradient revealed an interesting trend (Figure 3). At higher elevations/latitudes (63° to 82° effective latitude), the phenology of larval emergence converged toward optimal timing through the previous century, suggesting improving conditions for the budworm. Indeed, at the highest latitudes/elevations, average asynchrony has declined from approximately 10 days at the start of the previous century to 5 days in recent years. At intermediate effective latitudes (between 58° and 62°), the timing of larval emergence was distributed evenly around optimal synchrony and showed no trend through time. At lower elevation/latitudes, however, synchrony diverged suggesting these areas have gradually become less suitable for western spruce budworm. At the lowest effective latitudes 100 years ago, mean synchrony was optimal, but larval emergence has become increasingly asynchronous through time reaching approximately 7 days in recent years.
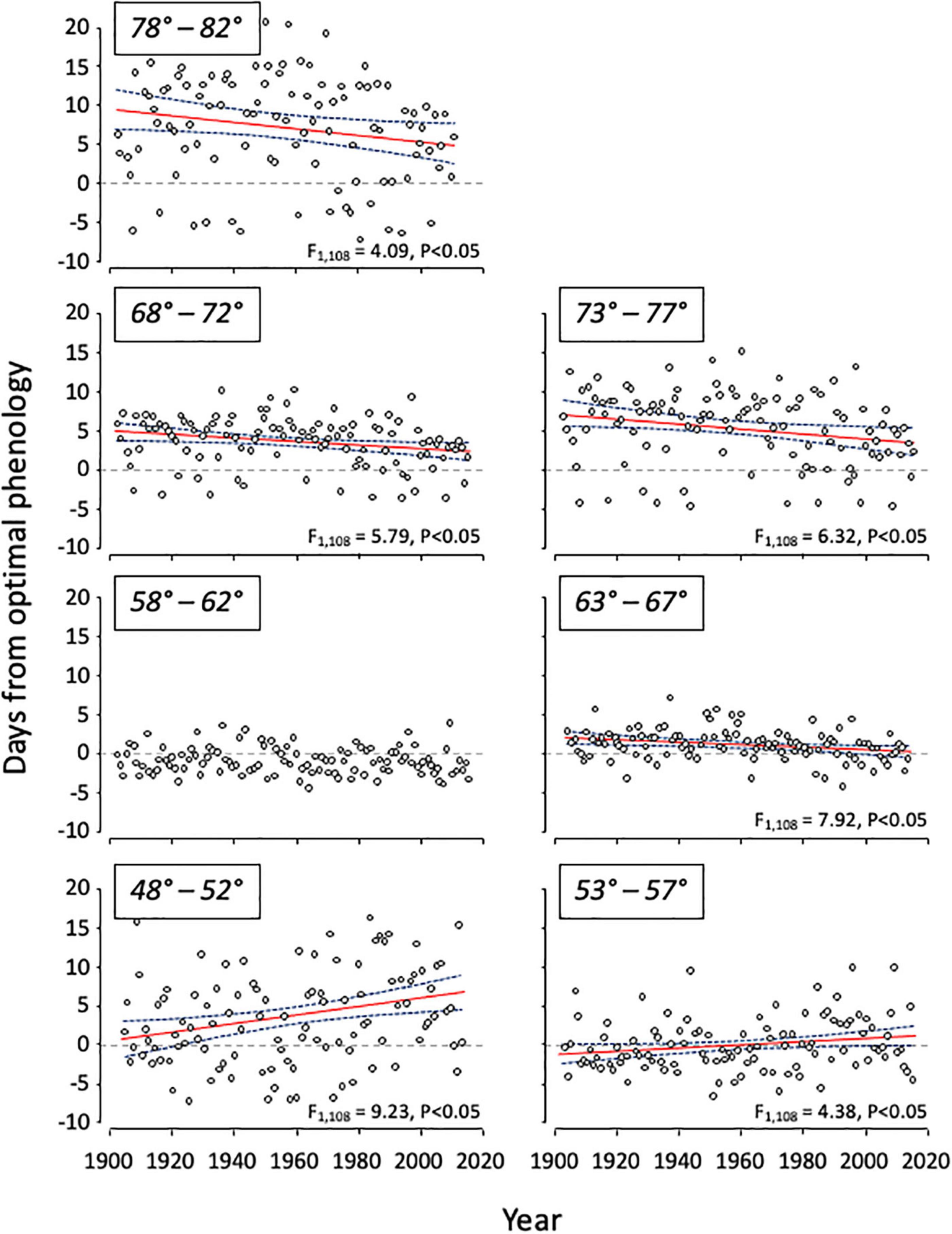
Figure 3. Linear regressions, with 95% confidence intervals, of deviation from optimal phenology (i.e., predicted difference between dates of Douglas-fir budburst and larval emergence) versus year for the western spruce budworm in British Columbia, Canada. Data were binned into equal intervals of 5° effective latitude, and annual averages were calculated within bins, with sample size of 108 in each bin.
Discussion
Western spruce budworm outbreaks in Douglas-fir forests of western Canada have rapidly shifted to higher latitudes and elevations while gradually retracting from the southern and western portions of its range since the beginning of the previous century. Evidence of this is clear in the spatial pattern of outbreaks detected during forest health surveys, and as predicted, in shifts in the timing of optimal budburst-larval emergence synchrony. Our results do not suggest that western spruce budworm populations have never occurred in northern portion of the Douglas-fir distribution, nor completely disappeared from the south. Indeed, several dendrochronological studies have found evidence of western spruce budworm activity throughout the range of Douglas-fir (Swetnam and Lynch, 1989, 1993; Campbell et al., 2006; Axelson et al., 2015). However, our analyses indicate that the propensity for populations to increase to detectable outbreak levels has shifted to higher latitudes and elevations during the previous 100 + years. Dendrochronology is a correlative technique that detects periods during which non-climate related events such as herbivorous insect activity may affect tree growth. It cannot be used to estimate herbivorous insect population levels (Swetnam and Lynch, 1993), and therefore may record defoliation events that fall below the level of detectability by forest health surveys. In support of this assertion, Campbell et al. (2006) found evidence for two western spruce budworm defoliation events in central BC, yet only the most recent one in their study, occurring between 1985 and 1995, was detected. Similarly, Alfaro et al. (2018) detected evidence of western spruce budworm defoliation of Douglas-fir on Vancouver Island in decades after 1930 when the last outbreak was recorded there.
Our analyses suggest a clear relationship between northward range expansion of the western spruce budworm and climate-change induced improvements in phenological synchrony. At higher latitudes/elevations, there was a consistent trend toward convergence in the timing of larval emergence and the initiation of host bud development over the previous century. At the beginning of our simulation (1900) the phenological mismatch was 7 to 10 days in the Douglas-fir forests at the highest latitude/elevations. A mismatch of this magnitude can cause significant amounts of mortality and limit the potential for outbreaks by early season folivores due to declines in the quality of foliage (Carroll, 1999; Jepsen et al., 2011; Fuentealba et al., 2017; Despland, 2018). However, through the previous century, the phenological mismatch decreased to < 5 days even in the most northerly Douglas-fir habitats. Improved synchrony may facilitate better access to the more nutritious and digestible new foliage leading to improved western spruce budworm development and survival (Shepherd, 1992; Chen et al., 2001, 2003), although evidence suggests that the requirement for phenological synchrony by western spruce budworm is a function of the physical state and not the nutritional condition of developing buds (Nealis and Nault, 2005; Nealis and Régnière, 2009; Nealis, 2012).
The results of this study largely complement rather than contradict our understanding of the spatial dynamics of western spruce budworm outbreaks. Variation in optimal synchrony is expected to affect the survival while foraging of newly emerged second-instar larvae following winter diapause (Shepherd, 1992). This assertion is in keeping with evidence that overall generation survival in populations of western spruce budworm is most affected by mortality of young, non-feeding larvae (Nealis and Régnière, 2021). Whereas Régnière and Nealis (2019) suggested low population fitness in lower latitudes/elevations at the beginning of the previous century, in part due to high levels of resource depletion by overwintering larvae, we found larval emergence-budburst synchrony to be optimal in that region at that time suggesting increased survival of newly emerged larvae while foraging for suitable buds may offset mortality from winter exhaustion. The evidence of widespread outbreaks in the southwest at this time support this conclusion. The expansion toward the north and higher elevations by outbreak populations is both in keeping with the simulations by Régnière and Nealis (2019) and our assessments of optimal synchrony. Indeed, the very high rates of northward expansion by populations above the median distribution suggests that budworms may be benefiting from both the phenological matching of later larval instars with new foliage prior to lignification (Nealis and Régnière, 2016; Régnière and Nealis, 2019) and our finding of the convergence toward optimal of larval emergence and bud development. Unfortunately, the overall northward shift in optimal synchrony, and the concomitant change in western spruce budworm outbreak distributions, does not entirely support the conclusion of Alfaro et al. (2018) that the disappearance of outbreaks at lower latitudes and elevations is a consequence of localized fragmentation of Douglas-fir forests.
In seasonal environments, phenological asynchrony has been suggested as an effective defense against herbivory for some populations and species of trees (Chen et al., 2003; Tikkanen and Julkunen-Tiitto, 2003; Pureswaran et al., 2015; Bellemin-Noël et al., 2021). Indeed, for Douglas-fir, resistance to defoliation by western spruce budworm has been linked to delays in the timing of budburst (Chen et al., 2001, 2003). Altered synchrony as a consequence of climate change may nullify the efficacy of a phenological mismatch and cause increased herbivore impacts to historically resistant hosts (Pureswaran et al., 2015, 2018; Fuentealba et al., 2017; Bellemin-Noël et al., 2021). As synchrony between the insect and host continue to converge at higher latitudes and elevations, historically resistant trees may become increasingly susceptible, resulting in further expansion of western spruce budworm into regions not historically prone to outbreaks.
Improved phenological matching in the north was accompanied by diverging synchrony at lower latitudes/elevations, reinforcing the northern range shift by outbreaking budworm populations over the past century. This result is consistent with Thomson and Benton (2007) who found that warming in the southwest portion of BC (i.e., Vancouver Island) after 1930 was associated with reduced budworm population densities, putatively a consequence of phenological mismatching. Indeed, our simulation suggests an increase in asynchrony between larval emergence and budburst of approximately 5 days since 1900. If the trend continues, it is likely that western spruce budworm outbreaks will become rare over large portions of southern BC. Although climate change-induced range retractions by irruptive insects are less common than expansions (Wilson et al., 2005), phenological mismatches have been implicated in decreases in outbreak frequencies for several defoliator species (Visser and Holleman, 2001; Johnson et al., 2010).
Range changes by irruptive folivores in pursuit of shifting phenological synchrony with their hosts is a commonly predicted (Bale et al., 2002; van Asch and Visser, 2007; Pureswaran et al., 2015; Despland, 2018), but infrequently documented consequence of climate change (but see Jepsen et al., 2009, 2011). For the western spruce budworm, we have shown that the shift by outbreak populations to higher latitudes and elevations is associated with both convergence and divergence in phenological matching with Douglas-fir. This is an outcome of differential responses to temperature by the insect and its host tree. Although both are reliant upon degree day accumulation, Douglas-fir bud development is putatively dependent upon an additional photoperiodic threshold (Linkosalo et al., 2006). Thus, the potential for warming to cause directional shifts in phenological matching between irruptive early season folivores and their host trees may be contingent upon the degree to which each depends on different components of their environments for their development (van Asch and Visser, 2007; Both et al., 2009); an aspect that should be considered in future research into the role of climate change in outbreaks by irruptive folivores in seasonal environments.
Data Availability Statement
Publicly available datasets were analyzed in this study. This data can be found here: https://www2.gov.bc.ca/gov/content/industry/forestry/managing-our-forest-resources/forest-health/aerial-overview-surveys and https://www2.gov.bc.ca/gov/content/industry/forestry/managing-our-forest-resources/forest-inventory/data-management-and-access.
Author Contributions
AT and AC conceived and designed the study and wrote the manuscript. AT conducted the analyses. Both authors contributed to the article and approved the submitted version.
Funding
This research was funded in form of grants from the Future Forest Ecosystem Science Council of British Columbia and the Natural Sciences and Engineering Research Council of Canada (Discovery Grant RGPIN-2015-04376) to AC.
Conflict of Interest
The authors declare that the research was conducted in the absence of any commercial or financial relationships that could be construed as a potential conflict of interest.
Publisher’s Note
All claims expressed in this article are solely those of the authors and do not necessarily represent those of their affiliated organizations, or those of the publisher, the editors and the reviewers. Any product that may be evaluated in this article, or claim that may be made by its manufacturer, is not guaranteed or endorsed by the publisher.
Acknowledgments
We would like to acknowledge all of the members of the UBC Forest Insect Disturbance Ecology Lab for their support and enthusiasm for ecological research.
References
Alfaro, R. I., and Maclauchlan, L. E. (1992). A method to calculate the losses caused by western spruce budworm in uneven-aged Douglas fir forests of British Columbia. For. Ecol. Manage. 55, 295–313.
Alfaro, R. I., van Akker, L., Berga, J., Van Hezewijk, B., Zhang, Q.-B., Hebdac, R., et al. (2018). Change in the periodicity of a cyclical forest defoliator: an indicator of ecosystem alteration in Western Canada. For. Ecol. Manage. 430, 117–125.
Axelson, J. N., Smith, D. J., Daniels, L. D., and Alfaro, R. I. (2015). Multicentury reconstruction of western spruce budworm outbreaks in central British Columbia, Canada. For. Ecol. Manage. 335, 235–248.
Ayres, M. P., and Lombardero, M. J. (2000). Assessing the consequences of global change for forest disturbance from herbivores and pathogens. Sci. Total Environ. 262, 263–286. doi: 10.1016/s0048-9697(00)00528-3
Bale, J. S., Masters, G. J., Hodkinson, I. D., Awmack, C., Bezemer, T. M., Brown, V. K., et al. (2002). Herbivory in global climate change research: direct effects of rising temperature on insect herbivores. Glob. Change Biol. 8, 1–16.
Battisti, A., Stastny, M., Buffo, E., and Larsson, S. (2006). A rapid altitudinal range expansion in the pine processionary moth produced by the 2003 climatic anomaly. Glob. Change Biol. 12, 662–671.
BC Ministry of Forests Lands, Natural Resource Operations and Rural Development (2022a). Aerial Overview Surveys. Available online at: https://www2.gov.bc.ca/gov/content/industry/forestry/managing-our-forest-resources/forest-health/aerial-overview-surveys (accessed March 7, 2022).
BC Ministry of Forests Lands, Natural Resource Operations and Rural Development (2022b). VRI Data Management and Access. Available online at: https://www2.gov.bc.ca/gov/content/industry/forestry/managing-our-forest-resources/forest-inventory/data-management-and-access (accessed March 7, 2022).
Beckwith, R. C., and Burnell, D. G. (1982). Spring larval dispersal of western spruce budworm (Lepidoptera: Tortricidae) in North-Central Washington. Environ. Entomol. 11, 828–832.
Bellemin-Noël, B., Bourassa, S., Despland, E., Grandpré, L. D., and Pureswaran, D. S. (2021). Improved performance of the eastern spruce budworm on black spruce as warming temperatures disrupt phenological defences. Glob. Change Biol. 27, 3358–3366. doi: 10.1111/gcb.15643
Both, C., Van Asch, M., Bijlsma, R. G., van den Burg, A. B., and Visser, M. E. (2009). Climate change and unequal phenological changes across four trophic levels: constraints or adaptations? J. Anim. Ecol. 78, 73–83. doi: 10.1111/j.1365-2656.2008.01458.x
Bylund, H. (1999). Climate and the population dynamics of two insect outbreak species in the north. Ecol. Bull. 47, 54–62.
Cade, B. S., and Noon, B. R. (2003). A gentle introduction to quantile regression for ecologists. Front. Ecol. Environ. 1:412–420.
Cade, B. S., Terrell, J. W., and Schroeder, R. L. (1999). Estimating effects of limiting factors with regression quantiles. Ecology 80, 311–323.
Caffarra, A., and Donnelly, A. (2011). The ecological significance of phenology in four different tree species: effects of light and temperature on bud burst. Int. J. Biometeorol. 55, 711–721. doi: 10.1007/s00484-010-0386-1
Campbell, R., Smith, D. J., and Arsenault, A. (2006). Multicentury history of western spruce budworm outbreaks in interior Douglas-fir forests near Kamloops, British Columbia. Can. J. For. Res. 36, 1758–1769.
Carroll, A. L. (1999). Physiological adaptation to temporal variation in conifer foliage by a caterpillar. Can. Entomol. 131, 659–669.
Carroll, A. L., and Quiring, D. (2003). Herbivory modifies conifer phenology: induced amelioration by a specialist folivore. Oecologia 136, 88–95. doi: 10.1007/s00442-003-1240-5
Chen, Z., Clancy, K. M., and Kolb, T. E. (2003). Variation in budburst phenology of Douglas-fir related to western spruce budworm (Lepidoptera: Tortricidae) fitness. J. Econ. Entomol. 96, 377–387. doi: 10.1603/0022-0493-96.2.377
Chen, Z., Kolb, T. E., and Clancy, K. M. (2001). Mechanisms of Douglas-fir resistance to western spruce budworm defoliation: bud burst phenology, photosynthetic compensation and growth rate. Tree Physiol. 21, 1159–1169. doi: 10.1093/treephys/21.16.1159
Clancy, K. M., Wagner, M. R., and Tinus, R. W. (1988). Variation in host foliage nutrient concentrations in relation to western spruce budworm herbivory. Can. J. For. Res. 18, 530–539.
Cudmore, T. J., Björklund, N., Carroll, A. L., and Lindgren, B. S. (2010). Climate change and range expansion of an aggressive bark beetle: evidence of higher reproductive success in naïve host tree populations. J. Appl. Ecol. 47, 1036–1043.
Cullingham, C. I., Cooke, J. E. K., Dang, S., Davis, C. S., Cooke, B. J., and Coltman, D. W. (2011). Mountain pine beetle host-range expansion threatens the boreal forest. Mol. Ecol. 20, 2157–2171. doi: 10.1111/j.1365-294X.2011.05086.x
Despland, E. (2018). Effects of phenological synchronization on caterpillar early-instar survival under a changing climate. Can. J. For. Res. 48, 247–254.
Deutsch, C. A., Tewksbury, J. J., Huey, R. B., Sheldon, K. S., Ghalambor, C. K., Haak, D. C., et al. (2008). Impacts of climate warming on terrestrial ectotherms across latitude. Proc. Natl. Acad. Sci. U.S.A. 105, 6668–6672. doi: 10.1073/pnas.0709472105
Fuentealba, A., Pureswaran, D., Bauce, É, and Despland, E. (2017). How does synchrony with host plant affect the performance of an outbreaking insect defoliator? Oecologia 184, 847–857. doi: 10.1007/s00442-017-3914-4
Harris, J. W. E., Alfaro, R. I., Dawson, A. F., and Brown, R. G. (1985). The Western Spruce Budworm in British Columbia 1909-1983. Canadian Forestry Service Information Report BC-X-257. Victoria, BC: Agriculture Canada, Ministry of State for Forestry, Pacific Forest Research Centre, 32.
Hickling, R., Roy, D. B., Hill, J. K., and Thomas, C. D. (2005). A northward shift of range margins in British Odonata. Glob. Change Biol. 11, 502–506.
Hopkins, A. D. (1919). The Bioclimatic Law as applied to entomological research and farm practise. Sci. Mon. 8, 496–513.
Howe, M., Raffa, K. F., Aukema, B. H., and Carroll, A. L. (2022). Numbers matter: how irruptive bark beetles initiate transition to self-sustaining behavior during landscape-altering outbreaks. Oecologia 198, 681–698. doi: 10.1007/s00442-022-05129-4
Hughes, I. (2000). Biological consequences of global warming: is the signal already apparent? Trends Ecol. Evol. 15, 56–61. doi: 10.1016/s0169-5347(99)01764-4
Jepsen, J. U., Biuw, M., Ims, R. A., Kapari, L., Schott, T., Petter, O., et al. (2013). Ecosystem impacts of a range expanding forest defoliator at the forest-tundra Ecotone. Ecosystems 16, 561–575.
Jepsen, J. U., Hagen, S. B., Ims, R. A., and Yoccoz, N. G. (2008). Climate change and outbreaks of the geometrids Operophtera brumata and Epirrita autumnata in subarctic birch forest: evidence of a recent outbreak range expansion. J. Anim. Ecol. 77, 257–264. doi: 10.1111/j.1365-2656.2007.01339.x
Jepsen, J. U., Hagen, S. B., Karlsen, S.-R., and Ims, R. A. (2009). Phase-dependent outbreak dynamics of geometrid moth linked to host plant phenology. Proc. R. Soc. B 276, 4119–4128. doi: 10.1098/rspb.2009.1148
Jepsen, J. U., Kapari, L., Hagen, S. B., Schott, T., Vindstad, O. P. L., Nilssen, A. C., et al. (2011). Rapid northwards expansion of a forest insect pest attributed to spring phenology matching with sub-Arctic birch. Glob. Chang. Biol. 17, 2071–2083.
Johnson, D. M., Büntgen, U., Frank, D. C., Kausrudd, K., Haynes, K. J., Liebhold, A. M., et al. (2010). Climatic warming disrupts recurrent Alpine insect outbreaks. Proc. Natl. Acad. Sci. U.S.A. 107, 20576–20581. doi: 10.1073/pnas.1010270107
Koenker, R. W. (2006). Quantreg: Quantile Regression. R Package Version 3⋅90. Available online at: http://www.cran.r-project.org (accessed April 22, 2022).
Kramer, K. (1994). A modelling analysis of the effects of climatic warming on the probability of spring frost damage to tree species in The Netherlands and Germany. Plant Cell Environ. 17, 367–377.
Kurz, W. A., Dymond, C. C., Stinson, G., Rampley, G. J., Neilson, E. T., Carroll, A. L., et al. (2008). Mountain pine beetle and forest carbon: feedback to climate change. Nature 452, 987–990. doi: 10.1038/nature06777
Lawrence, R. K., Mattson, W. J., and Haack, R. A. (1997). White spruce and the spruce budworm: defining the phenological window of susceptibility. Can. Entomol. 129, 291–318.
Liang, L. (2016). Beyond the bioclimatic law: geographic adaptation patterns of temperate plant phenology. Prog. Phys. Geogr. 40, 811–834.
Linkosalo, T., Häkkinen, R., and Hänninen, H. (2006). Models of the spring phenology of boreal and temperate trees: is there something missing? Tree Physiol. 26, 1165–1172. doi: 10.1093/treephys/26.9.1165
Lombardo, J. A., Sullivan, B. T., Myers, S. W., and Ayres, M. P. (2022). Are southern pine forests becoming too warm for the southern pine beetle? Agric. For. Meteorol. 315:108813. doi: 10.1016/j.agrformet.2022.108813
Maclauchlan, L. E., Brooks, J. E., and Hodge, J. C. (2006). Analysis of historic western spruce budworm defoliation in south central British Columbia. For. Ecol. Manage. 226, 351–356.
Maclauchlan, L. E., Daniels, L. D., Hodge, J. C., and Brooks, J. E. (2018). Characterization of western spruce budworm outbreak regions in the British Columbia Interior. Can. J. For. Res. 48, 783–802.
McMorran, A. (1973). Effects of pre-storage treatment on survival of diapausing larvae of the spruce budworm Choristoneura fumiferana (Lepidoptera: Tortricidae). Can. Entomol. 105, 1005–1009.
Miller-Rushing, A. J., Høye, T. T., Inouye, D. W., and Post, E. (2010). The effects of phenological mismatches on demography. Philos. Trans. R. Soc. B Biol. Sci. 365, 3177–3186. doi: 10.1098/rstb.2010.0148
Nealis, V. G. (2012). The phenological window for western spruce budworm: seasonal decline in resource quality. Agric. For. Entomol. 14, 340–347.
Nealis, V. G., and Nault, J. R. (2005). Seasonal changes in foliar terpenes indicate suitability of Douglas-fir buds for western spruce budworm. J. Chem. Ecol. 31, 683–696. doi: 10.1007/s10886-005-3538-8
Nealis, V. G., and Régnière, J. (2009). Risk of dispersal in western spruce budworm. Agric. For. Entomol. 11, 213–223. doi: 10.1111/1744-7917.12407
Nealis, V. G., and Régnière, J. (2016). Why western spruce budworms travel so far for the winter. Ecol. Entomol. 41, 633–641.
Nealis, V. G., and Régnière, J. (2021). Ecology of outbreak populations of the western spruce budworm. Ecosphere 12:e03667. doi: 10.1002/ecs2.3667
Pörtner, H. O., and Farrell, A. P. (2008). Physiology and climate change. Science 322, 690–692. doi: 10.1016/j.virol.2017.08.015
Pureswaran, D. S., De Grandpré, L., Paré, D., Taylor, A., Barrette, M., Morin, H., et al. (2015). Climate-induced changes in host tree–insect phenology may drive ecological state-shift in boreal forests. Ecology 96, 1480–1491.
Pureswaran, D. S., Neau, M., Marchand, M., Grandpré, L. D., and Kneeshaw, D. (2018). Phenological synchrony between eastern spruce budworm and its host trees increases with warmer temperatures in the boreal forest. Ecol. Evol. 9, 576–586. doi: 10.1002/ece3.4779
Quinn, B. K. (2017). A critical review of the use and performance of different function types for modeling temperature-dependent development of arthropod larvae. J. Therm. Biol. 63, 65–77. doi: 10.1016/j.jtherbio.2016.11.013
Quiring, D. T. (1992). Rapid change in suitability of white spruce for a specialist herbivore, Zeiraphera canadensis, as a function of leaf age. Can. J. Zool. 70, 2132–2138.
R Core Team (2014). R: A Language and Environment for Statistical Computing. Vienna: R Foundation for Statistical Computing.
Rasmann, S., Pellissier, L., Defossez, E., Jactel, H., and Kunstler, G. (2014). Climate-driven change in plant–insect interactions along elevation gradients. Funct. Ecol. 28, 46–54.
Rebaudo, F., and Rabhi, V. B. (2018). Modeling temperature-dependent development rate and phenology in insects: review of major developments, challenges, and future directions. Entomol. Exp. Applicata 166, 607–617.
Régnière, J. (1996). A generalized approach to landscape-wide seasonal forecasting with temperature-driven simulation models. Environ. Entomol. 25, 869–881.
Régnière, J., and Logan, J. A. (1996). “Landscape-wide projection of temperature-driven processes for seasonal pest management decision support: a generalized approach,” in Decision Support Systems for Forest Pest Management. FRDA, Report No. 260, eds T. L. Shore and D. A. MacLean (Victoria, BC: Canadian Forest Service and BC Ministry of Forests), 43–56.
Régnière, J., and Nealis, V. G. (2018). Two sides of a coin: host-plant synchrony fitness trade-offs in the population dynamics of the western spruce budworm. Insect Sci. 25, 117–126.
Régnière, J., and Nealis, V. G. (2019). Influence of temperature on historic and future population fitness of the western spruce budworm, Choristoneura occidentalis. Int. J. Pest Manage. 65, 228–243.
Régnière, J., Saint-Amant, R., and Béchard, A. (2013). BioSIM 10 – User’s Manual. Canadian Forest Service Inf. Rep. LAU-X-137. Quebec, QC: Canadian Forest Service, Laurentian Forestry Centre, 66.
Richardson, A. D., Hufkens, K., Li, X., and Ault, T. R. (2019). Testing Hopkins’ bioclimatic law with PhenoCam data. Appl. Plant Sci. 7:e1228. doi: 10.1002/aps3.1228
Royama, T. (1984). Population dynamics of the spruce budworm Choristoneura fumiferana. Ecol. Monogr. 54, 429–462.
Safranyik, L., Carroll, A. L., Régnèire, J., Langor, D. W., Riel, W. G., Shore, T. L., et al. (2010). Potential for range expansion of mountain pine beetle into the boreal forest of North America. Can. Entomol. 142, 415–442.
Sambaraju, K. R., Carroll, A. L., and Aukema, B. H. (2019). Multiyear weather anomalies associated with range shifts by the mountain pine beetle preceding large epidemics. For. Ecol. Manage 438, 86–95.
Saxe, H., Cannell, M. G. R., Johnsen, Ø., Ryan, M. G., and Vourlitis, G. (2001). Tree and forest functioning in response to global warming. New Phytol 149, 369–399. doi: 10.1046/j.1469-8137.2001.00057.x
Shepherd, R. F. (1992). Relationships between attack rates and survival of western spruce budworm, Choristoneura occidentalis Freeman (Lepidoptera: Tortricidae), and bud development of Douglas-fir, Pseudotsuga menziesii (Mirb.) Franco. Can. Entomol. 124, 347–358.
Simler-Williamson, A. B., Rizzo, D. M., and Cobb, R. C. (2019). Interacting effects of global change on forest pest and pathogen dynamics. Annu. Rev. Ecol. Evol. Syst. 50, 381–403.
Swetnam, T. W., and Lynch, A. M. (1989). A tree-ring reconstruction of western spruce budworm history in the southern Rocky Mountains. For. Sci. 35, 962–986.
Swetnam, T. W., and Lynch, A. M. (1993). Multicentury, regional-scale patterns of western spruce budworm outbreaks. Ecol. Monogr. 63, 399–424.
Thomson, A. J., and Benton, R. (2007). A 90-year sea warming trend explains outbreak patterns of western spruce budworm on Vancouver Island. For. Chron. 83, 867–869.
Thomson, A. J., and Moncrieff, S. M. (1982). Prediction of bud burst in Douglas-fir by degree-day accumulation. Can. J. For. Res. 12, 448–452.
Thomson, A. J., Shepherd, R. F., Harris, J. W. E., and Silversides, R. H. (1984). Relating weather to outbreaks of western spruce budworm Choristoneura occidentalis (Lepidoptera: Tortricidae), in British Columbia. Can. Entomol. 116, 375–381.
Tikkanen, O.-P., and Julkunen-Tiitto, R. (2003). Phenological variation as protection against defoliating insects: the case of Quercus robur and Operophtera brumata. Oecologia 136, 244–251. doi: 10.1007/s00442-003-1267-7
U.S. Geological Survey’s Center for Earth Resources Observation and Science (2010). 30 arc-second DEM of North America. ArcGIS Online, Data Basin Dataset. Available online at: http://databasin.org/datasets/d2198be9d2264de19cb93fe6a380b69c (accessed March 11, 2022).
van Asch, M., and Visser, M. E. (2007). Phenology of forest caterpillars and their host trees: the importance of synchrony. Annu. Rev. Entomol. 52, 37–55. doi: 10.1146/annurev.ento.52.110405.091418
Van Sickle, A., Fiddick, R. I., and Wood, C. S. (2001). The forest insect and disease survey in the Pacific Region. J. Entomol. Soc. B. C. 98, 169–176.
Van Sickle, G. A., Alfaro, R. I., and Thomson, A. J. (1983). Douglas-fir height growth affected by western spruce budworm. Can. J. For. Res. 13, 445–450.
Visser, M. E., and Holleman, L. J. M. (2001). Warmer springs disrupt the synchrony of oak and winter moth phenology. Proc. R. Soc. Lond. B 268, 289–294. doi: 10.1098/rspb.2000.1363
Volney, W. J. (1994). Multi-century regional western spruce budworm outbreak patterns. Trees 9, 43–45. doi: 10.1016/0169-5347(94)90265-8
Weed, A. S., Ayres, M. P., and Hicke, J. A. (2013). Consequences of climate change for biotic disturbances in North American forests. Ecol. Monogr. 83, 441–470.
Keywords: phenological synchrony, western spruce budworm, Douglas-fir, outbreaks, range expansion, range contraction, climate change
Citation: Tai AR and Carroll AL (2022) In the Pursuit of Synchrony: Northward Shifts in Western Spruce Budworm Outbreaks in a Warming Environment. Front. For. Glob. Change 5:895579. doi: 10.3389/ffgc.2022.895579
Received: 14 March 2022; Accepted: 09 May 2022;
Published: 26 May 2022.
Edited by:
Sigrid Netherer, University of Natural Resources and Life Sciences Vienna, AustriaReviewed by:
Vincent Nealis, Canadian Forest Service, CanadaManuela Branco, University of Lisbon, Portugal
Copyright © 2022 Tai and Carroll. This is an open-access article distributed under the terms of the Creative Commons Attribution License (CC BY). The use, distribution or reproduction in other forums is permitted, provided the original author(s) and the copyright owner(s) are credited and that the original publication in this journal is cited, in accordance with accepted academic practice. No use, distribution or reproduction is permitted which does not comply with these terms.
*Correspondence: Allan L. Carroll, allan.carroll@ubc.ca