- Fenner School of Environment & Society, The Australian National University, Canberra, ACT, Australia
Old growth is a critical growth stage in many forest types globally. It has many key ecological roles including biodiversity conservation, carbon storage and the provision of services such as water production. The extent of old growth forest has been declining in many ecosystems around the world, with major ecological and ecosystem service consequences. Important insights about such declines, as well as the structure, function and conservation of old growth forest, can be gained from detailed cross-sectional and longitudinal studies of different age cohorts within a given forest ecosystem. In this review article, we outline key insights into the characteristics of, and threats to old growth forests, using the Mountain Ash (Eucalyptus regnans) forests of the Central Highlands of Victoria, south-eastern Australia as a detailed case study. These forests are dominated by the tallest flowering plants on earth and have been subject to several decades of intense study. These studies show that old growth Mountain Ash forests are characterized by (among other features): giant trees (approaching 100 m tall and sometimes exceeding 20 m in circumference), numerous trees with hollows, an understorey of Acacia and rainforest trees, a range of plant and animal species that are rare or absent in younger aged stands, and moist, nutrient-rich soils. The area of old growth Mountain Ash forest has declined to 1.16% of the ∼141,000 ha area occupied by ash-type forests in the Central Highlands region. This is up to 60 times less than it was at the time of European colonization ∼220 years ago. The loss of old growth has major implications for bird, mammal and other biodiversity, as well as for carbon storage and water production for human consumption. The main drivers of old growth decline are recurrent wildfire, widespread clearcutting, and a logging-fire interaction in which cut and then regenerated forests become more flammable and are at significantly elevated risk of burning at high (stand replacing) severity. Climate change is also a driver of old growth decline both through elevating the mortality of large old living trees and underpinning an increase in the frequency of high severity wildfire. These interacting drivers mean that restoring old growth Mountain Ash forest will be an ecological and policy challenge. We argue that a first step must be to cease all commercial logging in the Mountain Ash ecosystem to allow new cohorts of old growth forest to be recruited and thereby expand the extent of the old growth estate. In addition, the Government of Victoria should revert to a past definition of old growth that made it easier for forest to qualify for protection. Given there are high risks of recurrent high-severity wildfire in the existing Mountain Ash forest estate which is dominated by highly flammable young regrowth forest, new technologies (such as the use of drones and satellites) are needed to rapidly detect and then suppress ignitions before fires become large and difficult to control. Mountain Ash forests have provided an important natural laboratory for understanding the dynamics, management and conservation of old growth forest. They have also helped generate some valuable general perspectives likely to be relevant to other forest ecosystems globally. These include: (1) the critical value of multi-facetted cross-sectional and longitudinal studies in quantifying attributes of, and threats to, old growth forest, (2) the need for a carefully crafted definition of old growth that will typically be ecosystem-specific and based on the time required to develop key ecosystem attributes (e.g., large old trees), (3) the importance of rigorous protection measures because poor decisions that result in the loss of old growth now will take prolonged periods to rectify, and (4) setting protection levels that are relative to the existing spatial coverage of remaining old growth and the extent and impacts of stressors driving old growth decline.
Introduction
Old growth forest has long been recognized as an important growth stage of many forest types globally (Franklin et al., 1981; Ambrose, 1982; Shao and Zhao, 1998; Sillett et al., 2000; Aakala et al., 2008; Colangelo et al., 2021). This is because of the many ecological and ecosystem service values of old growth forest, including as habitat for biodiversity (Bart and Forsman, 1992; Lindenmayer and Franklin, 2002; Betts et al., 2017; Phalan et al., 2019; Jones et al., 2021), for long-term carbon storage (Luyssaert et al., 2008; Keith et al., 2009; Moomaw et al., 2019), and the provision of clean air and water (Vertessy et al., 2001; Watson et al., 2018).
Despite the numerous key ecological values of old growth forests, their spatial extent has declined rapidly in many areas around the world (Vora, 1994; Watson et al., 2018; Lindenmayer and Taylor, 2020; McDowell et al., 2020). Several factors have contributed to these declines including (among others): land clearing for agriculture, industrial logging, fire, insect attack and climate change (Crowther et al., 2015; Lindenmayer and Laurance, 2017; Watson et al., 2018; McDowell et al., 2020). These drivers can act independently, or in combination, and reduce the spatial extent and erode the integrity of old growth forest ecosystems (Buma, 2015; Lindenmayer et al., 2022d).
Old growth stages of forest ecosystems can be structurally and compositionally complex environments (Franklin et al., 1981, 2002; Burgman, 1996; Gilhen-Baker et al., 2022). However, how they are defined, the range of ecological and other roles they play, and the threats they face can vary markedly between ecosystems (Burgman, 1996; Lindenmayer and Franklin, 2002; van Pelt, 2007; Martin et al., 2021). Many studies show that it is not possible to develop a generic definition of old growth forest that can be applied uncritically to all forest types and all regions. As an example, in British Columbia, Canada, some studies in coastal areas have defined old growth stands as those exceeding 250 years old, whereas in interior forests, old growth stands are defined as those older than 140 years [e.g., see MacKinnon and Vold (1998)]. In a further example, some old growth stands of Douglas Fir (Pseudotsuga menziesii) forests in the Pacific Northwest of the United States are defined as exceeding 210 years, whereas markedly different age cohorts are used to distinguish old growth in forests dominated by other tree species (van Pelt, 2007). However, most definitions of old growth are indicative of the advanced age of dominant overstorey trees (Dyne, 1991; van Pelt, 2007; Gilhen-Baker et al., 2022) and the paucity of recent severe disturbances (Woodgate et al., 1994; Hunter and Schmiegelow, 2011) often in ecosystems where major disturbances (typically wildfires) are stand-replacing or partially stand-replacing events (Franklin et al., 1981; van Pelt, 2007; Lindenmayer et al., 2019b). Beyond such generalizations, ecosystem-specific definitions of old growth will be underpinned by key elements of stand structure such as tree canopy shape and density, the development of tree-hollows, stocking rates of trees, the presence of large quantities of coarse woody debris (including in streams), and the presence and abundance of particular plants such as epiphytes and ferns (see Franklin et al., 1981; van Pelt, 2007).
A combination of cross-sectional or chronosequence investigations, longitudinal studies (sensu Cunningham and Lindenmayer, 2016) and other kinds of work (e.g., predictive modeling and simulation modeling) can provide a comprehensive understanding of the unique characteristics of old-growth forests, identify their key threats and determine the most effective way to both conserve existing and facilitate the recruitment of new cohorts of old growth forest. In this paper, we outline the unique characteristics and ecological dynamics of old-growth forests and identify key threats to their preservation, conservation, and recruitment using Mountain Ash (Eucalyptus regnans) forests of mainland south-eastern Australia as a detailed case study. To do this, we draw on key insights generated from approximately four decades of investigations in these forests, including cross-sectional and longitudinal studies as well as modeling (e.g., Ball et al., 1999; Tulloch et al., 2018; Cary et al., 2021; Lindenmayer et al., 2022a). Old growth Mountain Ash forest was formerly defined as stands of dominant overstorey eucalypt trees exceeding 120 years old (Macfarlane and Seebeck, 1991). This is the age when large Eucalyptus trees first begin to develop cavities (Ambrose, 1982) and become critical nesting and denning resources for an array of forest-dependent species in these ecosystems (Lindenmayer, 1997; Lindenmayer et al., 2017a). More recently, old growth Mountain Ash forest has been redefined as stands of overstorey eucalypt trees exceeding 250 years (Office of the Conservation Regulator, 2019), although there is limited ecological principal for such changes, especially for threatened cavity-dependent species (Blair et al., 2018). For the purposes of this paper, on an ecological basis, we define old growth Mountain Ash forests as stands of overstorey eucalypts exceeding 120 years old.
Old growth Mountain Ash forests are spectacular (Figure 1) and support the tallest flowering trees on earth (approaching 100 m in height) (Ashton, 1975). Mountain Ash forests have been the target of detailed ecological and ecosystem service studies for almost four decades (Lindenmayer et al., 2015, 2022a; Bowd et al., 2021c; Bowd E. J. et al., 2021; Bowd et al., 2022), with some key long-term studies (e.g., temporal changes in populations of large old trees and populations of biota) (Lindenmayer et al., 2020) as well as a number of chronosequence investigations [e.g., disturbance effects on plant communities and soil nutrients and composition; (Bowd et al., 2018, 2019)]. Mountain Ash is an obligate seeder tree species that is vulnerable to disturbance at short intervals, with almost complete stand replacement occurring after high-severity disturbances including wildfire and clearcut logging (Ashton, 1981). We discuss how regimes of these natural and human disturbances can influence the age class structure of Mountain Ash forests, including the spatial extent of old growth forests at stand and landscape scales. We summarize ways in which this critically important growth stage can be better protected, especially through the cessation of widespread clearcut (industrial) logging, and the recruitment of existing areas of advanced regrowth that will be a key source of future stands of old growth forest. Finally, we present some general lessons from the substantial body of work in Australian Mountain Ash forests that may help guide old growth conservation efforts in other forest ecosystems globally.
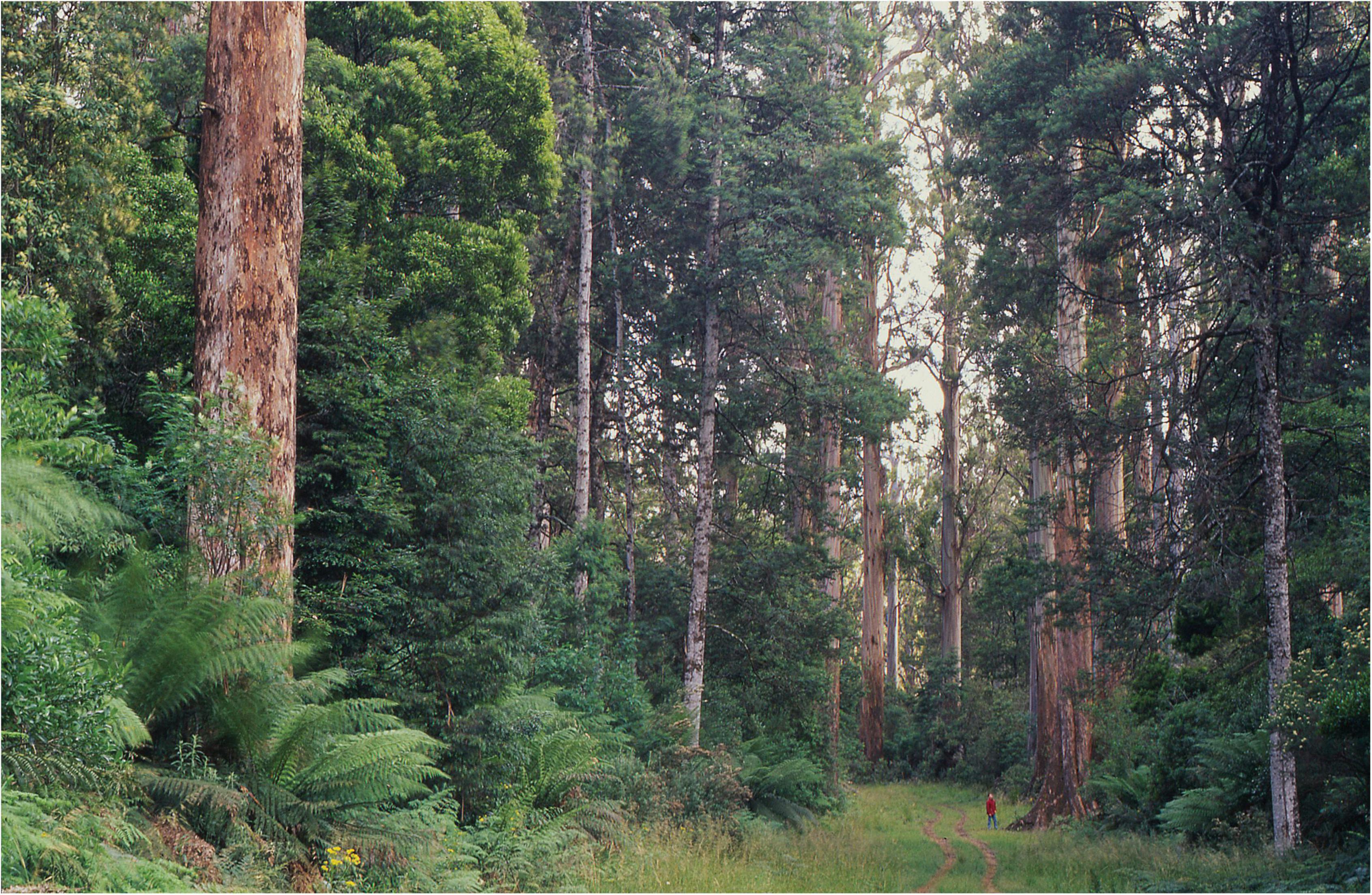
Figure 1. A stand of old growth Mountain Ash forest with an image of one of the authors (DL) highlighting the diameter and height of the trees. The image shows site 470 – one of a network of long-term sites where fauna, flora and vegetation structure have been studied for approximately four decades (Photo by Esther Beaton).
Background
Our overview of the importance of old growth Mountain Ash forests focuses on the Central Highlands of Victoria, a ∼80 km × 60 km region located approximately 120 km north-east of Melbourne, south-eastern Australia (Figure 2). The Mountain Ash forests of the Central Highlands of Victoria are a specifically defined and recognized ecosystem type (Burns et al., 2015) and they cover approximately 141,000 ha in the region. Over the past 40 years, the fauna and flora of these forests, and their responses to human and natural disturbance and post-perturbation recovery, have been well-documented (Flint and Fagg, 2007; Lindenmayer et al., 2015; Bowd et al., 2021a; Bowd E. J. et al., 2021; Bowd et al., 2022). This includes critical long-term insights generated by repeated biodiversity and vegetation surveys conducted across 183 long-term field sites (see Figure 2). The characteristics, dynamics, and ecological values of old growth stands have been a vital part of this work (e.g., Franklin et al., 1981; Lindenmayer et al., 2000a,2019a, 2021, 2022a,b; van Pelt, 2007; Bowd et al., 2019) and these topics are a key focus of this article.
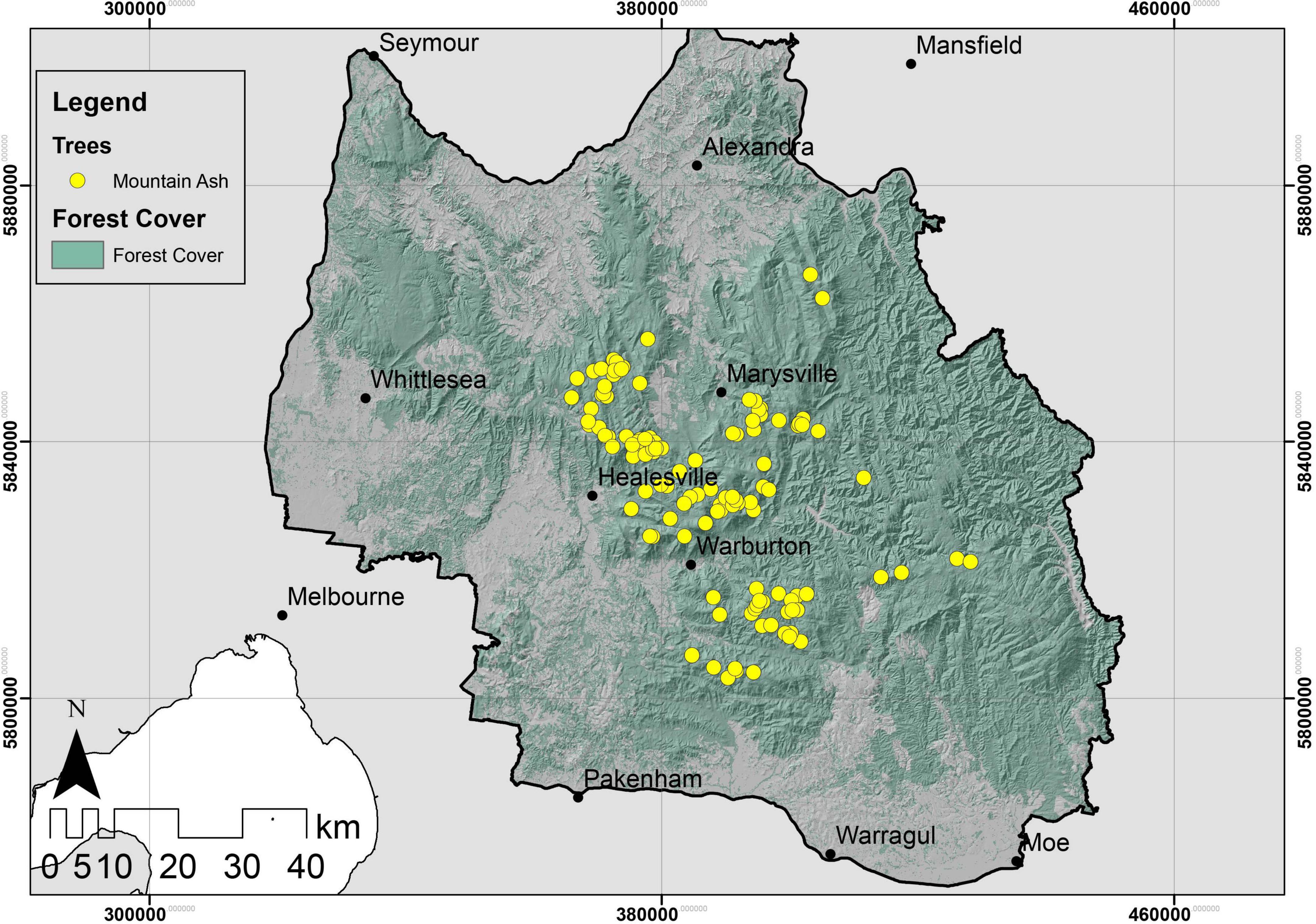
Figure 2. Map of Central Highlands region with long-term field sites where data on biodiversity and vegetation structure have been gathered (as indicated by colored dots).
Disturbance Regimes and Old Growth Mountain Ash Forests
Fire is the primary form of natural disturbance in Mountain Ash forests (Lindenmayer et al., 2022d). Mountain Ash trees depend on specific fire regimes to regenerate and maintain their functional integrity. Mountain Ash trees are often killed by high severity wildfire, but rapidly regenerate after fire from canopy-stored seed, often as even-aged cohorts of trees (Smith et al., 2014). The natural regime is rare, high-severity, stand-replacing wildfire that occurs on average every 75–150 years (Cary et al., 2021) but ranges from 30 to 300+ years depending on the location in the landscape (Mackey et al., 2002). Old growth forests typically occur in cooler, wetter and more sheltered parts of Mountain Ash-dominated landscapes (Mackey et al., 2002).
In the long (>200 years) absence of fire, Mountain Ash forests can transition into cool temperate rainforests dominated by Southern Sassafras (Atherosperma moschatum) and Myrtle Beech (Nothofagus cunninghamii) (Lindenmayer et al., 2000b). Conversely, frequent, high-severity wildfire (<20–30 intervals) can preclude stands from reaching sexual maturity and developing viable seed stores (Von Takach Dukai et al., 2018), resulting in regeneration failure and eventual ecological collapse (Lindenmayer et al., 2011; Burns et al., 2015). This can lead to Mountain Ash forests being replaced by Acacia spp. woodland (Lindenmayer et al., 2011) – sometimes termed the “interval squeeze” problem (Enright et al., 2015; Lindenmayer et al., 2022d). Evidence for the development of this phenomenon has been described in several other ecosystems around the world, with anthropogenically-driven climatic changes resulting in increased forest wildfires at a global scale (Fonseca et al., 2019; Canadell et al., 2021; van Oldenborgh et al., 2021). These include the wet eucalypt forests of north-eastern Victoria and Tasmania (Enright et al., 2015; Furlaud et al., 2021), North-American subalpine lodgepole pine forests (Turner et al., 2019), and Canadian conifer forests (Buma et al., 2013).
Moderate-low severity fires in Mountain Ash forests are partial stand-replacing events and lead to the development of multi-aged stands comprising living and dead overstorey trees (Lindenmayer and McCarthy, 1998). The severity of wildfires in Mountain Ash forests is influenced by the age of the forest at the time it is burnt. The highest severity fires occur in young (7–36 years old) stands, whereas the lowest severity fires are in stands which exceed ∼100 years old (Taylor et al., 2014). Indeed, no stands of old growth are purely a single age, but rather support trees from two or more age cohorts, including large old fire-scarred living trees (Lindenmayer et al., 2000a). In addition, fires in older stands typically produce more biological legacies (sensu Franklin et al., 2000) than fires in young stands (Lindenmayer et al., 2019b), and support a more diverse vegetation assemblage and accelerated recovery rate (Bowd et al., 2021a).
The biological legacies remaining in burnt stands provide critical habitat elements for birds, mammals, vascular plants and bryophytes (Lindenmayer et al., 2019b). Therefore, the habitat value of regenerating Mountain Ash forests after fire is strongly influenced by the age of the forest prior to a fire (Figure 3). For instance, forests regenerating after fire in prior old growth forests are structurally heterogeneous, supporting many large, fire-killed hollow bearing trees amongst dense cohorts of young Eucalyptus, Acacia and other tree species (Lindenmayer et al., 2019b; Bowd et al., 2021a) (Figure 3). This structurally and compositionally diverse post-fire environment provides key resources for biota including habitat, food, and foraging substrates, facilitating the recovery of many animal species. In contrast, regenerating forest stands burnt at earlier successional stages (like those created after clearcut logging; see below) are typically homogenous and inconsistent with the habitat requirements of many species (Bowd et al., 2021a) (Figure 3). For some species, the habitat value of these stands may take over a century to recover after fire due to the absence of critically important hollow-bearing trees that can take 170 or more years to develop (Lindenmayer et al., 2019b).
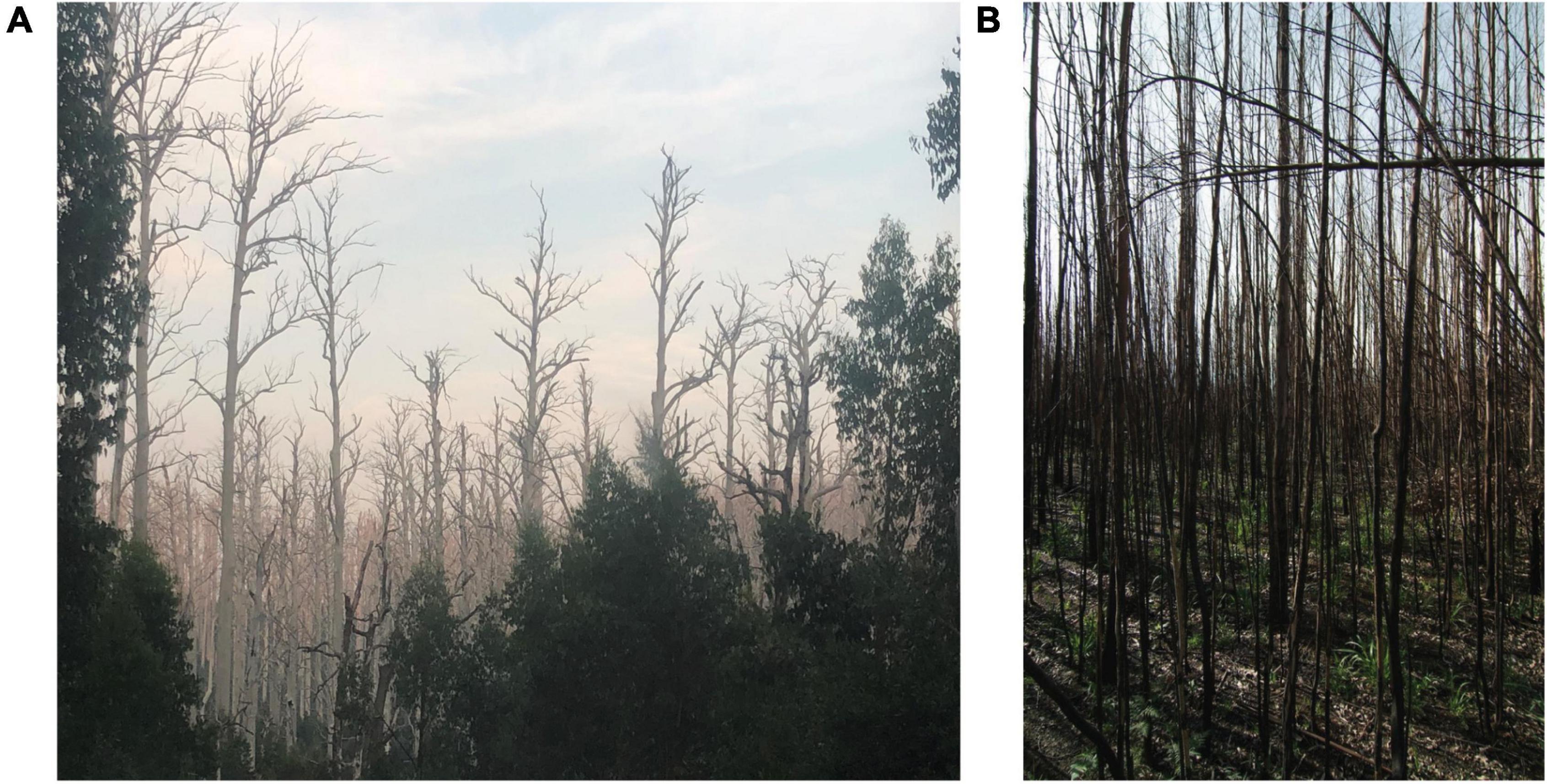
Figure 3. Post-fire structural differences in forests that were at (A) old-growth and (B) young (<20 years) successional stages at the time of wildfire in 2009. [Photo (A) was taken by Elle Bowd, Photo (B) was taken by the late David Blair].
Industrial logging, primarily widespread clearcutting, is the dominant form of human disturbance in Mountain Ash forests (Lindenmayer et al., 2022d). Mountain Ash forests have been logged for more than 150 years with clearcutting being the conventional silvicultural system employed for the past 50 years (Flint and Fagg, 2007). Mountain Ash forests are located predominately on State Government-owned land, and Timber Release Plans specify the location and size of “cutblocks” or harvest units as well as where logging exclusion areas occur (e.g., VicForests, 2019b). The clearcutting approach is relatively simple and entails: (1) all merchantable trees being removed offsite for subsequent processing as sawn timber or for pulpwood, (2) logging slash being left on the forest floor for one or more years to dry, (3) a high-intensity regeneration burn being applied to consume the logging slash and create a bed of ashes in which Mountain Ash seed is aerially dropped on the cutblock to instigate stand regeneration (Flint and Fagg, 2007). The typical size for cutblocks ranges from 15–40 ha and varies with topography (Department of Natural Resources and Environment, 1996). Clearcutting resets stand age to zero with the specified rotation age until the next logging operation being ∼80 years.
Clearcutting is relatively efficient in producing large quantities of pulpwood and timber. However, it also has a number of significant, negative environment impacts. These include: (1) eroding biodiversity, such as populations of arboreal marsupials, birds and resprouting native tree ferns, shrubs and trees (Bowd et al., 2018; Lindenmayer et al., 2019a,2020), (2) generating carbon emissions, including smoke pollution during the burning of logging slash which greatly reduces the air-quality of surrounding communities (Lindenmayer and Taylor, 2018), (3) depleting key soil nutrients and altering soil structure (Bowd et al., 2019), (4) altering the soil microbiome such as by reducing the diversity of fungal symbionts (Bowd et al., 2022), (5) reducing water yields from watersheds (Vertessy et al., 2001; Taylor et al., 2019), (6) fragmenting patterns of forest cover (Taylor and Lindenmayer, 2020), and (7) increasing levels of forest flammability (Taylor et al., 2014, 2020). Some of these effects are at the stand level (Bowd et al., 2019), others are landscape level effects (Lindenmayer et al., 2020, 2022d), and yet others are at an ecosystem level (such as the elevated risk of ecosystem collapse in logged forest) (Lindenmayer and Sato, 2018; Lindenmayer et al., 2022d).
Definitions of Old Growth Mountain Ash Forest
The identification of old growth forest is dependent on how it is defined (Burgman, 1996; van Pelt, 2007). The Victorian Government developed the following definition following the work by Woodgate et al. (1994):
Old-growth forest is forest which contains significant amounts of its oldest growth stage – usually senescing trees – in the upper stratum and has been subject to any disturbance, the effect of which is now negligible (Department of Natural Resources and Environment, 1996, p. 3).
As outlined above, the definition of old growth in Victoria has changed in recent years in some forest types (DEPI, 2013; VicForests, 2013; Office of the Conservation Regulator, 2019). In Mountain Ash forests, old growth forest used to be defined as stands that were 120 or more years old. Now stands that are 250 years old or older are considered to be old growth by the Victorian Government (VicForests, 2013). The minimum patch size for protection of stands of old growth Mountain Ash forest from logging used to be 5 ha (with patches smaller than this allowed to be logged). However, recent changes in prescriptions have brought minimum patch sizes for protection from logging down to 1 ha (Office of the Conservation Regulator, 2019).
Current definitions of old growth Mountain Ash forest focus on the age of the dominant overstorey trees, in part because tree and stand age (based on stem diameter) is relatively easily determined from surveys on-the-ground (Lindenmayer et al., 2017a) and in aerial surveys. This, in turn, facilitates mapping and forest management planning, such as the location of regrowth forests available for logging (e.g., VicForests, 2019a). However, some elements of the understorey, particularly those that resprout after wildfires such as tree ferns, can be comparatively old, even when the overstorey trees are relatively young (Blair et al., 2017b). As an example, tree ferns can exceed 250 years old even when the overstorey eucalypts are less than one-third of that age (Mueck et al., 1996). However, in line with Victorian Government planning and management practice, we have used the age of overstorey eucalypt trees to guide the definition of old growth Mountain Ash forest. Specifically, based on their critical ecological values (such as the presence of numerous large old hollow-bearing trees; see below), we define old growth forests as stands that are older 120 years old – which is the age this species first begins to develop cavities (Ambrose, 1982; Lindenmayer et al., 1993).
Critical Features of Old Growth Mountain Ash Forest
Old growth Mountain Ash has some distinctive structural features that are often rare or absent in younger stands (Figures 4, 5 and Table 1). Detailed analyses across a chronosequence of stands of different age (Lindenmayer et al., 2000a) revealed that relative to younger aged stands, old growth forests: (1) support significantly more large old hollow-bearing trees, (2) larger numbers of tree ferns, (3) better developed hanging bark microhabitats, (4) a better developed understory of cool temperate rainforest dominated by Myrtle Beech, and (5) a greater number of vegetation layers leading to higher levels of vertical heterogeneity in stand structural complexity (sensu Brokaw and Lent, 1999). In addition, rates of collapse of large old trees tend to be slower in old growth Mountain Ash forests than in stands of younger age, likely because they are less exposed to climatic conditions such as elevated windspeeds (Lindenmayer et al., 2018a). The soils of old growth Mountain Ash forests typically support higher levels of key soil nutrients such as plant available nitrogen, phosphorus and have greater measures of carbon and soil moisture, relative to younger forests with a more recent history of disturbance (Bowd et al., 2019). Finally, old growth forests are characterized by cooler, less variable microclimatic conditions in comparison to young stands, particularly those that are 10+ years old (Furlaud et al., 2021; Lindenmayer et al., 2022a).
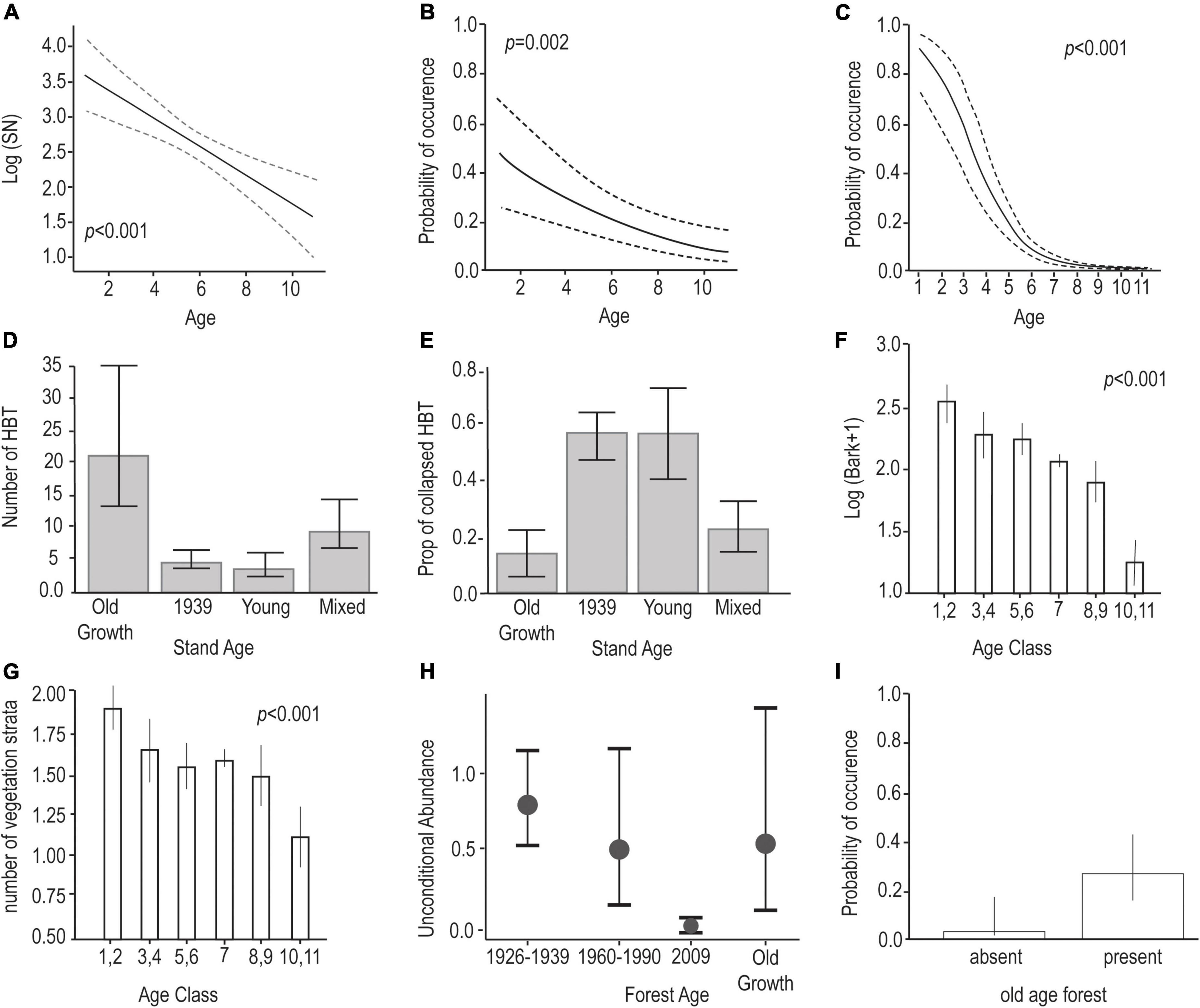
Figure 5. Differences in a selection of key structural attributes of stands of Mountain Ash forest of different ages in the Central Highlands of Victoria based on detailed field-based measurements. Old growth stands correspond to Age class 1 on the x-axis and stand age relationships shown for: (A) the stocking rate of rainforest understorey (Nothofagus cunninghamii). (B) The probability of occurrence of rainforest. (C) The presence of multi-aged forest (as a function of the number of age classes present). (D) The abundance of hollow-bearing trees. (E) The probability of collapse of hollow-bearing trees. (F) The abundance of bark streamers hanging from lateral branches. (G) The number of vegetation strata. (H) The abundance of the Greater Glider. (I) The presence of the Yellow-bellied Glider.
Critical Values of Old Growth Mountain Ash Forest
Several studies have underscored the many ecological and ecosystem values of old growth Mountain Ash forest (Table 1). Old growth Mountain Ash forest provides critical habitat for many elements of biodiversity. For example: (1) Site occupancy by many bird species is significantly greater in old growth forest than in younger aged cohorts (Lindenmayer et al., 2019a). And, (2) Species of arboreal marsupials, including species of conservation concern like the Southern Greater Glider (Petauroides volans) and Yellow-bellied Glider (Petaurus australis) are strongly associated with old growth forest (Lindenmayer et al., 1990b,1999; Lefoe et al., 2021). In addition, many species are intimately associated with structural attributes of stands that are significantly more likely to occur in old growth forests. As an example, the occurrence of the Mistletoebird (Dicaeum hirundinaceum) is closely tied to the occurrence of mistletoe which is found primarily in old growth Mountain Ash forest but rare in younger stands (Lindenmayer et al., 2015). The Tree Martin (Petrochelidon nigricans) is strongly associated with the dead tops of very large old living and/or dead trees. Similarly, old growth stands support significantly more large old hollow-bearing trees than young stands (Lindenmayer et al., 2000a) and these trees are critical nesting sites for many species of cavity-dependent vertebrates such as arboreal marsupials. Indeed, there are strong links between the occurrence (Lindenmayer et al., 2020) and richness (Bowd E. J. et al., 2021) of these species and the abundance of hollow-bearing trees.
Large trees store disproportionally large amounts of carbon across global forest ecosystems and therefore play critical roles in the mitigation of climate change (Mildrexler et al., 2020). Conversely, as a result of altered disturbance regimes, forest degradation and the loss of large old trees can produce significant carbon emissions. Alarmingly, in recent years, net carbon losses have been documented in some Amazonian forests (Gatti et al., 2021) and boreal forests (Walker et al., 2019). That is, these forests may have transitioned from a carbon sink to a carbon source (Walker et al., 2019; Gatti et al., 2021). Mountain Ash forests are some of the most carbon-dense forests in the world, with older forests characterized by substantially higher levels of above-ground biomass carbon than younger aged forests (Keith et al., 2009). In addition, the soils in old growth forests have a higher percentage of organic carbon than the soils in forests with a more recent disturbance history (Bowd et al., 2019). The protection of these forests is important for carbon storage in the current period of rapid, global climatic change and predicted increases in wildfires (and subsequent carbon emissions).
A suite of studies have also demonstrated that old growth Mountain Ash forests are characterized by significantly greater water yields than younger forests (Vertessy et al., 2001). The age of forests in water catchments therefore has major implications for water yields for human consumption (Vertessy et al., 2001; Taylor et al., 2019).
Threats to Old Growth Mountain Ash Forest
Temporal Changes in Old Growth Forest
Lindenmayer and Taylor (2020) found that since 1995 there has been a reduction of 77% in the amount of old growth forest across a wide range of forest types across Victoria. The Wet and Damp Ecological Vegetation Class to which Mountain Ash forest belongs has been particularly heavily disturbed by both fire and logging (Lindenmayer and Taylor, 2020). In the Central Highlands region of Victoria, the spatial extent of the Mountain Ash ecosystem has changed little in the past 100 years (Burns et al., 2015). However, the age class structure has been radically altered and only 1.16% of the Mountain Ash ecosystem is now old growth. Not only is the amount of old growth remaining limited in spatial extent (encompassing just 1,887 ha), it is also highly fragmented and dispersed across 147 different patches, most of which are less than 3 ha in size. Many of these remaining old growth patches are close to cutblocks or roads (Taylor and Lindenmayer, 2020).
Logging and Wildfire as Drivers of Old Growth Decline
Wildfire and logging are the two major drivers of decline of old growth Mountain Ash forest, which have collectively led to landscapes being dominated by regrowth vegetation (Lindenmayer et al., 2022d). There were large-scale wildfires in Mountain Ash forests in 1824, 1851, 1926, 1932, 1939, 1983, and 2009. Smaller scale wildfires occurred in 1908, 1918, 1919, 1948 and 2019. Empirical data suggest that the frequency of high-severity wildfires is increasing in Mountain Ash forests, in part as a result of climate change in eastern Australia (Canadell et al., 2021) and also as a consequence of elevated flammability in young stands regenerated after logging (Taylor et al., 2014, see below). Modeling by Cary et al. (2021) indicates that current patterns of recurrent wildfire in Mountain Ash forests will occur at sufficient frequency to virtually completely preclude the recruitment of old growth stands.
Stands of old growth Mountain Ash forest greater than 5 ha in size (and more recently exceeding 1 ha in size) have been excluded from logging for more than 20 years. However, up until 1995, past logging operations removed extensive areas of old growth forest. Indeed, more than 120 sawmills used to operate in the ∼170,400 ha ash-type eucalypt forest estate of the Central Highlands region (Commonwealth of Australia and Department of Natural Resources and Environment, 1997; Lindenmayer et al., 2015). Stand reconstruction data suggest that up to 60% of the Mountain Ash forest in the Central Highlands of Victoria may have been old growth prior to large-scale logging operations (Lindenmayer and McCarthy, 2002). This is ∼ 60 times more old growth than currently exists.
Interactions Between Logging and Fire
Logging and wildfire also can produce interactive and cumulative effects on old growth Mountain Ash forests which have exacerbated their decline (Lindenmayer et al., 2022d). For instance, logging elevates the risk of high severity wildfire which can lead to stand replacement. Empirical studies by Taylor et al. (2014, 2020) after the 2009 wildfires in the Central Highlands of Victoria showed that logged and regenerated forests burnt at significantly higher severity than intact, unlogged forest (Figure 6). The paucity of old growth forest in the Central Highlands region and, conversely, the predominance of younger more flammable regrowth stands (<82 years old) that comprise almost all (∼99%) of the Mountain Ash forest estate, means that there is a high risk of recurrent wildfire in the landscape (Lindenmayer et al., 2022d). Recurrent widespread conflagrations in such flammable fire-prone landscapes mean that not only are existing small, remaining patches at risk of being burnt, but there is also little chance that regrowth forest will escape fire for long enough to eventually become old growth (Lindenmayer et al., 2011; Cary et al., 2021). Such recurrent fire dynamics in highly flammable landscapes have been termed a “landscape trap” (Lindenmayer et al., 2011, 2022d). The landscape trap phenomenon is also thought to be developing in other forests worldwide. These include the wet forests of north-eastern Victoria and Tasmania (Enright et al., 2015; Furlaud et al., 2021), tropical forests in South America (Cochrane and Laurance, 2008), the obligate-seeding dry woodlands of south west Western Australia (Gosper et al., 2018), and temperate forests in western North America (Zald and Dunn, 2017), southern South America (Paritsis et al., 2015; Tiribelli et al., 2018) and New Zealand (Kitzberger et al., 2016).
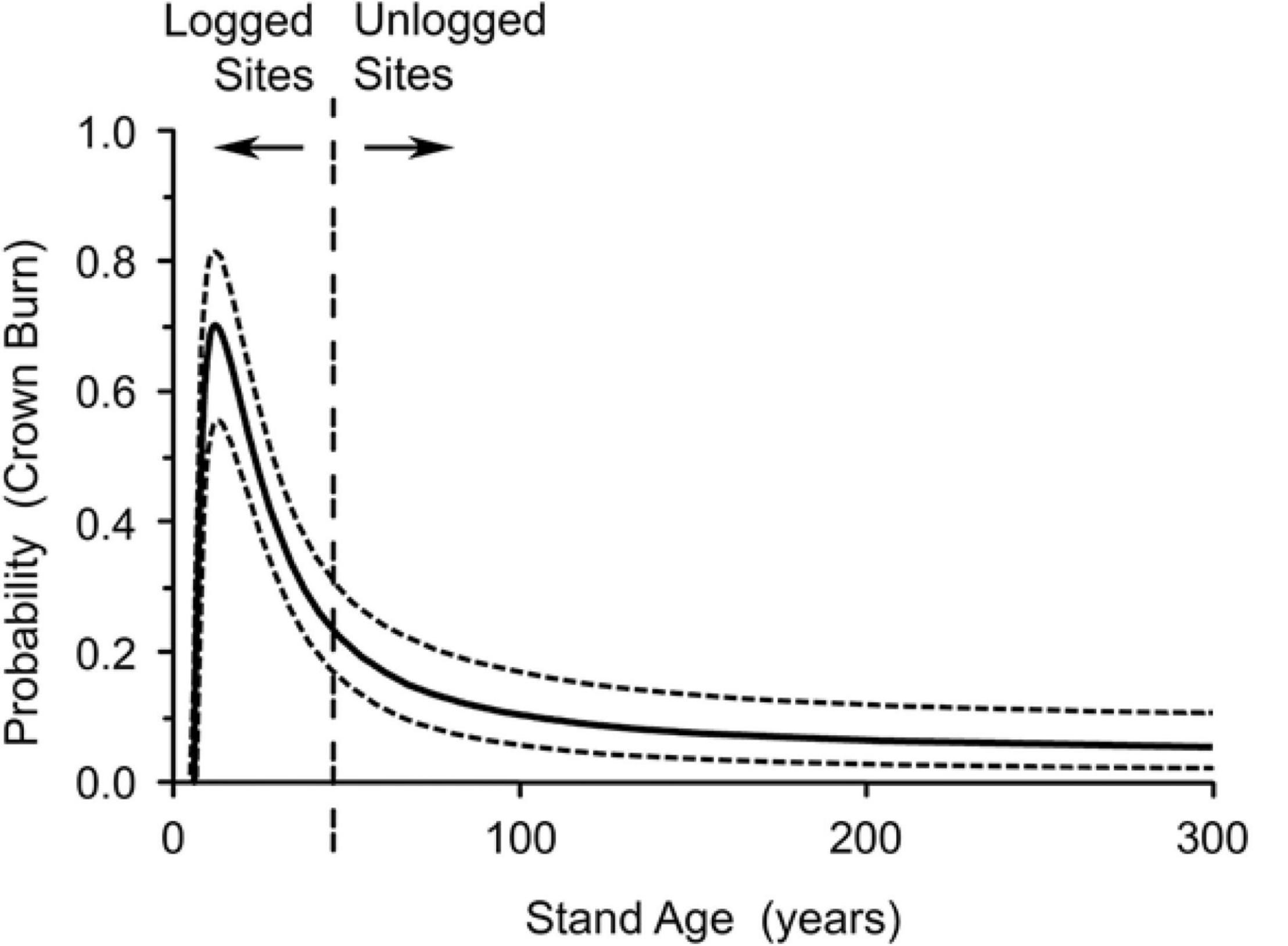
Figure 6. Non-linear relationship between stand age and the probability of canopy fire or crown burn. The probability of crown burn peaks at ∼40 years before declining as stands approach 80–100 years old. Redrawn from data in Taylor et al. (2014). The solid line corresponds to mean respond and the dashed line represented the lower and upper bounds of the 95% confidence interval.
Climate Change and Old Growth Forest
Beyond the effects of fire and logging, climate change also has a marked effect on old growth Mountain Ash forest. For example, Lindenmayer et al. (2012) found evidence of elevated mortality of large old living Mountain Ash trees during the drought in south-eastern Australia in the 2000s, most likely because of extreme temperatures and exceptionally low levels of rainfall at this time which creating conditions outside the natural bioclimatic domain occupied by the species (Lindenmayer et al., 1996). It has long been feared that ongoing climate change in the Central Highlands of Victoria may mean the region will eventually become entirely unsuitable for supporting Mountain Ash forest (Lindenmayer et al., 1991b). Of course, climate change is interacting with other drivers of decline in Mountain Ash forest. These include wildfire, with the prevalence of high-severity fire increasing with increasing temperatures associated with climate change (Canadell et al., 2021; van Oldenborgh et al., 2021).
Strengthened Protection of Old Growth Mountain Ash Forest
Revert to Previous Definitions of Old Growth Forest (That Are Ecologically Appropriate)
A change in the definition of old growth Mountain Ash forests has meant lower levels of protection for advanced growth stands (i.e., those 120–250 years old), which now require an additional 130 years of growth before prescriptions to protect them can take effect (Blair et al., 2017a). This significantly weakens the protection of large old trees and, in turn, the protection of denning habitat for cavity tree-dependent animal species of conservation concern like Leadbeater’s Possum (Gymnobelideus leadbeateri) and the Southern Greater Glider (Petauroides volans) (Blair et al., 2018).
We argue that because stands of old growth Mountain Ash are already rare (Lindenmayer and Taylor, 2020), the Victorian Government must revert to the original tree age definition of 120 years for stands to qualify as old growth. This change is important because trees occupied by arboreal marsupials of conservation concern are typically at least 120–170 years old (Lindenmayer et al., 2017a), and hence somewhat younger than the 250 years set out by government criteria for the definition of old growth (VicForests, 2013).
Reset Minimum Patch Size Levels for Improved Old Growth Protection
A key issue for the conservation of stands of old growth Mountain Ash forest is the definition of the minimum size of patches that must be excluded from logging. The minimum size of patches which can be protected from logging has been reduced from 5 to 1 ha (Office of the Conservation Regulator, 2019). However, many small patches of large old trees and individual large old trees are still at risk of being logged (Lindenmayer, 2017a). Individual large old trees are critical habitat for a wide range of species, including arboreal marsupials and birds of conservation concern. We argue that the definition for the minimum patch size for the protection of old growth patches needs to be further reduced from 1 ha to the scale of a single individual large old tree [defined here as any stem that is 120 years or older because this is when Mountain Ash trees first begin to develop cavities; (Ambrose, 1982; Lindenmayer et al., 1993)]. This change to better protect individual large old trees is especially important given the rapid rate at which existing populations of large old trees are collapsing in Mountain Ash forests (Lindenmayer et al., 2018a). We suggest that individual large old trees will need to be protected by a buffer of 100 m of unlogged forest. Our recommended buffering distance of 100 m is based on three key criteria: (1) empirical data on the accelerated rates of destruction and/or collapse of hollow-bearing trees on logged and regenerated sites (Lindenmayer et al., 1990a,2016), (2) the risk of collapse of trees in unlogged forest adjacent to logged areas (Lindenmayer et al., 1997, 2018b), and (3) the protection of potentially suitable foraging habitat and movement patterns by cavity-tree dependent threatened arboreal marsupials around nesting sites in unlogged forest (Lindenmayer et al., 2017b). The location of a given tree would act as the centroid of such buffers.
Cease Industrial Logging
Logging has been a major past contributor to the decline of old growth Mountain Ash forest directly through its removal, and indirectly by precluding the recruitment of new old growth stands. Logging also contributes to fire severity (Figure 6) which, in turn, can further reduce the extent of the old growth forest estate. The complete removal of logging from Mountain Ash landscapes therefore represents an important step in better protecting old growth forest. This protection will increase the chance that the limited remaining patches of old growth forest may persist in the future and will give advanced regrowth the best opportunity to reach old growth stages. The Government of Victoria has signaled it will end the native forest logging industry by 2030. In the interim, the restoration of the Mountain Ash ecosystem will benefit from strategic decisions on limiting logging in wetter and more sheltered parts of the landscape where previously analyses have indicated old growth forest was most likely to occur (see Mackey et al., 2002). It will also be important to set new targets for the proportion of the landscape that should be old growth forest. Given the potential impacts of future fire on stand age class distribution in the landscape, targets of substantially more than 60% will be required. This recommendation is based on congruence with historical levels of old growth coverage in Mountain Ash forests (Lindenmayer and McCarthy, 2002) and the fact that large amounts of existing forest (even if protected) will likely be lost to future frequent fire thereby making it challenging to reach set levels of old growth cover [see Cary et al. (2021)]. Modeling in other forest types around the world have been instructive in terms of identifying past levels of old growth cover and, in turn, the target amounts of future cover of this growth stage (e.g., for boreal forest in central Canada; Bélisle et al., 2011).
We argue that the case to cease logging and better protect Mountain Ash forests will be strengthened by highlighting the ecosystem service values of old growth stands, particularly the extent of carbon storage in such areas (Keith et al., 2009) as well as the high levels of water production for human consumption (Taylor et al., 2019). Indeed, in a formal economic and environmental accounting study, Keith et al. (2017) showed that for the Mountain Ash forests of the Central Highlands of Victoria, the economic value (in terms of contribution to regional GDP) of the water exceeded the value of the timber and pulpwood by more than 25 times and the value of carbon more than four times. Hence, the economic case for forest protection (and the cessation of logging) is compelling (Lindenmayer, 2017b).
Finally, there do not appear to be viable opportunities to employ silvicultural practices to promote the development of old growth characteristics in Mountain Ash forests, unlike in some other forest types around the world (e.g., see Bauhus et al., 2009). This is, in part, because activities like thinning in Mountain Ash forests can sometimes make stand more prone to high severity fire (Taylor et al., 2020) and at the same time have negative effects on some key mesic attributes of stand structure like the development of a cool temperate rainforest understorey. In addition, the extent of roads and other infrastructure needs to create access to logged stands can further fragment already highly fragmented Mountain Ash landscapes (Taylor and Lindenmayer, 2020) with corresponding negative impacts on some threatened forest-dependent species (Lindenmayer et al., 2020).
Better Protect Existing Old Growth and Advanced Regrowth Forest From Fire
Mountain Ash forest landscapes are highly flammable because of the predominance of young regrowth forest (Lindenmayer et al., 2022d). Repeated reburning risks the limited remaining old growth forest being incinerated in future fires (Lindenmayer et al., 2022d). This is a particular problem given the impacts of climate change on future fire in the region and elsewhere in south-eastern Australia (Canadell et al., 2021). Given such risks, we argue there is a need to develop new approaches to more quickly detect ignitions as soon as they occur and then rapidly suppress fires when the chances of extinguishing them are greatest. Satellite and other sensor systems, drones, and autonomous aerial vehicles will be important innovations in new generation fire detection and suppression capability (Roldán-Gómez et al., 2021). In addition, there will be value in embracing fire smart management actions (sensu Hirsch et al., 2001) to integrate forest management and fire management in an attempt to reduce the area burnt by wildfires. For example, in the case of Mountain Ash forests, this may entail not logging or otherwise disturbing areas (e.g., by semi-clearing them for so-called fire breaks) with a well developed cool temperate rainforest understorey where mesic conditions can sometimes limit fire spread in the landscape.
Do Not Salvage Log Old Growth Forests in the Event of Wildfire
In the event of old growth forests being burnt in a wildfire, we argue that they should not be subject to post-fire salvage logging. This is because burnt old growth forests typically support significant numbers of important biological legacies such as large old fire-scarred living and dead trees that can eventually become important nesting and denning sites for a wide range of cavity-dependent species (Lindenmayer and Ough, 2006). The post-fire persistence of animal and plant biota on burnt sites, as well as post-fire recolonization and regeneration patterns, can be substantially impaired by salvage logging operations (Bowd et al., 2018; Lindenmayer et al., 2018c)– a problem documented in numerous forest types globally (Thorn et al., 2018). Notably, the most species-rich and ecologically functional early successional forests will often be those which were old growth prior to disturbance (Lindenmayer et al., 2019b). This highlights the importance of landscapes supporting substantial areas of old growth forest in the event of future disturbances such as wildfires.
General Lessons for Old Growth Forest Protection
Our case study has focused largely on the importance of old growth Mountain Ash forests in mainland south-eastern Australia. However, there are some important general perspectives for other forest ecosystems that can be drawn from the body of work in Mountain Ash forests. First, many of the key insights generated from this body of work have been underpinned by cross-sectional and longitudinal studies spanning multiple inter-related topics (e.g., various kinds of disturbances, interactions between disturbance, biological legacies, and post-disturbance recovery). Such an array of different kinds of studies provide for a comprehensive understanding of the disturbance responses and recovery of forests that is often absent from a single, short-term “snapshot” investigation. For example, the importance of old growth Mountain Ash forest in influencing patterns of post-disturbance recovery and the abundance and quality of biological legacies has been made possible only through prolonged monitoring before and after perturbations (Bowd E. J. et al., 2021). The value of such kinds of monitoring in the context of old growth forest conservation and management has been established for many ecosystems globally [reviewed by Lindenmayer and Likens (2018)].
Second, detailed multi-facetted studies of old growth forest are needed to unravel key drivers of decline. This diagnosis will necessarily involve mechanistic understanding of ecological processes and dynamics in other age classes to enable stand age effects to be clearly elucidated. As an example, stand age-related interactions between fire severity and logging have been recently identified in Mountain Ash forests (Lindenmayer et al., 2022d). This required documenting contrasts in fire severity among many sites in several ages of forest (Taylor et al., 2014, 2020). Notably, similar patterns of high flammability in forests of intermediate age have now been found in many other forest types around the world, with old growth stands those subject to the lowest severity fire (Paritsis et al., 2015; Zald and Dunn, 2017; Gosper et al., 2018; Tiribelli et al., 2018; Furlaud et al., 2021; Zylstra et al., 2022).
Third, how old growth forest is defined matters (Franklin et al., 2002; van Pelt, 2007), including for the conservation of threatened species associated with such growth stages (Scotts, 1991; Bart and Forsman, 1992; Jones et al., 2021). Definitions will vary between ecosystems given ecosystem-specific differences in the rates at which key characteristics develop such as the presence of large trees, cavities in those trees, and numerous other features (Franklin et al., 1981; Burgman, 1996; Lindenmayer et al., 2000a; van Pelt, 2007; Gilhen-Baker et al., 2022). For example, the development of cavities in Australian eucalypts has sometimes been used to determine when trees and stands can be considered to be old growth forest. However, different tree species develop cavities at different rates and therefore at different ages (Lindenmayer et al., 1993; Gibbons and Lindenmayer, 2002). Similarly, attributes like mistletoe which in Mountain Ash forest are typically associated with old growth stands can be common in much younger vegetation in other forest and woodland types. In addition, animal species (e.g., the Southern Greater Glider) which in Mountain Ash ecosystems is often strongly associated with old growth forest (Lindenmayer et al., 1990b,2022b) do not show such associations in other parts of their distribution (Youngentob et al., 2013). Such ecosystem-specific differences in old growth characteristics and old growth associated taxa reinforce the need to carefully consider how old growth forest is defined and the ecological basis for such classification. This is because what is appropriate for one ecosystem may be entirely unsuitable for another (Burgman, 1996; van Pelt, 2007).
Differences in the definition of old growth forest have important ramifications for their protection, not only for old growth forest per se, but also for the plant and animal species which are strongly associated with the key structural attributes of old growth forest (e.g., cavity-dependent fauna). Indeed, the changes in definition of old growth Mountain Ash forest have had substantial negative impacts on efforts to conserve species of conservation concern like Leadbeater’s Possum and the Southern Greater Glider (Blair et al., 2018; Ashman et al., 2021). On this basis, resource managers and policy makers need to very carefully consider the definitions they construct for old growth forest and ensure that they align with the key objectives of conservation and management efforts.
Finally, many forest types globally are experiencing declines in old growth forest (McDowell et al., 2020), not unlike what has been described in this paper for Mountain Ash forest. As old-growth forests require considerable time periods to develop (sometimes up to 1,000 years; see van Pelt, 2007), poor decisions on their protection taken now will have negative implications for decades and even centuries to come. This highlights a need not only to be very conservative about the definition of old growth forest, but also to plan well ahead for new cohorts of old growth forest to replace existing areas if and/or when they are lost. In many cases, this will demand reducing the number of stressors in a landscape that can lead to the loss of old growth, particularly those stressors over which we have direct control such as logging operations. On this basis, levels of protection will need to be shaped by the spatial extent of old growth in a given ecosystem, and the prevalence of stressors driving decline. Stronger conservation measures will be appropriate in landscapes and ecosystems where old growth forest is rare and stressors are pervasive and widespread. For instance, in fire-prone ecosystems, this may include the application of new technologies (e.g., drones and satellites) that rapidly detect and then suppress fire in patches of old-growth (or stands approaching these ages) at high-risk of being destroyed.
Conclusion
Mountain Ash forest is one of the world’s iconic ecosystems and supports the tallest flowering plants on earth. Recurrent wildfire and extensive clearcut logging have radically altered the age class structure of these forests over the past 150 years (Lindenmayer and Taylor, 2020). As a result, old growth Mountain Ash forest is now extremely rare and covers only 1.16% of the spatial extent of the ecosystem. This is up to 60 times less than it was prior to European settlement. The predominance of young, flammable regrowth forest means that repeated reburning at short inter-fire intervals may prevent the recruitment of new areas of old growth forest and lock the ecosystem into a perpetually young state. To avoid the development of such a landscape trap (sensu Lindenmayer et al., 2022d), new policies to boost forest protection are urgently required, particularly the removal of large-scale industrial logging. Such policies are critical to restore the array of ecological and ecosystem service values of old growth Mountain Ash forest.
Mountain Ash forests have provided an important natural laboratory for understanding the dynamics, management and conservation of old growth forest as well as generating some valuable general perspectives relevant to other forest ecosystems globally. These include: (1) the critical value of cross-sectional investigations and long-term, multi-facetted studies, (2) the need for a carefully crafted definition of old growth that will typically be ecosystem-specific, (3) the importance of rigorous protection measures as poor decisions that result in the loss of old growth will take prolonged periods of forest recovery to rectify, and (4) setting protection levels relative to the existing spatial coverage of remaining old growth and the extent and impacts of stressors driving old growth decline.
Data Availability Statement
The original contributions presented in the study are included in the article/supplementary material, further inquiries can be directed to the corresponding author/s.
Author Contributions
All authors listed have made a substantial, direct, and intellectual contribution to the work, and approved it for publication.
Conflict of Interest
The authors declare that the research was conducted in the absence of any commercial or financial relationships that could be construed as a potential conflict of interest.
Publisher’s Note
All claims expressed in this article are solely those of the authors and do not necessarily represent those of their affiliated organizations, or those of the publisher, the editors and the reviewers. Any product that may be evaluated in this article, or claim that may be made by its manufacturer, is not guaranteed or endorsed by the publisher.
Acknowledgments
We thank Lachlan McBurney and the late Dave Blair for their outstanding field work in gathering some of the key datasets which underpin this manuscript. Tabitha Boyer provided expert editorial assistance in manuscript preparation.
References
Aakala, T., Kuuluvainen, T., Gauthier, S., and Grandpre, D. L. (2008). Standing dead trees and their decay-class dynamics in the northeastern boreal old growth forests of Quebec. Forest Ecol. Manage. 255, 410–420.
Ambrose, G. J. (1982). An Ecological and Behavioural Study of Vertebrates Using Hollows in Eucalypt Branches. Melbourne: La Trobe University. [Ph.D.Thesis].
Ashman, K. R., Watchorn, D. J., Lindenmayer, D. B., and Taylor, M. I. (2021). Is Australia’s environmental legislation protecting threatened species? A case study of the national listing of the Greater Glider. Pacif. Conserv. Biol. 1–13. doi: 10.1071/PC20077
Ashton, D. H. (1981). “Fire in tall open forests (wet sclerophyll forests),” in Fire and the Australian Biota, eds A. M. Gill, R. H. Groves, and I. R. Noble (Canberra, ACT: Australian Academy of Science), 339–366.
Ashton, D., and Willis, E. J. (1982). “Antagonisms in the regeneration of Eucalyptus regnans in the mature forest,”,” in The plant community as a working mechanism, ed. E. I. Newman (Oxford: Blackwell), 113–128.
Ashton, D. H. (1975). The root and shoot development of Eucalyptus regnans F. Muell. Aus. J. Bot. 23, 867–887.
Ball, I. R., Lindenmayer, D. B., and Possingham, H. P. (1999). A tree hollow dynamics simulation model. Forest Ecol. Manage. 123, 179–194.
Bart, J., and Forsman, E. D. (1992). Dependence on Northern Spotted Owl Strix occidentalis caurina on old growth forests in western U.S.A. Biol. Conserv. 62, 95–100. doi: 10.1016/0006-3207(92)90930-l
Bauhus, J., Puettmann, K., and Messier, C. (2009). Silviculture for old-growth attributes. Forest Ecol. Manage. 258, 525–537.
Bélisle, A. C., Gauthier, S., Cyr, D., Bergeron, Y., and Morin, H. (2011). Fire regime and old-growth boreal forests in central Quebec, Canada: an ecosystem management perspective. Silva Fennica 45, 889–908.
Betts, M. G., Phalan, B., Frey, S., Rousseau, J., and Yang, Z. (2017). Old-growth forests buffer climate-sensitive bird populations from warming. Divers. Distrib. 24, 439–447. doi: 10.1111/ddi.12688
Blair, D., McBurney, L., and Lindenmayer, D. B. (2018). Failing to conserve Leadbeater’s Possum and its Mountain Ash forest habitat. Aus. Zool. 39, 443–448. doi: 10.7882/az.2018.008
Blair, D., McBurney, L., Lindenmayer, D. B., Banks, S., and Blanchard, W. (2017a). “The Leadbeater’s Possum Review”. Canberra: The Australian National University.
Blair, D. P., Blanchard, W., Banks, S. C., and Lindenmayer, D. B. (2017b). Non-linear growth in the tree ferns, Dicksonia antarctica and Cyathea australis. PLoS One 12:e0176908. doi: 10.1371/journal.pone.0176908
Bowd, E., Blair, D. P., and Lindenmayer, D. B. (2021a). Prior disturbance legacy effects on plant recovery post-high severity wildfire. Ecosphere 12:e03480.
Bowd, E., Blanchard, W., McBurney, L., and Lindenmayer, D. B. (2021b). Direct and indirect fire impacts on forest biodiversity. Ecosphere 12:e03823. doi: 10.1002/ecs2.3823
Bowd, E., McBurney, L., and Lindenmayer, D. B. (2021c). Temporal patterns of vegetation recovery after wildfire in two obligate seeder ash forests. Forest Ecol. Manage. 496:119409.
Bowd, E. J., Banks, S. C., Bissett, A., May, T. W., and Lindenmayer, D. B. (2021). Direct and indirect disturbance impacts in forests. Ecol. Lett. 24, 1225–1236. doi: 10.1111/ele.13741
Bowd, E., Lindenmayer, D. B., May, T., Bissett, A., and Banks, S. (2022). Fire and logging regimes alter functional communities of fungi. Mol. Ecol. in press.
Bowd, E. J., Banks, S. C., Strong, C. L., and Lindenmayer, D. B. (2019). Long-term impacts of wildfire and logging on forest soils. Nat. Geosci. 12, 113–118. doi: 10.1038/s41561-018-0294-2
Bowd, E. J., Lindenmayer, D. B., Banks, S. C., and Blair, D. P. (2018). Logging and fire regimes alter plant communities. Ecol. Appl. 28, 826–841. doi: 10.1002/eap.1693
Brokaw, N. V., and Lent, R. A. (1999). ““Vertical structure,”,” in Managing Biodiversity in Forest Ecosystems, ed. M. Hunter (Cambridge: Cambridge University Press), 373–399.
Buma, B. (2015). Disturbance interactions: characterization, prediction, and the potential for cascading effects. Ecosphere 6:art70.
Buma, B., Brown, C. D., Donato, D. C., Fontaine, J. B., and Johnstone, J. F. (2013). The Impacts of Changing Disturbance Regimes on Serotinous Plant Populations and Communities. BioScience 63, 866–876. doi: 10.1525/bio.2013.63.11.5
Burgman, M. A. (1996). Characterisation and delineation of the Eucalypt old-growth forest estate in Australia - a review. Forest Ecol. Manage. 83, 149–161.
Burns, E. L., Lindenmayer, D. B., Stein, J., Blanchard, W., McBurney, L., Blair, D., et al. (2015). Ecosystem assessment of mountain ash forest in the Central Highlands of Victoria, south-eastern Australia. Aus. Ecol. 40, 386–399. doi: 10.1111/aec.12200
Canadell, J. G., Meyer, C. P., Cook, G. D., Dowdy, A., Briggs, P. R., Knauer, J., et al. (2021). Multi-decadal increase of forest burned area in Australia is linked to climate change. Nat. Commun. 12:6921. doi: 10.1038/s41467-021-27225-4
Cary, G., Blanchard, W., Foster, C. N., and Lindenmayer, D. B. (2021). Effects of altered fire regimes on critical timber production and conservation rotations. Int. J. Wildland Fire 30, 322–328.
Cochrane, M. A., and Laurance, W. F. (2008). Synergisms among fire, land use, and climate change in the Amazon. Ambio 37, 522–527. doi: 10.1579/0044-7447-37.7.522
Colangelo, M., Camarero, J., Gazola, A., Piovesan, G., Borghetti, M., Baliva, M., et al. (2021). Mediterranean old-growth forests exhibit resistance to climate warming. Sci. Total Environ. 801:149684. doi: 10.1016/j.scitotenv.2021.149684
Commonwealth of Australia and Department of Natural Resources and Environment. (1997). Comprehensive Regional Assessment - Biodiversity. Central Highlands of Victoria. Canberra: The Commonwealth of Australia and Department of Natural Resources and Environment.
Crowther, T. W., Glick, H. B., Covey, K. R., Bettigole, C., Maynard, D. S., Thomas, S. M., et al. (2015). Mapping tree density at a global scale. Nature 525, 201–205. doi: 10.1038/nature14967
Cunningham, R., and Lindenmayer, D. B. (2016). Approaches to landscape scale inference and design issues. Curr. Landsc. Ecol. Rep. 2, 42–50. doi: 10.1002/ece3.1782
Department of Natural Resources and Environment. (1996). Code of Practice. Code of Forest Practice for timber production. Revision No. 2, November 1996. Melbourne: Department of Natural Resources and Environment.
DEPI. (2013). State of the Forests Report 2013. Melbourne: Department of Environment and Primary Industries.
Dyne, G. (1991). ““Attributes of old growth forest in Australia”,” in Working Paper, ed. G. R. Dyne (Canberra: Bureau of Rural Resources, Department of Primary Industries and Energy).
Enright, N. J., Fontaine, J. B., Bowman, D. M., Bradstock, R. A., and Williams, R. J. (2015). Interval squeeze: altered fire regimes and demographic responses interact to threaten woody species persistence as climate changes. Front. Ecol. Environ. 13:265–272. doi: 10.1890/140231
Flint, A., and Fagg, P. (2007). “Mountain Ash in Victoria’s State Forests. Silviculture reference manual No. 1”. Melbourne: Department of Sustainability and Environment.
Fonseca, M., Alves, L. M., Aguiar, A. P., Arai, E., Anderson, L. O., Rosan, T. M., et al. (2019). Effects of climate and land-use change scenarios on fire probability during the 21st century in the Brazilian Amazon. Glob. Change Biol. 25, 2931–2946. doi: 10.1111/gcb.14709
Franklin, J. F., Cromack, K. J., Denison, W., McKee, A., Maser, C., Sedell, J., et al. (1981). “Ecological Attributes of Old-Growth Douglas-Fir Forests”, in USDA Forest Service General (Portland: Pacific Northwest Forest and Range Experimental Station). Technical Report PNW-118. doi: 10.1093/treephys/27.6.871
Franklin, J. F., Lindenmayer, D. B., MacMahon, J. A., McKee, A., Magnuson, J., Perry, D. A., et al. (2000). Threads of continuity. Conserv. Prac. 1, 8–17.
Franklin, J. F., Spies, T. A., van Pelt, R., Carey, A. B., Thornburgh, D. A., Berg, D. R., et al. (2002). Disturbances and the structural development of natural forest ecosystems with silvicultural implications, using Douglas-fir forests as an example. Forest Ecol. Manage. 155, 399–423. doi: 10.1016/s0378-1127(01)00575-8
Furlaud, J. M., Prior, L. D., Williamson, G. J., and Bowman, D. M. J. S. (2021). Fire risk and severity decline with stand development in Tasmanian giant Eucalyptus forest. Forest Ecol. Manage. 502:119724. doi: 10.1016/j.foreco.2021.119724
Gatti, L. V., Basso, L. S., Miller, J. B. M., Domingues, L. G., Cassol, H. L., Tejada, G., et al. (2021). Amazonia as a carbon source linked to deforestation and climate change. Nature 595, 388–393.
Gibbons, P., and Lindenmayer, D. B. (2002). Tree Hollows and Wildlife Conservation in Australia. Melbourne: CSIRO Publishing.
Gilhen-Baker, M., Roviello, V., Beresford-Kroeger, D., and Roviello, G. N. (2022). Old growth forests and large old trees as critical organisms connecting ecosystems and human health. Environ. Chem. Lett. 20, 1529–1538. doi: 10.1007/s10311-021-01372-y
Gosper, C. R., Yates, C. J., Crook, G. D., Harvey, J. M., Liedloff, A. C., McCaw, L., et al. (2018). A conceptual model of vegetation dynamics for the unique obligate-seeder eucalypt woodlands of south-western Australia. Aus. Ecol. 43, 681–695. doi: 10.1111/aec.12613
Hirsch, K., Kafka, V., TJlmstra, C., McAlpine, R., Hawkes, B., Stegehuis, H., et al. (2001). Fire-smart forest management: a pragmatic approach to sustainable forest management in fire-dominated ecosystems. Forestry Chron. 77, 357–363.
Hunter, M. L., and Schmiegelow, F. K. (2011). Wildlife, Forests and Forestry. Boston: Prentice Hall.
Jones, G. M., Kramer, H. A., Berigan, W. J., Whitmore, S. A., Gutiérrez, R. J., and Peery, M. Z. (2021). Megafire causes persistent loss of an old-forest species. Anim. Conserv. 24, 925–936.
Keith, H., Mackey, B. G., and Lindenmayer, D. B. (2009). Re-evaluation of forest biomass carbon stocks and lessons from the world’s most carbon-dense forests. Proc. Natl. Acad. Sci. 106, 11635–11640. doi: 10.1073/pnas.0901970106
Keith, H., Vardon, M., Stein, J. A. R., Stein, J. L., and Lindenmayer, D. B. (2017). Ecosystem accounts define explicit and spatial trade-offs for managing natural resources. Nat. Ecol. Evol. 1, 1683–1692. doi: 10.1038/s41559-017-0309-1
Kitzberger, T., Perry, G. L., Paritsis, J., Gowda, J. H., Tepley, A. J., Holz, A., et al. (2016). Fire-vegetation feedbacks and alternative stabe states: common mechanisms of temperate forest vulnerability to fire in southern South Ameria and New Zealand. N. Zealand J. Bot. 54, 247–272.
Lefoe, M., Rendall, A. R., McKinnon, F., and Whisson, D. A. (2021). Logging and wildfire limit the distribution of a vulnerable arboreal mammal. Forest Ecol. Manage. 503:119773.
Lindenmayer, D. B. (1997). Differences in the biology and ecology of arboreal marsupials in forests of southeastern Australia. J. Mammal. 78, 1117–1127. doi: 10.1007/s00442-014-2997-4
Lindenmayer, D. B. (2017a). Conserving large old trees as small natural features. Biol. Conserv. 211, 51–59.
Lindenmayer, D. B. (2017b). Halting natural resource depletion: engaging with economic and political power. Econ. Labour Relat. Rev. 28, 41–56.
Lindenmayer, D. B., Blair, D. P., McBurney, L., and Banks, S. C. (2015). Mountain Ash: Fire, Logging and the Future of Victoria’s Giant Forests. Melbourne: CSIRO Publishing.
Lindenmayer, D. B., Blanchard, W., Blair, D., and McBurney, L. (2018a). The road to oblivion – quantifying pathways in the decline of large old trees Forest Ecology and Management. Forest Ecol. Manage. 430, 259–264.
Lindenmayer, D. B., Blanchard, W., Blair, D., McBurney, L., Stein, J., and Banks, S. C. (2018b). Empirical relationships between tree fall and landscape-level amounts of logging and fire. PLoS One 13:e0193132. doi: 10.1371/journal.pone.0193132
Lindenmayer, D. B., McBurney, L., Blair, D., Wood, J., and Banks, S. C. (2018c). From unburnt to salvage logged: quantifying bird responses to different levels of disturbance severity. J. Appl. Ecol. 55, 1626–1636.
Lindenmayer, D. B., Blanchard, W., Blair, D., McBurney, L., and Banks, S. C. (2016). Environmental and human drivers of large old tree abundance in Australian wet forests. Forest Ecol. Manage. 372, 266–235. doi: 10.1016/j.foreco.2016.04.017
Lindenmayer, D. B., Blanchard, W., Blair, D., McBurney, L., and Banks, S. C. (2017a). Relationships between tree size and occupancy by cavity-dependent arboreal marsupials. Forest Ecol. Manage. 391, 221–229. doi: 10.1016/j.foreco.2017.02.014
Lindenmayer, D. B., McBurney, L., Blair, D. P., and Banks, S. C. (2017b). Inter-den tree movements by Leadbeater’s Possum. Aus. Zool. 39, 464–468. doi: 10.7882/AZ.2017.028
Lindenmayer, D. B., Blanchard, W., Blair, D., McBurney, L., Taylor, C., Scheele, B., et al. (2020). The response of arboreal marsupials to long-term changes in forest disturbance. Anim. Conserv. 24, 246–258. doi: 10.1111/acv.12634
Lindenmayer, D. B., Westgate, M. J., Scheele, B. C., Foster, C. N., and Blair, D. P. (2019b). Key perspectives on early successional forests subject to stand-replacing disturbances. Forest Ecol. Manage. 454:117656.
Lindenmayer, D. B., Blanchard, W., Blair, D., Westgate, M. J., and Scheele, B. C. (2019a). Spatio-temporal effects of logging and fire on forest birds. Ecol. Appl. 29:e01999.
Lindenmayer, D. B., Blanchard, W., Bowd, E., McBurney, L., Ashman, K., and Blair, D. (2021). What factors influence the extent and abundance of midstorey Acacia in Mountain Ash forests? Aus. Ecol. 46, 532–544. doi: 10.1111/aec.13002
Lindenmayer, D. B., Blanchard, W., McBurney, L., Blair, D., Banks, S., Likens, G. E., et al. (2012). Interacting factors driving a major loss of large trees with cavities in a forest ecosystem. PLoS One 7:e41864. doi: 10.1371/journal.pone.0041864
Lindenmayer, D. B., Taylor, C., and Bowd, E. (2022d). Interacting fire, logging and climate change has sprung a landscape trap in Victoria’s montane ash forests. Plant Ecology. doi: 10.1007/s11258-021-01217-2
Lindenmayer, D. B., Blanchard, W., McBurney, L., Bowd, E., Youngentob, K., Marsh, K., et al. (2022a). Stand age related differences in forest microclimate. Forest Ecol. Manage. 510:120101. doi: 10.1016/j.foreco.2022.120101
Lindenmayer, D. B., McBurney, L., Blanchard, W., Marsh, K., Bowd, E., Watchorn, D., et al. (2022b). Elevation, disturbance, and forest type drive the occurrence of a specialist arboreal folivore. PLoS One 17:e0265963. doi: 10.1371/journal.pone.0265963
Lindenmayer, D. B., Cunningham, R. B., and Donnelly, C. F. (1997). Decay and collapse of trees with hollows in eastern Australian forests: impacts on arboreal marsupials. Ecol. Appl. 7, 625–641. doi: 10.1890/1051-0761(1997)007[0625:dacotw]2.0.co;2
Lindenmayer, D. B., Cunningham, R. B., Donnelly, C. F., and Franklin, J. F. (2000a). Structural features of old growth Australian montane ash forests. Forest Ecol. Manage. 134, 189–204.
Lindenmayer, D. B., Mackey, B. G., Cunningham, R. B., Donnelly, C. F., Mullen, I. C., McCarthy, M. A., et al. (2000b). Factors affecting the presence of the cool temperate rain forest tree myrtle beech (Nothofagus cunninghamii) in southern Australia: integrating climatic, terrain and disturbance predictors of distribution patterns. J. Biogeograph. 27, 1001–1009. doi: 10.1046/j.1365-2699.2000.00443.x
Lindenmayer, D. B., Cunningham, R. B., Donnelly, C. F., Tanton, M. T., and Nix, H. A. (1993). The abundance and development of cavities in Eucalyptus trees: a case-study in the montane forests of Victoria, southeastern Australia. Forest Ecol. Manage. 60, 77–104.
Lindenmayer, D. B., Cunningham, R. B., and McCarthy, M. A. (1999). The conservation of arboreal marsupials in the montane ash forests of the Central Highlands of Victoria, south-eastern Australia: VIII. Landscape analysis of the occurrence of arboreal marsupials. Biol. Conserv. 89, 83–92.
Lindenmayer, D. B., Cunningham, R. B., Nix, H. A., Tanton, M. T., and Smith, A. P. (1991a). Predicting the abundance of hollow-bearing trees in montane ash forests of southeastern Australia. Aus. J. Ecol. 16, 91–98. doi: 10.1111/j.1442-9993.1991.tb01484.x
Lindenmayer, D. B., Nix, H. A., McMahon, J. P., Hutchinson, M. F., and Tanton, M. T. (1991b). The conservation of Leadbeater’s Possum. Gymnobelideus leadbeateri (McCoy): a case study of the use of bioclimatic modelling. J. Biogeograph. 18, 371–383. doi: 10.2307/2845479
Lindenmayer, D. B., Cunningham, R. B., Tanton, M. T., Smith, A. P., and Nix, H. A. (1990b). The habitat requirements of the Mountain Brushtail Possum and the Greater Glider in the montane ash-type eucalypt forests of the Central Highlands of Victoria. Aus. Wildlife Res. 17, 467–478. doi: 10.1071/wr9900467
Lindenmayer, D. B., Cunningham, R. B., Tanton, M. T., and Smith, A. P. (1990a). The conservation of arboreal marsupials in the montane ash forests of the Central Highlands of Victoria, southeast Australia: II. Biol. Conserv. 54, 133–145. doi: 10.1016/0006-3207(90)90138-f
Lindenmayer, D. B., and Franklin, J. F. (2002). Conserving Forest Biodiversity: A Comprehensive Multiscaled Approach. Washington DC: Island Press.
Lindenmayer, D. B., Hobbs, R. J., Likens, G. E., Krebs, C., and Banks, S. (2011). Newly discovered landscape traps produce regime shifts in wet forests. Proc. Natl.l Acad. Sci. 108, 15887–15891. doi: 10.1073/pnas.1110245108
Lindenmayer, D. B., and Laurance, W. (2017). The ecology, distribution, conservation and management of large old trees. Biol. Rev. 92, 1434–1458. doi: 10.1111/brv.12290
Lindenmayer, D. B., and Likens, G. E. (2018). Effective Ecological Monitoring. Melbourne: CSIRO Publishing.
Lindenmayer, D. B., Mackey, B. G., and Nix, H. A. (1996). The bioclimatic domains of four species of commercially important eucalypts from south-eastern Australia. Aus. Forestr. 59, 74–89. doi: 10.1080/00049158.1996.10674672
Lindenmayer, D. B., and McCarthy, M. (1998). Multi-aged mountain ash forest, wildlife conservation and timber harvesting. Forest Ecol. Manage. 104, 43–56. doi: 10.1016/s0378-1127(97)00231-4
Lindenmayer, D. B., and McCarthy, M. A. (2002). Congruence between natural and human forest disturbance: a case study from Australian montane ash forests. Forest Ecol. Manage. 155, 319–335. doi: 10.1016/s0378-1127(01)00569-2
Lindenmayer, D. B., and Ough, K. (2006). Salvage logging in the montane ash eucalypt forests of the Central Highlands of Victoria and its potential impacts on biodiversity. Conserv. Biol. 20, 1005–1015. doi: 10.1111/j.1523-1739.2006.00501.x
Lindenmayer, D. B., and Sato, C. (2018). Hidden collapse is driven by fire and logging in a socioecological forest ecosystem. Proc. Natl. Acad. Sci. 115, 5181–5186. doi: 10.1073/pnas.1721738115
Lindenmayer, D. B., and Taylor, C. (2020). Extensive recent wildfires demand more stringent protection of critical old growth forest. Pacif. Conserv. Biol. 26, 384–394. doi: 10.1071/PC20037
Luyssaert, S., Schulze, E.-D., Borner, A., Knohl, A., Hessenmoller, D., Law, B. E., et al. (2008). Old-growth forests as global carbon sinks. Nature 455, 213–215. doi: 10.1038/nature07276
Macfarlane, M. A., and Seebeck, J. H. (1991). ““Draft Management Strategies for the Conservation of Leadbeater’s Possum, Gymnobelideus leadbeateri, in Victoria”,” in Technical Report Series 111, Arthur Rylah Institute for Environmental Research, eds M. A. Victoria and J. H. Seebeck (Melbourne: Department of Conservation and Environment).
Mackey, B., Lindenmayer, D. B., Gill, A. M., McCarthy, M. A., and Lindesay, J. A. (2002). Wildlife, Fire and Future Climate: A Forest Ecosystem Analysis. Melbourne: CSIRO Publishing.
MacKinnon, A., and Vold, T. (1998). Old-growth forests inventory for British Columbia. Can. Nat. Areas J. 18, 309–318.
Martin, M., Grondin, P., Lambert, M. C., Bergeron, Y., and Morin, H. (2021). Compared to Wildfire, Management Practices Reduced Old-Growth Forest Diversity and Functionality in Primary Boreal Landscapes of Eastern Canada. Front. Forests Glob. Change 4:639397. doi: 10.3389/ffgc.2021.639397
McDowell, N. G., Allen, C. D., Anderson-Teixeira, K., Aukema, B. H., Bond-Lamberty, B., Chini, L., et al. (2020). Pervasive shifts in forest dynamicsin a changing world. Science 368:964.
Mildrexler, D. J., Berner, L. T., Law, B. E., Birdsey, R. A., and Moomaw, W. R. (2020). Large Trees Dominate Carbon Storage in Forests East of the Cascade Crest in the United States Pacific Northwest. Front. Forests Glob. Change 3:594274. doi: 10.3389/ffgc.2020.594274
Moomaw, W. R., Masino, S. A., and Faison, E. K. (2019). Intact forests in the United States: proforestation mitigates climate change and serves the greatest good. Front. Forests Glob. Change 2:27. doi: 10.3389/ffgc.2019.00027
Mueck, S. G., Ough, K., and Banks, J. C. (1996). How old are wet forest understories? Aus. J. Ecol. 21, 345–348. doi: 10.1111/j.1442-9993.1996.tb00619.x
Office of the Conservation Regulator. (2019). “Old Growth Forest Field Identification Procedure”. Melbourne, Australia: Department of Environment, Land, Water and Planning.
Paritsis, J., Veblen, T. T., and Holz, A. (2015). Positive fire feedbacks contriutes to shifts from Nothofagus pumilio forests to fire-prone shrublans in Patagonia. J. Vegetat. Sci. 26, 89–101. doi: 10.1111/jvs.12225
Phalan, B., Northrup, J. M., Yang, Z., Deal, R. L., Rousseau, J., Soies, T. A., et al. (2019). Impacts of the Northwest Forest Plan on forest composition and bird populations. Proc. Natl. Acad. Sci. U S A. 116, 3322–3327. doi: 10.1073/pnas.1813072116
Roldán-Gómez, J. J., González-Gironda, E., and Barrientos, A. (2021). A Survey on Robotic Technologies for Forest Firefighting: applying Drone Swarms to Improve Firefighters’ Efficiency and Safety. Appl. Sci. 11:363. doi: 10.3390/app11010363
Scotts, D. J. (1991). ““Old-growth forests: their ecological characteristics and value to forest-dependent vertebrate fauna of south-east Australia,”,” in Conservation of Australia’s forest fauna, ed. D. Lunney (Sydney: Royal Zoological Society of N.S.W), 147–159. doi: 10.7882/rzsnsw.1991.014
Shao, G., and Zhao, G. (1998). Protection versus harvest of old growth forests on Changbai Mountain (China and North Korea): a remote sensing application. Nat. Areas J. 18, 358–365.
Sillett, S. C., McCune, B., Peck, J. E., Rambo, T. R., and Ruchty, A. (2000). Dispersal limitations of epiphytic lichens result in species dependent on old-growth forests. Ecol. Appl. 10, 789–799. doi: 10.1890/1051-0761(2000)010[0789:dloelr]2.0.co;2
Smith, A. L., Blair, D., McBurney, L., Banks, S. C., Barton, P. S., Blanchard, W., et al. (2014). Dominant drivers of seedling establishment in a fire-dependent obligate seeder: climate or fire regimes? Ecosystems 17, 258–270. doi: 10.1007/s10021-013-9721-9
Taylor, C., Blair, D., Keith, H., and Lindenmayer, D. B. (2019). Modelling water yields in response to logging and Representative Climate Futures. Sci. the Total Environ. 688, 890–902. doi: 10.1016/j.scitotenv.2019.06.298
Taylor, C., Blanchard, W., and Lindenmayer, D. B. (2020). Does forest thinning reduce fire severity in Australian eucalypt forests? Conserv. Lett. 14:e12766.
Taylor, C., and Lindenmayer, D. B. (2020). Temporal fragmentation of a critically endangered ecosystem. Aus. Ecol. 45, 340–354. doi: 10.1111/aec.12863
Taylor, C., McCarthy, M. A., and Lindenmayer, D. B. (2014). Non-linear effects of stand age on fire severity. Conserv. Lett. 7, 355–370. doi: 10.1111/conl.12122
Thorn, S., Bassler, C., Burton, P., Cahall, R., Campbell, J. L., Castro, J., et al. (2018). Impacts on salvage logging on biodiversity: a meta-analysis. J. Appl. Ecol. 55, 279–289. doi: 10.1111/1365-2664.12945
Tiribelli, F., Morales, J. M., Gowda, J. H., Mermoz, M., and Kitzberger, T. (2018). Non-additive effects of alternative stable states on landscape flammability in NW Patagonia: fire history and simulation modelling evidence. Int. J. Wildland Fire 28, 149–159.
Tulloch, A. I. T., McDonald, J., Cosier, P., Sbrocchi, C., Stein, J., Lindenmayer, D. B., et al. (2018). Using ideal distributions of the time since habitat was disturbed to build metrics for evaluating landscape condition. Ecol. Appl. 28, 709–720. doi: 10.1002/eap.1676
Turner, M. G., Braziunas, K. H., Hansen, W. D., and Harvey, B. J. (2019). Short-interval severe fire erodes the resilience of subalpine lodgepole pine forests. Proc. Natl. Acad. Sci. 116, 11319–11328. doi: 10.1073/pnas.1902841116
van Oldenborgh, G.-J., Krikken, F., Lewis, S., Leach, N. J., Lehner, F., Saunders, K. R., et al. (2021). Attribution of the Australian bushfire risk to anthropogenic climate change. Nat. Haz. Earth Syst. Sci. 21, 941–960.
van Pelt, R. (2007). “Identifying Mature and Old Forests in Western Washington”. Olympia: Washington State Department of Natural Resources.
Vertessy, R. A., Watson, F. G. R., and O’Sullivan, S. K. (2001). Factors determining relations between stand age and catchment water balance in mountain ash forests. Forest Ecol. Manage. 143, 13–26. doi: 10.1016/S0378-1127(00)00501-6
Von Takach Dukai, B., Lindenmayer, D. B., and Banks, S. C. (2018). Environmental influences on growth and reproductive maturation of a keystone forest tree: implications for obligate seeder susceptibility to frequent fire. Forest Ecol. Manage. 411, 108–119.
Vora, R. S. (1994). Integrating old-growth forest into managed landscapes: a Northern great Lakes perspective. Nat. Areas J. 14, 113–123.
Walker, X. J., Baltzer, J. L., Cumming, S. G., Day, N. J., Ebert, C., Goetz, S., et al. (2019). Increasing wildfires threaten historic carbon sink of boreal forest soils. Nature 572, 520–523. doi: 10.1038/s41586-019-1474-y
Watson, J. E., Evans, T., Venter, O., Williams, B., Tulloch, A., Stewart, C., et al. (2018). The exceptional value of intact forest ecosystems. Nat. Ecol. Evol. 2, 599–610. doi: 10.1038/s41559-018-0490-x
Woodgate, P., Peel, W., Ritman, K. T., Coram, J. E., Brady, A., Rule, A. J., et al. (1994). A Study of the Old-Growth Forests of East Gippsland. Melbourne, Australia: Department of Conservation and Natural Resources.
Youngentob, K. N., Wood, J., and Lindenmayer, D. B. (2013). The response of arboreal marsupials to landscape context over time: a large-scale fragmentation study revisited. J. Biogeograph. 40, 2082–2093. doi: 10.1111/jbi.12158
Zald, S. J., and Dunn, C. (2017). Severe fire weather and intensive forest management increase fire severity in a multi-ownership landscape. Ecosphere 28, 1068–1080. doi: 10.1002/eap.1710
Keywords: old growth, forest, forest management, environmental protections, Australia
Citation: Lindenmayer D and Bowd E (2022) Critical Ecological Roles, Structural Attributes and Conservation of Old Growth Forest: Lessons From a Case Study of Australian Mountain Ash Forests. Front. For. Glob. Change 5:878570. doi: 10.3389/ffgc.2022.878570
Received: 18 February 2022; Accepted: 22 April 2022;
Published: 12 May 2022.
Edited by:
Pablo Donoso, Austral University of Chile, ChileReviewed by:
Alvaro G. Gutierrez, University of Chile, ChileFrédérik Doyon, Université du Québec en Outaouais, Canada
Copyright © 2022 Lindenmayer and Bowd. This is an open-access article distributed under the terms of the Creative Commons Attribution License (CC BY). The use, distribution or reproduction in other forums is permitted, provided the original author(s) and the copyright owner(s) are credited and that the original publication in this journal is cited, in accordance with accepted academic practice. No use, distribution or reproduction is permitted which does not comply with these terms.
*Correspondence: David Lindenmayer, David.Lindenmayer@anu.edu.au