- 1Centre for Boreal Research, Northern Alberta Institute of Technology, Peace River, AB, Canada
- 2School of Science and the Environment/Boreal Ecosystem Research Facility, Memorial University of Newfoundland and Labrador, Corner Brook, NL, Canada
Boreal forests across Canada and other geographic areas globally have vast networks or densities of seismic lines, pipelines, access roads, utility corridors, and multipurpose trails collectively termed “linear disturbances” or “linear features.” Additionally, large areas of disturbances attributed to resource harvesting represent a major anthropogenic impact on the global boreal forest ecosystem. Restoration of these disturbed areas is currently a significant component of global boreal forest management strategies. A key to successful restoration or re-vegetation of these disturbed sites is the availability of highly adaptive native planting materials to grow and establish on the disturbed sites, particularly in varying abiotic stressors or severe environmental conditions. Abiotic stress includes non-living environmental factors, including salinity, drought, waterlogging or extreme temperatures, adversely affecting plant growth, development, and establishment on field sites. Herein, we present the concept of nanopriming native boreal seeds with microgram concentrations of carbon nanoparticles (CNPs) as an effective approach to improve the propagation and vigor of native boreal forest species. Priming refers to the technique of hydrating seeds in solutions or in combination with a solid matrix to enhance the rate at which they germinate and their germination uniformity. Seed priming has been shown to increase seed vigor in many plant species. In this mini-review, we will provide a brief overview of the effect of nanopriming on seed germination and seed vigor in agricultural plants and native boreal forest species, indicating the potential future applications of CNPs on native boreal species for use in forest reclamation or restoration.
Introduction
Boreal forest, the world’s largest terrestrial biome, represents significant land areas in Canada, China, Finland, Japan, Norway, Russia, Sweden, and the United States (Larsen, 1980). Boreal forest ecosystems develop under several climate conditions, including a short growing season and freezing winter temperatures (Sykes and Prentice, 1995). These environment factors result in relatively low tree species diversity, with only a few genera being dominant across the biome, e.g., Larix, Pinus, Picea, Populus, Betula, and Alnus (Johnston, 2017). Boreal forests represent the largest pool of living biomass on the terrestrial surface. The aboveground net primary productivity of boreal forest in North America ranges from 25 to 675 g C m–2 y–1, with an annual mean of 296 g C m–2 y–1 (Gower et al., 2001).
Seed germination constitutes a fundamental part of plant growth and development. Some boreal forest species of the Northern hemisphere exhibit deep dormancy, which prevents seeds from germinating when environmental conditions are unfavorable, thus, allowing more seedlings to survive over time (Herranz et al., 2010; Baskin and Baskin, 2014; Hu et al., 2018). Seed dormancy is a mechanism that ensures seeds are able to germinate at times when environmental conditions are ideally suited for germination and seedling establishment (Baskin and Baskin, 2014). As such, germination is often synchronized with appropriate environmental cues, such as temperature, moisture, and photoperiod, to improve the establishment and survival of the germinant (Baskin and Baskin, 2004; Bewley et al., 2013b).
Seeds are known to exhibit different types of dormancy, including physical, morphological, or physiological (Bewley et al., 2013a; Baskin and Baskin, 2014). Physical dormancy is often associated with the inability of the seed to exchange gases and imbibe water due to the presence of militating physical barriers such as an impermeable seed coat. Morphological dormancy is observed in seeds with an immature embryo. Physiological dormancy is characterized by the release of inhibiting hormones, preventing the initiation of germination under different prevailing environmental conditions (Bewley et al., 2013a; Baskin and Baskin, 2014). The hormonal balance of endogenous abscisic acid and gibberellin compositions constitute vital factors inducing the germination of dormant seeds (Finch-Savage and Leubner-Metzger, 2006). A number of native boreal tree species that have been identified as important for land reclamation are challenging to propagate from seeds because of seed dormancy or seedling vigor issues (Smreciu et al., 2013; Ali et al., 2020a,b; Arnott et al., 2021).
For instance, green alder (Alnus viridis) is known to exhibit physiological dormancy with germination rates of less than 55% (Kaur et al., 2016). The embryos of these seeds are characterized by an inability of the radicles to emerge from the seed. This is considered to be a vital factor in this type of dormancy (physiological), which may be influenced by the seed coat or endosperm. Several strategies have been applied to successfully overcome the challenges associated with different seed dormancy of native forest species (e.g., Kaur et al., 2016; Mackenzie and Naeth, 2019; Deng et al., 2021). These include scarification, stratification, hormone treatments, and seed priming methods. Recently, nanotechnology has been demonstrated to be an effective approach to alleviate seed dormancy in several crop species (e.g., Villagarcia et al., 2012; Tiwari et al., 2014; Javed et al., 2021) and a few native boreal forest species (Ali et al., 2020a,b). This mini-review focuses on current studies that have examined the effect of carbon-based nanoparticles (CNPs) on seed germination of crops and native boreal forest species. Finally, the review provides an outlook on potential future applications of CNPs on native boreal species for use in forest reclamation or restoration.
Nanotechnology in the Context of Plant Propagation
Nanotechnology has the potential to revolutionize the way ecologists approach plant propagation to improve plant regeneration during forest reclamation, food, and crop production (Parisi et al., 2015; Servin et al., 2015). NPs used in this sector are atomic or molecular aggregates with dimensions between 1 and 100 nm (Ball, 2002; Roco, 2003). In fact, NPs are prepared directly from natural compounds or synthetic materials by grinding or milling, high-pressure homogenization, and sonication to reduce the particle to nanometer scale (Podsiadlo et al., 2007; Vasquez et al., 2008). The small size of the particles can drastically modify the physicochemical properties of seeds compared to the bulk material (Nel et al., 2006). NPs are also observed to be present in the ecosystem and can originate from a variety of sources. Origins of NPs include manufacturing processes of different industries, treatment and disposal of wastewater, and incorporation of biosolids into the soil (Morales-Díaz et al., 2017). Engineered NPs can be grouped into four primary groups based on the materials used: (1) carbon, (2) metal, (3) dendrimers, and (4) composites (Majhi and Yadav, 2021). However, the ideal NPs used for plant propagation should be economical, eco-friendly, biocompatible, and non-toxic (Liu and Lal, 2015; Mahakham et al., 2016). Plant-based materials are good candidates for synthesizing biocompatible NPs due to the biochemical diversity of plant extracts, non-toxic phytochemical constitutes, non-pathogenicity, low cost, and flexibility in reaction parameters compared to chemically synthesized nanoparticles (Mahakham et al., 2016; Singh et al., 2016).
In recent years, carbon-based NPs (CNPs) (fullerene and carbon nanotubes) have been applied as seed pre-treatment agents to promote seed germination, seedling vigor, and growth in crops (e.g., Villagarcia et al., 2012; Tiwari et al., 2014; Mahakham et al., 2017). CNPs were shown to enter the seed and alter various biological pathways, which modified their physicochemical properties and resulted in different effects on plant growth (Nadiminti et al., 2013). Rapid and uniform seed germination and seedling emergence are essential determinants of successful stand establishment (Rajjou et al., 2012; Chen and Arora, 2013). Seed germination begins with water uptake by the mature dry seed (imbibition) and terminates with the elongation of the embryonic axis, usually the radicle, through the seed envelope, ultimately resulting in the protrusion of root and shoot (Rajjou et al., 2012; Baskin and Baskin, 2014).
Plant responses to treatments with CNPs were observed to vary depending on the chemical composition of the CNPs, concentration, aggregation state (Liu et al., 2009), metabolic abilities of the treated plant species, plant growth environmental conditions (Schultz et al., 2015), and exposure time (Mrakovcic et al., 2013). However, a thorough understanding of the effects induced by CNPs on plant physiology at the molecular level is still lacking. In addition, the methods used for detecting such nanostructures in plant tissues are not well established, and most of them are time-consuming and labor-intensive. Existing detection methods for NPs usually lead to the destruction of samples in the process of detecting the presence of nanomaterials. As a result, the same plant samples cannot be assessed for genomic/proteomic analysis (Khodakovskaya et al., 2011).
Effect of Nanoparticles on the Germination of Agricultural Species
Several studies have demonstrated the beneficial effects of different nanomaterials in improving germination and growth in many agricultural plant species (e.g., Mondal et al., 2011; Smirnova et al., 2012; Javed et al., 2021). CNTs are either single (0.1 μm in length and 1–2 nm in diameter) or multiple (1 mm long and 20 nm in diameter) layers of carbon in a cylindrical arrangement (Li et al., 1996; Lin et al., 2002; Hata et al., 2004; Aslani et al., 2014; Liu et al., 2014). Single-walled carbon nanotubes (SWCNTs) were shown to stimulate germination and enhance the growth of tomato and onion plants. This result was linked to improved gas exchange and water uptake, possibly facilitated by the cylindrical shape of SWCNTs (Haghighi and Teixeira da Silva, 2014). Multi-walled carbon nanotubes (MWCNTs) were also shown to induce water and essential nutrient uptake, thereby enhancing seed germination and plant growth (e.g., Villagarcia et al., 2012; Lahiani et al., 2013; Tiwari et al., 2014). For instance, MWCNTs application by air spray significantly increased the seed germination of valuable crops, such as barley, soybean, and corn, penetrating the seed coat and facilitating the water absorption (Lahiani et al., 2013). While many positive impacts of CNTs on seed germination, seedling vigor, and growth have been reported, several studies have suggested potential negative effects of CNTs due to their toxicity (Pourkhaloee et al., 2011). Pourkhaloee et al. (2011) found the highest concentration (40 mg L–1) of SWCNT in growth medium decreased in Salvia macrosiphon Boiss., and Capsicum annuum L. seedling growth, suggesting the possible toxicity threshold. These negative impacts may be avoided by choosing the best concentrations of CNTs for each species. In fact, the effects of CNTs vary depending on the concentration of CNTs, and more importantly, the plant species used. For instance, Zhang et al. (2017) reported that lower concentration (5 mg L–1) of MWCNTs in growth medium did not show any effect on rice seedlings, whereas 20 mg L–1 of MWCNTs showed the highest average sprout length. Tiwari et al. (2014) found that high concentration (>20 mg L–1) in growth medium could depress the growth of maize. On the other hand, the exposure of maize plants to 50 mg L–1 of Carboxylic acid-functionalized MWCNTs (COOH-MWCNTs) resulted in increases in dry biomass (Zhai et al., 2015).
Effect of Nanoparticles on Seed Germination of Native Boreal Forest Species
Though nanotechnology has been used extensively on crops, to date, this has not been widely tested on native boreal forest species, particularly in the context of improving seed germination, vigor, and growth performance for application in forest reclamation or restoration.
A few studies have looked at the effect of nanoparticles on seed germination of native boreal forest species. Recent studies from the Northern Alberta Institute of Technology (Center for Boreal Research) and the Memorial University (School of Science and the Environment, Grenfell Campus) tested the effectiveness of selected CNPs to improve seed germination and seedling vigor in green alder (Alnus viridis), a native boreal forest species commonly used in land reclamation (Ali et al., 2020a), as well as their effect on two non-dormant boreal species, aspen (Populus tremuloides) and fireweed (Chamerion angustifolium) (Sobze and Thomas, 2022). In these native plant studies, the priming method was used, with nanoparticles as the priming agent. Seed priming is a technique to improve seed germination and seedling vigor by rehydrating seeds and triggering metabolic process during the early phase of germination (Paparella et al., 2015). This technique is widely used because it is both highly effective and inexpensive (Hasanuzzaman and Fotopoulos, 2019). Aspen and fireweed seeds may lose viability quickly or develop into less vigorous seedlings if seeds are not appropriately handled after collection or during storage. Although aspen and fireweed seeds do not require any form of pre-treatment for breaking dormancy, treatment with CNTs may be beneficial in improving seedling vigor, as well as the viability of older seed collections. Improved seedling vigor is crucial because it increases the capacity of the seed to germinate and establish on a range of sites with varying environmental conditions.
Germination of Green Alder (Alnus viridis) Treated With Nanoparticles
The effect of NPs on seed germination of green alder was tested by nanopriming the seeds with various concentrations of graphene, multi-walled carbon nanotube functionalized with COOH-MWCNTs, and non-functionalized MWCNT (Ali et al., 2020a). The authors found that seeds primed with NPs and germinated without stratification had poor germination (less than 40%) but performed better than the control seeds germinated without any form of treatment (10%). In contrast, stratified seeds treated with functionalized COOH-MWCNT produced the best results (90%) followed by those treated with non-functionalized MWCNT (83%) and graphene (72%) (Figure 1). The germination of stratified-control seeds was significantly lower (57%) than those treated with any type of CNTs and stratification. These findings demonstrated that CNTs could improve the germination of dormant boreal seeds. Since stratified seeds treated with CNTs germinated far better (90%) than non-stratified (35%), we assume that NPs significantly contribute to breaking seed dormancy and germination, but should not be used as the only treatment. As reported by other authors (Villagarcia et al., 2012; Tiwari et al., 2014), the NPs may have induced water uptake through the seed coat to enhance breaking seed dormancy and seed germination. Based on these results, cold stratification likely remains essential for breaking the dormancy of green alder seeds. The research team also found no difference between green alder seeds treated with 20 and 40 mg mL–1 of CNTs (Figure 1). This indicates that the effect of CNTs does not increase with their concentration in the solution. However, it is difficult to conclude that 20 mg mL–1 is the optimum concentration since the team did not test the effect of lower concentrations with green alder seeds in this study.
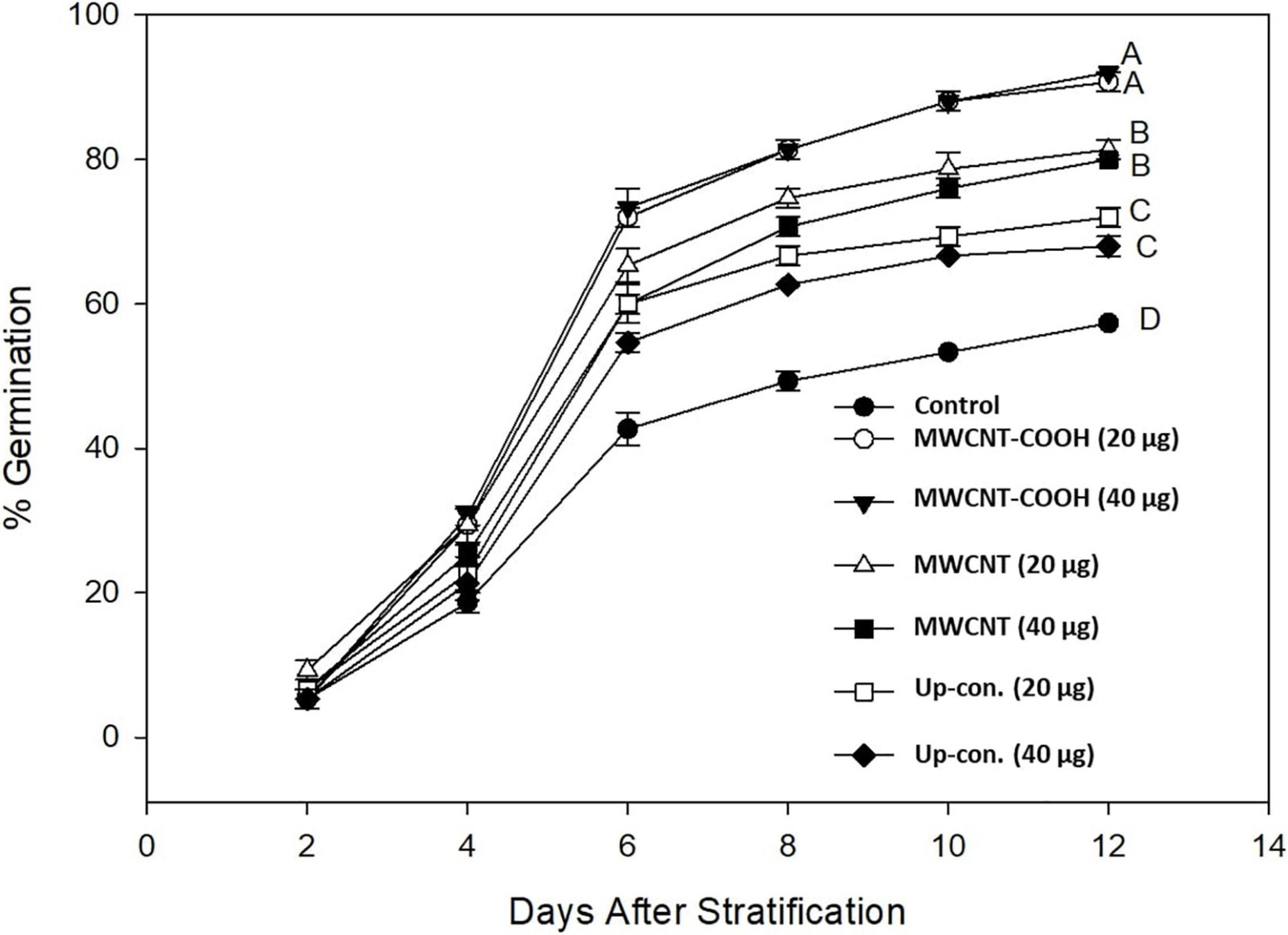
Figure 1. The germination percentage (%) of green alder seeds primed with three concentrations of carbon nanoparticles (control, 20, and 40 μg mL–1). Values represent means ± SE of 4 replicates, consisting of 50 seeds. MWCNT, multi-wall carbon nanotube; MWCNT-COOH, multi-wall carbon nanotube functionalized with the carboxylic group; Up-con. NP, Up-conversion nano phosphors or graphene; Control, seeds imbibed with de-ionized water. Adapted from Ali et al. (2020a), CC-BY 4.0.
Germination and Seedling Vigor of Fireweed (Chamerion angustifolium) and Aspen (Populus tremuloides) Treated With Nanoparticles
Imbibing aspen and fireweed seeds in the presence of 0, 1, 10, 20, and 40 μg mL–1 of various CNPs (graphene, MWCNT, and hydroxide (OH) functionalized MWCNT (OH-MWCNT), or polyethylene glycol (PEG) was observed to be effective in increasing germination (Figure 2) and seedling vigor in fireweed. Concentrations of 1 or 10 μg mL–1 were found to be most effective in improving fireweed growth and germination performance. In aspen, polyethylenglycol (PEG) functionalized MWCNT (PEG-MWCNT) was found to be the most effective in enhancing germination and seedling vigor. The concentration of 40 μg mL–1 gave the best response in aspen seeds imbibed with PEG-MWCNT (Sobze and Thomas, 2022).
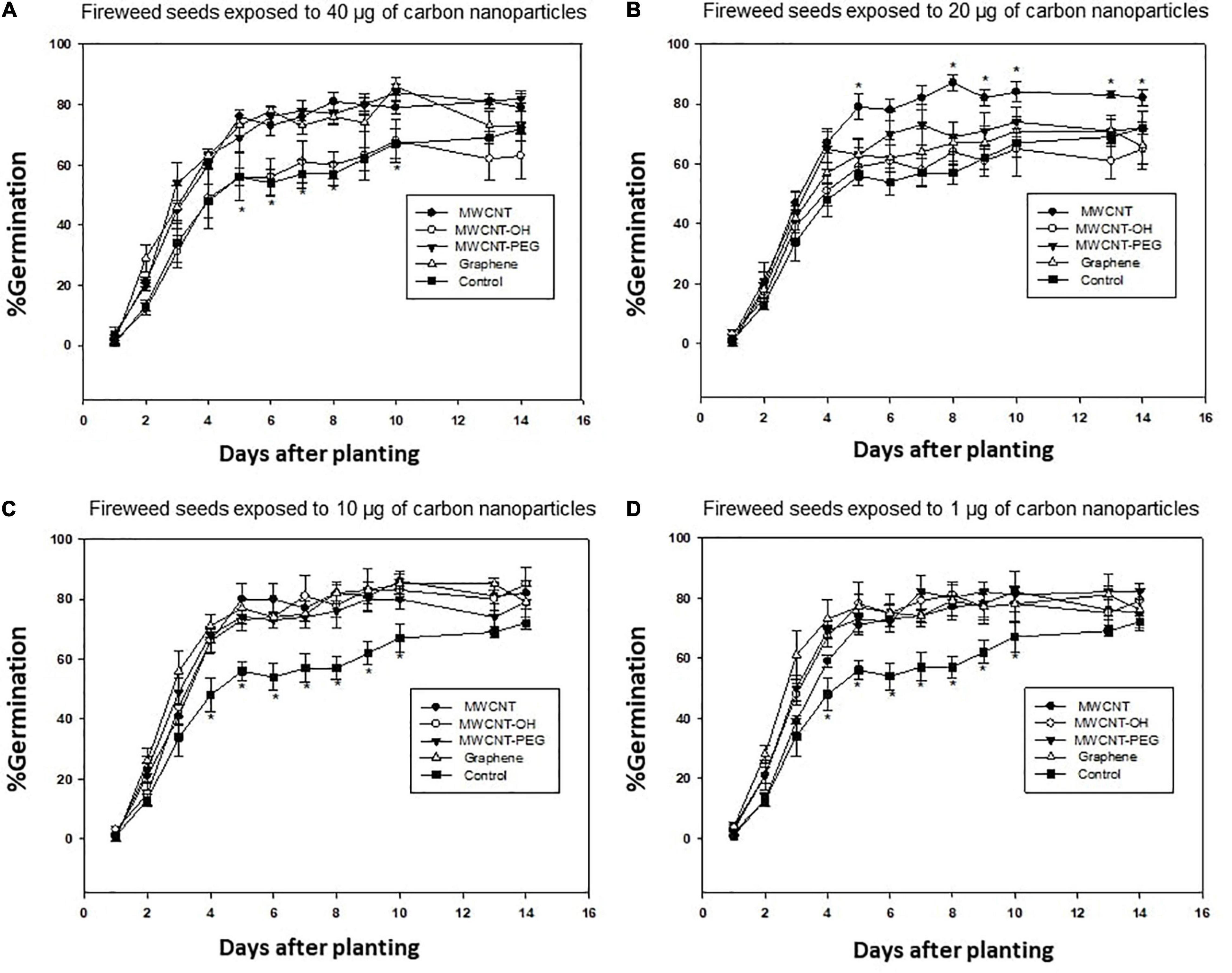
Figure 2. The germination percentage (%) of fireweed seeds primed in different concentrations of carbon nanoparticles: (A) 40μg mL–1, (B) 20μg mL–1, (C) 10μg mL–1, and (D) 1μg mL–1. Values represent means ± SE of 4 replicates, each consisting of 50 seeds. MWCNT, multi-wall carbon nanotube; MWCNT-OH, multi-wall carbon nanotube functionalized with a hydroxyl group; MWCNT-PEG, multi-wall carbon nanotube functionalized with polyethylene glycol. Control, seeds imbibed with de-ionized water. *Represents significant difference at α = 0.05. Adapted from Sobze and Thomas (2022), with permission from Northern Alberta Institute of Technology.
Seed nanopriming with a low concentration of CNTs was an effective technique to enhance germination, seedling vigor, or biomass accumulation in fireweed and aspen. These findings have potential applications in the development of novel seed treatment techniques and plant products to improve the germination rate, growth, and establishment of these species on disturbed boreal forest sites. The data obtained indicate that there is the potential to use CNPs at low concentrations (1–10 μg mL–1) to enhance the establishment of fireweed on disturbed boreal forest sites with varying adverse environmental conditions. For aspen, PEG-MWCNT at 40 μg mL–1 should be used, while for fireweed, 1 or 10 μg mL–1 of either MWCNT, OH-MWCNT, PEG-MWCNT, or graphene should be used.
Discussion
The information presented indicates that selected CNPs treatments significantly improved seed germination in several plant species, including the native boreal forest species, green alder, which has challenging seed dormancy issues with low germination rates (Ali et al., 2020a; Sobze and Thomas, 2022). Anthropogenic disturbance and fragmentation of global forest ecosystems are recognized as major threats to biodiversity and essential ecosystem services. To mitigate any potential environmental and social impacts, it is important to achieve forest establishment within the natural range of ecotypes found in the forest seed zones, capable of supporting wildlife habitats similar to pre-disturbance conditions (Rowland et al., 2009). The challenges associated with native boreal species germination and establishment must be considered for effective reclamation purposes and biodiversity conservation. As such, we believe nanoparticles (NPs) could be an excellent source of improving the germination rate and propagation of many native boreal forest species. General benefits of this technology include a new approach to enhance plant propagation and efficient use of limited native boreal seed resources through an improvement in germination and seedling stock quality. Very low amounts (microgram quantities) of NPs have been shown to confer beneficial effects to the tested plants reported in our study (Ali et al., 2020a; Sobze and Thomas, 2022) and the literature (e.g., Mondal et al., 2011; Smirnova et al., 2012; Javed et al., 2021), indicating this is a very promising technology with low inputs and cost. The ultimate benefit is that select CNPs could be used to produce superior boreal planting materials (seeds and stock plants) ideal for boreal forest reclamation across a range of field sites following industrial disturbances. As a first step, NPs imbibed seeds (aspen and fireweed) should be tested under adverse conditions in greenhouse, growth chambers, and field sites to determine the effectiveness of the improved seed vigor on seed germination, growth, and establishment when exposed to adverse fields or environmental conditions. The size and concentrations of the NPs used to achieve the most effective responses would be an appropriate direction for future work. Moreover, further studies are required with other species not only in boreal regions, but also in other geographical regions, to confirm the effectiveness of NPs on both germination and seedling vigor. For instance, a recent study from the tropics found MWCNTs in the burned wood of native plants, Pinus oocarpa, and P. pseudostrobus, following forest wildfire (Lara-Romero et al., 2017). These MWCNTs produced by wildfire were applied to other native plants such as Eysenhardtia polystachya and found to be effective to speed up its germination time (Juárez-Cisneros et al., 2020). Many fire-adapted plant species are difficult to germinate due to their impermeable and hard-coated seeds (Moreira and Pausas, 2012; Baskin and Baskin, 2014). Applying NPs could possibly increase water uptake during seed imbibition by penetrating seed coat and cell wall, leading to seed germination improvement, which can assist reforestation process and biodiversity conservation.
Author Contributions
All authors listed have made a substantial, direct, and intellectual contribution to the work, and approved it for publication.
Conflict of Interest
The authors declare that the research was conducted in the absence of any commercial or financial relationships that could be construed as a potential conflict of interest.
Publisher’s Note
All claims expressed in this article are solely those of the authors and do not necessarily represent those of their affiliated organizations, or those of the publisher, the editors and the reviewers. Any product that may be evaluated in this article, or claim that may be made by its manufacturer, is not guaranteed or endorsed by the publisher.
References
Arnott, A., Galagedara, L., Thomas, R., Cheema, M., and Sobze, J.-M. (2021). The potential of rock dust nanoparticles to improve seed germination and seedling vigor of native species: a review. Sci. Total Environ. 775:145139. doi: 10.1016/j.scitotenv.2021.145139
Aslani, F., Bagheri, S., Muhd Julkapli, N., Juraimi, A. S., Hashemi, F. S., Baghdadi, A., et al. (2014). Effects of Engineered Nanomaterials on Plants Growth: an Overview. Sci. World J. 2014:e641759. doi: 10.1155/2014/641759
Ali, M. H., Azim, K. F., Somana, S. R., Hasan, M. K., Foysal, M. J., Chowdhury, T. A., et al. (2020a). Carbon Nanoparticles Functionalized with Carboxylic Acid Improved the Germination and Seedling Vigor in Upland Boreal Forest Species. Nanomaterials 10:176. doi: 10.3390/nano10010176
Ali, M. H., Azim, K. F., Somana, S. R., Hasan, M. K., Foysal, M. J., Chowdhury, T. A., et al. (2020b). Carbon Nanotubes Improved the Germination and Vigor of Plant Species from Peatland Ecosystem Via Remodeling the Membrane Lipidome. Nanomaterials 10:1852. doi: 10.3390/nano10091852
Ball, P. (2002). Natural strategies for the molecular engineer. Nanotechnology 13, R15–R28. doi: 10.1088/0957-4484/13/5/201
Baskin, C. C., and Baskin, J. M. (2014). Seeds: Ecology, Biogeography, and Evolution of Dormancy and Germination, 2nd edn. Oxford: Academic Press.
Baskin, J. M., and Baskin, C. C. (2004). A classification system for seed dormancy. Seed Sci. Res. 14, 1–16. doi: 10.1079/SSR2003150
Bewley, J. D., Bradford, K. J., Hilhorst, H. W. M., Nonogaki, H., et al. (2013b). “Environmental Regulation of Dormancy and Germination,” in Seeds: Physiology of Development, Germination and Dormancy, 3rd Edition, ed. J. D. Bewley (New York, NY: Springer), 299–339. doi: 10.1007/978-1-4614-4693-4_7
Bewley, J. D., Bradford, K. J., Hilhorst, H. W. M., Nonogaki, H., et al. (2013a). “Dormancy and the Control of Germination,” in Seeds: Physiology of Development, Germination and Dormancy, 3rd Edition, ed. J. D. Bewley (New York, NY: Springer), 247–297. doi: 10.1007/978-1-4614-4693-4_6
Chen, K., and Arora, R. (2013). Priming memory invokes seed stress-tolerance. Environ. Exp. Bot. 94, 33–45. doi: 10.1016/j.envexpbot.2012.03.005
Deng, S., Deng, Z., Wang, X., Lu, H., and Xue, H. (2021). Effects of Temperature, Scarification, Stratification, Phytohormones, and After-Ripening on the Dormancy and Germination of Eucommia ulmoides Oliv. Seeds. Forests 12:1593. doi: 10.3390/f12111593
Finch-Savage, W. E., and Leubner-Metzger, G. (2006). Seed dormancy and the control of germination. New Phytol. 171, 501–523. doi: 10.1111/j.1469-8137.2006.01787.x
Gower, S. T., Krankina, O., Olson, R. J., Apps, M., Linder, S., Wang, C., et al. (2001). Net Primary Production and Carbon Allocation Patterns of Boreal Forest Ecosystems. Ecol. Appl. 11, 1395–1411.
Haghighi, M., and Teixeira da Silva, J. A. (2014). The effect of carbon nanotubes on the seed germination and seedling growth of four vegetable species. J. Crop. Sci. Biotechnol. 17, 201–208. doi: 10.1007/s12892-014-0057-6
Hasanuzzaman, M., and Fotopoulos, V. (2019). Priming and Pretreatment of Seeds and Seedlings: Implication in Plant Stress Tolerance and Enhancing Productivity in Crop Plants. Singapore: Springer, doi: 10.1007/978-981-13-8625-1
Hata, K., Futaba, D. N., Mizuno, K., Namai, T., Yumura, M., Iijima, S., et al. (2004). Water-Assisted Highly Efficient Synthesis of Impurity-Free Single-Walled Carbon Nanotubes. Science 306, 1362–1364. doi: 10.1126/science.1104962
Herranz, J. M., Ferrandis, P., and Martínez-Duro, E. (2010). Seed germination ecology of the threatened endemic Iberian Delphinium fissum subsp. sordidum (Ranunculaceae). Plant Ecol. 211, 89–106. doi: 10.1007/s11258-010-9775-0
Hu, D., Baskin, J. M., Baskin, C. C., Yang, X., and Huang, Z. (2018). Ecological role of physical dormancy in seeds of Oxytropis racemosa in a semiarid sandland with unpredictable rainfall. J. Plant Ecol. 11, 542–552. doi: 10.1093/jpe/rtx063
Javed, R., Arslan Ahmad, M., Gul, A., Ahsan, T., Cheema, M., et al. (2021). “Chapter Seven - Comparison of chemically and biologically synthesized nanoparticles for the production of secondary metabolites, and growth and development of plants,” in Comprehensive Analytical Chemistry, eds S. K. Verma and A. K. Das (Amsterdam: Elsevier), 303–329. doi: 10.1016/bs.coac.2021.02.002
Johnston, C. A. (2017). “Legacy of Beaver–Human Interaction,” in Beavers: Boreal Ecosystem Engineers, ed. C. A. Johnston (Cham: Springer International Publishing), 1–11. doi: 10.1007/978-3-319-61533-2_1
Juárez-Cisneros, G., Gómez-Romero, M., Campos-Garcia, J., and Villegas, J. (2020). Multi-walled carbon nanotubes produced after forest fires improve germination and development of Eysenhardtia polystachya. PeerJ 8:e8634. doi: 10.7717/peerj.8634
Kaur, J., Schoonmaker, A. L., and Sobze, J.-M. (2016). Length of cold stratification period affects germination in green alder (Alnus viridis (Chaix) DC. subsp. crispa (Aiton) Turrill) seed collected from northwestern Alberta. Native Plants J. 17, 95–102. doi: 10.3368/npj.17.2.95
Khodakovskaya, M. V., de Silva, K., Nedosekin, D. A., Dervishi, E., Biris, A. S., Shashkov, E. V., et al. (2011). Complex genetic, photothermal, and photoacoustic analysis of nanoparticle-plant interactions. Proc. Nat. Acad. Sci. U. S. A. 108, 1028–1033. doi: 10.1073/pnas.1008856108
Lahiani, M. H., Dervishi, E., Chen, J., Nima, Z., Gaume, A., Biris, A. S., et al. (2013). Impact of Carbon Nanotube Exposure to Seeds of Valuable Crops. ACS Appl. Mater. Interfaces 5, 7965–7973. doi: 10.1021/am402052x
Lara-Romero, J., Campos-Garcia, J., Dasgupta-Schubert, N., Borjas-García, S., Tiwari, D., Paraguay-Delgado, F., et al. (2017). Biological effects of carbon nanotubes generated in forest wildfire ecosystems rich in resinous trees on native plants. PeerJ 5:e3658. doi: 10.7717/peerj.3658
Larsen, J. A. (1980). “1 - Introduction: Boreal Ecology and Ecosystems Analysis,” in The Boreal Ecosystem, ed. J. A. Larsen (Cambridge: Academic Press), 1–18. doi: 10.1016/B978-0-12-436880-4.50006-6
Li, W. Z., Xie, S. S., Qian, L. X., Chang, B. H., Zou, B. S., and Zhou, W. Y. (1996). Large-Scale Synthesis of Aligned Carbon Nanotubes. Science 274, 1701–1703. doi: 10.1126/science.274.5293.1701
Lin, Y., Rao, A. M., Sadanadan, B., Kenik, E. A., and Sun, Y. P. (2002). Functionalizing Multiple-Walled Carbon Nanotubes with Aminopolymers. J. Phys. Chem. B 106, 1294–1298. doi: 10.1021/jp013501v
Liu, D., Zhang, Y., Sun, X., and Chang, P. R. (2014). Recent advances in bio-sourced polymeric carbohydrate/nanotube composites. J. Appl. Polym. Sci. 131:40359. doi: 10.1002/app.40359
Liu, J., Aruguete, D. M., Murayama, M., and Hochella, M. F. Jr. (2009). Influence of Size and Aggregation on the Reactivity of an Environmentally and Industrially Relevant Nanomaterial (PbS). Environ. Sci. Technol. 43, 8178–8183. doi: 10.1021/es902121r
Liu, R., and Lal, R. (2015). Potentials of engineered nanoparticles as fertilizers for increasing agronomic productions. Sci. Total Environ. 514, 131–139. doi: 10.1016/j.scitotenv.2015.01.104
Mackenzie, D. D., and Naeth, M. A. (2019). Effect of plant-derived smoke water and potassium nitrate on germination of understory boreal forest plants. Can. J. For. Res. 49, 1540–1547. doi: 10.1139/cjfr-2019-0016
Mahakham, W., Sarmah, A. K., Maensiri, S., and Theerakulpisut, P. (2017). Nanopriming technology for enhancing germination and starch metabolism of aged rice seeds using phytosynthesized silver nanoparticles. Sci. Rep. 7:8263. doi: 10.1038/s41598-017-08669-5
Mahakham, W., Theerakulpisut, P., Maensiri, S., Phumying, S., and Sarmah, A. K. (2016). Environmentally benign synthesis of phytochemicals-capped gold nanoparticles as nanopriming agent for promoting maize seed germination. Sci. Total Environ. 573, 1089–1102. doi: 10.1016/j.scitotenv.2016.08.120
Majhi, K. C., and Yadav, M. (2021). “Synthesis of inorganic nanomaterials using carbohydrates,” in Green Sustainable Process for Chemical and Environmental Engineering and Science, eds Inamuddin Dr. R. Boddula and A. Asiri (Amsterdam: Elsevier), 109–135. doi: 10.1016/B978-0-12-821887-7.00003-3
Mondal, A., Basu, R., Das, S., and Nandy, P. (2011). Beneficial role of carbon nanotubes on mustard plant growth: an agricultural prospect. J. Nanopart. Res. 13:4519. doi: 10.1007/s11051-011-0406-z
Morales-Díaz, A. B., Ortega-Ortíz, H., Juárez-Maldonado, A., Cadenas-Pliego, G., González-Morales, S., and Benavides-Mendoza, A. (2017). Application of nanoelements in plant nutrition and its impact in ecosystems. Adv. Nat. Sci. 8:013001. doi: 10.1088/2043-6254/8/1/013001
Moreira, B., and Pausas, J. G. (2012). Tanned or Burned: the Role of Fire in Shaping Physical Seed Dormancy. PLoS One 7:e51523. doi: 10.1371/journal.pone.0051523
Mrakovcic, M., Absenger, M., Riedl, R., Smole, C., Roblegg, E., and Fröhlich, L. F. (2013). Assessment of Long-Term Effects of Nanoparticles in a Microcarrier Cell Culture System. PLoS One 8:e56791. doi: 10.1371/journal.pone.0056791
Nadiminti, P. P., Dong, Y. D., Sayer, C., Hay, P., Rookes, J. E., and Boyd, B. J. (2013). Nanostructured Liquid Crystalline Particles As an Alternative Delivery Vehicle for Plant Agrochemicals. ACS Appl. Mater. Interfaces 5, 1818–1826. doi: 10.1021/am303208t
Nel, A., Xia, T., Mädler, L., and Li, N. (2006). Toxic Potential of Materials at the Nanolevel. Science 311, 622–627. doi: 10.1126/science.1114397
Paparella, S., Araújo, S. S., Rossi, G., Wijayasinghe, M., Carbonera, D., and Balestrazzi, A. (2015). Seed priming: state of the art and new perspectives. Plant Cell Rep. 34, 1281–1293. doi: 10.1007/s00299-015-1784-y
Parisi, C., Vigani, M., and Rodríguez-Cerezo, E. (2015). Agricultural Nanotechnologies: what are the current possibilities? Nano Today 10, 124–127. doi: 10.1016/j.nantod.2014.09.009
Podsiadlo, P., Kaushik, A. K., Arruda, E. M., Waas, A. M., Shim, B. S., and Xu, J. (2007). Ultrastrong and Stiff Layered Polymer Nanocomposites. Science 318, 80–83. doi: 10.1126/science.1143176
Pourkhaloee, A., Haghighi, M., Saharkhiz, M. J., Jouzi, H., and Doroodmand, M. M. (2011). Carbon Nanotubes Can Promote Seed Germination via Seed Coat Penetration. Seed Technol. 33, 155–169.
Rajjou, L., Duval, M., Gallardo, K., Catusse, J., Bally, J., and Job, C. (2012). Seed Germination and Vigor. Annu. Rev. Plant Biol. 63, 507–533. doi: 10.1146/annurev-arplant-042811-105550
Roco, M. C. (2003). Broader Societal Issues of Nanotechnology. J. Nanopart. Res. 5, 181–189. doi: 10.1023/A:1025548512438
Rowland, S. M., Smith, D. F., Blakney, G. T., Corilo, Y. E., Hendrickson, C. L., and Rodgers, R. P. (2009). Recreating a functioning forest soil in reclaimed oil sands in northern alberta: an approach for measuring success in ecological restoration. J. Environ. Qual. 38, 1580–1590. doi: 10.2134/jeq2008.0317
Schultz, C., Powell, K., Crossley, A., Jurkschat, K., Kille, P., and Morgan, A. J. (2015). Analytical approaches to support current understanding of exposure, uptake and distributions of engineered nanoparticles by aquatic and terrestrial organisms. Ecotoxicology 24, 239–261. doi: 10.1007/s10646-014-1387-3
Servin, A., Elmer, W., Mukherjee, A., De La Torre-Roche, R., Hamdi, H., White, J. C., et al. (2015). A review of the use of engineered nanomaterials to suppress plant disease and enhance crop yield. J. Nanopart. Res. 17:92. doi: 10.1007/s11051-015-2907-7
Singh, P., Kim, Y. J., Zhang, D., and Yang, D. C. (2016). Biological Synthesis of Nanoparticles from Plants and Microorganisms. Trends Biotechnol. 34, 588–599. doi: 10.1016/j.tibtech.2016.02.006
Smirnova, E., Gusev, A., Zaytseva, O., Sheina, O., Tkachev, A., and Kuznetsova, E. (2012). Uptake and accumulation of multiwalled carbon nanotubes change the morphometric and biochemical characteristics of Onobrychis arenaria seedlings. Front. Chem. Sci. Eng. 6:132–138. doi: 10.1007/s11705-012-1290-5
Smreciu, A., Gould, K., and Wood, S. (2013). Boreal Plant Species for Reclamation of Athabasca Oil Sands Disturbances - Updated December 2014. Edmonton, Alberta: University of Alberta, doi: 10.7939/R3MC8RK9B
Sobze, J.-M., and Thomas, R. (2022). The Use of Nanomaterials to Improve Propagation of Native Boreal Species. Technical Note #46. Northern Alberta Institute of Technology, Centre for Boreal Research. Available online at: https://publicdocs.nait.ca/sites/pd/_layouts/15/docidredir.aspx?ID=4NUSZQ57DJN7-208515216-21514
Sykes, M. T., and Prentice, I. C. (1995). Boreal forest futures: modelling the controls on tree species range limits and transient responses to climate change. Water Air Soil Pollut. 82, 415–428. doi: 10.1007/BF01182851
Tiwari, D. K. N., Dasgupta-Schubert, L. M., Villaseñor Cendejas, J., Villegas, L., Carreto Montoya, S. E., and Borjas García, B. (2014). Interfacing carbon nanotubes (CNT) with plants: enhancement of growth, water and ionic nutrient uptake in maize (Zea mays) and implications for nanoagriculture. Appl. Nanosci. 4, 577–591. doi: 10.1007/s13204-013-0236-7
Vasquez, Y., Henkes, A. E., Bauer, J. C., and Schaak, R. E. (2008). Nanocrystal conversion chemistry: a unified and materials-general strategy for the template-based synthesis of nanocrystalline solids. J. Solid State Chem. 181, 1509–1523. doi: 10.1016/j.jssc.2008.04.007
Villagarcia, H., Dervishi, E., de Silva, K., Biris, A. S., and Khodakovskaya, M. V. (2012). Surface Chemistry of Carbon Nanotubes Impacts the Growth and Expression of Water Channel Protein in Tomato Plants. Small 8, 2328–2334. doi: 10.1002/smll.201102661
Zhai, G., Gutowski, S. M., Walters, K. S., Yan, B., and Schnoor, J. L. (2015). Charge, Size, and Cellular Selectivity for Multiwall Carbon Nanotubes by Maize and Soybean. Environ. Sci. Technol. 49, 7380–7390. doi: 10.1021/acs.est.5b01145
Keywords: seed nanopriming, carbon nanoparticles, seed germination, seed vigor, growth, native boreal tree species
Citation: Sobze J-M, Galagedara L, Cheema M, Thomas R and Inoue S (2022) The Potential of Carbon Nanoparticles as a Stimulant to Improve the Propagation of Native Boreal Forest Species: A Mini-Review. Front. For. Glob. Change 5:872780. doi: 10.3389/ffgc.2022.872780
Received: 09 February 2022; Accepted: 27 April 2022;
Published: 31 May 2022.
Edited by:
Sean Michael Gleason, Agricultural Research Service (USDA), United StatesReviewed by:
Wuttipong Mahakham, KhonKaen University, ThailandPratibha Acharya, Benson Hill Biosystems, Inc., United States
Copyright © 2022 Sobze, Galagedara, Cheema, Thomas and Inoue. This is an open-access article distributed under the terms of the Creative Commons Attribution License (CC BY). The use, distribution or reproduction in other forums is permitted, provided the original author(s) and the copyright owner(s) are credited and that the original publication in this journal is cited, in accordance with accepted academic practice. No use, distribution or reproduction is permitted which does not comply with these terms.
*Correspondence: Sahari Inoue, c2lub3VlQGxha2VoZWFkdS5jYQ==