- 1Hawkesbury Institute for the Environment, Western Sydney University, Penrith, NSW, Australia
- 2Centre for Carbon, Water and Food, School of Life and Environmental Sciences, The University of Sydney, Camden, NSW, Australia
Root trait plasticity can facilitate plant adjustment to water shortages, but the impact of altered traits on belowground carbon (C) cycling is mostly unknown. While drought and nutrient availability can alter root morphological and chemical traits that may affect root decomposition, direct assessments of drought mediated changes on decomposability are not available. We exposed four tree species contrasting in drought stress tolerance and root traits to three dry-down and recovery periods (over 5 months after 11 months of growth in well-watered conditions) under high and low nutrient conditions. We then assessed early stage root decomposability in relation to their morphology and chemistry as well as implications for CO2 release when accounting for effects on root biomass. While each species showed a unique set of responses, drought generally reduced root diameter and increased nitrogen concentration. We found limited evidence that morphological responses to drought were counteracted by high nutrient supply. Results indicated that the degree of association between morphological and nutrient root trait responses to drought and decomposability varied with different species. However, across these contrasting woody species, drought-induced increases in nitrogen and phosphorus concentrations were associated with drought-induced increases in early stage root decomposability. When accounting for changes in root biomass, estimated overall C loss through root decomposition increased with drought stress. Our experimental results demonstrate that changes in tree root traits with drought can enhance C loss via root decomposition, and with other factors being equal, drought may potentially contribute to a positive feedback to climate change. Our findings contribute empirical evidence to help disentangle the multiple factors involved in root contribution to C balances at the ecosystem level.
Introduction
Plant root responses to climate change can impact ecosystem carbon (C) cycling in highly diverse ways that are just beginning to be understood (Bardgett et al., 2014; Bardgett, 2017). Among climate events, droughts are presumed to have among the strongest impacts on terrestrial C cycling (Frank et al., 2015). Reduced precipitation frequency and amounts, and longer dry periods (drought), are expected to increase plant stress particularly in mid-latitudes (IPCC, 2013). These changes in precipitation regimes are particularly poised to impact roots via shifts in both water and nutrient availability in soil. Root traits mediate plant productivity under drought (Comas et al., 2013) and root trait shifts can help support plants adjust to water and associated nutrient shortages (Pierik and Testerink, 2014). Root-mediated impacts on plant performance can impact ecosystem C cycling via aboveground C exchange; however, root responses can also have direct effects on belowground C cycling.
Roots traits are major drivers of soil C sequestration and balance while living but also after death (Faucon et al., 2017). Living roots deposit C into their vicinity, influencing the decomposition of surrounding soil organic matter (Cheng et al., 2014) and providing a source of C for microbial C assimilation and stabilization and soil C formation (Sokol et al., 2019). Once roots die, however, their impacts on C cycling continue as they become substrates for decomposition and C release to the atmosphere, and sources for soil C formation. The effects of climate change-driven shifts in water and nutrient availability on morphological, chemical or architectural root traits have potential to modify these belowground processes. To understand and model the mechanisms by which climate change can affect ecosystem C balance and potential feedbacks to climate, it is necessary to tease apart its impact on individual processes. Here we focus on the impacts of water and nutrient availability on root chemistry, morphology and their relation to root decomposability and C release. Studies of drought impacts on decomposition and climate feedback mechanisms have largely focused on direct effects of moisture on microbial activity and ecosystem respiration, leaving the indirect responses via changes in plant root traits as a large gap in our knowledge.
Responses of root morphology to reductions in water availability have been assessed by various studies and while some common responses have been observed, high variation exists among studies, species and functional groups. A recent synthesis of 128 field studies found overall trends for decreased root biomass, root length and root length density and increased root diameter with drought (Zhou et al., 2018). In contrast, in another meta-analysis, Zhang et al. (2019) observed decreased diameter with reduced precipitation (not necessarily to drought levels). Specifically for woody species, Zhou et al. (2018) found increased specific root length but decreased overall root length with no effect on root diameter. Wang et al. (2020) observed that decreased precipitation reduced fine root biomass of herbaceous but not woody species. In a study focused on woody species, Olmo et al. (2014) found increases in specific root length and tissue density, but in contrast to Zhou et al. (2018), they found decreases in root diameter. In addition to changes in morphology, the chemistry, including elemental composition of roots, can also be impacted by drought stress. In a summary of observed and expected responses to drought, Brunner et al. (2015) noted that root C and phosphorus (P) concentrations could decrease, while nitrogen (N) could increase. Olmo et al. (2014), however, saw a clear decrease in N concentration in woody species. Root responses thus appear to differ for woody plants, and may further vary with ecological strategies to cope with drought (Nikolova et al., 2020).
Changes in root morphology and nutrient content with drought are likely highly interrelated and also dependent on nutrient availability. Changes in root morphology with drought can facilitate plant access to water, but also nutrients, as nutrient access is limited by water availability (Brunner et al., 2015; Zhou et al., 2018). Indeed, there are common root physiological responses to water and nutrient availability (Kudoyarova et al., 2015), and nutrient supplementation is used as a means to ameliorate impacts of drought (Bardhan et al., 2021). While nutrient supplementation could be expected to counteract impacts of drought on nutrient content, it may also counteract impacts on morphology. Direct manipulations to assess this dependency and its impacts on root decomposition are needed, particularly in woody species.
Root morphology and nutrient and C chemistry can influence root susceptibility to decomposition (decomposability). Nutrient-related traits have been frequently studied and appear to regulate root decomposability across large suites of species. Root decomposability increases with N concentration (Roumet et al., 2016; See et al., 2019; Bonanomi et al., 2021; Jiang et al., 2021) and P concentration (Smith et al., 2014; See et al., 2019; Jiang et al., 2021). C traits, particularly the concentration of acid unhydrolizable compounds including lignin and phenolics, have been found to be a good predictor of root decomposition (Prieto et al., 2016; Roumet et al., 2016; See et al., 2019; Bonanomi et al., 2021; Jiang et al., 2021). Morphological traits have been less commonly studied and patterns are less clear. For example, thinner roots can lead to faster or slower decomposition depending on the stage of decay (Hobbie et al., 2010) or on mycorrhizal type (Jiang et al., 2021). Decomposition has been found to increase with specific root length (Roumet et al., 2016), but decrease with specific root area (Smith et al., 2014). Some of the discrepancies may arise from the differences between woody and herbaceous growth forms, as controls on their root decomposition are likely to differ (See et al., 2019). As drought drives within-species variation in various root traits, changes in decomposability could be expected, but it is unknown if responses vary across species.
Understanding the effects of climate factors on plant traits and their susceptibility to decomposition is critical to understand impacts on the C balance (Cornwell et al., 2008; Laliberté, 2017). Very few studies have directly assessed how particular climate factors shape decomposability of roots. Warming and elevated CO2 increased decomposability in grasslands (Sindhoj et al., 2000; Nelson et al., 2017), while long-term rainfall exclusion increased decomposability in a Mediterranean forest (Garcia-Palacios et al., 2016). In these studies, the mechanisms by which the climate factor affected decomposability remain unclear. The missing link is how the impact of drought and other climate factors on root morphology and chemistry subsequently impacts root decomposability and resulting C release. Predictions of decomposability based on expected impacts of drought on root traits may be imprecise as they require assumptions of the direction of responses; furthermore, different trait responses may cause counteracting impacts. Brunner et al. (2015) proposed that the expected decreases in root diameter with drought could potentially enhance their decomposability. However, very fine roots of tree species decomposed more slowly than thicker roots (Sun et al., 2013), which suggests that the expectation of higher decomposability of thinner, longer roots may not apply. Similarly, the impacts of a stress such as drought on chemical recalcitrance of tissues would be expected to reduce decomposability (Suseela and Tharayil, 2018); however, the few observations available indicate higher decomposability with warming and drought (Garcia-Palacios et al., 2016; Nelson et al., 2017). Direct assessment of drought-mediated changes on decomposability are not available. Moreover, to scale these effects, it is necessary to also consider the impact of drought on root biomass, as overall effects on root C loss via decomposition and C remaining in soil will depend on the balance between impacts on decomposability of the tissues and impacts on standing biomass stocks.
In order to address these knowledge gaps, we asked: (1) What are the main and combined impacts of drought and nutrient availability on tree root morphology, chemistry and decomposability?; and (2) What is the effect of changes in decomposability combined with changes in biomass on the overall release of CO2? For this we exposed four tree species from different functional groups, differing in drought stress tolerance and root traits, to periodic water shortage under high and low nutrient conditions. We expected that the degree of chemical, morphological and biomass response of roots would depend on the intrinsic drought stress tolerance of species. Specifically, we hypothesized that for these woody species (1) drought would increase specific root length (longer roots per unit mass) and tissue density and decrease root diameter and N concentration, but that these effects would be counteracted by high nutrient availability, which would reduce stress in terms of nutrient access; (2) thinner and longer roots associated with drought would be more easily decomposable, thereby releasing more C as CO2 per unit of root biomass, but if drought decreased N content this effect would be counteracted, and (3) the enhanced release of C back to the atmosphere with increased tissue decomposition would be balanced by the reduction of root biomass with drought.
Materials and Methods
Experimental Design
The study utilized the rainout shelter facility at Western Sydney University, Richmond, NSW, Australia (long. 150°44′23″E, lat. 33°36′39″S, mean annual temperature = 17°C and mean annual precipitation = 730 mm). The facility comprises 6 rainout shelters (12 m long × 8 m wide × 7 m tall), each possessing a light-transparent retractable roof and curtains which close automatically when rainfall is detected, preventing rain from falling into the shelter (Drake et al., 2017) and thus allowing full control of water supply. A 1 m gap at the bottom of the shelter maintains air flow during rainfall. Following rainfall events, the curtains retract so that temperature and humidity return to ambient field conditions.
Four evergreen tree species were selected to be contrasting in terms of water use. Casuarina cunninghamiana (CACU, N-fixer), is a native evergreen tree found in high water availability sites; Pinus radiata (PIRA) is an exotic gymnosperm with high drought tolerance; Eucalyptus tereticornis (EUTE) is a widespread native along the Australian Eastern coast, where seasonal drought is naturally common; Eucalyptus sideroxylon (EUSI) also native, is typically slower-growing and expected to be more tolerant to drought than EUTE (Drake et al., 2017). In November 2011 5-month old seedlings were planted in 75 L bags filled with 90-100 kg of field soil (one seedling per bag). The soil was a local loamy sand (pH = 6.2, 14 g kg–1 organic C, 1.2 g kg–1 total N, and 0.24 g kg–1 total P). When plants died during the first month they were replaced with spare seedlings. In each of the six shelters there were 4 bags of each species for each of 4 treatments: low water and low nutrient supply (LW-LN), low water and high nutrient supply (LW-HN), high water and low nutrient supply (HW-LN), and high water and high nutrient supply (HW-HN). Thus, 4 species × 2 water treatments × 2 nutrient treatments × 6 replicates (one in each shelter) amounted to 96 trees in total.
All bags received N, P, K, and S fertilizer (Nitrophoska Special®) 6 months after transplanting. Bags with high nutrient supply received additional fertilizer at 10 and 13 months (for details of fertilization regime see Dijkstra et al., 2016). A total of 9.0, 3.7, 10.4, and 5.8 g m–2 of N, P, K, and S were added to the bags with the high nutrient supply treatment, which was 4 times the amount added to the bags with the low nutrient supply treatment.
For the first 11 months of growth since transplanting (until October 2012), soil moisture was maintained at field capacity in all bags by watering bags on a daily basis. At this point (the Austral Spring-Summer), the low water availability treatment was implemented as three periods of reduced water. Bags with low water supply received reduced water during the period 8 October–2 November 2012 (first dry-down), 19 November–21 December 2012 (second dry-down), and after 28 January 2013 until drought-induced plant mortality (third dry-down). The dry-down periods involved passive drying to a minimum target volumetric content of 0.05 m3 m–3 followed by controlled re-wetting to field capacity, while during the final dry down no water was added (Drake et al., 2017). On all other dates, soil moisture was maintained at conditions similar to the high water supply treatment. Volumetric soil moisture was monitored for each species in the low and high water supply treatments with high nutrient availability (Dijkstra et al., 2016).
Sampling and Analyses
After 16 months of growth, in late March 2013, during the final dry down period before plant death, a 5-cm diameter, 20-cm core was taken from each bag. All roots in the core were hand-picked and refrigerated and total dry weight of soil in the core was measured. To evaluate root morphology, all roots were washed and arranged for digital scanning and images analyzed automatically with WinRhizo Pro software (Regents Instruments, Inc., Quebec) for length, diameter, volume, surface area and branching. Scanned roots were then washed, dried and weighed. We assessed root average diameter (mm), specific root length as the ratio of root length to root dry mass (SRL; cm mg–1), root tissue density as mass per unit volume (RTD; g cm3), specific surface area as the ratio of area to dry mass (SSA; cm2 g–1), and branching as the number of forks per unit of mass (number mg–1). Following morphological assessment a subsample was ground for C, N, and P analysis. C and N were determined with Dumas combustion using a Vario EL Cube CHNOS Elemental analyzer, Hanau, Germany. P was determined via Kjeldahl digestion using 3 mL pure H2SO4 and 2 mL H2O2, 30% and a Berghof’s microwave digester with speed wave 4. P concentrations of the Kjeldahl digests were colorimetrically analyzed at 880 nm after a molybdate reaction in a discrete analyzer (AQ2, SEAL Analytical, Ltd., Milwaukee, WI, United States and EPA135 method).
All remaining roots were later incubated in the laboratory to quantify decomposition measured as CO2 production. For incubation, dried roots were homogenized by cutting into < 1 cm length and mixed. A subsample of 60 mg of roots was mixed with 30 g of autoclaved and oven-dried sand (1:500 ratio by weight) in plastic cups. Plastic cups were placed in gas 400 ml air-tight jars for incubation with 20 ml of water in the bottom to maintain humidity. To initiate incubations, root-sand mixes were inoculated with 3 ml of soil slurry (1:10 soil:water, 250 μm filtered and previously kept at 25°C for 3 days to activate microbial activity). Soil used to produce the slurry was an even mix of air-dried soils generated by combining subsamples from all field soil cores from all bags. This approach allowed us to fully isolate the experimental impacts on root morphology and remove impacts on soil microbial communities, while still using the native soil microbial community. In 17 samples, for which there was less than 60 mg roots available, the ratio of root to sand to slurry was adjusted proportionally. Immediately after inoculum addition, jars were ventilated outdoors to bring CO2 concentrations to atmospheric conditions, capped and kept at 25°C. Three blank jars (all components minus the roots and inoculum) were maintained in parallel. Rate of CO2 release was estimated at day 3, samples quickly ventilated again, capped and sampled again at day 5 and this was repeated at day 16. CO2 was measured in a 12 ml headspace subsample transferred with airtight syringes to pre-evacuated gas chromatography vials. CO2 was measured on an Agilent Technologies G1888 greenhouse gas analyzer.
Statistical Analysis
We used ANOVA to test for effects of species, water supply and nutrient supply and their interactive effects on root biomass and morphological and chemical variables. The rain out shelter was included as a blocking factor, but it did not explain a significant amount of variation. Repeated measures ANOVA was used to assess impacts of treatments on decomposition over the incubation time. No interactive effects of time were detected so the CO2 release over the three incubation periods were combined into a cumulative release across the full incubation and divided by the number of days to calculate mean decomposition rate. Three-way ANOVA was used for mean decomposition rate as for root biomass, chemistry and morphology. We used Pearson correlation to explore relationships between root morphology and chemistry with decomposition for individual species. To investigate the association of the impacts of water availability on root traits with those of root decomposition across species we used principal component analysis on the responses of all variables to water availability. Responses were calculated as the ratio between the value of each variable at LW over HW for each species and each nutrient condition in each rainout shelter (4 species × 2 nutrient conditions × 6 shelters).
Results
Root Biomass and Morphology
Root biomass was highest in CACU, and similar across other species, while PIRA exhibited large root diameter and low specific root length. Biomass significantly decreased under low nutrients (LN) in all species, but particularly EUTE, where it was reduced by 88% (Figure 1A and Table 1). Root biomass was not affected by water availability. In contrast, all morphological parameters responded to water availability but the combination of variables affected were dependent on species or nutrient availability. Root diameter was reduced under low water availability (LW) across all species (Figure 1B and Table 1) but this effect was more marked in EUSI and PIRA (marginally significant Species by Water interaction; Table 1). LW increased SRL in EUSI (Figure 1C and Table 1). RTD was increased by LW in CACU and PIRA, but decreased with LN overall (Figure 1D and Table 1). SSA and branching showed interactive effects of species by water and by nutrients. SSA increased in LW for EUSI and EUTE, but only under LN in EUTE; in contrast, LW reduced SSA in CACU and did not impact PIRA (Figure 1E and Table 1). LW increased branching (# forks per unit of mass) in EUSI and PIRA, when under LN for the later and LN reduced branching for EUSI and PIRA, when under HW for the later (Figure 1F and Table 1).
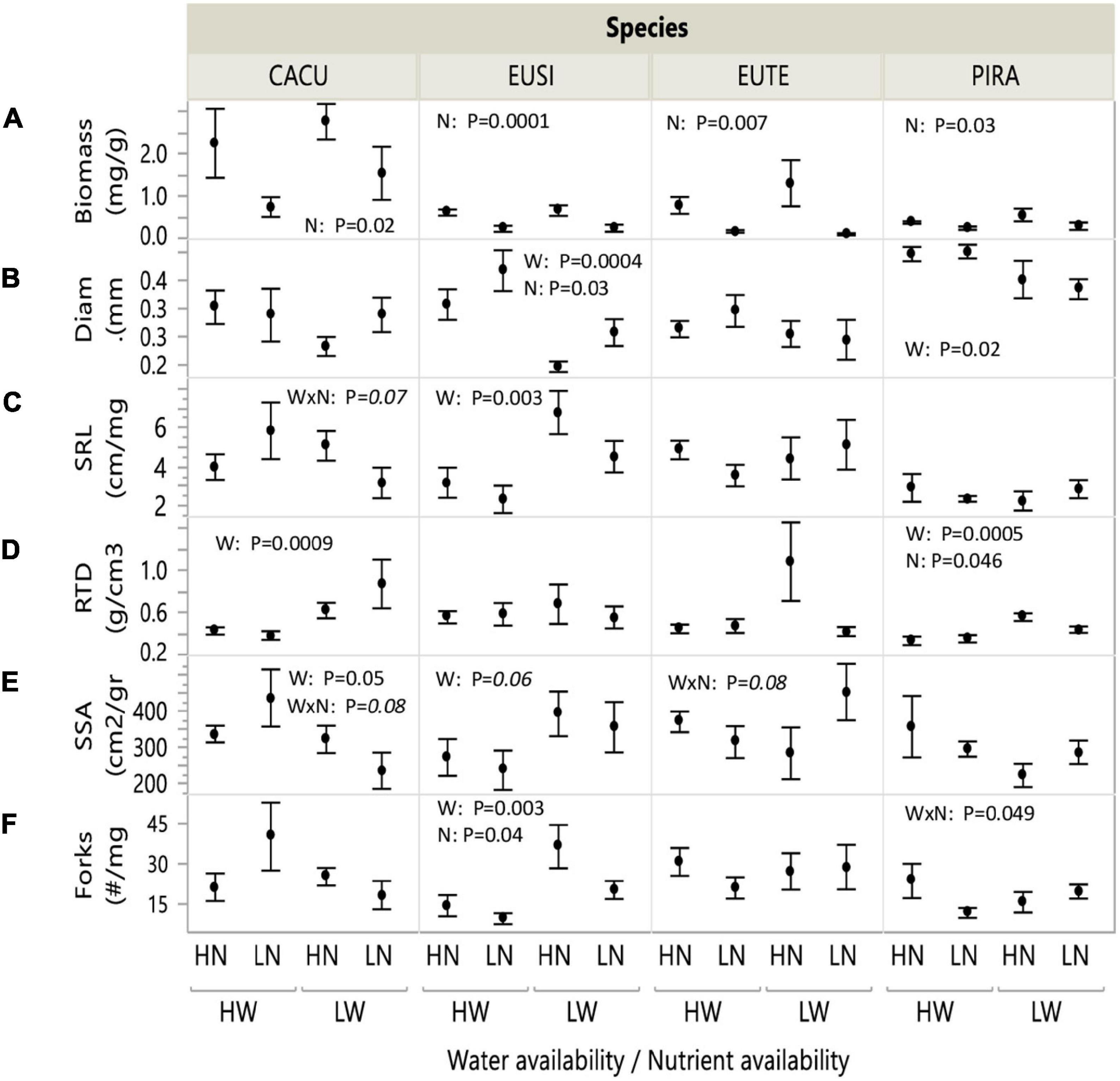
Figure 1. Root biomass and morphological parameters in response to water and nutrient availability treatments. Water (W): Low/High water availability (LW/HW), Nutrients (N): Low/High nutrient availability (LN/HN). (A) Biomass; (B) diameter; (C) specific root length (SRL); (D) root tissue density (RTD); (E) specific surface area (SSA); (F) number of forks (branching points). Data points represent averages across shelters/replicates with standard error (n = 6). P-values from two-way ANOVA with interactions for each species. Italics indicate marginal significance. See Table 1 for analysis across species. CACU, Casuarina cunninghamiana; EUSI, Eucalyptus sideroxylon; EUTE, Eucalyptus tereticornis; PIRA, Pinus radiata.
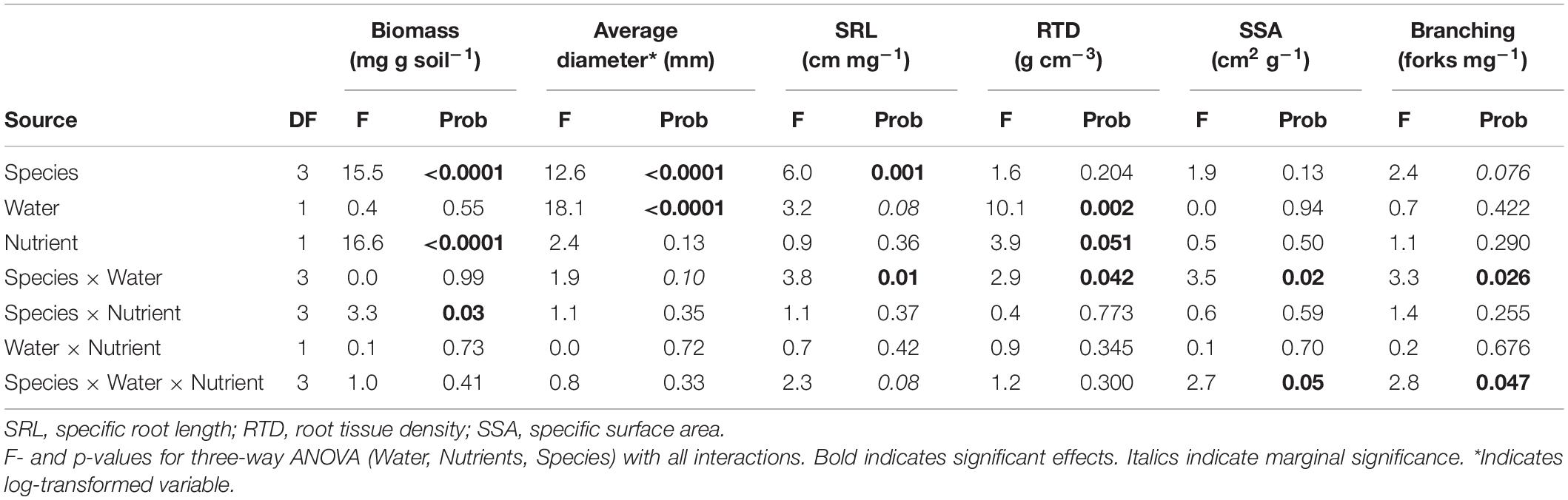
Table 1. Results from statistical analysis of impacts of water and nutrient availability across plant species on root biomass and morphological parameters.
Root Chemistry
Root chemistry contrasted significantly across species (Figure 2, and Table 2). CACU and PIRA had the highest N concentration and consequently the lowest C/N ratios; thus, the eucalypts (EUSI and EUTE) had the lowest N concentration. PIRA showed the highest P concentration and EUSI the lowest. EUTE had the lowest N/P ratio. Root chemistry responded to water and nutrient availability but there were no interactive effects of water and nutrients. Reduced water availability (LW) led to higher N concentration across species (12% increase on average, but with a stronger effect on EUTE and PIRA) and thus lower C/N ratios (main effect of Water, Table 2). An increase of P concentration with LW of 9% on average was marginally significant, but occurred in all species except EUSI. Root N was reduced under LN, especially for EUSI, generating a higher C/N ratio (Species by Nutrient interaction, Table 2 and Figure 2).
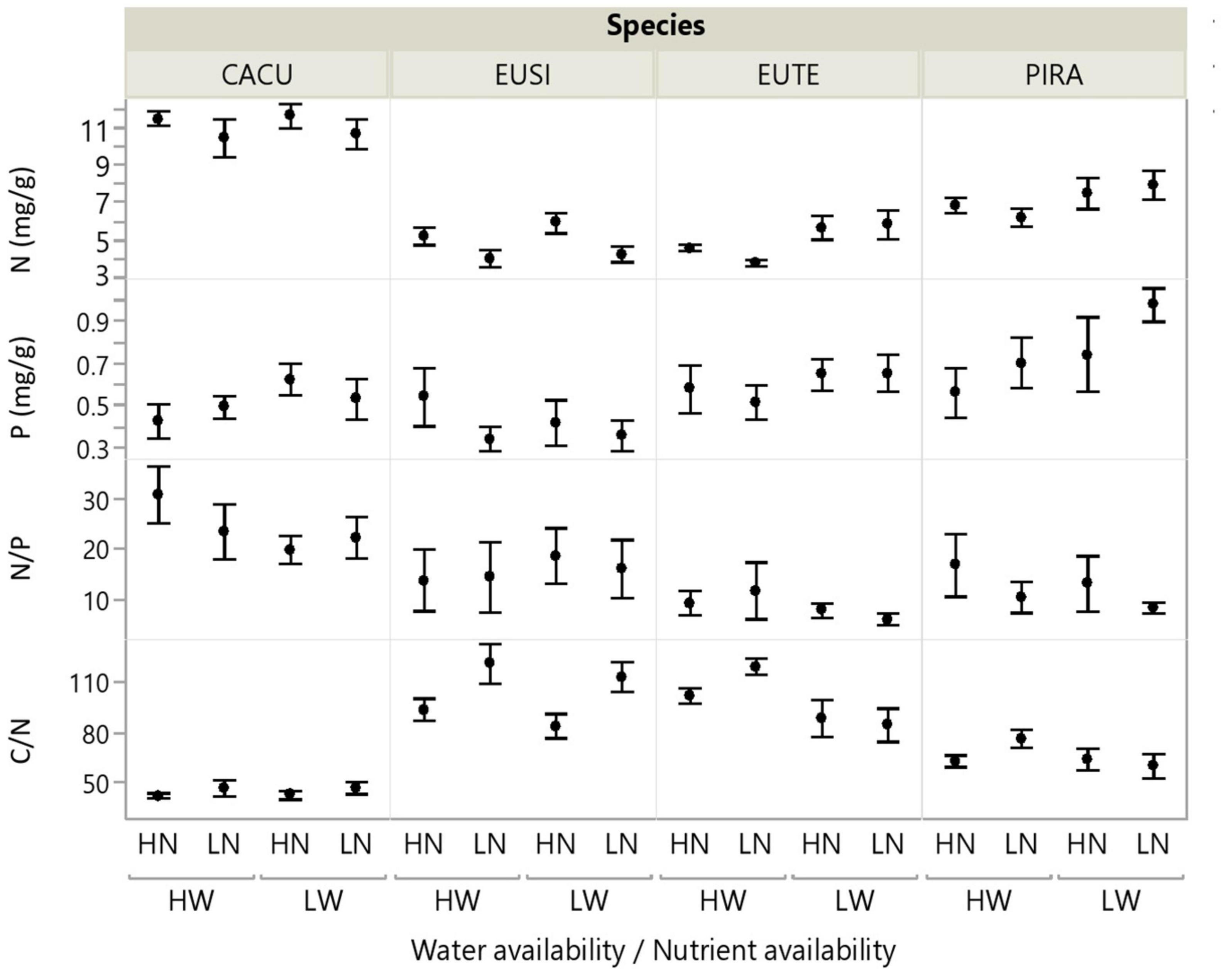
Figure 2. Root chemistry in response to water and nutrient availability treatments for each of the studied species. Water (W), Low/High water availability (LW, HW); Nutrients (N), Low/High nutrient availability (LN, HN). Data points represent averages across shelters/replicates with standard error (n = 6). See Table 2 for statistical test across species. CACU, Casuarina cunninghamiana; EUSI, Eucalyptus sideroxylon; EUTE, Eucalyptus tereticornis; PIRA, Pinus radiata.
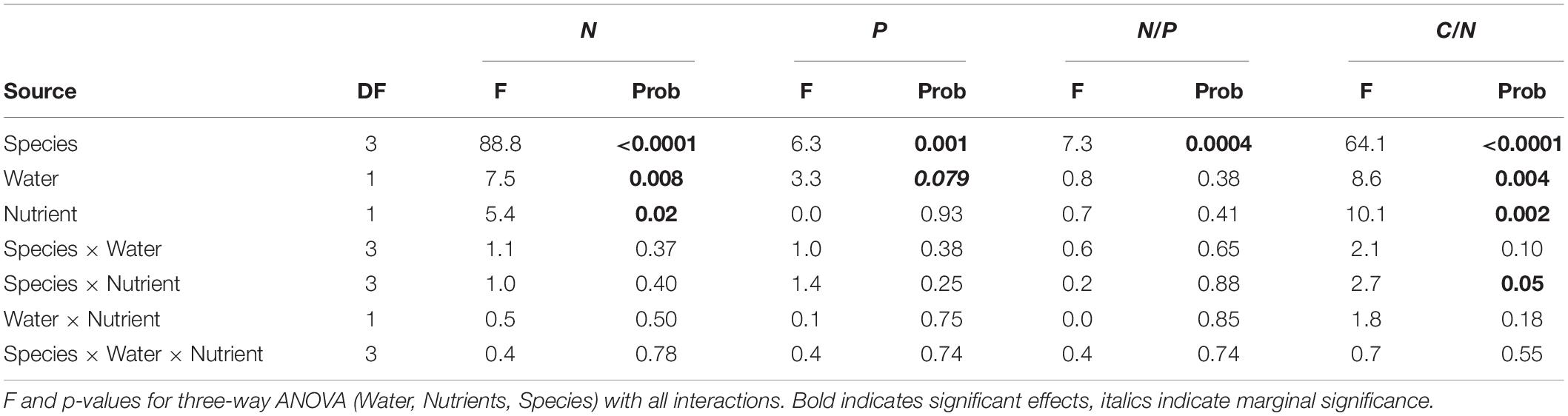
Table 2. Results from statistical analysis of impacts of water and nutrient availability across plant species on root chemistry (N and P content, N/P and C/N).
Root Decomposition and Relationships With Root Traits
C-CO2 release rates were consistent with other lab incubation measurements of early root decomposition via CO2 release (Nelson et al., 2017), CO2 release and mass loss (Ma et al., 2016) and with field observed mass loss of forest roots (Ostertag and Hobbie, 1999). Root decomposition was not affected by nutrient availability but was impacted by water availability with the magnitude of the effect being dependent on species (Figure 3). Decomposition rates were significantly increased under LW conditions for CACU (by 43%; p = 0.0003), PIRA (by 42%; p = 0.0042) and for EUTE when under HN (by 54%; p = 0.019, t-test).
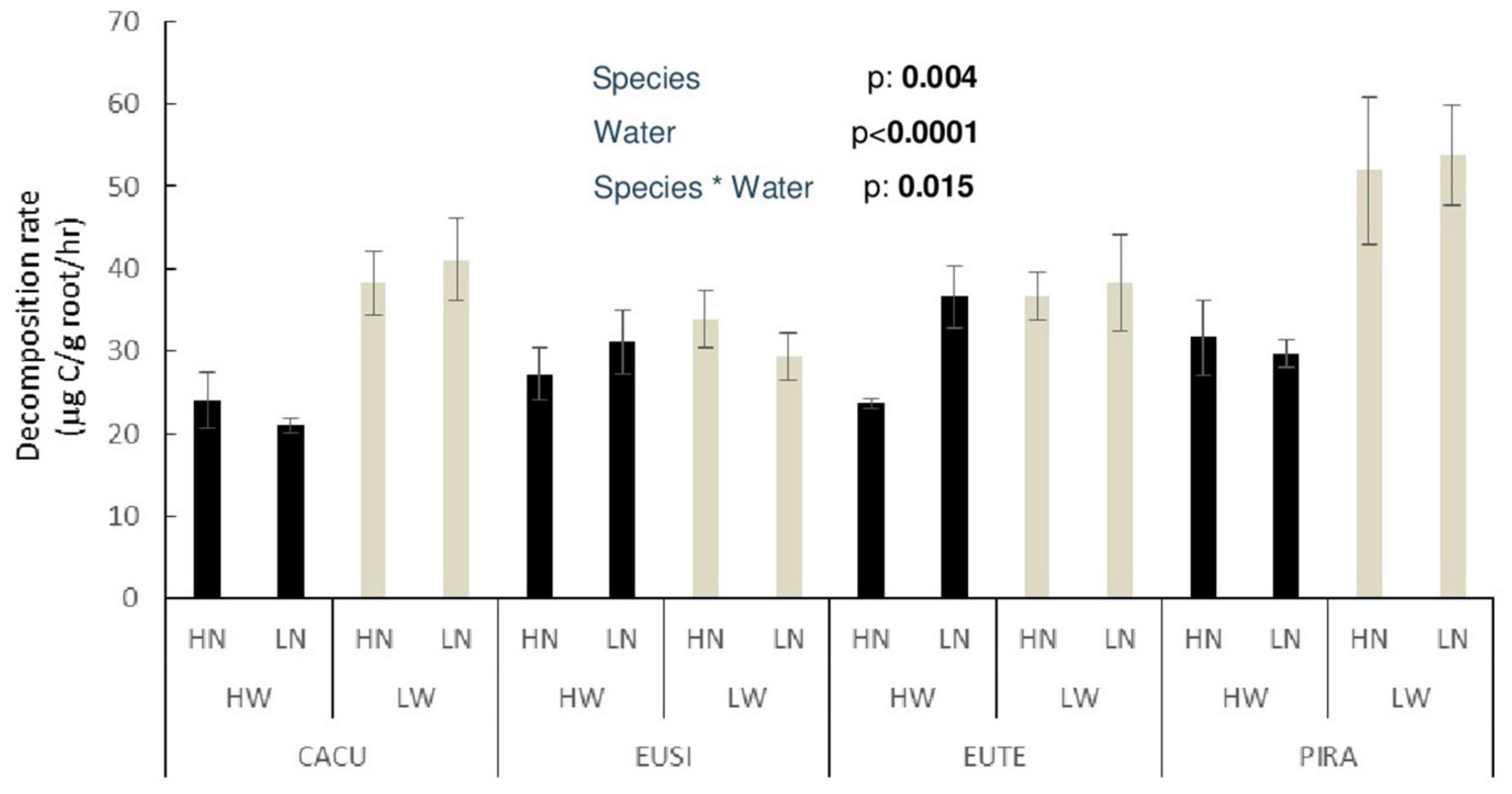
Figure 3. Decomposition rate of roots produced under water and nutrient availability treatments for each of the studied species. Values are mean decomposition rates as CO2 release from roots incubated in sand per gram of root ± standard error (n = 6) across the full incubation duration. HW, high water, LW, low water, HN, high nutrient, LN, low nutrient. p-values are from significant effects from three-way ANOVA (Water, Nutrients, Species) with interactions. CACU, Casuarina cunninghamiana; EUSI, Eucalyptus sideroxylon; EUTE, Eucalyptus tereticornis; PIRA, Pinus radiata.
We examined relationships of roots traits and decomposition within species and found significant albeit species dependent relationships (Table 3). Decomposition rate of CACU was positively related to RTD. Decomposition of EUTE and PIRA was positively related to N content. In addition, PIRA showed strong relationships with C and C/N and with morphological traits, including a negative relationship with diameter and positive relationships with SRL and branching.
We also investigated the coordination between the responses to water availability (ratio of LW to HW values) of root traits and decomposition across species (Figure 4). The responses of SSA, RTD, SRL, and branching were positively related to PC1 while the response of diameter was negatively related, reflecting that across species the reductions in diameter with LW corresponded with increases in SSA, RTD, and SRL and branching. The response of decomposition was positively associated to PC2 together with N and P, indicating that across species increases in decomposition with LW occurred in conjunction with increases in N and P concentrations.
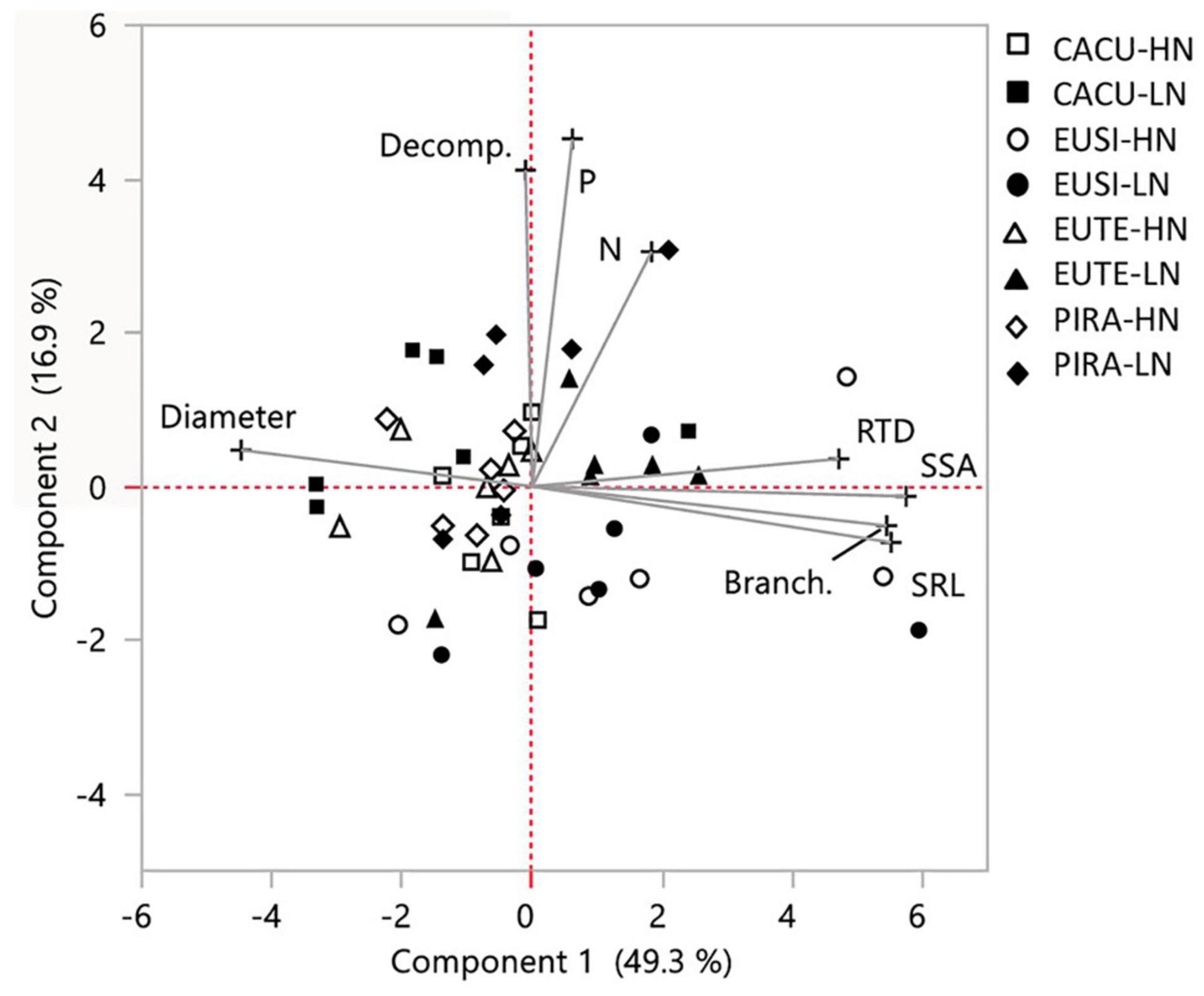
Figure 4. Principal component analysis on the responses to water availability of root chemical and morphological traits and root decomposition. Responses to water availability assessed as the ratio between the value of each variable at LW (low water) and HW (high water). Data points correspond to responses within each experimental shelter (replicates) for each species and each nutrient condition (HN, high nutrients; LN, low nutrients). SRL, specific root length; RTD, root tissue density; SSA, specific surface area; Branching, number of forks/branching points; P, Phosphorus concentration; N, Nitrogen concentration. Values in parentheses: percentage of variation explained by each component. CACU, Casuarina cunninghamiana; EUSI, Eucalyptus sideroxylon; EUTE, Eucalyptus tereticornis; PIRA, Pinus radiata.
Integrated Impact of Changes Belowground on CO2 Release From Decomposition
We applied the measured decomposition rates per mass to the measured biomass pools to estimate the potential CO2 release from root decomposition per gram of soil thus accounting for the responses of biomass to treatments. Integrated CO2 release was impacted independently by nutrient and water availability. Low nutrients (LN) reduced CO2 release across all species by up 60% on average (Figure 5). The effect of water availability was dependent on species as LW significantly increased CO2 release for CACU (p = 0.014) and PIRA (p = 0.034) but not for EUSI or EUTE.
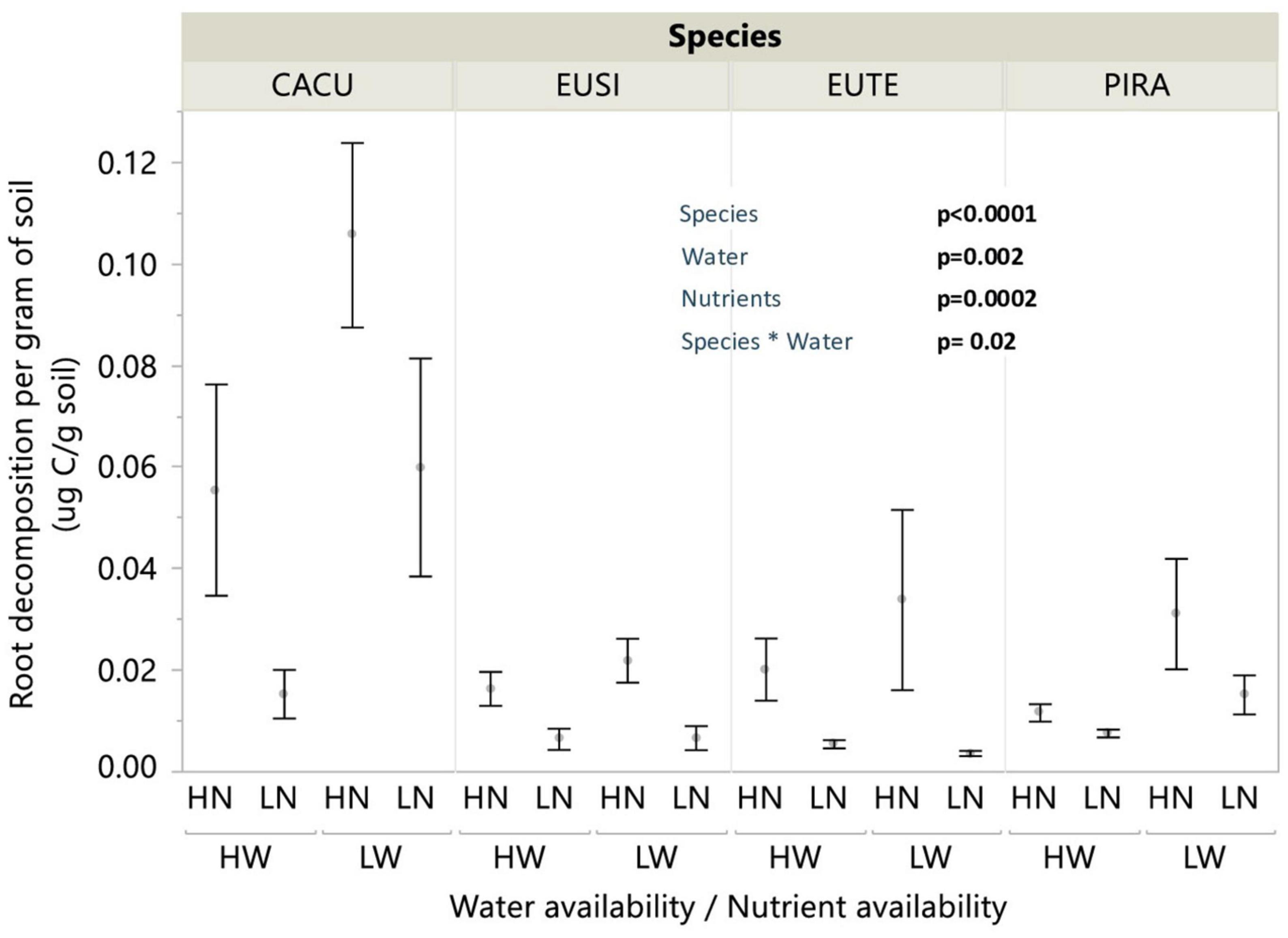
Figure 5. Integrated impact of changes belowground on C release from root decomposition in response to water and nutrient availability treatments N. Values are root decomposition per gram of root applied to the total root biomass per gram of soil. Water (W), Low/High water availability (HW, LW); Nutrients (N), Low/High nutrient availability (LN, HN). Data points represent averages across shelters/replicates with standard error (n = 6). P-values are from significant effects from three-way ANOVA (Water, Nutrients, Species) with interactions. CACU, Casuarina cunninghamiana; EUSI, Eucalyptus sideroxylon; EUTE, Eucalyptus tereticornis; PIRA, Pinus radiata.
Discussion
We investigated the impacts of drought on tree root traits and biomass across different levels of nutrient availability, the linkages of these impacts with their decomposability, and the consequences for release of CO2 from decomposition. While root biomass remained unchanged with drought, we observed chemical and morphological responses. While each species showed a unique set of responses, drought generally reduced root diameter and increased N concentration. Responses of root traits were linked to decomposability. For individual species, morphological and nutrient responses were associated with changes in decomposition. In contrast, across species, drought effects on decomposability were most closely associated with shifts in nutrient concentrations. Specifically, drought increased early stage root decomposability in most species and this response was associated with increases in nutrient concentrations. When accounting for responses in root biomass, for some species, shifts in root decomposability with drought were sufficient to increase their potential net CO2 release back to the atmosphere.
Drought had no strong impacts on root biomass, even though it reduced total plant biomass (Dijkstra et al., 2016). Thus, while this indicates drought increased the root to shoot ratio, as is commonly found (Ledo et al., 2018), our results indicate that the belowground responses to drought manifested in the chemistry and morphology of roots rather than in biomass. As expected, the degree and direction of response were species dependent; however, there were some general responses across species. Drought increased N concentration and decreased C/N, which agrees with the expectations by Brunner et al. (2015) for trees and with observations for herbaceous species (de Vries et al., 2016). Drought also reduced diameter overall, which is in agreement with the observations by Olmo et al. (2014) for woody species, and across growth forms (Zhang et al., 2019). This diameter response was most marked for EUSI. EUSI is the eucalypt expected to be most tolerant to drought and indeed showed the smallest decline in total biomass (Dijkstra et al., 2016). EUSI also responded strongly across other morphological parameters suggesting that its high responsiveness in root morphology was an effective strategy for drought tolerance. The expectation of high tissue density was not met for EUSI, but it occurred strongly in CACU and PIRA (as well as EUTE, HN) and is in agreement with Olmo et al. (2014) for woody species. Thus, our findings indicated some common responses across contrasting woody species, although the particular suite of responsive traits were unique to each species.
We hypothesized that enhanced nutrient availability would reduce the response of root traits to drought. This would show up as an interactive effect where the impact of drought would be lower under high nutrient availability (HN). However, there were no indications of high nutrient availability modifying the impact of drought on root chemical traits. In the case of root morphological traits, there was some, although limited evidence of species dependent interactions where high nutrient availability reduced or eliminated the effects of drought (CACU SRL and SSA; EUTE SSA; and PIRA branching). The observation of counteracting effects in three of the four species suggests that it may be common for woody species, that morphological responses to drought are reduced when nutrient supply is high. Our study helps explain the variation in observed morphological responses to drought in previous synthesis studies (e.g., Zhou et al., 2018) and calls for analyses comparing responses across or controlling for nutrient availability levels. The fact that only some trait responses to drought were counteracted by high nutrient supply, poses the question of whether it is possible to identify patterns regarding drought-responding traits that are driven primarily by lack of access to nutrients or, alternatively, to water.
We found that drought (LW) increased root decomposability in three of the four species. Increased decomposability with drought is in agreement with the very few similar observations available (Garcia-Palacios et al., 2016) and general hypothesis by Brunner et al. (2015). We hypothesized that changes in decomposability would be linked with the impacts of drought on root morphology and chemistry, and thinner and longer roots would decompose faster, but this could be counteracted by potential decreases in nutrient content. For CACU, EUTE and PIRA, the species whose decomposition was affected by drought, some relationships were evident. For the drought tolerant PIRA, we found strong support for the expectation that thinner and longer roots would be associated with faster decomposition (Roumet et al., 2016) with positive relationships with diameter and SRL. In turn, increases in N concentration with drought led to a positive relationship between decomposability and N in EUTE and PIRA. Increases in decomposability with increased root N content are consistent with other observations (Prieto et al., 2016; Roumet et al., 2016). In contrast, for EUSI, the various changes in root morphology with drought (decreased diameter, increased SRL, SSA and branching) did not lead to changes in decomposability as EUSI decomposed similarly with and without drought. Our results thus indicate varying degrees of association between root traits responses to drought and decomposability for different species.
When we analyzed responses across species, we found that morphological responses were not associated with root decomposability. It was the responses of root chemistry that were most closely linked to decomposition so that increased decomposability co-occurred with increases in N and P content (Figure 4 and Table 2), whereas in cases of small or no shifts in nutrients, (EUSI) decomposability did not change. Greater decomposability with higher N (Roumet et al., 2016; See et al., 2019; Bonanomi et al., 2021; Jiang et al., 2021) and P concentrations (Smith et al., 2014; See et al., 2019; Jiang et al., 2021) have been observed. Our data suggest that drought can cause changes in decomposability via changes in nutrient chemistry so that for the species which respond to drought with increases in nutrient content, this is likely to lead to increases in root decomposability. Together, our observations indicate that while for some species morphological responses can be related to changes in decomposition (PIRA, CACU), across these contrasting woody species, shifts in nutrient concentration are more important drivers of drought-induced shifts in root decomposability than morphological changes.
The increase in root decomposability with drought observed in most conditions suggests greater release of C per unit of root biomass back to the atmosphere. However, a greater overall net release of C would also depend on the impacts of drought on root biomass and turnover. Although we did not quantify direct effects on turnover (growth, death, decomposition in situ), the measurements of biomass allowed us to test the hypothesis that enhanced release of C as CO2 back to the atmosphere with increased decomposability would be counteracted by the reduction of root biomass with drought. We found that the potential CO2 release, accounting for root biomass, was greater under drought for CACU and PIRA. This was due to these species showing the strongest increase in decomposability combined with no reduction in root biomass with drought. These results indicate that for those species the shifts in root decomposability with drought due to changes in root traits were sufficient to increase their potential CO2 release back to the atmosphere. Changes in root decomposability could also have additional consequences for C cycling, such as soil organic matter formation as greater decomposability could impact the amount of root-derived C that may ultimately become stabilized in the soil. Increase in decomposability with drought is a specific mechanism that should be considered when assessing the role of roots in belowground C cycling and potential feedbacks to climate change.
While studies of effects of drought on roots traits and separate studies of root trait control of decomposition are available, our study is unique in that it directly and empirically links the environmental stress, the root response and the microbial decomposition process within and across contrasting species. We demonstrated that while for some species morphological responses are related to changes in decomposition (PIRA, CACU), across these contrasting woody species it is the changes in tree root nutrient concentrations with drought that are associated with shifts in their decomposability. When integrated with changes in biomass, these changes in decomposability can shift the potential contribution of roots to the C balance at the ecosystem scale and, with other factors being equal, may contribute to more C release and thus a positive feedback to climate change. By isolating root traits, decomposability and biomass, our findings help disentangle the multiple factors involved in root contribution to C balances at the ecosystem level. Our observations were of responses of young trees (ca. 2 years) and of early stages of root decomposition of their roots. It is yet to be assessed whether shifts in decomposability are sustained in later stages, but our results indicate such investigation is warranted. Our findings enhance our empirical understanding of belowground plant trait responses to drought and how they might influence feedbacks to climate via C cycling in soil. So far, this has been mainly explored in aboveground traits (Anderegg et al., 2019), but we critically need this information for inclusion of roots in Earth system models (Warren et al., 2015).
Data Availability Statement
The original contributions presented in the study are included in the article/supplementary material, further inquiries can be directed to the corresponding author/s.
Author Contributions
DT designed the field experiment which was managed by CM. YC and FD conceived and conducted the belowground study, while SB conducted the root incubations and root chemistry analyses. YC and SB analyzed the data. YC wrote the manuscript with input from all co-authors. All authors contributed to the article and approved the submitted version.
Funding
This work was supported by a grant from the Australian Research Council (FT100100779) awarded to FD and ARC grants (DP0879531 and LP0989881) to DT.
Conflict of Interest
The authors declare that the research was conducted in the absence of any commercial or financial relationships that could be construed as a potential conflict of interest.
Publisher’s Note
All claims expressed in this article are solely those of the authors and do not necessarily represent those of their affiliated organizations, or those of the publisher, the editors and the reviewers. Any product that may be evaluated in this article, or claim that may be made by its manufacturer, is not guaranteed or endorsed by the publisher.
Acknowledgments
We thank Burhan Amiji, and Craig Barton for support with management and maintenance of the ROS facility. We thank Hero Tahaei, Alberto Canarini, Julius Sagun, and Pushpinder Matta for assistance with field and laboratory work.
References
Anderegg, W. R. L., Trugman, A. T., Bowling, D. R., Salvucci, G., and Tuttle, S. E. (2019). Plant functional traits and climate influence drought intensification and land–atmosphere feedbacks. Proc. Natl. Acad. Sci. U.S.A. 116, 14071–14076. doi: 10.1073/pnas.1904747116
Bardgett, R. D. (2017). Plant trait-based approaches for interrogating belowground function. Biol. Environ. Proc. R. Irish Acad. 117B, 1–13. doi: 10.3318/bioe.2017.03
Bardgett, R. D., Mommer, L., and De Vries, F. T. (2014). Going underground: root traits as drivers of ecosystem processes. Trends Ecol. Evol. 29, 692–699. doi: 10.1016/j.tree.2014.10.006
Bardhan, K., York, L. M., Hasanuzzaman, M., Parekh, V., Jena, S., and Pandya, M. N. (2021). Can smart nutrient applications optimize the plant’s hidden half to improve drought resistance? Physiol. Plant. 172, 1007–1015. doi: 10.1111/ppl.13332
Bonanomi, G., Idbella, M., Zotti, M., Santorufo, L., Motti, R., Maisto, G., et al. (2021). Decomposition and temperature sensitivity of fine root and leaf litter of 43 mediterranean species. Plant Soil 464, 453–465.
Brunner, I., Herzog, C., Dawes, M. A., Arend, M., and Sperisen, C. (2015). How tree roots respond to drought. Front. Plant Sci. 6:547. doi: 10.3389/fpls.2015.00547
Cheng, W., Parton, W. J., Gonzalez-Meler, M. A., Phillips, R., Asao, S., et al. (2014). Synthesis and modeling perspectives of rhizosphere priming. New Phytol. 201, 31–44. doi: 10.1111/nph.12440
Comas, L., Becker, S., Cruz, V. M., Byrne, P. F., and Dierig, D. A. (2013). Root traits contributing to plant productivity under drought. Front. Plant Sci. 4:442. doi: 10.3389/fpls.2013.00442
Cornwell, W. K., Cornelissen, J. H., Amatangelo, K., Dorrepaal, E., Eviner, V. T., and Godoy, O. (2008). Plant species traits are the predominant control on litter decomposition rates within biomes worldwide. Ecol. Lett. 11, 1065–1071. doi: 10.1111/j.1461-0248.2008.01219.x
de Vries, F. T., Brown, C., and Stevens, C. J. (2016). Grassland species root response to drought: consequences for soil carbon and nitrogen availability. Plant Soil 409, 297–312. doi: 10.1007/s11104-016-2964-4
Dijkstra, F. A., Carrillo, Y., Aspinwall, M. J., Maier, C., Canarini, A., and Tahaei, H. (2016). Water, nitrogen and phosphorus use efficiencies of four tree species in response to variable water and nutrient supply. Plant Soil 406, 187–199. doi: 10.1007/s11104-016-2873-6
Drake, J. E., Reichstein, M., Bahn, M., Thonicke, K., Frank, D., and Mahecha, M. D. (2017). Stomatal and non-stomatal limitations of photosynthesis for four tree species under drought: a comparison of model formulations. Agric. For. Meteorol. 247, 454–466.
Faucon, M.-P., Houben, D., and Lambers, H. (2017). Plant functional traits: soil and ecosystem services. Trends Plant Sci. 22, 385–394. doi: 10.1016/j.tplants.2017.01.005
Frank, D., Reichstein, M., Bahn, M., Thonicke, K., Frank, D., and Mahecha, M. D. (2015). Effects of climate extremes on the terrestrial carbon cycle: concepts, processes and potential future impacts. Glob. Change Biol. 21, 2861–2880. doi: 10.1111/gcb.12916
Garcia-Palacios, P., Prieto, I., Ourcival, J. M., and Hattenschwiler, S. (2016). Disentangling the litter quality and soil microbial contribution to leaf and fine root litter decomposition responses to reduced rainfall. Ecosystems 19, 490–503. doi: 10.1007/s10021-015-9946-x
Hobbie, S. E., Oleksyn, J., Eissenstat, D. M., and Reich, P. B. (2010). Fine root decomposition rates do not mirror those of leaf litter among temperate tree species. Oecologia 162, 505–513. doi: 10.1007/s00442-009-1479-6
IPCC (2013). Climate Change 2013: the Physical Science Basis. Contribution of Working Group I to the Fifth Assessment Report of the Intergovernmental Panel on Climate Change. NY: Cambridge University Press.
Jiang, L., Wang, H. M., Li, S. G., Fu, X. L., Dai, X. Q., Yan, H., et al. (2021). Mycorrhizal and environmental controls over root trait-decomposition linkage of woody trees. New Phytol. 229, 284–295. doi: 10.1111/nph.16844
Kudoyarova, G. R., Dodd, I. C., Veselov, D. S., Rothwell, S. A., and Veselov, S. (2015). Common and specific responses to availability of mineral nutrients and water. J. Exp. Bot. 66, 2133–2144. doi: 10.1093/jxb/erv017
Laliberté, E. (2017). Below-ground frontiers in trait-based plant ecology. New Phytol. 213, 1597–1603. doi: 10.1111/nph.14247
Ledo, A., Paul, K. I., Burslem, D. F. R. P., Ewel, J. J., Barton, C., and Battaglia, M. (2018). Tree size and climatic water deficit control root to shoot ratio in individual trees globally. New Phytol. 217, 8–11. doi: 10.1111/nph.14863
Ma, C., Xiong, Y., Li, L., and Guo, D. (2016). Root and leaf decomposition become decoupled over time: implications for below- and above-ground relationships. Funct. Ecol. 30, 1239–1246.
Nelson, L., Blumenthal, D. M., Williams, D. G., and Pendall, E. (2017). Digging into the roots of belowground carbon cycling following seven years of Prairie Heating and CO2 Enrichment. (PHACE). Wyoming USA Soil Biol. Biochem. 115, 169–177.
Nikolova, P. S., Bauerle, T. L., Häberle, K.-H., Blaschke, H., Brunner, I., and Matyssek, R. (2020). Fine-root traits reveal contrasting ecological strategies in european beech and norway spruce during extreme drought. Front. Plant Sci. 11:1211. doi: 10.3389/fpls.2020.01211
Olmo, M., Lopez-Iglesias, B., and Villar, R. (2014). Drought changes the structure and elemental composition of very fine roots in seedlings of ten woody tree species. implications for a drier climate. Plant Soil 384, 113–129.
Ostertag, R., and Hobbie, S. E. (1999). Early stages of root and leaf decomposition in Hawaiian forests: effects of nutrient availability. Oecologia 121, 564–573. doi: 10.1007/s004420050963
Pierik, R., and Testerink, C. (2014). The art of being flexible: how to escape from shade. salt, and drought. Plant Physiol. 166, 5–22. doi: 10.1104/pp.114.239160
Prieto, I., Stokes, A., and Roumet, C. (2016). Root functional parameters predict fine root decomposability at the community level. J. Ecol. 104, 725–733. doi: 10.1111/1365-2745.12537
Roumet, C., Birouste, M., Picon-Cochard, C., Ghestern, M., Osman, N., et al. (2016). Root structure-function relationships in 74 species: evidence of a root economics spectrum related to carbon economy. New Phytol. 210, 815–826. doi: 10.1111/nph.13828
See, C. R., Luke, McCormack, M., Hobbie, S. E., Flores-Moreno, H., Silver, W. L., et al. (2019). Global patterns in fine root decomposition: climate, chemistry, mycorrhizal association and woodiness. Ecol. Lett. 22, 946–953. doi: 10.1111/ele.13248
Sindhoj, E., Hansson, A. C., Andren, O., Katterer, T., Marissink, M., and Pettersson, R. (2000). Root dynamics in a semi-natural grassland in relation to atmospheric carbon dioxide enrichment, soil water and shoot biomass. Plant Soil 223, 253–263.
Smith, S. W., Woodin, S. J., Pakeman, R. J., Johnson, D., and van der Wal, R. (2014). Root traits predict decomposition across a landscape-scale grazing experiment. New Phytol. 203, 851–862. doi: 10.1111/nph.12845
Sokol, N. W., Kuebbing, S. E., Karlsen-Ayala, E., and Bradford, M. A. (2019). Evidence for the primacy of living root inputs, not root or shoot litter, in forming soil organic carbon. New Phytol. 221, 233–246. doi: 10.1111/nph.15361
Sun, T., Mao, Z. J., Dong, L. L., Hou, L. L., Song, Y., and Wang, X. W. (2013). Further evidence for slow decomposition of very fine roots using two methods: litterbags and intact cores. Plant Soil 366, 633–646.
Suseela, V., and Tharayil, N. (2018). Decoupling the direct and indirect effects of climate on plant litter decomposition: accounting for stress-induced modifications in plant chemistry. Glob. Change Biol. 24, 1428–1451. doi: 10.1111/gcb.13923
Wang, P., Huang, K. L., and Hu, S. J. (2020). Distinct fine-root responses to precipitation changes in herbaceous and woody plants: a meta-analysis. New Phytol. 225, 1491–1499. doi: 10.1111/nph.16266
Warren, J. M., Hanson, P. J., Iversen, C. M., Kumar, J., Walker, A. P., and Wullschleger, S. D. (2015). Root structural and functional dynamics in terrestrial biosphere models – evaluation and recommendations. New Phytol. 205, 59–78. doi: 10.1111/nph.13034
Zhang, X., Xing, Y. J., Yan, G. Y., Han, S. J., and Wang, Q. G. (2019). Effects of precipitation change on fine root morphology and dynamics at a global scale: a meta-analysis. Can. J. Soil Sci. 99, 1–11. doi: 10.1139/cjss-2018-0114
Keywords: decomposition, drought, root traits, root morphology, root nutrients, nutrient availability, woody plants, climate change feedbacks
Citation: Carrillo Y, Tissue DT, Bruna S, Maier C and Dijkstra FA (2022) Drought Impacts on Tree Root Traits Are Linked to Their Decomposability and Net Carbon Release. Front. For. Glob. Change 5:836062. doi: 10.3389/ffgc.2022.836062
Received: 15 December 2021; Accepted: 28 January 2022;
Published: 16 March 2022.
Edited by:
Douglas Godbold, University of Natural Resources and Life Sciences Vienna, AustriaReviewed by:
Xiao-Tao Lu, Institute of Applied Ecology (CAS), ChinaJörg Luster, Swiss Federal Institute for Forest, Snow and Landscape Research (WSL), Switzerland
Copyright © 2022 Carrillo, Tissue, Bruna, Maier and Dijkstra. This is an open-access article distributed under the terms of the Creative Commons Attribution License (CC BY). The use, distribution or reproduction in other forums is permitted, provided the original author(s) and the copyright owner(s) are credited and that the original publication in this journal is cited, in accordance with accepted academic practice. No use, distribution or reproduction is permitted which does not comply with these terms.
*Correspondence: Yolima Carrillo, y.carrillo@westernsydney.edu.au