- 1Department of Wood Science and Engineering, Oregon State University, Corvallis, OR, United States
- 2School of the Environment and Sustainability, University of Michigan, Ann Arbor, MI, United States
The chemical modification of wood is gaining popularity as a treatment to increase wood durability, particularly in jurisdictions where restrictions on the use of chemical impregnation are increasing. This work measures the impact of thermal modification of western hemlock lumber durability against decay fungi. Western hemlock lumber was thermally modified using a cycle with temperatures ranging from 80 to 170°C and was cut into standard 19 mm blocks for testing according to AWPA method E10. Performance of thermally modified wood was measured against two brown rot fungi, Rhodonia placenta and Gloeophyllum trabeum and one white rot fungus, Trametes versicolor over an 8, 12, and 16-week incubation period. Unmodified western hemlock wood, untreated southern pine and copper azole-treated southern pine were included for comparison. Thermal modification resulted in a slight improvement in durability against Gloeophyllum trabeum, but not Rhodonia placenta compared to untreated western hemlock which caused 50.3 and 52.2% mass loss in thermally modified western hemlock after 16 weeks, respectively. T. versicolor caused a 21.2% mass loss after 16 weeks, but this value was not statistically different than untreated western hemlock (p = 0.46). For comparison, copper azole-treated southern pine showed only an average of 0 and 15% mass loss when exposed to G. trabeum or R. placenta for 16 weeks, respectively. Together, these data indicate that while there may be some protective effect of thermally modifying western hemlock, further development of treatment processes are needed to improve the durability of thermally modified western hemlock.
Introduction
Most wood preservation methods include the application of a chemical biocide to the wood in a pressure or non-pressure process. However, wood preservation technologies that directly modify the wood rather than impregnate it with biocides are gaining greater interest in areas where chemical treatments are heavily restricted. To date these methods have proven successful in a relatively limited range of wood species. As such there is an interest in improving wood modification technologies to increase the durability of the final product and expand this technology into a wider range of wood species.
Thermal modification of wood is one approach to improving wood durability. Thermal modification of wood is done by heating wood in a low-oxygen environment to facilitate chemical and structural alteration (Sandberg et al., 2017). Chemical modifications such as covalent crosslinking in lignin and partial polysaccharide decomposition reduce hygroscopicity and increase the resistance of wood to fungal decay (Tjeerdsma et al., 1998; Alen et al., 2002; Kamden et al., 2002; Marcon et al., 2021). Modification can be done by a variety of different processes that all facilitate the heating of wood to between 160 and 260°C (Gerardin, 2016; Sandberg et al., 2017). Thermal treatment processes differ in a wide variety of parameters such as water content of the treatment chamber, use of oils in heating, atmospheric gas composition, heating and drying cycles and more (Militz, 2002). Process conditions can be modified to alter the characteristics of the final product.
Thermal modification of wood improves durability against decay fungi for above ground contact applications. This effect has been shown for a variety of wood species involving many different chemical processes, but the much of the research done to date has focused on European species (Alen et al., 2002; Kamden et al., 2002; Miklecic and Jirous-Rajkovic, 2016; Luptakova et al., 2019). Near complete resistance to fungal decay shown by near zero percent mass loss upon fungal exposure can be achieved if thermally modified wood is sufficiently treated. In European beech, % mass loss due to decay fungi begins to significantly decline as the treatment cycle is increased over 200°C (Hakkou et al., 2006). Higher temperatures yield greater wood mass loss, which has been used as a parameter to evaluate thermally modified wood durability due to its inverse relationship with mass loss due to fungal decay (Candelier et al., 2015). As interest in thermal modification grows in North America, testing thermal modification technologies on a greater variety of North American species using varied processes is necessary to improve these products.
This study describes durability testing of thermally modified, Tsuga heterophylla (western hemlock) wood using an American Wood Protection Association (AWPA) E10 soil bottle test (American Wood Protection Association [AWPA], 2020). The test used 19 mm blocks cut from the thermally modified and control western hemlock lumber that was left over following bending strength tests. The test compared the decay resistance of thermally modified western hemlock with untreated western hemlock, untreated southern pine, and southern pine treated with copper azole to UC3B retention levels against two brown rot fungi and one white rot fungus.
Materials and Methods
Thermal Modification of Western Hemlock Wood
Each piece of western hemlock lumber was cut into two matching test specimens of identical dimensions. One piece of the matching lumber specimens was designated as the kiln-dried KW sample, while the second matching specimen was designated as the thermally-modified TW sample. The TW specimens were loaded into a hydrothermal reactor and treated according to the conditions listed in Table 1 (Song et al., 2019). The treatment conditions, including parameters like temperature, time and pressure, were based on previous research conducted in the Natural Resources Research Institute at the University of Minnesota in Duluth (UMDNRRI). Upon completion of the process, the samples were conditioned in a standard environment with steam to a moisture content of 4.5% prior to structure and strength property characterization. Following the strength testing, the control and thermally modified samples were cut into standard 19 mm blocks for testing according to AWPA method E10.
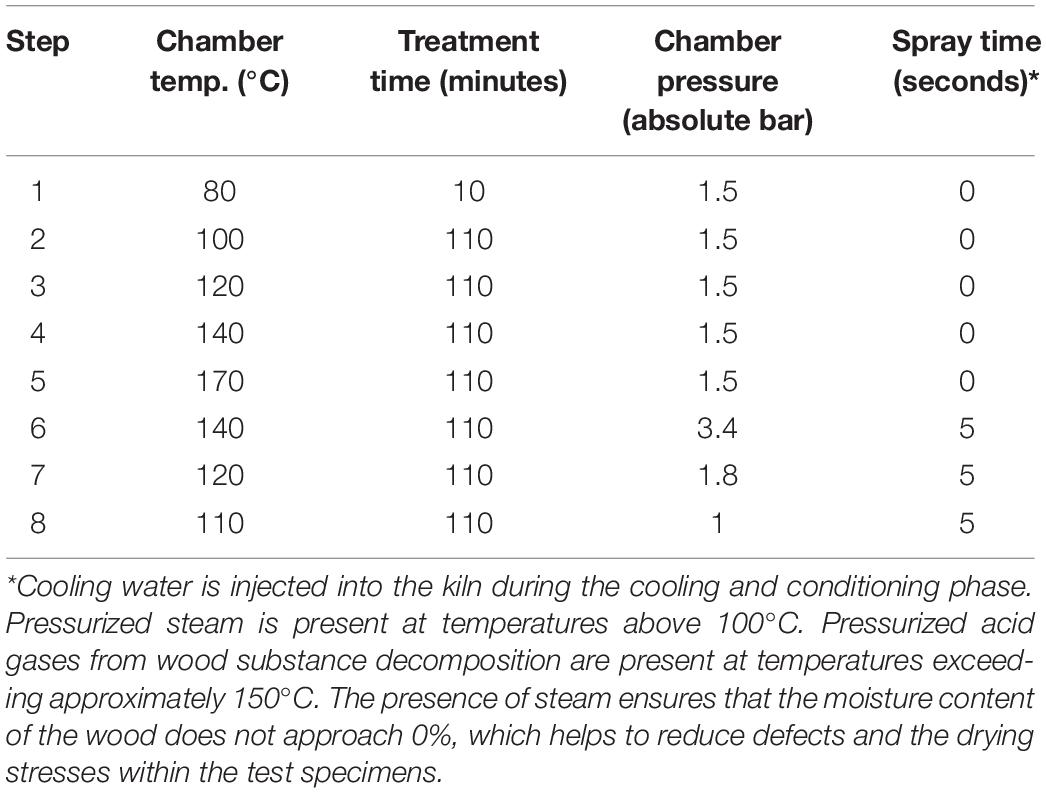
Table 1. Treatment conditions for the thermal modification process used for western hemlock in this study derived from Song et al. (2019).
Soil Bottle Decay Tests
Soil bottle decay tests were prepared according to AWPA E10 standards. Decay tests were run for three timepoints, 8, 12, and 16 weeks and 10 replicate blocks per treatment per timepoint were measured. Blocks were oven-dried at 50°C for 72 h and weighed to the nearest 0.001 g prior to soaking in distilled water until their moisture contents reached 30–40%. Moistened blocks were sterilized in an autoclave at 121°C for 1 h, cooled and placed into colonized decay chambers described below.
Decay chambers (473 mL French squares) were half-filled with a custom soil blend of 45% sandy loam soil (40% sand, 40% silt, and 20% clay), 42% organic amendments (∼14% each of composted dairy manure, horse manure, and Douglas-fir bark) and ∼13% organic soil building conditioner (Gardner and Bloome®, Carson, CA, United States). Strips of western hemlock [Tsuga heterophylla (Raf) Sarg.] for brown rot or red alder (Alnus rubra Bong) for white rot test fungi were placed on the soil surface, and the bottles were sterilized in an autoclave at 121°C for 100 min. The bottles were inoculated with 5 mm malt agar disks from the actively growing edges of cultures for the two brown rot fungi Gloeophyllum trabeum (Pers.: Fr.) Murr. (isolate # Madison 617) and Rhodonia placenta (Fr) Niemela, Larss, and Schagel (Isolate No. Mad 698) or the white rot fungus Trametes versicolor (L. ex Fr.) Pilát (Isolate # R-105). Inoculated bottles were incubated at 28°C until test fungi completely covered the feeder strips (∼10 days). Sterile blocks were then placed on the surfaces of the feeder strips. The bottles were loosely capped and incubated at 28°C for 8, 12, or 16 weeks for blocks and 16 weeks only for no fungus controls.
At the end of the incubation period, samples were removed, scraped clean of adhering mycelium and oven-dried at 50°C for 72 h and reweighed to determine mass loss. The difference between initial and final oven-dry weight was used as a measure of the decay resistance of each material. The degree of resistance to fungal attack was assessed using the scale described in ASTM D2017-05 (2014), where 0–10% weight loss is considered highly resistant to decay, 11–24% weight loss is resistant, 25–44% is moderately resistant, and > 45% is slightly or non-resistant.
A subset of 19 mm southern pine sapwood blocks was treated with copper azole-C (CA-C) in a vacuum chamber to AWPA UC3B retentions (0.06 pcf, 1.0 kg/m3) to serve as a positive, decay resistant control. Retentions were determined gravimetrically by solution uptake. Untreated southern pine was included as a positive decay control at each timepoint and an uninoculated control for each wood treatment was run in parallel to the 16-week timepoint.
Results and Discussion
Untreated southern pine and untreated western hemlock blocks showed an average mass loss across all fungi of 55.2 and 49.5% after 16 weeks, respectively (Table 2 and Figure 1). The white rot fungus caused relatively less mass loss over this period, averaging 44.2 and 29.2% for southern pine and western hemlock, respectively. These values were well within a normal range for untreated wood and indicate the fungal strains used in this test exhibited sufficient vigor. These data indicate that western hemlock is not resistant to attack by brown rot fungi but shows a moderate level of resistance to attack by a white rot fungus. Treatment of southern pine blocks with CA-C resulted in blocks that had an average uptake retention of 1.12 kg/m3 (0.07 pcf), meeting the UC3B standard. CA-C treated blocks prevented mass loss entirely in Gloeophyllum trabeum and Trametes versicolor cultures. An average of 15% mass loss was observed in Rhodonia placenta cultures, likely because this fungus is known to be more copper tolerant than the other fungi used in this test.
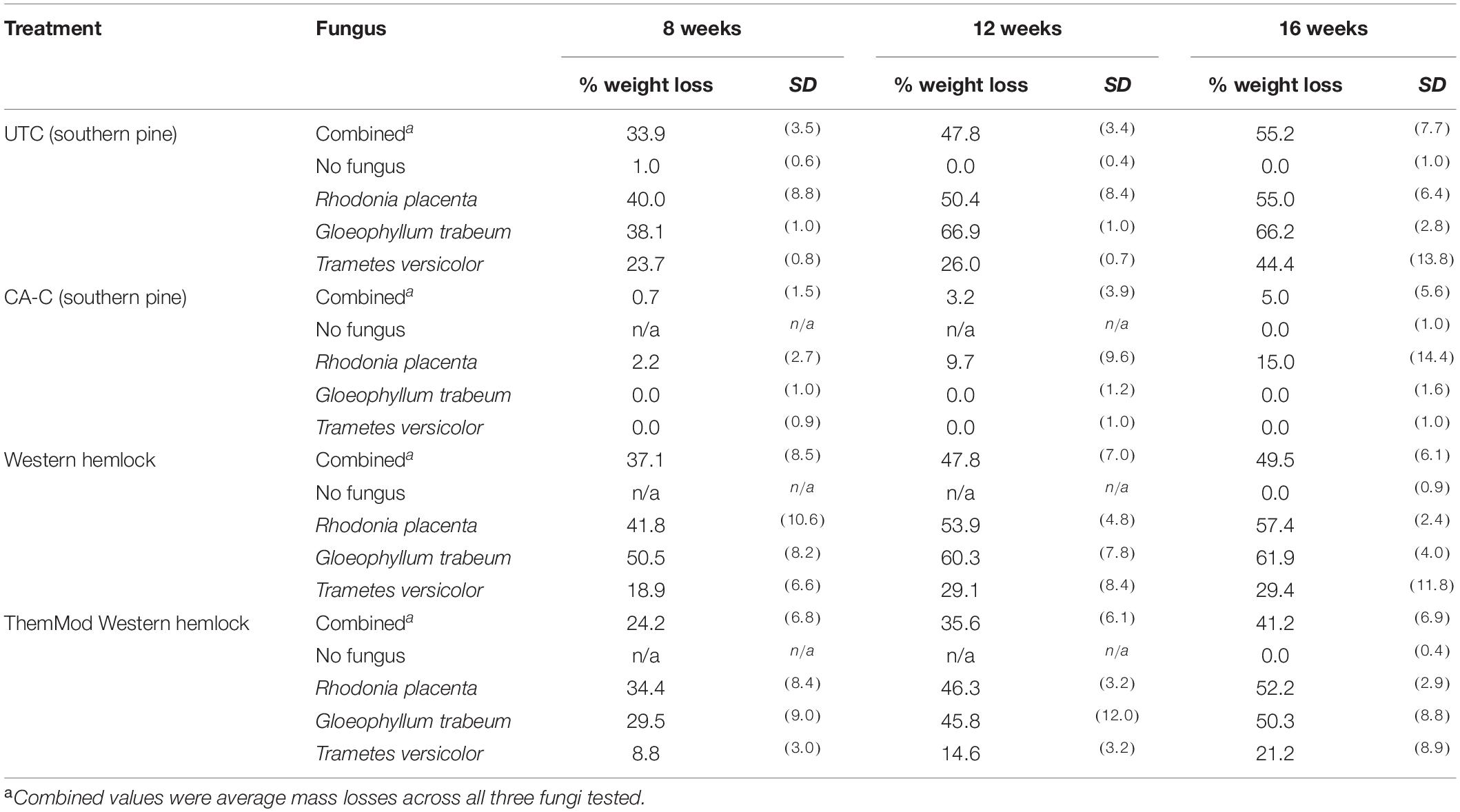
Table 2. Mass loss in 19 mm blocks after exposure to one of two brown rot fungi or one white rot fungus for 8, 12, or 16 weeks.
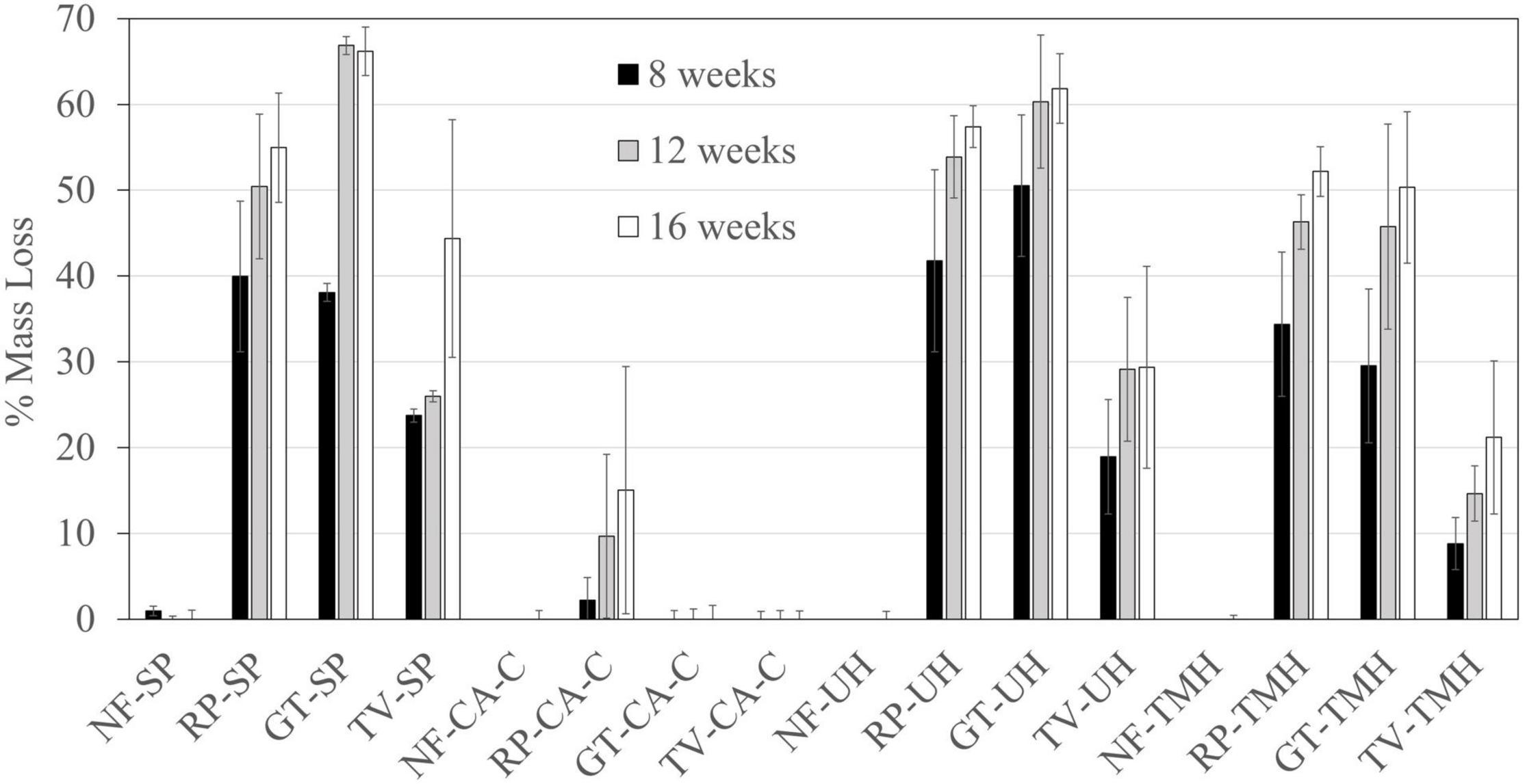
Figure 1. Mass loss percentages after 8, 12, and 16 weeks of decay for thermally modified western hemlock (TMH), untreated western hemlock (UH), copper azole-treated southern pine (CA-C), and southern pine (SP) degraded by Rhodonia placenta (RP), Gloeophyllum trabeum (GT), or Trametes versicolor (TV). Error bars are one standard deviation of 10 replicate blocks.
Thermally modified western hemlock showed an overall average mass loss of 41.2% across all test fungi after 16 weeks. As was seen with untreated western hemlock, brown rot fungi caused much higher mass losses than the white rot fungus. Mass loss in thermally modified western hemlock exposed to R. placenta for 16 weeks was an average of 52.5% and was not statistically different from the 58% mass loss observed for unmodified western hemlock (p < 0.05, Tukey’s test). Exposure to G. trabeum caused 50.3% mass loss, significantly less than the 61.9% mass loss measured on untreated western hemlock. Similarly, to western untreated western hemlock, mass losses were lower for the white rot fungus, 21.2% for TM western hemlock vs. 30% for untreated western hemlock, although this result was not statistically different.
These data show that the thermal modification process used in this study caused a modest increase in durability against one brown rot fungus compared to untreated western hemlock. Mass loss levels were reduced against the two other test fungi, but not enough to be statistically significant. According to the definitions outlined in ASTMD-2017-5, thermally modified western hemlock in this study would be considered resistant to white rot fungi, and non-resistant to both brown rot fungi. By contrast untreated western hemlock would be considered moderately resistant to white rot decay and non-resistant to brown rot fungi.
Despite the limited improvements in durability seen here, thermal modification of western hemlock has been shown to make the wood less hygroscopic, a characteristic that is would suggest better durability in above ground contact applications (Song et al., 2019). Modest durability increases seen here are lower than what is expected based on some previous testing of thermally modified wood (Kamden et al., 2002; Hakkou et al., 2006). Factors that may have contributed to the difference in durability is treatment temperature and resulting mass loss. Thermally modified wood tested in this study only showed about a 3% density loss and was treated at temperatures below 200°C. Previous work on thermally modified beech wood showed that inhibition of fungal growth greatly accelerated after wood was modified at temperatures above 200°C (Hakkou et al., 2006). Additionally, mass losses of about 10% are necessary to fully inhibit brown rot decay on thermally modified wood (Candelier et al., 2015). Treatment temperature and mass loss was kept low for wood tested in this study to maintain structural integrity of the modified hemlock, which does deteriorate at higher mass loss values (Song et al., 2019). Further alteration of the modification cycle in future work would help develop a process for western hemlock that balances greater durability increases with the maintenance of structural properties of wood necessary for load-bearing applications such as decking.
Conclusion
While some improvement in durability was measured, thermal modification was nowhere near as effective as treatment with copper azole or other thermal treatments in the literature. The results, show that thermal modification is able to improve western hemlock durability, against at least one brown rot fungus (G. trabeum). As a result, TM western hemlock produced here represents a step toward making this product appropriate for exterior end-uses where the lumber is not in direct contact with the ground. However, the relatively modest improvement in durability compared to previous work on thermal modification indicates that treatment cycle severity used to produce material tested here was too low. Higher temperatures in the treatment cycle, for example from 200 to 210°C for conifer lumber, would likely further improve the durability of thermally modified western hemlock. Future research investigating the impacts of different process control conditions on durability of western hemlock could lead to significant improvements its durability and a commercially viable wood product.
Data Availability Statement
The original contributions presented in the study are included in the article/supplementary material, further inquiries can be directed to the corresponding author/s.
Author Contributions
IE: thermal modification. JC: decay tests. GP and IE: manuscript preparation. All authors contributed to the article and approved the submitted version.
Conflict of Interest
The authors declare that the research was conducted in the absence of any commercial or financial relationships that could be construed as a potential conflict of interest.
The handling editor declared a past co-authorship with one of the authors GP.
Publisher’s Note
All claims expressed in this article are solely those of the authors and do not necessarily represent those of their affiliated organizations, or those of the publisher, the editors and the reviewers. Any product that may be evaluated in this article, or claim that may be made by its manufacturer, is not guaranteed or endorsed by the publisher.
References
Alen, R., Kotilainen, R., and Zaman, A. (2002). Thermochemical behavior of Norway spruce (Picea abies) at 180-225 degrees C. Wood Sci. Technol. 36, 163–171. doi: 10.1007/s00226-001-0133-1
American Wood Protection Association [AWPA] (2020). American Wood Protection Association 2020 Book of Standards. Birmingham, AL: American Wood Protection Association.
Candelier, K., Thevenon, M., Petrissans, A., Dumarcay, S., Gerardin, P., and Petrissans, M. (2015). “A review of the analytical methods used in determining the decay resistance of heat treated wood,” in Proceedingo fo the 46th IRG Annual Meeting, IRG/WP 15-40690, (Cardiff), 46.
Gerardin, P. (2016). New alternatives for wood preservation based on thermal and chemical modification of wood- a review. Ann. For. Sci. 73, 559–570. doi: 10.1007/s13595-015-0531-4
Hakkou, M., Petrissans, M., Gerardin, P., and Zoulalian, A. (2006). Investigations of the reasons for fungal durability of heat-treated beech wood. Polym. Degrad. Stab. 91, 393–397. doi: 10.1016/j.polymdegradstab.2005.04.042
Kamden, D. P., Pizzi, A., and Jermannaud, A. (2002). Durability of heat-treated wood. Holz-Als Roh-und Werkst. 60, 1–6. doi: 10.1007/s00107-001-0261-1
Luptakova, J., Kacik, F., Mitterova, I., and Zachar, M. (2019). Influence of temperature of thermal modification on the fire-technical characteristics of spruce wood. Bioresources 14, 3795–3807. doi: 10.15376/biores.14.2.3795-3807
Marcon, B., Tondi, G., Procino, L., and Goli, G. (2021). Thermal modification kinetics and chemistry of poplar wood in dry and saturated steam media. Holzforschung 75, 721–730. doi: 10.1515/hf-2020-0166
Miklecic, J., and Jirous-Rajkovic, V. (2016). Influence of thermal modification on surface properties and chemical composition of beech wood (Fagus sylvatica L.). Drv. Ind. 67, 65–71. doi: 10.5552/drind.2016.1520
Militz, H. (2002). “Thermal treatment of wood: European processes and their background,” in Proceeding of the IRG Annual Meeting, IRG/WP 02-40241, (Cardiff), 33.
Sandberg, D., Kutnar, A., and Mantanis, G. (2017). Wood modification technologies - a review. Iforest-Biogeosci. For. 10, 895–908. doi: 10.3832/ifor2380-010
Song, K., Ganguly, I., Eastin, I., Dou, C., Bura, R., and Dichiara, A. (2019). Structure-property relationships of hydrothermally treated western hemlock. SN Appl. Sci. 1:84. doi: 10.1007/s42452-018-0096-6
Keywords: wood modification, wood biodeterioration, wood protection, brown rot, white rot
Citation: Presley G, Cappellazzi J and Eastin I (2022) Durability of Thermally Modified Western Hemlock Lumber Against Wood Decay Fungi. Front. For. Glob. Change 5:813080. doi: 10.3389/ffgc.2022.813080
Received: 11 November 2021; Accepted: 10 February 2022;
Published: 08 March 2022.
Edited by:
Christian Brischke, University of Göttingen, GermanyReviewed by:
Mingliang Jiang, Chinese Academy of Forestry, ChinaSun Fangli, Zhejiang Agriculture and Forestry University, China
Copyright © 2022 Presley, Cappellazzi and Eastin. This is an open-access article distributed under the terms of the Creative Commons Attribution License (CC BY). The use, distribution or reproduction in other forums is permitted, provided the original author(s) and the copyright owner(s) are credited and that the original publication in this journal is cited, in accordance with accepted academic practice. No use, distribution or reproduction is permitted which does not comply with these terms.
*Correspondence: Gerald Presley, Z2VyYWxkLnByZXNsZXlAb3JlZ29uc3RhdGUuZWR1