- 1Departamento de Producción Vegetal, Cátedra de Dasonomía, Facultad de Agronomía, Universidad de Buenos Aires, Buenos Aires, Argentina
- 2Grupo de Estudios Biofísicos y Ecofisiológicos (GEBEF), Instituto de Biociencias de la Patagonia (INBIOP), Consejo Nacional de Investigaciones Científicas y Técnicas (CONICET)-Universidad Nacional de la Patagonia San Juan Bosco (UNPSJB), Comodoro Rivadavia, Argentina
- 3Departamento de Biología, Universidad Nacional de la Patagonia San Juan Bosco (UNPSJB), Comodoro Rivadavia, Argentina
- 4Instituto de Silvicultura y Manejo de Bosques (INSIMA), Consejo Nacional de Investigaciones Cientificas y Tecnicas (CONICET), Universidad Nacional de Santiago del Estero (UNSE), Santiago del Estero, Argentina
- 5Instituto de Ecología Regional (IER), Consejo Nacional de Investigaciones Científicas y Técnicas (CONICET), Universidad Nacional de Tucumán, San Miguel de Tucumán, Argentina
- 6Instituto de Ecología, Genética y Evolución, Universidad de Buenos Aires - Consejo Nacional de Investigaciones Científicas y Técnicas (CONICET), Buenos Aires, Argentina
- 7Department of Biology, University of Miami, Coral Gables, FL, United States
- 8Consejo Nacional de Investigaciones Científicas y Técnicas (CONICET), Departamento de Ingeniería Forestal, Facultad de Ingeniería, Universidad Nacional de la Patagonia San Juan Bosco (UNPSJB), Esquel, Argentina
Stem capacitance and water storage are known to play an important role in the water economy of trees by acting as an intermediate water source for transpiring leaves. The bark, in addition to be involved in protection and mechanical support of the trees, can also serve as a water reservoir. We examined the relationship between inner bark and sapwood biophysical properties in tree species occurring in forests at the opposite ends of a moisture gradient in subtropical South America. We also assessed the relationships between wood density, growth rate and the magnitude of the water reservoir. The inner bark thickness varied between 1.89 and 0.50 cm across species and sites and there were not significant differences between forests. Inner bark capacitance of species from the dry forest was higher than sapwood capacitance, and the opposite was found in the moist forest. Sapwood capacitance (262 ± 80.8 Kg m−3 MPa−1) was significantly higher in the moist forest compared to the dry forest (41.9 ± 4.5 Kg m−3 MPa−1), while the opposite was found for inner bark (50.7 ± 8.4 and 83.1 ± 11.4 Kg m−3 MPa−1, respectively). Inner bark capacitance and density were linear and positively correlated across species, while for sapwood the relationship was well-described by a negative exponential function. In species with higher percentage of inner bark, the time lags in the daily contractions of bark and sapwood tissues were lower. Relative growth rate was negatively correlated with inner bark and sapwood density and positively with daily stored water used and percentage of inner bark across species and sites. Our results suggest that sapwood is a relevant water storage tissue in the trees of the moist forest while inner bark is important for tree functioning in the dry forest. High stem capacitance and water storage are needed to cope with short dry spells or seasonal periods of water deficit, and for maintaining growth rates. These stem properties will be more relevant under climatic scenarios with more frequent extreme drought events or seasonal reduction in precipitation in these forest ecosystems.
Introduction
The bark of woody plants plays a central role of protection against dehydration, fire, insects, diseases, provide mechanical support and storage of water, non-structural carbohydrates and other compounds (Scholz et al., 2007; Romero and Bolker, 2008; Midgley et al., 2010; Brando et al., 2012). Apart from plant size (Paine et al., 2010; Poorter et al., 2014), fire regime was originally described as the most important factor explaining bark thickness at a global scale (Ryan et al., 1988). Particularly, fire regimes can explain the thickness of the outer bark (dead cells) but provide little information of the living inner bark tissues (Rosell, 2016). The inner bark of trees, on the other hand, tends to be thicker in dry environments or sites with low soil water availability than in humid areas, and in environments with high temperatures as well. This suggests that water storage is also an important function of the inner bark in environments with low water availability and high evapotranspiration (Rosell, 2016).
Parenchyma cells in both the inner bark and sapwood serve as large water storage reservoirs (Scholz et al., 2008), which can be temporary used to replace water transpired by leaves (Goldstein et al., 1998; Phillips et al., 2003). The daily contribution of sapwood water storage to water loss by transpiration is in the order of 10 to 50% depending on the species and tree size (Goldstein et al., 1998; Steppe and Lemeur, 2004; Scholz et al., 2007, 2008; Oliva Carrasco et al., 2015). The water stored in the inner bark can supply water to the sapwood via the rays of both phloem and xylem (Pfautsch et al., 2015). This may occur when the evaporative demand of the atmosphere is very high both on a daily and seasonal scale or when the water availability in the soil is low (Chapotin et al., 2006; Nardini et al., 2011). Water transported from the inner bark to the sapwood is also essential to supply the expanding leaves, flowers and fruits (Borchert, 1994; Chapotin et al., 2006; di Francescantonio et al., 2018). Radial movements of water lead to changes in tissue volume in response to the discharge and recharge of internal water storages in the stems of woody species. These changes show typical daily patterns of contraction and expansion of the stem diameter (Scholz et al., 2008) that correlate with changes in volumetric water content of sapwood (Oliva Carrasco et al., 2015). It has been indicated that storage function of the bark is determined by the amount of tissue rather than tissue type (Rosell et al., 2014). If the selection pressure favors a thick inner bark in areas where storage water is an adaptive advantage, the thickness of the inner bark should correlate negatively with water availability and positively with air saturation deficit (Srivastava, 1964; Rosell and Olson, 2014).
There are numerous studies on morphological and functional characteristics of the bark and sapwood in different environments (Paine et al., 2010; Poorter et al., 2014; Rosell et al., 2014; Rosell, 2016). Tissue density serve as a proxy for many ecophysiological properties as it takes into account the structure and composition of the tissue. Combinations of cell types affect water and carbohydrate transport and storage, stress resistance, and ultimately tree growth (Scholz et al., 2007). There is a well-established negative relationship (trade-off) between wood density and growth rate (Chave et al., 2009), but the relationship between inner bark density and tree growth remain poorly studied. Poorter et al. (2014) observed a closed association between wood and bark traits in neotropical forests, with species having either high density tissues or high water content tissues and thick bark. These authors have also found that bark characteristics of species from dry and moist forests were more similar than bark characteristics of coexisting species in the same type of forest. In that study, however, differences between the inner and outer portion of the bark were not assessed. Subtropical woody species were in general less studied and there is little information linking biophysical properties of the inner bark with the storage of water and sapwood characteristics. Particularly important is separating living from non-living bark tissues, which may contribute differently to metabolic processes and water supply to the transpirational stream.
We examined the relationship between bark and sapwood biophysical properties in tree species occurring in forests at the opposite ends of a moisture gradient in subtropical South America. We studied seven tree species in a dry forest in the Chaco region and 10 species in a moist forest of the semideciduous Atlantic Forest. Particularly we focused on inner bark and sapwood density and capacitance, and its relation with bark thickness. We also assessed the relationship between sapwood and inner bark density, growth rate and water use of reservoirs for all the species studied. Both inner bark and sapwood function as water reservoirs in trees but contribute differently due to species-specific anatomical characteristics. Because bark has in general lower tissue density and a greater water content than sapwood, it would also have a larger capacitance and will deliver a greater amount of water to maintain a favorable water balance than the corresponding sapwood tissue in any particular species. We expected species from dry forests to have greater sapwood density and lower sapwood capacitance than species from humid forests, which could be partially compensated by inner bark thickness or capacitance. Therefore, we expected species in the dry forests to have a relatively thicker inner bark than species from the moist forest which would contribute in a greater proportion to the water balance of the trees, or a similar inner bark thickness but with a greater capacitance. If there were a trade-off between stem tissue density and growth rate, a negative correlation between both sapwood and inner bark density and stem growth rates across species could be expected independently of the type of forest.
Materials and Methods
Study Area and Plant Species
The research was carried out in forests at the extremes of a water availability gradient in subtropical Argentina corresponding to dry and moist Holdrige life zones (Derguy et al., 2019). The driest site is located in the Pizarro National Reserve (RNP, 24° 08′ S, 64° 05′ W) and the moist forest was located in the Iguazú National Park (PNI, 25° 31′ S, 54° 08′ W). Rainfall in the dry forest ranges between 750 and 900 mm per year, concentrated between November and March (Loto and Bravo, 2020), while in the moist forest average annual rainfall is ~2,000 mm uniformly distributed throughout the year (Supplementary Figure 1A). Mean monthly air saturation deficits (ASD) show a similar pattern in both forests (Supplementary Figure 1B), but reaching higher values in the dry forest, particularly during summer. However, maximum ASD could be as high as 4 kPa in the moist forest in dry hot summer days (Campanello et al., 2008). Although this is a subtropical moist forest, it is floristically linked to seasonally dry neotropical forests sharing many tree species and genus with that phytogeographic vegetation domain (Pennington et al., 2009).
The data in the PNI were collected between the years 2013 to 2016, while the data in the RNP were collected during the month of June 2016 in the dry season and in December 2016 and February 2017 in the wet season. Seventeen canopy tree species differing in wood biophysical properties, growth rates and leaf habits were studied (7 from the RNP and 10 from the PNI). All studied species are diffuse-porous mature trees (Table 1). The height of the main stem and diameter at breast height (DBH) were measured in healthy adult trees (DBH > 10 cm). Between 7 to 10 and 12 to 20 individuals were measured for stem height and DBH, respectively, depending on the species.
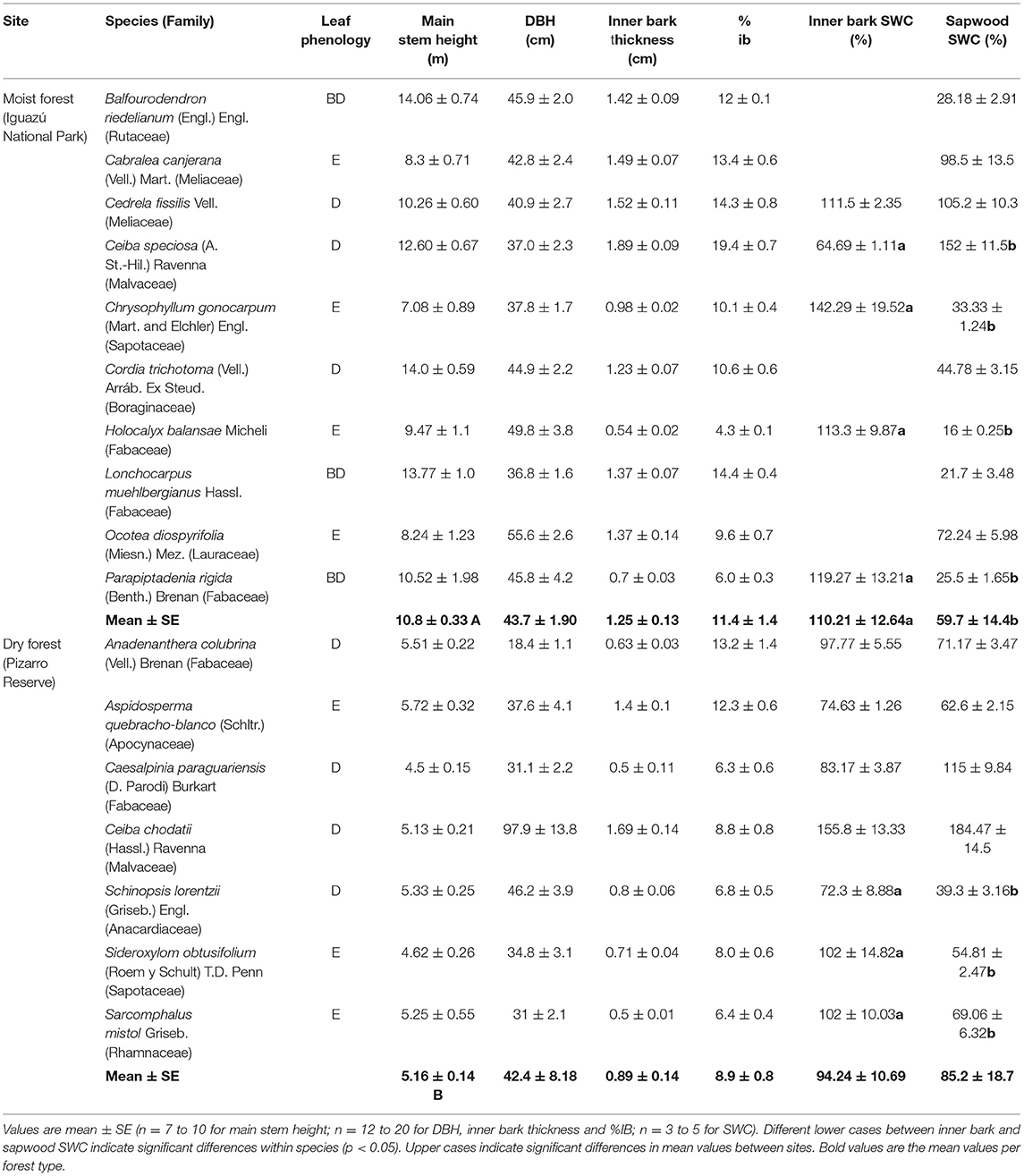
Table 1. Leaf phenology (D, deciduous; BD, brevideciduous; E, evergreen), main stem height, diameter at breast height (DBH), inner bark thickness, inner bark saturated water content (SWC) and sapwood SWC of the studied tree species from moist (Iguazú National Park) and dry forest (Pizarro Reserve) in the extremes of a precipitation gradient in northern Argentina.
Inner Bark, Sapwood and Sap Flow Measurements
Inner bark thickness was measured in 12 to 20 individuals per species. All dead bark tissues of the trees were carefully removed. A piece of 1 cm2 of the inner bark was taken with the help of a chisel. The thickness of each sample was recorded with an electronic digital caliper with a precision of 0.5 mm. The percentage of inner bark (% ib) was obtained taking into account the area of each tissue respect to the total cross section area of the individual.
Between 3 and 5 individuals having a similar DBH within each species were used for the estimation of hydraulic capacitance, tissue density and sap flow measurements. Most of the individuals selected had a DBH between 30 and 45 cm. In the case of moist forest, inner bark capacitance was estimated only in five species (Ceiba speciosa, Cedrela fissilis, Parapiptadenia rigida, Holocalyx balansae and Chrysophyllum gonocarpum).
Inner bark and sapwood density (ρ) were measured by the water-displacement method on tree core samples of the main stem (outer bark was previously removed). Tissue density was calculated as
where M is the dry mass of the sample (oven dried at 60°C for 72 h) and V is the sample volume. Volume was estimated by submerging the sample in a container with distilled water resting on a digital balance with a 0.001 g precision. The sample was kept submerged during measurements until saturation with the help of a very small needle, without touching the walls of the container. It was not possible to determine the inner bark density in two species of the moist forest (Balfourodendron riedelianum and Ocotea diospyrifolia) since the samples disintegrate and lose their shape completely, making the determination of tissues volumes very difficult and imprecise.
To determine tissue capacitance cylinders of the inner bark and sapwood were obtained with a 5-mm increment borer in the main stem of trees, sealed in glass vials and transported to the laboratory. Samples were allowed to hydrate in distilled water for <2 h to avoid over saturation. After hydration, samples were quickly blotted to remove excess water, placed in the caps of thermocouple psychrometer chambers (JRD Merrill Specialty Equipment, Logan, UT, USA), samples were weighed and then sealed inside the chamber for determination of water potential isotherms. The psychrometer chambers were then placed in an insulated water bath and allowed to equilibrate for at least 3 h before measurements with a dew point microvoltmeter (HR-33T, Wescor, Logan, UT, USA). Measurements were repeated at frequent intervals until the water potential values stabilized. The chambers were opened and the samples were allowed to dehydrate for different time intervals, reweighed in the psychrometer caps, resealed inside the psychrometer chambers and allowed to equilibrate for another determination of water potential. Moisture release curves were generated by plotting water potential against the relative water content. The relationships between sapwood water potential and relative water content were used to calculate inner bark and sapwood capacitance as described in Meinzer et al. (2003). Capacitance was normalized by the tissue volume to facilitate comparison of absolute amounts of water released per unit decline in water potential. Inner bark and sapwood capacitance (C; kg m−3 MPa−1) was calculated as follows:
where W is the weight of water per unit volume of tissue and Ψ is the water potential of the tissue (Scholz et al., 2007).
To calculate the contribution of water storage to daily transpiration sap flow was measured with the heat dissipation method (Granier, 1985, 1987; Clearwater et al., 1999) near the base of the main stem and in a branch in each tree. Temperature differences between probes were recorded every 10 s and 10 min averages were stored in solid- state storage modules (SM192, Campbell Scientific, Logan, UT, USA) connected to data loggers (CR 10×, Campbell Scientific). Corrections for natural temperature gradients between probes were made according to Do and Rocheateu (2002). Wound effects were minimize by measuring during short periods of time (from 7 to 15 days) after installation. Sapwood cross-sectional area of stems and branches were obtained by the dye-injection method. Sapwood areas were determined from the dyed portion of cylinders extracted with an 5 mm increment borer. Whole-crown transpiration was calculated according to Goldstein et al. (1998). The contribution of storage water to daily transpiration was obtained as the percentage of the discharged water related to the sap flow at the base of the stem. A more detailed description of methods and calculations can be found in Oliva Carrasco et al. (2015).
Sapwood and Inner Bark Diameter Variations
The stem diameter variations were measured in 3 to 5 individuals per species in each one of the 17 species studied. While for simultaneous variations (inner bark and sapwood) between 2 and 3 individual per species were measured in 3 evergreen species (Sideroxylon obtusifolium and Aspidosperma quebracho–blanco in the dry forest, and H. balansae in the moist forest) and 3 deciduous species (Schinopsis lorentzii in the dry forest, and C. speciosa and C. fissilis in the moist forest).
Diurnal microvariations in stem radius (inner bark and sapwood) were continuously monitored with point electronic dendrometers with very low temperature sensitivity (0.27 μm °C−1) (ZN11- T-WP; Zweifel Consulting, Hombrechtikon, Switzerland). The electronic displacement sensor (linear motion potentiometer) was in contact with the tissues. Two sensors were installed per tree, one of them in contact with the inner bark (outer bark was removed) and the other in contact with the sapwood, consequently diurnal radius variations mainly reflected changes in water content in both the xylem and active bark tissues. Other factors such as turgor drive growth may be involved (Steppe et al., 2006) but the magnitude of diurnal variations should be relatively small compared with changes in water content in both xylem and living cells in the bark. The sensor in the sapwood was maintained for shorter periods, as water loss by the xylem is generally higher and measurements may be erroneous after a few days. Time lags were calculated by finding the highest cross correlation of time series between inner bark and sapwood diameters (Sevanto et al., 2002).
Volumetric Water Content (VWC) and Tree Growth
Stem volumetric water content was measured simultaneously with diameter variations. Moisture sensors based on the FDR technology (Model GS3, Decagon Devices, Inc., Pullman, WA, USA) were installed in the main stem sapwood at breast height. The details of sensor installation and calibration have been described by Hao et al. (2013). Because laboratory and factory calibrations were very similar, factory calibration was used in this study. Data were obtained using EM50 data loggers (Decagon Devices, Inc.) every 10 min for different time periods.
Tree growth rate in the humid forest was measured with band dendrometers installed at 1.3 m (DBH) in 12 individuals per species. Diameter increment was measured monthly from February 2012 to February 2015. In the dry forest growth rate was measured with a tree caliper in all the individuals of the different species present in 0.4 ha permanent plots from 2007 to 2012. The mean annual increment for DBH was calculated for the different species and tree size classes. Growth rate of the individuals was then estimated by taking into account the DBH of the particular tree species and considering the relative growth rate of the corresponding DBH size class.
Analyses
To test for differences between sites in the biophysical properties a Student's test were performed by using the SPSS 11.5 statistical package (SPSS, Chicago IL, USA). All data were tested for normal distribution and homogeneity of variance. Correlations between two variables were performed with linear or non-linear regression analysis. The regressions were fitted by using Sigma Plot 14 software (Systat Softwate, Inc., Chicago, IL, USA).
Results
There were significant differences between sites in main stem height. Forest in the dry extreme of the precipitation gradient had trees with lower main stem height [t(15): 5.69; p < 0.0001] (Table 1). Diameter at breast height (DBH) was similar between sites (Table 1). The inner bark thickness varied between 1.89 and 0.50 cm across species and sites, and there were no significant differences between moist and dry forests (Table 1). No correlation between inner bark thickness and tree diameter or stem height was found across species and sites. There was a positive linear relationship between DBH and bark thickness for each one of the species studied. The percentage contribution of inner bark is fairly constant for individuals with different tree diameter within each species. The contribution from inner bark to DBH tended to be lower in the dry forest; however there were no significant differences (Table 1).
Inner bark exhibited lower interspecific variability in density (0.28 to 0.79 g cm−3) than sapwood (0.21 to 1.19 g cm−3) (Figure 1A). All study species had lower inner bark density than sapwood density, except Ceiba chodatti (Figure 1A). Mean inner bark densities in the moist and dry forest were 30% and 40%, respectively, lower than mean sapwood density in each case [t(12): 2.32; p = 0.05 and t(12): 3.82; p = 0.0025, for moist and dry forest, respectively]. There were no significant differences in inner bark and sapwood density between sites (Figure 1B).
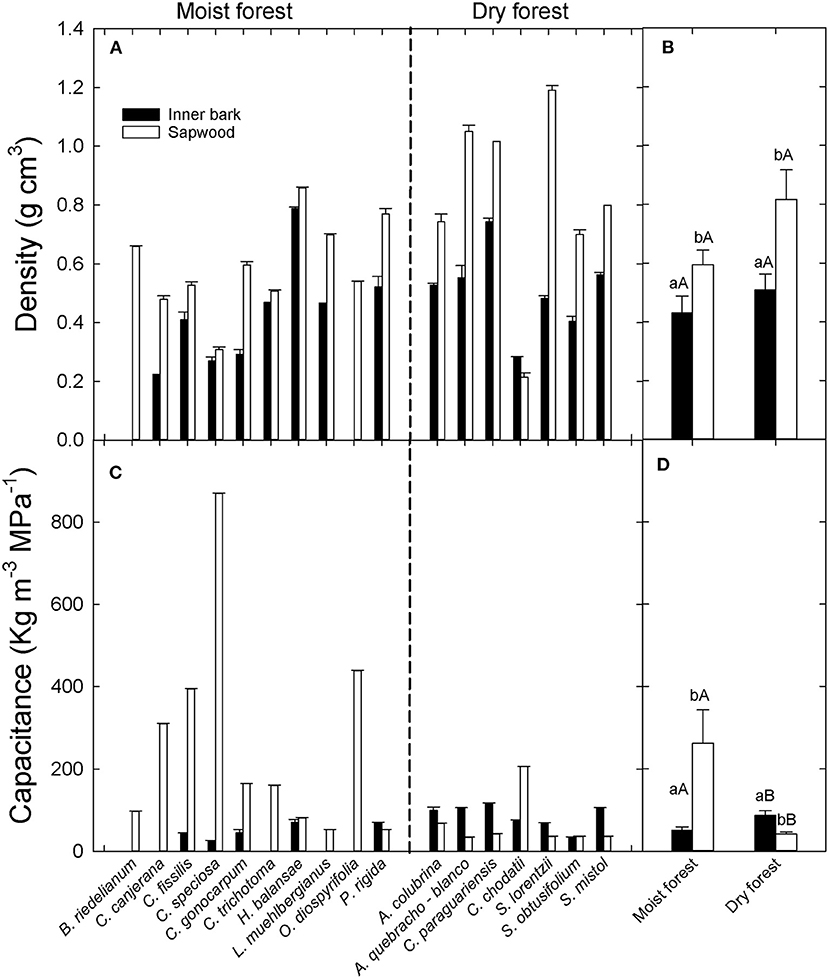
Figure 1. (A) Inner bark and sapwood density per species (A) and per site (B) and inner bark and sapwood capacitance per species (C) and per site (D) in two forests in the extremes of a precipitation gradient in northern Argentina. Different lower cases in (B,D) indicate significant differences between inner bark and sapwood within site. Different upper cases indicate significant differences in inner bark or sapwood between sites.
Inner bark capacitance did not show a defined pattern compared to sapwood capacitance across species (Figure 1C). Similar to density, inner bark capacitance showed lower interspecific variability than sapwood capacitance (28 to 120 Kg m−3 MPa−1 and 33 to 870 Kg m−3 MPa−1). While all species (except C. chodatii) from the dry forest exhibited higher inner bark capacitance than sapwood, most species in the wet extreme of the gradient had substantially lower capacitance in the inner bark. Significant differences in capacitance between tissues were found only in the dry forest (Figure 1D). Inner bark capacitance was similar between sites but sapwood capacitance (262 ± 80.8 Kg m−3 MPa−1) was significantly higher in the moist forest than in the dry forest (41.9 ± 4.5 Kg m−3 MPa−1) (U:2000; p > 0.0001) (Figure 1D).
Inner bark capacitance and thickness were correlated with inner bark density across species and sites (Figure 2). However, inner bark capacitance and density were linear and positively correlated (Figure 2A) while for bark thickness there was a negative exponential relationship (Figure 2B). A positive relationship between inner bark and sapwood capacitance was also observed across species and sites (data not shown: y = 0.17 + 0.43x; R2 = 0.51; p = 0.003). On the other hand, sapwood capacitance and contribution of water storage to daily transpiration (%) were negatively and exponentially correlated with sapwood density across species and sites (Figures 3A,B, respectively). There was no correlation between the contribution of stored water to transpiration and inner bark density and capacitance.
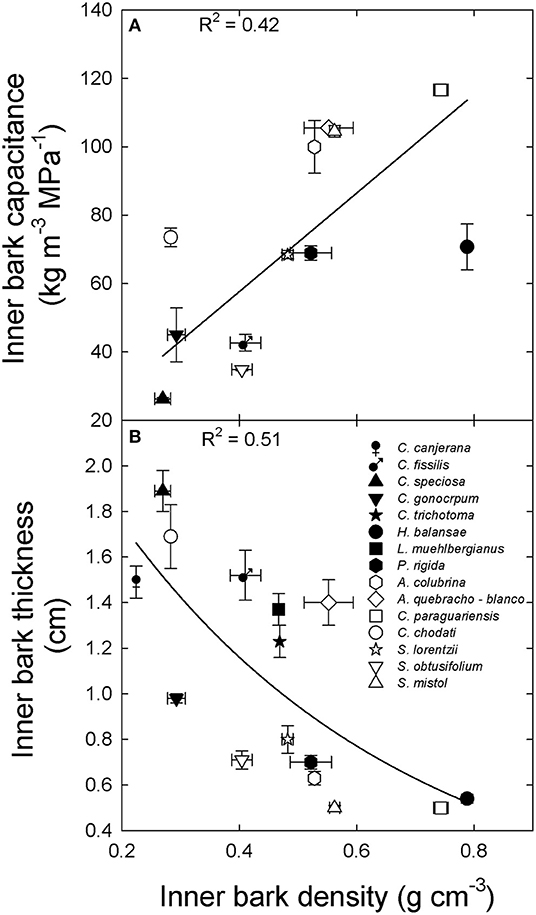
Figure 2. Relationship between (A) inner bark capacitance and (B) inner bark thickness and inner bark density for tree species from the extremes of a precipitation gradient in northern Argentina. Black symbols correspond to wet extreme and white symbols to dry extreme. Lines are the functions fixed to the data [(A) y = 144x, p < 0.001; (B) y = 2.6 exp−2x; p < 0.001].
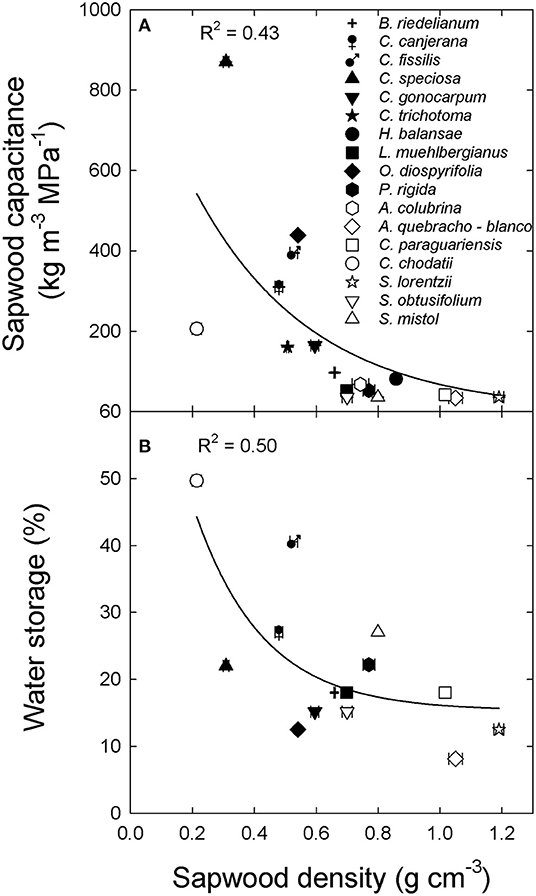
Figure 3. (A) Sapwood capacitance and (B) contribution of water storage to daily transpiration (water storage) and sapwood density for tree species from the extremes of a precipitation gradient in northern Argentina. Black symbols correspond to wet extreme and white symbols to dry extreme. The lines are the functions fitted to the data [(A) y = y= 144x, p < 0.001; (B) y = 15.3 + 76.5 exp−4.5x, p < 0.001].
Saturated water content tended to be higher in inner bark than in sapwood in both extremes of the precipitation gradient; however the difference was statistically significant only in the case of the moist forest [t(13): −2.24; p = 0.04] (Table 1). Both sites exhibited similar SWC of inner bark and sapwood.
Patterns of contraction and expansion of inner bark and sapwood for six tree species exhibiting different combinations of sapwood and inner bark capacitance are shown in Figure 4. Species with high inner bark capacitance (e.g. A. quebracho-blanco and H. balansae) exhibited a decrease in inner bark radius when sapwood radius increased. The opposite tendency was observed when sapwood radio decreased (Figures 4A,E). On the other hand, species with low inner bark capacitance exhibited inner bark contraction simultaneously with sapwood contraction (e.g., C. speciosa and C. fissilis) or expansion (e.g. S. lorentzi and S. obtusifolium). In addition, the species C. speciosa, that has high sapwood capacitance and low inner bark capacitance (Figure 4C) showed a similar behavior in the contraction and expansion of inner bark and sapwood than species with low sapwood capacitance but high inner bark capacitance (e.g. A. quebracho-blanco, Figure 4A).
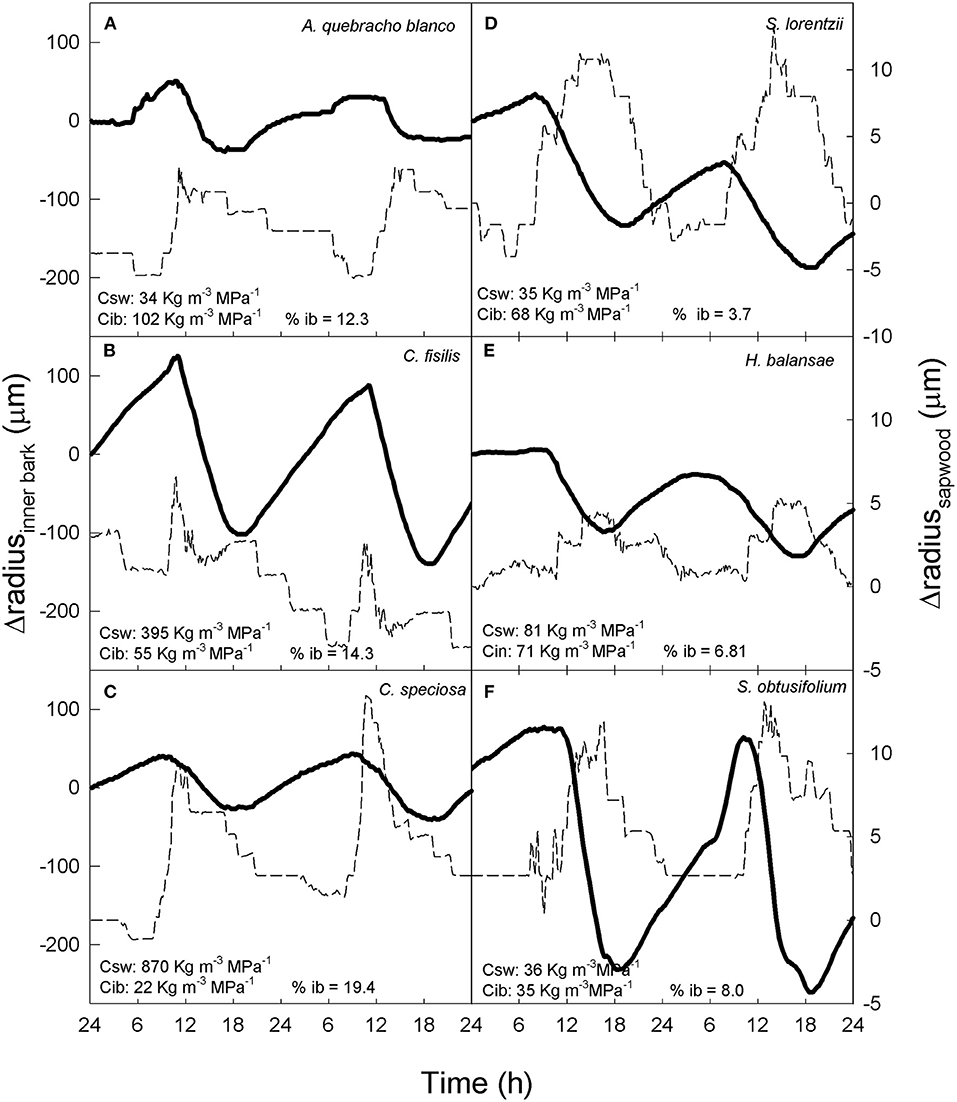
Figure 4. (A–F) Changes in radius of inner bark (solid line) and sapwood (dashed line) in six trees species with different sapwood capacitance (Csw), inner bark capacitance (Cib) and percentage of inner bark (% ib).
In summary, the patterns of contraction between both tissues were related to the percentage of inner bark (Figure 4). Although in all study species inner bark radius decreased before sapwood, species with higher percentage of inner bark exhibited a radius decline of inner bark more coupled to the radius decline of sapwood (Figures 4A–C). On the other hand, in species with low percentage of inner bark contraction of both tissues occurred at very different time (Figures 4D–F). The lag time between contraction of inner bark and sapwood was linear and negatively correlated with the percentage of inner bark across six species including dry and moist forests (Figure 5). Similar to contraction, inner bark exhibited earlier expansion than sapwood, however there was no relationship between time lags in the expansion of tissues and percentage of inner bark.
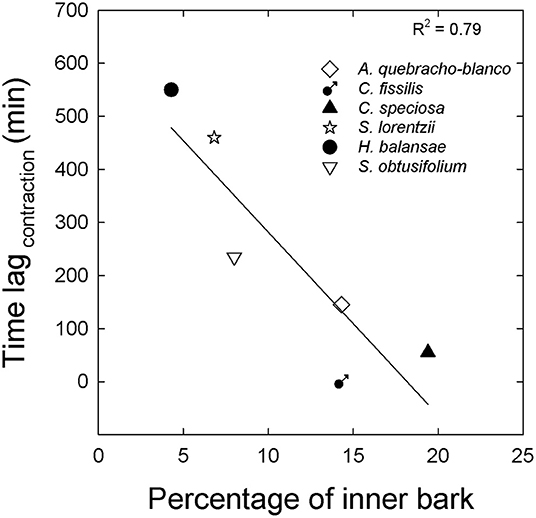
Figure 5. Lag time between inner bark contraction and sapwood contraction in relation to the percentage of inner bark for three typical species from moist forest (close symbols) and three typical species from dry forest (open symbols). The line in A is the linear function fitted to the data y = 623–34x; p < 0.001.
Relative growth rate decreased exponentially with sapwood and linearly with inner bark density (Figures 6A,B) across species and sites. Inversely, an exponentially positive relationship was found between growth rate and the contribution of water storage to daily transpiration (Figure 6C), and percentage of inner bark (Figure 6D).
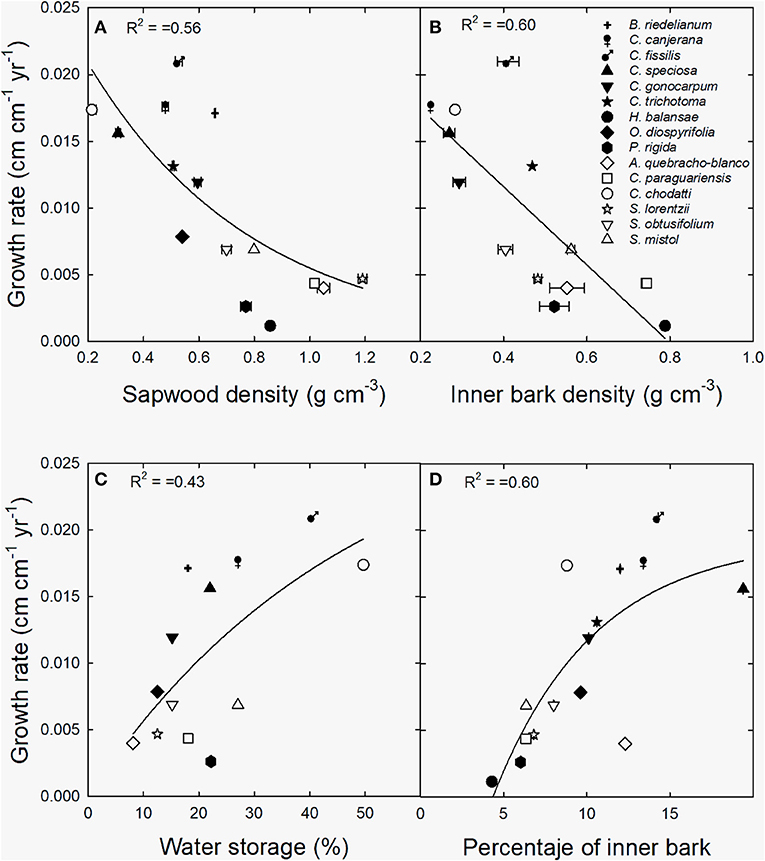
Figure 6. Relationship between growth rate and (A) sapwood density, (B) inner bark density, (C) contribution of water storage to daily transpiration (water storage) and (D) percentage of inner bark. Black symbols correspond to wet extreme and white symbols to dry extreme. The lines are the functions fitted to the data [(A) y = 0.38 + 2.8 exp−0.83x, p < 0.0001; (B) y = 0.023–0.029x, p < 0.01; (C) y = 0.03*(1-exp−0.021x), p < 0.001; (D) y = −0.020 + 0.039*(1–exp−0.16x), p < 0.001].
Discussion
Convergence in sapwood and inner bark functionality for species from two subtropical forests at the extremes of a precipitation gradient was observed in this study. Contrary to the general assumption that the inner bark is thicker in dry than in moist forests, we found no differences among sites for the subtropical species studied. However, the results suggest that different compensating mechanisms may operate to withstand water deficits, particularly sapwood appeared to be a relevant water storage tissue in the moist forest while inner bark was more important in the dry forest. As expected, tissue density and water storage were important characteristics for growth in trees that experience short dry spells or seasonal droughts.
Convergence in Sapwood and Inner Bark Functional Traits
Previous studies found different results when relating bark thickness in trees of dry forests compared to wet or moist forest (Poorter et al., 2014; Rosell and Olson, 2014). However those studies did not differentiate between inner and outer bark. Because, outer bark is influenced by fire frequency and damage protection (Paine et al., 2010; Lawes et al., 2013; Rosell, 2016) it might hinder the potential association of inner bark with traits related to carbon and water storage. In this study differences in inner bark thickness were not observed. We also expected a higher contribution of inner bark to DBH in the dry forest, supposing more water could be storage to supply the transpiration stream, but percentage inner bark tended to be higher in the moist forest although differences were not significant. Instead, capacitance differed between sites, and was higher in trees in the dry forest. Scholz et al. (2011) indicated that the size of a tissue is related with the water storage capacity; however, it does not imply that a higher amount of water can be withdrawn and used from that tissue. In this case, a greater inner bark capacitance in the dry forest could contribute to compensate the lower investment in this type of tissue. On the contrary, trees in the moist forest showed larger sapwood capacitance than in the dry forest. Higher sapwood capacitance in trees of the moist forests could partially compensate for the effects of path length resistance and gravity on xylem tension (Ryan and Yoder, 1997; Koch et al., 2004) as the trees are taller than in the dry forest. A positive correlation between tree height and sapwood capacitance has been observed across 35 tree species including angiosperms and gymnosperms (Scholz et al., 2011).
Due to the overlapping storage function of sapwood and inner bark, and considering they are both ontogenetically linked, morpho-physiological characters of these tissues can be expected to be related across species (Rosell, 2019). Anatomical differences are responsible of functional convergence and trade-offs among biophysical characters of these tissues. For example, sapwood density is lowered by relative quantity of parenchyma cells that are capable of stored water, and thus a less dense tissue can store and deliver more water if required. In the case of inner bark, a positive correlation of capacitance and density was observed. Different anatomical traits could affect the inner bark density and capacitance. For example the ability of parenchyma cells to release water depends on cell wall elasticity which enables changes in cell volume (Scholz et al., 2007; Jupa et al., 2016). On the other hand, tissue density could be affected by wall thickness, cell size and packing degree. This positive relationship between inner bark capacitance and density suggest no trade-off between mechanical support and ability to release water of the tissue. However, a negative relationship between inner bark density and thickness was observed, possibly indicating that trees with low inner bark density need a thicker bark to compensate for functions other than water storage and carbohydrate translocation. Indeed, inner bark provides mechanical support and protection against herbivory (Niklas, 1999; Rosell et al., 2015). Different type of cells (e.g., sclereids, fibers, sieve tubes, axial and ray parenchyma) support the multiple functions while affecting tissue density (Angyalossy et al., 2016).
Daily Patterns of Tissue Contraction and Expansion
The contribution of inner bark to stem was related to sapwood capacitance across species, and partially explained the different patterns of daily contraction and expansion of both stem tissues. Daily fluctuations in stem tissues showed the typical pattern of contractions during the day and expansion in the night, which is associated to water discharge and recharge of reservoirs (Scholz et al., 2007; Oliva Carrasco et al., 2015). These tissues are tightly interconnected and osmotic potential changes in the floem strongly influence xylem water potentials, and vice versa (Hölttä et al., 2006, 2009). Water is withdrawn from the bark to the xylem when the tension increments during the day, and the xylem deliver water to the bark at night when water reservoirs recharge. This water movement explains the patterns of water contraction of tissues during the day and expansion at night. In trees having a higher contribution of inner bark, daily contraction of sapwood and inner bark were more coupled than for trees with low percentage of inner bark. A greater percentage of inner bark could contribute to the early and coupled peak of sapwood respect to the inner bark expansion in some species (e.g., C. fissilis in the moist forest and A. quebracho-blanco in the dry forest). Inner bark is a low hydraulic resistance tissue with high water content that can rapidly deliver water to the transpirational stream. A decoupling of tissue expansion and contraction, as observed in H. balansae and S. lorentzii, has been also reported for other species, and could indicate a high radial hydraulic resistance between both tissues, or changes in sugar content in the phloem (De Schepper and Steppe, 2010; Sevanto et al., 2011; Mencuccini et al., 2013). Hydraulic resistance and sugar content are both affected by environmental variables as both depend on temperatures and physiological activity (De Schepper and Steppe, 2010; Steppe et al., 2012). In a seasonal basis, apart of being an indication of physiological processes such as leaf flushing (Chapotin et al., 2006; di Francescantonio et al., 2018), stem contraction can occur as a result of water reservoir depletion under low water availability (Zweifel et al., 2001; Drew et al., 2008; Steppe et al., 2008; King et al., 2013; Köcher et al., 2013), and might be an indication of tree responses to variable climate conditions.
Stem Tissue Characteristics, Water Storage and Growth Rates
In closed-canopy moist forests, where solar radiation is limited, sustaining growth is important for trees to access the upper canopy. Instead, in dry forest water availability is the principal limitation and a more conservative strategy of water use could be expected. According to the results on tissue capacitance and on percentage inner bark, sapwood would contribute more to water relations maintenance and storage in the moist forest while inner bark would be more relevant for trees in the dry forest. Indeed, contrary to the expectation of general higher capacitance for inner bark than for sapwood, there was a tendency of inner bark capacitance to be lower than sapwood capacitance in the moist forest. This observation suggests that sapwood could be more suitable source of water to buffering daily water deficit while water storage in the inner bark could buffer longer term water demand. Thus, sapwood water reservoirs could release water more efficiently to transpiration stream under transient and sporadic dry spells occurring in the moist forest (Oliva Carrasco et al., 2015). A rapid water release allows a fast daily response and the maintenance of a large water potential gradient and daily photosynthesis to keep high growth rates. Water released from inner bark to xylem might be more gradual and may contribute to water use and the maintenance of a favorable water balance in species exposed to a seasonal drought in the dry forests.
In this study expected differences in sapwood and inner bark density between moist and dry forests were not observed. However, these variables were negatively related to growth rate across tree species and sites, indicating there are differences in construction costs and growth strategies across species in both types of forests. The negative correlation between sapwood density and growth rates has been largely studied for different tropical forests (see for example, Burslem and Whitmore, 1999; Enquist et al., 1999; Muller-Landau, 2004; Kunstler et al., 2016). Wood density reflect a combination of anatomical elements that determine water transport capacity and tissue resistance to different stressful conditions (Bucci et al., 2004; Scholz et al., 2007). On the other hand, bark is associated to water transport, non-structural carbohydrates storage, mechanical resistance and damage defense. A recent study in the Brazilian savanna has shown that inner bark characteristics reflect different metabolic and growth strategies of trees (Loram-Lourenço et al., 2020). In the present study, the negative correlation between inner bark density and growth rate reinforces this idea, and shows the importance of storage capacity as determinant of tree growth in the subtropical forests studied.
Stored water use and percentage of inner bark also correlated positively with growth rates in this study. On one side, the using of water reservoirs may sustain transpiring leaves and thus the maintenance of high photosynthesis rates during the day by avoiding hydraulic failure (Goldstein et al., 1998), which might translate into increased growth rates as observed in the studied species. On the other hand, it is known the role of inner bark as a non-structural carbohydrates storage tissue, which support metabolic processes such as turgor maintenance and growth (Rosell et al., 2021). For example, bark density correlated positively with carbon assimilation and growth across 31 tree species of the Brazilian savanna (Loram-Lourenço et al., 2020). We have previously shown that the use of water stored in stem tissues is specially relevant during leaf flushing in trees of the moist forest (di Francescantonio et al., 2018). The results obtained in the present study reinforce the importance that water storage and inner bark function have in both subtropical ecosystems for tree growth.
Conclusion
In this study we linked both xylem and living bark tissue properties and highlighted its role for the maintenance of water balance and growth in subtropical species. We found that in dry forests the inner bark can release water to the transpirational stream due its high capacitance, while in moist forests that experience only sporadic and transient water deficits, the stored water in the sapwood can be more rapidly released. However, we observed a combination of plant traits that allow trees of both forest types to cope with drought. Trees with reduced inner bark capacitance can compensate for this by having a high proportion of this tissue that allows the release of stored water to vessel xylem to supply the leaf water demand. These results indicate that not only the intrinsic stem tissue properties but also the amount of inner bark are key drought resistance factors in subtropical trees with contrasting precipitation.
Data Availability Statement
The raw data supporting the conclusions of this article will be made available by the authors, without undue reservation.
Author Contributions
LC: conceptualization, data collection, analyses, and manuscript revision. SB: conceptualization, data collection, analyses, and manuscript edition. FS: conceptualization, data collection, and manuscript revision. DL and IG: data collection and manuscript revision. GG: conceptualization, funding acquisition, analyses, and manuscript revision. PC: conceptualization, funding acquisition, analyses, and manuscript writing. All authors contributed to the article and approved the submitted version.
Funding
The Consejo Nacional de Investigaciones Científicas y Técnicas (CONICET) and Agencia Nacional de Promoción Científica y Tecnológica (PICT 0001 PITEC NA007/006, PICT 2014 0039) provided funding for this research.
Conflict of Interest
The authors declare that the research was conducted in the absence of any commercial or financial relationships that could be construed as a potential conflict of interest.
Publisher's Note
All claims expressed in this article are solely those of the authors and do not necessarily represent those of their affiliated organizations, or those of the publisher, the editors and the reviewers. Any product that may be evaluated in this article, or claim that may be made by its manufacturer, is not guaranteed or endorsed by the publisher.
Acknowledgments
We would like to thank Oscar Lezcano, Raul Sanchez, Franco Sosa, Abraham Rojas and staff from Pizarro National Reserve. We also thank the Administración de Parques Nacionales (APN) for permitting access to the Iguazu National Park. We are grateful to editor and reviewers that contributed to improve the manuscript.
Supplementary Material
The Supplementary Material for this article can be found online at: https://www.frontiersin.org/articles/10.3389/ffgc.2022.793385/full#supplementary-material
References
Angyalossy, V., Pace, M. R., Evert, R. F., Marcati, C. R., Oskolski, A. A., Terrazas, T., et al. (2016). IAWA list of microscopic bark features. IAWA J. 37, 517–615. doi: 10.1163/22941932-20160151
Borchert, R. (1994). Water status and development of tropical trees during seasonal drought. Trees 8, 115–125. doi: 10.1007/BF00196635
Brando, P. M., Nepstad, D. C., Balch, J. K., Bolker, B., Christman, M. C., Coe, M., et al. (2012). Fire-induced tree mortality in a neotropical forest: the roles of bark traits, tree size, wood density and fire behavior. Glob. Change Biol. 18, 630–641. doi: 10.1111/j.1365-2486.2011.02533.x
Bucci, S. J., Goldstein, G., Meinzer, F. C., Scholz, F. G., Franco, A. C., and Bustamante, M. (2004). Functional convergence in hydraulic architecture and water relations of tropical savanna trees from leaf to whole plant. Tree Physiol. 24, 891–899. doi: 10.1093/treephys/24.8.891
Burslem, D. F. R. P., and Whitmore, T. C. (1999). Species diversity, susceptibility to disturbance and tree population dynamics in tropical rain forests. J. Veg. Sci. 10, 767–776. doi: 10.2307/3237301
Campanello, P. I., Gatti, M. G., and Goldstein, G. (2008). Coordination between water transport efficiency and photosynthetic capacity in canopy tree species under different growth irradiances. Tree Physiol. 28, 85–94. doi: 10.1093/treephys/28.1.85
Chapotin, S. M., Razanameharizaka, J. H., and Holbrook, N. M. (2006). Baobab trees (Adansonia) in Madagascar use stored water to flush new leaves but not to support stomatal opening before the rainy season. New Phytol. 169, 549–559. doi: 10.1111/j.1469-8137.2005.01618.x
Chave, J., Coomes, D., Jansen, S., Lewis, S. L., Swenson, N. G., and Zanne, A. E. (2009). Towards a worldwide wood economics spectrum. Ecol Lett. 12, 351–366. doi: 10.1111/j.1461-0248.2009.01285.x
Clearwater, M. J., Meinzer, F. C., Andrade, J. L., Goldstein, G., and Holbrook, N. M. (1999). Potential errors in measurement of no uniform sap flow using heat dissipation probes. Tree Physiol. 19, 681–687. doi: 10.1093/treephys/19.10.681
De Schepper, V., and Steppe, K. (2010). Development and verification of a water and sugar transport model using measured stem diameter variations. J. Exp. Bot. 61, 2083–2099 doi: 10.1093/jxb/erq018
Derguy, M. R., Frangi, J. L., Drozd, A. A., Arturi, M. F., and Martinuzzi, S. (2019). Holdridge life zone map: Republic of Argentina. Gen. Tech. Rep. IITF-GTR-51. San Juan, PR: U.S. Department of Agriculture, Forest Service, International Institute of Tropical Forestry. 48. doi: 10.2737/IITF-GTR-51
di Francescantonio, D., Villagra, M., Goldstein, G., and Campanello, P. I. (2018). Leaf phenology and water-use patterns of canopy trees in Northern Argentinean subtropical forests. Tree Physiol. 38, 1841–1854. doi: 10.1093/treephys/tpy072
Do, F., and Rocheateu, A. (2002). Influence of natural temperature gradients on measurements of xylem sap flow with thermal dissipation probes. 1. Field observations and possible remedies. Tree Physiol. 22, 641–648. doi: 10.1093/treephys/22.9.641
Drew, D. M., O'Grady, A. P., Downes, G. M., Read, J., and Worledge, D. (2008). Daily patterns of stem size variation in irrigated and unirrigated Eucalyptus globulus. Tree Physiol. 28, 1573–1581. doi: 10.1093/treephys/28.10.1573
Enquist, B. J., West, G. B., Charnov, E. L., and Brown, J. H. (1999). Allometric scaling of production and life-history variation in vascular plants. Nature 401, 907–911. doi: 10.1038/44819
Goldstein, G., Andrade, J. L., Meinzer, F. C., Holbrook, N. M., Cavalier, J., Jackson, P., et al. (1998). Stem water storage and diurnal patterns of water use in tropical forest canopy trees. Plant Cell Environ. 21, 397–406. doi: 10.1046/j.1365-3040.1998.00273.x
Granier, A. (1985). Une nouvelle méthode pour la mesure du flux de séve brute dans le tronc des arbres. Ann Sci Forest. 42,193–200. doi: 10.1051/forest:19850204
Granier, A. (1987). Evaluation of transpiration in a Douglas fir stand by mean of sap flow measurement. Tree Physiol. 3, 309–320. doi: 10.1093/treephys/3.4.309
Hao, G.-H., Wheeler, J. K., Holbrook, N. M., and Goldstein, G. (2013). Investigating xylem embolism formation, refilling and water storage in tree trunks using frequency domain reflectometry. J. Exp. Bot. 64, 2321–2332. doi: 10.1093/jxb/ert090
Hölttä, T., Mencuccini, M., and Nikinmaa, E. (2009). Linking phloem function to structure: analysis with a coupled xylem-phloem transport model. J. Theor. Biol. 259, 325–337. doi: 10.1016/j.jtbi.2009.03.039
Hölttä, T., Vesala, T., Sevanto, S., Perämäki, M., and Nikinmaa, E. (2006). Modeling xylem and phloem water flows in trees according to cohesion theory and Münch hypothesis. Trees 20, 67–78. doi: 10.1007/s00468-005-0014-6
Jupa, R., Plavcová, L., Gloser, V., and Jansen, S. (2016). Linking xylem water storage with anatomical parameters in five temperate tree species. Tree Physiol. 36, 756–769. doi: 10.1093/treephys/tpw020
King, G., Fonti, P., Nievergelt, D., Buentgen, U., and Frank, D. (2013). Climatic drivers of hourly to yearly tree radius variations along a 6 degrees C natural warming gradient. Agric. For. Meteorol. 168, 36–46. doi: 10.1016/j.agrformet.2012.08.002
Koch, G., Sillett, S., Jennings, G., and Davis, S. D. (2004). The limits to tree height. Nature 428, 851–854. doi: 10.1038/nature02417
Köcher, P., Horna, V., and Leuschner, C. (2013). Stem water storage in five coexisting temperate broad-leaved tree species: significance, temporal dynamics and dependence on tree functional traits. Tree Physiol. 33, 817–832. doi: 10.1093/treephys/tpt055
Kunstler, G., Falster, D., Coomes, D. A., Hui, F., Kooyman, R. M., Laughlin, D. C., et al. (2016). Plant functional traits effects on competition. Nature 529, 204–207. doi: 10.1038/nature16476
Lawes, M. J., Midgley, J. J., and Clarke, P. J. (2013). Costs and benefits of relative bark thickness in relation to fire damage: a savanna/forest contrast. J. Ecol. 101, 517–524. doi: 10.1111/1365-2745.12035
Loram-Lourenço, L., Farnese dos Santos, F., Ferreira de Sousa, L., Ferreira Barros Alves, R. D., Pereira de Andrade, M. C., da Silva Almeida, S. E., et al. (2020). A structure shaped by fire, but also water: ecological consequences of the variability in bark properties across 31 species from the Brazilian Cerrado. Front. Plant Sci. 10:1718. doi: 10.3389/fpls.2019.01718
Loto, D., and Bravo, S. (2020). Species composition, structure, and functional traits in Argentine Chaco forests under two different disturbance histories. Ecol. Indic. 113, 106232. doi: 10.1016/j.ecolind.2020.106232
Meinzer, F. C., James, S. A., Goldstein, G., and Woodruff, D. (2003). Whole-tree water transport scales with sapwood capacitance in tropical forest canopy trees. Plant Cell Environ. 26, 1147–1155. doi: 10.1046/j.1365-3040.2003.01039.x
Mencuccini, M., Höltt,ä, T., Sevanto, S., and Nikinmaa, E. (2013). Concurrent measurements of change in the bark and xylem diameters of trees reveal a phloem-generated turgor signal. New Phytol. 198, 1143–1154. doi: 10.1111/nph.12224
Midgley, J. J., Lawes, M. J., and Chamaillé-Jammes, S. (2010). TURNER REVIEW No. 19. Savanna woody plant dynamics: the role of fire and herbivory, separately and synergistically. Aust. J. Bot. 58, 1–11. doi: 10.1071/BT09034
Muller-Landau, H. C. (2004). lnterspecific and inter-site variation in wood specific gravity of tropical trees. Biotropica 36, 20–32. doi: 10.1111/j.1744-7429.2004.tb00292.x
Nardini, A., Gullo, M., and Salleo, S. (2011). Refilling embolized xylem conduits: is it a matter of phloem unloading? Plant Sci. 180, 604–611. doi: 10.1016/j.plantsci.2010.12.011
Oliva Carrasco, L., Bucci, S. J., di Francescantonio, D., et al. (2015). Water storage dynamics in the main stem of subtropical tree species differing in wood density, growth rate and life history traits. Tree Physiol. 35, 354–365. doi: 10.1093/treephys/tpu087
Paine, C. E. T., Stahl, C., Courtois, E. A., Patiño, S., Sarmiento, C., and Baraloto, C. (2010). Functional explanations for variation in bark thickness in tropical rain forest trees. Funct. Ecol. 24, 1202–1210. doi: 10.1111/j.1365-2435.2010.01736.x
Pennington, R. T., Lavin, M., and Oliveira-Filho, A. (2009). Woody plant diversity, evolution, and ecology in the tropics: perspectives from seasonally dry tropical forests. Ann. Rev. Ecol. Evolut. Syst. 40, 437–457. doi: 10.1146/annurev.ecolsys.110308.120327
Pfautsch, S., Renard, J., Tjoelker, M. G., and Salih, A. (2015). Phloem as capacitor: radial transfer of water into xylem of tree stems occurs via symplastic transport in ray parenchyma. Plant Physiol. 167, 963–971. doi: 10.1104/pp.114.254581
Phillips, N. G., Ryan, M. G., Bond, B. J., McDowell, N. G, Hinckley, T. M., and Cermak, J. (2003). Reliance on stored water increase swith tree size in three species in the Pacific Northwest. Tree Physiol. 23, 237–245. doi: 10.1093/treephys/23.4.237
Poorter, L., McNeil, A., Hurtado, V. H., Prins, H. H. T., and Putz, F. E. (2014). Bark traits and life-history strategies of tropical dry- and moist forest trees. Funct. Ecol. 28, 232–242. doi: 10.1111/1365-2435.12158
Romero, C., and Bolker, B. M. (2008). Effects of stem anatomical and structural traits on responses to stem damage: an experimental study in the Bolivian Amazon. Can. J. For. Res. 38, 611–618. doi: 10.1139/X07-205
Rosell, J. A. (2016). Bark thickness across the angiosperms: more than just fire. New Phytol. 211, 90–102. doi: 10.1111/nph.13889
Rosell, J. A. (2019). Bark in woody plants: understanding the diversity of a multifunctional structure. Integr. Comp. Biol. 59, 535–547. doi: 10.1093/icb/icz057
Rosell, J. A., Castorena, M., Laws, C. A., and Westoby, M. (2015). Bark ecology of twigs vs. main stems: functional traits across eighty-five species of angiosperms. Oecologia 178, 1033–1043. doi: 10.1007/s00442-015-3307-5
Rosell, J. A., Gleason, S., M?ndez-Alonzo, R., Chang, Y., and Westoby, M. (2014). Bark functional ecology: evidence for tradeoffs, functional coordination, and environment producing bark diversity. New Phytol. 201, 486–497. doi: 10.1111/nph.12541
Rosell, J. A., and Olson, M. E. (2014). The evolution of bark mechanics and storage across habitats in a clade of tropical trees. Am. J. Bot. 101, 764–777. doi: 10.3732/ajb.1400109
Rosell, J. A., Piper, F. I., Jiménez-Vera, C., Vergílio, P. C. B., Marcati, C. R., Castorena, M., et al. (2021). Inner bark as a crucial tissue for non-structural carbohydrate storage across three tropical woody plant communities. Plant Cell Environ. 44, 156–170. doi: 10.1111/pce.13903
Ryan, K. C., Peterson, D. L., and Reinhardt, E. D. (1988). Modeling long-term fire-caused mortality of Douglas fir. For. Sci. 34, 190–199.
Ryan, M. G., and Yoder, B. J. (1997). Hydraulic limits to tree height and tree growth. BioScience 47, 235–242. doi: 10.2307/1313077
Scholz, F. G., Bucci, S. J., Goldstein, G., Meinzer, F. C., Franco, A. C., and Miralles- Wilhelm, F. (2007). Biophysical properties and functional significance of stem water storage tissues in Neotropical savanna trees. Plant Cell Environ. 30, 236–248. doi: 10.1111/j.1365-3040.2006.01623.x
Scholz, F. G., Bucci, S. J., Goldstein, G., Meinzer, F. C., Franco, A. C., and Miralles- Wilhelm, F. (2008). Temporal dynamics of stem expansion and contraction in savanna trees: withdrawal and recharge of stored water. Tree Physiol. 28, 469–480. doi: 10.1093/treephys/28.3.469
Scholz, F. G., Phillips, N. G., Bucci, S. J., Meinzer, F. C., and Goldstein, G. (2011). “Hydraulic capacitance; biophysic and functional significance of internal water sources in relation to tree size,” in Size- and Age-Related Changes in Tree Structure, eds F. C. Meinzer, B. Lachenbruch, and T. E. Dawson TE (New York, NY: Springer), 341–361. doi: 10.1007/978-94-007-1242-3_13
Sevanto, S., Hölttä, T., and Holbrook, N. M. (2011). Effects of the hydraulic coup- ling between xylem and phloem on diurnal phloem diameter variation. Plant Cell Environ. 34, 690–703. doi: 10.1111/j.1365-3040.2011.02275.x
Sevanto, S., Vesala, T., Perämäki, M, and Nikinmaa, E. (2002). Time lags for xylem and stem diameter variations in a Scots pine tree. Plant Cell Environ. 25, 1071–1077. doi: 10.1046/j.1365-3040.2002.00884.x
Srivastava, L. M. (1964). Anatomy, chemistry, and physiology of bark. Int. Rev. For. Res. 1, 203–277. doi: 10.1016/B978-1-4831-9975-7.50010-7
Steppe, K., Cochard, H., Lacointe, A., and Ameglio, T. (2012). Could rapid diameter changes be facilitated by a variable hydraulic conductance? Plant Cell Environ. 35, 150–157. doi: 10.1111/j.1365-3040.2011.02424.x
Steppe, K., De Pauw, D. J. W., and Lemeur, R. (2008). Validation of a dynamic stem diameter variation model and the resulting seasonal changes in calibrated parameter values. Ecol. Model. 218, 247–259. doi: 10.1016/j.ecolmodel.2008.07.006
Steppe, K., De Pauw, D. J. W., Lemeur, R., and Vanrolleghem, P. A. (2006). A mathematical model linking tree sap flow dynamics to daily stem diameter fluctuations and radial stem growth. Tree Physiol. 26, 257–273. doi: 10.1093/treephys/26.3.257
Steppe, K., and Lemeur, R. (2004). An experimental system for analysis of the dynamic sap-flow characteristics in young trees: Results of a beech tree. Funct. Plant Biol. 31, 83–92. doi: 10.1071/FP03150
Keywords: Atlantic Forest, capacitance, Chaco forest, density, tree growth rate, stem contraction, water storage
Citation: Carrasco LO, Bucci SJ, Scholz FG, Loto D, Gasparri I, Goldstein G and Campanello PI (2022) Biophysical Properties of Inner Bark and Sapwood in Tree Species From Forests With Contrasting Precipitation in Subtropical South America. Front. For. Glob. Change 5:793385. doi: 10.3389/ffgc.2022.793385
Received: 12 October 2021; Accepted: 20 March 2022;
Published: 14 April 2022.
Edited by:
Alicia Correa, University of Giessen, GermanyReviewed by:
Wakana Azuma, Kobe University, JapanJose Climent, Instituto Nacional de Investigación y Tecnología Agroalimentaria (INIA), Spain
Copyright © 2022 Carrasco, Bucci, Scholz, Loto, Gasparri, Goldstein and Campanello. This is an open-access article distributed under the terms of the Creative Commons Attribution License (CC BY). The use, distribution or reproduction in other forums is permitted, provided the original author(s) and the copyright owner(s) are credited and that the original publication in this journal is cited, in accordance with accepted academic practice. No use, distribution or reproduction is permitted which does not comply with these terms.
*Correspondence: Paula I. Campanello, cGNhbXBhbmVsbG9AZ21haWwuY29t