- 1Landscape Ecology and Environmental Systems Analysis, Institute of Geoecology, Technische Universität Braunschweig, Braunschweig, Germany
- 2Geography Department, Humboldt-Universität zu Berlin, Berlin, Germany
- 3Chair of Forest Growth and Yield Science, Technische Universität München, Munich, Germany
- 4Department of Evolutionary Biology and Environmental Studies, University of Zurich, Zurich, Switzerland
- 5Department of Biology, University of Konstanz, Konstanz, Germany
- 6Centre for the Advanced Study of Collective Behaviour, University of Konstanz, Konstanz, Germany
- 7Department of Collective Behavior, Max Planck Institute of Animal Behavior, Konstanz, Germany
- 8Norwegian Institute for Nature Research, Trondheim, Norway
- 9SLU Swedish Species Information Centre, Swedish University of Agricultural Sciences, Uppsala, Sweden
- 10Berlin-Brandenburg Institute of Advanced Biodiversity Research (BBIB), Berlin, Germany
The global increase in demand for wood products, calls for a more sustainable management of forests to optimize both the production of wood and the conservation of forest biodiversity. In this paper, we evaluate the status and future trends of forest birds in Central European forests, assuming different forest management scenarios that to a varying degree respond to the demand for wood production. To this end, we use niche models (Boosted Regression Trees and Generalized Linear Models) to model the responses of 15 forest bird species to predictors related to forest stand (e.g., stand volume of specific tree species) and landscape structure (e.g., percentage cover), and to climate (bioclimatic variables). We then define five distinct forest management scenarios, ranging from set-aside to productivity-driven scenarios, project them 100 years into the future, and apply our niche models into these scenarios to assess the birds’ responses to different forest management alternatives. Our models show that the species’ responses to management vary reflecting differences in their ecological niches, and consequently, no single management practice can benefit all species if applied across the whole landscape. Thus, we conclude that in order to promote the overall forest bird species richness in the study region, it is necessary to manage the forests in a multi-functional way, e.g., by spatially optimizing the management practices in the landscape.
Introduction
Forests cover nearly one third of the planetary land area and they hold over 80% of the global terrestrial biodiversity (Aerts and Honnay, 2011). European forests, however, are largely reduced in comparison to their original extent, and are generally subjected to intensive management for the global demand of wood products (Bengtsson et al., 2000). Forest decline results in forest biodiversity decline, which jeopardizes their functioning and provisioning of ecosystem services. Thus, it is important to sustainably manage European forests that secure the long-term functioning of these ecosystems, while also responding to the increasing global demand of wood products (Duffy, 2009; Aerts and Honnay, 2011; Mori et al., 2017).
Most forestry practices, such as thinning or harvesting, involve disturbances to the forest ecosystem, directly affecting their structure, composition, function and dynamics. Consequently, managed forests are usually simplified ecosystems, with largely unfavorable conditions for many forest dependent species, eventually leading to ecosystems dominated by common or generalist species (Bengtsson et al., 2000; Czeszczewik et al., 2015; Bouvet et al., 2016). However, specific management actions can also diversify forest structures in the horizontal and vertical directions, promoting species diversity (Toraño Caicoya et al., 2018). It is established that sustainable forest management should mimic natural disturbances and forest dynamics, to maintain forest characteristics and diversity (Bengtsson et al., 2000; Lindenmayer et al., 2006). Natural forests are generally heterogeneous, structurally diverse, with a high compositional diversity, and a considerable amount of deadwood (Lindenmayer et al., 2006; Czeszczewik et al., 2015). These forests typically have a considerable density of habitat trees (i.e., standing live or dead trees with cavities, bark pockets, large dead branches, cracks), providing habitat for diverse and species rich communities of forest birds (Bütler et al., 2013; Bouvet et al., 2016; Reise et al., 2019).
Forest management particularly affects the presence and density of old, typically large, senescent trees, and dead standing trees. These structures are necessary for cavity-nesting birds, such as woodpeckers that also find the majority of their food (mostly insects) in these structures. Habitat trees have a long formation time and are therefore rare, unless specifically spared in cutting operations. High levels of tree retention tend to have a positive influence on bird species richness, especially that of canopy and cavity-nesting birds which benefit from a high level of tree retention particularly in mixed and broadleaved forests (Basile et al., 2019). The vertical structural heterogeneity, including the height distribution of trees, and the number of tree layers, influences bird species richness through the provisioning of a variety of nesting and foraging sites, and food sources (Heidrich et al., 2020). These vertical structures also influence the local climate, light conditions and shelter, potentially affecting the reproductive success and predation risk of birds (Aikens et al., 2013). Moreover, also horizontal structural heterogeneity, e.g., tree density and size (diameter) distribution, influences local climate and light conditions, and therefore site suitability for bird species with different ecologies. For instance, the Wood Warbler (Phylloscopus sibilatrix) have been shown to prefer semi-open over dense forests (Mallord et al., 2012), while the Black Woodpecker (Dryocopus martius) is rather associated with forest tree diversity (Pirovano and Zecca, 2014). Diverse forests with several tree species are expected to host more bird species than monocultures, by offering a number of nesting places and food sources for a variety of bird species (Basile et al., 2019). However, the positive effect of high tree species diversity may be partly reduced by higher predation in such forests (Batáry et al., 2014). Finally, the presence of particular tree species, such as oaks, may additionally influence local bird communities. Bird species are strongly associated with or specialized in particular tree species or tree genera, such as the Golden Oriole (Oriolus oriolus) and the Middle Spotted Woodpecker (Dendrocoptes medius), which are associated with oaks.
Forest management can be modified in terms of intensity, timing and type of forestry activities, for the joint promotion of biodiversity and economic values (Basile et al., 2019). Especially in Central Europe, various types of continuous-cover forestry (CCF) management schemes have the potential to result in both high biodiversity and profitable forestry, as habitat for species is provided continuously through time. In CCF, wood production is not necessarily lower than in traditional rotation forestry that aims to maximize wood production via intensive harvesting of even-aged monocultures (Bianchi et al., 2020). Rotation forestry, however, is associated with low biodiversity values after the final felling, which may partially recover as the forest grows back. Nevertheless, applying rotation forestry to at least a portion of the landscape may promote species diversity as it can emulate beneficial natural processes like senescence and collapse (Zeller et al., 2021). The recently developed multifunctional forest management concept (Schwaiger et al., 2019) aims at securing multiple functions or ecosystems services that the forest provides, including biodiversity and wood production, as well as climate risk mitigation and carbon storage via a continuous crown cover and increased structural and tree species heterogeneity. For a more targeted promotion of biodiversity, the kind of trees known to be important to many species or specialist species, e.g., large and/or old trees, can be spared in cutting operations (Augustynczik et al., 2018). Extensive conservation, where natural disturbances drive the forest dynamics, is expected to lead to high biodiversity values, but at the expense of the economic outcome.
In this paper, we evaluate the status and future trends of forest birds in Central European forests, according to different forest management scenarios that represent existing and potential forestry regimes, and cover the abovementioned range of structures. Thus, scenarios that include novel silviculture practices that result in highly structured mixed stands are expected to increase bird occurrence. CCF, which is currently standard practice in Central European forestry may also improve or maintain current levels of bird species occurrence and diversity. Intensive silviculture scenarios with rotation forestry and even-aged management are expected to affect birds negatively. However, the effects of different forest management approaches on bird diversity across the landscape remain poorly understood. The specific challenges include, among others, the high level of landscape fragmentation (typical of Central Europe), new species mixtures, an increased proportion of old forest stands with high standing volumes, and a high demand for multifunctional forestry (Schindler et al., 2014).
Forest birds are good indicator species to assess the ecological value and diversity of forest ecosystems (Venier and Pearce, 2004; Gil-Tena et al., 2007) as they represent several trophic levels, are widespread, diverse, sensitive to environmental change, and easy to identify and survey (Leaver et al., 2019). To this end, we use an environmental niche modeling approach to establish the association between the bird occurrence patterns and forest structure. We applied these models to investigate the effects of different forest structural conditions, corresponding to the considered management scenarios simulated into the future. Specifically, we want to study trade-offs derived from intensive forest management, focusing on high wood production together with more multifunctionality oriented approaches, and the associated impacts that the application of such regimes may have on bird diversity. Although the assessment of forest management effects on forest birds are not novel (see e.g., Price et al., 2020), the use of niche modeling combined with forest management scenario projections to this aim is new and provides new insights into possible future management alternatives.
Methodology
Study Area
This study leveraged bird census and forest structure data of the Canton of Zurich, Switzerland (1,729 km2), located in the eastern part of the Swiss plateau (Figure 1). The climate is continental with strong influence from the Atlantic, having cold winters and warm summers, a mean annual temperature of 9.9°C, and mean annual precipitation of 1,179 mm. Forests cover ca. 31% of the surface and span from deciduous to subalpine conifer forests. Coniferous forests are the most common in the region, with dominance of Norway Spruce (Picea abies; 38%) and Silver Fir (Abies alba; 12%); the most common broadleaved tree species is European Beech (Fagus sylvatica), with a coverage of 23% (Amt für Landschaft und Natur, 2020).
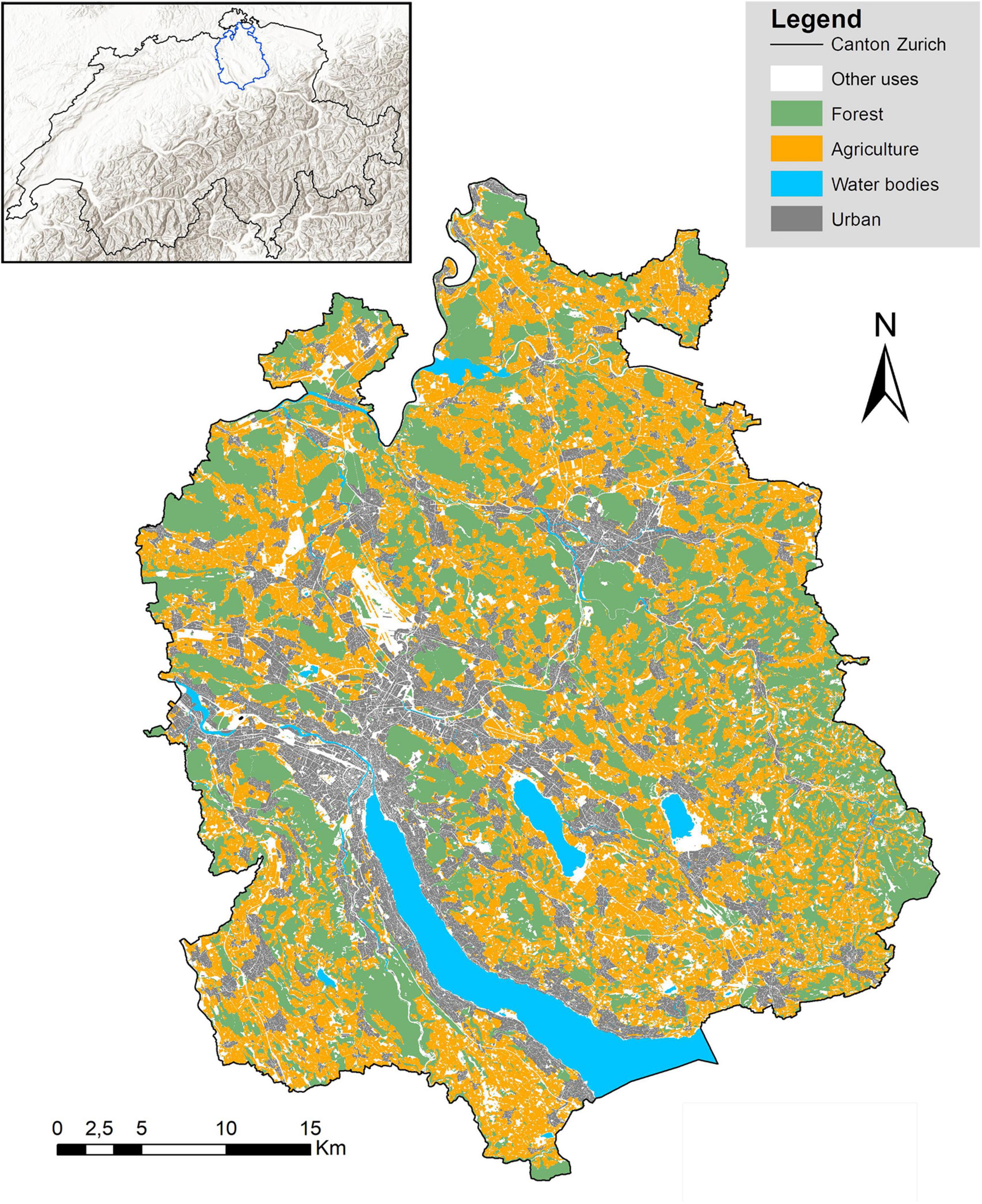
Figure 1. Our study area is the Canton of Zurich (blue line in the inset at the top left), in Switzerland (black line in the same inset). The main land use types within the study area are agricultural, forest and urban land. Data from Amt für Landschaft und Natur (2010).
The forests in the Canton of Zurich are representative for forests of the central European continental biogeographical region (Sabatini et al., 2021), which dominates Central Europe and the plains and valleys surrounding the Alps. These forests are mostly dominated by the abovementioned species, namely Norway spruce, European Beech and Silver Fir, as well as Scots Pine (Pinus sylvestris) and oak (Quercus sp.; Leuschner and Ellenberg, 2017).
Bird Data
We used data from the breeding bird atlas of the Canton of Zurich 2008 (Weggler et al., 2009), with a total of 1,440 habitat polygons (with sizes ranging from 6.4 to 80.2 ha; mean = 49.24 ± 0.30). We only used polygons, covering both forested and mixed use polygons that included forests. These data were collected from 2006 to 2008, using transect counts conducted by 250 trained volunteer ornithologists. Each polygon was surveyed 5 times between the 20th of March and 30th of June, for a period of 30 min, in the early hours of the day (from sunrise until 10 am), with every bird seen or heard recorded. We analyzed data from 15 forest bird species (Table 1; Keller et al., 2010), covering different habitat preferences and ranging from rare to common in the study region (prevalence values between 0.024 and 0.915). To reduce uncertainty in the data we reduced the original relative abundance information into presence and absence (abundance ≥ 0 converted to presence).
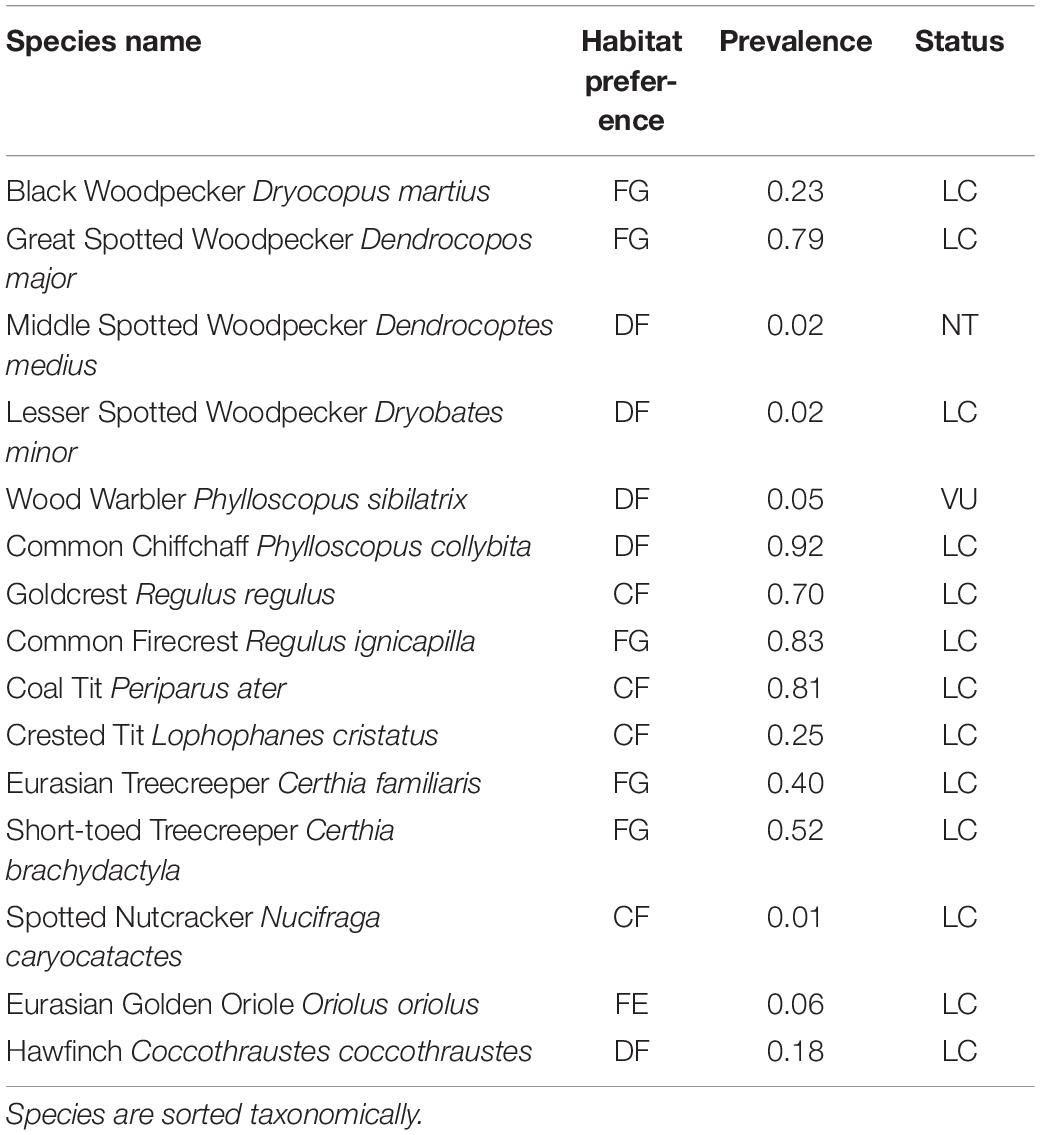
Table 1. List if forest bird species studied; respective habitat preference (FG, Forest generalist; DF, Deciduous forests; CF, Coniferous forests; FE, Forest edges); prevalence in the study region and conservation status in Switzerland (Keller et al., 2010; Knaus et al., 2021): LC, Least Concern; NT, Near Threatened; VU, Vulnerable).
Environmental Data
We chose predictors for our ecological niche models relating to forest stand structure, landscape structure, and climate, likely of relevance for the 15 bird species, as described below.
Two sources of forest inventory data were available for this study: the national forest inventory of Switzerland and the forest inventory of the Canton of Zurich (Brassel and Lischke, 2001). The sample densities of the national inventories are 1 × 1 km for the first inventory (Brändli, 1996), and 1.4 × 1.4 km for the second and third inventories (Brassel and Lischke, 2001; Brändli, 2010), using a system of concentric plots for different diameter classes (200 m2 for diameter at breast height (dbh) < 12 cm, 500 m2 for dbh < 36 cm and an auxiliary plot for ingrowth). The data from the Canton of Zurich has a denser grid, with circular inventory plots at each 80 × 300 m and a fixed sized of 300 m2. The main data set used for the simulation was the one from the Canton of Zurich, due to the denser grid, while we used the national forest inventory data to calibrate the dbh-height relationship for not measured heights in the Canton’s data.
We selected forest variables that are likely of ecological relevance for the focal species, and possible to produce during simulations of future forest dynamics and management with the forest simulator SILVA (Pretzsch et al., 2002; Pretzsch et al., 2008). The selected variables (Table 2) were calculated for three scales. These are, the entire stand, each tree species group (broadleaves and conifers), and each of the (most common) individual tree species that are implemented in SILVA: Norway Spruce, Silver Fir, Scots Pine, European Beech, Common and Sessile Oak (Quercus robur and Q. petrea, considered together due to their structural similarities), as well as European Larch (Larix decidua). Additionally, the North American Douglas Fir (Pseudotsuga menziesii), although not very common in the study area, was considered for the future scenarios due to the increasing interest in its use in Europe (Isaac-Renton et al., 2014). The mean value of each predictor among the inventory plots within each forest stand was assigned to the respective stand (Amt für Landschaft und Natur, 2010). As forest stands (delineated on the forest map described below) were considerably smaller than the bird sampling polygons, the variables needed to be compiled to the bird polygon level by calculating the area-weighted mean value of all contained forest stands (for all variables), and the respective standard deviation as a measure of heterogeneity (for the stand level variables only). Tree density is the total number of trees divided by the area of the inventory plot; crown coverage is the ratio between the space occupied by crowns and the plot area; tree volume is calculated as a function of the tree’s basal area, tree height, and species-specific form factors; and tree diversity is the Shannon diversity index calculated from the tree density values for each tree species.
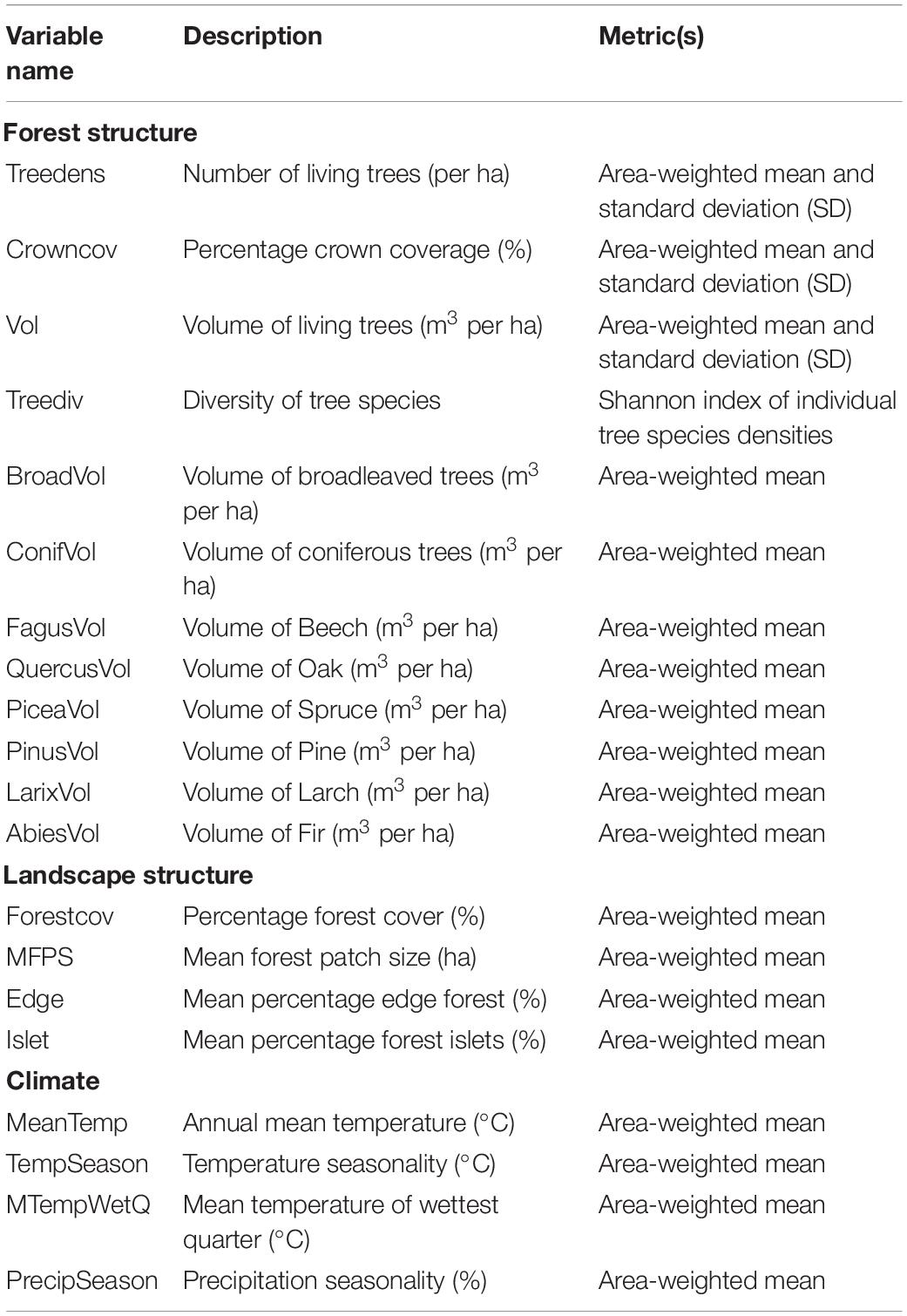
Table 2. Predictor variables depicting forest structure, landscape structure, and climate used as candidates in the niche modeling process.
The landscape structure variables were calculated based on forest stand data (Table 2). Percentage forest cover and mean forest patch size were directly derived from a forest map, compiled after an aerial photographic survey, and obtained from the Amt für Landschaft und Natur (2010). The remaining three variables were calculated using the Guidos Toolbox (Soille and Vogt, 2009; Vogt and Riitters, 2017), considering a forest edge width of 50 m. Forest islets are small, isolated “islands” of forests within the open landscape. The four climatic variables (Table 2) chosen were Annual mean temperature, Temperature seasonality (annual range in temperature), Mean temperature of wettest quarter and Precipitation seasonality (annual range in precipitation), and were extracted from the CHELSA “bioclim” variable dataset (Karger et al., 2017).
Forest Simulations With SILVA
Forest simulations were performed using the forest simulator SILVA (Pretzsch et al., 2002, 2008) which was developed to support practitioners of sustainable forest management. SILVA is a single-tree-based model that is distance-dependent (tree positions matter) and age-independent. The time scale to be simulated ranges from 5 years up to a rotation period. SILVA is optimized for the most important tree species in central Europe in pure and mixed stands. With local adjustment, e.g., based on inventory data, it can be tuned for about 80% of the central European forests.
The core equation for estimating the site dependent height growth potential is a Chapman-Richards function:
which describes a tree’s potential height hpot at a given age t dependent on three site-dependent (and species specific) parameters a, k, and p (Kahn, 1994; Pretzsch et al., 2002). Using height-age relationships from long term research data from experimental plots located in the Canton of Zurich (data provided by the Swiss Federal Institute for Forest, Snow and Landscape Research -WSL), new parameters (a, k, and p) were fitted for each tree species and groups abovementioned and the corresponding curves were implemented in SILVA. The long-term research offered the advantage of covering a broad age-height range for all species used in SILVA. The 90% quantile was used to fit the potential growth curves to avoid biases caused by thinning experiments.
SILVA simulates growth of single trees in forested conditions. As the competition among trees is evaluated in a spatially explicit way, the model can cover a broad range of existing, and also novel, silvicultural concepts. SILVA can simulate for even-aged or uneven-aged mixed and monospecific forests. The model is generally applied stand-wise, but, as it is the case in this study, it can also be applied on a landscape level where grid-based forest inventory data are available.
For model initialization, we grouped all structure data from the inventory plots into representative strata based on the main dominant species, diameter distribution, top height and land tenure type. Each stratum was simulated independently to improve computing performance. Once the simulations were ready, the structural information was transferred to each inventory point. In a second step, the structure variables were aggregated for each of homogenous forest patch (∼0.6 ha) as described in section “Environmental Data.”
Forest Management Scenarios
We investigated the difference in predicted bird occurrence patterns between future potential and realistic forest management scenarios. We selected five different scenarios which cover the main silvicultural regimes applied in Central Europe. Each scenario is described by harvesting intensities and the main types of silviculture. Thus, we can simulate silvicultural regimes ranging from wood production oriented, involving high harvest intensities, to more conservation-oriented silvicultural regimes with a higher emphasis on biodiversity (close-to-nature) or protection (set-asides). The scenarios are (see below for their description): Continuous cover; Multifunctional; Wood production; Habitat tree; and Set-aside (Table 3). Each scenario was simulated for 100 years, in 5-year intervals.
The Continuous cover scenario simulates an intensified version of continuous cover regimes that are widely applied by Central European forest offices. Productive monocultures, including Norway Spruce and Scots Pine stands, are managed using selective thinning including the future crop tree thinning concept (Bücking et al., 2007), while Beech stands are managed according to the shelter-wood concept, with different intensities depending on site quality. Thus, the main objective of this scenario is the sustainable wood production with a focus on forest stability and resilience. As silviculture in the Canton of Zurich is similar to the one applied in Bavaria, we decided to use prescriptions that are already included in SILVA for the Bavarian State Office.
The Multifunctional scenario is designed to balance different forest ecosystem functions and services simultaneously. These include wood production together with groundwater recharge, carbon sequestration and biodiversity. Thus, the predominant objectives of this scenario include a continuous crown cover and an active promotion of high structural heterogeneity and tree species diversity, including a high share of deciduous species. Specifically, silviculture is applied as follows: up to a tree height of 12 m, the stand is simulated through a stem number reduction that must not exceed a total volume of 15 m3/ha. Selective thinning is conducted at tree heights between 12 and 32 m, to remove up to 55 m3/ha in deciduous stands and up to 70 m3/ha in coniferous stands. The target diameter harvest phase starts at tree heights of 32 m and removed between 80 and 144 m3/ha of conifers and 70 m3/ha in deciduous-dominated stands. All the conducted treatments are applied in turns of 10 years (Toraño Caicoya et al., 2018). In this scenario, natural regeneration is the main contributor to regrowth. To improve species diversity, Scots Pine (500 trees/ha) is planted during the regeneration phase in the conifer-dominated stands. In the deciduous-dominated stands, additional to the natural regeneration (Poschenrieder et al., 2018), Scots Pine (250 trees/ha) and Douglas Fir (250 trees/ha) are planted during the regeneration phase to increase the stand’s multifunctionality, and additionally European Beech (6,000 trees/ha) is planted to ensure that the desired species mix is achieved.
The Wood production scenario focuses on the production of wood, where the volume of harvested wood is the first priority. It assumes an increase in the demand of wood products in Europe within the next 100 years. In this scenario, stem reduction is applied on deciduous tree species, removing up to 25 m3/ha, while no stem reduction is applied to conifer-dominated stands. In the deciduous stands, a selective thinning is applied at a tree height of 12–17 m, and thinning from below for a tree height of 17–30 m, removing up to a total of 25 m3/ha. The final felling is conducted by a light selective thinning and a target diameter felling of trees with a dbh diameter between 20 and 200 cm and a removal of up to 500 m3 of the standing volume. In the conifer stands, strong selective thinning and target diameter felling is applied in two height phases. During the first phase from 12 to 19 m, up to 60 m3/ha are removed, targeting conifers with a dbh diameter between 40 and 200 cm and broadleaves with diameters > 5 cm. During the second phase, up to 120 m3/ha are removed for diameters between 50 and 200 cm for all species. The final felling phase aims at trees with a height > 31 m, applying a light selective thinning and a target diameter felling with a removed standing volume of up to 500 m3/ha and a diameter between 5 and 200 cm for deciduous trees and a diameter between 20 and 200 cm for conifers. In addition to the natural regeneration (Poschenrieder et al., 2018), Norway Spruce (4,000 trees/ha) and Douglas Fir (100 trees/ha) are planted during the regeneration phase.
The Habitat tree scenario is a variation of the multifunctional scenario. This scenario aims at increasing the number of large trees remaining in the stand. For all tree species, residual large trees are promoted, however, with a preference for broadleaves and Oak in particular. To achieve this, the regeneration phase is modified as follows: for stands with a broadleaved species as dominant species, the maximum diameter during the target diameter felling is reduced for any possible admixture of oak and broadleaves until 60 cm, and leaving 20% of the volume for the oak and 15% for the rest of the broadleaved species standing. The intensity of the selective thinning for oak (being a light demanding species) is intensified to favor its growth. For the stands with a conifer species as dominant species, the multifunctional scenario remains unaltered for the conifer admixtures, but no treatment is applied to any broadleaved admixture in the regeneration phase. In summary, in broadleaved stands around 15–20% of the broadleaves are allowed to grow until they reach their natural mortality, favoring trees with dbh diameter > 15 cm, and in conifer stands all broadleaves in the admixture are left without management.
The Set-aside scenario, in which no management was conducted and the forest dynamics was driven solely by natural mortality and regeneration, was used as the reference scenario. The bird responses in the other scenarios were compared to the bird responses the Set-aside scenario.
Niche Modeling
The bird occurrence data were fitted to the environmental data using Boosted Regression Trees (BRTs; Friedman et al., 2000; Elith et al., 2008). BRTs combine decision trees and boosting in a single algorithm. While decision trees relate a response to their predictors through recursive binary splits, boosting combines many simple models to give improved predictive performance. This way, BRTs work as an additive regression model where many individual decision tree models are fitted in a forward stagewise fashion (Elith et al., 2008). Thus, they are very flexible and capable of dealing with complex (non-linear) responses, and are robust to missing data. Also, they include a stochastic element in the boosting process, which by randomly selecting subsamples (bag fraction) to fit the data at each iteration, makes them robust against overfitting (Friedman, 2002). For this reason, BRTs are highly performing and are a primary choice for ecological niche (or habitat) modeling (e.g., Elith et al., 2006).
We used BRTs as implemented in R version 3.6.2 (R Development Core Team, 2020), using code from the package “gbm” version 3.1.5 (Ridgeway, 2019). We ran the BRTs using the recommended bag fraction of 0.5 (Elith et al., 2008). The BRT parameterization is done by defining three main parameters: the maximum number of splits in each decision tree (tree complexity); the number of trees to use (model complexity); and the model regularization (or shrinkage) parameter (Elith et al., 2008). The ideal parameter set depends on the particular data used, so we first defined the number of trees to be used for each case via cross-validation by maximizing the model log likelihood. The tree complexity and shrinkage parameters were defined via a heuristic search of every possible pair combination, again in a cross-validation procedure, by optimizing each model’s area under the receiver operating characteristics curve (AUC; Swets, 1988).
Initially, we fitted one model per species using all 23 predictors considered. In a second step, we simplified the models, based on the initial models’ variable contributions, and by ensuring that highly correlated variables (r ≥ 0.7) were excluded, thus avoiding further modeling problems (Dormann et al., 2013). In the final (reduced) models, we included six forest structural, two landscape structural, and two climatic variables per model, and in this way gave emphasis to forest structural variables (whose effect is investigated), while acknowledging the role of landscape and climate (Table 4). The final BRT models were reiterated using Generalized Linear Models (GLMs; Hosmer and Lemeshow, 2000) to assess consistency on the species responses (when using a more parsimonious modeling approach). The GLMs were developed by testing to include linear and quadratic terms for all of the ten predictors included in the final models for each species, and then running a multi-model selection (Burnham and Anderson, 2004) based on the Akaike Information Criterion (AIC; Akaike, 1974). This analysis was performed in the R package “MuMIn” version 1.43.17 (Barton, 2020).
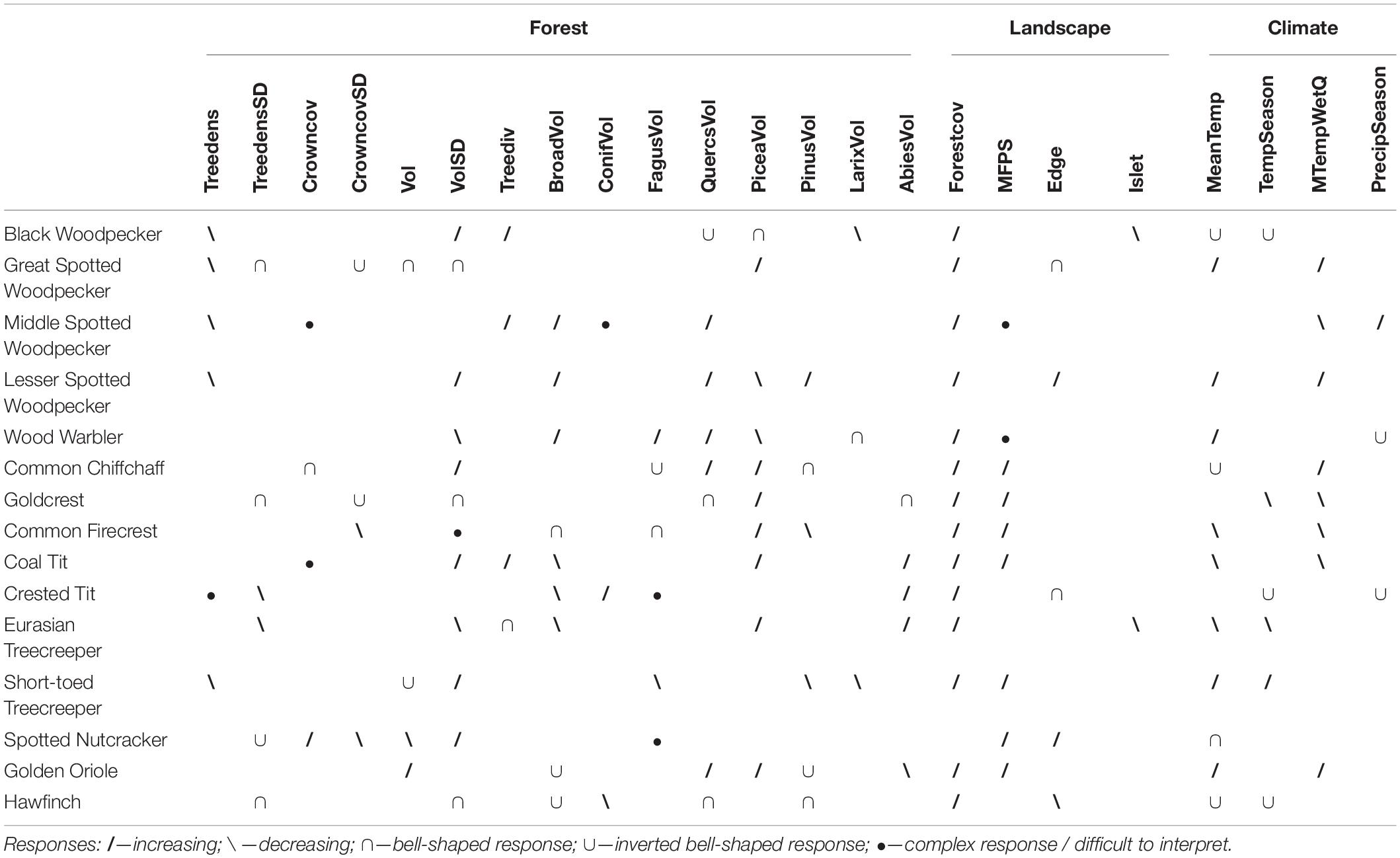
Table 4. Predictors used in each of the species’ niche models, and type of response of each species to each predictor.
All models were checked for ecological plausibility, and evaluated using a 10-fold cross-validation procedure, optimizing AUC. The BRT models were used to interpret the species responses to the environment, and all models with an AUC value ≥ 0.7 were used to project the species occurrences onto the future forest management scenarios. Both the BRTs and GLMs are described according to the ODMAP protocol for reporting species distribution models (Zurell et al., 2020; see Supplementary Information 1).
Results
Niche Models and Scenario Projections
The BRT models achieved cross-validated AUC values between 0.706 and 0.914. Forest structural parameters were generally highly influential, contributing the most for eight species models, and the second most for the remaining models (Table 5). The GLM model performances ranged from AUC values of 0.622–0.918, while seven of the 15 models did not achieve a sufficient AUC value (0.7) to be further used for prediction or inference.
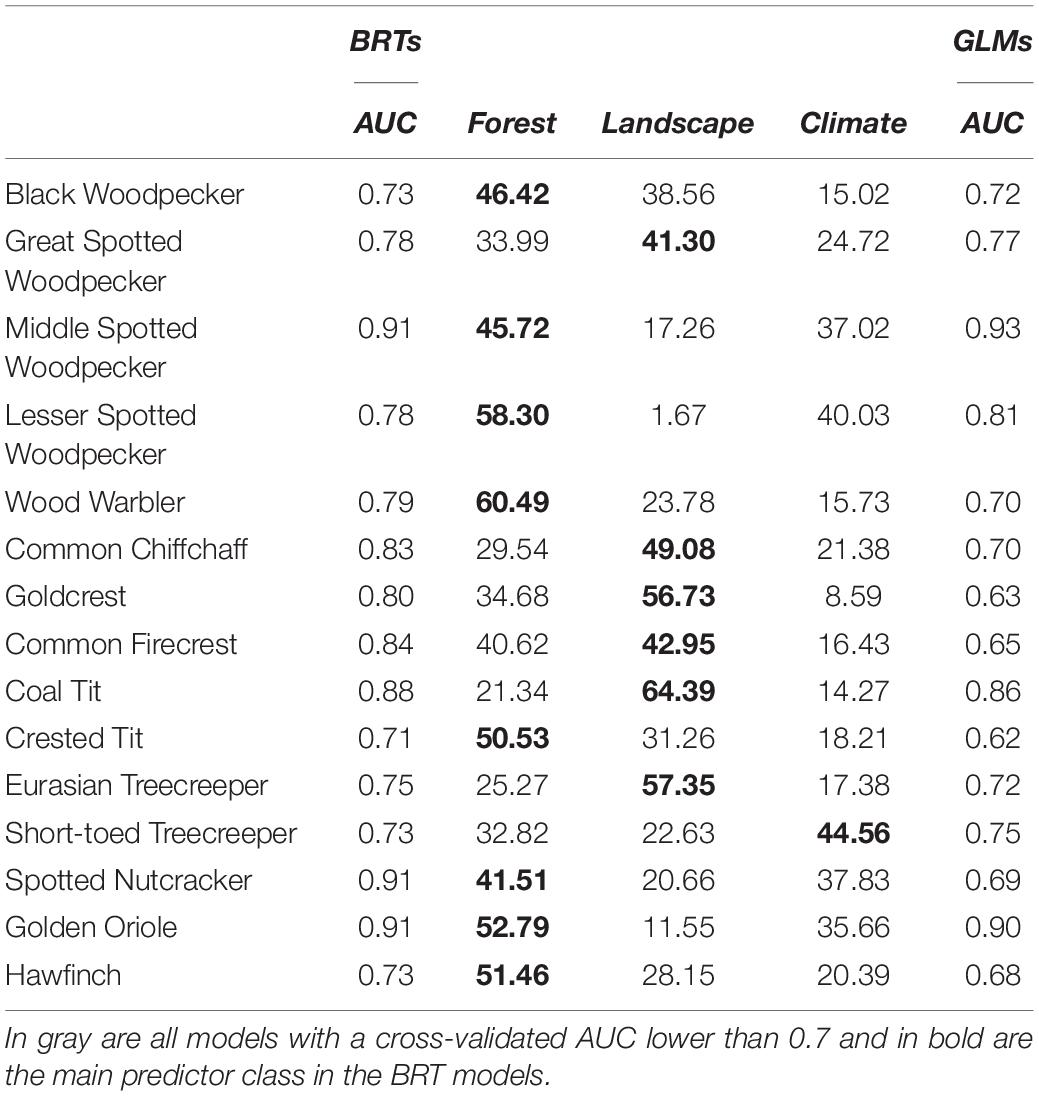
Table 5. Summary table of the BRT model performances, respective contribution of forest, landscape and climate predictors, and GLM model performances.
Within the forest structural variables, the most commonly selected variable (in the BRT models) was the variation (standard deviation) in stand volume (selected in N = 12 models), followed by (mean) volumes of spruce (N = 10 models), broadleaved species (N = 9 models) and oak (N = 8 models). Several bird species showed clear responses to forest structural parameters, including the Middle Spotted Woodpecker and the Golden Oriole (associated with areas with high oak volumes), the Lesser Spotted Woodpecker (high broadleaved and oak volumes), the Wood Warbler (high oak and larch volumes), the Common Firecrest (high spruce volumes), the Crested Tit (low broadleaved volumes), the Spotted Nutcracker (selecting areas with high crown cover) and the Hawfinch (associated with areas with intermediate variation in tree density). We note that in the seven GLM models with acceptable results (AUC higher or equal to 0.7), the species responses identified were qualitatively similar to those of the BRT models—see Supplementary Informations 2, 3 for further details on BRT variable importance and the individual species responses to the environmental variables (partial dependency plots and variable contributions).
The distinct trajectories of forest structural changes among the scenarios are visualized via the development of the standing volume (Figure 2, left) and the tree species diversity (Shannon index; Figure 2, right). The largest final standing volume was achieved by the Set-aside scenario (∼770 m3/ha), followed by Continuous cover (∼600 m3/ha). Multifunctional and Habitat tree followed similar trends, with a small reduction after the first simulation period and a constant increase afterward. The Wood production scenario showed the highest cyclic fluctuations due to the dominance of rotation forestry. In contrast, final tree species diversity was highest in the Set-aside scenario, closely followed by Continuous cover. Both progressively recovered from a drastic decrease after the first simulation periods. Multifunctional and Habitat followed again similar trends, showing the most stable trajectories. The largest changes were observed in the Wood production scenario, showing the trends of rotation forestry, with drastic decreases of diversity after each final harvest.
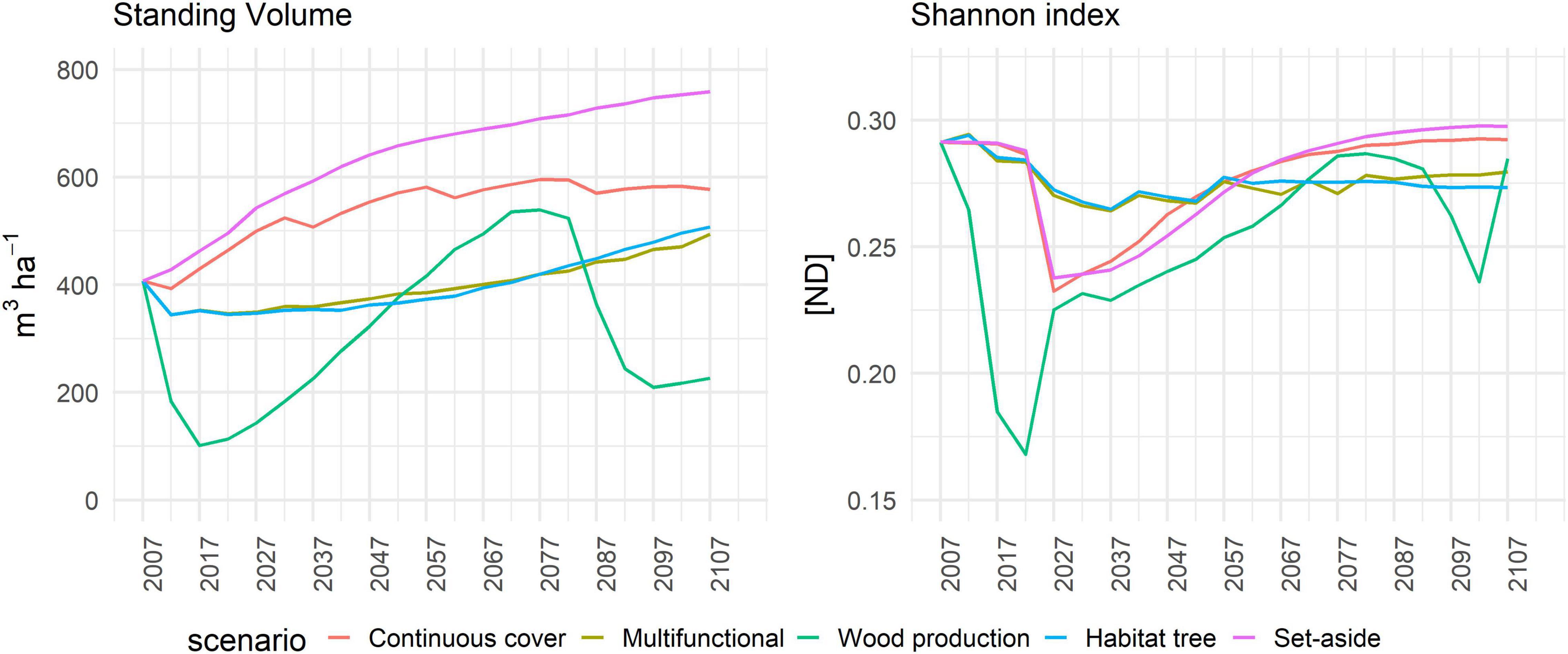
Figure 2. Development of the standing volume (left) and the mean Shannon index for each scenario over time.
Bird species showed variable responses to the different management scenarios (Figure 3). Coal Tit, Common Chiffchaff and Goldcrest had almost no change in their mean probabilities of occurrence across the study area and the time period of analysis. In contrast, Black Woodpecker, Great Spotted Woodpecker, Lesser Spotted Woodpecker, Common Firecrest, and Short-toed Treecreeper showed a consistent decrease in their occurrence probabilities in relation to the starting point. The occurrence of Golden Oriole seemed to benefit from short-term management actions (first 25-year time period) of most scenarios (apart from the Wood production scenario), although their mean occurrence probabilities in all scenarios decreased in the intermediate and long-term (50–100 years). Middle Spotted Woodpecker, Crested Tit, Eurasian Treecreeper, and Hawfinch had variable responses depending on the management regimes. While Middle Spotted Woodpecker benefited particularly from the scenarios that promoted the establishment of large oak trees within the forest (i.e., Habitat tree and Multifunctional), Crested Tit and the Eurasian Treecreeper rather benefited from the Wood production scenario. Hawfinch, while also benefitting from the two scenarios that promote oak trees, had a fluctuating response to the Wood production management scenario. Wood Warbler and Spotted Nutcracker generally benefited from all analyzed forest management scenarios, although to a different degree. While the vulnerable Wood Warbler greatly benefitted from the oak tree promoting scenarios, the Spotted Nutcracker benefitted from the Continuous cover and the Set-aside scenarios.
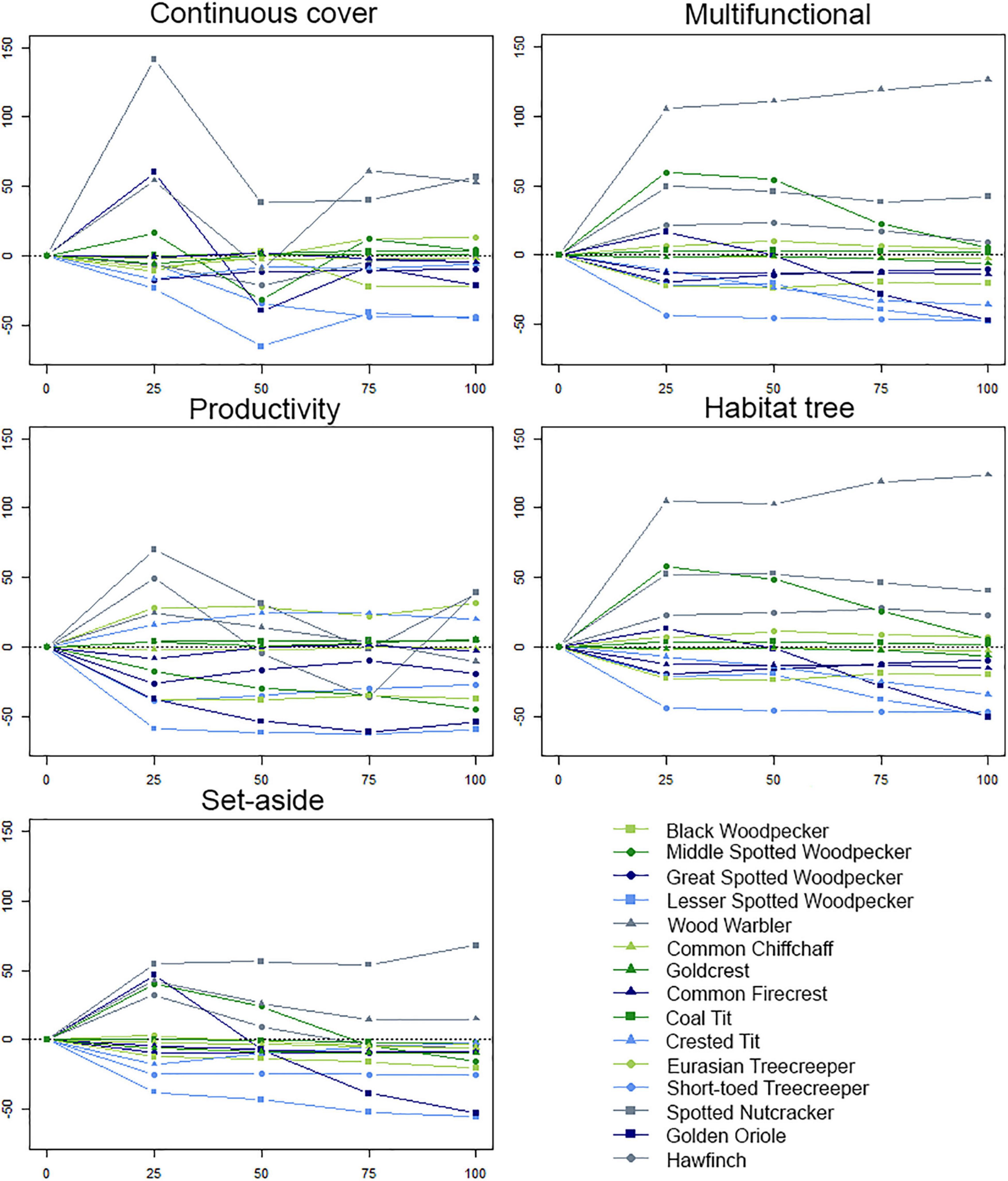
Figure 3. Change in mean predicted probability of occurrence (in percentage) of our studied species across the entire study area, for the five forest management scenarios considered, 100 year into the future.
Discussion
Our analyses showed that forest structure (reflecting stand-level forest management) is generally more important for the occurrence of forest birds than landscape structural variables (e.g., forest cover), corroborating findings from previous studies (e.g., Villard et al., 1999; Smith et al., 2008). Species with the strongest responses to landscape variables were mostly those that were very common in the study region (prevalence above 0.7) and responded primarily to the percentage cover of forest. Additionally, also the Eurasian Tree-creeper responded mostly to forest cover, corroborating previous findings (Suorsa et al., 2005). The Short-toed Tree-creeper, mostly responded to climatic variables, by selecting areas with higher annual mean temperatures. As our study is restricted to a relatively small region, this preference is more likely to reflect the regional altitude gradient and differences in forest management. In higher altitudes, forest management is dominated by selection cutting-CCF, which results in high standing volumes, presence of big trees and diverse vertical structures.
Species with different ecological niches respond differently to forest management, confirming the findings of other studies (Bengtsson et al., 2000). This has direct implications for biodiversity management and conservation (Lelli et al., 2019). Indeed, depending on the conservation objective, one needs to impose different forest management to meet the ecological needs of individual bird species (e.g., Bouvet et al., 2016; Kellner et al., 2016). The most relevant observed species responses to the considered management scenarios are described below.
Strong fluctuations in the mean occurrence of Spotted Nutcracker and Hawfinch in the Continuous cover and Wood production scenarios may reflect strong structural variation. Under rotation forestry management regimes, standing volumes accumulate, and are drastically reduced after harvest. The Wood production scenario promotes conifer trees (mostly Norway Spruce), and a high average proportion of small trees in the stand during longer periods. This pattern reflects that plantation occurs after final felling, with subsequent thinnings from above, which promotes the future crop trees (not affecting the dominant individuals). This kind of forest structure promotes the occurrence of Crested Tit and European Treecreeper. The opposite response was observed in species whose occurrences tend to decrease for all scenarios (e.g., Black and Lesser Spotted Woodpecker, Short-toed Tree-creeper). Due to the high standing volumes in the mountainous part of the study area, mainly due to spruce monocultures planted simultaneously during the 1950s, any management that is applied simultaneously on the entire landscape will result in the decrease in standing volume and increase in vertical structures. Such development leads to overall decreases in forest bird occurrences. For this reason, the Continuous cover and the Set-aside scenarios are those which generally promote the occurrence of forest birds, by maintaining or increasing the standing volume, also generally promote the occurrence of forest birds.
The Habitat tree scenario, while derived from the Multifunctional scenario, was specifically designed to retain large trees, specifically promoting oaks. Indeed, this management difference resulted in an increased occurrence of Hawfinch in the former scenario, when compared to the latter. However, the highest occurrence probabilities of Hawfinch were observed for the year 100 of the Wood production scenario. This pattern is most likely a direct consequence of the high variability in tree densities across the landscape that result from dominance of rotation forestry.
A clear effect of differing management strategies was reflected by the occurrence of Middle Spotted Woodpecker and Golden Oriole. For these two species, a high proportion of broadleaves, and especially the proportion of oak had a positive impact on their occurrences. In the case of Golden Oriole, the current high levels of standing volume seemed to be ideal, so any reduction of this volume does cause a decrease on their occurrence in the landscape. Thus, Golden Oriole mostly benefitted from the Set-aside scenario, followed closely by the Continuous cover scenario. The Middle Spotted Woodpecker benefitted from a high proportion of broadleaves, structures and especially oak, and thus from the Multifunctional and the Habitat tree scenarios.
Overall, Multifunctional and Habitat tree scenarios supported the occurrence of forest birds via highly structured forests and low fluctuations in tree volume and diversity (Figure 2) corroborating the findings of previous work (Bütler et al., 2013). Particularly, Wood Warblers, with a “vulnerable” conservation status (Knaus et al., 2021), positively responded to both of these forest management scenarios. Indeed, this species has been shown to respond to a high share of broadleaved trees and a shifting mosaic of stand ages and structures, which these two scenarios create (Mallord et al., 2012; Huber et al., 2017). Thus, these silvicultural regimes can both provide a steady supply of timber products and non-woody ecosystem services, and also help in the conservation of vulnerable bird species. Nevertheless, more intense productive regimes, that are characterized by lower standing volumes and trees of smaller dimensions, can favor some species, e.g., the Spotted Nutcracker. A combination of management regimes at the landscape level, that either provide many ecosystem services or are oriented primarily to production or conservation, may improve the overall provision of ecosystem services (balancing their trade-offs), while also promoting the conservation of a high number of species.
While the GLMs mostly corroborated the BRT findings, there were a few differences in the observed responses, directly derived from the respective model architectures. Indeed, one notable difference between the two modeling approaches is the way they deal with scarce values (with few observations) close to the end of the data ranges (outliers). While BRT tend to extend the (unaltered) adjacent predicted value into these data regions, GLMs are affected by these outliers, potentially compromising the predictions. This effect is clearly seen in the case of the Lesser Spotted Woodpecker, where the model estimates an extreme increasing response of the species to high oak volumes, which is more likely resulting from the model’s fit on data dense regions (low oak volume values) than from the species ecology. This can limit the use of these models for extrapolating, e.g., into future scenarios.
Finally, climate change could also have an effect on both forest growth (and hence the relation between management and structure) and the bird species. Our methodological approach did not encompass climate change, and thus, to implement this in future assessments would be beneficial.
Conclusion
Different forest management practices have a direct impact on forest structure, thereby having varying effects on forest bird species. The bird species’ responses to management vary as they depend on their ecological niches. Thus, no single management practice can benefit all species.
The conservation or promotion of a particular species (or species group), requires therefore a fit-to-purpose management strategy considering the species’ ecological requirements. To conserve a diverse forest bird community, it is necessary to manage forests in a way to promote diversity in habitat structure, such as by mimicking natural forest disturbances and dynamics, and promoting development of trees that could serve as habitat.
In particular, in subalpine forests with high standing volumes, any management that is applied simultaneously on the entire landscape will tend to decrease the standing volume and increase vertical structures, resulting in a general decline on forest bird occurrences. Thus it is necessary to spatially optimize the management practices in the landscape to promote the overall forest bird species diversity. This approach may allow creating the necessary diversity of habitats through time for different bird species.
Data Availability Statement
Publicly available datasets were analyzed in this study. This data can be found here: The bird and forest datasets are available through the respective data owners (see article for details).
Author Contributions
PL and BS contributed to the concept and design of the study. PH and MG aggregated the bird data. PL, AD, and LG conducted the analyses on the bird data and the species distribution modeling. AT simulated the forest data and contributed in the interpretation of the results. PL drafted the manuscript. All authors participated in revising and editing the manuscript, and approved it for publication.
Funding
The project GREENFUTUREFOREST was funded by the 2015–2016 BiodivERsA COFUND call for research proposals, with the national funders (i) German Federal Ministry of Education and Research (BMBF), grants 01LC1610A (TU Braunschweig) and 01LC1610B (TU München); (ii) the Swiss National Research council ERA-Net BiodivERsA 31BD30_172465 (University of Zurich); (iii) the Research Council of Norway grant 268624 (NINA Oslo); and (iv) The Swedish Research Council Formas grant 2016-1949 (SLU). We acknowledge support by the Open Access Publication Funds of Technische Universität Braunschweig.
Conflict of Interest
The authors declare that the research was conducted in the absence of any commercial or financial relationships that could be construed as a potential conflict of interest.
Publisher’s Note
All claims expressed in this article are solely those of the authors and do not necessarily represent those of their affiliated organizations, or those of the publisher, the editors and the reviewers. Any product that may be evaluated in this article, or claim that may be made by its manufacturer, is not guaranteed or endorsed by the publisher.
Acknowledgments
We would like to thank Denise Luethy, Christa Schmid, and Charles Henry (Amt für Landschaft und Natur, Canton of Zurich) to provide us with forest GIS data, Martin Weggler and Bird Life Zurich for providing us with the bird inventory data. We would like to thank Christian Ginzler and David Ian Forrester (Swiss Federal Institute for Forest, Snow and Landscape Research—WSL), for providing the necessary data for the calibration of SILVA in the Canton of Zurich.
Supplementary Material
The Supplementary Material for this article can be found online at: https://www.frontiersin.org/articles/10.3389/ffgc.2022.786556/full#supplementary-material
References
Aerts, R., and Honnay, O. (2011). Forest restoration, biodiversity and ecosystem functioning. BMC Ecol. 11:29. doi: 10.1186/1472-6785-11-29
Aikens, K. R., Timms, L. L., and Buddle, C. M. (2013). Vertical heterogeneity in predation pressure in a temperate forest canopy. PeerJ 1:e138. doi: 10.7717/peerj.138
Akaike, H. (1974). A new look at the statistical model identification. IEEE Trans. Automat. Control 19, 716–723. doi: 10.1109/tac.1974.1100705
Amt für Landschaft und Natur (2010). Luftbild-Bestandeskarte. Available online at: http://www.wald.kanton.zh.ch (accessed January, 2018).
Amt für Landschaft und Natur (2020). Züricher Wald. Zahlen und Fakten. [Online]. Available online at: https://aln.zh.ch/internet/baudirektion/aln/de/wald/zuercher_wald/zahlen_fakten.html (accessed March 30, 2020).
Augustynczik, A. L. D., Yousefpour, R., Rodriguez, L. C. E., and Hanewinkel, M. (2018). Conservation costs of retention forestry and optimal habitat network selection in Southwestern Germany. Ecol. Econ. 148, 92–102. doi: 10.1016/j.ecolecon.2018.02.013
Barton, K. (2020). MuMIn: Multi-Model Inference. R Package Version 1.43.17. Available online at: https://CRAN.R-project.org/package=MuMIn (accessed May, 2020).
Basile, M., Mikusiński, G., and Storch, I. (2019). Bird guilds show different responses to tree retention levels: a meta-analysis. Glob. Ecol. Conserv. 18:e00615. doi: 10.1016/j.gecco.2019.e00615
Batáry, P., Fronczek, S., Normann, C., Scherber, C., and Tscharntke, T. (2014). How do edge effect and tree species diversity change bird diversity and avian nest survival in Germany’s largest deciduous forest? For. Ecol. Manag. 319, 44–50. doi: 10.1016/j.foreco.2014.02.004
Bengtsson, J., Nilsson, S. G., Franc, A., and Menozzi, P. (2000). Biodiversity, disturbances, ecosystem function and management of European forests. For. Ecol. Manag. 132, 39–50. doi: 10.1016/s0378-1127(00)00378-9
Bianchi, S., Huuskonen, S., Siipilehto, J., and Hynynen, J. (2020). Differences in tree growth of Norway spruce under rotation forestry and continuous cover forestry. For. Ecol. Manag. 458:117689. doi: 10.1016/j.foreco.2019.117689
Bouvet, A., Paillet, Y., Archaux, F., Tillon, L., Denis, P., Gilg, O., et al. (2016). Effects of forest structure, management and landscape on bird and bat communities. Environ. Conserv. 43, 148–160. doi: 10.1017/s0376892915000363
Brändli, U. (1996). Die häufigsten Waldbäume der Schweiz. Ergebnisse aus dem Landesforstinventar 1983–85: Verbreitung, Standort und Häufigkeit von 30 Baumarten. Birmensdorf, Eidgenössische Forschungsanstalt für Wald, Schnee und Landschaft WSL. Bern: Bundesamt für Umwelt.
Brändli, U. E. (2010). Schweizerisches Landesforstinventar. Ergebnisse der dritten Erhebung 2004–2006. Birmensdorf, Eidgenössische Forschungsanstalt für Wald, Schnee und Landschaft WSL. Bern: Bundesamt für Umwelt.
Brassel, P., and Lischke, H. E. (2001). Swiss National Forest Inventory: Methods and Models of the Second Assessment.. Birmensdorf, Eidgenössische Forschungsanstalt für Wald, Schnee und Landschaft WSL. Bern: Bundesamt für Umwelt.
Bücking, M., Moshammer, R., and Roeder, A. (2007). Wertholzproduktion bei der Fichte mittels kronenspannungsarm gewachsener Z-Bäume. Mitteilungen aus der Forschungsanstalt für Waldökologie und Forstwirtschaft Rheinland-Pfalz, (62/07). Mainz: Ministerium für Umwelt, Forsten und Verbraucherschutz.
Burnham, K. P., and Anderson, D. R. (2004). Multimodel inference: understanding AIC and BIC in model selection. Sociol. Methods Res. 33, 261–304. doi: 10.1177/0049124104268644
Bütler, R., Lachat, T., Larrieu, L., and Paillet, Y. (2013). “Habitat trees: key elements for forest biodiversity,” in Integrative Approaches as an Opportunity for the Conservation of Forest Biodiversity, eds D. Kraus and F. Krumm (Freiburg: European Forest Institute), 84–91.
Czeszczewik, D., Zub, K., Stanski, T., Sahel, M., Kapusta, A., and Walankiewicz, W. (2015). Effects of forest management on bird assemblages in the Bialowieza Forest, Poland. iForest Biogeosci. For. 8, 377–385. doi: 10.1016/j.scitotenv.2020.142084
Dormann, C. F., Elith, J., Bacher, S., Buchmann, C., Carl, G., Carré, G., et al. (2013). Collinearity: a review of methods to deal with it and a simulation study evaluating their performance. Ecography 36, 27–46. doi: 10.1111/j.1600-0587.2012.07348.x
Duffy, J. E. (2009). Why biodiversity is important to the functioning of real-world ecosystems. Front. Ecol. Environ. 7:437–444. doi: 10.1890/070195
Elith, J., Graham, C. H., Anderson, R. P., Dudík, M., Ferrier, S., Guisan, A., et al. (2006). Novel methods improve prediction of species’ distributions from occurrence data. Ecography 29, 129–151.
Elith, J., Leathwick, J. R., and Hastie, T. (2008). A working guide to boosted regression trees. J. Anim. Ecol. 77, 802–813. doi: 10.1111/j.1365-2656.2008.01390.x
Friedman, J. H. (2002). Stochastic gradient boosting. Comput. Stat. Data Anal. 38, 367–378. doi: 10.1016/s0167-9473(01)00065-2
Friedman, J., Hastie, T., and Tibshirani, R. (2000). Additive logistic regression: a statistical view of boosting. Ann. Stat. 28, 337–407.
Gil-Tena, A., Saura, S., and Brotons, L. (2007). Effects of forest composition and structure on bird species richness in a Mediterranean context: implications for forest ecosystem management. For. Ecol. Manag. 242, 470–476. doi: 10.1016/j.foreco.2007.01.080
Heidrich, L., Bae, S., Levick, S., Seibold, S., Weisser, W., Krzystek, P., et al. (2020). Heterogeneity–diversity relationships differ between and within trophic levels in temperate forests. Nat. Ecol. Evol. 4, 1204–1212. doi: 10.1038/s41559-020-1245-z
Huber, N., Kéry, M., and Pasinelli, G. (2017). Occupancy dynamics of the Wood Warbler Phylloscopus sibilatrix assessed with habitat and remote sensing data. IBIS 159, 623–637. doi: 10.1111/ibi.12472
Isaac-Renton, M. G., Roberts, D. R., Hamann, A., and Spiecker, H. (2014). Douglas-fir plantations in Europe: a retrospective test of assisted migration to address climate change. Glob. Change Biol. 20, 2607–2617. doi: 10.1111/gcb.12604
Kahn, M. (1994). Modellierung der Höhenentwicklung ausgewählter Baumarten in Abhängigkeit vom Standort. Dissertation. München: Forstwissenschaftliche Fakultet der Universität der Universität München und Bayerische forstliche Versuchs-und Forschungsanstalt.
Karger, D. N., Conrad, O., Böhner, J., Kawohl, T., Kreft, H., Soria-Auza, R. W., et al. (2017). Climatologies at high resolution for the earth’s land surface areas. Sci. Data 4:170122.
Keller, V., Gerber, A., Schmid, H., Volet, B., and Zbinden, N. (2010). in Rote Liste Brutvögel.Gefährdete Arten der Schweiz, Stand 2010, ed. B. U. S. Vogelwarte, Sempach: Bundesamt für Umwelt.
Kellner, K. F., Ruhl, P. J., Dunning, J. B., Riegel, J. K., and Swihart, R. K. (2016). Multi-scale responses of breeding birds to experimental forest management in Indiana, USA. For. Ecol. Manag. 382, 64–75. doi: 10.1016/j.foreco.2016.10.008
Knaus, P., Antoniazza, S., Keller, V., Sattler, T., Schmid, H., and Strebel, N. (2021). Rote Liste 2021 der Brutvögel: Grundlagen, Hintergründe der Einstufungen und Dokumentation der Arten. Sempach: Schweizerische Vogelwarte.
Leaver, J., Mulvaney, J., Ehlers Smith, D. A., Ehlers Smith, Y. C., and Cherry, M. I. (2019). Response of bird functional diversity to forest product harvesting in the Eastern Cape, South Africa. For. Ecol. Manag. 445, 82–95. doi: 10.1016/j.foreco.2019.04.054
Lelli, C., Bruun, H. H., Chiarucci, A., Donati, D., Frascaroli, F., Fritz, Ö., et al. (2019). Biodiversity response to forest structure and management: comparing species richness, conservation relevant species and functional diversity as metrics in forest conservation. For. Ecol. Manag. 432, 707–717. doi: 10.1016/j.foreco.2018.09.057
Leuschner, C., and Ellenberg, H. (2017). Ecology of Central European Forests: Vegetation Ecology of Central Europe, Vol. I. Berlin: Springer.
Lindenmayer, D. B., Franklin, J. F., and Fischer, J. (2006). General management principles and a checklist of strategies to guide forest biodiversity conservation. Biol. Conserv. 131, 433–445. doi: 10.1016/j.biocon.2006.02.019
Mallord, J. W., Charman, E. C., Cristinacce, A., and Orsman, C. J. (2012). Habitat associations of Wood Warblers Phylloscopus sibilatrix breeding in Welsh oakwoods. Bird Study 59, 403–415. doi: 10.1080/00063657.2012.727780
Mori, A. S., Lertzman, K. P., and Gustafsson, L. (2017). Biodiversity and ecosystem services in forest ecosystems: a research agenda for applied forest ecology. J. Appl. Ecol. 54, 12–27. doi: 10.1111/1365-2664.12669
Pirovano, A. R., and Zecca, G. (2014). Black Woodpecker Dryocopus martius habitat selection in the Italian Alps: implications for conservation in Natura 2000 network. Bird Conserv. Int. 24, 299–315. doi: 10.1017/s0959270913000439
Poschenrieder, W., Biber, P., and Pretzsch, H. (2018). An inventory-based regeneration biomass model to initialize landscape scale simulation scenarios. Forests 9:212. doi: 10.3390/f9040212
Pretzsch, H., Biber, P., and Ďurský, J. (2002). The single tree-based stand simulator SILVA: construction, application and evaluation. For. Ecol. Manag. 162, 3–21. doi: 10.1016/s0378-1127(02)00047-6
Pretzsch, H., Grote, R., Reineking, B., Rötzer, T., and Seifert, S. (2008). Models for forest ecosystem management: a European perspective. Ann. Bot. 101, 1065–1087. doi: 10.1093/aob/mcm246
Price, K., Daust, K., Lilles, E., and Roberts, A.-M. (2020). Long-term response of forest bird communities to retention forestry in northern temperate coniferous forests. For. Ecol. Manag. 462:117982. doi: 10.1016/j.foreco.2020.117982
R Development Core Team (2020). R: A Language and Environment for Statistical Computing. 3.6.2 ed. Vienna: R Foundation for Statistical Computing.
Reise, J., Kukulka, F., Flade, M., and Winter, S. (2019). Characterising the richness and diversity of forest bird species using National Forest Inventory data in Germany. For. Ecol. Manag. 432, 799–811. doi: 10.1016/j.foreco.2018.10.012
Sabatini, F. M., Bluhm, H., Kun, Z., Aksenov, D., Atauri, J. A., Buchwald, E., et al. (2021). European primary forest database v2.0. Sci. Data 8:220.
Schindler, S., Sebesvari, Z., Damm, C., Euller, K., Mauerhofer, V., Schneidergruber, A., et al. (2014). Multifunctionality of floodplain landscapes: relating management options to ecosystem services. Landscape Ecol. 29, 229–244. doi: 10.1007/s10980-014-9989-y
Schwaiger, F., Poschenrieder, W., Biber, P., and Pretzsch, H. (2019). Ecosystem service trade-offs for adaptive forest management. Ecosyst. Serv. 39:100993. doi: 10.1016/j.ecoser.2019.100993
Smith, K. M., Keeton, W. S., Donovan, T. M., and Mitchell, B. (2008). Stand-level forest structure and avian habitat: scale dependencies in predicting occurrence in a heterogeneous forest. For. Sci. 54, 36–46. doi: 10.1093/forestscience/54.1.36
Soille, P., and Vogt, P. (2009). Morphological segmentation of binary patterns. Pattern Recogn. Lett. 30, 456–459. doi: 10.1016/j.patrec.2008.10.015
Suorsa, P., Huhta, E., Jäntti, A., Nikula, A., Helle, H., Kuitunen, M., et al. (2005). Thresholds in selection of breeding habitat by the Eurasian treecreeper (Certhia familiaris). Biol. Conserv. 121, 443–452. doi: 10.1016/j.biocon.2004.05.014
Swets, J. A. (1988). Measuring the accuracy of diagnostic systems. Science 240, 1285–1293. doi: 10.1126/science.3287615
Toraño Caicoya, A., Biber, P., Poschenrieder, W., Schwaiger, F., and Pretzsch, H. (2018). Forestry projections for species diversity-oriented management: an example from Central Europe. Ecol. Process. 7:23.
Venier, L. A., and Pearce, J. L. (2004). Birds as indicators of sustainable forest management. For. Chronicle 80, 61–66. doi: 10.5558/tfc80061-1
Villard, M.-A., Trzcinski, M. K., and Merriam, G. (1999). Fragmentation effects on forest birds: relative influence of woodland cover and configuration on landscape occupancy. Conserv. Biol. 13, 774–783. doi: 10.1046/j.1523-1739.1999.98059.x
Vogt, P., and Riitters, K. (2017). GuidosToolbox: universal digital image object analysis. Eur. J. Remote Sens. 50, 352–361. doi: 10.1080/22797254.2017.1330650
Weggler, M., Baumberger, C., Widmer, M., Schwarzenbach, Y., and Bänziger, R. (2009). Zürcher Brutvogelaltas 2008 – Aktuelle Brutvogelbestände im Kanton Zürich 2008 und Veränderungen seit 1988. Zurich: ZVS/BirdLife Zürich.
Zeller, L., Caicoya, A. T., and Pretzsch, H. (2021). Analyzing the effect of silvicultural management on the trade-off between stand structural heterogeneity and productivity over time. Eur. J. For. Res. 140, 615–634. doi: 10.1007/s10342-020-01350-z
Keywords: forestry, sustainable management, forest birds, niche modeling, scenario projections
Citation: Leitão PJ, Toraño Caicoya A, Dahlkamp A, Guderjan L, Griesser M, Haverkamp PJ, Nordén J, Snäll T and Schröder B (2022) Impacts of Forest Management on Forest Bird Occurrence Patterns—A Case Study in Central Europe. Front. For. Glob. Change 5:786556. doi: 10.3389/ffgc.2022.786556
Received: 30 September 2021; Accepted: 25 February 2022;
Published: 22 March 2022.
Edited by:
Stefan Zerbe, Free University of Bozen-Bolzano, ItalyReviewed by:
Gintautas Mozgeris, Vytautas Magnus University, LithuaniaChristian Temperli, Swiss Federal Institute for Forest, Snow and Landscape Research (WSL), Switzerland
Copyright © 2022 Leitão, Toraño Caicoya, Dahlkamp, Guderjan, Griesser, Haverkamp, Nordén, Snäll and Schröder. This is an open-access article distributed under the terms of the Creative Commons Attribution License (CC BY). The use, distribution or reproduction in other forums is permitted, provided the original author(s) and the copyright owner(s) are credited and that the original publication in this journal is cited, in accordance with accepted academic practice. No use, distribution or reproduction is permitted which does not comply with these terms.
*Correspondence: Pedro J. Leitão, cGVkcm8ubGVpdGFvQHVuaS1sZWlwemlnLmRl