- 1School of Applied Sciences, Edinburgh Napier University, Edinburgh, United Kingdom
- 2Adam Smith Business School, University of Glasgow, Glasgow, United Kingdom
- 3Department of Oceanography and Hydrography, Kenya Marine and Fisheries Research Institute, Mombasa, Kenya
The functional and ecological importance of dead wood in terrestrial forests is widely recognized and researched. In contrast, much less is known about dead wood in mangrove forests, despite its known or demonstrated contribution to key ecological processes including nutrient cycling and seedling recruitment. In addition, mangrove dead wood provides an important service for millions of people; harvesting wood for fuel is widespread in mangroves and is often vital for the lives and wellbeing of people living close to these forests. Limited information on stocks and production, and the drivers of these, means that understanding and managing the supply of this service is difficult. Here we conduct a systematic review of the literature on dead wood stocks and production in mangrove ecosystems. Four hundred and seventy-five subject articles were found, with large gaps in geography, species, and forest type. After excluding records that were not relevant to our study and those from mass mortality events, 68 studies remained. We also added new data from 9 sites in Kenya, to provide overall estimates of mean (± SD) stocks of dead wood of 16.85 ± 25.35 Mg ha−1 standing and 29.92 ± 36.72 Mg ha−1 downed. Our analysis shows that potentially, higher stocks of dead wood might be found in forests without evidence of human impact. Average mean production with 95% CI was 6.30, 3.10–11.40 Mg ha−1 yr−1. Estimates of daily wood use were applied to give likely demands on wood from mangrove dependent communities. This review reveals the paucity of research on mangrove dead wood, hence these estimates of average stocks and productivity remain very limited and thus, further work on the dynamics of dead wood in mangroves and the ecological effects of its removal is needed.
Introduction
Mangrove forests are communities of trees and shrubs found in the intertidal zone in the tropics and subtropics (Lugo and Snedaker, 1974). With a global area of 13.76 million hectares (Bunting et al., 2018), mangroves contribute about 0.3% of the world's forest cover (FAO, 2016). Mangroves provide numerous provisioning, supporting, regulating and cultural services to coastal populations and have been conservatively valued between USD33,000 and 57,000 per hectare per year (Sathirathai and Barbier, 2001). Despite their relevance, between 35 and 50% of the pre-industrial area of mangrove forest has been lost. Whilst global decline of these forests continues, the rate of loss has slowed to ~0.16% yr−1 (Friess et al., 2019; FAO, 2020) possibly as a result of increasing attention to conservation and growing recognition of the role of mangroves as natural carbon sinks.
The Food and Agriculture Organization (FAO) defines dead wood (DW) as “All non-living woody biomass not contained in the litter, either standing, lying on the ground, or in the soil” (FRA, 2015). Dead wood has received increasing attention in terrestrial forestry over the past two decades, reflecting the growing knowledge of its importance in forest ecology. Many organisms rely wholly or partly on the presence of DW (Heilmann-Clausen and Christensen, 2004; Seibold and Thorn, 2018). For example, Siitonen (2001) found that DW habitats supported 20–25% of all forest dwelling species in Finland. In addition to directly providing habitat, DW influences nutrient cycling and retention, pedogenesis and plant recruitment dynamics in forests. Monitoring systems designed to measure and promote forest biodiversity, such as those adopted by the European Environment Agency, now use DW as an indicator of ecological quality (Söderberg et al., 2014) and research is devoted to ways of increasing DW quantity and diversity in forests (e.g., Christensen et al., 2005).
DW in mangrove forests has received much less research and policy attention, despite evidence of its importance in a range of ecological processes, including those found in terrestrial forests but also some of particular or sole relevance to tidal forests. For example Romero et al. (2005) studied DW decomposition and its contribution to nutrient dynamics at a Florida site which, like many mangroves, is subject to major disturbance from tropical cyclones that can result in sudden depositions of large volumes of DW. They found that such incidences have “profound” impacts on nutrient dynamics. In particular, downed wood can be a major source of nitrogen and phosphorus in forests that are limited by these nutrients.
DW is also an important component of the carbon stocks and flows in mangrove forests. Robertson and Daniel (1989) produced one of the few estimates of both stock and production dynamics of mangrove DW. In this study, carried out in Australia, the authors reported thata mature, mixed Rhizophora spp. forest had a mean aboveground DW stock of 14.2 Mg ha−1 (9.4 Mg fallen and 4.8 Mg standing), compared to 403 Mg ha−1 dry weight living biomass. Hence DW may be a significant part of the carbon stock in many mangrove forests; note that these figures do not include dead (belowground) roots. Buried DW may be a larger carbon stock than aboveground DW in many forests; for example Tamooh et al. (2008) found 32.6 Mg ha−1 of dead roots (compared with 35.8 Mg ha−1 live roots) in a mature Kenyan Rhizophora spp forest.
These ecological functions—mediating supplies of key nutrients such as nitrogen and phosphorus, supporting a range of fauna and acting as a store of carbon—are shared with terrestrial forests. In contrast, other processes which involve mangrove DW are unique or of special importance to these aquatic habitats. For example, DW can influence stream and tidal flow patterns, changing how aquatic fauna access and use the forest and influencing the accumulation of sediment (Allen et al., 2000). Mangrove propagules are dispersed by floating in the water; the passive trapping of propagules by woody debris is important in the recruitment of new trees and in the recovery of damaged or cleared areas (McKee et al., 2007).
In addition to its ecological importance, DW is a crucial resource for many human communities living in or adjacent to mangrove forests. Biomass remains the main source of fuel for billions of people; those close to mangroves often preferentially collect mangrove wood, for convenience but also because it has high density, burns at high temperatures and can produce desirable flavors (Huxham et al., 2017). For example, at Gazi Bay in Kenya, more than 70% of households rely on wood collected from local forests, including the mangroves, and use an average of 1.2 kg per capita per day, spending an average of 22 h per month collecting this wood (Jung and Huxham, 2018). Understanding the importance of this ecosystem service requires sensitivity to the social and cultural context. The labor of fuelwood collection in Africa is performed overwhelmingly by poor women and girls. Standard economic assessments may underestimate the value of this fuelwood provision and the opportunity costs, such as time not spent studying or at school, suffered by the girls (Huxham et al., 2015). Cash income is strongly correlated with mangrove fuelwood use. In a review of case studies of mangrove communities from around the world, Huxham et al. (2017) found ~ 90% of households using mangrove wood in Vietnam, Indonesia, The Gambia and Cameroon, whilst none reported using mangrove wood for fuel in a Mexican study, where people could afford alternatives. Hence, supplies of fuelwood (which is mostly but not exclusively dead) are vital resource for some communities and irrelevant for others. So, understanding and managing supplies of mangrove DW to dependent communities requires understanding of cultural, social and gender contexts. Wood collection is a significant driver of mangrove degradation and destruction. Chowdhury et al. (2017) found that wood collection, including for fuel use, was implicated in 44% of the cases of degradation that they studied globally and in 90% of those cases from Africa. Therefore, the supply of DW from mangroves has important implications for the health and welfare of millions of people and for the conservation of mangrove forests.
At present, there is limited information on the stocks, and less on the productivity, of DW in mangroves and on the factors that drive these variables (Sitoe et al., 2014; Kauffman et al., 2016). To our knowledge there are no published attempts at estimating what might be sustainable levels of DW harvest from a mangrove forest, despite the importance of this for mangrove conservation in most countries that support mangroves. In this study we aim at filling this research gap by pursuing the following objectives: (a) conducting a systematic literature review to identify the current estimates for above-ground DW stocks and productivity in mangroves and explore the influence of potential drivers, including location, species and impacts of human use, on DW; (b) comparing literature values on stock, and different methods for estimating productivity; (c) exploring the possible implications of DW estimates for management of mangrove forests.
Methods
We used four methods to collect data on above-ground mangrove DW stocks and production: (a) a systematic review of published literature; (b) incorporation of any relevant data and papers used in the IPCC (2014), which provides default values for carbon assessments including of DW under IPCC Tier 1 assumptions, which were not already included during the initial review; (c) analysis of published forest data on mangroves in Kenya, collected by members or colleagues of the current team using consistent methodology at all sites; (d) search and analysis of published mangrove forest data on publicly accessible databases. Key aims were to estimate the average DW stocks and production in mangroves, along with the variance around these averages, to understand the influence of likely ecological and anthropogenic drivers of these variables and to identify research gaps and/or inconsistencies.
Systematic Literature Review
Search Strategy and Identification of Relevant Studies
Four search engines including the Institute for Scientific Information (ISI) “Web of Science,” CAB Abstracts, ProQuest and JSTOR were used to find potentially relevant publications, which were either written in English or included an English abstract. There was no limitation on date of publication. The initial search term was:
“TOPIC: (mangrove* AND (biomass OR carbon OR productivity OR production)) OR TOPIC: ((dead wood OR standing dead wood OR downed wood) AND (biomass OR carbon OR production OR productivity)) AND TOPIC: (mortality) AND TITLE: (mangrove* AND ((dead wood OR standing dead wood OR downed wood) OR (biomass OR carbon OR production OR productivity)))”
A total of 9,754 articles were found with this inquiry. A quick inspection showed that most of these articles were irrelevant given the versatility in use of the terms “production,” “productivity” and “mortality”; therefore, the search was refined by excluding most research areas, leaving the following core disciplines: “Environmental Sciences and Ecology, Water Resources, Fisheries, Marine Freshwater Biology, Meteorology, Atmospheric Sciences, Geography, Biodiversity Conservation, Social Sciences and Other Topics, Forestry, Energy Fuels, Plant Sciences, Oceanography, and Social Issues.”
Screening and Eligibility Criteria
The refined search resulted in 8,252 articles (Figure 1). Articles whose titles were relevant where selected and their abstracts were all independently read and categorized. Duplicates were excluded and the remaining articles were categorized into three groups: “definitely relevant,” “possibly relevant” and “definitely not relevant.” The “definitely relevant” class contained articles where the abstract clearly mentioned DW stock and/or production. The “possibly relevant” group mainly consisted of papers with mention of mangrove biomass and/or carbon, litter production and standing litter. As mangrove biomass and carbon studies, and litter production studies, usually focus on live biomass and leaf litter rather than wood litter, respectively, it was generally not possible to determine the relevance of these articles by only reading the abstract. Categorizations made by individual readers were compared to check for consistency; there was conformity in all the categorizations between readers.

Figure 1. Description of steps taken to find and refine literature along with the number of relevant publications found at each point.
A total of 283 articles were categorized as “definitely relevant” and “possibly relevant.” The reference lists of these were then scrutinized for any additional relevant studies that had not been identified during the initial literature search. A further 192 articles were found to be possibly relevant and were available online. Thus, 475 articles were read in full for relevant data and information.
Data Extraction and Quality Assessment
During the full-text reading, data on the following quantitative and qualitative variables were collected: location (country, site, and coordinates), dominant mangrove species, stock of total DW (standing and/or downed), basal area and density of standing DW, biomass of standing dead and downed wood, production of DW, mangrove tree mortality rate and wood decomposition rate. Additionally, any evidence of human impact (such as wood removal activities including fuelwood collection or shrimp production) and management status, the type of forest (planted or natural stand) and the methodologies used in the studies were recorded. Data from studies undertaken after mass mortality events such as typhoons were excluded since these events can result in sudden, very large and unrepresentative stocks of DW (Stephenson et al., 2011). Similarly, articles that only covered litter production were set aside. Eventually, 67 of the 475 articles were found to have data relevant to our Research Topic.
Data Summary and Analysis
Retrieved data were converted to common units of measurement: Mg dry mass ha−1 and Mg dry mass ha−1 year−1 in the case of DW stock and production data, respectively. Where information was given on downed wood volume these data were converted into mass (Mg ha−1) using wood density values provided by Simpson (1996). Where basal area (m2 ha−1) and stem density (stems ha−1) were given the allometric equation provided by Clough and Scott (1989) was adopted to calculate standing DW (Mg ha−1) using Equation 1.
where A and B were regression constants and varied between species and tree components, and D was the average DBH (cm) in the stand (or in case of basal area, it was DBH ha−1) (Clough and Scott, 1989). Table 1 gives some examples of A and B values used.
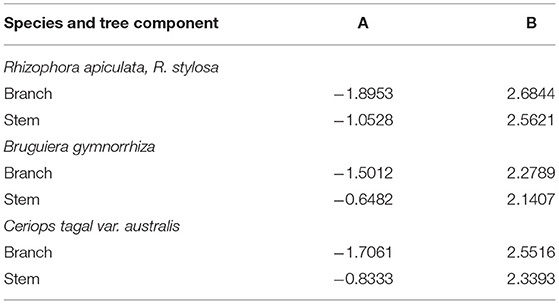
Table 1. Regression constants for calculating aboveground biomass of different mangrove species (Clough and Scott, 1989).
Tree biomass (Mg) was calculated and multiplied by the relevant stem density to get a unit area value (Mg ha−1). DBH (cm) was calculated from basal area (Equation 2) where this was given; where it was not stated, but the average DBH for trees in a study was, this was applied to standing tree stock data.
Production of mangrove DW was estimated using three approaches:
1) It was directly recorded where it was explicitly measured or calculated by the primary authors.
2) Where mortality rates of trees were given for a site these were used to infer DW production. Percentage mortality rates were used in combination with biomass values to calculate annual DW production rates (Mg ha−1 yr−1). Where biomass was not provided, it was calculated from mean DBH (cm) using equation 1 (Clough and Scott, 1989) as indicated earlier for the case of stocks.
3) In some cases, papers did not directly give productivity or mortality rates but rather presented self-thinning or mortality models. Where appropriate relevant data on DBH/stem density etc was also presented, mortality rates were derived and used to calculate productivity as described in 2.
IPCC Wetlands Supplement
The most authoritative collection of information that is currently published on mangrove DW is the IPCC (2014) which lists studies that include default values of DW can be used in Tier 1 assessments of carbon stocks in mangroves. These papers were checked for any information in addition to that discovered during the literature review. This process added one additional paper to the list of those reviewed.
Additional Field Data
Three of the authors (ML, KJ, and HM) work to support the Mikoko Pamoja and Vanga Blue Forest projects which are two mangrove conservation initiatives based in southern Kenya (ACES, 2021). The projects collect monitoring data from 25 permanent forest plots, which include information on DW. These data were used to add three additional sites to this analysis. Further, data collected from six other mangrove sites in Kenya (Mugi and Kairo, 2021; Figure 2) were also used. These were collected using the same field protocols from temporary plots on various occasions between 2015 and 2020; the forest assessment methods described by Kauffman and Donato (2012) were applied. The studies covered 74% of the mangroves in Kenya where nine species have been documented in a horizontal species zonation typical of the Western Indian Ocean (WIO) region (Bosire et al., 2015; GoK, 2017). The sites are drawn from along the whole Kenyan coast. The most northerly sites are near Lamu (Figure 2), an area which contains > 60 % of the mangrove coverage in Kenya with relatively structurally complex forest formations (GoK, 2017). Further south, the low-lying estuarine system of Tana River (Figure 2) is dominated by distinctive stands of mangroves and associated species, and dwarf Avicennia marina stands on the landward (GoK, 2017). At the southern coast of Kenya, mangroves are dominated by mixed species stands (Mungai et al., 2019), with near pristine Rhizophora dominated stands on Sii Island at the southern-most part of the country (GoK, 2017). Human-induced losses and degradation of mangroves in Kenya have been widely reported (Kirui et al., 2013; Bosire et al., 2014; GoK, 2017; Mungai et al., 2019).
To calculate biomass at these sites, the bespoke equation developed by Cohen (2014) was used:
Equation 3 estimates the biomass of standing live trees. It was also used in the estimation of dead tree biomass where it was combined with corresponding decomposition constants given by Kauffman and Donato (2012), where in standing dead trees in decay status 1 and 2, 2.5% and 15% were subtracted based on the leaf and branch biomass loss, respectively. The formula for conical volume was multiplied by species-specific wood density values obtained from Simpson (1996) to calculate the biomass of dead trees in decay status 3 (Equation 4).
where H is the tree height (in cm),
DBH is the diameter at 130 cm from the ground,
and, q is the wood density: 0.867, 0.780, 0.803, 0.741, 0.700 and 0.661 g cm−3 for R. mucronata, S. alba, C. tagal, B. gymnorrhiza, Xylocarpus granatum and A. marina, respectively (Simpson, 1996).
Swamp and GlobAllomeTree Database
SWAMP is a database containing information from mangroves and peatlands across 27 countries; most of these datasets are publicly accessible and some include data on DW (SWAMP Database Management – SWAMP, n.d). GlobAllomeTree is a web platform for sharing and providing access to tree allometric equations, including mangroves. All relevant, accessible datasets and equations were scrutinized for additional data, but no sites not already included in the other searches were found.
Statistical Analyses
Statistical analyses were conducted using Microsoft Excel and R statistical software (version 4.0.2). Summary statistics were applied to characterize data retrieved from literature and those added from our local sites. Stocks of dead wood and productivity were compared between forests with and without evidence of human impact using ANOVA or non-parametric equivalents where appropriate.
When estimating average productivity, the paucity of data and uncertainty about underlying mechanisms and statistical distributions suggested caution when using parametric statistics. Hence bootstrapping (with the “boot” function in R) was used to produce an average (with non-parametric 95% CIs).
Results
Study Characteristics
The refined literature search revealed that the first studies written on mangrove DW were published in the 1930s with a gradual increase in research on mangrove DW in the 21st century. Between 1932 and 2000, there were 254 scientific works published on mangrove dead wood, and since 2000, this number quadrupled. However, further investigation showed that most of these publications were irrelevant to the present study as they did not cover stocks or production rates of DW. The 68 articles found to be relevant to our study (67 from “the literature search” and an additional one from the IPCC wetlands supplement) were published between 1978 and 2021 (Figure 3, Appendix 1), with only 8 published in the 20th century. All subsequent discussion of published literature concerns only these 68 articles.
Relevant studies came from 38 countries, with Africa and Asia each having eleven (11), seven (7) in the Americas, three (3) in the Caribbean, five (5) in Oceania and one (1) in the Middle East (Figure 4, Supplementary Table 1). Twenty (20) of the publications reported on multiple sites while the rest were conducted at single sites, although larger forests were often stratified into different areas. As can be observed from Figure 4, the publications represented a relatively small area of the global mangrove coverage; from the 118 countries with mangroves (Giri et al., 2011), 32 % were represented.
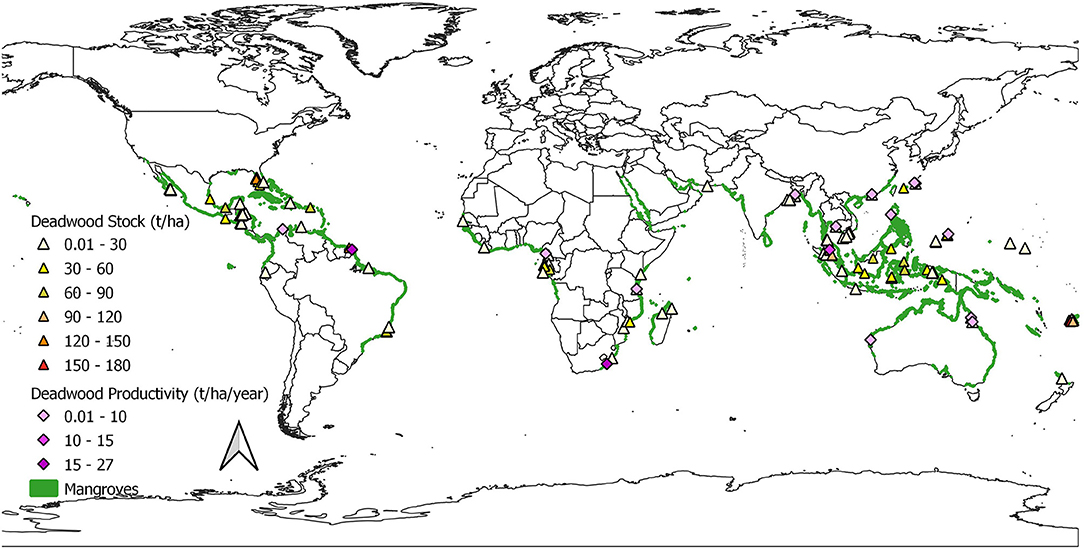
Figure 4. Study sites for dead wood stocks and production. Stock values are a combination of total, standing, and downed wood biomass.
A majority (95%) of the publications reported on primary studies undertaken in the respective sites in either temporary or permanent sample plots. The planar intersect technique (Van Wagner, 1968; Allen et al., 2000) was the most common procedure for assessing stocks of downed wood whereas forest productivity assessments reported wood litter from the litter traps technique. Cases of standing DW used tree measurement techniques described by Kauffman and Donato (2012). Only one of the publications (Steinke et al., 1995) reported use of a destructive sampling method to measure DW, which involved removal and sorting of above ground living and DW material from sampling plots. A summary of the data on DW production, standing dead and downed wood in mangrove forests is provided as Supplementary Table 1.
Mangrove DW stocks were reported as standing dead, downed, total DW, or a combination of these. Data on stocks of downed and standing wood were more frequent (34 and 25 articles, respectively) as compared to reports of total DW stock for which only 9 articles could be found from the search.
An indication of human impact and/or the management status of the forest stands was given in 44 articles (although in most cases information was scanty and qualitative, for example a comment in the site description that a forest “showed signs of cutting” or “was a protected area”). Such information was used to categorize stands as “protected” (where this was stated explicitly and there was no contradictory information, for example stating protection was not enforced), “exploited” (where this was explicitly stated in the article) or “no evidence” (where no information on levels of human impact was given). Twenty-four articles described their sites as either protected areas with prohibited deforestation or as having no evidence of mangrove wood removal/human impact. Extraction of wood for fuel and/or timber, and to support fisheries or shrimp aquaculture were the most frequent reported land uses. The least described forms of use included exploitation for medicinal purposes, oil palm plantation and salt production.
Statistical Synthesis
Stock of Dead Wood in Mangrove Forests
Standing stocks of DW were reported from sites in the Americas (7), Africa (6), Asia (4), the Caribbean (3), Oceania (3), and Middle East (1) (Supplementary Table 1). The mean (± SD) biomass from these sites was 16.85 ± 25.35 Mg ha−1 (median = 9.4, IQR: 1.98–18.27 Mg ha−1). The largest stock of standing DW was 143.2 Mg ha−1, reported in an Avicennia germinas dominated stand in French Guiana, while the lowest stocks (<0.03 Mg ha−1) were reported from mangroves with intensive human impact at Mombasa, Kenya and managed forests in Fiji (Supplementary Table 1). Relatively low values of standing DW were also reported in two stands in Australia: 0.78 Mg ha−1 in a young Rhizophora dominated forest in Missionary Bay, and 1.91 Mg ha−1 in Port Douglas.
Downed wood biomass was reported in 34 articles (76 sites); 12 of them studied Asian stands, 11 in the Americas, six in Oceania, four in Africa and one in the Caribbean (Supplementary Table 1). The mean biomass of downed wood was 29.92 ± 36.72 Mg ha−1 (median = 15.84, IQR: 7.40–34.00 Mg ha−1); the highest (between 115.00 and 179.20 Mg ha−1) were reported in Rhizophoraceae spps stands in Fiji. The lowest stock (0.26 Mg ha−1) was recorded in an A. marina dominated stand in Sofala Bay, Mozambique where there was evidence of wood extraction for fuel, charcoal and building material.
There were nine cases in which total DW was reported, and it averaged (mean ± SD) 31.76 ± 24.68 Mg ha−1 (IQR: 12.65–44.18 Mg ha−1). Six of the articles studied sites in Asia, while the others were from Africa (2) and the Americas (1). The highest stock of total mangrove DW, 85.40 Mg ha−1, was reported in Bunaken National Park in Indonesia, while the lowest, 2.40 Mg ha−1, was reported from Mexico.
Data on total aboveground mangrove DW (combining standing and downed) were collated and/or derived from values of downed wood, total and standing DW found in 57 articles (120 sites) and the additional nine sites in Kenya. The total stock of DW averaged (mean ± SD) 29.65 ± 35.32 Mg ha−1 (median = 16.40 IQR: 6.80–34.80 Mg ha−1). The heavily exploited site of Mombasa, Kenya and a forest in the USA recorded the lowest values of DW stocks, 0.03 and 0.16 Mg ha−1, respectively. The highest stocks were reported in a French Guiana stand (143.20 Mg ha−1), and in Fiji with values between 135.20 Mg ha−1 and 180.4 Mg ha−1. The lowest mean was reported from the Middle East (1.06 Mg ha−1) in contrast to the Oceania where the widest range and the highest average were reported. The stocks of DW were significantly different between the regions; (Figure 5; Kruskal–Wallis test χ2 = 20.53, df = 5, p < 0.001). Post-hoc Dunn's test with Bonferroni adjustments showed stocks of DW were significantly higher in Asia and Oceania than Africa (0.002 and 0.026, respectively). No other differences were statistically significant.
The sites reporting adverse human impacts and extraction of mangrove wood had significantly lower stocks of DW (mean 32.08 ± 33.50; median = 20.90 IQR: 6.80–40.00 Mg ha−1) as compared to those reported to be protected (mean = 41.66 ± 46.50; median = 28.95 IQR: 8.01–55.70 Mg ha−1; Figure 6; Kruskal–Wallis test χ2 = 6.22, df = 2, p = 0.045).
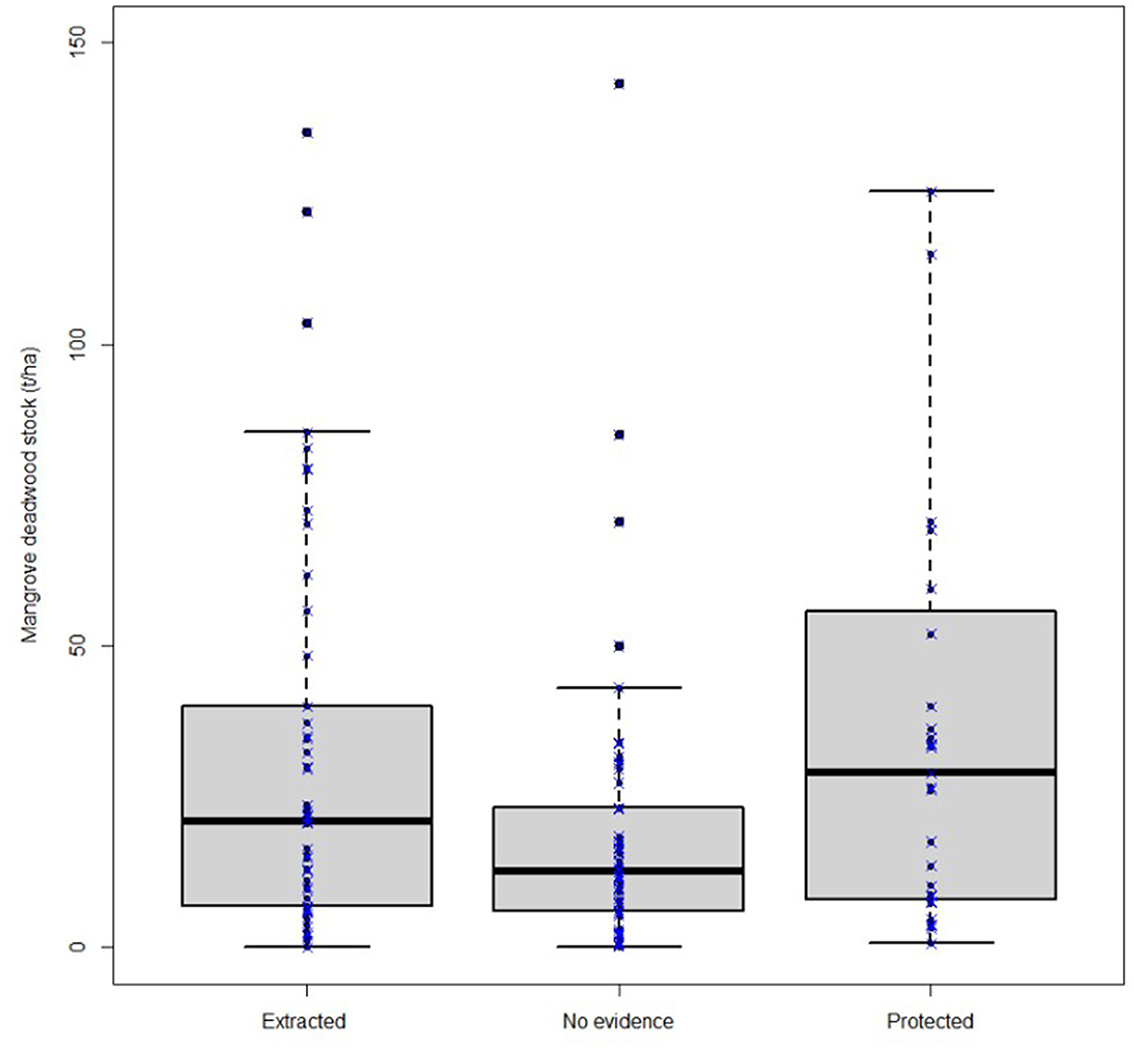
Figure 6. Mangrove dead wood stocks Mg ha−1 under different reported human impacts based on available evidence from the literature review.
Production of Dead Wood in Mangrove Forests
In our study, we found only three sites—in Australia and China—that explicitly reported the rate of production of DW in mangroves (Supplementary Table 1). In addition to these, data on mortality rates were used to estimate DW production at 12 sites and mortality/thinning models could be applied to two sites; there were no significant differences among medians from these three estimates (Kruskal–Wallis test) (Table 2). The spatial distribution of the sites was: Africa (3), Asia (7), Oceania (5), and Americas (2) (Figure 4), with the most recent of the studies in 2019 in Japan, and the oldest data reported (1986) for a site in Malaysia. Highest and lowest rates of mangrove DW production were 26.68 Mg ha−1 yr−1 for a Bruguiera species forest in Japan and 0.01 Mg ha−1 yr−1 for a young Rhizophora spp Australian stand, respectively.
Given the limited data and uncertainties over the underlying distribution, bootstrapping (10,000 iterations) was used to estimate an average and non-parametric 95% confidence intervals (using the BCa procedure) for these productivity data of 6.30 (3.10–11.40) Mg ha−1 yr−1. Assuming a per capita consumption of 1.2 Kg day−1 (Jung and Huxham, 2018) these estimates suggest a community of 1,000 people would need a forest of between 38.4 and 141.3 ha in size to provide their fuelwood needs sustainably.
Discussion
The current study estimates averages of mangrove above-ground DW stock (29.65 ± 35.32 Mg ha−1 (mean ± SD); based on information from 129 sites) and production (6. 30: 3.10–11.40 Mg ha−1 yr−1 (mean with 95% non-parametric CI); based on information from 16 sites) derived from a dataset with values from Africa, Americas, Caribbean, Oceania and Middle East. However, given the small number of relevant studies and patchy geographic coverage these estimates need to be treated as very provisional. For example regions, including West Africa and West America, are not well-represented in the literature, even though these areas constitute a considerable percentage of the total global mangrove coverage (Spalding et al., 2010). Hence a key finding of the current work is that mangrove DW is relatively under-researched and deserves further study.
Forest DW is an important part of the global carbon pool (IPCC, 2014). It accounts for around 8% (~73 Pg) of all carbon in terrestrial forests (Pan et al., 2011). It may rival or exceed other major carbon pools in individual forests. For example, the carbon found in the DW pool in boreal forests represents almost twice (178%) that carbon found in the soil pool (with 27% and 43% for temperate and tropical forests respectively) (Pan et al., 2011). The data presented here suggest that per unit area stocks of DW in mangroves are within the range (although toward the bottom end) of those found in other forests. The IPCC gives median DW stocks of 18.2, 43.4, 34.7, and 10.7 Mg ha−1 for tropical, evergreen and deciduous forests (IPCC, 2003; Table 3.2.2), and for mature mangroves (IPCC, 2014; Table 4.7), respectively; although stocks in particular forests may far exceed these. There is evidence that DW is increasing in many forest types, both in total amounts and as a proportion of total pools, as degradation and disturbance spreads. Pan et al. (2011) report a “large sink increase” of deadwood in boreal forests over the decade up to 2007, caused by increasing climate-related disturbances and further suggest increased “dead biomass production” in tropical forests. Case studies of logging and other intense anthropogenic disturbance typically show increases in DW. For example, DW increased from 55 Mg ha−1 in intact Brazilian rainforest, to 75 Mg ha−1 with reduced-impact logging, to almost 110 Mg ha−1 in a logged forest (Keller et al., 2004). In a study of carbon stocks at different times following logging in Indonesian mangroves, Murdiyarso et al. (2021) found DW stocks immediately following logging that were double those in protected forests (39.73 vs. 19.98 Mg C ha −1 respectively). Although stocks may be comparable, it is likely that above ground DW is not proportionally as important a pool in most mangroves as in most other forest types, principally because of the dominance of the soil carbon pool in mangroves, which often exceeds 90% of the total carbon present (Gress et al., 2017). Our estimates of mangrove DW do not include below ground data and hence underestimate total stocks and productivity. Including information on below ground DW may have major impacts on estimates of total stocks at some sites. For example, at Gazi Bay in Kenya, there are 32.5 Mg ha−1 of below ground dead roots in natural Rhizophora spp dominated stands (Tamooh et al., 2008), which exceeds the aboveground stocks, 0.62 Mg ha−1, reported here. However, soil carbon is likely to remain the dominant pool at most sites even if below ground DW is included.
The wide variation in the estimates of DW reported here could represent a sparse sample from a large and variable population but might also imply use of dissimilar measurement/monitoring systems in mangrove DW stock and production assessments. The protocols for estimating mangrove DW stocks described (Kauffman and Donato, 2012) are now widely used and will help to address historical differences in methods; their adoption and refinement within IPCC guidelines means that inconsistency in methods does not appear to be a major problem in comparing DW stock estimates between sites. Instead, the spread in the values from different studies is probably related to a wide range of biological, geographical and anthropogenic factors, including the age and structural characteristics of the mangrove forests. For example, Robertson and Daniel (1989) reported that DW stock for a mature stand was 8-fold that of a young stand at a similar protected site in Australia (14.89 and 1.81 Mg ha−1, respectively). Terrestrial stands have similarly been found to contain very variable amounts of DW controlled by natural forest dynamics and human impact (Harmon et al., 1986; Sandström et al., 2019). Meta-analytical examination of the drivers of this variance (between, for example, forests of different ages, species and geomorphological settings) is an obvious research goal but was not possible here given the paucity of data. In contrast to the measurement of stocks, there are no standard methodological approaches for establishing mangrove DW production rates (which is a much harder variable to estimate, but which is more relevant for consideration of fuelwood extraction and management and also contributes to our understanding of mangroves as a carbon sink). Further theoretical and empirical work on DW productivity in mangroves is highlighted by this review as an important target for future research.
One motivation for the current work, and justification for a focus on above ground DW, was to contribute toward understanding and managing human needs for dead wood from mangroves (which is principally for fuelwood). The data presented here (that excludes cases of instantaneous high mortality) show that mangrove forests exposed to human exploitation are generally lower in total and standing DW stocks than those that are relatively undisturbed. Logic, along with multiple site-specific studies looking at human impact on mangroves (for example (Huxham et al., 2017; Chow, 2018; Adanguidi et al., 2020) and relevant reviews (e.g., Chowdhury et al., 2017; Huxham et al., 2017), suggest that extraction of fuelwood will lower stocks of DW and when intense could have a range of impacts on forest ecology; if fuelwood collection includes the cutting of living biomass then it may quickly threaten the sustainability of a forest. Given the importance of this ecosystem service for the lives of millions of people, along with the implications of excessive fuelwood collection for mangrove conservation, it is surprising that so little attention has been given to it, from either scientific or management perspectives. There are examples of the sustainable management of fuelwood and charcoal extraction from mangroves. For example McNally et al. (2011) describe how management of mangroves in the Saadani National Park, Tanzania, has reduced unsustainable cutting, leading to a 5% reduction in households using mangrove fuelwood. This was combined with an overall increase in income from more productive shrimp capture fisheries. Importantly, the reduction in fuelwood use was recorded predominantly in richer households, which could afford to shift to alternative sources of fuel, so this example appears to show how mangrove conservation can lead to enhanced economic opportunities for local communities, without penalizing the poor and whilst still permitting the use of fuelwood by those who need it most. A very different model of sustainable use comes from the Matang mangrove in Malaysia, which has been producing commercial charcoal for more than a century (Goessens et al., 2014). In addition, communities around the world have used mangrove fuelwood sustainably for a long time (Bosire et al., 2015), applying a wide range of customary management practices. Hence, we can learn from these examples to help manage fuelwood at other sites; doing so will usually require much better information on levels and productivity of DW than is available at present.
Conclusion
The present study adds to knowledge on mangrove DW stocks and production and reveals the current paucity of information and research on these topics, both globally and particularly for some major regions.
The data presented has evidenced lower DW stocks in mangroves compared to terrestrial counterparts, although the values available fall within the very large range reported in other tropical forests. Research on terrestrial forests has shown the influence of latitude, age and species on DW stocks and production. The importance of such drivers in mangroves remains unknown and should be the subject of future research. More studies within and across regions are necessary to make clear patterns and to quantify the ecological roles and thresholds of DW stocks and production in mangrove forests. Whereas, simple methods for quantifying stocks are available and widely used, estimation of DW production in mangroves remains a challenge and has rarely been attempted. Lastly, the importance of mangrove fuelwood to millions of people should encourage further work on understanding production and on using this understanding to assist with sustainable management of this valuable resource.
Data Availability Statement
The original contributions presented in the study are included in the article/Supplementary Material, further inquiries can be directed to the corresponding author/s.
Author Contributions
MH, LM, and DK were involved in the conception and development of the presented review. LM and DK performed the literature search under the supervision of MH. JK, MH, and LM were involved in the conceptualization, supervision, collection, and analysis of the data from the Kenya sites. LM performed the analysis for the presented review and together with MH designed figures. DK developed the images. LM took lead in writing the manuscript in consultation with MH. All authors provided critical feedback on the manuscript.
Funding
We appreciate the financial support accorded to LM by Mr. Derek Moss during the development of this manuscript. We would also like to acknowledge the financial assistance received toward the collection of the different Kenyan forest data sets: PEW Charitable Trust (00032875), Western Indian Ocean Marine Scientists Association (WIOMSA) through Cities and Coast Program (Grant No. OP/2018/01), World Wildlife Fund for Nature (Grant No. BMZ 68776), and the World Bank through the Kenya Coastal Development Project (Grant No. P094692).
Conflict of Interest
The authors declare that the research was conducted in the absence of any commercial or financial relationships that could be construed as a potential conflict of interest.
Publisher's Note
All claims expressed in this article are solely those of the authors and do not necessarily represent those of their affiliated organizations, or those of the publisher, the editors and the reviewers. Any product that may be evaluated in this article, or claim that may be made by its manufacturer, is not guaranteed or endorsed by the publisher.
Acknowledgments
We wish to extend our thanks to Gavin Ballantyne for proof reading and his insightful comments on the draft manuscript. We are also grateful to the Mikoko Pamoja and Vanga Blue Forest project communities for providing forest data for Gazi and Vanga mangrove sites, and to Kenya Marine and Fisheries Research Institute (KMFRI) for the rest of the Kenyan sites' data. Special thanks to the staff and teams from Kenya who provided expert and technical support in the collection of these data. Jason Cole of the US Forest service provided helpful advice on the SWAMP dataset. We also appreciate the invaluable input from the reviewers.
Supplementary Material
The Supplementary Material for this article can be found online at: https://www.frontiersin.org/articles/10.3389/ffgc.2022.767337/full#supplementary-material
References
ACES. (2021). Available online at: www.aces-org.co.uk (accessed March 02, 2022).
Adanguidi, J., Padonou, E. A., Zannou, A., Houngbo, S. B. E., Saliou, I. O., and Agbahoungba, S. (2020). Fuelwood consumption and supply strategies in mangrove forests - insights from RAMSAR sites in Benin. Forest Policy Econ, 116, 102192. doi: 10.1016/j.forpol.2020.102192
Allen, J. A., Ewel, K. C., Keeland, B. D., Tara, T., and Smith, T. J. (2000). Downed wood in Micronesian mangrove forests. Wetlands 20, 169–176. doi: 10.1672/0277-5212(2000)020[0169:DWIMMF]2.0.CO;2
Bosire, J. O., Kaino, J. J., Olagoke, A. O., Mwihaki, L. M., Ogendi, G. M., Kairo, J. G., et al. (2014). Mangroves in peril: unprecedented degradation rates of peri-urban mangroves in Kenya. Biogeosciences 11, 2623–2634. doi: 10.5194/bg-11-2623-2014
Bosire, J. O., Lang'at, J. K. S., Kirui, B. Y. K., Kairo, J. G., Mugi, L. M., Hamza, A. J., et al. (2015). “Mangroves of Kenya,” in Mangroves of the Western Indian Ocean: Status and Management, eds J. O. Bosire, M. M. Mangora, S. Bandeira, A. Rajkaran, R. Ratsimbazafy, C. Appadoo, et al. (Zanzibar Town: WIOMSA), 15–30.
Bunting, P., Rosenqvist, A., Lucas, R., Rebelo, L.-M., Hilarides, L., Thomas, N., et al. (2018). The Global mangrove watch—a new 2010 global baseline of mangrove extent. Remote Sens. 10, 1669. doi: 10.3390/rs10101669
Chow, J. (2018). Determinants of household fuelwood collection from mangrove plantations in coastal Bangladesh. Forest Policy Econ. 96, 83–92. doi: 10.1016/j.forpol.2018.08.007
Chowdhury, R. R., Uchida, E., Chen, L., Osorio, V., and Yoder, L. (2017). “Anthropogenic drivers of mangrove loss: geographic patterns and implications for livelihoods,” in Mangrove Ecosystems: A Global Biogeographic Perspective: Structure, Function, and Services, eds V. H. Rivera-Monroy, S. Y. Lee, E. Kristensen, and R. R. Twilley (Cham: Springer International Publishing), 275–300. doi: 10.1007/978-3-319-62206-4_9
Christensen, M., Hahn, K., Mountford, E. P., Ódor, P., Standovár, T., Rozenbergar, D., et al. (2005). Dead wood in European beech (Fagus sylvatica) forest reserves. Forest Ecol. Manag. 210, 267–282. doi: 10.1016/j.foreco.2005.02.032
Clough, B. F., and Scott, K. (1989). Allometric relationships for estimating above-ground biomass in six mangrove species. Forest Ecol. Manag. 27, 117–127. doi: 10.1016/0378-1127(89)90034-0
Cohen, R. (2014). Estimating the above-ground biomass of mangrove forests in Kenya (Ph.D. Thesis). Edinburgh: The University of Edinburgh.
FAO. (2016). Global Forest Resources Assessment. Rome: Food and Agriculture Organization of the United Nations.
FAO. (2020). Global Forest Resources Assessment 2020 - Key Findings. Rome: Food and Agriculture Organization of the United Nations.
Friess, D. A., Rogers, K., Lovelock, C. E., Krauss, K. W., Hamilton, S. E., Lee, S. Y., et al. (2019). The state of the world's mangrove forests: past, present, and future. Annu. Rev. Environ. Resour. 44, 89–115. doi: 10.1146/annurev-environ-101718-033302
Giri, C., Ochieng, E., Tieszen, L. L., Zhu, Z., Singh, A., Loveland, T., et al. (2011). Status and distribution of mangrove forests of the world using earth observation satellite data. Glob. Ecol. Biogeogr. 20, 154–159. doi: 10.1111/j.1466-8238.2010.00584.x
Goessens, A., Satyanarayana, B., Van Der Stocken, T., Zuniga, M. Q., Mohd-Lokman, H., Sulong, I., et al. (2014). Is matang mangrove forest in Malaysia sustainably rejuvenating after more than a century of conservation and harvesting management? PLoS ONE 9, e105069. doi: 10.1371/journal.pone.0105069
Gress, S. K., Huxham, M., Kairo, J. G., Mugi, L. M., and Briers, R. A. (2017). Evaluating, predicting and mapping belowground carbon stores in Kenyan mangroves. Glob. Change Biol. 23, 224–234. doi: 10.1111/gcb.13438
Harmon, M. E., Anderson, N. H., Franklin, J. F., Cline, S. P., Swanson, F. J., Aumen, N. G., et al. (1986). Harmon_1986.pdf. Adv. Ecol. Res. 15, 133–302. doi: 10.1016/S0065-2504(08)60121-X
Heilmann-Clausen, J., and Christensen, M. (2004). Does size matter? On the importance of various dead wood fractions for fungal diversity in Danish beech forests. Forest Ecol. Manag. 201, 105–117. doi: 10.1016/j.foreco.2004.07.010
Huxham, M., Dencer-Brown, A., Diele, K., Kathiresan, K., Nagelkerken, I., and Wanjiru, C. (2017). “Mangroves and people: local ecosystem services in a changing climate,” in Mangrove Ecosystems: A Global Biogeographic Perspective: Structure, Function, and Services, eds V. H. Rivera-Monroy, S. Y. Lee, E. Kristensen, and R. R. Twilley (Springer International Publishing), 245–274. doi: 10.1007/978-3-319-62206-4_8
Huxham, M., Emerton, L., Kairo, J., Munyi, F., Abdirizak, H., Muriuki, T., et al. (2015). Applying Climate Compatible Development and economic valuation to coastal management: a case study of Kenya's mangrove forests. J. Environ. Manag. 157, 168–181. doi: 10.1016/j.jenvman.2015.04.018
IPCC (2003). Good Practice Guidance for Land Use, Land-Use Change and Forestry, eds F. Penman, J., Gytarsky, M., Hiraishi, T., Krug, T., Kruger, D., Pipatti, R., et al. Tokyo: Institute for Global Environmental Strategies (IGES) for the IPCC.
IPCC (2014). 2013 Supplement to the 2006 IPCC Guidelines for National Greenhouse Gas Inventories: Wetlands, eds T. Hiraishi, T. Krug, K. Tanabe, N. Srivastava, J. Baasansuren, M. Fukuda, and T. G. Troxler (Cham: IPCC).
Jung, J., and Huxham, M. (2018). Firewood usage and indoor air pollution from traditional cooking fires in Gazi Bay, Kenya. Biosci. Horizons 11, hzy014. doi: 10.1093/biohorizons/hzy014
Kauffman, J. B., and Donato, D. C. (2012). Protocols for the Measurement, Monitoring and Reporting of Structure, Biomass and Carbon Stocks in Mangrove Forests. Bogor, Indonesia.
Kauffman, J. B., Hernandez Trejo, H., del Carmen Jesus Garcia, M., Heider, C., and Contreras, W. M. (2016). Carbon stocks of mangroves and losses arising from their conversion to cattle pastures in the Pantanos de Centla, Mexico. Wetlands Ecol. Manag. 24, 203–216. doi: 10.1007/s11273-015-9453-z
Keller, M., Palace, M., Asner, G. P., Pereira, R., and Silva, J. N. M. (2004). Coarse woody debris in undisturbed and logged forests in the eastern Brazilian Amazon. Glob. Change Biol. 10, 784–795. doi: 10.1111/j.1529-8817.2003.00770.x
Kirui, K. B., Kairo, J. G., Bosire, J. O., Viergever, K. M., Rudra, S., Huxham, M., et al. (2013). Mapping of mangrove forest land cover change along the Kenya coastline using Landsat imagery. Ocean Coastal Manag. 83, 19–24. doi: 10.1016/j.ocecoaman.2011.12.004
Lugo, A. E., and Snedaker, S. C. (1974). The ecology of mangroves. Annu. Rev. Ecol. Syst. 5, 39–64. doi: 10.1146/annurev.es.05.110174.000351
McKee, K. L., Rooth, J. E., and Feller, I. C. (2007). Mangrove recruitment after forest disturbance is facilitated by herbaceous species in the Caribbean. Ecol. Applic. 17, 1678–1693. doi: 10.1890/06-1614.1
McNally, C. G., Uchida, E., and Gold, A. J. (2011). The effect of a protected area on the tradeoffs between short-run and long-run benefits from mangrove ecosystems. Proc. Natl. Acad. Sci. U. S. A. 108, 13945–13950. doi: 10.1073/pnas.1101825108
Mugi, L. M., and Kairo, J. G. (2021). Dataset for mangrove dead wood in Kenya. Zenodo. doi: 10.5281/zenodo.5591883
Mungai, F., Kairo, J., Mironga, J., Kirui, B., Mangora, M., Koedam, N., et al. (2019). Mangrove cover and cover change analysis in the transboundary area of Kenya and Tanzania during 1986–2016. J. Indian Ocean Region 15, 157–176. doi: 10.1080/19480881.2019.1613868
Murdiyarso, D., Sasmito, S.D., Sillanpää, M., MacKenzie, R., and Gaveau, D. (2021). Mangrove selective logging sustains biomass carbon recovery, soil carbon, and sediment. Sci. Rep. 11, 12325. doi: 10.1038/s41598-021-91502-x
Pan, Y., Birdsey, R. A., Fang, J., Houghton, R., Kauppi, P. E., Kurz, W. A., et al. (2011). A large and persistent carbon sink in the world's forests. Science 333, 988–93. doi: 10.1126/science.1201609
Robertson, A. I., and Daniel, P. A. (1989). Decomposition and the annual flux of detritus from fallen timber in tropical mangrove forests. Limnol. Oceanogr. 34, 640–646. doi: 10.4319/lo.1989.34.3.0640
Romero, L. M., Smith, T. J., and Fourqurean, J. W. (2005). Changes in mass and nutrient content of wood during decomposition in a south Florida mangrove forest. J. Ecol. 93, 618–631. doi: 10.1111/j.1365-2745.2005.00970.x
Sandström, J., Bernes, C., Junninen, K., Lõhmus, A., Macdonald, E., Müller, J., et al. (2019). Impacts of dead wood manipulation on the biodiversity of temperate and boreal forests. A systematic review. J. Appl. Ecol. 56, 1770–1781. doi: 10.1111/1365-2664.13395
Sathirathai, S., and Barbier, E. B. (2001). Valuing mangrove conservation in southern Thailand. Contemp. Econ. Policy 19, 109–122. doi: 10.1111/j.1465-7287.2001.tb00054.x
Seibold, S., and Thorn, S. (2018). The Importance of Dead-Wood Amount for Saproxylic Insects and How It Interacts with Dead-Wood Diversity and Other Habitat Factors. Cham: Springer, 607–637. doi: 10.1007/978-3-319-75937-1_18
Siitonen, J. (2001). Coarse woody debris and saproxylic organisms : fennoscandian boreal forests as an example. Ecol. Bull. 49, 11–41. doi: 10.2307/20113262
Simpson, W. (1996). Method to Estimate Dry-Kiln Schedules and Species Groupings: Tropical and Temperate Hardwoods. Madison, WI: U.S. Department of Agriculture, Forest Service, Forest Products Laboratory.
Sitoe, A. A., Mandlate, L. J. C., and Guedes, B. S. (2014). Biomass and carbon stocks of Sofala Bay mangrove forests. Forests 5, 1967–1981. doi: 10.3390/f5081967
Söderberg, U., Wulff, S., and Ståhl, G. (2014). The choice of definition has a large effect on reported quantities of dead wood in boreal forest. Scand. J. Forest Res. 29, 252–258. doi: 10.1080/02827581.2014.896940
Spalding, M., Kainuma, M., and Collins, L. (2010). World Atlas of Mangroves. London: Routledge. doi: 10.4324/9781849776608
Steinke, T. D., Ward, C. J., and Rajh, A. (1995). Forest structure and biomass of mangroves in the Mgeni estuary, South Africa. Hydrobiologia 295, 159–166. doi: 10.1007/BF00029123
Stephenson, N. L., Van Mantgem, P. J., Bunn, A. G., Bruner, H., Harmon, M. E., O'Connell, K. B., et al. (2011). Causes and implications of the correlation between forest productivity and tree mortality rates. Ecol. Monogr. 81, 527–555. doi: 10.1890/10-1077.1
SWAMP Database Management – SWAMP. Available online at: https://www2.cifor.org/swamp/database/database-management/ (accessed March 1, 2022).
Tamooh, F., Huxham, M., Karachi, M., Mencuccini, M., Kairo, J. G., and Kirui, B. (2008). Below-ground root yield and distribution in natural and replanted mangrove forests at Gazi bay, Kenya. Forest Ecol. Manag. 256, 1290–1297. doi: 10.1016/j.foreco.2008.06.026
Appendix
Appendix 1. Research areas excluded during the “Web of Science” search.
“Mathematical computational biology; History; Endocrinology metabolism; Cardiovascular system, Cardiology; Evolutionary biology; Imaging science photographic technology; Infectious diseases; Communication; Materials science; Demography; Behavioral sciences; Health care sciences, services; Pathology; Polymer science; Geochemistry, geophysics; Education; Educational research; Public administration; Oncology; Mycology; Life sciences, biomedicine, other topics; Instruments, instrumentation; Sport sciences; Science technology, other topics; Automation control systems; Physiology; Psychology; Microscopy; Physical geography; Neurosciences, neurology; Cell biology; Women's studies; Entomology; Dermatology; Nutrition dietetics; Veterinary sciences; Integrative complementary medicine; Microbiology; Immunology; Spectroscopy; Developmental biology; Anthropology; Urban studies; Biotechnology, applied microbiology; Paleontology; Public environmental occupational health; Government law; Electrochemistry; Reproductive biology; Biophysics; Mining, mineral processing; Genetics heredity; Optics; Chemistry; Parasitology; Radiology, nuclear medicine, medical imaging; Geology; Sociology; General internal medicine; Anatomy morphology; physics; History, philosophy of science; Pharmacology, pharmacy; Operations Research, management science; Toxicology; Information science, library science; Research experimental medicine; Archaeology; Telecommunications; Physical sciences, other topics; International relations; Tropical medicine; Engineering; Gastroenterology, hepatology; Area studies; Hematology; Construction building technology; Mathematics; Respiratory system; Geriatrics gerontology; Food science technology; Computer science; Remote sensing; Biochemistry, molecular biology; Architecture; Art; Zoology; Business economics.”
Keywords: woody biomass, forest, mangrove, standing dead wood, downed wood, carbon, biomass
Citation: Mugi LM, Kiss D, Kairo JG and Huxham MR (2022) Stocks and Productivity of Dead Wood in Mangrove Forests: A Systematic Literature Review. Front. For. Glob. Change 5:767337. doi: 10.3389/ffgc.2022.767337
Received: 30 August 2021; Accepted: 14 April 2022;
Published: 10 May 2022.
Edited by:
Sigit Sasmito, National University of Singapore, SingaporeReviewed by:
Meriadec Sillanpaa, National University of Singapore, SingaporeEmilio Vilanova, University of California, Berkeley, United States
Copyright © 2022 Mugi, Kiss, Kairo and Huxham. This is an open-access article distributed under the terms of the Creative Commons Attribution License (CC BY). The use, distribution or reproduction in other forums is permitted, provided the original author(s) and the copyright owner(s) are credited and that the original publication in this journal is cited, in accordance with accepted academic practice. No use, distribution or reproduction is permitted which does not comply with these terms.
*Correspondence: Lilian Mwihaki Mugi, lilian.mwihaki@napier.ac.uk; mwihakimugi@yahoo.com