- 1Department of Entomology and Plant Pathology, University of Tennessee, Knoxville, TN, United States
- 2Department of Plant Pathology, Tropical Research and Education Center, University of Florida, Homestead, FL, United States
- 3Department of Plant Sciences, University of Tennessee, Knoxville, TN, United States
Thousand cankers disease (TCD), first documented in the western United States in the early 2000s, has spread into nine western and seven eastern states in the United States and northern Italy. TCD incidence and severity differ between eastern and western United States outbreak localities. Black walnut (Juglans nigra) trees, introduced into both urban and plantation settings in the western United States, have been severely impacted as evident by the documented high disease incidence and mortality. However, in eastern United States localities, where J. nigra is native, host-pathogen-vector interactions resulted in two different outcomes: trees either die or partly recover followed by infection. Recent genetic studies on the TCD causal agent, Geosmithia morbida, indicate the spatial genetic structure and high levels of genetic diversity among United States populations. Using detached branch inoculation assays, we reported differential virulence among 25 G. morbida isolates collected across the current distribution range of the disease. As a proxy for virulence, the canker area was measured to 7 days after inoculation. Varying degrees of virulence were observed among tested G. morbida isolates, which was partly explained by their genetic provenance (genetic clusters). Isolates that grouped within genetic cluster 2 (n = 7 from the eastern United States and n = 6 from the western United States; mean = 210.34 mm2) induced significantly larger cankers than isolates that grouped within genetic cluster 1 (n = 12; all western United States isolates; mean = 153.76 mm2). Canker sizes varied among isolates within each genetic cluster and were not correlated with a geographic region (eastern vs. western United States) but rather to the isolated state of origin. Mean canker size also differed in response to isolates that originated from different tree host species. G. morbida isolates collected from Juglans major induced statistically smaller cankers when compared to isolates recovered from undetermined Juglans species but not from J. nigra. In sum, the increased mortality reported for western United States walnut tree populations cannot be explained by a higher virulence of local G. morbida. Plausible explanations for the observed disparity include environmental conditions, such as prolonged drought, greater population densities of walnut twig beetle causing a higher number of inoculation events to individual trees, and multiple introductions of G. morbida originating from multiple locations. Future experimental evaluation should be undertaken to quantify the influence of these factors on the local epidemics.
Introduction
Thousand cankers disease (TCD) is a disease complex that involves interactions between walnut twig beetle (WTB), Pityophthorus juglandis Blackman (Coleoptera: Curculionidae: Scolytinae), a canker-causing fungal pathogen, Geosmithia morbida Kolarik, Freeland, Utley, and Tisserat (Ascomycota: Hypocreales: Bionectriaceae), and susceptible host plants, walnut (Juglans spp. L.) and wing nut (Pterocarya spp.) Kunth (Tisserat et al., 2009, 2011; Kolarik et al., 2011; Hishinuma et al., 2016). Although most Juglans and Pterocarya species are susceptible, symptoms are more severe in Juglans nigra L., the eastern United States native black walnut (Utley et al., 2013; Rugman-Jones et al., 2015; Hishinuma et al., 2016; Hefty et al., 2018). External symptoms of TCD include wilting, flagging, crown thinning, and branch dieback followed by the emergence of epicormic shoots (Tisserat et al., 2009). Internal symptoms include numerous localized small cankers within the phloem that can coalesce and girdle the tree (Kolarik et al., 2011; Tisserat et al., 2011). Vertical and horizontal phloem-restricted galleries beneath the bark provide evidence of beetle feeding and reproduction (Tisserat et al., 2011; Seybold et al., 2019).
Fungal members of the genus Geosmithia Pitt are ubiquitous and have been reported from North and South America, Europe, Asia, and Australia (Kolarík et al., 2008, 2017; Chahal et al., 2017). The majority of Geosmithia spp. are associated with bark and ambrosia beetles infesting diverse host plants, including coniferous and hardwoods (Chahal et al., 2017; Kolarík et al., 2017; Schuelke et al., 2017; Huang et al., 2019). Although most Geosmithia spp. are saprophytes, a few species can act as weak pathogens (Schuelke et al., 2017), including G. morbida (Kolarik et al., 2011; Kolarík et al., 2017), which was the first pathogenic species described in this genus. The second one was Geosmithia sp. 41 (initially identified as Geosmithia pallida by Lynch et al., 2014), the causal agent of foamy bark disease on Quercus agrifolia Nee. (California live oak) and other oak species (Huang et al., 2019). Although the associations of Geosmithia spp. with bark and ambrosia beetles are documented (Huang et al., 2017; Jankowiak and Bilański, 2018), the details of the ecological interactions among Geosmithia spp., its beetle vector(s), and host plant(s) are not fully understood (Huang et al., 2019). This is true for the TCD pathosystem, in which the nutritional role of G. morbida is still largely unknown. G. morbida has only been reported to colonize hosts in the Juglandaceae family (Tisserat et al., 2009). However, the fungus has been recently recovered from other subcortically active beetles besides WTB (Chahal et al., 2019; Moore et al., 2019), suggesting that the fidelity of G. morbida to WTB as the most functional beetle associate may have been overestimated. Additional work is needed to elucidate the potential role of other insect species in the efficient transmission of the pathogen and on the development of disease on susceptible hosts (Chahal et al., 2019).
Although, by 2009, TCD was documented in most of the states of western United States (Tisserat et al., 2009, 2011), the first detection in the eastern United States (Knoxville, Tennessee) was recorded in 2010, representing an imminent threat to J. nigra in urban and forest settings (Grant et al., 2011). The initial occurrence of TCD in the western United States is spatially pertinent since it overlaps with the native range of the TCD vector, WTB (Kolarik et al., 2011; Sitz et al., 2021). Following the initial discovery of TCD in Tennessee, members of this disease complex were subsequently reported in Indiana, Maryland, North Carolina, Ohio, Pennsylvania, and Virginia (Hansen et al., 2011; Hadziabdic et al., 2014; Daniels et al., 2016), as well as in Italy (Montecchio and Faccoli, 2014; Moricca et al., 2019). Forest Inventory and Analysis (FIA) projections estimated that TCD could have been present in the eastern United States at least 10 years before the first report was made (Randolph et al., 2013). In the eastern United States, J. nigra mortality attributed to TCD has been very low (<5%) in comparison with mortality rates in western states where ~60% of J. nigra populations have been removed (Newton et al., 2009; Tisserat et al., 2011; Randolph et al., 2013; Seybold et al., 2019). Furthermore, recovery of some J. nigra trees following TCD infection has been documented in the eastern United States (Griffin, 2015; Seybold et al., 2019). Slow TCD progression and partial recovery have also been observed in affected populations distributed in the western United States (Seybold et al., 2019) but at lower rates compared to the eastern United States. In the western United States, the high tree mortality of Juglans spp. has been observed in diverse habitats, including agricultural plantations, urban forests, and wildland settings (Seybold et al., 2019). These patterns were not observed in the eastern United States, where the majority of affected trees are distributed in the urban landscape, along roads, and in recreational parks, suggesting that resistance to G. morbida may be naturally present in native, J. nigra trees (Griffin, 2015; Seybold et al., 2019; Sitz et al., 2021). We hypothesized that differences in TCD outcome observed between eastern and western United States are due to differential virulence present in G. morbida populations.
Population studies have revealed high genetic diversity and spatial structure among G. morbida populations distributed across the United States (Hadziabdic et al., 2014; Zerillo et al., 2014). G. morbida populations collected across the entire geographic distribution of TCD can be grouped into two distinct genetic clusters (Hadziabdic et al., 2014; Hadziabdic et al., unpublished). This grouping not only indicates the complexity of the pathogen inoculum but also supports earlier suggestions that G. morbida, such as WTB, is likely native to North America (Sitz et al., 2021). The current working hypothesis states that G. morbida evolved in close association with at least one native Juglans species within the western United States range of WTB and that G. morbida was present, but undetected, in the eastern United States for a long time before it was first documented (Hadziabdic et al., 2014; Zerillo et al., 2014; Sitz et al., 2021). The study by Sitz et al. (2021) supported this hypothesis by reporting regional resistance and variation in host responses to G. morbida infestations in J. nigra trees. Their results indicated higher resistance in J. nigra populations in the western and central ranges of the native distribution, where TCD has not been documented (Sitz et al., 2021).
The objective of this study was to determine whether isolates collected from different localities have different virulence profiles. We hypothesized that differences in G. morbida virulence are correlated to their genetic cluster assignment and that their genetic grouping can explain the difference in TCD incidence and severity observed between eastern and western states. Using canker size as a proxy for virulence, our specific objectives were a) to measure canker size resulting from G. morbida inoculation into J. nigra host branches and b) to compare canker size variation as a function of genetic clustering and geographic and host origin.
Materials and Methods
Selection of G. morbida Isolates
Preliminary genetic profiling from early examinations of this data set led to the selection of the 25 G. morbida isolates that were used in this study. Isolates were chosen based on their genetic diversity and assignment probability to belong to one of the assigned genetic clusters (Hadziabdic et al., unpublished data; Supplementary Figure S1). More specifically, these isolates were chosen as representatives of five putative genetic clusters and were used to initiate the experiments. Subsequent addition of G. morbida isolates from additional states led to a reanalysis of the data and reorganization of genetic variability across the United States into two genetic clusters (Hadziabdic et al., unpublished data; Supplementary Figure S1) that are consistent with the previous study (Zerillo et al., 2014). Thus, experiments reported here were conducted with 12 isolates from genetic cluster 1 (all western United States isolates) and 13 isolates representing genetic cluster 2 (n = 7 from the eastern United States and n = 6 from the western United States) (Table 1).
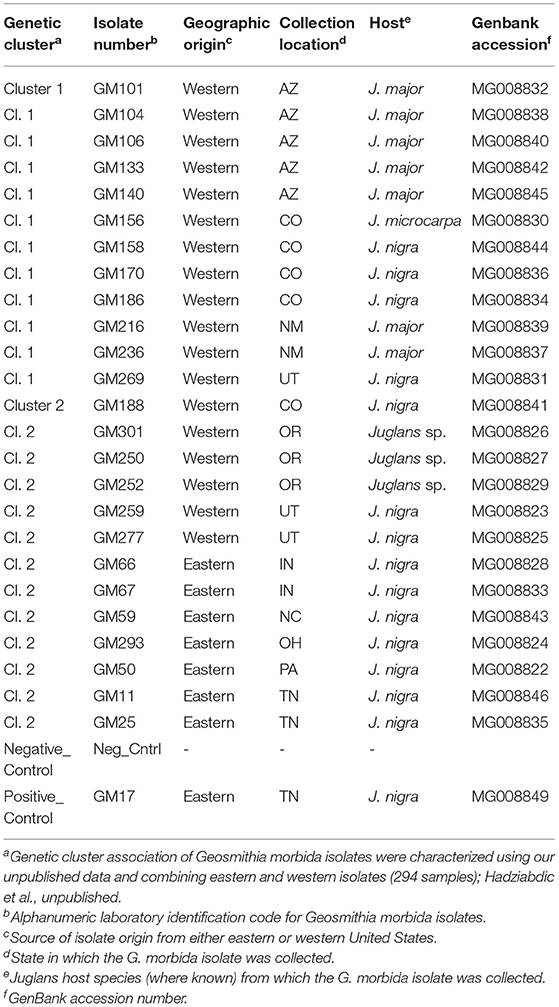
Table 1. Geosmithia morbida isolates selected for inoculation experiment per genetic groupings of Hadziabdic et al. unpublished.
Molecular Confirmation of Selected Axenic Isolates
Axenic single-spore cultures were obtained from all the 25 G. morbida isolates following established protocols (Hadziabdic et al., 2014). All isolates were confirmed as G. morbida through morphological (prior to this study) and molecular identification. In brief, mycelial plugs from each isolate were grown in Difco™ Potato Dextrose Broth at room temperature (25°C) for 3 weeks following established protocols (Gazis et al., 2018). DNA was extracted from harvested mycelium as described previously (Gazis et al., 2018; Chahal et al., 2019). The Internal Transcribed Spacer (ITS) region was amplified using the primers ITS1F (Gardes and Bruns, 1993) and ITS4R (White et al., 1990). Each PCR reaction-mix (final volume 25 μl) contained 12.5 μl GoTaq®G2 Hot Start Master Mix (Promega Corp., Madison, WI, USA), 1.25 μl 10 mM reverse primer, 1.25 μl 10 mM forward primer, 1 μl dimethyl sulfoxide (DMSO, Sigma–Aldrich, St Louis, MO, USA), 1 μl of genomic DNA (~25 ng/μl), and double-distilled water to complete the total volume of 25 μl. The PCR thermal cycle started with an initial denaturation step of 2 min at 94°C followed by 15 cycles of denaturation for 30 s at 94°C, annealing for 30 s at 65°C, and primer extension for 1 min at 72°C; followed by 30 cycles of 30 s at 94°C, 30 s at 48°C, and 1 min at 72°C; and followed by a final elongation for 10 min at 72°C. The amplifications of PCR products were confirmed with gel electrophoresis. PCR products were sent to MCLAB laboratories (www.mclab.com) for cleaning and sequencing. Sequencher TM 4.9 (Gene Codes Corp., Ann Arbor, MI, USA) was used to assess the quality of the chromatograms and assemble the strands into contigs. Basic Local Alignment Search Tool (BLAST) was used to compare the ITS sequences deposited in the NCBI nucleotide database. Generated ITS sequences were deposited to GenBank (Table 1).
Preparation of J. nigra Branch Sections
Detached branch assays were used to limit the potential confounding effects inherent in using field trees, such as differences in host genotype and microclimates. Two mature (>35-year-old) J. nigra trees located at Middle Tennessee Research & Education Center, Spring Hill, Tennessee, USA (latitude/longitude 35°43'01.3” N, 86°57'19.3” W; 35°43'03.0” N, 86°57'18.7” W) were selected as a source of branch sections for each of the two inoculation experiments that were repeated. For each inoculation treatment, each of two replicates was completed using branch sections taken from a single tree. None of the TCD complex members have been reported in Spring Hill, or from Maury and Williamson counties in Tennessee where Spring Hill is located. Symptoms of TCD were not observed on trees at the Spring Hill site. Once branches were detached, 10 cut branch sections (~1 m length) from each tree were visually inspected for the presence of TCD signs and symptoms. During the examination, a portion of the outer bark layer from branch sections was peeled to verify that cankers, phloem discoloration, lesions, or necrotic areas nor galleries of other wood-boring beetles were present. Collected tree branches were also verified to be free of G. morbida using the molecular protocol described by Oren et al. (2018). In brief, three individual samples each containing approximately 150 mg of drilled inner wood shavings were collected from portions of 10 different branches that were harvested from each tree (Supplementary Figure S2). Samples were stored at −20°C until processed. DNA extractions, PCR amplifications, and amplicons were conducted as described by Oren et al. (2018). Molecular detection of G. morbida from source branches was negative for pathogen DNA, indicating that prior to this experiment, selected branch sections were free from G. morbida infection. The cut branches were stored at 4°C (~5 days) prior to inoculation. Branches were cut into branch sections (length = 8 cm, diameter = 2–3 cm) using a handsaw and surface-sterilized with 70% ethanol (Supplementary Figure S3). Cut ends of inoculated branch sections were then dipped into paraffin wax and wrapped with aluminum foil to prevent evaporation and preserve moisture.
Inoculations of J. nigra Branch Sections With G. morbida Isolates
Fungal isolates were grown in Petri dishes (10 cm diameter, 1.5 cm depth) containing 20 ml of half-strength PDA at 25°C for 4 weeks. G. morbida isolates GM17, which is a well-characterized strain used in multiple previous studies (e.g., host-plant inoculations, development of molecular detection protocols, and comparative genetics and genomics experiments) was used as a positive control. Agar-only plugs were inserted into wounds as a negative control. Once branch pieces had dried following surface sterilization, sections were chosen randomly for G. morbida inoculation experiments (Supplementary Figure S3). Using a cordless drill with a 3.175-mm diameter bit, two ~2-mm-deep wounds were offset on opposing sides of six separate branch sections per fungal isolate. Branch sections were inoculated by using a sterile, 4-mm diameter (o.d.) Humboldt Brass Cork Borer (Fisher Scientific, Pittsburgh, PA, USA) to remove a piece of fungal agar plug, which was then picked using a sterile scalpel, inverted, and inserted completely into each of the two opposing wounds per branch section. Colonized agar plugs were obtained from the edge of the growing colonies, and all plugs were completely covered by the fungus. Wounded branch sections were wrapped with Parafilm (M Laboratory Film, Bemis, Neenah, WI, USA), and the diameter of each inoculated branch section was recorded using a digital caliper (Lyman Products Corp., Middletown, CT, USA). Inoculated branch sections were incubated at 25°C in darkness for 7 days.
Canker Measurements
To visualize cankers in the phloem of cut branches before extensive tissue oxidation occurred, lesions were assessed to 7 days after inoculation (7 DAI). A sterile scalpel was used to shave the outer bark from around the wounding site exposing the cankers (Supplementary Figure S3), and the digital images of exposed cankers beside a reference scale were taken immediately. Image processing and analysis software, ImageJ (https://imagej.nih.gov/ij/) (Schneider et al., 2012), was used to measure canker area from digital photographs. The software was calibrated to standardize each photograph to the reference scale included in each photograph.
Confirmation of Pathogenicity via Modified Koch's Postulates
To confirm G. morbida as the causal agent of the observed cankers, a modified version of Koch's postulates was conducted using the rapid molecular detection method described by Oren et al. (2018). Three of six replicated branch sections used in each isolate inoculation were randomly selected, and one of the inoculation sites was selected for molecular screening. Under sterile conditions, drill shavings of phloem and wood tissues were collected only from the margins of the cankers, excluding the inoculation wound site. Sample collection, DNA extraction, and PCR amplification for pathogen detection were performed as described by Oren et al. (2018).
Statistical Analysis
The effects of the genetic cluster, isolates, Juglans host, location (state), and region (eastern vs. western United States) (Table 1) on canker size were each analyzed using the mixed model analysis for split-plot design with the individual J. nigra trees as the experiment unit for the whole plot effects. Diagnostic analysis was conducted to assess model assumptions. The rank transformation was applied if diagnostic analysis exhibited violation of normality and equal variance assumptions. Post-hoc multiple comparisons were performed with Tukey's adjustment. Statistical significance was identified at P < 0.05. Analyses were conducted in SAS 9.4 TS1M7 for Windows 64x (SAS Institute Inc., Cary, NC, USA).
Results
Confirmation of Koch's Postulates
From 150 samples tested (25 G. morbida isolates across six replicates), 147 were positive for the presence of G. morbida DNA in tissues collected from the margins of cankers (98% G. morbida detection rate). Phloem margins from darkened damage-response areas of six agar-only control samples were also tested, and G. morbida DNA was not detected from any of these samples.
Effect of the Variation in Branch Section Diameter on Canker Area
Branch sections of 2–3 cm diameter (average of 2.5 cm) were used for inoculations. The potential effect of variation in branch section diameter on the canker area was analyzed. Canker area did not differ in response to branch diameter (P = 0.569); therefore, branch diameters were pooled for further analyses.
Effect of Genetic Cluster, State, and Host Origin of Fungal Isolate on Canker Size
Overall, G. morbida isolates from genetic cluster 2 induced significantly larger cankers than the ones from cluster 1, yielding a mean canker area of 210.34 mm2 and 153.76 mm2, respectively (P = 0.034; Figure 1). Canker sizes did not differ significantly among isolates within cluster 1 (P =0.080), but canker sizes differed among isolates within cluster 2 (P = 0.0003). All G. morbida isolates resulted in the formation of cankers with areas that were significantly larger than responses observed in the negative control (effect of wound in isolation), except for isolates GM140 (P = 0.184) and GM158 (P = 0.196) (affiliated with cluster 1) and GM11 (P = 0.623) and GM59 (P = 0.063) (affiliated with cluster 2) (Figure 2). These isolates induced cankers; however, their smaller lesion areas were not statistically different from lesions produced by the wounding alone (Figure 2). Irrespective of genetic cluster association, all G. morbida isolates were variable in the mean canker sizes observed 7 DAI (P < 0.001). Despite variability observed across mean canker sizes from all isolates (Figure 2), differences were observed in mean canker sizes when analyzed according to the states in which the G. morbida isolates were collected (P < 0.001). At 7 DAI, the largest mean canker areas were induced by isolates GM 293 (OH), GM 277 (UT), GM 50 (PA), GM 259 (UT), and GM 301 (OR) (Figure 2). The largest canker sizes were observed across a mixture of western (OR and UT) with eastern (IN, OH, and PA) states. Similar variability was also evident in the state origins of isolates that induced smaller canker areas. However, we caution that for several states (OH, PA, and NC), only a single isolate could be obtained for assays, which restricts the validity of predictions that may be inferred regarding isolates from these states. When the results from these three state isolates were removed from data analysis, the effect of canker size on collection location (AZ, CO, IN, NM, OR, TN, and UT) was significant (P = 0.0019). To address this limitation to the experimental design, a comparison of canker areas was analyzed according to the eastern vs. western geographic region from which isolates originated and canker sizes among G. morbida isolates did not statistically differ (P = 0.927). Thus, our results indicate that the geographic origin of isolates is not a good predictor of expected virulence. Mean canker areas observed between geographic groups were 188 ± 8.6 mm2 for eastern and 181 ± 4.4 mm2 for western isolates (data not shown).
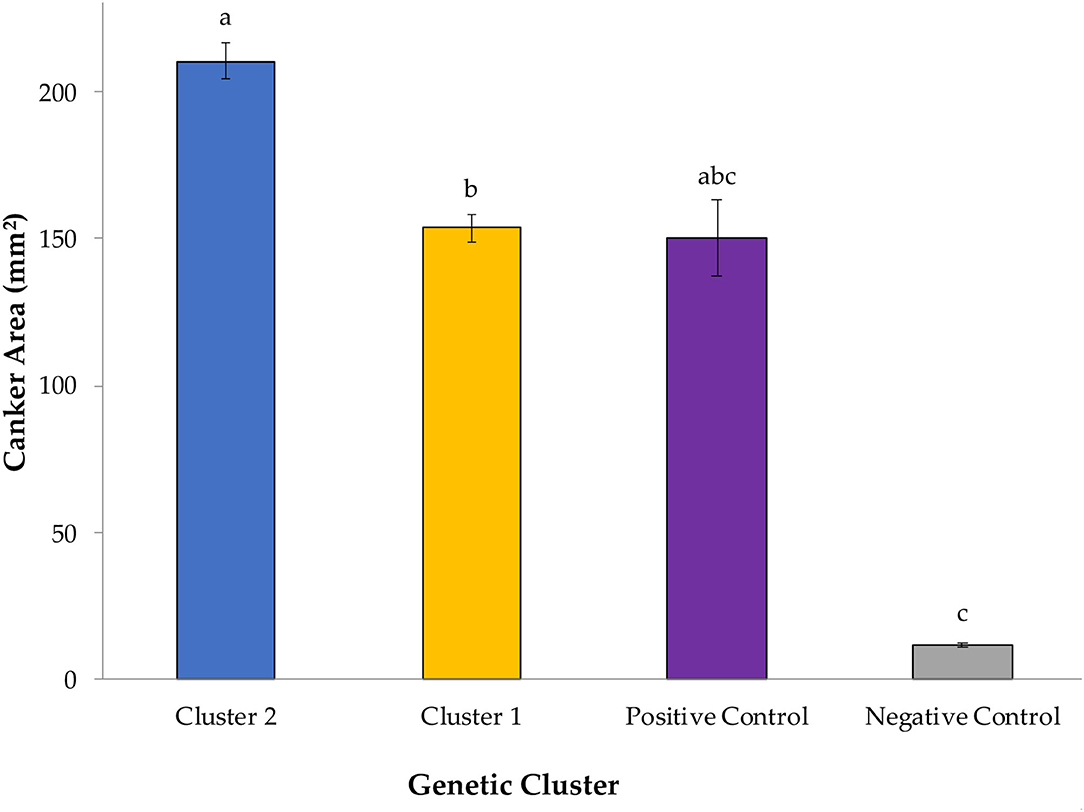
Figure 1. Mean canker sizes were observed at 7 days after inoculation (DAI) with Geosmithia morbida isolates representing 2 genetic clusters, as were characterized in the studies by Hadziabdic et al. (2014) and Hadziabdic et al. (unpublished data, Supplementary Figure S1). In this study, 12 G. morbida isolates represented genetic cluster 1 and 13 G. morbida isolates represented genetic cluster 2. Within cluster or control group, treatment mean separations were made using least square mean estimates calculated from rank-transformed canker areas and differ significantly (P < 0.05; Tukey's honestly significant difference) when indicated with different letters. Mean canker areas and standard errors of genetic clusters and controls depicted here reflect non-transformed data measured across assays performed on both assayed trees.
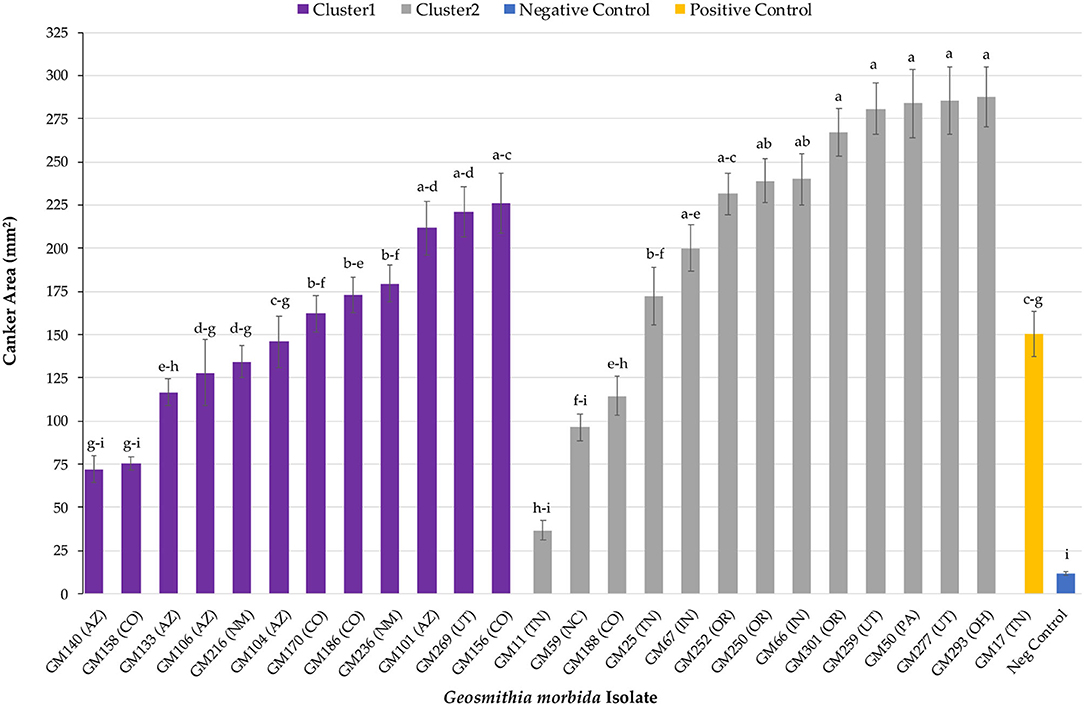
Figure 2. Mean canker area observed across all G. morbida isolates. For each isolate, treatment mean separations were made using least square mean estimates calculated from rank-transformed canker areas and differ significantly (P < 0.05; Tukey's honestly significant difference) when indicated with different letters. Mean canker areas and standard errors depicted here reflect non-transformed data measured across both assayed trees.
Finally, the observed mean canker areas differed when isolates were grouped by origin from different Juglans sp. hosts (P = 0.048). Mean canker area of isolates collected from Juglans major induced statistically smaller cankers when compared to isolates recovered from undetermined Juglans spp. (P = 0.045) but not from J. nigra (P = 0.134) (Figure 3). Cankers induced by the isolate recovered from Juglans microcarpa, which were excluded from statistical comparisons due to limited sample size, yielded intermediate-sized cankers averaging 225.81 ± 17.28 mm2.
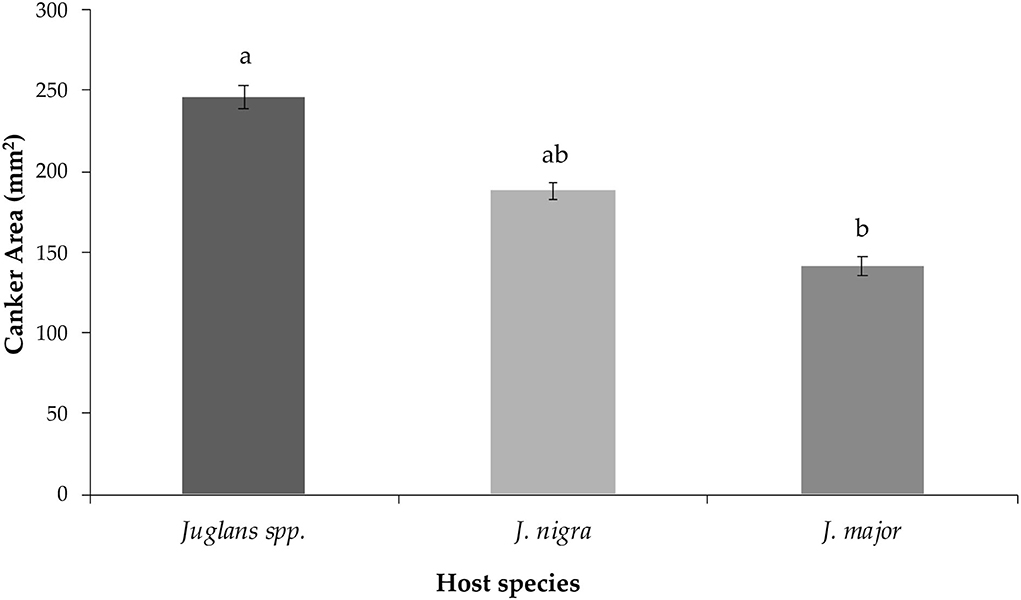
Figure 3. Mean canker area measured for lesions induced by G. morbida isolates that were grouped based on Juglans species host source as follows: undetermined Juglans spp., Juglans major, and Juglans nigra. Within species, treatment mean separations were made using least square mean estimates calculated from rank-transformed canker areas and differ significantly (P < 0.05; Tukey's honestly significant difference) when indicated with different letters. Non-transformed data and standard errors are presented.
Discussion
All tested G. morbida isolates induced canker formation. However, the geographic origin of the isolates (eastern vs. western origin) was not correlated to their virulence and could not be used to provide meaningful insights into the different disease outcomes observed between the two regions. While our data indicate that G. morbida isolates assigned to genetic cluster 2 (mixture of eastern and western isolates) yielded cankers with a larger mean size than isolates in cluster 1 (western isolates only), the virulence of G. morbida may only be partially explained by their genetic grouping into distinct clusters (clustering herein was based on G. morbida microsatellites, Hadziabdic et al., 2014, unpublished data). Isolates belonging to the same cluster induced a wide range of canker sizes. After 7 days, canker areas induced by G. morbida isolates in cluster 2 ranged in size from 36 to 287 mm2 (from isolates GM11 and GM293, respectively) compared with canker areas from 72 to 225 mm2 (isolates GM140 and GM156, respectively) induced by G. morbida isolates from cluster 1. Overall, G. morbida isolates from genetic cluster 2 yielded cankers that were more variable in size than isolates from genetic cluster 1. Significant differences were observed among mean canker sizes according to the states from which the G. morbida isolates were collected. Given the variability observed in canker sizes at 7 DAT, however, state provenance of the isolates was not a reliable predictor of potential virulence. This observation is further evidence in support of the hypothesis that multiple introductions of G. morbida have occurred across time and which have originated from multiple locations (Hadziabdic et al., 2014; Zerillo et al., 2014). Given the lack of statistical significance observed when comparing canker sizes induced by western vs. eastern isolates, our hypothesis for explaining the greater TCD severity seen in the western localities was not supported by these laboratory assays. Similarly, results do not support anecdotal observations that G. morbida has been less virulent in the eastern United States. Factors, including environmental conditions, host-plant genetics, and interactions with other fungi and microorganisms, are likely to contribute to virulence across the distributed range of G. morbida. We also acknowledged that the lack of resolution could be partly due to the low number of isolates from eastern (n = 7) in comparison with western (n = 18) states.
Detached branch and stem assays are often used in pathogenicity assays for fungi affecting shrubs (Wagner et al., 2006), woody ornamentals (Guo et al., 2015), and forest trees (Amponsah et al., 2011; Sessa et al., 2017) as rapid alternatives to in planta inoculations. Detached branch assays can be as reliable as in vivo assays for pathogenicity, virulence, and germplasm screening experiments (Smith, 1996; Kohpina et al., 2000; Dodd et al., 2005; Stewart et al., 2005; Baskarathevan et al., 2012). These assays are also particularly appropriate when regulated or quarantined organisms, such as G. morbida, are being studied. In addition, detached tissue assays are easier to handle and offer greater reproducibility by enabling the use of branch sections with uniform diameters allowing appropriate replication, consistent inoculum delivery, and greater accuracy in the quantification of disease symptoms (Kohpina et al., 2000; Hüberli et al., 2002). However, this system is not without its flaws or biological shortcomings. Disadvantages of detached tissue assays are that host-defense mechanisms may be compromised and detached branches may not adequately reflect the whole plant response (Sessa et al., 2017). For example, larger cankers were observed on detached branches of necrotic grapevine tissue compared to results from in vivo branch inoculations, which shows the importance of host-plant defense response in mitigating disease severity (Amponsah et al., 2011).
Virulence can play an important role in pathogen emergence and reemergence, potential host switches, and range expansion, thus making it very challenging to control and contain further spread (Sacristán and García-Arenal, 2008). Our study demonstrated variable virulence outcomes for isolates from both of our genetic clusters. This mixed result is consistent with the variable virulence that was observed by Tran et al. (2020), in which the virulence of the fungal pathogens Monilinia fructicola and Monilinia laxa on Japanese plum, Prunus salicina cv. “Fortune,” did not correlate to pathogen genotypes. Similar results have been reported in other studies using in planta and detached branch assays, wherein differences in virulence were not correlated with genetic diversity of canker-causing fungal pathogens (Baskarathevan et al., 2012; Billones-Baaijens et al., 2013; Elena et al., 2015). The geographic origin of fungal isolates also was not associated with the virulence of canker-forming Neonectria ditissima fungus in apple trees (Campos et al., 2017) nor for various canker-forming Botryosphaeriaceae fungal pathogens that infect multiple host plants, including softwood and hardwood tree species (Mohali et al., 2007; Piškur et al., 2011; Félix et al., 2017).
Zerillo et al. (2014) used both single nucleotide polymorphisms and microsatellite markers to genotype G. morbida isolates across 17 geographic regions distributed across eastern and western United States with results that revealed the presence of four genetically distinct groups that were clustered into three different geographic regions. Based on this information, Sitz et al. (2017) conducted an in planta pathogen virulence field study using isolates from three genetic clusters. In their study, the virulence of G. morbida (using canker size as proxy) isolates was evaluated when inoculated into branches of standing trees. The authors also assessed canker formation when branches were co-inoculated with Fusarium solani species complex (FSSC). This latter step was undertaken because FSSC members have been recovered with G. morbida and might be associated with disease severity in the late stages of the disease (Tisserat et al., 2009; Montecchio et al., 2015).
Similar to our study, canker sizes induced by G. morbida (only) isolates from Colorado were not significantly correlated within the genetic cluster of the isolate (Sitz et al., 2017). Unlike our study, however, no significant difference in canker size was observed between genetic clusters (Sitz et al., 2017). The 183 mm2 canker area that was averaged among all G. morbida isolates used in our study was much larger than the 87 mm2 mean canker area reported by Sitz et al. (2017). From the co-inoculation efforts of Sitz et al. (2017) with G. morbida and FSSC members, the authors found that these pathogens do not yield a synergistic response. In our own preliminary studies on co-inoculation of juvenile J. nigra trees with F. solani and with eastern United States (TN), the isolates of G. morbida yielded contradictory results (data not shown). We caution that the specific role of FSSC members in tree mortality remains under-examined and should be a focus of future pathogenicity efforts. In sum, other factors including differences in environmental conditions, inoculation method, incubation period, host-plant age and condition, and in planta vs. detached branch assays all are likely to have contributed to different results found between Sitz et al.'s (2017) study and our study. Ideally, our ability to resolve these questions would require in-field, in planta assays using isolates from across the distributed range of G. morbida and inoculated into clonal Juglans germplasm.
Forest pathosystems are complex, especially when an insect vector is involved or more than one pathogen species contribute to the disease, as occurs in Ceratocystis wilt of ‘ohi‘a (Hughes et al., 2020), beech leaf disease (Burke et al., 2020; Ewing et al., 2021), black pod of cocoa (Guest, 2007), and TCD on walnuts and wing nuts (Tisserat et al., 2009; Montecchio et al., 2015). The potential for multiple pathogen interactions makes it difficult to identify and document the role of each organism in tree decline. Interestingly, the F. solani isolate that was originally recovered from Colorado (Tisserat et al., 2009) did not yield a synergistic response in terms of canker size when co-inoculated with G. morbida on J. nigra branches (Sitz et al., 2017). Sitz et al. (2017) found that cankers induced by Fusarium alone were not different from the cankers induced by negative control inoculations. Members of FSSC have also been found in diseased J. nigra and Juglans regia trees in Italy where TCD has been recently introduced (Montecchio et al., 2015). Montecchio et al. (2015) observed that cankers induced by a Fusarium isolate from FSSC-25 alone were similar to cankers induced by Fusarium and G. morbida co-inoculations and were significantly larger than negative control inoculations. Hence, their results suggest Fusarium as a contributing pathogen to early stages of TCD in Italy (Montecchio et al., 2015). Differences in virulence results of Fusarium isolates in both studies (Montecchio et al., 2015; Sitz et al., 2017) are similar to our observed differences in G. morbida inoculation trails. These inconsistencies may be explained by the differences in F. solani/G. morbida strains used in testing trials, genotype, and age of host, environmental conditions, type of propagules used as inoculum, and incubation period. In future, a large-scale study that involves the inoculations of multiple strains of Fusarium from different TCD-associated FSSC groups on diverse J. nigra genotypes could help articulate the functional role of Fusarium in TCD etiology and epidemiology.
We also examined if virulence was correlated to the tree host species. If TCD members (host, pathogen, and vector) exist in isolation, pathogen populations could have adapted to particular hosts and, therefore, respond differently when inoculated into J. nigra. The isolates used in this study were recovered from cankers on J. major, J. microcarpa, J. nigra, and unidentified Juglans spp. Canker sizes were significantly different when isolates were analyzed by host. Isolates collected from J. major induced significantly smaller cankers compared to isolates collected from other Juglans spp. but not from J. nigra. This finding is not surprising since the TCD vector, i.e., WTB, is native to the southwestern United States, and it has been hypothesized that both G. morbida and WTB have coevolved in a native host, Arizona walnut (J. major) (Zerillo et al., 2014; Rugman-Jones et al., 2015; Seybold et al., 2019). One plausible explanation is that G. morbida isolates from J. major might have reduced virulence on that particular host due to a coevolutionary relationship, and the concept of host-jumping into other Juglans spp. could provide further clues into the current virulence and migration patterns of G. morbida. This idea was further elaborated in a recent study by Sitz et al. (2021) that included 640 trees from wild and selected J. nigra families and found that improved seedlings (improved selections for enhanced growth and timber quality) exhibited larger canker sizes when compared to wild trees from the same provenance. Unfortunately, breeding efforts (Beineke, 1989; McKenna and Coggeshall, 2018; Sitz et al., 2021) may have resulted in nontargeted selection for reduced defense response against G. morbida infection. The authors further proposed that differences in TCD dynamics between J. nigra tree populations distributed in the western range of the species natural distribution vs. populations distributed in the central region (wild trees in the regions without TCD) reflect enhanced genetic resistance as a result of coevolution with G. morbida (Sitz et al., 2021).
Conclusion
Our results indicated varying degrees of virulence among tested G. morbida isolates, which was partly explained by their genetic provenance (state-of-origin). From the variables we tested here, genetic clustering (G. morbida isolates from genetic cluster 2 induced significantly larger cankers), state of origin, and Juglans host species could provide some explanation regarding the differences observed in G. morbida canker sizes. Although significant differences in canker size were noted when results from isolates were pooled within genetic cluster, host, geographic, and state-of-origin factors, a high level of variability was observed across most of these tested variables. Differential TCD severity and incidence between the western and eastern regions of the United States might instead result from other biotic (Gazis et al., 2018; Chahal et al., 2019) and abiotic factors (Griffin, 2015), or a combination of both (Seybold et al., 2019). The infestation level of WTB is likely a critical factor in determining tree mortality, as tree decline and death occur due to cambium-girdling caused by the coalescence of G. morbida cankers formed as a consequence of numerous beetle inoculations and WTB gallery formations (Tisserat et al., 2011). Hence, the lower levels of WTB infestation will result in less G. morbida inoculations and less likelihood of lesion coalescence to occur. In the eastern United States, WTB populations have been declining (Chahal et al., 2019), which in turn may be limiting the impact of TCD where J. nigra is native (Seybold et al., 2019).
Data Availability Statement
The raw data supporting the conclusions of this article will be made available by the authors upon request, without undue reservation.
Author Contributions
DH, RG, WK, and MW conceived and designed the experiments including the major conceptual ideas and proof outline. KC and MW collected samples. KC carried out the experiments. RG contributed to the processes. All authors contributed to the interpretation of the results, manuscript writing, editing, and provided critical feedback to shape the experiments, analyses, and finally produce the manuscript.
Funding
This research was conducted in partial fulfillment of an MS degree for K.C. from The University of Tennessee and was funded by the Woodtiger Foundation as part of the Evaluating Impact of Thousand Cankers Disease on Black Walnut: Risk Assessment, Biological Control, and Development of Novel Assessment Tools project. Additional support was provided by the USDA National Institute of Food and Agriculture Hatch Project #1009630: TEN00495.
Conflict of Interest
The authors declare that the research was conducted in the absence of any commercial or financial relationships that could be construed as a potential conflict of interest.
The reviewer PB declared a past collaboration with the author DH to the handling editor at the time of review.
The reviewer BD declared a shared affiliation with the author RG to the handling editor at the time of review.
Publisher's Note
All claims expressed in this article are solely those of the authors and do not necessarily represent those of their affiliated organizations, or those of the publisher, the editors and the reviewers. Any product that may be evaluated in this article, or claim that may be made by its manufacturer, is not guaranteed or endorsed by the publisher.
Acknowledgments
We thank Mr. Kevin Thompson (Director, Middle Tennessee Research & Education Center, Spring Hill, TN) for his help in collecting J. nigra branches. We are thankful to Sara Collins (University of Tennessee, Knoxville, TN, USA) for her assistance in laboratory work and to Sun Xiaocun (University of Tennessee, Knoxville, TN, USA) for statistical consulting and analyses.
Supplementary Material
The Supplementary Material for this article can be found online at: https://www.frontiersin.org/articles/10.3389/ffgc.2022.726388/full#supplementary-material
References
Amponsah, N. T., Jones, E. E., Ridgway, H. J., and Jaspers, M. V. (2011). Identification, potential inoculum sources and pathogenicity of botryosphaeriaceous species associated with grapevine dieback disease in New Zealand. Eur. J. Plant Pathol. 131, 467. doi: 10.1007/s10658-011-9823-1
Baskarathevan, J., Jaspers, M. V., Jones, E. E., Cruickshank, R. H., and Ridgway, H. J. (2012). Genetic and pathogenic diversity of Neofusicoccum parvum in New Zealand vineyards. Fungal Biol. 116, 276–288. doi: 10.1016/j.funbio.2011.11.010
Beineke, W. F. (1989). Twenty years of black walnut genetic improvement at Purdue University. North. J. Appl. For. 6, 68–71. doi: 10.1093/njaf/6.2.68
Billones-Baaijens, R., Jones, E. E., Ridgway, H. J., and Jaspers, M. V. (2013). Virulence affected by assay parameters during grapevine pathogenicity studies with Botryosphaeriaceae nursery isolates. Plant Pathol. 62, 1214–1225. doi: 10.1111/ppa.12051
Burke, D. J., Hoke, A. J., and Koch, J. (2020). The emergence of beech leaf disease in Ohio: Probing the plant microbiome in search of the cause. For. Pathol. 50, e12579. doi: 10.1111/efp.12579
Campos, J. D. S., Bogo, A., Sanhueza, R. M. V., Casa, R. T., Silva, F. N. D., Cunha, I. C. D., et al. (2017). European apple canker: morphophysiological variability and pathogenicity in isolates of Neonectria ditissima in southern Brazil. Ciência Rural. 47. doi: 10.1590/0103-8478cr20160288
Chahal, K., Gazis, R., Klingeman, W., Hadziabdic, D., Lambdin, P., Grant, J., et al. (2019). Assessment of alternative candidate subcortical insect vectors from walnut crowns in habitats quarantined for thousand cankers disease. Environ. Entomol. 48, 882–893. doi: 10.1093/ee/nvz064
Chahal, K., Gazis, R. O., Grant, J., Hadziabdic, D., Lambdin, P., Klingeman, W., et al. (2017). Preliminary assessment of insect-associated Geosmithia species in Tennessee. Phytopathology. 107, 113.
Daniels, D., Nix, K., Wadl, P., Vito, L., Wiggins, G., Windham, M., et al. (2016). Thousand cankers disease complex: a forest health issue that threatens Juglans species across the US. Forests. 7, 260. doi: 10.3390/f7110260
Dodd, R. S., Hüberli, D., Douhovnikoff, V., Harnik, T. Y., Afzal-Rafii, Z., and Garbelotto, M. (2005). Is variation in susceptibility to Phytophthora ramorum correlated with population genetic structure in coast live oak (Quercus agrifolia)? New Phytologist. 165, 203–214. doi: 10.1111/j.1469-8137.2004.01200.x
Elena, G., Garcia-Figueres, F., Reigada, S., and Luque, J. (2015). Intraspecific variation in Diplodia seriata isolates occurring on grapevines in Spain. Plant Pathol. 64, 680–689. doi: 10.1111/ppa.12296
Ewing, C. J., Slot, J., Benítez, M. S., Rosa, C., Malacrin,ò, A., Bennett, A., et al. (2021). The foliar microbiome suggests that fungal and bacterial agents may be involved in the Beech Leaf Disease Pathosystem. Phytobiomes J. 5, 335–349. doi: 10.1094/PBIOMES-12-20-0088-R
Félix, C., Pinto, G., Amaral, J., Fernandes, I., Alves, A., and Esteves, A. C. (2017). Strain-related pathogenicity in Diplodia corticola. For. Pathol. 47, e12366. doi: 10.1111/efp.12366
Gardes, M., and Bruns, T. D. (1993). ITS primers with enhanced specificity for basidiomycetes: application to the identification of mycorrhizae and rusts. Mol. Ecol. 2, 113–118. doi: 10.1111/j.1365-294X.1993.tb00005.x
Gazis, R., Poplawski, L., Klingeman, W., Boggess, S. L., Trigiano, R. N., Graves, A. D., et al. (2018). Mycobiota associated with insect galleries in walnut with thousand cankers disease reveals a potential natural enemy against Geosmithia morbida. Fungal Biology 122, 241–253. doi: 10.1016/j.funbio.2018.01.005
Grant, J. F., Windham, M. T., Haun, W. G., Wiggins, G. J., and Lambdin, P. L. (2011). Initial assessment of thousand cankers disease on black walnut, Juglans nigra, in eastern Tennessee. Forests. 2, 741–748. doi: 10.3390/f2030741
Griffin, G. J. (2015). Status of thousand cankers disease on eastern black walnut in the eastern United States at two locations over 3 years. For. Pathol. 45, 203–214. doi: 10.1111/efp.12154
Guest, D. (2007). Black pod: diverse pathogens with a global impact on cocoa yield. Phytopathology. 97, 1650–1653. doi: 10.1094/PHYTO-97-12-1650
Guo, Y., Olsen, R. T., Kramer, M., and Pooler, M. (2015). Effective bioassays for evaluating boxwood blight susceptibility using detached stem inoculations. HortScience. 50, 268–271. doi: 10.21273/HORTSCI.50.2.268
Hadziabdic, D., Vito, L. M., Windham, M. T., Pscheidt, J. W., Trigiano, R. N., and Kolarik, M. (2014). Genetic differentiation and spatial structure of Geosmithia morbida, the causal agent of thousand cankers disease in black walnut (Juglans nigra). Curr. Genet. 60, 75–87. doi: 10.1007/s00294-013-0414-x
Hansen, M. A., Bush, E., Day, E., Griffin, G., and Dart, N. (2011). Walnut Thousand Cankers Disease Alert. Blacksburg, Virginia: Virginia Cooperative Extension. Available online at: https://www.vdacs.virginia.gov/pdf/techpestalert.pdf.
Hefty, A. R., Aukema, B. H., Venette, R. C., Coggeshall, M. V., McKenna, J. R., and Seybold, S. J. (2018). Reproduction and potential range expansion of walnut twig beetle across the Juglandaceae. Biol. Invasions. 20, 2141–2155. doi: 10.1007/s10530-018-1692-5
Hishinuma, S. M., Dallara, P. L., Yaghmour, M. A., Zerillo, M. M., Parker, C. M., Roubtsova, T. V., et al. (2016). Wingnut (Juglandaceae) as a new generic host for Pityophthorus juglandis (Coleoptera: Curculionidae) and the thousand cankers disease pathogen, Geosmithia morbida (Ascomycota: Hypocreales). Can. Entomol. 148, 83–91. doi: 10.4039/tce.2015.37
Huang, Y. T., Kolarík, M., Kasson, M. T., and Hulcr, J. (2017). Two new Geosmithia species in G. pallida species complex from bark beetles in eastern USA. Mycologia. 109, 790–803. doi: 10.1080/00275514.2017.1410422
Huang, Y. T., Skelton, J., Johnson, A. J., Kolarík, M., and Hulcr, J. (2019). Geosmithia species in southeastern USA and their affinity to beetle vectors and tree hosts. Fungal Ecol. 39, 168–183. doi: 10.1016/j.funeco.2019.02.005
Hüberli, D., Tommerup, I. C., Colver, M. C., Colquhoun,C, I. J., and Hardy, G. E. S. J. (2002). Temperature and inoculation method influence disease phenotypes and mortality of Eucalyptus marginata clonal lines inoculated with Phytophthora cinnamomi. Australas. Plant Pathol. 31, 107–118. doi: 10.1071/AP01078
Hughes, M. A., Juzwik, J., Harrington, T., and Keith, L. (2020). Pathogenicity, symptom development and colonization of Metrosideros polymorpha by Ceratocystis lukuohia. Plant Dis. 104, 2233–2241. doi: 10.1094/PDIS-09-19-1905-RE
Jankowiak, R., and Bilański, P. (2018). Geosmithia species associated with fir-infesting beetles in Poland. Acta Mycologica. 53, 1115. doi: 10.5586/am.1115
Kohpina, S., Knight, R., and Stoddard, F. L. (2000). Evaluating faba beans for resistance to ascochyta blight using detached organs. Aust. J. Exp. Agric. 40, 707–713. doi: 10.1071/EA99168
Kolarik, M., Freeland, E., Utley, C., and Tisserat, N. (2011). Geosmithia morbida sp. nov., a new phytopathogenic species living in symbiosis with the walnut twig beetle (Pityophthorus juglandis) on Juglans in USA. Mycologia. 103, 325–332. doi: 10.3852/10-124
Kolarík, M., Hulcr, J., Tisserat, N., De Beer, W., Kostovčík, M., Kolaríkov,á, Z., et al. (2017). Geosmithia associated with bark beetles and woodborers in the western USA: taxonomic diversity and vector specificity. Mycologia. 109, 185–199. doi: 10.1080/00275514.2017.1303861
Kolarík, M., Kubátová, A., Hulcr, J., and PaŽoutová, S. (2008). Geosmithia fungi are highly diverse and consistent bark beetle associates: evidence from their community structure in temperate Europe. Microbial Ecol. 55, 65–80. doi: 10.1007/s00248-007-9251-0
Lynch, S. C., Wang, D. H., Mayorquin, J. S., Rugman-Jones, P. F., Stouthamer, R., and Eskalen, A. (2014). First report of Geosmithia pallida causing foamy bark canker, a new disease on coast live oak (Quercus agrifolia), in association with Pseudopityophthorus pubipennis in California. Plant Dis. 98, 1276–1276. doi: 10.1094/PDIS-03-14-0273-PDN
McKenna, J. R., and Coggeshall, M. V. (2018). The genetic improvement of black walnut for timber production. in Plant Breeding Reviews. Goldman, I. (ed). New Jersey: John Wiley and Sons, Inc. 41, 263–290. doi: 10.1002/9781119414735.ch6
Mohali, S., Slippers, B., and Wingfield, M. J. (2007). Identification of Botryosphaeriaceae from Eucalyptus, Acacia and Pinus in Venezuela. Fungal Div. 25, 103–125. Available online at: https://www.fungaldiversity.org/fdp/sfdp/25-7.pdf
Montecchio, L., and Faccoli, M. (2014). First record of thousand cankers disease Geosmithia morbida and walnut twig beetle Pityophthorus juglandis on Juglans nigra in Europe. Plant Dis. 98, 696. doi: 10.1094/PDIS-10-13-1027-PDN
Montecchio, L., Faccoli, M., Short, D. P. G., Fanchin, G., Geiser, D. M., and Kasson, M. T. (2015). First report of Fusarium solani phylogenetic species 25 associated with early stages of thousand cankers disease on Juglans nigra and Juglans regia in Italy. Plant Dis. 99, 1183–1183. doi: 10.1094/PDIS-01-15-0103-PDN
Moore, M., Juzwik, J., Miller, F., Roberts, L., and Ginzel, M. D. (2019). Detection of Geosmithia morbida on numerous insect species in four eastern states. Plant Health Prog. 20, 133–139. doi: 10.1094/PHP-02-19-0016-RS
Moricca, S., Bracalini, M., Benigno, A., Ginetti, B., Pelleri, F., and Panzavolta, T. (2019). Thousand cankers disease caused by Geosmithia morbida and its insect vector Pityophthorus juglandis first reported on Juglans nigra in Tuscany, Central Italy. Plant Dis. 103, 369–369. doi: 10.1094/PDIS-07-18-1256-PDN
Newton, L., Fowler, G., Neeley, A. D., Schall, R. A., and Takeuchi, Y. (2009). Pathway assessment: Geosmithia sp. and Pityophthorus juglandis Blackman movement from the western into the eastern United States. US Department of Agriculture, Animal and Plant Health Inspection Service. Available online at: http://thousandcankers.com/wp-content/uploads/2018/08/APHIS_Geosmithia_10_2009.pdf.
Oren, E., Klingeman, W., Gazis, R., Moulton, J., Lambdin, P., Coggeshall, M., et al. (2018). A novel molecular toolkit for rapid detection of the pathogen and primary vector of thousand cankers disease. PloS ONE. 13, e0185087. doi: 10.1371/journal.pone.0185087
Piškur, B., Pavlic, D., Slippers, B., Ogris, N., Maresi, G., Wingfield, M. J., et al. (2011). Diversity and pathogenicity of Botryosphaeriaceae on declining Ostrya carpinifolia in Slovenia and Italy following extreme weather conditions. Eur. J. For. Res. 130, 235–249. doi: 10.1007/s10342-010-0424-x
Randolph, K. C., Rose, A. K., Oswalt, C. M., and Brown, M. J. (2013). Status of black walnut (Juglans nigra L.) in the eastern United States in light of the discovery of thousand cankers disease. Castanea. 78, 2–14. doi: 10.2179/12-024
Rugman-Jones, P. F., Seybold, S. J., Graves, A. D., and Stouthamer, R. (2015). Phylogeography of the walnut twig beetle, Pityophthorus juglandis, the vector of thousand cankers disease in North American walnut trees. PLoS ONE. 10, e0118264. doi: 10.1371/journal.pone.0118264
Sacristán, S., and García-Arenal, F. (2008). The evolution of virulence and pathogenicity in plant pathogen populations. Mol. Plant Pathol. 9, 369–384. doi: 10.1111/j.1364-3703.2007.00460.x
Schneider, C. A., Rasband, W. S., and Eliceiri, K. W. (2012). NIH Image to ImageJ: 25 years of image analysis. Nat. Methods. 9, 671–675. doi: 10.1038/nmeth.2089
Schuelke, T. A., Wu, G., Westbrook, A., Woeste, K., Plachetzki, D. C., Broders, K., et al. (2017). Comparative genomics of pathogenic and nonpathogenic beetle-vectored fungi in the genus Geosmithia. Genome Biol. Evol. 9, 3312–3327. doi: 10.1093/gbe/evx242
Sessa, L., Abreo, E., Bettucci, L., and Lupo, S. (2017). Diversity and virulence of Diaporthe species associated with wood disease symptoms in deciduous fruit trees in Uruguay. Phytopathol. Mediterr. 56, 431–444.
Seybold, S. J., Klingeman, I. I. I. W. E, Hishinuma, S. M., Coleman, T. W., and Graves, A. D. (2019). Status and impact of walnut twig beetle in urban forest, orchard, and native forest ecosystems. J. For. 117, 152–163. doi: 10.1093/jofore/fvy081
Sitz, R. A., Luna, E. K., Caballero, J. I., Tisserat, N. A., Cranshaw, W. S., McKenna, J. R., et al. (2021). Eastern black walnut (Juglans nigra L.) originating from native range varies in their response to inoculation with Geosmithia morbida. Front. For. Glob. Change. 4, 627911. doi: 10.3389/ffgc.2021.627911
Sitz, R. A., Luna, E. K., Caballero, J. I., Tisserat, N. A., Cranshaw, W. S., and Stewart, J. E. (2017). Virulence of genetically distinct Geosmithia morbida isolates to black walnut and their response to coinoculation with Fusarium solani. Plant Dis. 101, 116–120. doi: 10.1094/PDIS-04-16-0535-RE
Smith, B. J. (1996). Detached stem assay to evaluate the severity of stem blight of rabbiteye blueberry (Vaccinium ashei). In VI International Symposium on Vaccinium Culture. 446, 457–464. doi: 10.17660/ActaHortic.1997.446.66
Stewart, P. J., Clark, J. R., and Fenn, P. (2005). Detached cane assay of resistance to botryosphaeria cane canker (Botryosphaeria dothidea) in eastern US blackberry genotypes. Int. J. Fruit Sci. 5, 57–64. doi: 10.1300/J492v05n04_07
Tisserat, N., Cranshaw, W., Leatherman, D., Utley, C., and Alexander, K. (2009). Black walnut mortality in Colorado caused by the walnut twig beetle and thousand cankers disease. Plant Health Prog. 1–10. doi: 10.1094/PHP-2009-0811-01-RS
Tisserat, N., Cranshaw, W., Putnam, M. L., Pscheidt, J., Leslie, C. A., Murray, M., et al. (2011). Thousand cankers disease is widespread in black walnut in the western United States. Plant Health Prog. 1–4. doi: 10.1094/PHP-2011-0630-01-BR
Tran, T. T., Li, H., Nguyen, D. Q., Sivasithamparam, K., Jones, M. G. K., and Wylie, S. J. (2020). Comparisons between genetic diversity, virulence and colony morphology of Monilinia fructicola and Monilinia laxa isolates. J Plant Pathol. 1–9. doi: 10.1007/s42161-020-00498-2
Utley, C., Nguyen, T., Roubtsova, T., Coggeshall, M., Ford, T. M., Grauke, L. J., et al. (2013). Susceptibility of walnut and hickory species to Geosmithia morbida. Plant Dis. 97, 601–607. doi: 10.1094/PDIS-07-12-0636-RE
Wagner, S., Kaminski, K., and Werres, S. (2006). “Inoculation trials with Phytophthora ramorum on Moorland species”, in Second Science Symposium: The State of Our Knowledge (p. 520). Gen. Tech. Rep. PSW-GTR-196. Albany, CA: Pacific Southwest Research Station, Forest Service, U.S. Department of Agriculture. doi: 10.2737/PSW-GTR-196
White, T. J., Bruns, T., Lee, S. J. W. T., and Taylor, J. (1990). Amplification and direct sequencing of fungal ribosomal RNA genes for phylogenetics. PCR Protocols: A Guide to Methods and Applications. 18, 315–322. doi: 10.1016/B978-0-12-372180-8.50042-1
Keywords: cankers, Geosmithia morbida, pathogenicity, thousand cankers disease, virulence, Pityophthorus juglandis, walnut twig beetle, Juglans nigra
Citation: Chahal K, Gazis R, Klingeman W, Lambdin P, Grant J, Windham M and Hadziabdic D (2022) Differential Virulence Among Geosmithia morbida Isolates Collected Across the United States Occurrence Range of Thousand Cankers Disease. Front. For. Glob. Change 5:726388. doi: 10.3389/ffgc.2022.726388
Received: 16 June 2021; Accepted: 02 March 2022;
Published: 31 March 2022.
Edited by:
Kimberly Wallin, North Dakota State University, United StatesReviewed by:
Braham Dhillon, University of Florida, United StatesIsabel Alvarez Munck, United States Forest Service (USDA), United States
Pierluigi Bonello, The Ohio State University, United States
Copyright © 2022 Chahal, Gazis, Klingeman, Lambdin, Grant, Windham and Hadziabdic. This is an open-access article distributed under the terms of the Creative Commons Attribution License (CC BY). The use, distribution or reproduction in other forums is permitted, provided the original author(s) and the copyright owner(s) are credited and that the original publication in this journal is cited, in accordance with accepted academic practice. No use, distribution or reproduction is permitted which does not comply with these terms.
*Correspondence: Denita Hadziabdic, ZGhhZHppYWImI3gwMDA0MDt1dGsuZWR1
†Present Address: Karandeep Chahal, Department of Plant, Soil, and Microbial Sciences, Michigan State University, East Lansing, MI, United States