- 1DRACONES Research Group, Department of Engineering and Agricultural Sciences, School of Agricultural and Forestry Engineering, University of León, León, Spain
- 2Department of Organisms and Systems Biology, Polytechnic School of Mieres, University of Oviedo, Mieres, Spain
- 3Unit for Sustainable Forest and Environmental Management, University of Santiago de Compostela, Lugo, Spain
Introduction: The Asian chestnut gall wasp (Dryocosmus kuriphilus) is a major pest of chestnut trees worldwide, seriously affecting chestnut cultivation. Information concerning the effects of gall wasp attack on diameter growth of chestnut trees is currently scarce and limited to coppice stands and to use of the growth of a non-target control species (unaffected by the pest) for reference purposes. The effects of the pest on widely-spaced plantations (grown at a much lower density than chestnut coppices) and the use of explicitly-observed annual infestation rate data remain to be explored.
Methods: In the present study, we analyzed the impact of the chestnut gall wasp on the diameter growth of chestnut trees, using data from 16 experimental plots established in widely-spaced plantations located in good quality sites. Two of the plots are in plantations where a susceptible hybrid chestnut clone and a chestnut clone resistant to the gall wasp coexist, whereas the remaining 14 plots are in Castanea sativa plantations where the level of gall wasp infestation varies across trees and years. The plots were surveyed to determine the diameter growth of the trees and the level of infestation during 5 years (2017–2021).
Results: The infestation level corresponding to the theoretical damage threshold was surpassed inmost plots during the study period. Nevertheless, there were no differences in the growth of attacked and unaffected plants in the two plots planted with hybrid clones with contrasting susceptibility to the gall wasp. The attack had a modest effect in C. sativa plots, with a mean reduction in annual basal area increment of 9.9%.
Discussion: These findings apparently contradict previous reports of a marked reduction in radial growth of chestnut coppice trees due to gall wasp attack. The difference in findings may be related to increased compensation for herbivory with increasing levels of resources (especially light) in the plantations under study, which were less dense than previously studied stands. The study outcomes add to existing knowledge on the impact of chestnut gall wasp on wood formation and may have implications regarding planting site recommendations and subsequent stand management.
1. Introduction
In Europe almost 1.8 million hectares of chestnut forest, which corresponds to two-thirds of the total chestnut-growing area (Manetti et al., 2020), is devoted to timber production. Most of this forest (around 80%) is managed using the coppice system, characterized by relatively short rotations and sparse silvicultural treatments (e.g., thinning). In Spain, the existing 100,000 ha of stands managed for timber production is divided approximately equally between coppice and high forest (Conedera et al., 2004).
In NW Spain, numerous chestnut tree plantations have been established in the last 20 years. Moreover, new plantations are currently being established, with more than 400,000 chestnut trees presently produced annually (Xunta de Galicia, 2020). Approximately half of these trees are sweet chestnut (Castanea sativa Mill.) and the other half are Euro-Japanese hybrid clones. Clonal forestry techniques have been applied to chestnut plantations in order to produce plants that are resistant to ink disease and also to generate certain productive traits such as vigorous growth, stem form and apical dominance (Fernández-López, 2011). The main objective of the plantations is usually to produce high quality round-wood at intermediate rotation ages, so that plantation density is usually low (280–625 trees per hectare).
Dryocosmus kuriphilus Yasumatsu (Hymenoptera, Cynipidae) is one of the major key insect pests in chestnut orchards and forests (e.g., Fernandes et al., 2022). This pest, commonly known as the Asian chestnut gall wasp, is native to China and can affect species of the genus Castanea and hybrid clones. Nevertheless, a wide range of variability among clones regarding the level of infestation by D. kuriphilus has been reported, suggesting a genotype-dependent variation in susceptibility (Sartor et al., 2015; Míguez-Soto et al., 2018; Castedo-Dorado et al., 2021; Lombardero et al., 2022). The same applies to the European populations of C. sativa, suggesting that the differences are due to specific features of individual trees within the populations (Bombi et al., 2018).
D. kuriphilus galling activity prevents or inhibits the development of normal shoots and leads to the production of abnormal plant structures (Maltoni et al., 2012). In the long term, the decreased capacity of developing buds can result in malformation of the branch architecture and general deterioration of the crown (Gehring et al., 2018a). As a result of the reduction in the leaf area of the affected trees, a gradual loss of the photosynthetic biomass and a decrease in tree vigor are expected to occur (Kato and Hijii, 1997). Additionally, the replacement of plant tissue damaged by insects requires plant resources, influencing plant allocation patterns, which in turn can affect radial growth (Waring, 1987; Cipollini et al., 2014).
Different studies have quantified the effect of the gall wasp attack on the yield of chestnut products. The gall wasp can reduce nut production by up to 80% in heavily infested trees (Battisti et al., 2014) and can also reduce the chestnut component of honey (Gehring et al., 2018b). Regarding timber yield, the pest has been found to cause a marked reduction in radial growth, although studies of the impact of the gall wasp are scarce (but see Mariotti et al., 2013; Marcolin et al., 2021).
These studies (op. cit.) focused on coppice stands, in which stand density is much higher (stem density >600 trees ha−1, with some plots surpassing 3,000 trees ha−1) than in chestnut plantations. As tree responses to herbivores are mediated by resource conditions (e.g., light, water and nutrients) (e.g., Maschinski and Whitham, 1989; Hawkes and Sullivan, 2001), it can be hypothesized that widely spaced high forest stands, where there is less competition for these resources, will be less impacted by the pest. The quality of the site, related to nutrient availability, may also play a role in compensating for herbivory (Coley et al., 1985; Maschinski and Whitham, 1989; Lombardero et al., 2016).
Furthermore, Marcolin et al. (2021) indirectly assessed both the gall wasp outbreak evolution and the effect of the pest on tree growth. In order to assess the former, these researchers used the time since the gall wasp and its specific parasitoid (Torymus sinensis Kamijo) arrived at each site, whereas for the latter they used the growth of a non-target control species (unaffected by the pest) as a baseline reference to account for the effect of the local environment. Therefore, the effects of the explicitly observed annual infestation rate relative to healthy reference chestnut trees remain to be explored.
Here, we used data from manual band dendrometers to determine the effects of Asian chestnut gall wasp on annual basal area increment of chestnut trees from low density plantations established in good quality sites. We sought to evaluate the following hypotheses: (i) the insect activity has less impact in widely spaced high forest stands (i.e. under conditions of reduced intraspecific competition), opposite to the high competition in coppice stands, and (ii) the site quality may also play a role in compensating for the pest-induced damage.
2. Materials and methods
2.1. Study area
Sixteen study plots were established in spring 2017 in an inland area of Galicia (NW Spain) to monitor the damage caused by D. kuriphilus to chestnut trees. The plots were relatively close to each other: the mean distance between each plot was 15 km and the maximum distance between the two most widely separated plots was 55 km. The climatic conditions were therefore similar in all plots (Figure 1). The climate diagrams for the years under study for the weather station of Campus de Lugo, in the vicinity of one of the study plots (see Figure 1; data available at https://www.meteogalicia.gal/observacion/estacionshistorico/historico.action?idEst=10053) is shown in Supplementary Figure 1.
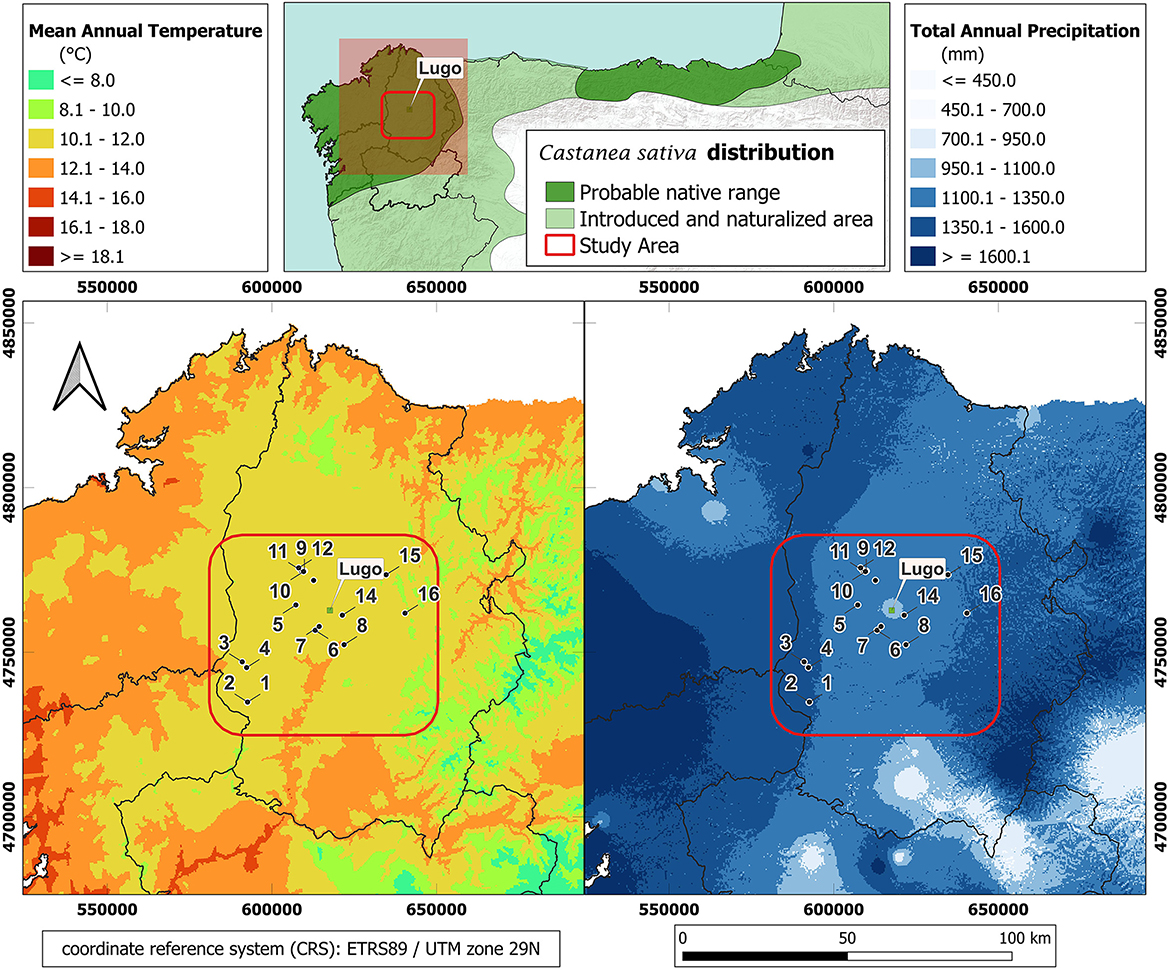
Figure 1. Spatial distribution of the 16 study plots superimposed on the mean values of mean annual temperature and total annual precipitation. Source: Ninyerola et al. (2005).
The plots were established in plantations for wood production or both wood and fruit production, with a wide plant spacing ranging from 4 × 4 to 8 × 8 m. There were no grafted trees and no treatments for fruit production (e.g., irrigation, fertilization or crown formation pruning) had been carried out. All of the plots were established in private chestnut plantations, and the forest owners provided some information about the plantation history. The plantations were carried out since the turn of the century and were established on former agricultural land.
The presence of D. kuriphilus was first reported in the center of the study area (the surroundings of the city of Lugo) in 2014 (Pérez-Otero and Mansilla, 2014). We estimate that the wasp arrived in the plots in 2015 and 2016, although this is not certain. In spring 2017, D. kuriphilus-induced galls were present in all 16 of the study plots.
In two closely located plots, “Esperante” and “O Corgo” (numbers 7 and 8 in Figure 1), we initially found trees with numerous galls and also trees without any galls. The owners reported that they had planted two hybrid chestnut clones, although they did not know which specific clones were used. Genetic analysis (identification of molecular markers) of two trees of each class, carried out by Functional Genomics and Sequencing Platform (RIAIDT) of the University of Santiago de Compostela, confirmed that the trees belong to different hybrid clones: “324” and “502” in “Esperante” and “324” and “HS” in “O Corgo.” No galls were found in trees of clone “324,” which is known to be resistant to the gall wasp (Míguez-Soto et al., 2018; Castedo-Dorado et al., 2021; Lombardero et al., 2022), whereas galls were present in trees of both “502” and “HS” clones. In the remaining 14 plots, we found that the level of gall wasp attack varied across the trees. The planted material from these plots was not analyzed for identification purposes, because the owners reported that native sweet chestnut trees (C. sativa) had been planted.
Field releases of Torymus sinensis, the major biocontrol agent used against the chestnut gall wasp, have been performed since 2015 in the study area, with release of small quantities in the first 3 years (2,000, 67,200 and 151,200 individuals for the whole Galicia region for years 2015, 2016 and 2017, respectively) (Pérez-Otero et al., 2017). The percentage of parasitism by T. sinensis in Galicia was low until 2019, probably due to the low population density of the releases (Nieves-Aldrey et al., 2019). More successful establishment was observed in 2020 and 2021, when the percentage of parasitism in the study area reached 25% in some locations (Xunta de Galicia, 2021).
2.2. Field measurements
We assessed a number of trees in each plot between spring of 2017 and November 2021. In the “Esperante” and “O Corgo” plots, 10 trees of each clone were evaluated. In the remaining 14 plots, we selected 6 trees with similar diameter at breast height and different levels of infestation at the beginning of the study period: 3 trees with a lower level and 3 trees with a higher level. The level of infestation varied notably in the following years in some trees, so that the initial classification of trees could not be used in the analysis. In some plots, the data on some trees had to be discarded because the trees had died or were severely damaged by other factors during the study period.
When the study plots were established, the diameter at breast height (d) of each tree was measured with a caliper, and total tree height (h) was measured with a digital hypsometer. In addition, from July 2017 onwards, girth at breast height growth was recorded via permanent measuring tapes marked with Vernier scales of 0.1 mm resolution (Type D1 Permanent Tree Girth, UMS, Germany). In the “Esperante” and “O Corgo” plots, the girth tapes were examined monthly between the beginning of April and the beginning of November. In the remaining plots, the tapes were revisited annually, at the end of the growing period (November). To avoid diurnal biases due to high temperatures, in summer all measurements were made early in the morning. We opted to use permanent bands instead of a caliper to characterize secondary tree growth to prevent errors in identifying the exact location of the measurement point. Moreover, the owners did not allow us take stem cores from the trees, to prevent damaging the basal log (which is the most valuable from an economic point of view) or causing wounds that could foster chestnut blight infections [Cryphonectria parasitica (Murrill) Barr]. Girth growth data from year 2017 was not used for analysis as we installed the band dendrometers in July and therefore we did not record the growth throughout all the growing season.
To assess secondary growth, girth increment data were transformed to diameter increments assuming a cylindrical stem shape. Annual basal area increment (BAI) was calculated from tree diameters as the difference between consecutive basal areas (BA = π/4·d2); bark growth was assumed to be null during the 4-year interval. We used BAI instead of diameter growth because it is a more biologically meaningful descriptor of growth trends (Biondi and Qeadan, 2008). As we were dealing with only four stem growth intervals, age-detrending individual tree diameter measurement series for removing non-climatic trends was not considered.
From initial tree measurements, we estimated the dominant height of each plot (H) as the average height of the thickest trees in the plot. Subsequently, and using information on stand age provided by the owners, we estimated the site index (SI) as the dominant height of the plot at a reference age of 45 years, according to the site index curves reported by Patrício and Nunes (2017) for high chestnut forest stands in the nearby region of Northern Portugal. In addition, we used the number of stems per hectare (N) and H to calculate the relative spacing (RS), which is a measure of stand density. The relative spacing index was calculated as the ratio of the average distance between trees and the dominant height, expressed as a percentage. Assuming a square spacing, the index can be calculated as follows: RS (%) = . As dominant height varies over years, RS was estimated for 2018–2021 by projecting annually H of each plot through the site index curves of Patrício and Nunes (2017). The main stand features of the 16 plots are shown in Table 1.
The level of infestation by the gall wasp on each tree was evaluated in July in four of the 5 years under study (2017, 2018, 2020, and 2021) (the trees were not able to be examined in 2019). In 2017 and 2018, the infestation level was evaluated in two different ways. First, the same observer (MJL) visually estimated the percentage of crown affected by D. kuriphilus at 10% intervals and then assigned a severity index (from 0 to 5) based on the level of damage (number of galls). In the largest trees, the galls were observed with the aid of binoculars. The level of attack (LoA) was calculated by dividing the percentage of crown affected by D. kuriphilus by 100 and multiplying the value thus obtained by the severity index. Subsequently, four small branches were randomly selected in each tree, although where possible each faced a different direction within the tree crown. We used 4 m telescopic pruning shears to cut the branches, thus allowing access to branches that were 6–7 m above the ground. Branches in the sunlit tree crown were thus able to be sampled in all cases. In each branch, we located and examined the shoot that had grown in the previous growing season. We counted both the number of newly developed buds (i.e., developed in the current growing season) and the number of galls on these buds along the length of the old shoot. We used these data to calculate two commonly used infestation indices for each tree: (i) the ratio between the number of galls and the number of buds in 2019 (GpB; e.g., Sartor et al., 2009, 2015); and (ii) the ratio between the number of attacked buds (i.e. buds with the presence of galls) and the total number of buds (AB; Kotobuki et al., 1985). LoA was strongly correlated with both GpB and AB (Supplementary Figure 2), and therefore in 2020 and 2021 we chose to assess the level of infestation by using only the latter two indices. During the last diameter growth survey, in November 2021, we remeasured tree height in the “Esperante” and “O Corgo” plots in order to estimate the primary tree growth during the study period.
2.3. Statistical analysis
The annual BAI measurements were square-root transformed prior to analysis to improve normality and homoscedasticity. As the growth data series is short (4 years) and only 2 plots included unaffected trees, the relationship between climate variables and diameter growth could not be established. The Standardized Precipitation Index (SPEI) (Vicente-Serrano et al., 2010) was considered a climate covariate to assess the impact of climate on the diameter growth in all annual BAI models. Given the sensitivity of chestnut to summer climate conditions, SPEI was calculated over a period of 3 months and averaged across the summer months (June, July, August; SPEIJJlA) (Marcolin et al., 2021). We assumed that the hybrid clones and C. sativa will behave the same way in response to climate because no specific information is available on the topic and because the same growth pattern was observed in young chestnut plantations throughout the region using hybrid clones and C. sativa (Álvarez-Álvarez et al., 2010).
ANOVA was applied to the data from the “Esperante” and “O Corgo” plots (which include both attacked and unaffected trees) to test the influence of the gall wasp attack on annual BAI and periodic height increment. We considered the factors attack and plot, and their interaction, as explanatory variables. In order to take into account the size of the tree, we also included the value of the basal area of each tree (BA) at the beginning of the current growth season (for the annual BAI model) and the value of tree height at the time of the first measurement (h_2017, for periodic height increment model) as covariates.
Annual BAI data from the 14 plots where C. sativa was present (which include trees with different levels of infestation) were analyzed using a linear mixed model. We included SPEIJJlA, individual-tree basal area (BA), site index (SI), relative spacing (RS) and the infestation level (given by GpB or AB) as fixed factors. The plot was included in the model as a random effect to account for the correlated BAI data from the same plot. A random effect for individual trees could not be included because of the short length of the growth series. The “lme” function of the R package “nlme” (Pinheiro et al., 2022) was used to fit the linear mixed model using the restricted maximum likelihood (REML) method.
The variable indicating the infestation level (GpB or AB) was selected by comparing the value of the Akaike information criterion (AIC) of the fitted models using each variable independently. The configuration of the random effect (random intercept or random intercept and slope) was determined following the procedure described by Zuur et al. (2009). Model validity was assessed by plotting the residuals against fitted values and the predictive variables and the quantile-quantile distribution of the residuals. Both marginal and conditional R2 were used as goodness-of-fit metrics, representing the proportion of the variance explained by the fixed effects, and by the combined fixed and random effects (i.e., the entire model), respectively (Nakagawa and Schielzeth, 2013). The relative importance of each fixed-effect predictor was calculated using the “relaimpo” package (Grömping, 2006). All analyses were carried out with R statistical software (R Core Team, 2022).
3. Results
The D. kuriphilus infestation generally increased between 2017 and 2018, peaked in 2020 and then decreased in 2021 (Figure 2 for GpB and AB; Supplementary Figure 3 for LoA). In 2017, susceptible hybrid clones in the “Esperante” and “O Corgo” plots already displayed high levels of infestation, possibly due to earlier arrival of the wasp. The epidemic peak corresponding to the 2020 growing season was common for all plots except those mentioned above and the “Castroverde” and “Masoucos” plots.
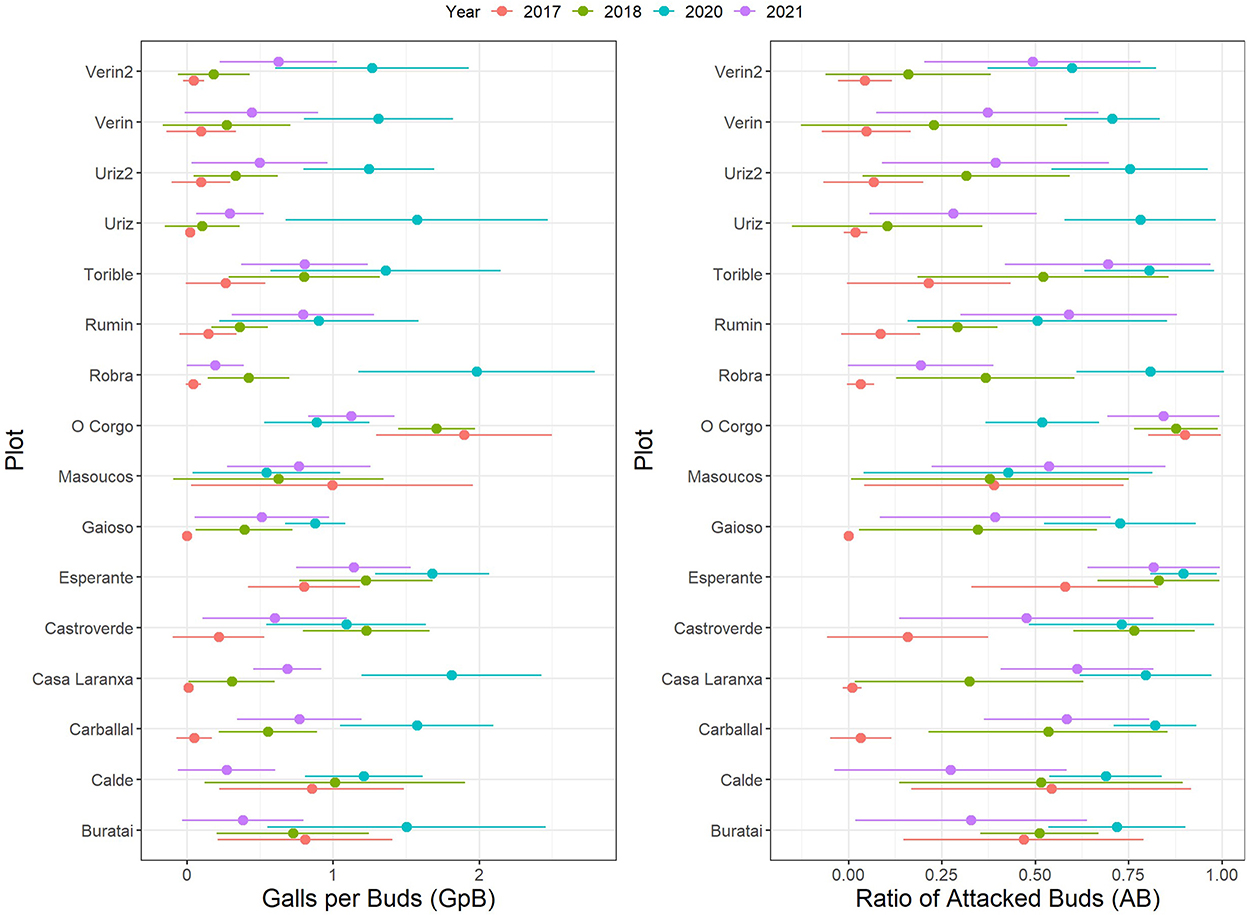
Figure 2. Changes in GpB and AB infestation indices in the 16 study plots throughout the study period. Note that in the “Esperante” and “O Corgo” plots, the infestation level corresponds to hybrid clone trees susceptible to the gall wasp.
In the two plots in which intensive monitoring of diameter growth was conducted (“Esperante” and “O Corgo”), the growth peak corresponds to the months of June, July and August, both for the attacked and unaffected trees (Supplementary Figure 4). For these plots, there was no significant difference in the mean of individual-tree basal area at plot establishment [ANOVA, F(1, 18) = 0.20, p = 0.65 and F(1, 18) = 0.37, p = 0.55]; nevertheless, in the “Esperante” plot, mean tree height was significantly higher for attacked trees [ANOVA, F(1, 18) = 20.3 p = 0.0003] (Supplementary Figure 5).
The mean annual BAI was around 60 and 40 cm2 in “Esperante” and “O Corgo,” respectively, for the 4 years evaluated, with a generally lower mean value for the attacked trees (Figure 3A). The ANOVA revealed that the individual-tree basal area at the beginning of the growth period (BA), the plot and SPEIJJlA were highly statistically significant variables (p < 0.01) for explaining the annual BAI. The effect of the attack was not statistically significant (p = 0.07), and the effect of the interaction between the plot and the attack was modest (p = 0.04) (Table 2).
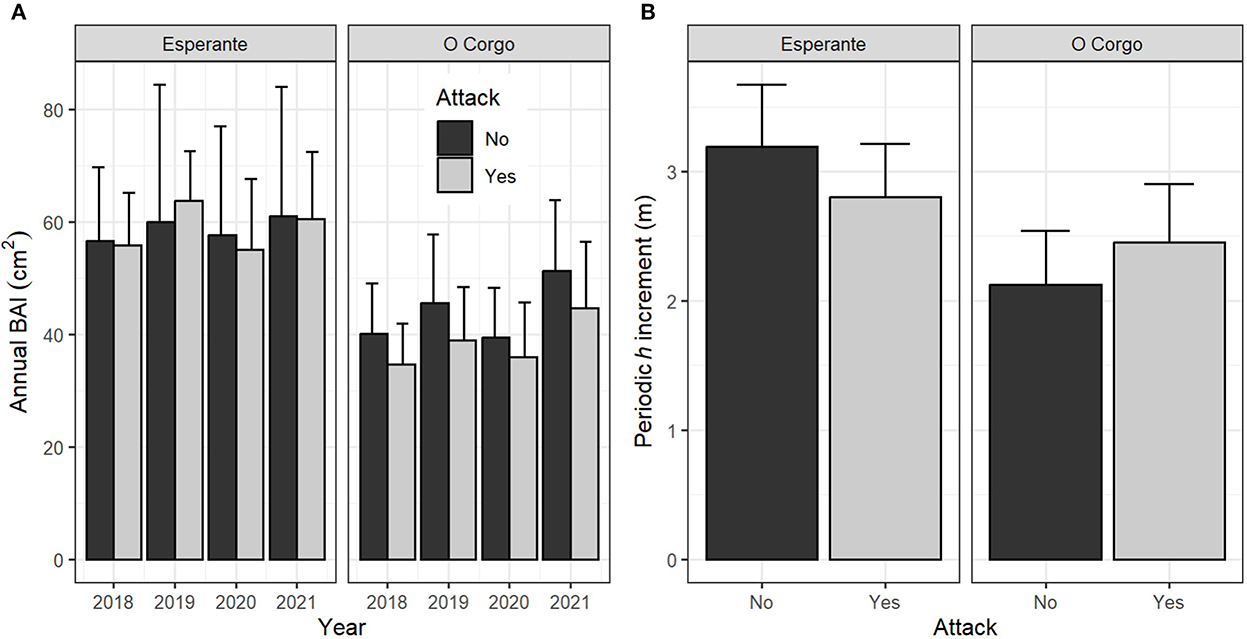
Figure 3. Bar plot with error bars (SE) for (A) annual BAI, and for (B) the periodic height increment (4-year period) for the two plots that include both attacked and unaffected trees.
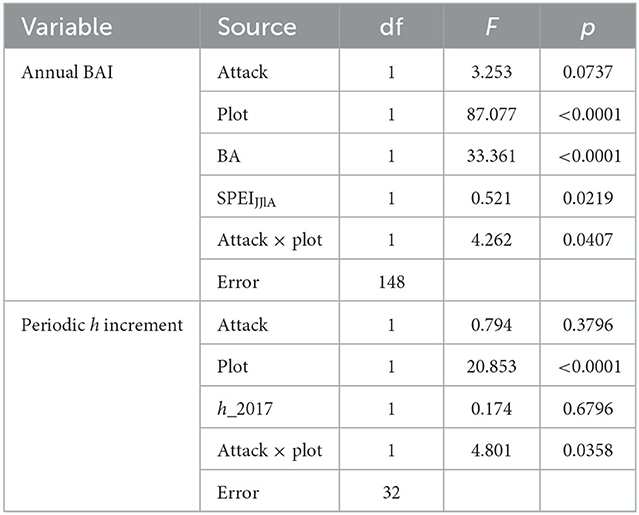
Table 2. ANOVA results testing for patterns in annual BAI and periodic height increment for the two plots that include both attacked and unaffected trees.
The trend in periodic height increment varied between plots: a higher mean height growth of attacked trees was observed in the “O Corgo” plot, whereas the opposite was observed in the “Esperante” plot (Figure 3B). The ANOVA results confirmed that the plot was statistically significant, but not the initial height (h_2017) nor the attack factor in isolation or the plot × attack interaction (Table 2).
For the fourteen plots where C. sativa was present, mean annual BAI was lowest in 2020 and highest in 2021, although with a high level of variability (Figure 4A). There appeared to be slight decrease in annual BAI when the infestation level increased (as indicated by the ratio of attacked buds -AB), although the relationship was weak (Figure 4B).
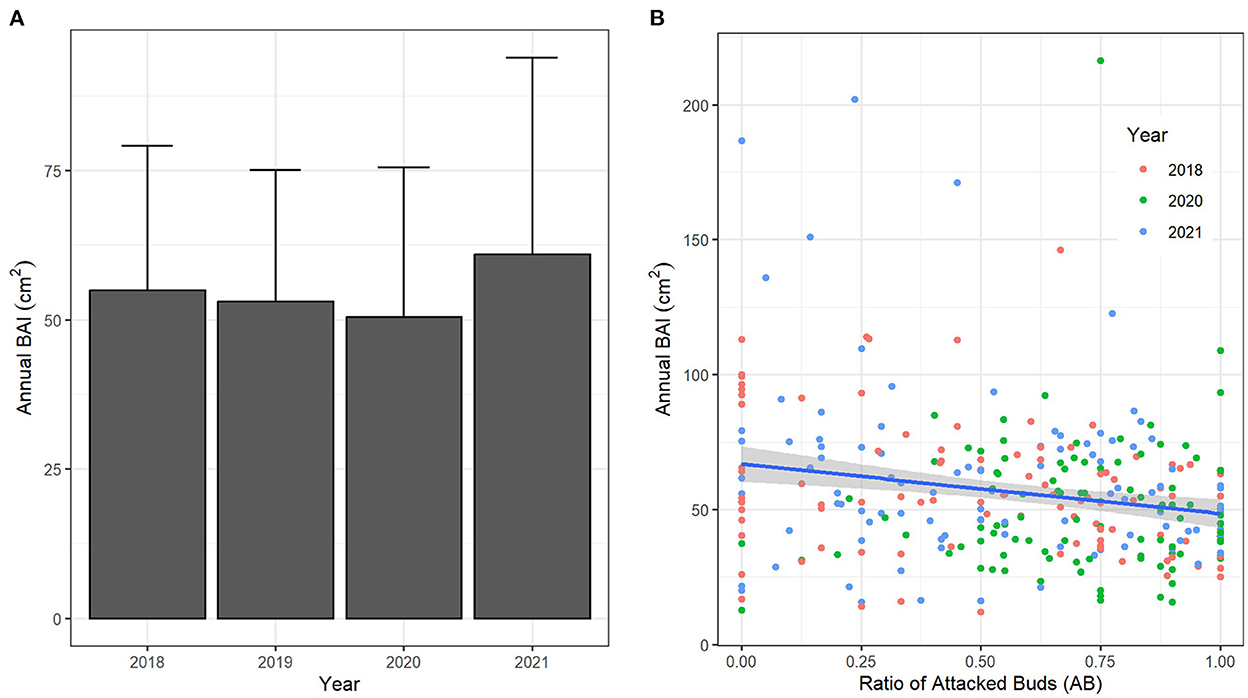
Figure 4. (A) Bar plot with error bars (SE) for annual BAI for the 14 plots where C. sativa was present and trees had different levels of infestation. (B) Graphical relationship between the annual BAI and the ratio of attacked buds (AB) for each tree. The 95% confidence level interval for predictions from a linear model is indicated in gray.
The ratio of attacked buds (AB) yielded a lower AIC value than the number of galls per bud (GpB) in the linear mixed effect model explaining the annual BAI and was therefore used in subsequent modeling. According to the results of the mixed model, only the Standardized Precipitation Evapotranspiration Index averaged over the three summer months (SPEIJJlA), the individual tree basal area at the beginning of the growing period (BA) and the relative spacing (RS) highly statistically significantly explained the annual BAI. The effect of the infestation level (AB) was modest (p = 0.030), and the negative sign of the parameter estimates for this variable is logical (Table 3). These results are consistent with the relative importance of the variables: BA was by far the relatively most important variable, followed by the RS and SPEIJJlA. The relative importance of the infestation level, given by AB, was modest, only 5.83% (Table 3). According to the results of the mixed model, the average reduction in BAI for all the plots was −9.86% [95% CI (−9.10, −10.63%)], with mean losses of the single plot spanning from −6.06% in “Uriz” [−3.45, −8.68%] to −13.42% [−11.91%, −14.93%] in “Castroverde.”
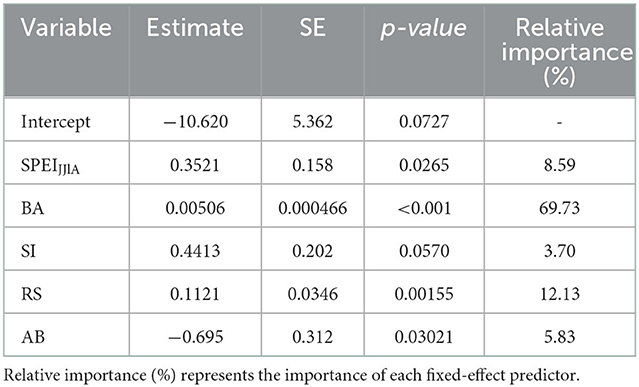
Table 3. Parameter estimates and the corresponding p-values of the linear mixed-effects models fitted to annual BAI.
The model provided a good fit to the data, as indicated by the fact that the residuals did not depart significantly from normality and the relatively high proportion of variance explained (marginal R2 = 0.468; conditional R2 = 0.670).
4. Discussion
We assessed the impact of D. kuriphilus on the growth of chestnut trees in two types of plots managed as high forest. Two of the plots corresponded to hybrid clone trees that were either attacked or unaffected by the gall wasp, while in the other 14 plots trees were affected by variable levels of infestation. For both type of plots, Standardized Precipitation Evapotranspiration Index averaged over June, July and August, and individual tree basal area at the beginning of the growth period were statistically significant variables influencing the annual BAI. The importance of temperature extremes and total precipitation in summer months on secondary growth confirms previous findings in sweet chestnut studies (Rubio et al., 1997; Fonti and García-González, 2004; Álvarez-Álvarez et al., 2010; Camarero et al., 2018; Marcolin et al., 2021). On the other hand, the importance of the tree size (i.e., BA) in explaining BAI has long been established (e.g., Monserud and Sterba, 1996; Hasenauer, 2006; Burkhart and Tomé, 2012; Vospernik, 2021).
In assessing tree responses to stressors such as herbivores, trees that are assumed to have no damage or trees with assumed optimal growth can be used for reference purposes (Dobbertin, 2005). At a local level, the scarcity of healthy C. sativa trees that are not affected by gall wasp attack makes it difficult to find specimens that can be used as reference trees. In the present study, two plots included trees of a hybrid clone that have not been attacked, which are potentially suitable for use as reference trees to assess the effect of the pest on growth. We did not find any evidence of the effect of the attack and only a modest effect of the attack × plot interaction (Table 2). The weak significance of this interaction suggests that the attack had slightly different effects on growth in the two plots. We acknowledge that these results could be partly mediated by the genotype, although the average basal areas of trees from each pair of clones within each plot at the beginning of our study were not significantly different (Supplementary Figure 5). The same rationale applies to periodic height increment, where the attack × plot interaction was weakly significant. It has been reported that genotype can influence the vigor and apical dominance of the hybrid clones under study (Fernández-López et al., 2009), thus potentially leading to a different growth pattern of the clones during the study period.
Regarding the results from the 14 C. sativa plots, the goodness of fit of the fitted linear mixed model is considered appropriate, taking into account that the fixed factors explained almost 50% of the existing variability, which reached almost 70% when random effects were considered. The model highlights the importance of the relative spacing index (RS) and the low significance of the site index (SI) in explaining annual BAI. The positive sign of the parameter estimate for RS reveals that a higher annual BAI is expected at lower stand densities (i.e., higher RS value, less tree competition). These results are consistent with the findings indicating that secondary growth is enhanced in trees in low density stands (e.g., Burkhart and Tomé, 2012). The absence of significance of SI in our study could be masked by the narrow range of site indices among the plots analyzed. Site indices in the study plots varied in a relatively narrow range (22–28 m) (Table 1), within the range of 14 to 26 m for which the site index curves have been developed for high forests in the NW Iberian Peninsula (Patrício and Nunes, 2017).
Previous studies have shown that repeated attack by the chestnut gall wasp affects the secondary growth of chestnut coppice trees (Mariotti et al., 2013; Marcolin et al., 2021), with an average annual BAI reduction of 60% during the epidemic (Marcolin et al., 2021). By contrast, the results of the present study indicate that the secondary growth of chestnut trees under the conditions of the study plots was scarcely affected by the pest (only an average 9.9% decrease of annual BAI was observed). Other authors have highlighted the resilience of chestnut trees in response to isolated events of damage by herbivores (e.g., Camarero et al., 2018). Our findings seem to confirm the resilience of chestnut trees even beyond 5–6 years of attacks and when the infestation level corresponding to the theoretical damage threshold is surpassed. This threshold was established by Gyoutoku and Uemura (1985) as 30% of AB and by 0.3 GpB by Gehring et al. (2018c) and was clearly surpassed in most plots during the study period.
The present findings are consistent with the continuum of responses hypothesis (Maschinski and Whitham, 1989), which assumes that the probability of compensation for herbivory decreases as competition with neighbors (mainly for light and water) increases and nutrient availability decreases (e.g., Björkman et al., 2000; Guillet and Bergström, 2006; Helbig et al., 2021). As chestnut is considered poorly competitive and light-demanding (Covone and Gratani, 2006; Conedera et al., 2021), the different conditions of competition for light resources may mainly explain the apparently contradictory findings of the present study and of the studies by Mariotti et al. (2013) and Marcolin et al. (2021).
Wood formation in chestnut trees has not been widely studied (but see Cufar et al., 2011; Marini et al., 2019). Nevertheless, it has been established that the development of earlywood vessels begins some 2–3 weeks before the development of leaves, as in other ring-porous species (Sass-Klaassen et al., 2011; González-González et al., 2014). This is consistent with the monthly diameter measurements in our two experimental plots, in which we recorded diameter growth even in March and April (see Supplementary Figure 4), although bud break does not occur in the study area until the beginning of May. Earlywood represents around a quarter of xylem ring width (Cufar et al., 2011) and its development draws on reserves accumulated in the previous growing season (Ermich, 1959). Formation of latewood, which represents a large part of chestnut tree xylem rings, starts in June and continues in the summer months, and is associated with summer precipitation (Cufar et al., 2011). Therefore, chestnut trees may compensate for growth by increasing the photosynthesis rate in these months. As photosynthesis is enhanced in sunlit parts of the crown (sunlit leaves) (Proietti et al., 2000), in widely spaced trees less of the crown will be shaded and photosynthetic activity will therefore be higher, increasing the plant's capacity to grow by increasing carbohydrate production (e.g., Thomson et al., 2003), and subsequently masking the effect of pest attack. The present results could also partly explain the findings reported by Marcolin et al. (2021), who found that the gall wasp attack had higher relative importance in reducing BAI in young coppices (denser) than in old coppices (less dense).
Regarding other limiting resources (e.g., nutrients, water), all of our plots were established on former agricultural land, in which soil and nutrients do not usually restrict growing chestnut (Álvarez-Álvarez et al., 2010). This is reflected in the high site indices in our study plots, relative to those in the NW Iberian Peninsula (Patrício and Nunes, 2017). Finally, competition can reduce water availability through increased stand transpiration and rainfall interception (Bréda et al., 2006; Inagaki et al., 2008; Slodičák et al., 2011), worsening plant water status and therefore tending to restrain compensatory growth (McNaughton et al., 1983).
In addition to the availability of resources, we recognize that other factors related to the differences in the infestation level and the combined effect of the attack of the gall wasp with other phytosanitary problems may also help to explain the differences between our results and those obtained in the above-mentioned studies. Although Marcolin et al. (2021) did not provide information on gall wasp infestation level in each plot, it seemed to be higher than in the present study. Thus, at the peak of the outbreak these authors reported a systematic, gradual activation of dormant buds in an attempt to restore the lost crown biomass and, accordingly, large number of suckers. Taking into account that these stress-induced reactions were not apparent in our trees, it is therefore reasonable to argue that our study plots suffered a comparatively lower level of infestation (Gehring et al., 2018a). On the other hand, although chestnut blight disease [Cryphonectria parasitica (Murrill) Barr] was present in these plots, we avoided selecting trees with blight symptoms in order to separate the effects of the two stressors. D. kuriphilus infestation occurring in conjunction with other stressors such as chestnut blight can result in additive negative effects on tree growth (Meyer et al., 2015; Schuldt et al., 2017; Rigling and Prospero, 2018). Alternatively, after intense wasp attack, if the vitality of chestnut trees decreases, the trees may become more vulnerable to pathogens, which in turn weaken their competitive fitness (Ugolini et al., 2014).
In summary, in widely spaced high forest chestnut stands located in good quality sites, annual reductions in BAI induced by the gall wasp were modest, reaching only 9.9% on average. This apparently contradicts previous findings in much denser chestnut coppices stands, in which a marked reduction in radial growth was observed. Due to the differences in density between these two types of stands, we suggest that inter-tree competition, mainly for light resources, may mediate the impact of attack by the Asian chestnut gall wasp on chestnut tree growth. Additionally, nutrient availability may also have helped chestnut trees to compensate for the pest-induced damage. The study findings suggest that maintaining a lower stand density and promoting plantations in high quality sites may mitigate the impact of gall wasp attack on secondary growth.
Data availability statement
The data that support the findings of this study are available from the corresponding author upon reasonable request.
Author contributions
FC-D: conceptualization, data acquisition, data curation, formal analysis, investigation, methodology, and writing—original draft. PÁ-Á: data acquisition, data curation, resources, formal analysis, and writing—review and editing. ML: conceptualization, data acquisition, funding acquisition, methodology, project administration, resources, and writing—review and editing. All authors contributed to the article and approved the submitted version.
Funding
This work was supported by FEDER/Spanish Ministry of Economy and Competitiveness Research (grant AGL2016-76262-R).
Acknowledgments
Diana Blanco and Elva Rico provided assistance in the field.
Conflict of interest
The authors declare that the research was conducted in the absence of any commercial or financial relationships that could be construed as a potential conflict of interest.
Publisher's note
All claims expressed in this article are solely those of the authors and do not necessarily represent those of their affiliated organizations, or those of the publisher, the editors and the reviewers. Any product that may be evaluated in this article, or claim that may be made by its manufacturer, is not guaranteed or endorsed by the publisher.
Supplementary material
The Supplementary Material for this article can be found online at: https://www.frontiersin.org/articles/10.3389/ffgc.2022.1095185/full#supplementary-material
References
Álvarez-Álvarez, P., Díaz-Varela, E., Cámara-Obregón, A., and Afif-Khouri, E. (2010). Relating growth and nutrition to site factors in young chestnut plantations established on agricultural and forest land in northern Spain. Agrofor. Syst. 79, 291–301. doi: 10.1007/s10457-010-9313-z
Battisti, A., Benvegnù, I., Colombari, F., and Haack, R. A. (2014). Invasion by the chestnut gall wasp in Italy causes significant yield loss in Castanea sativa nut production. Agr. Forest Entomol. 16, 75–79. doi: 10.1111/afe.12036
Biondi, F., and Qeadan, F. (2008). A theory-driven approach to tree-ring standardization: defining the biological trend from expected basal area increment. Tree-Ring Res. 64, 81–96. doi: 10.3959/2008-6.1
Björkman, C., Hoglund, S., Eklund, K., and Larsson, S. (2000). Effects of leaf beetle damage on stem wood production in coppicing willow. Agric. For. Entomol. 2, 131–139. doi: 10.1046/j.1461-9563.2000.00058.x
Bombi, P., Fedi, C., Zapparoli, M., Cammarano, M., Guidolotti, G., Pallozzi, E., et al. (2018). Infestation potential of Dryocosmus kuriphilus Yasumatsu, 1951 (Hymenoptera: Cinipidae) in different natural populations of Castanea sativa Miller: an experimental ex situ test. Int. J. Pest Manage. 65, 147–153. doi: 10.1080/09670874.2018.1483091
Bréda, N., Huc, R., Granier, A., and Dreyer, E. (2006). Temperate forest trees and stands under severe drought: a review of ecophysiological responses, adaptation processes and long-term consequences. Ann. For. Sci. 63, 625–544. doi: 10.1051/forest:2006042
Burkhart, H. E., and Tomé, M. (2012). Modeling Forest Trees and Stands. New York, NY: Springer Science and Business Media.
Camarero, J. J., Álvarez-Taboada, F., Hevia, A., and Castedo-Dorado, F. (2018). Radial growth and wood density reflect the impacts and susceptibility to defoliation by gypsy moth and climate in radiata pine. Front. Plant Sci. 9, 1582. doi: 10.3389/fpls.2018.01582
Castedo-Dorado, F., Álvarez-Álvarez, P., Cuenca Valera, B., and Lombardero, M. J. (2021). Local-scale dispersal patterns and susceptibility to Dryocosmus kuriphilus in different Castanea species and hybrid clones: insights from a field trial. New For. doi: 10.1007/s11056-021-09893-8
Cipollini, D., Walters, D., and Voelckel, C. (2014). Cost of resistance in plants: from theory to evidence. Annual Plant Rev. 47, 263–307. doi: 10.1002/9781118829783.ch8
Coley, P. D., Bryant, J. P., and Chapin, F. S. (1985). Resource availability and plant antiherbivore defense. Science 230, 895–899. doi: 10.1126/science.230.4728.895
Conedera, M., Krebs, P., Gehring, E., Wunder, J., Hülsmann, L., Abegg, M., et al. (2021). How future-proof is sweet chestnut (Castanea sativa) in a global change context? For. Ecol. Manage. 494, 119320. doi: 10.1016/j.foreco.2021.119320
Conedera, M., Manetti, M. C., Giudici, F., and Amorini, E. (2004). Distribution and economic potential of the sweet chestnut (Castanea sativa Mill.) in Europe. Ecol. Mediterr. 30, 179–193. doi: 10.3406/ecmed.2004.1458
Covone, F., and Gratani, L. (2006). Age-related physiological and structural traits of chestnut coppices at the Castelli Romani Park (Italy). Ann. For. Sci. 63, 239–247. doi: 10.1051/forest:2006002
Cufar, K., Cherubini, M., Gricar, J., Prislan, P., Spina, S., and Romagnoli, M. (2011). Xylem and phloem formation in chestnut (Castanea sativa Mill.) during the 2008 growing season. Dendrochronologia 29, 127–134. doi: 10.1016/j.dendro.2011.01.006
Dobbertin, M. (2005). Tree growth as indicator of tree vitality and of tree reaction to environmental stress: a review. Eur. J. Forest Res. 124, 319–333. doi: 10.1007/s10342-005-0085-3
Ermich, K. (1959). The investigations of the seasonal course of the diameter growth of Pinus sylvestris L. and Quercus robur L. Acta. Soc. Bot. Pol. 28, 15–63. doi: 10.5586/asbp.1959.002
Fernandes, P., Colavolpe, M. B., Serrazina, S., and Costa, R. L. (2022). European and American chestnuts: an overview of the main threats and control efforts. Front. Plant Sci. 13, 951844. doi: 10.3389/fpls.2022.951844
Fernández-López, J. (2011). Identification of the genealogy of interspecific hybrids between Castanea sativa, Castanea crenata and Castanea mollissima. For. Syst. 20, 65–80. doi: 10.5424/fs/2011201-9136
Fernández-López, J., Miranda-Fontaíña, M. E., Furones-Pérez, P., and Míguez, B. (2009). Clonal Forestry of Chestnut in Northern Spain. Centro de Investigación Ambiental (CINAM-Lourizán) Pontevedra. Xunta de Galicia. Available online at: https://lourizan.xunta.gal/es/centro/departamentos/departamento-de-silvicultura-y-mejora/publicaciones (accessed July 4, 2021).
Fonti, P., and García-González, I. (2004). Suitability of chestnut earlywood vessel chronologies for ecological studies. New Phytol. 163, 77–86. doi: 10.1111/j.1469-8137.2004.01089.x
Gehring, E., Bellosi, B., Quacchia, A., and Conedera, M. (2018a). Assessing the impact of Dryocosmus kuriphilus on the chestnut tree: branch architecture matters. J. Pest Sci. 91, 189–202. doi: 10.1007/s10340-017-0857-9
Gehring, E., Bellosi, B., Quacchia, A., and Conedera, M. (2018c). Evaluating Dryocosmus Kuriphilus-induced damage on Castanea sativa. J. Vis. Exp. 138, 57564. doi: 10.3791/57564-v
Gehring, E., Kast, C., Kilchenmann, V., Bieri, K., Gehrig, R., Pezzatti, P. B., et al. (2018b). Impact of the Asian Chestnut gall wasp, Dryocosmus kuriphilus (Hymenoptera, Cynipidae), on the chestnut component of honey in the Southern Swiss Alps. J. Econ. Entomol. 111, 43–52. doi: 10.1093/jee/tox338
González-González, B. D., Rozas, V., and García-González, I. (2014). Earlywood vessels of the sub-Mediterranean oak Quercus pyrenaica have greater plasticity and sensitivity than those of the temperate Q. petraea at the Atlantic–Mediterranean boundary. Trees Struct. Funct. 28, 237–252. doi: 10.1007/s00468-013-0945-2
Grömping, U. (2006). Relative importance for linear regression in R: the package relaimpo. J. Stat. Soft. 17, 1–27. doi: 10.18637/jss.v017.i01
Guillet, C, and Bergström, R. (2006). Compensatory growth of fast-growing willow (Salix) coppice in response to simulated large herbivore browsing. Oikos 113, 33–42. doi: 10.1111/j.0030-1299.2006.13545.x
Gyoutoku, Y., and Uemura, M. (1985). Ecology and biological control of the chestnut gall wasp, Dryocosmus kuriphilus Yasumatsu (Hymenoptera: Cynipidae). 1. Damage and parasitization in Kumamoto Prefecture. Proceed. Assoc. Plant Protect. Kyushu 31, 213–215. doi: 10.4241/kyubyochu.31.213
Hasenauer, H. (2006). “Concepts within tree growth modelling,” in Sustainable Forest Management-Growth Models for Europe. Chap. 1, ed H. Hasenauer (Springer, Berlin), 64–70.
Hawkes, C. V., and Sullivan, J. J. (2001). The impact of herbivory on plants in different resource conditions: a meta-analysis. Ecology 82, 2045–2058. doi: 10.2307/2680068
Helbig, C. E., Müller, M. G., and Landgraf, D. (2021). Effects of leaf loss by artificial defoliation on the growth of different poplar and willow varieties. Forests 12, 1224. doi: 10.3390/f12091224
Inagaki, Y., Kuramoto, S., Torii, A., Shinomiya, Y., and Fukata, H. (2008). Effects of thinning on leaf-fall and leaf-litter nitrogen concentration in hinoki cypress (Chamaecyparis obtusa Endlicher) plantation stands in Japan. For. Ecol. Manage. 255, 1859–1867. doi: 10.1016/j.foreco.2007.12.007
Kato, K., and Hijii, N. (1997). Effects of gall formation by Dryocosmus kuriphilus Yasumatsu (Hym., Cynipidae) on the growth of chestnut trees. J. Appl. Entomol. 121, 9–15. doi: 10.1111/j.1439-0418.1997.tb01363.x
Kotobuki, K., Mori, K., and Sato, Y. (1985). 2 methods to estimate the tree damage by chestnut gall wasp Dryocosmus-kuriphilus. Bull. Fruit. Tree Res. Stn. A 2, 29–36.
Lombardero, M. J., Ayres, M. P., Álvarez-Álvarez, P., and Castedo-Dorado, F. (2022). Defensive patterns of chestnut genotypes (Castanea spp.) against the gall wasp, Dryocosmus kuriphilus. Front. For. Glob. Change 5, 1046606. doi: 10.3389/ffgc.2022.1046606
Lombardero, M. J., Ayres, M. P., Bonello, P., Cipollini, D., and Herms, D. A. (2016). Effects of defoliation and site quality on growth and defenses of Pinus pinaster and P. radiata. For. Ecol. Manage 382, 39–50. doi: 10.1016/j.foreco.2016.10.003
Maltoni, A., Mariotti, B., and Tani, A. (2012). Case study of a new method for the classification and analysis of Dryocosmus kuriphilus Yasumatsu damage to young chestnut sprouts. iForest 5, 50–59. doi: 10.3832/ifor0598-008
Manetti, M. C., Marcolin, E., Pividori, M., Zanuttini, R., and Conedera, M. (2020). “Coppice woodlands and chestnut wood technology,” in The chestnut Handbook. Crop and Forest Management, Eds G. Beccaro, A. Alma, G. Bounous, and J. Gomes-Laranjo (Boca Raton, London, New York, NY: CRC Press), 275–295.
Marcolin, E., Pividori, M., Colombari, F., Manetti, M. C., Pelleri, F., Conedera, M., et al. (2021). Impact of the Asian gall wasp (Dryocosmus kuriphilus) on the radial growth of the European chestnut (Castanea sativa). J. Appl. Ecol. 58:1212–1124. doi: 10.1111/1365-2664.13861
Marini, F., Battipaglia, G., Manetti, M. C., Corona, P., and Romagnoli, M. (2019). Impact of climate, stand growth parameters, and management on isotopic composition of tree rings in chestnut coppices. Forests 10, 1148. doi: 10.3390/f10121148
Mariotti, B., Antonello, L., Tani, A., Vannuccini, M., and Maltoni, A. (2013). Impact of Dryocosmus kuriphilus (Yatsumatsu) Attack on Chestnut Coppices Growth. ISHS II European Congress on Chestnut 9-12 October 2013. Available online at: http://www.studioeureco.com/wp-content/uploads/2013/10/posterDEBRECEN_2013.pdf (accessed July 4, 2021).
Maschinski, J., and Whitham, T. G. (1989). The continuum of plant responses to herbivory: the influence of plant association, nutrient availability, and timing. Am. Nat. 134, 1–19. doi: 10.1086/284962
McNaughton, S. J., Wallace, L. L., and Coughenour, M. B. (1983). Plant adaptation in an ecosystem context: effects of defoliation, nitrogen, and water on growth of an African C4 sedge. Ecology 64, 307–318. doi: 10.2307/1937078
Meyer, J. B., Gallien, L., and Prospero, S. (2015). Interaction between two invasive organisms on the European chestnut: does the chestnut blight fungus benefit from the presence of the gall wasp? FEMS Microbiol. Ecol. 91, fiv122. doi: 10.1093/femsec/fiv122
Míguez-Soto, B., Martínez Chamorro, E., and Fernández López, J. (2018). Tolerancia a la avispa del castaño (Dryocosmus kuriphilus) en variedades tradicionales de fruto e híbridos interespecíficos. Xunta de Galicia. Centro de Investigación Forestal de Lourizán. Available online at: https://lourizan.xunta.gal/es/transferencias/tolerancia-la-avispa-del-castano-dryocosmus-kuriphilus-en-variedades-tradicionales-de (accessed July 21, 2022).
Monserud, R. A., and Sterba, H. (1996). A basal area increment model for individual trees growing in even and uneven-aged forest stands in Austria. For. Ecol. Manage. 80, 57–80. doi: 10.1016/0378-1127(95)03638-5
Nakagawa, S., and Schielzeth, H. (2013). A general and simple method for obtaining R2 from generalized linear mixed-effects models. Methods Ecol. Evol. 2, 133–142. doi: 10.1111/j.2041-210x.2012.00261.x
Nieves-Aldrey, J. L., Gil-Tapetado, D., Gavira, O., Boyero, J. R., Polidori, C., Lombardero, M. J., et al. (2019). Torymus sinensis Kamijo, a biocontrol agent against the invasive chestnut gall wasp Dryocosmus kuriphilus Yasumatsu in Spain: its natural dispersal from France and the first data on establishment after experimental releases. For. Syst. 28, e001. doi: 10.5424/fs/2019281-14361
Ninyerola, M., Pons, X., and Roure, J. M. (2005). Atlas Climático Digital de la Península Ibérica. Metodología y aplicaciones en bioclimatología y geobotánica. Bellaterra: Universidad Autónoma de Barcelona.
Patrício, M. S., and Nunes, L. (2017). Density management diagrams for sweet chestnut high-forest stands in Portugal. iForest 10, 865–870. doi: 10.3832/ifor2411-010
Pérez-Otero, R., Crespo, D., and Mansilla, J. P. (2017). Dryocosmus kuriphilus Yasumatsu, 1951 (Hymenoptera: Cynipidae) in Galicia (NW Spain): pest dispersion, associated parasitoids and first biological control attempts. Arquivos Entomolóxicos 17, 439–448.
Pérez-Otero, R., and Mansilla, J. P. (2014). El cinípido del castaño Dryocosmus kuriphilus Yasumatsu, 1951 llega a Galicia (NO de la Península Ibérica). Arquivos Entomolóxicos 12, 33–36.
Pinheiro, J., Bates, D., and R Core Team (2022). nlme: Linear and Nonlinear Mixed Effects Models. R package version 3.1-161. Available online at: https://CRAN.R-project.org/package=nlme (accessed October 21, 2022).
Proietti, P., Palliotti, A., Famiani, F., Antognozzi, E., Ferranti, F., Andreutti, R., et al. (2000). Influence of leaf position, fruit and light availability on photosynthesis of two chestnut genotypes. Sci. Hortic. 85, 63–73. doi: 10.1016/S.0304-4238(99)00129-6
R Core Team (2022). R: A Language and Environment for Statistical Computing. R Foundation for Statistical Computing, Vienna, Austria. Available online at: https://www.R-project.org/ (accessed October 21, 2022).
Rigling, D., and Prospero, S. (2018). Cryphonectria parasitica, the causal agent of chestnut blight: invasion history, population biology and disease control. Mol. Plant Pathol. 19, 7–20. doi: 10.1111/mpp.12542
Rubio, A., Escudero, A., and Gandullo, J. M. (1997). Sweet chestnut silviculture in an ecological extreme of its range in the west of Spain (Extremadura). Ann. For. Sci. 54, 667–680. doi: 10.1051/forest:19970707
Sartor, C., Botta, R., Mellano, M. G., Beccaro, G. L., Bounous, G., Marinoni, D. T., et al. (2009). Evaluation of susceptibility to Dryocosmus kuriphilus Yasumatsu (Hymenoptera: Cynipidae) in Castanea sativa Miller and in hybrid cultivars. Acta. Hort. 815, 289–297. doi: 10.17660/ActaHortic.2009.815.38
Sartor, C., Dini, F., Torello Marinoni, D., Mellano, M. G., Beccaro, G. L., Alma, A., et al. (2015). Impact of the Asian wasp Dryocosmus kuriphilus (Yasumatsu) on cultivated chestnut: Yield loss and cultivar susceptibility. Sci. Hortic. 197, 454–460. doi: 10.1016/j.scienta.2015.10.004
Sass-Klaassen, U., Sabajo, C. R., and Ouden, J. D. (2011). Vessel formation in relation to leaf phenology in pedunculate oak and European ash. Dendrochronologia 29, 171–175. doi: 10.1016/j.dendro.2011.01.002
Schuldt, A., Hönig, L., Li, Y., Fichtner, A., Härdtle, W., Oheimb, G., et al. (2017). Herbivore and pathogen effects on tree growth are additive, but mediated by tree diversity and plant traits. Ecol. Evol. 7, 7462–7474. doi: 10.1002/ece3.3292
Slodičák, M., Novák, J., and Dušek, D. (2011). Canopy reduction as a possible measure for adaptation of young Scots pine stand to insufficient precipitation in Central Europe. For. Ecol. Manage. 262, 1913–1918. doi: 10.1016/j.foreco.2011.02.016
Thomson, V. P., Cunningham, S. A., Ball, M. C., and Nicotra, A. B. (2003). Compensation for herbivory by Cucumis sativus through increased photosynthetic capacity and efficiency. Oecologia 134, 167–175. doi: 10.1007/s00442-002-1102-6
Ugolini, F., Massetti, L., Pedrazzoli, F., Tognetti, R., Vecchione, A., Zulini, L., et al. (2014). Ecophysiological responses and vulnerability to other pathologies in European chestnut coppices, heavily infested by the Asian chestnut gall wasp. For. Ecol. Manage. 314, 38–49. doi: 10.1016/j.foreco.2013.11.031
Vicente-Serrano, S. M., Beguería, S., and López-Moreno, J. I. (2010). A multiscalar drought index sensitive to global warming: the standardized precipitation evapotranspiration index. J. Clim. 23, 1696–1718. doi: 10.1175/2009JCLI2909.1
Vospernik, S. (2021). Basal area increment models accounting for climate and mixture for Austrian tree species. For. Ecol. Manage. 480, 118725. doi: 10.1016/j.foreco.2020.118725
Waring, W. H. (1987). Characteristics of trees predisposed to die. Bioscience 37, 569–574. doi: 10.2307/1310667
Xunta de Galicia (2020). Estadísticas Forestales. Consellería do Medio Rural, Xunta de Galicia. Available online at: https://mediorural.xunta.gal/es/recursos/estadisticas/estadisticas-forestales (accessed July 4, 2022).
Xunta de Galicia (2021). Dlan de actuacións de control do Dryocosmus kuriphilus. Solta do parasitoide (Torymus sinensis) e recollida bugallas 2021, Xunta de Galicia. Available online at: https://mediorural.xunta.gal/sites/default/files/temas/forestal/sanidade-forestal/210825_PLAN_ACTU_TS_2021_DEF%2523.pdf (accessed July 4, 2022).
Keywords: chestnut tree pest, infestation level, compensation for herbivory, high forest, basal area increment
Citation: Castedo-Dorado F, Álvarez-Álvarez P and Lombardero MJ (2023) The impact of the Asian chestnut gall wasp (Dryocosmus kuriphilus) on chestnut tree growth may be mediated by site resources. Front. For. Glob. Change 5:1095185. doi: 10.3389/ffgc.2022.1095185
Received: 10 November 2022; Accepted: 28 December 2022;
Published: 13 January 2023.
Edited by:
Juan A. Martin, Universidad Politecnica de Madrid, SpainReviewed by:
Diego Gallego, University of Alicante, SpainAndrea Battisti, University of Padua, Italy
Copyright © 2023 Castedo-Dorado, Álvarez-Álvarez and Lombardero. This is an open-access article distributed under the terms of the Creative Commons Attribution License (CC BY). The use, distribution or reproduction in other forums is permitted, provided the original author(s) and the copyright owner(s) are credited and that the original publication in this journal is cited, in accordance with accepted academic practice. No use, distribution or reproduction is permitted which does not comply with these terms.
*Correspondence: Fernando Castedo-Dorado, ZmNhc2RAdW5pbGVvbi5lcw==
†ORCID: Fernando Castedo-Dorado orcid.org/0000-0002-1656-5255
Pedro Álvarez-Álvarez orcid.org/0000-0002-6898-8137
María Josefa Lombardero orcid.org/0000-0002-3245-896X