- 1Department of Botany, Kumaun University, Nainital, Uttarakhand, India
- 2Department of Forestry and Natural Resources, HNB Garhwal University, Srinagar, Uttarakhand, India
- 3Department of Forestry, Mizoram University, Aizwal, Mizoram
The belowground systems of trees have a major role in forest functioning through absorption of water and nutrient cycling. This study deals with the fine root dynamics including fine root biomass, necromass, production, turnover, and nutrient return in transitional Sal (Shorea robusta Gaertn. f.) dominated sub-tropical forest ecosystems of Central Himalaya, India. Four sites namely, Site-1 (Kaladhungi), Site-2 (Fatehpur), Site-3 (Ranibagh), Site-4 (Amritpur) were selected in Sal forest within an elevational range between 405 and 580 m above sea level. The dominant and associated co-dominant species were selected from each site for the estimation of fine root dynamics by using sequential core and ingrowth core methods. The results revealed that the fine root biomass, necromass, and production were significantly (p < 0.05) affected by location, seasons, and soil properties. The fine root biomass and production decreased with increasing soil depth and also influenced by stand characteristics including tree density and basal area. The rainy season was most productive with maximum fine root biomass (507.37 kg ha–1) as well as fine root production (600.26 kg ha–1 season–1) in the dominant tree species S. robusta. Among the associated co-dominant tree species highest fine root biomass (330.48 kg ha–1) and fine root production (410.04 kg ha–1 season–1) was reported for Tectona grandis L. during the rainy season, while lowest fine root biomass (126.72 kg ha–1) and fine root production (195.59 kg ha–1 season–1) in the Glochidion velutinum Wight tree species during the winter season. Annual fine root production ranged from 460.26 to 1583.55 kg ha–1 yr –1, while turnover rate varied from 1.37 to 4.45 yr–1 across all the studied sites. The fine roots added carbon input of 154.38 to 564.20 kg ha–1 yr–1 and nitrogen input of 6.58 to 24.34 kg ha–1 yr–1 to the soil through annual flux. The study improves our understanding on fine root parameters under the influence of sites, soils and seasonal and spatial variation. The return of nutrients to the soil through fluxes from the roots illustrates the role of fine roots in carbon and nitrogen cycling of the forests and this potential can be harnessed to assess the long-term carbon and nitrogen pool estimations in forests and to plan and manage the forest ecosystems.
Introduction
The forest ecosystems have four major sources that are responsible for the flux of nutrients within the ecosystem namely aboveground, belowground components, litter, and soil (Persch et al., 2015). Among these, the belowground parts have a pivotal role in the functioning of a forest ecosystem and contribute more than 30% of net primary productivity of the terrestrial ecosystems but majority of the studies mainly focus on the contribution of aboveground parts (Mikieleko et al., 2021). Fine roots have a diameter of ≤2 mm are involved in the nutrient acquisition, organic matter accumulation, and elemental flow within the forest ecosystems (Wang et al., 2016) and are mainly concentrated in the soil surface due to the higher concentrations of soil organic matter and rapid microbial activities in the upper horizon of soil (Bargali et al., 2019; Manral et al., 2022). Fine roots have a key role in soil carbon cycling through rapid turnover and root exudates (Pausch and Kuzyakov, 2018; Keller et al., 2021). These fine roots thorough carbon and nitrogen fluxes also helps for the natural regeneration of forests which ultimately conserve the biodiversity, mitigating the atmospheric carbon, and provide multiple ecosystem goods, and services (Chazdon et al., 2020). Efforts to restore the degraded and lost ecosystems are the need of today (Chazdon and Brancalion, 2019). Natural regeneration of forests is an intersection point for conservation and restoration goals (Chazdon, 2019).
Vegetation, soil characteristics and availability of resources determines the development, distribution and proliferation of root system in trees (Weemstra et al., 2020; Awasthi et al., 2022a). Distinct seasonality in the sub-tropical regions alters the resource availability in different seasons resulting in temporal and spatial variability in fine roots (Cusack et al., 2021; Karki et al., 2022). Fine roots have a crucial role in fluxes of carbon and nitrogen in forest ecosystems (Malhi et al., 2017). The fine root biomass and production constitutes a small fraction of tree biomass but the carbon inputs to the soil from fine roots are 1.4 times higher than the inputs from the aboveground components due to the rapid turnover rate of fine roots in most of the terrestrial ecosystems (Ding et al., 2019).
The Bhabhar region which is an alluvial belt with permeable sediments composed of sub-tropical Sal forests that mark a transitional zone between hills and terai; thus have a peculiar topography and vegetation composition. This region also having several ecologically and economically important species that contributes to the nutrient accumulation and nutrient cycling, cardinally and is under immense anthropogenic pressures. The aboveground components of the species have been explored and well documented, while the belowground systems still remain unfathomed (Persch et al., 2015). Fine roots are immensely important component of the forest ecosystems yet the detailed information on fine root dynamics in the Himalayan terrain is limited to some regions only (Usman et al., 2000; Garkoti, 2012; Karki et al., 2021a; Verma et al., 2021). The objectives of the present study were to study the fine root dynamics in Sal dominated forest ecosystem and to assess their role in organic matter and nitrogen accumulation and turnover in soil.
Materials and methods
Study sites and climate
The study was carried out in the Bhabhar region of Nainital district in Central Himalaya, India. An area of 1 ha was established in Shorea robusta Gaertn. f. dominated forests at four sites viz. Site-1 (Kaladhungi), Site-2 (Fatehpur), Site-3 (Ranibagh), and Site-4 (Amritpur) varying in topography and soil characteristics and located between 405 and 580 m asl (Figure 1). The characteristics of the sites are given in Table 1. The study was conducted during August 2017–2019. The study area comes under sub-tropical zone with peculiar climatic regimes with three distinct seasons namely, rainy (July–October), winter (November–February), and summer (March–June). During the 1st year the mean temperature ranged between 9 (January) and 38°C (June) and annual rainfall varied from 0 (October) to 577.6 mm (July), while during the 2nd year the mean temperature ranged from 5 (December) to 40°C (June) and annual rainfall ranged from 5 (January) to 558 mm (July). The climatic specifics are mentioned in Figures 2, 3.
Vegetation and soil sampling
The vegetation was sampled by placing 10 (10 m × 10 m) quadrats for tree species and the density and basal area were determined by following Misra (1968). Soil samples were collected from the three depths (0–20, 20–40, and 40–60 cm) by using a soil corer in random manner in each season and the soil samples were collected in replicates of three, brought to the laboratory and air-dried. Soil texture that indicates the relative content of particles of various sizes, such as sand, silt, and clay in the soil was determined by passing the soil through a series of sieves having different mesh sizes (Indian Standard, 1965). Soil pH was determined by mixing 20 g of soil sample with 40 ml of distilled water in a ratio of 1:2 and by stirring the suspension thoroughly using the glass rod. The mixture was then kept as such for 1 h. The combined electrode was inserted into supernatant and pH was recorded and before taking each new reading the electrode was washed and cleaned using distilled water following Jackson (1958). Bulk density refers to weight of dry soil per unit of volume typically expressed in gm cm–3. To determine the bulk density, a steel augur of known volume was used to collect soil samples from different soil depths, which were brought to the laboratory and oven dried at 60°C till constant weight and bulk density was determined by dividing oven-dried weight of soil by volume (πr2h), following Black (1965). Water holding capacity refers to the ability of a certain soil texture to physically hold water against the force of gravity and was determined by using nickel sieves with water saturated soil in filter papers following Piper (1950). Soil moisture content was determined by collecting 50 g of fresh soil samples (in triplicates), which were oven dried, weighed, and moisture content was calculated on the basis of dry weight following Jackson (1958). Soil porosity that represents void or empty pore spaces in the soil which is of crucial importance for conduction of water, air, and nutrients into the soil was determined by substracting, dividing bulk density values by a factor of 2.6 and multiplying with 100 following Kumar (2000). Soil organic carbon was estimated by using Walkley and Black (1934) procedure based on rapid dichromate oxidation. Soil nitrogen was determined by using Kjeldhal digestion method following defined standard ecological protocols (Subbiah and Asija, 1956).
Sampling of fine roots
For fine root estimation, at each location fine roots of dominant and co-dominant tree species were collected at 1 and 1.5 m distance from tree bole at 3 depths (0–20, 20–40, and 40–60 cm) through stratified random sampling. The fine root biomass (FRB) was estimated through sequential soil core method which is a direct method that equates root biomass using a steel auger having an internal diameter of 7.5 cm and a length of 60 cm. This method is simple in operational principle and provides highly accurate details of spatial and temporal variability of fine roots. The fine roots were extracted seasonally (rainy, winter, summer) up to the depth of 60 cm (0–20, 20–40, and 40–60 cm soil depth) using the steel auger. Roots were extracted from soil core and the hole was refilled with root-free soil. Depth wise root samples were collected from different directions and were pooled. Individual cores were placed in separate bags and taken to the laboratory to separate the fine roots. Organic material was separated from the roots by passing the individual soil cores through a sequence of sieves. The living and dead roots were hand sorted from the residues remaining in each sieve on the basis of color and texture and fine root biomass and necromass were determined. Fine root production (FRP) was estimated through ingrowth core method (Lukac and Godbold, 2010; Karki et al., 2022). The ingrowth core method is also a direct method which is based on the assumption that the disturbances to the roots and soil do not affect the root in growth and is highly economical and efficient. In this method, first time sampling of roots was done by using soil corer. Roots were collected and ingrowth cores (nylon net with mesh size of 2 mm and mesh openings) were installed vertically in holes of 60 cm depth where the soil had been removed with a corer, and the ingrowth cores were filled with root-free soil from the same stand and left in the field for about four months’ time interval which is optimal duration for assessing the ingrowth of fine roots. Thus, root-free cores were installed three times in each year during the study for the estimation of seasonal FRP. The cylindrical ingrowth core with mesh openings allows only the entry of fine roots, soil microorganisms into it due to its defined mesh size. The subsequent growth of roots in this nylon net was measured and used to estimate fine root production in the field. The collected roots from the respective depths and distances in each site were brought to the laboratory, pooled depth wise and were separated from debris and other organic material, cleaned and divided into live and dead roots on the basis of keen visual inspection and ultimately oven dried at 60°C for 48 h. The oven dried root samples were weighed, grinded, and kept in sealed plastic bags for carbon (Jackson, 1958) and nitrogen (micro-Kjeldhal method) analysis. At each location 3 individuals of each tree species were selected, marked and fine roots were collected seasonally, resulting in a total of 432 fine root samples each year {3(Trees) × 2(species) × 4(sites) × 3(depths) × 2(distances) × 3(seasons)}. Three individuals of each tree species were selected to unify the sampling procedure and to reduce the chances of biasness and errors in the study. The field sampling procedures were designed in such a manner that the clumping or overlapping of sampling locations and fine roots of different trees can be avoided. As the samples for each tree species were collected from a region where mostly the individuals of the same tree species were present within the study area to avoid any confusion in fine root collection and to capture the spatial heterogeneity of root distribution in the studied sites. Annual fine root production was calculated by summing the seasonal fine root production (Lopez et al., 2001). Fine root turnover was determined by dividing the annual fine root ingrowth by maximum fine root biomass depth wise and distance wise (Dahlmand and Kucera, 1965; Karki et al., 2021b). Mean fine root biomass and production and turnover values were determined depth wise seasonally and fine root datasets were prepared site wise for 2 years.
The annual carbon and nitrogen flux from the fine roots were calculated by multiplying the annual fine root production (kg ha–1 yr–1) with the carbon and nitrogen concentrations (%) in the fine roots following Xia et al. (2015) that yields the annual carbon and nitrogen input into the soil through fine roots.
Statistical analysis
SPSS 25 was used to establish relationship between vegetational attributes and fine root dynamics among the experimental sites, across the seasons and year. PAST3 (Paleontological Statistics Software for Education and Data Analysis) statistical package was used to determine the impact of soil properties on fine root dynamics.
Results
Vegetation and soil characteristics
The vegetational analysis revealed the dominance of S. robusta in all the sites and presence of co-dominant species like Mallotus philippensis (Lam.) Muell.-Arg at Kaladhungi, Glochidion velutinum Wight at Fatehpur, Holarrhena pubescens Wall. ex G.Don at Ranibagh and Tectona grandis L. at Amritpur. Across the locations total tree density ranged between 620 (Fatehpur) and 810 ind ha–1 (Ranibagh). Highest total basal area was reported in Amritpur (29.52 m2 ha–1) and lowest in Fatehpur (25.51 m2 ha–1). The soil was deep, well drained, neutral to slightly alkaline, coarse-loamy/fine loam, mainly composed of poorly sorted unconsolidated sediments like pebbles, gravels, sand, and silt with intervening clay layers which makes it highly porous. The percent sand content in the soil ranged from 32.13 (Ranibagh) to 39.18% (Amritpur), clay content varied from 23.20 (Kaladhungi) to 38.21% (Fatehpur) and silt content ranged between 26.01 (Fatehpur) and 40.43% (Kaladhungi). Soil moisture content was recorded lowest at Fatehpur (9.95%) and highest at Kaladhungi (13.56%). The maximum water holding capacity was recorded at Kaladhungi (57.10%), while, minimum at Amritpur (43.27%). Bulk density was highest at Fatehpur (1.33 g cm–3) and lowest at Amritpur (1.25 g cm–3). Porosity was highest at Kaladhungi and Amritpur (50.08%), while lowest at Fatehpur (48.80%). Soil pH ranged from slightly acidic (5.41) in Amritpur to slightly basic (7.49) in Ranibagh. The soil organic carbon content varied from 1.29 (Kaladhungi) to 2.74% (Amritpur), while total nitrogen content of the soil was reported to be maximum at Amritpur (0.25%) and minimum (0.17%) at Kaladhungi (Table 1).
Distribution pattern of fine roots
All the locations showed similar pattern of fine root distribution. Vertically, highest fine root dispersal was recorded in the uppermost layer (262.16–362.03 kg ha–1) which decreased with increasing soil depth during both the experimental years in all the species (Figures 4A–D). Horizontally, fine roots were more concentrated at 1 m distance (124.52–356.62 kg ha–1) from tree bole as compared to 1.5 m distance (110.75–313.75 kg ha–1) though the differences were statistically not significant (Figures 4A–D).
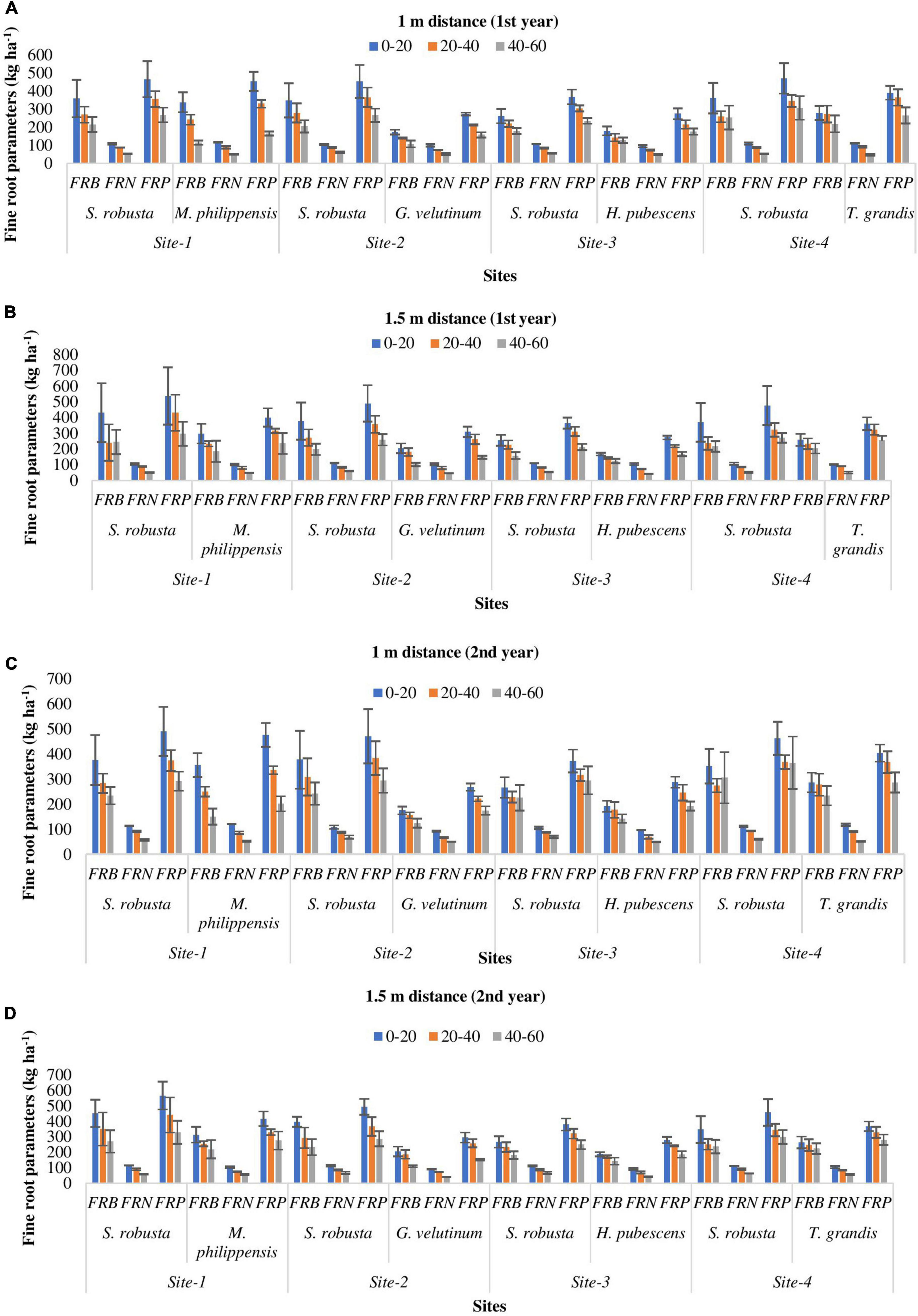
Figure 4. Spatial variability (A–D) in fine roots parameters (kg ha– 1) of trees in Sal forest sites. FRB, fine root biomass; FRN, fine root necromass; FRP, fine root production.
Fine root biomass
During the 1st year, at 1 m distance from the tree base FRB of S. robusta was maximum at Amritpur (362.03 kg ha–1) and minimum at Ranibagh (179.65 kg ha–1), while at 1.5 m distance from the tree base, highest value of FRB was reported at Kaladhungi (431.91 kg ha–1) and lowest FRB was reported at Ranibagh (158.52 kg ha–1). During the 2nd year, at 1 m distance from the tree base, the highest FRB was recorded at Fatehpur (377.90 kg ha–1), and lowest FRB was reported at Ranibagh (225.64 kg ha–1). The value of FRB at 1.5 m distances from the tree base was recorded to be maximum at Kaladhungi (451.83 kg ha–1) whereas, minimum FRB was reported at Ranibagh (181.50 kg ha–1). For the co-dominant species at Kaladhungi, the highest values of FRB (338.97 and 298.66 kg ha–1) were reported for M. philippensis at 1 and 1.5 m distance from the tree base, respectively, while lowest FRB was observed at Fatehpur for G. velutinum, (107.16 kg ha–1 at 1 m distance and 102.19 kg ha–1 at 1.5 m distance from tree base), during the 1st year. During the 2nd year, maximum FRB was reported for M. philippensis at Kaladhungi while, lowest for G. velutinum at Fatehpur at both the distances from the tree base (Figures 4C, D).
Fine root necromass
Across the sites, the fine root necromass (FRN) of S. robusta was highest (109.05 kg ha–1) at Amritpur and lowest (52.31 kg ha–1) at Kaladhungi at the distance of 1 m from the tree base during the 1st year. At 1.5 m distance from the tree base, maximum FRN was reported at Amritpur (113.08 kg ha–1) and minimum (51.32 kg ha–1) at Kaladhungi. During the 2nd year, the maximum (114.46 kg ha–1 at 1 m distance and 114.83 kg ha–1 at 1.5 m distance) and minimum (57.48 kg ha–1 at 1 m distance and 58.86 kg ha–1 at 1.5 m distance) FRN was recorded at Kaladhungi. For the co-dominant species, during the 1st year at 1 m distance from the tree base, the maximum FRN was recorded at Kaladhungi for M. philippensis (116.34 kg ha–1) and minimum FRN was recorded at Amritpur for T. grandis (47.17 kg ha–1) while at 1.5 m distance, the highest (105.65 kg ha–1) and lowest FRN (44.02 kg ha–1) was recorded for H. pubescens at Ranibagh. During the 2nd year, at 1 m distance from the tree base, the highest FRN (120.12 kg ha–1) was recorded for M. philippensis at Kaladhungi while, lowest value of FRN (49.68 kg ha–1) was recorded for H. pubescens at Ranibagh. At 1.5 m distance from the tree base, the maximum FRN (105.02 kg ha–1) was recorded for T. grandis of Amritpur and minimum (40.88 kg ha–1) for G. velutinum at Fatehpur (Figures 4C, D).
Seasonal fine root production
During the 1st year, the fine root production of S. robusta across the locations at 1 m distance from the tree base was maximum (471.08 kg ha–1 season–1) at Amritpur and minimum (235.49 kg ha–1 season–1) at Ranibagh, while at 1.5 m distance from the tree base highest fine root production was reported at Kaladhungi (538.82 kg ha–1 season–1) and lowest (212.98 kg ha–1 season–1) at Ranibagh. During the 2nd year, at 1 m distance from the tree base, the highest (491.22 kg ha–1 season–1) and lowest (291.86 kg ha–1 season–1) FRP were recorded at Kaladhungi. The maximum value of FRP at 1.5 m distance from the tree base was recorded at Kaladhungi (566.67 kg ha–1 season–1) and minimum at Ranibagh (249.04 kg ha–1 season–1). Among the co-dominant tree species highest FRP was estimated for M. philippensis at Kaladhungi while, lowest FRP was recorded for G. velutinum at Fatehpur during both the studied years as well as at both the distances. At 1 m distance from the tree base, the maximum FRP was 455.32 kg ha–1 season–1 and minimum FRP was 158.10 kg ha–1 season–1, while at 1.5 m distance, the highest recorded FRP was 401.80 kg ha–1 season–1 and lowest FRP was 148.73 kg ha–1 season–1, during the 1st year. During the 2nd year, at 1 m distance from the tree base, the highest FRP was 476.74 kg ha–1 season–1 and lowest FRP was 174.83 kg ha–1 season–1. At 1.5 m distance from the tree base, the maximum FRP was 416.89 kg ha–1 season–1 and minimum FRP was 151.62 kg ha–1 season–1 (Figures 4C, D).
Seasonal variation in fine roots
Fine root biomass, production and turnover of dominant and associated species also varied significantly (p < 0.05) across the seasons and years (p < 0.05).
Fine root biomass
During both the year, FRB of S. robusta, was maximum during the rainy season at Kaladhungi, while minimum during the winter season at Fatehpur. Among the co-dominant tree species, the highest FRB during 1st year was reported during rainy season for T. grandis (319.64 kg ha–1) at Amritpur, while lowest FRB was recorded during winter season for G. velutinum (126.72 kg ha–1). During 2nd year, the maximum FRB was observed for T. grandis (330.48 kg ha–1) at Amritpur and minimum FRB was reported for G. velutinum (126.72 kg ha–1) at Fatehpur (Figures 5A, B).
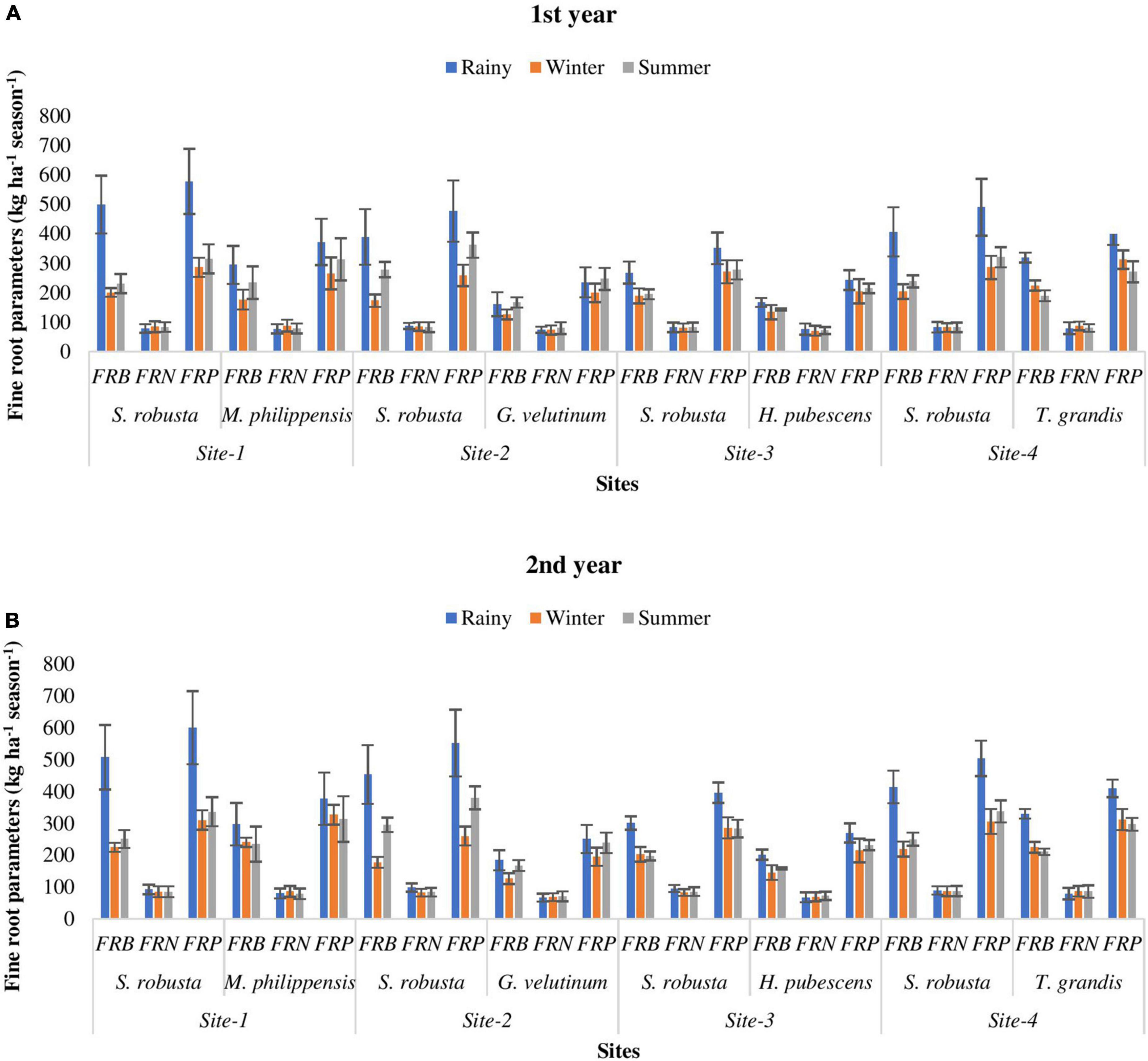
Figure 5. Temporal variability in fine root parameters (kg ha– 1 season– 1) among the experimental sites during 1st (A) and 2nd (B) year. FRB, fine root biomass; FRN, fine root necromass; FRP, fine root production.
Fine root necromass
During both the years, for S. robusta, the FRN was highest at Fatehpur during the rainy season and minimum at Ranibagh during the winter season. For the co-dominant tree species, during 1st year, the FRN was maximum during rainy season for T. grandis (79.87 kg ha–1) at Amritpur, and minimum during winter season for H. pubescens (71.06 kg ha–1) at Ranibagh. The FRN was highest at Kaladhungi for M. philippensis (80.18 kg ha–1) and lowest for G. velutinum (68.87 kg ha–1) at Fatehpur, during the 2nd year (Figures 5A, B).
Fine root production
During both the years, the FRP of S. robusta was maximum during rainy season at Kaladhungi, and minimum during winter season at Fatehpur. Across the years, during 1st year maximum FRP was 578.39 kg ha–1 season–1, while during 2nd year it was 600.26 kg ha–1 season–1. The minimum values of FRP were 258.91 and 260.54 kg ha–1 season–1 during 1st year and 2nd year, respectively. Among the co-dominant tree species, during 1st year, the FRP was maximum during rainy for T. grandis (399.50 kg ha–1 season–1) at Amritpur, and minimum during winter season for G. velutinum (200.30 kg ha–1 season–1) at Fatehpur. The highest FRP was observed at Amritpur for T. grandis (410.04 kg ha–1 season–1) and lowest FRP was reported for G. velutinum (195.59 kg ha–1 season–1) at Fatehpur, during 2nd year (Figures 5A, B).
Annual fine root production
In the present study, AFRP showed a decreasing trend with increasing soil depth. During the 1st year, annual fine root production of S. robusta ranged from 672.709 (Ranibagh) in deepest (40–60 cm) soil depth to 1559.935 kg ha–1 yr–1 (Kaladhungi) in uppermost (0–20 cm) soil depth. During the 2nd year, the AFRP for S. robusta varied from 816.547 (Ranibagh) to 1583.554 kg ha–1 yr–1 (Kaladhungi). During the 1st year, for the co-dominant tree species, the highest annual fine root production (1285.67 kg ha–1 yr–1) was recorded for M. philippensis (Kaladhungi) in 0–20 cm soil depth, while lowest AFRP (460.255 kg ha–1 yr–1) was observed for G. velutinum (Fatehpur) in 40–60 cm soil depth. During the 2nd year, AFRP ranged between 489.685 kg ha–1 yr–1 for G. velutinum (Fatehpur) in 40–60 cm soil depth to 1333.78 kg ha–1 yr–1 for M. philippensis (Kaladhungi) in 0–20 cm soil depth (Figure 6).
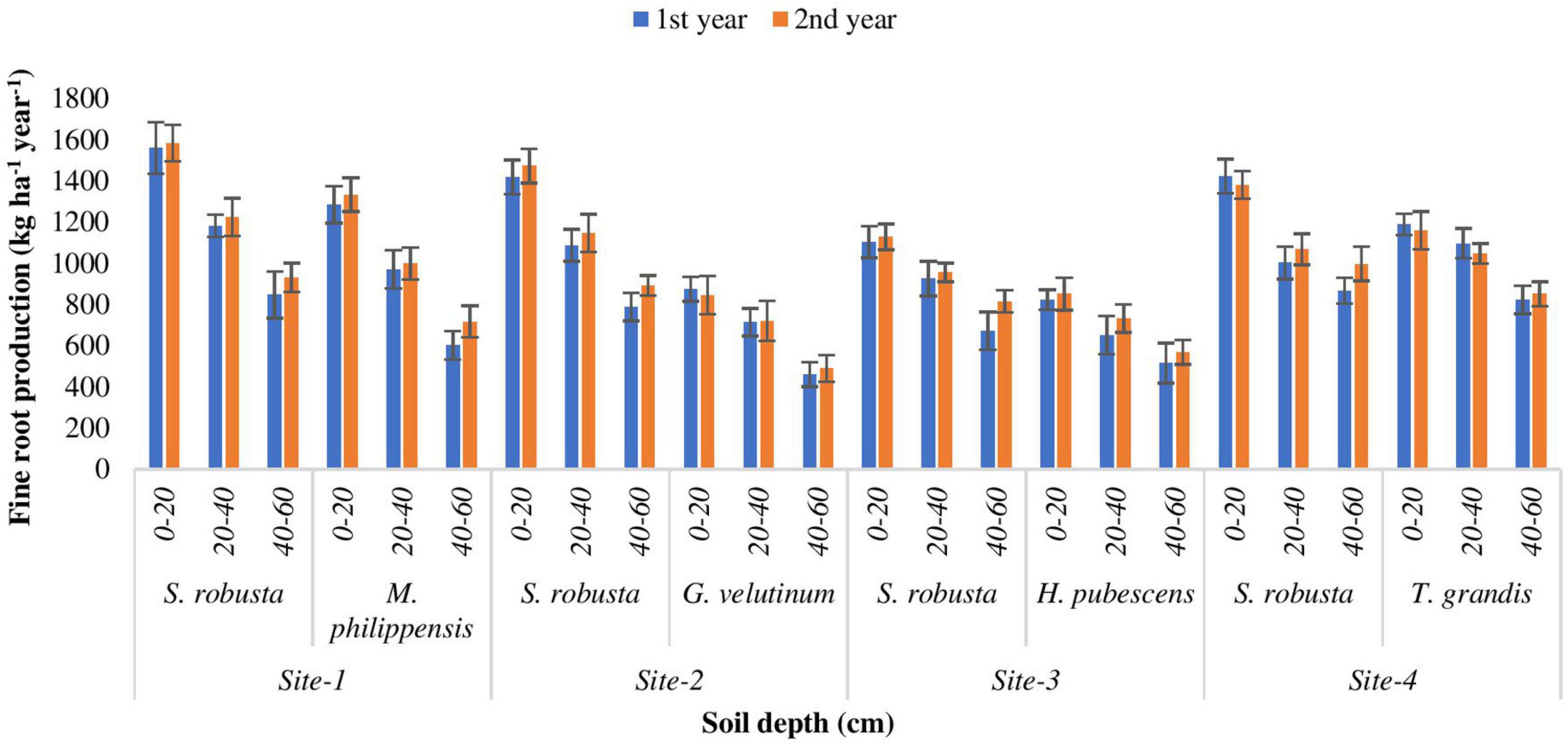
Figure 6. Variability in annual fine root production (AFRP) among the experimental sites (kg ha– 1 year– 1).
Turnover rate (yr–1)
The rate of fine root turnover was observed highest at upper most soil layer while, least at deeper soil depth in the present study. Fine root turnover rate of S. robusta ranged between 2.67 (Amritpur) and 3.43 yr–1 (Ranibagh) at 1 m distance from the tree base while, at 1.5 m distance from the tree base, the maximum turnover rate was reported at Ranibagh (3.45 yr–1) and minimum at Kaladhungi (2.47 yr–1) during the 1st year. During the 2nd year, at 1 m distance the turnover rate for S. robusta varied from 1.54 (Amritpur) to 3.33 yr–1 (Ranibagh), while at 1.5 cm distance from the tree base, the highest turnover rate was observed at Ranibagh (3.41 yr–1) and least at Amritpur (1.43 yr–1). Among the co-dominant tree species, during the 1st year at 1 m distance from the tree base turnover rate varied from 2.75 (for T. grandis at Amritpur) to 4.13 yr–1 (for G. velutinum) at Fatehpur, whereas at 1.5 m distance, the highest turnover rate (4.45 yr–1) was reported for H. pubescens at Ranibagh and lowest turnover rate (2.19 yr–1) was observed for M. philippensis at Kaladhungi. During the 2nd year, the turnover rate ranged from 1.54 (for T. grandis) at Amritpur to 3.92 yr–1 (for G. velutinum) in Fatehpur at 1 m distance whereas, at 1.5 m distance from the tree base the highest turnover rate (4.01 yr–1) was observed for H. pubescens at (Ranibagh) and lowest turnover rate (1.37 yr–1) was reported for T. grandis at Amritpur (Figures 7A, B).
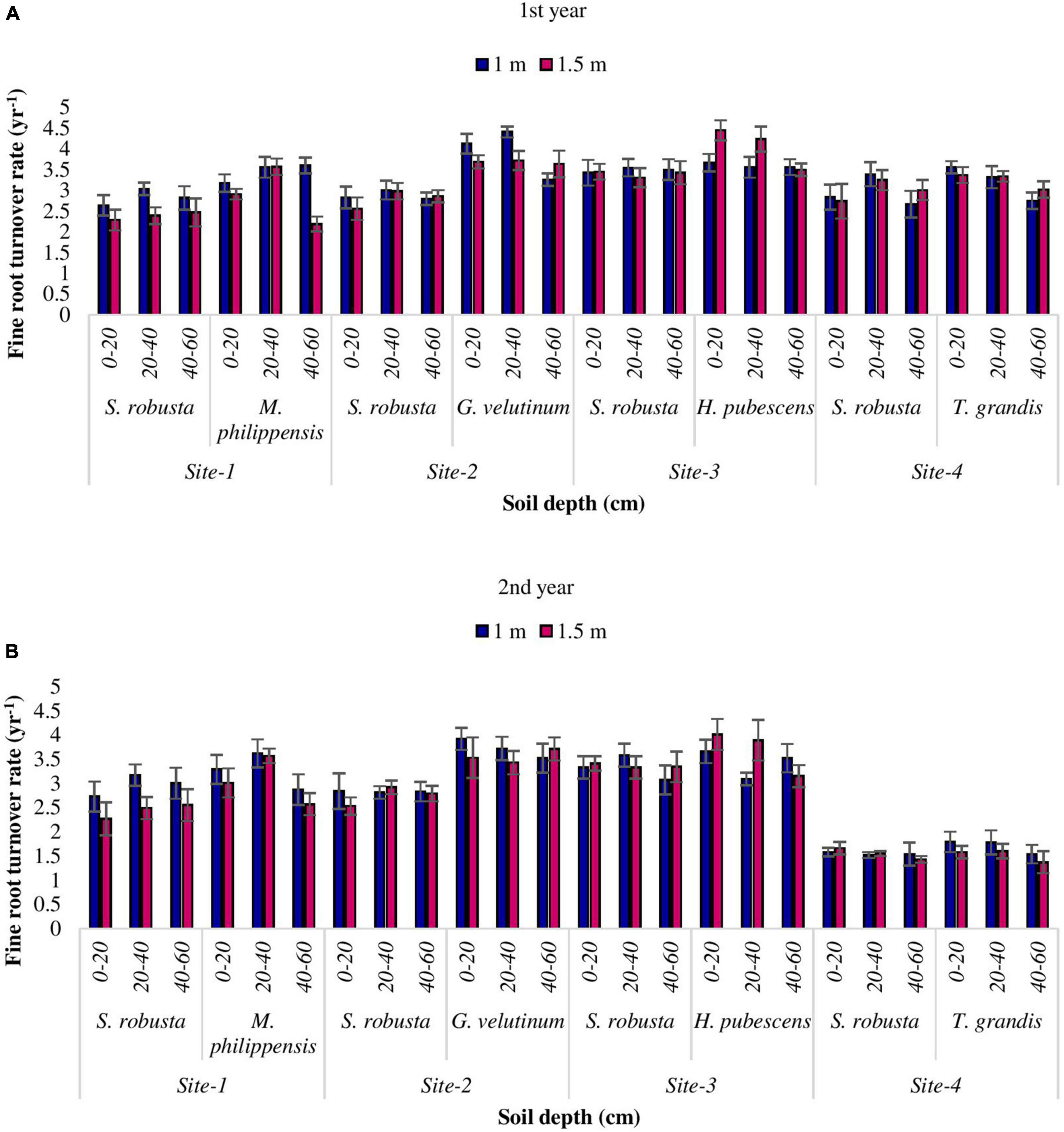
Figure 7. Variability in turnover rate (yr– 1) among the studied Sal forest sites during 1st (A) and 2nd year (B).
Nutrient return
Fine roots are an important source and sink for nutrients in terrestrial ecosystems. There were important differences in the nutrient concentrations (carbon and nitrogen) of roots as a function of soil depth with significant declines in concentration with depth (Tables 2, 3). The highest annual carbon flux (564.20 kg ha–1 yr–1) was recorded for S. robusta in 0–20 cm soil depth at Amritpur during 1st year as well as at Kaladhungi during the 2nd year. The lowest carbon flux was observed in 40–60 cm soil depth at site-3 (254.76 kg ha–1 yr–1) during 1st and 2nd year (307.10 kg ha–1 yr–1). Among the associated tree species, maximum carbon flux was reported for M. philippensis in 0–20 cm soil depth during 1st (427.16 kg ha–1 yr–1) as well as 2nd year (438.86 kg ha–1 yr–1) at Kaladhungi, while minimum flux was reported for G. velutinum in 40–60 cm soil depth during 1st year (154.38 kg ha–1 yr–1) and 2nd year (160.93 kg ha–1 yr–1) at Fatehpur (Figure 8).
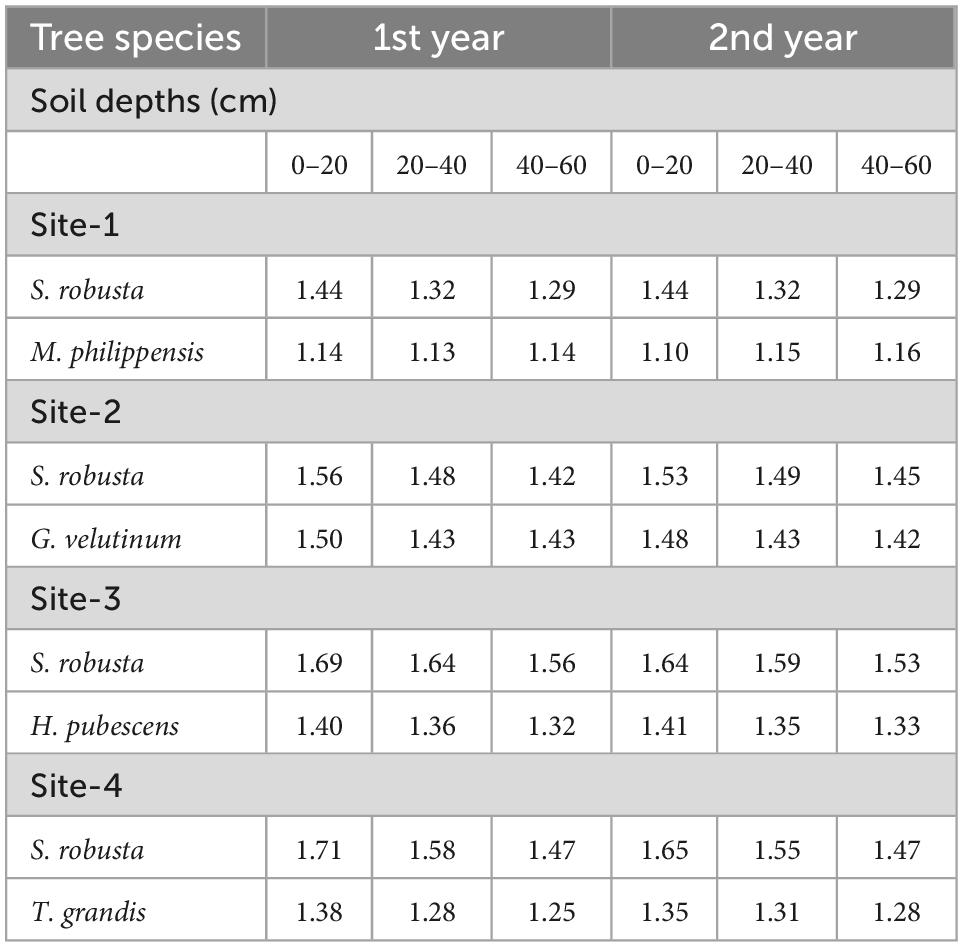
Table 3. Nitrogen concentration (%) in fine roots of trees under selected sub- tropical Sal forests.
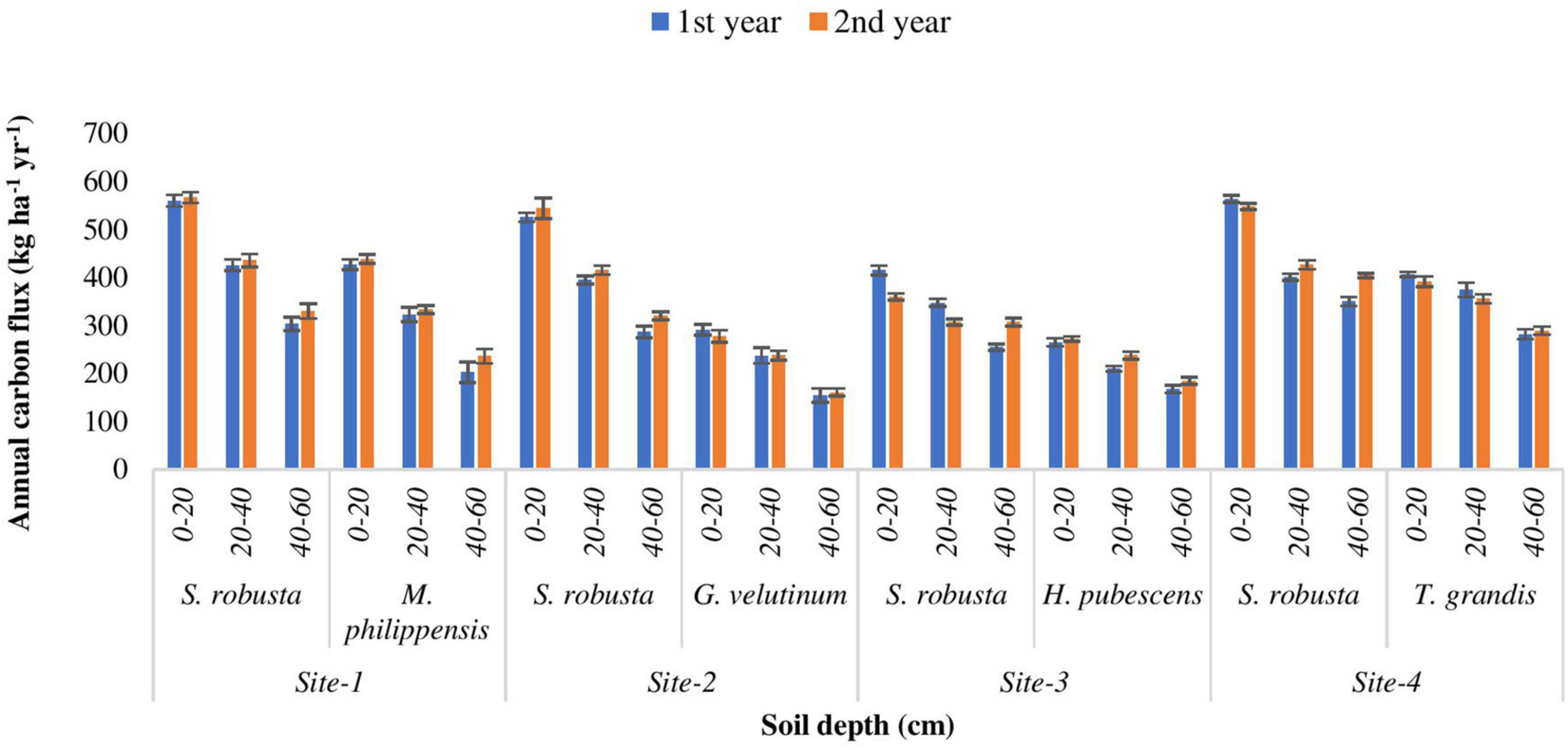
Figure 8. Annual carbon flux (kg ha– 1 yr– 1) into the soil via fine roots in sub- tropical Sal forest.
The maximum annual nitrogen flux was observed for S. robusta at in 0–20 cm soil depth at Amritpur (24.34 kg ha–1 yr–1) during the 1st year and at Kaladhungi (22.80 kg ha–1 yr–1) during the 2nd year. The lowest carbon flux was observed in 40–60 cm soil depth at Ranibagh during the 1st year (10.49 kg ha–1 yr–1) and at Kaladhungi during the 2nd year (12.01 kg ha–1 yr–1). Among the associated tree species highest annual nitrogen flux was reported for T. grandis during 1st (16.41 kg ha–1 yr–1) as well as 2nd year (15.65 kg ha–1 yr–1) at Amritpur, while minimum flux was reported for G. velutinum during 1st year (6.58 kg ha–1 yr–1) and 2nd year (6.97 kg ha–1 yr–1) at Fatehpur (Figure 9).
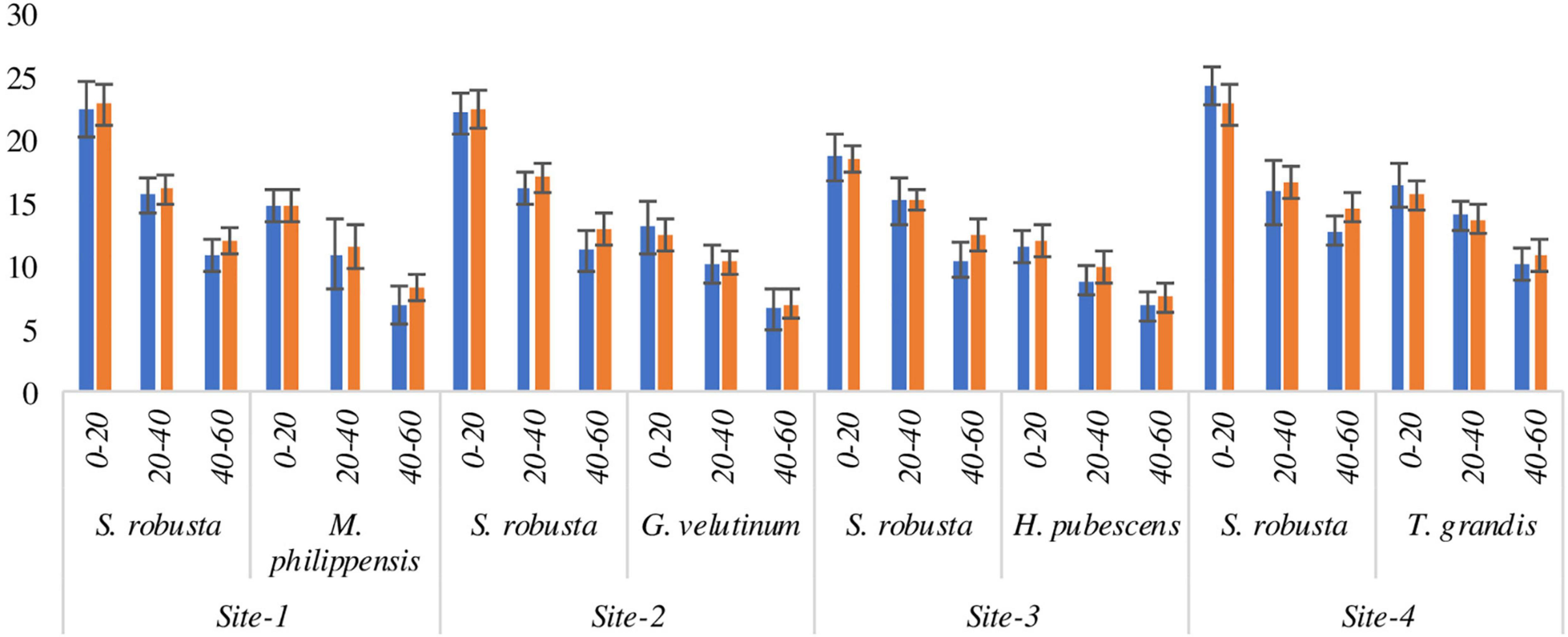
Figure 9. Annual nitrogen flux (kg ha– 1 yr– 1) into the soil via fine roots in sub- tropical Sal forest.
Discussion
The vertical gradient of fine root distribution revealed a distinct pattern with higher biomass, necromass and production in the superficial horizon of soil (0–20 cm) which declined subsequently in the deeper soil depths (20–40 and 40–60 cm). This pattern was observed probably because the top layer of soil (0–20 cm) is enriched with nutrient contents, organic matter which provides favorable microclimatic conditions for the expansion of root system. The different soil layers differ in their nutrient and water contents and thus have disparity in the distribution of fine roots (Sahu et al., 2013; Wang et al., 2016). The nutrient concentration of the surface layer is also supplemented by litter decomposition (Rosado et al., 2011; Oraon et al., 2018). Similar patterns of fine root distribution were also reported by Mikieleko et al. (2021) in semi-deciduous forest of Republic of Congo and Katayama et al. (2019) in tropical rainforest of Joshi and Garkoti (2021) in white oak forests of the Indian Central Himalayan region.
The present study revealed that spatial distribution of fine root biomass exhibited a peculiar pattern with highest fine root biomass (507.37 kg ha–1) and fine root production (600.26 kg ha–1 season–1) during the rainy season followed by summer and winter seasons, respectively. These seasonal variations in fine root biomass and production may be ascribed to the suitable moisture and temperature regimes as also supported by the climatic data obtained during the study periods. As the rainy season coincides with active growth period of many Himalayan tree species and during this season, the nutrients get dissolved in soil solution and percolate into deeper soil, thus to meet the demand of metabolic activities and growing vegetative structures, the root system elaborates resulting in higher fine root production. The temperature and moisture conditions are pertinent with respect to fine root growth as they are accountable for the carbon and nutrient allocation within terrestrial tropical and sub-tropical ecosystems. The significant relationship between fine root parameters and rainfall patterns in tropical forests of Ghana and chir-pine mixed banj-oak forests of Central Himalaya were also reported by Ibrahim et al. (2020) and Verma et al. (2021). Significant effect of temperature on fine root dynamics in sub-tropical forests was observed by Fu et al. (2015). The annual fine root production was higher for the dominant tree species S. robusta (672.71–1583.55 kg ha–1 yr–1) as compared to the associated co-dominant species (460.26–1333.78 kg ha–1 yr–1).
Across the sites, maximum fine root biomass was recorded at Kaladhungi (76.83–77.26%), while maximum FRN (28.10–28.13%) was recorded at Ranibagh during 1st as well as 2nd year (Figure 10). The FRB values obtained in the present study are comparable with the studies conducted in forests around the globe. Fine root biomass within a range of 1.1–5.8 t ha–1 in sub-tropical forests of China was observed by Yang et al. (2002) and Pei et al. (2018). The values of fine root biomass were reported from 174.3 to 516.6 g m–2 in protected natural forests of, in tropical Central Amazonian forest of Brazil by Singha et al. (2020), the FRB values ranged between 1.9 and 13.1 Mg ha–1 (Cordeiro et al., 2020) and in Central Himalayan chir-pine and banj-oak forests the FRB values were 24 to 972 kg ha–1 (Usman et al., 1997). Similar findings were also reported for fine root production from the deciduous forest of Central Himalaya (2.94–6.34 Mg ha ha–1 yr–1) by Garkoti (2011) and the agro-ecosystems of Central Himalaya (232.2–1905.9 kg ha–1 yr–1) by Karki et al. (2021c,2022).
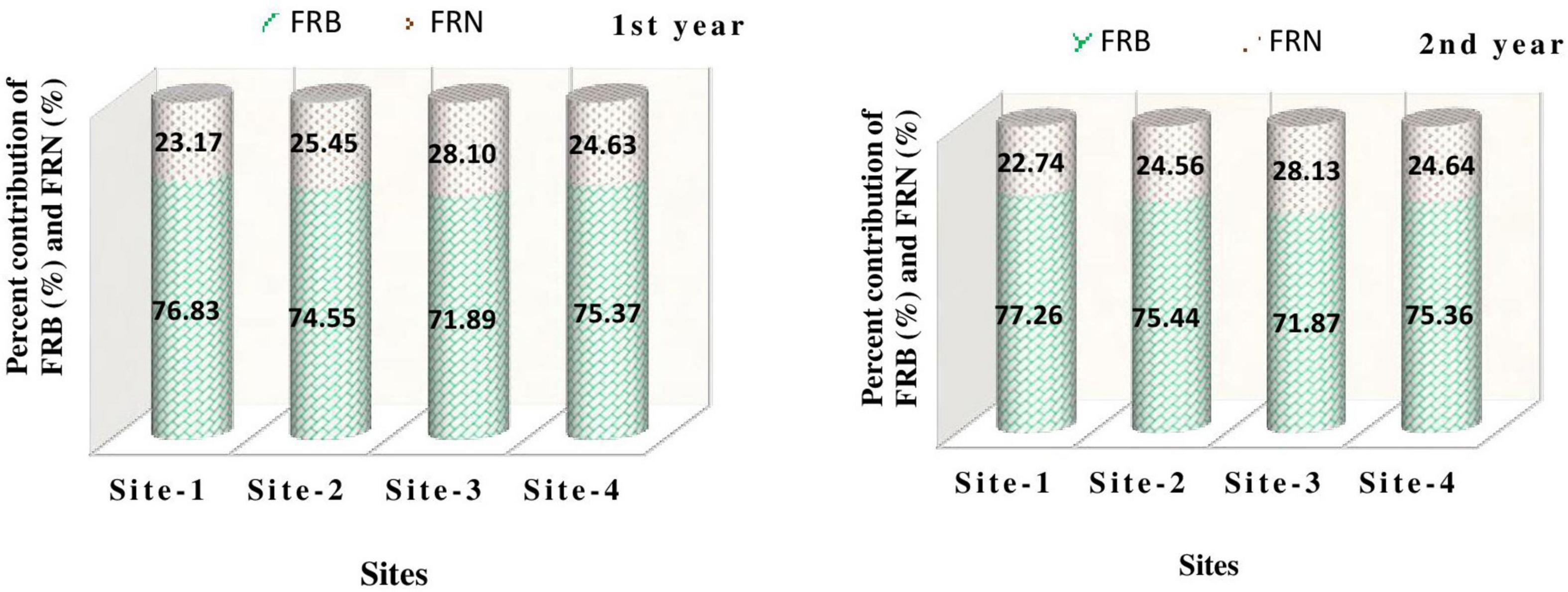
Figure 10. Percent contribution of fine root biomass (FRB) and fine root necromass (FRN) to fine root production among the study sites during the study period.
Turnover rate (yr–1) refers to the growth and dieback of fine roots and plays a crucial role in forest ecosystems as it provides carbon and nutrient inputs into the soil (Lukac, 2012). The water and nutrient accessibility to the growing vegetation in the forest ecosystems regulates the fine root turnover rates (Lima et al., 2010). The results showed no definite trend across the sites as well as depth the possibly due to the specific habitat conditions and climatic attributes as these variations are very evident in the Himalayan regions (Bargali et al., 2018). Similar findings from the global as comparison of turnover rates in boreal, temperate and tropical forests also reported by Finer et al. (2011). The values (1.37–4.45 yr–1) are comparable with the values (2.3–3.1 yr–1) reported by Ibrahim et al. (2020) and were comparatively higher than the values reported by Usman et al. (2000) and Verma et al. (2021) for chir- pine and banj-oak forests (0.67–0.84 yr–1) and Karki et al. (2021a) for agroforestry systems (1.38–2.67 yr–1) of Central Himalaya. The sub-tropical and tropical forests have higher fine root production and rapid turnover rates in comparison to the boreal and temperate forests (Wang et al., 2019).
Fine roots significantly contribute to the carbon and nitrogen inputs of the soil through fluxes, decomposition and mortality (Gautam and Mandal, 2018; Bibi et al., 2022). About 70% of soil organic carbon is sequestered by roots and is returned back to the soil on mortality (Finer et al., 2019). In the present study, the carbon fluxes from tree fine roots ranged between 154.38 and 564.20 kg ha–1 yr–1, while nitrogen fluxes varied from 6.58 to 24.34 kg ha–1 yr–1, during the study period. Similar findings were observed by Cornejo et al. (2021) for the carbon flux (171.26–211.72 g m–2 yr–1), nitrogen flux (2.83–7.67 g m–2 yr–1) and by Hertel et al. (2009) for carbon flux (127.2–232.8 g m–2 yr–1) in tropical forest stands varying in elevation and stand characteristics. Wapongnungsang, and Tripathi (2019) also reported that about 18–58% of soil nitrogen is returned to the soil through fine root mortality, turnover and decomposition. The slightly higher amount of carbon and nitrogen fluxes in the present study may be due to the vegetation and climatic conditions that through decomposition and exudates enhanced the soil nutrients and soil biota plays an important role in better growth of fine roots that ultimately resulted in higher fluxes on production and mortality (Keller et al., 2021; Awasthi et al., 2022b,c).
The forest structure including vegetation and floristic diversity (Ma and Chen, 2016), soil characteristics (Frouz et al., 2008) are also the crucial factors influencing fine root dynamics as soil properties can alter the root growth by regulating various physiological and chemical processes occurring within the ecosystem. Fine root dynamics is limited by various soil nutrients in the terrestrial ecosystems (Kochsiek et al., 2013). In the present study, Pearson’s correlation matrix with Bonferroni correction unveiled the effect of soil characteristics on fine root dynamics (Table 4). Fine root biomass, necromass and production of the dominant (S. robusta) and associated tree species showed strong negative correlation with soil depth and bulk density, while strong positive correlation with porosity across all the sites. Higher bulk density results in more compaction of the soil which makes it difficult for the roots to penetrate and expand thus the fine roots generally decline with increasing bulk density. The release of nutrients through litter decomposition and fluxes from the roots, results in higher quantities of the soil micronutrients such as carbon and nitrogen in the surface layer which subsequently decline in the deeper soil depths which may be the reason for declining biomass and production of fine roots (Bibi et al., 2022).
Soil pH also showed negative correlation with sand content, clay content, soil moisture content and fine root biomass and production of associated species. Bulk density and porosity were negatively correlated. Soil moisture content and water holding capacity of the soil showed negative correlation with silt content, while positive with clay content of the soil. The fine root parameters also showed positive correlation with sand content and soil organic matter, while negative correlation with clay content but the relationship was not significant. Sandy soils are considered to be more resource limited in comparison to clay or loam and the nutrient deficient soil promotes the growth and expansion of belowground components resulting in higher fine root biomass and production in sandy soil (Mosquera and Moreno-Hurtado, 2022). The higher organic matter contents in the soil results in improved water holding capacity thus helps in expansion of the root system (Lorenz et al., 2019). The resource availability in the soil regulates the fine root distribution and fine root biomass and it is positively correlated with soil organic carbon and negatively correlated with bulk density of the soil (Chen et al., 2016).
Fine root parameters showed significant (p < 0.05) variability among locations, seasons, years and their interactions (Table 5). These observations may be due to the changes in soil characteristics, microclimatic conditions, composition of vegetation that influences the temperature, moisture and nutrient availability and results in different fine root concentration and distribution site-wise and season-wise (An and Osawa, 2021). The highest fine root biomass and production observed during the rainy season is attributable to higher precipitation, suitable temperature for microbial activities resulting in ample nutrients through decomposition processes and exudates that supported the growth of fine roots. The low precipitation and temperature during the winter season resulted in low microbial activities; slower decomposition rates that might have subjected the fine roots to drought stress and reduce their growth.
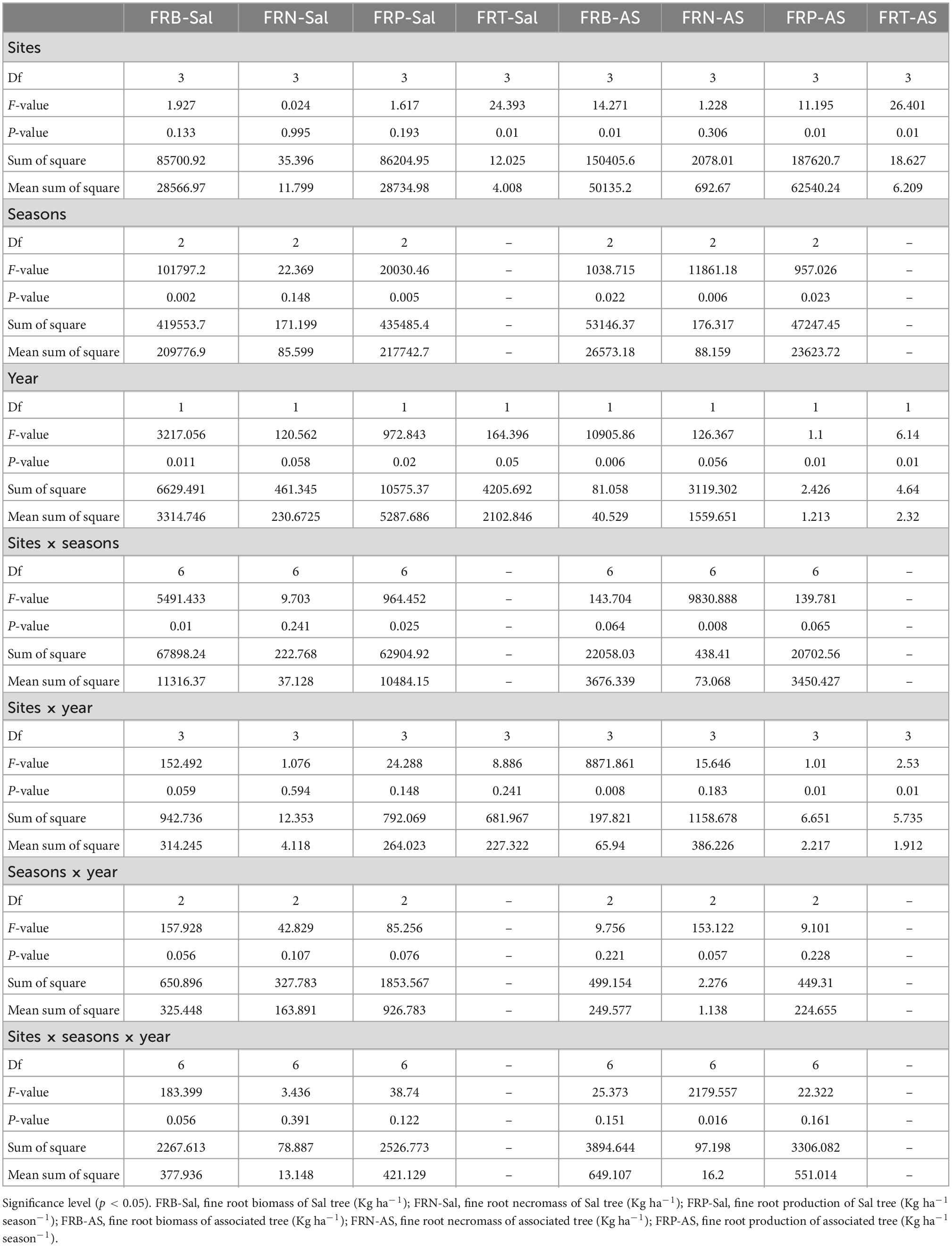
Table 5. Multivariate analysis of variance (MANOVA) table for fine root dynamics with sites, seasons, year and their interactions (Df, degrees of freedom).
In the present study, fine root parameters were also affected by stand density and basal area. The fine root biomass, necromass, production as well as turnover of the associated tree species significantly (p < 0.05) varied with tree density and total basal area, while in S. robusta significant variability with respect to density and basal area was only observed for fine root turnover (Table 6). The reason may be that the density and basal area of a forest regulate the quality and quantity of litter which in-turn manages the nutrient availability such as carbon and nitrogen inputs for growth of the inhabiting species resulting in expansion of root system (Verma et al., 2021). The above-ground and below-ground partitioning of biomass and resources is dependent on the stand density which regulates the ecosystem functioning and nutrient uptake and higher density results in higher fine root biomass (Ile et al., 2021). Zhou et al. (2018) also reported significant relationships between tree basal area and fine root biomass and considered tree basal area is an efficacious variable to estimate the fine root biomass in forest ecosystems.
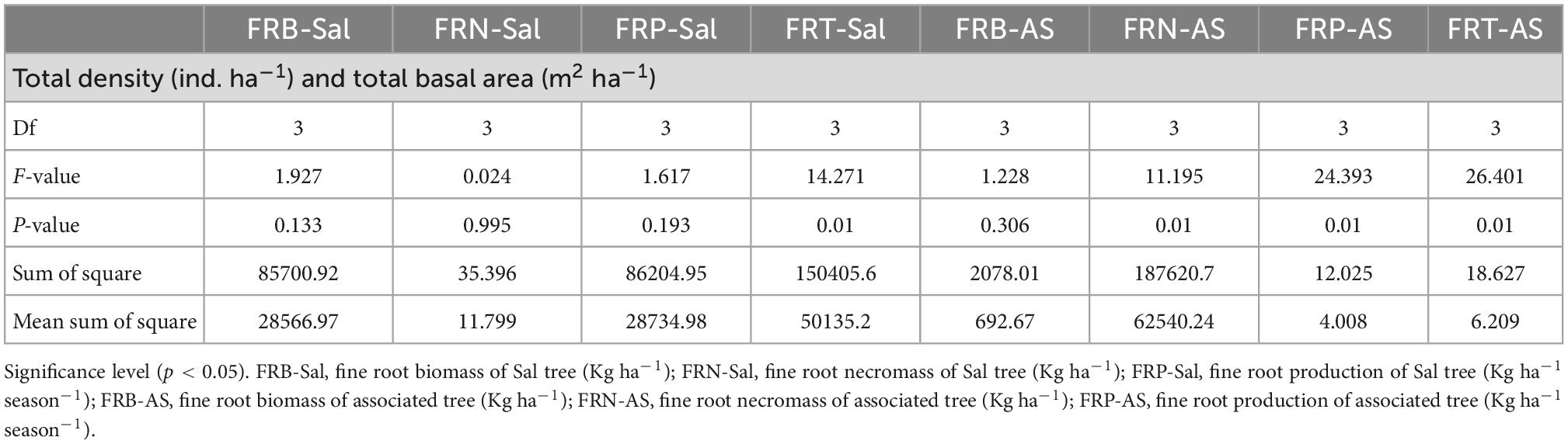
Table 6. One-way Analysis of variance (ANOVA) table for fine root dynamics with tree density and total basal area (Df, degrees of freedom).
Caveat of the study
The study enhanced the understanding on fine root dynamics and spatio-temporal relationship between fine roots and nutrients, but still extensive detailed studies covering wider spectrum, focusing on effect of anthropogenic disturbances, biotic and abiotic variations on fine roots and linking nutrient fluxes to biogeochemical cycling would be beneficial in developing overall ecosystem-scale understanding and management of forest ecosystems.
Conclusion
The study revealed that the fine root distribution in sub-tropical Sal forests was significantly affected by soil physical and chemical characteristics. The fine root dynamics of dominant as well as co-dominant tree species were significantly influenced by the site characteristics, distance, soil depth, seasons and climatic variables. At all the sites, fine root biomass and production was higher in the uppermost (0–20 cm) soil layer, which subsequently declined in the deeper soil depths (20–60 cm). Across the seasons a peak value was recorded in the rainy season due to better microclimatic conditions and nutrient availability. Fine root turnover showed inconsistent patterns across the depths and seasons. Fine roots play a cardinal role in return of nutrients to the soil which enriches the soil quality. The annual flux of carbon and nitrogen recorded in this study verified that the fine roots of the forests have a substantial role in conservation of huge quantities of carbon in the form of biomass, production, thus reducing the atmospheric carbon concentrations and through planning proper forest management regimes this potential can be harvested to mitigate atmospheric carbon contents.
Data availability statement
The original contributions presented in this study are included in this article/supplementary material, further inquiries can be directed to the corresponding author.
Author contributions
RP collected the data and prepared the manuscript. SB guided the research and edited the manuscript. KB reviewed, modified, and improved the manuscript. HK revised and edited the manuscript. MK and US helped in editing the manuscript. All authors contributed to the article and approved the submitted version.
Acknowledgments
We are grateful to the Head, Department of Botany for providing lab facilities and Uttarakhand Tea Development Board Bhowali, Nainital for analysis of soil samples.
Conflict of interest
The authors declare that the research was conducted in the absence of any commercial or financial relationships that could be construed as a potential conflict of interest.
Publisher’s note
All claims expressed in this article are solely those of the authors and do not necessarily represent those of their affiliated organizations, or those of the publisher, the editors and the reviewers. Any product that may be evaluated in this article, or claim that may be made by its manufacturer, is not guaranteed or endorsed by the publisher.
References
An, J. Y., and Osawa, A. (2021). Seasonal patterns of fine root dynamics and their contribution to net primary production in Hinoki cypress (Chamaecyparis obtusa) and Konara oak (Quercus serrata) forests. Trees 35, 255–271. doi: 10.1007/s00468-020-02030-6
Awasthi, P., Bargali, K., Bargali, S. S., and Jhariya, M. K. (2022a). Structure and functioning of Coriaria nepalensis dominated shrublands in degraded hills of Kumaun Himalaya. I. Dry matter dynamics. Land Degrad. Dev. 33, 1474–1494. doi: 10.1002/ldr.4235
Awasthi, P., Bargali, K., Bargali, S. S., and Khatri, K. (2022c). Nutrient return through decomposing Coriaria nepalensis litter in degraded hills of Kumaun Himalaya. India. Front. For. Glob. Change 5:1008939. doi: 10.3389/ffgc.2022.1008939
Awasthi, P., Bargali, K., Bargali, S. S., Khatri, K., and Jhariya, M. K. (2022b). Nutrient partitioning and dynamics in Coriaria nepalensis Wall dominated shrublands of degraded hills of Kumaun Himalaya. Front. For. Glob. Change 5:913127. doi: 10.3389/ffgc.2022.913127
Bargali, K., Manral, V., Padalia, K., Bargali, S. S., and Upadhyay, V. P. (2018). Effect of vegetation type and season on microbial biomass carbon in Central Himalayan forest soils. India. CATENA 171, 125–135. doi: 10.1016/j.catena.2018.07.001
Bargali, S. S., Padalia, K., and Bargali, K. (2019). Effects of tree fostering on soil health and microbial biomass under different land use systems in Central Himalaya. Land Degrad. Dev. 30, 1984–1998. doi: 10.1002/ldr.3394
Bibi, F., Tomlinson, K. W., Liu, C., Liu, C., Jin, Y., and Tang, J. (2022). Fine root production and soil available nutrients in Rubber monoculture versus Rubber–Flemingia macrophylla agroforestry. Forests 13:830. doi: 10.3390/f13060830
Chazdon, R. L. (2019). Towards more effective integration of tropical forest restoration and conservation. Biotropica 51, 463–472.
Chazdon, R. L., Lindenmayer, D., Manuel, R., Guariguata, M. R., Crouzeilles, R., Benayas, J. M. R., et al. (2020). Corrigendum: Fostering natural forest regeneration on former agricultural land through economic and policy interventions. Environ. Res. Lett. 15:099501. doi: 10.1088/1748-9326/ab97cc
Chazdon, R., and Brancalion, P. (2019). Restoring forests as a means to many ends. Science 365, 24–25.
Chen, L., Mu, X., Yuan, Z., Deng, Q., Chen, Y., Yuan, L. Y., et al. (2016). Soil nutrients and water affect the age-related fine root biomass but not production in two plantation forests on the Loess Plateau. China. J. Arid. Environ. 135, 173–180. doi: 10.1016/j.jaridenv.2016.09.003
Cordeiro, A. L., Norby, R. J., Andersen, K. M., Valverde-Barrantes, O., Fuchslueger, L., Oblitas, E., et al. (2020). Fine-root dynamics vary with soil depth and precipitation in a low-nutrient tropical forest in the Central Amazonia. Plant Environ. Int. 1, 1–14. doi: 10.1002/pei3.10010
Cornejo, N. S., Leuschner, C., Becker, J. N., Andreas, H., Costa, D. S., and Hertel, D. (2021). Climate implications on forest above- and belowground carbon allocation patterns along a tropical elevation gradient on Mt. Kilimanjaro (Tanzania). Oecologia 195, 797–812. doi: 10.1007/s00442-021-04860-8
Cusack, D. F., Addo-Danso, S. D., Agee, E. A., Andersen, K. M., Arnaud, M., Batterman, S. A., et al. (2021). Tradeoffs and synergies in tropical forest root traits and dynamics for nutrient and water acquisition: Field and modeling advances. Front. For. Glob. Change 4:704469. doi: 10.3389/ffgc.2021.704469
Dahlmand, R. C., and Kucera, C. L. (1965). Root productivity and turnover in native Prairie. Ecology 46, 84–89. doi: 10.2307/1935260
Ding, Y., Leppalammi-Kujansuu, J., and Helmisaari, H. S. (2019). Fine root longevity and below-and aboveground litter production in a boreal Betula pendula forest. For. Ecol. Manag. 431, 17–25. doi: 10.1016/j.foreco.2018.02.039
Finer, L., Ohashi, M., Noguchi, K., and Hirano, Y. (2011). Fine root production and turnover in forest ecosystems in relation to stand and environmental characteristics. For. Ecol. Manag. 262, 2008–2023. doi: 10.1016/j.foreco.2011.08.042
Finer, L., Zverev, V., Palviainen, M., Romanis, T., and Kozlov, M. V. (2019). Variation in fine root biomass along a 1000 km long latitudinal climatic gradient in mixed boreal forests of North-East Europe. For. Ecol. Manag. 432, 649–655. doi: 10.1016/j.foreco.2018.09.060
Frouz, J., Prach, K., Pizl, V., Hanel, L., Stary, J., Tajovsky, K., et al. (2008). Interactions between soil development, vegetation and soil fauna during spontaneous succession in post mining sites. Eur. J. Soil Biol. 44, 109–121. doi: 10.1016/j.ejsobi.2007.09.002
Fu, X., Wang, J., Di, Y., and Wang, H. (2015). Differences in fine-root biomass of trees and understory vegetation among stand types in subtropical forests. PLoS One 10:e0128894. doi: 10.1371/journal.pone.0128894
Garkoti, S. C. (2011). Fine root dynamics in three Central Himalayan high elevation forests ranging from closed canopied to open-canopied treeline vegetation. J. For. Res. 16, 136–143. doi: 10.1007/s10310-010-0218-5
Garkoti, S. C. (2012). Dynamics of fine root N, P and K in high elevation forests of Central Himalaya. For. Stud. China 14, 145–151. doi: 10.1007/s11632-012-0203-5
Gautam, T. P., and Mandal, T. N. (2018). Storage and flux of nutrients in disturbed and undisturbed Tropical moist forest of Eastern Nepal. Hindawi Int. J. For. Res. 4, 1–12. doi: 10.1155/2018/8516321
Hertel, D., Harteveld, M., and Leuschner, C. (2009). Conversion of a tropical forest into agroforest alters the fine root-related carbon flux to the soil. Soil Biol. Biochem. 41, 481–490. doi: 10.1016/j.soilbio.2008.11.020
Ibrahim, F., Adu-Bredu, S., Addo-Danso, S. D., Duah-Gyamfi, A., Manu, E. A., and Malhi, Y. (2020). Patterns and controls on fine-root dynamics along a rainfall gradient in Ghana. Trees 34, 917–929. doi: 10.1007/s00468-020-01970-3
Ile, O. J., Aguilos, M., Morkoc, S., Heitman, J., and King, J. S. (2021). Root biomass distribution and soil physical properties of short-rotation coppice American sycamore (Platanus occidentalis L.) Grown at different planting densities. Forests 12:1806. doi: 10.3390/f12121806
Joshi, R. K., and Garkoti, S. C. (2021). Influence of Nepalese alder on soil physico-chemical properties and fine root dynamics in white oak forests in the Central Himalaya. India. Catena 200:105140. doi: 10.1016/j.catena.2020.105140
Karki, H., Bargali, K., and Bargali, S. S. (2021a). Nitrogen mineralization patterns in Populus deltoides and Tectona grandis based agrisilvicultural practices in Central Himalaya, India. Vegetos 34, 86–93. doi: 10.1007/s42535-021-00195-0
Karki, H., Bargali, K., and Bargali, S. S. (2021b). Spatial and seasonal pattern of fine root biomass and turnover rate in different land use systems in Central Himalaya. India. Russ. J. Ecol. 52, 36–48. doi: 10.1134/S1067413621010070
Karki, H., Bargali, K., and Bargali, S. S. (2021c). Spatial and temporal trends in soil N-mineralization rates under the agroforestry systems in bhabhar belt of Kumaun Himalaya, India. Agrofor. Syst. 95, 1603–1617. doi: 10.1007/s10457-021-00669-9
Karki, H., Bargali, K., and Bargali, S. S. (2022). Dynamics of fine root and soil nitrogen in Mangifera indica based agroforestry systems in Central Himalaya, India. Land Degrad. Dev. 33, 3523–3538. doi: 10.1002/ldr.4406
Katayama, A., Kho, L. K., Makita, N., Kume, T., Matsumoto, K., and Ohashi, M. (2019). Estimating fine root production from ingrowth cores and decomposed roots in a Bornean tropical rainforest. Forests 10, 1–13. doi: 10.3390/f10010036
Keller, A., Brzostek, E. A., Craig, M. E., Fisher, J. B., and Phillips, R. P. (2021). Root-derived inputs are major contributors to soil carbon in temperate forests, but vary by mycorrhizal type. Ecol. Lett. 24, 626–635. doi: 10.1111/ele.13651
Kochsiek, A., Tan, S., and Russo, S. E. (2013). Fine root dynamics in relation to nutrients in oligotrophic Bornean rain forest soils. Plant Ecol. 214, 869–882.
Kumar, B. M. (2000). Ailanthus triphysa in the homegardens of Kerala, India: Occurrence, basal area, average standing stock of wood and diameter structure. Indian J. Agrofor. 2, 49–52.
Lima, T. T. S., Miranda, I. S., and Vasconcelos, S. S. (2010). Effects of water and nutrient availability on fine root growth in eastern Amazonian forest regrowth. Brazil. New Phytol. 187, 622–630. doi: 10.1111/j.1469-8137.2010.03299.x
Lopez, B., Sabate, S., and Gracia, C. A. (2001). Annual and seasonal changes in fine root biomass of Quercus ilex L. Plant Soil 230, 125–134. doi: 10.1023/A:1004824719377
Lorenz, K., Lal, R., and Ehlers, K. (2019). Soil organic carbon stock as an indicator for monitoring land and soil degradation in relation to United Nations’. Sustainable Development Goals. Land Degrad. Dev. 30, 824–838. doi: 10.1002/ldr.3270
Lukac, M. (2012). “Fine root turnover,” in Measuring roots, ed. S. Mancuso (Berlin: Springer), 363–373. doi: 10.1007/978-3-642-22067-818
Lukac, M., and Godbold, D. L. (2010). Fine root biomass and turnover in southern taiga estimated by root inclusion nets. Plant Soil 331, 505–513. doi: 10.1007/s11104-009-0271-z
Ma, Z., and Chen, H. Y. H. (2016). Effects of species diversity on fine root productivity in diverse ecosystems: A global meta-analysis. Global Ecol. Biogeogr. 25, 1387–1396. doi: 10.1111/geb.12488
Malhi, Y., Girardin, C. A., Goldsmith, G. R., Doughty, C. E., Salinas, N., Metcalfe, D. B., et al. (2017). The variation of productivity and its allocation along a tropical elevation gradient: A whole carbon budget perspective. New Phytol 214, 1019–1032. doi: 10.1111/nph.14189
Manral, V., Bargali, K., Bargali, S. S., Jhariya, M. K., and Padalia, K. (2022). Relationships between soil and microbial biomass properties and annual fluxof nutrients in Central Himalayan forests, India. Land Degrad. Dev. 33, 2014–2025. doi: 10.1002/ldr.4283
Mikieleko, E. F. K., Bocko, Y. E., Loubota-Panzou, G. J., and Loumeto, J. J. (2021). Fine roots dynamics in two forest strata of a semi-deciduous forest in Northern Republic of Congo. Open J. For. 11, 192–205. doi: 10.4236/ojf.2021.113013
Mosquera, H. Q., and Moreno-Hurtado, F. H. (2022). Effects of nutrient (N, P, K) fertilization on the dynamics of fine roots in tropical rain forests with different soil texture in the Colombian Pacific region. Rev. Biol. Trop. 70, 482–494. doi: 10.15517/rev.biol.trop.2022.47351
Oraon, P. R., Singh, L., and Jhariya, M. K. (2018). Forest floor biomass, litterfall and physico-chemical properties of soil along the anthropogenic disturbance regimes in tropics of Chhattisgarh. India. J. For. Environ. Sci. 34, 359–375. doi: 10.7747/JFES.2018.34.5.359
Pausch, J., and Kuzyakov, Y. (2018). Carbon input by roots into the soil: Quantification of rhizodeposition from root to ecosystem scale. Glob. Change Biol. 24, 1–12. doi: 10.1111/gcb.13850
Pei, Y., Lei, P., Xiang, W., Ouyang, S., and Xu, Y. (2018). Effect of stand age on fine root biomass, production and morphology in Chinese fir plantations in subtropical China. Sustainability 10:2280. doi: 10.3390/su10072280
Persch, S., Clendenning, J., Dawson, L., and Jourdan, C. (2015). Fine root dynamics within land-use change from tropical forests to agriculture: A systematic review protocol. Working Paper No. 200. Bogor: Center for International Forestry Research (CIFOR), 18. doi: 10.17528/cifor/005912
Rosado, B. H. P., Martins, A. C., Colomeu, T. C., Oliveira, R. S., Joly, C. A., and Aidar, M. P. M. (2011). Fine root biomass and root length density in a lowland and a montane tropical rain forest, SP, Brazil. Biota Neotr. 11, 203–209. doi: 10.1590/S1676-06032011000300018
Sahu, K. P., Singh, L., and Jhariya, M. K. (2013). Fine root biomass, forest floor and nutrient status of soil in an age series of teak plantation in dry tropics. The Bioscan 8, 1149–1152.
Singha, D., Brearley, F. Q., and Tripathi, S. K. (2020). Fine root and soil nitrogen dynamics during stand development following shifting agriculture in Northeast India. Forests 11:1236. doi: 10.3390/f11121236
Subbiah, B. V., and Asija, G. L. (1956). A rapid procedure for the determination of available nitrogen in soil. Curr. Sci. 25, 259–260.
Usman, S., Rawat, Y. S., Singh, S. P., and Garkoti, S. C. (1997). Fine root biomass production and turnover in evergreen forests of Central Himalaya. India. Oecol. Mont. 6, 4–8.
Usman, S., Singh, S. P., Rawat, Y. S., and Bargali, S. S. (2000). Fine root decomposition and nitrogen mineralisation patterns in Quercus leucotrichophora and Pinus roxburghii forests in Central Himalaya. For. Ecol. Manag. 131, 191–199. doi: 10.1016/S0378-1127(99)00213-3
Verma, A., Garkoti, S., Singh, S., Pal, S., and Kumar, M. (2021). Fine root production and nutrient dynamics in relation to stand characteristics of chir pine mixed banj oak forests in central Himalaya. Flora 279:151808. doi: 10.1016/j.flora.2021.151808
Walkley, A., and Black, I. A. (1934). An examination of Degtjareff method for determining soil organic matter and the proposed modification of the chromic acid titration method. Soil Sci. 37, 29–38. doi: 10.1097/00010694-193401000-00003
Wang, W., Mo, Q., Han, X., Hui, D., and Shen, W. (2019). Fine root dynamics responses to nitrogen addition depend on root order, soil layer, and experimental duration in a subtropical forest. Biol. Fert. Soils 55, 723–736. doi: 10.1007/s00374-019-01386-3
Wang, W., Wu, X. G., Hu, K., Liu, J. C., and Tao, J. P. (2016). Understorey fine root mass and morphology in the litter and upper soil layers of three Chinese subtropical forests. Plant Soil 406, 219–230. doi: 10.1007/s11104-016-2878-1
Wapongnungsang Tripathi, S. K. (2019). Fine root growth and soil nutrient dynamics during shifting cultivation in tropical semi-evergreen forests of northeast India. J. Environ. Biol. 40, 45–52. doi: 10.22438/jeb/40/1/MRN-813
Weemstra, M., Kiorapostolou, N., Ruijven, J., Mommer, L., Vries, J., and Sterck, F. (2020). The role of fine-rootmass, specific root length and life span in tree performance: A whole-tree exploration. Funct. Ecol. 34, 575–585. doi: 10.1111/1365-2435.13520
Xia, M., Talhelm, A. F., and Pregitzer, K. S. (2015). Fine roots are the dominant source of recalcitrant plant litter in sugar maple-dominated northern hardwood forests. New Phytol. 208, 715–726. doi: 10.1111/nph.13494
Yang, Y. S., Chen, G. S., He, Z. M., Chen, Y. X., and Guo, J. F. (2002). Production, distribution and nutrient return of fine roots in a mixed and a pure forest in subtropical China. Chinese J. Appl. Environ. Biol. 8, 223–233.
Keywords: fine root dynamics, Central Himalaya, ingrowth core, turnover rate, carbon flux, forest ecosystems
Citation: Pandey R, Bargali SS, Bargali K, Karki H, Kumar M and Sahoo UK (2023) Fine root dynamics and associated nutrient flux in Sal dominated forest ecosystems of Central Himalaya, India. Front. For. Glob. Change 5:1064502. doi: 10.3389/ffgc.2022.1064502
Received: 08 October 2022; Accepted: 19 December 2022;
Published: 09 January 2023.
Edited by:
Chonggang Xu, Los Alamos National Laboratory (DOE), United StatesReviewed by:
Manoj Kumar Jhariya, Sant Gahira Guru Vishwavidyalaya, IndiaDessie Assefa, Bahir Dar University, Ethiopia
Purabi Saikia, Central University of Jharkhand, India
Copyright © 2023 Pandey, Bargali, Bargali, Karki, Kumar and Sahoo. This is an open-access article distributed under the terms of the Creative Commons Attribution License (CC BY). The use, distribution or reproduction in other forums is permitted, provided the original author(s) and the copyright owner(s) are credited and that the original publication in this journal is cited, in accordance with accepted academic practice. No use, distribution or reproduction is permitted which does not comply with these terms.
*Correspondence: Surendra Singh Bargali, surendrakiran@rediffmail.com
†ORCID: Rachita Pandey, orcid.org/0000-0002-6123-2580; Surendra Singh Bargali, orcid.org/0000-0001-6341-0945; Kiran Bargali, orcid.org/0000-0002-8554-8803; Himani Karki, orcid.org/0000-0001-9288-9748