- 1Swiss Federal Institute for Forest, Snow and Landscape Research (WSL), Birmensdorf, Switzerland
- 2College of Water Sciences, Beijing Normal University, Beijing, China
Winter chilling, spring forcing temperature and photoperiod are the most important drivers explaining the spatial and temporal variability of spring phenology in temperate trees. However, how these factors interact with each other on dormancy release and spring budburst date remains unclear and varies greatly depending on species. Our knowledge is also limited as to whether heat accumulation of forcing temperatures that trigger bud break in spring is a linear or non-linear process. Here, we aimed at experimentally quantifying the effect of chilling, forcing, photoperiod and their interactions on the budburst dates of nine different temperate tree species from East Asia (near Beijing, China) and Central Europe (near Zurich, Switzerland), including six phylogenetically related species (same genus). We conducted a full factorial experiment in climate chambers using two chilling (low and high, i.e., 0 vs. 56 days at 2°C after sampling at the end of December), four forcing (5, 10, 15, and 20°C), and two photoperiod (8 vs. 16 h) treatments simultaneously in Beijing and Zurich. We found that species growing near Beijing responded more readily to forcing conditions than species of the same genus growing near Zurich regardless of chilling treatment. Budburst timing of most species but European beech was marginally, if at all, affected by photoperiod. Furthermore, our results suggest that linear heat accumulation, as commonly used with the growing degree hours (GDH) model, could result in accurate prediction of budburst date depending on the temperature threshold used as a basis for heat accumulation. Our results also demonstrate the important role of chilling in shaping the sensitivity and rate of forcing accumulation to trigger budburst and suggest that species-specific sigmoid relationship for accumulating heat that accounts for prior chilling exposure may yield better predictions of budburst dates. Our results suggest that deciduous trees may have adapted their chilling and forcing requirements in regards to the predictability of winter-spring transition and late spring frosts. A less predictable winter-spring transition, as observed in Central Europe, could have driven species evolution towards higher chilling and forcing requirements compared to species growing in a more predictable climate of Northeastern Asia. Our cross-continental experiment therefore suggests that the spring phenology of East Asian species is tighter coupled to spring forcing temperature than Central European forests.
Introduction
In boreal and temperate regions, the seasonality of the climate imposes trees to optimize growth initiation to avoid frost damages while maximizing the competitiveness for resources (light, nutrient, and water; reviewed in Vitasse et al., 2014). Therefore, temperate trees have developed complex mechanisms to set their spring phenology (reviewed in Delpierre et al., 2016) based on (i) previous exposure to cold temperature conditions (hereafter referred to as “chilling”), (ii) forcing temperatures in spring (temperature generally assumed to be higher than 5°C, hereafter referred to as “forcing”), and for some species (iii) photoperiod. There is ample evidence that all those factors could affect spring phenology of temperate trees (Laube et al., 2014; Flynn and Wolkovich, 2018). For example, temperate and boreal trees require lower forcing to trigger budburst with increasing exposure to chilling conditions (Coville, 1920), with a negative-exponential forcing-chilling relationship commonly found (e.g., Murray et al., 1989; Laube et al., 2014; Baumgarten et al., 2021). Thus, insufficient chilling increases the amount of forcing required for budburst exponentially (Harrington et al., 2010), which could potentially slow-down spring phenology advancement in response to increasing temperature (Fu et al., 2015). Predictions of deciduous tree spring phenology under increasing warming remain therefore challenging and require further experimental insights for a better understanding of winter dormancy progression.
Because of the tight link between temperature and growth, growing degree days (GDD) or growing degree hours (GDH) are common indicators of growth development such as time of budburst in temperate woody species (Murray et al., 1989). Although, the efficiency of forcing temperature accumulation over a temperature range has not yet been experimentally quantified for forest trees, various phenological models assume linear (e.g., GDH) or sigmoid (e.g., unified model) relationships to represent forcing temperature accumulation with increasing air temperature (Chuine, 2000).
Even though temperature (i.e., forcing efficiency and previous chilling) is the most important driver of budburst for most temperate and boreal tree species, budburst timing of some temperate and boreal species has also be shown to be mediated by photoperiod (Basler and Körner, 2012). Reliance on an invariable driver such as photoperiod could prevent from potential frost damage or resource deficiency in the early growth stage due to too early or too late budburst (Körner and Basler, 2010; Fu et al., 2019a), which is critical for temperate species, as any damage on young leaves can have several long-lasting effects (e.g., depletion of reserves, competition for light, water and nutrients, vulnerability to pathogens) that may determine the trajectory of species composition at saplings stages. In fact, longer photoperiods were found to speed up both dormancy release and budburst timing of various species (Hänninen, 1990; Partanen et al., 1998; Basler and Körner, 2012). Similarly, shorter photoperiod would prevent too precocious budburst under warmer spring conditions in regards to late spring frosts (Fu et al., 2019b). In addition, for some species, photoperiodic control was found to gain importance with decreasing chilling exposure (Malyshev et al., 2018).
Hence, photoperiodic sensitive species are likely to be more constraint in their adaptation to rising spring temperatures compared to photoperiodic insensitive species in order to benefit from a longer favorable window for their development. However, the photoperiodic sensitivity of species is difficult to quantify and seems to be more prevalent for species growing in ecosystems with shorter winters (Zohner et al., 2016, 2017; Geng et al., 2022) 2). Consequently, further warming, could result in contrasting effects within and between ecosystems depending on species specific chilling, forcing and photoperiod requirements and the ability to compensate deficient drivers, which might also change the susceptibility of late-spring frost damages in a given ecosystem.
Regions with a distinct transition from cool winter to warm summer might favor species that readily react to forcing temperatures whereas regions with high temperature fluctuation during the winter spring transition (from year to year but also within the season) might favor species that rely on additional cues such as chilling temperatures and/or photoperiod (Zohner et al., 2017; Geng et al., 2022). Consistently, North American trees seem to have a higher chilling and forcing requirement than Central European and East Asian species, matching the long-term risk of damaging frosts in these regions (Zohner et al., 2020). However, there are only few studies that have attempted to experimentally disentangle the effects of native climate and phylogeny (Gao et al., 2022). Here, we aimed to examine the effects of chilling, forcing and photoperiod, including their interactions, on budburst timing of nine different temperate tree species growing near Beijing in China and Zurich in Switzerland, with three pairs of phylogenetically related species (i.e., species belonging to the same genus). We used twigs cuttings subjected to either short or long chilling duration and exposed them into different constant forcing conditions in climate chambers set at 5, 10, 15, and 20°C with either short (8 h) or long (16 h) photoperiod. This experimental approach allowed us to test whether (i) phenological sensitivity to abiotic drivers has a phylogenetic component, (ii) the accumulation of forcing temperatures to trigger budburst follows a linear relationship, and (iii) to what extent forcing accumulation is mediated by chilling and/or photoperiod.
We expect species growing in a more predictable climate in terms of spring frosts with faster winter spring transition (i.e., Beijing region, Figure 1) to exhibit lower chilling and forcing requirements than species growing in a less predictable climate with longer winter spring transition with more temperature fluctuation around the freezing point (i.e., Zurich region). We expect an almost linear accumulation of forcing temperature to trigger budburst once chilling requirement is fulfilled, i.e., similar GDH at the time of budburst in the different forcing conditions for a given species. However, under limited chilling conditions, we predict higher GDH at the time of budburst compared to previous longer chilling conditions and expect GDH to increase with increasing forcing temperatures, especially for species with high chilling requirement, due to further completion of chilling requirement at lower forcing temperatures (for the forcing treatment 5°C and 10°C).
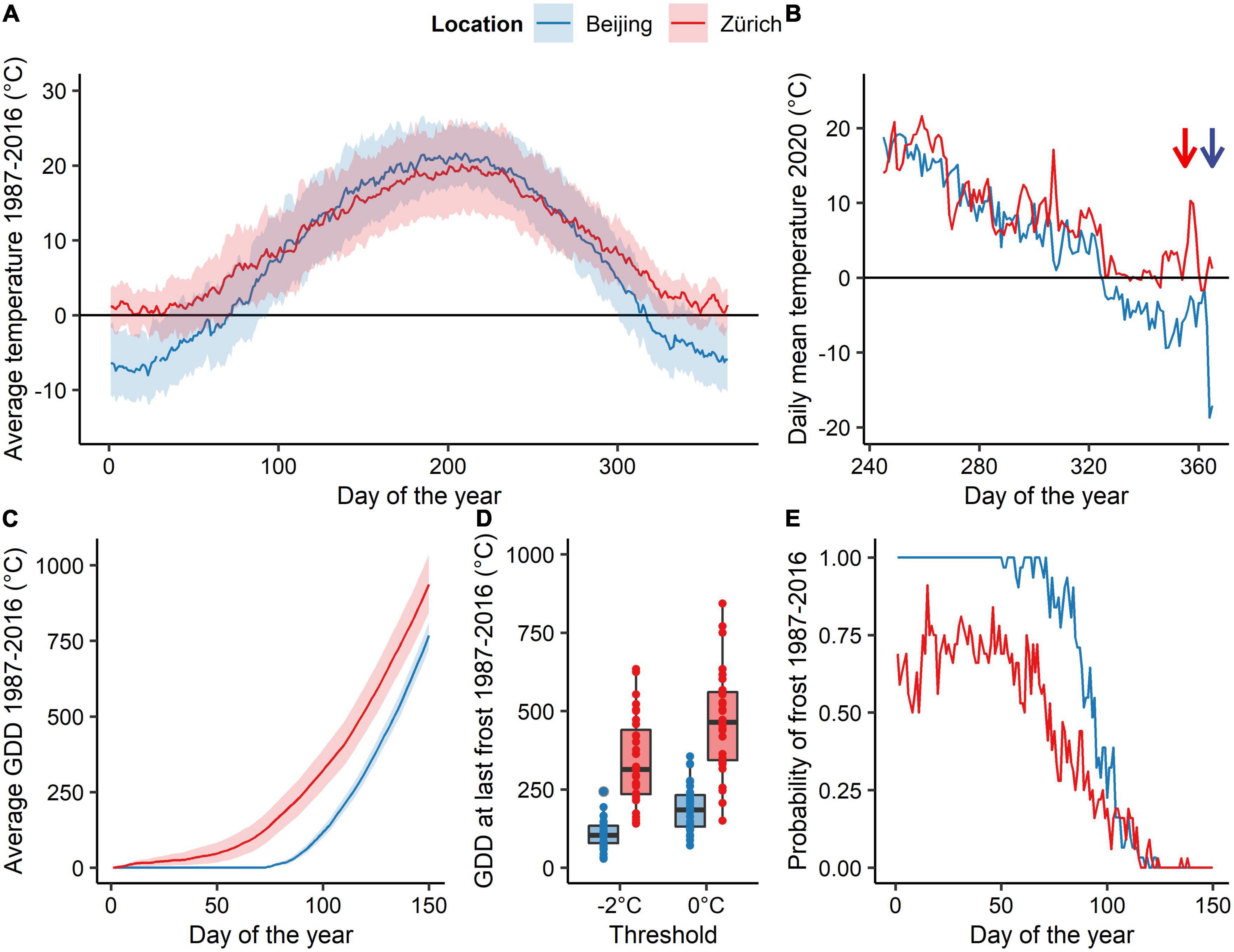
Figure 1. Climatic characteristics of the study sites near Beijing and Zurich. Data derive from one weather station closely located to the sampling area (A). Annual course of the average mean, minimum and maximum temperature, (B) daily average temperature of the autumn 2020 at the two study sites: The sampling dates (i.e., DoY = 350 for Zurich and DoY = 363 and 365 for Beijing) are indicated by arrows of the corresponding color. (C) Average GDD (mean ± se; threshold 0°C) accumulated during spring. (D) Accumulated growing degree days (threshold 0 °C) at the last spring frost of the year (either below −2°C or 0°C) during the period 1987-2016. (E) Long-term daily frost probability (i.e., daily minimum Temperatures < 0°C) during spring for the period 1987-2016.
Materials and methods
Study species and sites
We selected six common deciduous forest tree species in Switzerland and three common deciduous forest tree species in China with contrasting spring phenology. In Switzerland the species consisted of six species (i.e., Acer pseudoplatanus L., Betula pendula Roth and Carpinus betulus L., Fagus sylvatica L., Quercus robur L., and Tilia cordata Mill.), whereas in China the species consisted of three species, (i.e., Acer truncatum Bunge, Betula dahurica Pall. and Carpinus turczaninowii Hance). For clarity and brevity, hereafter, we refer to each species by its English name, i.e., Shantung maple (Acer truncatum), sycamore maple (Acer pseudoplatanus), Dahurian birch (Betula dahurica), silver birch (Betula pendula), Turczaninow hornbeam (Carpinus turczaninowii), European hornbeam (Carpinus betulus), European beech (Fagus sylvatica), Pedunculate oak (Quercus robur) and Small-leaved lime (Tilia cordata).
Central European species were sampled in a mature mixed forest near Zurich, Switzerland (47°21′ N, 8°27′ E; 580 m a.s.l.). The mean annual temperature of the site is 9.8°C (data from 1987 to 2016 weather station Zurich/Kloten, 428 m a.s.l. ∼15 km away from the study site). East Asian species’ twig cuttings were sampled in a mature mixed forest in the Jiufeng mountains in north-eastern China (40° 3′ N, 116° 5′ E; 1040 m a.s.l.) for Dahurian birch and Shantung maple, whereas Turczaninow hornbeam was sampled at lower elevation (∼650 m a.s.l.). The mean annual temperature of the higher Chinese site was 7.7 °C. For the Chinese sites typical near-surface air temperature lapse rates of the region (0.4°C per 100 m during winter and 0.6°C per 100 m during the rest of the year) were used to correct for differences in elevation between the sampling site and the weather station (Li et al., 2013). The long-term air temperature (1987-2016) over the winter season (December–February) was 1.4°C (−1.9 and 4.4°C; average minimum and maximum, respectively) in Zurich and −5.5°C (−9.4 and −0.1 °C) in the Jiufeng Mountains (Figure 1A). From beginning of February to end of April the average air temperature rose from 0.2 ± 4.9°C (mean ± sd) to 11.9 ± 3.3°C in Zurich and from −5.7 ± 2.6 to 12.8 ± 3 in the Jiunfeng Mountains.
Sampling
The sampling took place on 16 December 2020 in Switzerland and on 29 and 31 December 2020 in China (Figure 1B). For each species, we selected 8 healthy adult trees (>10 m height, > 30 years old) and harvested 16 twigs of ∼60 cm length with healthy buds per individual (i.e., a total of 768 twigs at the Swiss site and 384 twigs at the Chinese site). Twigs were harvested at the south-facing side of the crown using a 7-m long pole pruner. Twig cuttings were put into wet plastic bags in the field (to prevent desiccation) and recut at the bottom before placing them in tap water on the same day. The twigs were kept in a climate chamber set to 2°C (Switzerland) or in a laboratory at 6°C (China) over night. Then, all remaining leaves were removed manually to prevent desiccation, twigs were pruned to a length of ∼50 cm and labeled and placed into plastic boxes with deionized water to minimize the development of fungi and bacteria on the next day. Before the different treatments started on 17 December 2020 in Switzerland and on 1 January 2021 in China.
Experimental design: Chilling, forcing and photoperiod treatments
The effect of chilling, forcing temperature and photoperiod was tested on twigs that were exposed to constant forcing temperatures at 5, 10, 15, and 20°C under either 8h or 16h photoperiod (i.e., 8 treatments) immediately after the sampling, referred hereafter to as low chilling conditions (only natural chilling accumulated at the sampling date), and after keeping them at approximately 2°C (Switzerland: 1.2 ± 0.4 °C, China: 2.0 ± 0.7 °C) and 8h photoperiod for eight weeks, which aims at simulating full natural chilling conditions, referred hereafter to as high chilling treatment (natural chilling accumulated at the sampling date and 56 days of artificial chilling). We used photoperiods that equal the shortest (8 h, winter solstice) and longest (16 h, summer solstice) daylengths possible at the investigated sites to simulate most contrasting photoperiodic conditions.
The eight climate chambers used in Switzerland were equipped with halogen lamps (Philips MASTER TL-D) with photosynthetic photon flux density (PPFD) = ∼100 μmol m2 s–1, and the four climate chambers in China were equipped with LED (Philips Greenpower LED Toplighting) with PPFD = ∼161 μmol m2 s–1. Photoperiod was automatically set to 8 h and 16 h (shortest and longest day at this latitude) in the climate chambers in Switzerland whereas it was manually applied in China using black foil layers within the chambers that covered samples either from 5 pm to 9 am (8 h photoperiod) or from 1 am to 9 am (16 h photoperiod) as described in Wu et al. (2022a).
Vessel occlusion was prevented during the experiment by recutting the base of each cutting by about 0.5 cm and by replacing the deionized water every week for the 15°C and 20°C forcing treatment or every second week for the 5°C and 10°C forcing treatments. Temperatures for all treatments were recorded using HOBO temperature loggers (Switzerland: HOBO MX2203, China: HOBO MX2202, Onset Computer Corporation, Bourne, MA, USA). All climate chambers provided stable temperature treatments, and average temperatures were within ± 1°C of the target value (Supplementary Figure 1).
Phenology monitoring
Bud development was monitored twice a week using a four-stage categorical scale (Vitasse, 2013) at stage 0 (dormant bud), no bud development was visible; at stage 1 (bud swelling), buds were swollen and/or elongating; at stage 2 (budburst), bud scales were open and leaves were partially visible; at stage 3 (leaf-out), leaves had fully emerged from the buds but were still folded, crinkled or pendant, depending on species; and at stage 4 (leaf unfolded), at least one leaf was fully unfolded. The day of year for each stage was recorded for the earliest bud per twig. Each twig was recut and examined for intact buds and vessels at weekly intervals. Most species showed a high cutting survival regardless of the treatment (Supplementary Figure 2). However, low chilling (i.e., samples immediately placed into forcing conditions in December) and exposure to 20°C forcing temperature resulted in relatively high mortality for sycamore maple at both photoperiods and for European beech and small-leaved lime at low photoperiod (8 h). For several species, cuttings were not able to reach the phenological stage 4 at forcing temperature of 5°C. The percentage of buds that reached at least stage 2 was assessed for the Swiss species as a proxy for twig vitality (Supplementary Figure 3). This assessment was done 2 weeks after stage 2 was observed for cuttings exposed to 15 and 20°C forcing condition and 3 weeks after stage 2 was observed for cuttings exposed to 5 and 10°C forcing condition.
Data analysis and statistics
For the analysis, we focused on budburst because this stage represents the earliest clearly visible indicator of leaf-emergence for all species. In addition, later stages may depend on the availability of resources (established water and nutrient fluxes), which may be limited when using twig cuttings (Vitasse and Basler, 2014).
We evaluated the differences between treatments in terms of thermal time to budburst, i.e., the number of days to budburst under a given constant forcing temperatures and by accumulating growing degree hours (GDHs) until budburst for chilling and forcing conditions, using 0°C as forcing accumulation threshold. Temperatures were recorded with a time resolution of 30 min inside each climate chamber. Equation 1 was used to calculate GDH accumulation with t1 corresponding to the starting time of exposure to experimental forcing condition, tBB time of budburst and Tt temperature record at 30 min resolution.
We evaluated climate predictability by analyzing a 30-year time series of 2 m above ground air temperatures from 1987 to 2016 at two weather stations each close to the corresponding sampling sites (Beijing: 39° 56′ N, 116° 17′ E, 54 m a.s.l., Zurich: 47° 21′ N, 8° 27′ E 424 m a.s.l). For this analysis growing degree days (GDDs) have been used, which were calculated similar to GDHs but at daily resolution. Average forcing temperature accumulation, accumulated GDDs at last frost day (i.e., daily minimum temperature < 0 °C or < –2 °C), and frost probability (i.e., daily minimum temperature < 0 °C) were computed per day of the year (DoY; Figures 1C–E).
We fitted Bayesian generalized linear mixed effect models for each species with artificial chilling (2 conditions), forcing temperature (continuous), photoperiod (2 conditions) and every possible two-way interaction as fixed effects and donor tree identity as random effect and normal distributed priors. Bayesian inference models were chosen since they allow the assignment of a probability to a hypothesis using current experimental data (see Ellison, 2004; Trotta, 2008; van de Schoot et al., 2021). The dependent variables (i.e., number of forcing days and GDH accumulation at the stage of budburst) and the independent variable “forcing temperature” were transformed by the natural logarithm to normalize data and to accurately model the non-linear effects of forcing temperature on number of forcing days and accumulated GDH at budburst (see Wolkovich et al., 2021). All models were fit using the R package rstanarm (2.21.1) that provides an interface to the Stan C++ library for Bayesian estimation (Stan Development Team, 2022). Each model was run on four chains with 2000 iterations (400 warm-up iterations) and default priors. Sensitivity analysis show that changing priors do not affect the outcome (Supplementary Figures 4, 5). Ȓs and effective sample sizes (ESS) were used to assess model performance (Supplementary Tables 1, 2). Posterior draws (i.e., model outputs) were retrieved using the bayestestR package (0.11.0) and visualized using the bayesplot package (1.8.1, Supplementary Figures 6, 7). The statistical interpretation of the model was done using Bayes Factors (BF10). All analyses and plots were performed in R (R Core Team, 2022), v.4.1.1. We present median and corresponding 89% credible intervals in all figures and tables but the sensitivity analysis where 50% credible intervals are shown.
Results
Effect of chilling and photoperiod
The number of days at forcing conditions (forcing days) required to budburst significantly differed among species (Figure 2), consistently with the sequence observed in natural conditions. Specifically, we observed species of the Betula and Carpinus genera to bud burst more readily than the other species, regardless of site, with, for example, less than 5 weeks and 2 weeks to budburst when exposed to a forcing temperature of 20°C under low and high pre-chilling conditions, respectively (Supplementary Table 1). In addition, all Asian species showed shorter time to budburst compared to European species of the same genus regardless of chilling, photoperiod and forcing treatment Shantung maple, for instance, required similar time to budburst as silver birch and European hornbeam for most treatments (Figure 2). Overall, Dahurian birch required the least number of forcing days to budburst for any treatment, whereas sycamore maple, European beech and small-leaved-lime required the highest number of forcing days to budburst (Figure 2).
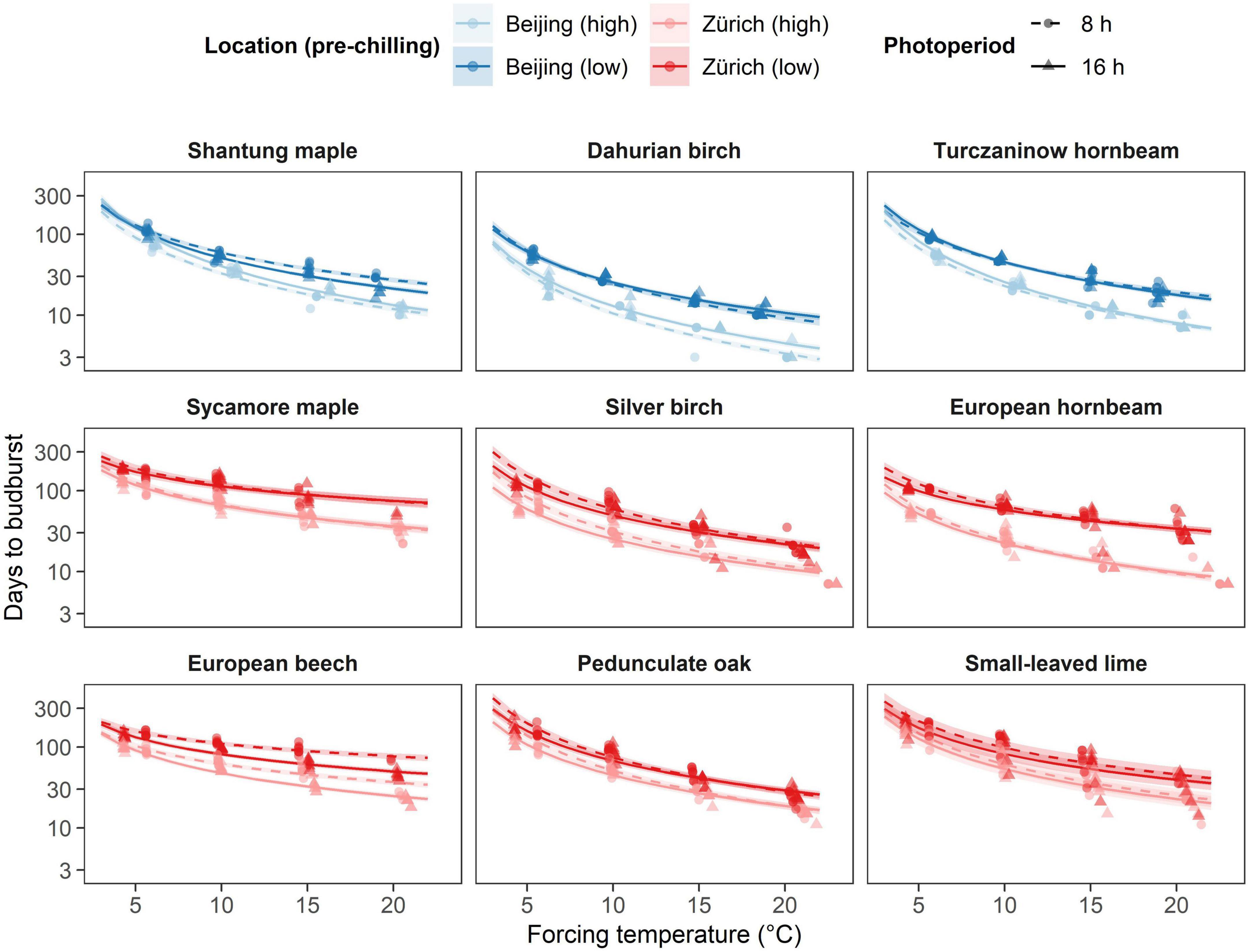
Figure 2. Effect of the different temperature treatments (forcing) on time to budburst (days) under different pre-chilling conditions and photoperiod. The predicted relationships from the final model are represented as solid lines with corresponding 0.89 credible intervals. Chilling treatments and locations are represented by colors, where blue indicated species growing near Beijing and red indicates species growing near Zurich. Pre-chilling conditions are indicated with lower brightness (high pre-chilling) and higher brightness (lower pre-chilling), whereas photoperiodic treatments are represented by solid lines and triangles (16 h photoperiod) and dashed lines and points (8 h photoperiod).
All species decreased the number of forcing days required to budburst with increasing temperature regardless of pre-chilling and photoperiodic condition. In addition, most species required more days to budburst under lower pre-chilling conditions and showed an increasing effect of higher pre-chilling conditions with increasing temperatures. Thus, most of the species responded faster to increasing temperature with additional eight weeks of artificial chilling (Figure 2). European hornbeam for instance required on average 2.4 times less days to budburst at 20°C (9.6 days) compared to 10°C (23.1 days) after higher pre-chilling and 1.8 times less days to budburst at 20°C (33.9 days) compared to 10°C (60.9 days) after lower-prechilling. However, in contrast to the other species, silver birch, pedunculate oak and small-leaved lime responded differently. Silver birch did not show an interaction between pre-chilling condition and forcing temperature, whereas no evidence for any effect of additional eight weeks of artificial chilling was found for pedunculate oak and small-leaved lime (Supplementary Table 1).
All Asian species showed a faster decrease in the number of forcing days with increasing temperature (i.e., higher temperature sensitivity) than European species of the same genus (Figure 2 and Supplementary Table 1). In addition, species from the birch and hornbeam genus and pedunculate oak showed a higher temperature sensitivity than the other species from the same site. Pedunculate oak, however, showed a temperature sensitivity similar to the Asian birch and hornbeam species at lower pre-chilling conditions but a lower temperature sensitivity than any Asian species at higher pre-chilling conditions. European beech showed the lowest temperature sensitivity of all species investigated (Figure 2 and Supplementary Table 1).
Silver birch, European beech and pedunculate oak showed distinct responses to photoperiodic treatments. Longer photoperiod (16 h) compared to a shorter photoperiod (8 h) reduced the number of days to budburst for silver birch regardless of forcing temperature and pre-chilling conditions. European beech showed an increasing photoperiodic effect with increasing temperature, whereas a decreasing photoperiodic effect with increasing temperature was observed for pedunculate oak. After higher pre-chilling conditions, European beech for example required on average 1.4 times more days to budburst at 8 h photoperiod and 20°C forcing temperature (+12 days), and pedunculate oak required 1.3 times more days to budburst at 8h photoperiod and 5°C forcing temperature (+20.9 days) (Figure 2 and Supplementary Table 1).
Growing degree hours requirement to budburst between European and Asian species
Overall, Asian species showed lower forcing requirements than European species of the same genus (Figure 3). This discrepancy vanished between European and Asian hornbeam species, but not for European birch and maple species at higher pre-chilling conditions. Overall, the Asian species Dahurian birch required by far the least growing degree hours (GDH) to budburst irrespective of the treatments, whereas the European species sycamore maple required the highest GDH to budburst at low chilling (Figure 3). Species from the birch and hornbeam genus required less growing degree hours (GDH) to bud burst than the other species of the same origin (Figure 3). High chilling evidently reduced GDH to budburst for most of the species but Dahurian birch, pedunculate oak and small-leaved lime. All maple and hornbeam species and European beech showed a diverging pattern between low and high pre-chilling conditions with increasing temperature. For these species, the effect of longer pre-chilling conditions increases with increasing forcing temperature (Figure 3 and Supplementary Table 2). European hornbeam exhibited stronger response to additional eight weeks of artificial chilling than any other species investigated and was able to reduce GDH by a factor of 2.6 at 20°C forcing temperature. In contrast, almost no difference between chilling treatments was found for Dahurian birch that did not reduce GDH at all at a temperature of 20°C.
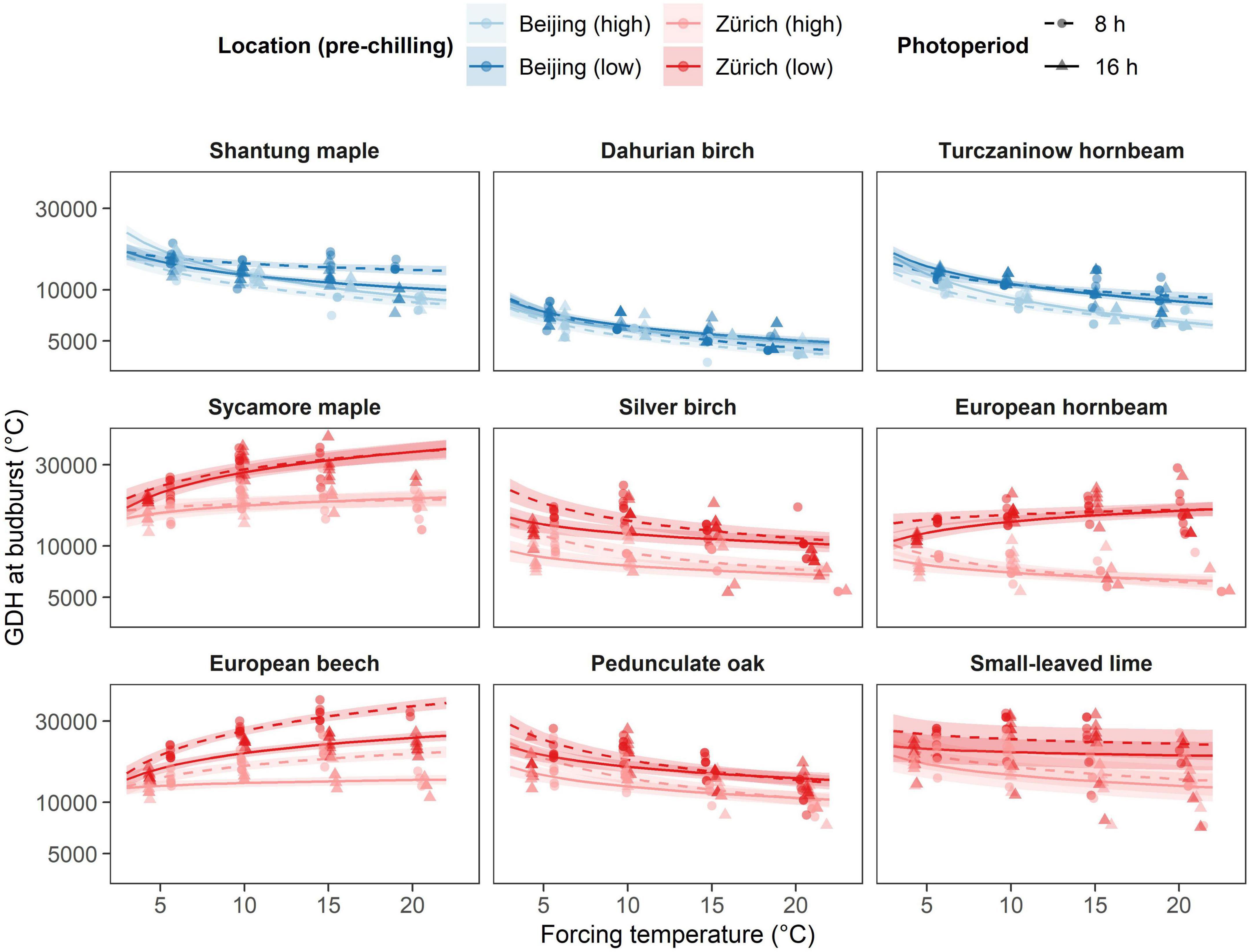
Figure 3. Effect of the different temperature treatments (forcing) on growing degree hours (GDH) under different pre-chilling conditions and photoperiod. The predicted relationships from the final model are represented as solid lines with corresponding 0.89 credible intervals. Chilling treatments and locations are represented by colors, where blue indicated species growing near Beijing and red indicates species growing near Zurich. Pre-chilling conditions are indicated with lower brightness (high pre-chilling) and higher brightness (lower pre-chilling), whereas photoperiodic treatments are represented by solid lines and triangles (16 h photoperiod) and dashed lines and points (8 h photoperiod).
Most species show a non-linear accumulation of forcing temperatures
A linear accumulation of GDH to predict budburst timing would result in similar GDH values across the different treatments. However, we found that all Asian species showed a reduction in GDH requirement with increasing temperature regardless of previous chilling and photoperiodic treatment (Supplementary Table 2 and Figure 3). This trend indicates a non-linear accumulation of forcing temperatures with higher forcing temperatures being more efficient than lower forcing temperatures. By contrasts, European species differed considerably regarding GDH requirement dependent on pre-chilling conditions. Silver birch, pedunculate oak and small-leaved lime decreased their GDH requirement with increasing temperature regardless of previous treatments similar to the Asian species (Figure 3). GDH requirement to budburst for European beech increased with increasing forcing temperature regardless of pre-chilling and photoperiodic conditions, whereas GDH requirement of sycamore maple increased with increasing forcing temperature only after lower pre-chilling conditions. Thus, chilling requirement was likely not fully satisfied for these two species even after high pre-chilling treatment. European hornbeam showed a somewhat intermediate response with GDH requirement increasing with increasing temperature at low pre-chilling conditions, whereas GDH requirement decreases with increasing temperature at high pre-chilling conditions (Figure 3).
In addition, we found a significant and distinct changes in GDH requirement depending on photoperiod for silver birch, pedunculate oak and European beech as already observed for the number of days to budburst (Figure 3). Silver birch required less GDH at 16 h photoperiod compared to 8 h photoperiod regardless of previous chilling and forcing temperature. The photoperiodic effect increased with increasing forcing temperature for European beech and decreased with increasing temperature for pedunculate oak (Figure 3 and Supplementary Table 2). In detail, European beech required on average almost 50% more GDH at 8 h photoperiod and 20°C forcing temperature for both pre-chilling conditions, whereas pedunculate oak required on average 25% more GDH at 8 h photoperiod and 5°C forcing temperature for both pre-chilling conditions.
Discussion
Although many experimental studies have focused on determining the range of chilling and photoperiod responsible for dormancy break (e.g., Malyshev et al., 2018; Fu et al., 2019a), experimental studies aiming at quantifying the efficiency and range of forcing temperature for a given previous chilling conditions and photoperiod are rare and were predominantly run with forcing temperatures slightly (max ∼5°C) warmer than ambient conditions (Flynn and Wolkovich, 2018; Wang et al., 2020). Thus, this cross-continental experimental offers a unique approach to compare the efficiency of different forcing, chilling and photoperiod and disentangle their individual effects on phylogenetically related species from different biogeographical regions using the same experimental settings and a wide range of constant forcing temperatures. Despite the shortcomings in transferring the outcome of this experiment to natural environments, we found that heat requirement to budburst declines substantially with increasing forcing temperature for most species under higher chilling conditions. Therefore, our results demonstrate the diverse, yet universal, effect of chilling on the rate of dormancy release in temperate deciduous tree species. East Asian species exhibited lower forcing requirement compared to European species of the same genus, especially under lower chilling conditions. Furthermore, we demonstrate that photoperiod can influence the amount of GDH required to budburst as well as the relationship between chilling and forcing for a few species, with the strongest effect found for European beech.
The effect of widely varying experimental chilling and forcing conditions
In line with previous experimental studies, we found that almost all tested species had a lower forcing requirement after being exposed to longer chilling conditions, i.e., after eight weeks at 2°C. This suggests that most of the species have not fulfilled their chilling requirement at the end of December, as also found in previous experimental studies (Laube et al., 2014; Man et al., 2017; Baumgarten et al., 2021). Surprisingly, we found distinct different chilling and forcing sensitivities among species of the same genus, which indicates that chilling and forcing requirement for dormancy release and budburst are not strongly conserved phylogenetic traits but rather depend on the native climate where the species has “recently” evolved. For instance, the Asian Shantung maple was the only species that appeared to have met its chilling requirement by the end of December, while sycamore maple, the European relative of Shantung maple, required the highest amount of chilling of all species studied. Birch and hornbeam genera required less forcing than most other species. However, European hornbeam showed high responsiveness to forcing temperatures only after exposure to longer chilling conditions, consistently with previous work (Baumgarten et al., 2021). Rather than a strong phylogenetic component explaining the amount of chilling and forcing required to budburst, we propose that these traits are instead plastic and have been shaped by evolution to match local or regional long-term climate conditions with respect to the risk of damaging late frost (Lenz et al., 2016; Gao et al., 2022). Species from eastern China seem to have both low chilling and forcing requirement, and thus are able to respond quickly to increasing temperatures in spring, which is consistent with the overall background climate that shows a high climate predictability with a fast transition from freezing temperatures to non-freezing temperatures during early spring. In contrast, European species have higher forcing and chilling requirement, ensuring that they do not respond too quickly to enhanced winter or early spring temperatures, as the risk of spring frosts is higher and much less predictable than in East Asia (Figures 1A,E). This fast transition from freezing temperatures to non-freezing temperatures might also explain why East-Asian species were not able to advanced their spring phenology to a stronger extent than Central European species per °C of warming so far (see Vitasse et al., 2009a; Dai et al., 2014). However, a higher winter chilling at the East-Asian site at the sampling date may have additionally magnified the strength of the observed trend (Figure 1B).
The combination of low chilling and high forcing temperatures (i.e., 15°C and 20°C) changed budburst sequence in some species. Pedunculate oak, for instance, substantially decreased its forcing requirement to budburst with increasing forcing temperature. Accordingly, the species required less forcing at high forcing temperatures than European beech for any treatment and less forcing than European hornbeam at low chilling. This finding is consistent with previous work that found European white oaks to respond strongly to warm spring temperatures by advancing budburst timing to a larger extent than silver birch or European beech (Vitasse et al., 2009b; Fu et al., 2013) and to be much less constrained by chilling requirement than European beech (Wenden et al., 2020). Thus, linear forcing accumulation with a base threshold of either 0°C or 5°C neglects the nonlinear forcing accumulation of temperate species at high forcing temperatures and may provide inaccurate predictions of processes happening inside the buds and cells. Therefore, further temperature increase is likely to alter budburst sequence of temperate deciduous trees due to their very specific requirements and sensitivity to chilling, forcing and photoperiod, with stronger phenological shifts expected for species having low chilling requirement and being insensitive to photoperiod (Laube et al., 2014; Hu et al., 2022). In contrast, species with higher chilling requirements and or being photoperiodic sensitive, as European beech, may advance their spring phenology much less, which in turn may affect their competitiveness with other species for a new window of opportunity for resources (light, water, nutrients).
Forcing accumulation, a linear process?
The fact that most species required less GDH at higher constant forcing temperature under higher pre-chilling condition, suggests a nonlinear accumulation of forcing temperature above 5°C with a higher temperature efficiency at higher forcing temperatures. This suggests that sigmoid models that incorporate chilling and forcing are likely better to represent plant physiology and may therefore be more suitable to predict budburst timing of temperate trees (Chuine, 2000). However, this should be taken with caution as a change of the forcing threshold in the GDH models might change the interpretation of temperature efficiency. For example, when changing the temperature threshold from 0°C to 2°C, none of the European species would show an increasing temperature sensitivity with increasing temperature and the effect of high chilling would be stronger for the Asian species (Supplementary Figure 8). This important issue calls for further experimental investigations about the relevant species-specific temperature thresholds that should be used as basis for heat accumulation to predict budburst.
Sycamore maple and European beech required more forcing at medium and high forcing temperatures (10, 15, and 20°C) compared to low forcing temperature (5°C) even after longer chilling exposure. This result is likely due to the high chilling requirement of the two species, which is likely not fully met even after being exposed to the longer pre-chilling treatment (Vitasse and Basler, 2012; Dantec et al., 2014). Because the range of chilling temperature seems to be efficient to release dormancy between −2°C and 10°C (Baumgarten et al., 2021), these species likely benefitted from additional chilling when placed at 5°C and to a lower extent also at 10°C, thereby decreasing the amount of heat required to budburst.
Species sensitivity to photoperiod
European beech was the species showing the most substantial change in temperature sensitivity under the different photoperiod treatments, confirming its sensitivity to photoperiod as previously shown in several experiments (Laube et al., 2014; Fu et al., 2019b). Interestingly, European beech showed a stronger photoperiodic effect at both warm temperatures and/or low chilling, suggesting that high chilling conditions act as a substitute for unmet photoperiodic cues, whereas longer photoperiod could also substitute for a lack of chilling, as shown in previous experiments (Caffarra and Donnelly, 2011; Laube et al., 2014). In addition to European beech, a photoperiodic effect was also found for silver birch and pedunculate oak. However, no relation between phylogeny nor successional stage on photoperiodic sensitivity was found as already emphasized by Way and Montgomery (2015). In fact, silver birch and pedunculate oak were sensitive to photoperiod to a small extent and slightly advanced their phenology from short (8 h) to very long photoperiod (16 h) particularly at low forcing temperatures. This outcome suggests that photoperiod is rather a cue that accelerates spring phenology for most temperate deciduous trees than an important driver per se (Fu et al., 2019a). An important future contribution to the field would be to examine species of the Fagaceae family, which includes the genera oak, beech and chestnut that have colonized large parts of the northern hemisphere. Combined with genetic analyses, it would help to understand how species have developed temperature and photoperiod sensitivity to avoid damaging spring frosts once they colonizing new areas (Zohner et al., 2016; Wu et al., 2022b).
East Asian species probably less vulnerable to late-spring frost damages
The climate of Beijing and its surrounding is continental with a fast transition from cold to warm air temperatures during spring (Figure 1A). Thus, the risk of false springs (i.e., when young leaves get damaged by frost) remain low in East Asia even for pioneer species such as Dahurian birch and Turczaninow hornbeam. This may explain why these species respond much more readily to forcing temperature compared to the European species of the same genus (i.e., silver birch and European hornbeam). In contrast to East Asian species, Central European species are exposed to a less predictable climate with late-spring frost being likely to occur in April and May (Figures 1C–E). Therefore, Central European species have developed a higher heat requirement to budburst which allows them to avoid flushing during unusual warm spells during late winter and early spring (Lenz et al., 2016; Bigler and Bugmann, 2018). A comparison between accumulated forcing at the last frost occurrence (below 0°C air temperature) and predicted forcing requirement to budburst found in our experiment (safety margin) revealed that East-Asian species would be seldom exposed to frost (positive safety margin) even when chilling conditions would be high, whereas Central European species has weaker or even negative safety margins, especially when they would be exposed to longer chilling conditions (Figure 4). This suggests that European tree species may have developed higher chilling requirements than Asian species due to the lower predictability of late spring frost in Central Europe. Nevertheless, the fast winter-spring transition might keep the vulnerability to frost induced damages low for East Asian species. Although beside of escaping freezing damage by being more conservative to increasing temperature with high, chilling and photoperiodic requirements less conservative plants may protect themselves also by producing high freezing resistant buds and leaves as often found for early flushing species (Lenz et al., 2013).
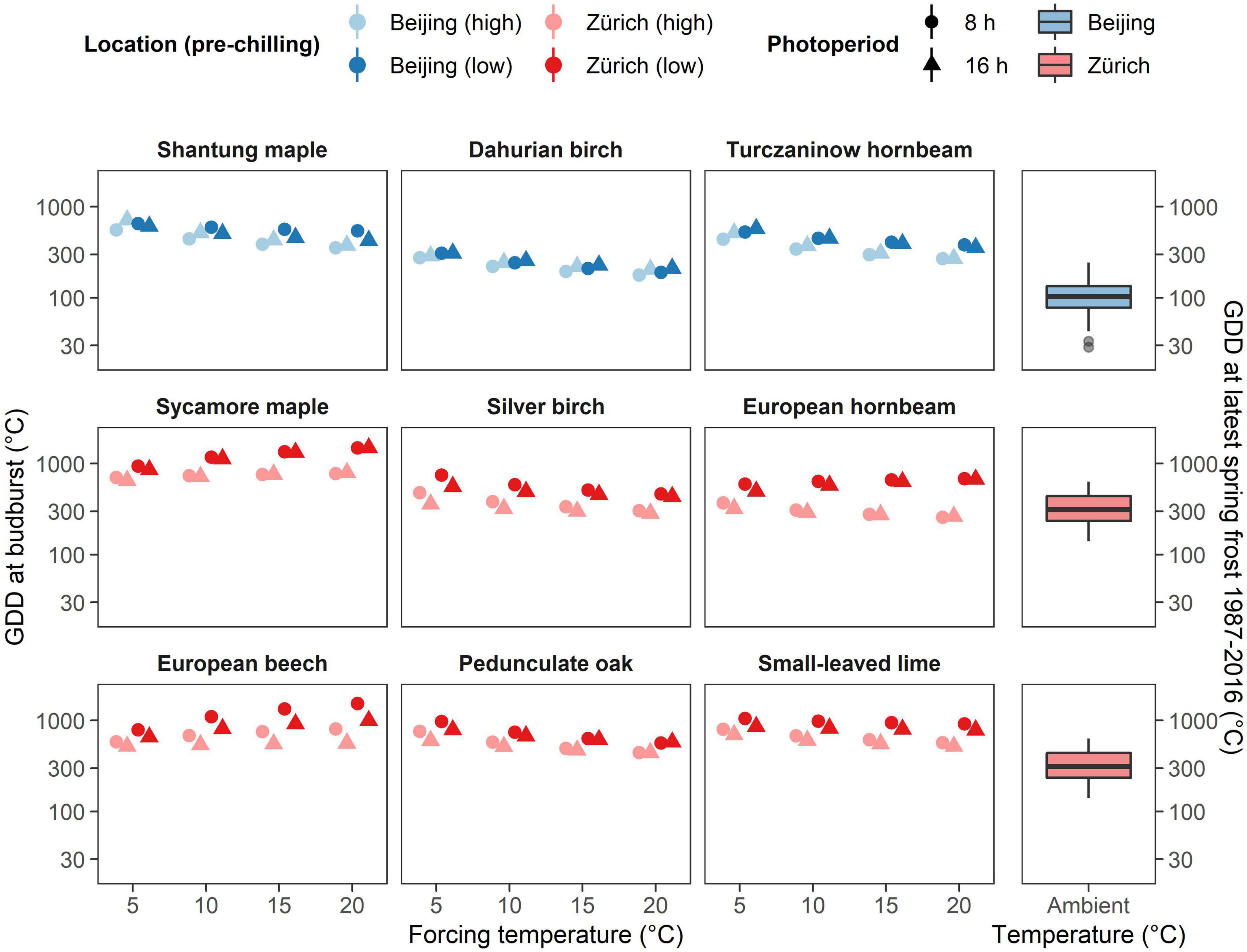
Figure 4. Required GDD to budburst of the nine species investigated under different forcing temperatures, pre-chilling conditions and photoperiod as predicted by the Bayesian mixed effect model (left) and the observed GDD at latest spring frost at the investigated sites (right). Note the higher distance between predicted GDD requirement and observed GDD near Beijing compared to predicted GDD requirement and observed GDD near Zurich. Note also the weaker influence of pre-chilling conditions (brightness) in East Asian species (upper row) compared to Central European species (lower two rows).
Limitations
Although we used ranges of chilling exposure, forcing temperatures and photoperiods that were very artificial with no fluctuations, these settings allowed us to disentangle their separate effect on spring phenology rather than providing accurate predictions about how spring phenology will advance under future climate warming. However, the absence of diurnal temperature fluctuation may have influenced the relationship between forcing and chilling, as it was demonstrated that diurnal temperature has more effect than night temperature (Fu et al., 2016).
Further, the difference between species in freezing resistance of newly formed tissue is also highly species-specific (Lenz et al., 2013) and the vulnerability of particular species to late-spring frost should also be adjusted to species-specific cold hardiness which remain to be assessed for some species investigated in this experiment, especially for the Asian species. We expect the new emerging leaves of East Asian trees to show a lower freezing resistance compared to Central European trees as they are overall less exposed to late-spring frosts. Further, some European species such as sycamore maple and European beech showed more conservative traits than all species investigated in this experiment with higher chilling requirement for dormancy release or a combination of high chilling and long photoperiods. Therefore, these species may better avoid frost-induced damages due to slower spring progression of spring phenology with increasing spring temperatures than more opportunistic species (Körner and Basler, 2010). However, they would not be able to take advantage of the “new window of opportunity” for growth and development which might be decisive at sapling stage for competition and for long-term establishment. We cannot rule-out an additional effect of more cumulative winter chilling of East Asian species compared to Central European at the sampling date (Figure 1B), whereas different light sources (i.e., halogen lamps in Zurich and LED light bulbs in Beijing) are unlikely to explain why photoperiodic effects were found for European species only since very low photon flux densities (∼ 100 μmol m2 s–1) were used in the experiment. Finally, we used a limited number of species in our experimental study, with only three Asian and six European species. To confirm the consistency of our findings, similar experiments should include more species from these regions and include additional regions with different winter-spring transitions such as eastern North America.
Conclusion
Our full factorial experiment including three species belonging to the same genus but growing either in Europe or in Asia and three other European species, showed that increasing winter and spring temperatures have manifold effects on the spring phenology of deciduous tree species. Further advance in the leaf-out is expected to continue in the future, especially for more opportunistic species that have lower chilling and photoperiodic requirements to budburst, whereas a lack of chilling and photoperiodic constrains are likely to slow down the advancement of more conservative species. Our cross-continental experiment further showed that East Asian species were in general found to be more responsive to forcing than European species of the same genus, consistently with the higher predictability of spring frosts in the eastern China. In view of these results, we expect that future climate warming will lead to an advance in spring phenology of East Asian species that is tightly coupled to spring temperature, which may increase carbon assimilation in the hitherto mostly temperature-limited forests of East Asian. Finally, photoperiod seems to play only a subordinate role compared to chilling and forcing for most species investigated in this experiment and will therefore likely has a limiting effect in the regulation of future phenological shifts in response to global warming.
Data availability statement
The datasets presented in this study can be found in online repositories. The names of the repository/repositories and accession number(s) can be found below: Dryad Digital Repository - doi: 10.5061/dryad.6gb90.
Author contributions
FB, YF, and YV designed this study. TF, SW, MW, and ZW conducted the research. MW analyzed the data and wrote the manuscript. YV was extensively revised the writing. All authors contributed to the article and approved the submitted version.
Funding
This research was supported by the Swiss National Science Foundation (research grant no. 315230_192712), the Velux Foundation (project grant no. 1119), the Chinese National Science Fund for Distinguished Young Scholars (grant no. 42025101), and the SwissForestLab (research grant no. SFL-17 P2). Open Access Funding provided by WSL - Swiss Federal Institute For Forest, Snow And Landscape Research.
Conflict of interest
The authors declare that the research was conducted in the absence of any commercial or financial relationships that could be construed as a potential conflict of interest.
Publisher’s note
All claims expressed in this article are solely those of the authors and do not necessarily represent those of their affiliated organizations, or those of the publisher, the editors and the reviewers. Any product that may be evaluated in this article, or claim that may be made by its manufacturer, is not guaranteed or endorsed by the publisher.
Supplementary material
The Supplementary Material for this article can be found online at: https://www.frontiersin.org/articles/10.3389/ffgc.2022.1063127/full#supplementary-material
References
Basler, D., and Körner, C. (2012). Photoperiod sensitivity of bud burst in 14 temperate forest tree species. Agric. For. Meteorol. 165, 73–81. doi: 10.1016/j.agrformet.2012.06.001
Baumgarten, F., Zohner, C. M., Gessler, A., and Vitasse, Y. (2021). Chilled to be forced: The best dose to wake up buds from winter dormancy. New Phytol. 230, 1366–1377. doi: 10.1111/nph.17270
Bigler, C., and Bugmann, H. (2018). Climate-induced shifts in leaf unfolding and frost risk of European trees and shrubs. Sci. Rep. 8:9865. doi: 10.1038/s41598-018-27893-1
Caffarra, A., and Donnelly, A. (2011). The ecological significance of phenology in four different tree species: Effects of light and temperature on bud burst. Int. J. Biometeorol. 55, 711–721. doi: 10.1007/s00484-010-0386-1
Chuine, I. (2000). A unified model for budburst of trees. J. Theor. Biol. 207, 337–347. doi: 10.1006/jtbi.2000.2178
Coville, F. V. (1920). The influence of cold in stimulating the growth of plants. Proc. Natl. Acad. Sci. U.S.A. 6, 434–435. doi: 10.1073/pnas.6.7.434
Dai, J., Wang, H., and Ge, Q. (2014). The spatial pattern of leaf phenology and its response to climate change in China. Int. J. Biometeorol. 58, 521–528. doi: 10.1007/s00484-013-0679-2
Dantec, C. F., Vitasse, Y., Bonhomme, M., Louvet, J. M., Kremer, A., and Delzon, S. (2014). Chilling and heat requirements for leaf unfolding in European beech and sessile oak populations at the southern limit of their distribution range. Int. J. Biometeorol. 58, 1853–1864. doi: 10.1007/s00484-014-0787-7
Delpierre, N., Vitasse, Y., Chuine, I., Guillemot, J., Bazot, S., Rutishauser, T., et al. (2016). Temperate and boreal forest tree phenology: From organ-scale processes to terrestrial ecosystem models. Ann. For. Sci. 73, 5–25. doi: 10.1007/s13595-015-0477-6
Ellison, A. M. (2004). Bayesian inference in ecology. Ecol. Lett. 7, 509–520. doi: 10.1111/j.1461-0248.2004.00603.x
Flynn, D. F. B., and Wolkovich, E. M. (2018). Temperature and photoperiod drive spring phenology across all species in a temperate forest community. New Phytol. 219, 1353–1362. doi: 10.1111/nph.15232
Fu, Y. H., Campioli, M., Deckmyn, G., and Janssens, I. A. (2013). Sensitivity of leaf unfolding to experimental warming in three temperate tree species. Agric. For. Meteorol. 181, 125–132. doi: 10.1016/j.agrformet.2013.07.016
Fu, Y. H., Liu, Y., De Boeck, H. J., Menzel, A., Nijs, I., Peaucelle, M., et al. (2016). Three times greater weight of daytime than of night-time temperature on leaf unfolding phenology in temperate trees. New Phytol. 212, 590–597. doi: 10.1111/nph.14073
Fu, Y. H., Piao, S., Zhou, X., Geng, X., Hao, F., Vitasse, Y., et al. (2019b). Short photoperiod reduces the temperature sensitivity of leaf-out in saplings of Fagus sylvatica but not in horse chestnut. Glob. Chang Biol. 25, 1696–1703. doi: 10.1111/gcb.14599
Fu, Y. H., Zhao, H., Piao, S., Peaucelle, M., Peng, S., Zhou, G., et al. (2015). Declining global warming effects on the phenology of spring leaf unfolding. Nature 526, 104–107. doi: 10.1038/nature15402
Fu, Y., Zhang, X., Piao, S., Hao, F., Geng, X., Vitasse, Y., et al. (2019a). Daylength helps temperate deciduous trees to leaf-out at the optimal time. Glob. Chang Biol. 25, 2410–2418. doi: 10.1111/gcb.14633
Gao, X., Dai, J., Shahzad, K., Wang, H., Tao, Z., and Alatalo, J. M. (2022). Association of spring phenological traits with phylogeny and adaptation to native climate in temperate plant species in Northeast China. Ecol. Indic. 143:109381. doi: 10.1016/j.ecolind.2022.109381
Geng, X., Zhang, Y., Fu, Y. H., Hao, F., Janssens, I. A., Peñuelas, J., et al. (2022). Contrasting phenology responses to climate warming across the northern extra-tropics. Fundam. Res. 2, 708–715. doi: 10.1016/j.fmre.2021.11.035
Hänninen, H. (1990). Modelling bud dormancy release in trees from cool and temperate regions. Acta Forestalia Fennica 213:7660. doi: 10.14214/aff.7660
Harrington, C. A., Gould, P. J., and St.Clair, J. B. (2010). Modeling the effects of winter environment on dormancy release of Douglas-fir. For. Ecol. Manag. 259, 798–808. doi: 10.1016/j.foreco.2009.06.018
Hu, Z., Wang, H., Dai, J., Ge, Q., and Lin, S. (2022). Stronger spring phenological advance in future warming scenarios for temperate species with a lower chilling sensitivity. Front. Plant Sci. 13:830573. doi: 10.3389/fpls.2022.830573
Laube, J., Sparks, T. H., Estrella, N., Hofler, J., Ankerst, D. P., and Menzel, A. (2014). Chilling outweighs photoperiod in preventing precocious spring development. Glob. Chang Biol. 20, 170–182. doi: 10.1111/gcb.12360
Lenz, A., Hoch, G., Körner, C., Vitasse, Y., and Russo, S. (2016). Convergence of leaf-out towards minimum risk of freezing damage in temperate trees. Funct. Ecol. 30, 1480–1490. doi: 10.1111/1365-2435.12623
Lenz, A., Hoch, G., Vitasse, Y., and Korner, C. (2013). European deciduous trees exhibit similar safety margins against damage by spring freeze events along elevational gradients. New Phytol. 200, 1166–1175. doi: 10.1111/nph.12452
Li, X., Wang, L., Chen, D., Yang, K., Xue, B., and Sun, L. (2013). Near-surface air temperature lapse rates in the mainland China during 1962-2011. J. Geophys. Res. Atmos. 118, 7505–7515. doi: 10.1002/jgrd.50553
Malyshev, A. V., Henry, H. A. L., Bolte, A., Arfin Khan, M. A. S., and Kreyling, J. (2018). Temporal photoperiod sensitivity and forcing requirements for budburst in temperate tree seedlings. Agric. For. Meteorol. 248, 82–90. doi: 10.1016/j.agrformet.2017.09.011
Man, R., Lu, P., and Dang, Q. L. (2017). Insufficient chilling effects vary among boreal tree species and chilling duration. Front. Plant Sci. 8:1354. doi: 10.3389/fpls.2017.01354
Murray, M. B., Cannell, M. G. R., and Smith, R. I. (1989). Date of budburst of fifteen tree species in britain following climatic warming. J. Appl. Ecol. 26, 693–700. doi: 10.2307/2404093
Partanen, J., Koski, V., and Hänninen, H. (1998). Effects of photoperiod and temperature on the timing of bud burst in Norway spruce (Picea abies). Tree Physiol. 18, 811–816. doi: 10.1093/treephys/18.12.811
R Core Team (2022). R: A language and environment for statistical computing. Vienna: R Foundation for Statistical Computing.
Stan Development Team (2022). Stan modeling language users guide and reference manual. Stan Development Team. *C.
Trotta, R. (2008). Bayes in the sky: Bayesian inference and model selection in cosmology. Contemp. Phys. 49, 71–104. doi: 10.1080/00107510802066753
van de Schoot, R., Depaoli, S., King, R., Kramer, B., Märtens, K., Tadesse, M. G., et al. (2021). Bayesian statistics and modelling. Nat. Rev. Methods Primers 1:1. doi: 10.1038/s43586-020-00001-2
Vitasse, Y. (2013). Ontogenic changes rather than difference in temperature cause understory trees to leaf out earlier. New Phytol. 198, 149–155. doi: 10.1111/nph.12130
Vitasse, Y., and Basler, D. (2012). What role for photoperiod in the bud burst phenology of European beech. Eur. J. For. Res. 132, 1–8. doi: 10.1007/s10342-012-0661-2
Vitasse, Y., and Basler, D. (2014). Is the use of cuttings a good proxy to explore phenological responses of temperate forests in warming and photoperiod experiments? Tree Physiol. 34, 174–183. doi: 10.1093/treephys/tpt116
Vitasse, Y., Delzon, S., Dufrêne, E., Pontailler, J.-Y., Louvet, J.-M., Kremer, A., et al. (2009a). Leaf phenology sensitivity to temperature in European trees: Do within-species populations exhibit similar responses? Agric. For. Meteorol. 149, 735–744. doi: 10.1016/j.agrformet.2008.10.019
Vitasse, Y., Lenz, A., and Korner, C. (2014). The interaction between freezing tolerance and phenology in temperate deciduous trees. Front. Plant Sci. 5:541. doi: 10.3389/fpls.2014.00541
Vitasse, Y., Porté, A. J., Kremer, A., Michalet, R., and Delzon, S. (2009b). Response of canopy duration to temperature changes in four temperate tree species: Relative contributions of spring and autumn leaf phenology. Oecologia 161, 187–198. doi: 10.1007/s00442-009-1363-4
Wang, H., Wang, H., Ge, Q., and Dai, J. (2020). The Interactive effects of chilling, photoperiod, and forcing temperature on flowering phenology of temperate woody plants. Front. Plant Sci. 11:443. doi: 10.3389/fpls.2020.00443
Way, D. A., and Montgomery, R. A. (2015). Photoperiod constraints on tree phenology, performance and migration in a warming world. Plant Cell Environ. 38, 1725–1736. doi: 10.1111/pce.12431
Wenden, B., Mariadassou, M., Chmielewski, F. M., and Vitasse, Y. (2020). Shifts in the temperature-sensitive periods for spring phenology in European beech and pedunculate oak clones across latitudes and over recent decades. Glob. Chang Biol. 26, 1808–1819. doi: 10.1111/gcb.14918
Wolkovich, E. M., Auerbach, J., Chamberlain, C. J., Buonaiuto, D. M., Ettinger, A. K., Morales-Castilla, I., et al. (2021). A simple explanation for declining temperature sensitivity with warming. Glob. Chang Biol. 27, 4947–4949. doi: 10.1111/gcb.15746
Wu, Z., Lin, C.-F., Wang, S., Gong, Y., Fu, Y. H., Tang, J., et al. (2022a). The sensitivity of ginkgo leaf unfolding to the temperature and photoperiod decreases with increasing elevation. Agric. For. Meteorol. 315:108840. doi: 10.1016/j.agrformet.2022.108840
Wu, Z., Wang, S., Fu, Y. H., Gong, Y., Lin, C. F., Zhao, Y. P., et al. (2022b). Spatial difference of interactive effect between temperature and daylength on Ginkgo budburst. Front. Plant Sci. 13:887226. doi: 10.3389/fpls.2022.887226
Zohner, C. M., Benito, B. M., Fridley, J. D., Svenning, J. C., and Renner, S. S. (2017). Spring predictability explains different leaf-out strategies in the woody floras of North America, Europe and East Asia. Ecol. Lett. 20, 452–460. doi: 10.1111/ele.12746
Zohner, C. M., Benito, B. M., Svenning, J.-C., and Renner, S. S. (2016). Day length unlikely to constrain climate-driven shifts in leaf-out times of northern woody plants. Nat. Clim. Chang. 6, 1120–1123. doi: 10.1038/nclimate3138
Keywords: tree phenology, chilling, photoperiod, temperature response, climate chamber, local adaptation
Citation: Walde MG, Wu Z, Fox T, Baumgarten F, Fu YH, Wang S and Vitasse Y (2022) Higher spring phenological sensitivity to forcing temperatures of Asian compared to European tree species under low and high pre-chilling conditions. Front. For. Glob. Change 5:1063127. doi: 10.3389/ffgc.2022.1063127
Received: 06 October 2022; Accepted: 17 November 2022;
Published: 01 December 2022.
Edited by:
Rongzhou Man, Ontario Ministry of Northern Development, Mines, Natural Resources and Forestry, CanadaReviewed by:
Xianliang Zhang, Agricultural University of Hebei, ChinaYanjun Du, Hainan University, China
Copyright © 2022 Walde, Wu, Fox, Baumgarten, Fu, Wang and Vitasse. This is an open-access article distributed under the terms of the Creative Commons Attribution License (CC BY). The use, distribution or reproduction in other forums is permitted, provided the original author(s) and the copyright owner(s) are credited and that the original publication in this journal is cited, in accordance with accepted academic practice. No use, distribution or reproduction is permitted which does not comply with these terms.
*Correspondence: Manuel. G. Walde, manuel.walde@wsl.ch
†These authors have contributed equally to this work and share first authorship