- 1Sustainable Forest Management Research Institute (iuFOR), University of Valladolid, Palencia, Spain
- 2Department of Plant Production and Forest Resources Higher Technical School of Agricultural Engineering of Palencia (ETSIIAA), University of Valladolid, Palencia, Spain
Introduction: Mediterranean forests are currently facing a surge in abiotic stressors such as droughts and massive fires as a result of climate crisis and human pressure. Susceptibility to biotic stressors has also increased, including a variety of pests and pathogens capable of weakening and potentially killing forest flora. Biodiversity of microbiome protects forests against declines as it increases trees’ resilience and adaptability.
Objectives: With the objective of analyzing the relationship between health status and fungal diversity, in the present work the mycobiota of declined and healthy specimens of keystone Mediterranean tree species is described and compared.
Methods: To this end, bark and wood from declining Spanish forests of Castanea sativa (chestnut), Quercus ilex (holm oak), Quercus suber (cork oak), and Quercus pyrenaica (pyrenean oak) were sampled and the Internal Transcribed Spacer (ITS1) genomic region was sequenced.
Results: Results showed a predominance of Ascomycota, Basidiomycota, and Mucoromycota in all samples. Alpha diversity at genus level was not affected by health status and was characterized by uneven, poorly distributed fungal communities dominated by a few genera. Differentially abundant (DA) genera between healthy and declined samples were found in chestnut (15), holm oak (6), and pyrenean oak (4) trees, but not in cork oak. Some genera known for their plant protection potential, such as Trichoderma, were found exclusively in healthy trees. Functional profiles revealed a predominance of phytopathogens and saprobes in all tree species, irrespective of their health status.
Discussion: This study emphasizes the importance of Mediterranean forests as biodiversity refuges and highlights the value of above-ground tissues as a valid approach to assess shifts in forests’ microbiome diversity in response to biotic and abiotic stressors.
1 Introduction
Forests are extremely valuable for the ecosystem services they provide. Forests act as biodiversity refuges and serve as important carbon sinks, but are experiencing severe degradation worldwide that threatens their very existence (Trumbore et al., 2015). Forest degradation is usually characterized by destruction of stand structure, dysregulation of functionality, and lowered productivity as a result of damaging human activities (Ghazoul et al., 2015; Vásquez-Grandón et al., 2018). As a result, forest resilience is heavily compromised. Forest decline can be listed as an important factor contributing to forest degradation, and encompasses reduction of growth rate and vigor of individual trees, loss of soil fertility, and ultimately, permanent death of trees (Zhu and Li, 2007).
Several factors contribute to forest decline, making it difficult to pinpoint specific causes. A variety of models of tree mortality have been conceptualized (Houston, 1981; Manion, 1991) and all of them share the idea that forest decline occurs when environmental stress factors interact with various biotic agents in forests at different stages of development. Declining trees often exhibit reduced water transport as a result of reduced radial growth, which creates an imbalance between the water-demanding foliage and the water-providing roots (Manion, 2003). This leads to alterations in carbon balance and systemic homeostasis and ends up increasing tree susceptibility to biotic attacks and other stressors (McDowell et al., 2008; Wang et al., 2012). This physiological disruption, alongside the continuous pressure exerted by human action and climate crisis, triggers a spiral of processes driven by interactions between abiotic and biotic stress factors that predispose, incite and contribute to stand-level decline (Manion, 1991; Amoroso et al., 2017).
Mediterranean forests rank among the terrestrial ecosystems most threatened by climate crisis and loss of biodiversity caused by changes in land use regime (Ochoa-Hueso et al., 2017; Newbold et al., 2020). Meteorological predictions for Iberian and Italian Peninsulas project severe decreases in precipitation, changes in rain patterns and rising temperatures in the following decades, which make the Mediterranean basin particularly prone to long drought periods and large-scale wildfires (Camia and Amatulli, 2009; Batllori et al., 2017; Turco et al., 2017). Post-fire soil is exposed to erosive agents and its recovery might be hindered if other extreme events, such as heavy rainfall, occur within the window of disturbance period (Morán-Ordóñez et al., 2020). Soil in Mediterranean forests is especially vulnerable to this kind of disturbances, as dry summers are followed by rain-intense autumns with 70–80% of the annual precipitation (Shakesby, 2011; Panagos et al., 2015).
Thus, edaphic instability and high frequency of climatic adversities impair the ability of Mediterranean trees to withstand other challenges such as insect pests and fungal diseases, which in turn contribute to the deterioration of tree health status. Inciting factors can also be of biotic origin, such as the Cerambyx welensii and Coraebus undatus beetles that create tunnels in oak trees and other deciduous species and for which neither preventive nor curative methods exist (Torres-Vila et al., 2013; Tiberi et al., 2016). These stressors not only affect tree health directly but also influence the composition of forest microbiota (Bowd et al., 2022). Compositional changes in the microbiome have a big influence on the severity of diseases affecting the host plant (Wei et al., 2018). Before decline starts, trees with higher microbial diversity pose more resistance against external disturbances. In vitro experiments have shown that several microbial communities can decrease the invasion success of pathogens (Jones et al., 2021). Core microbiome assemblies capable of buffering plants from extreme environmental conditions have also been identified (Timm et al., 2018). The relationship between trees and their microbiome is so close that considering them as a holobiont - a ‘functional entity formed by a macrobe and its long- and short-term associations with microbes and viruses’ (Gordon et al., 2013) - has been proposed as a necessity to combat forest decline (Bettenfeld et al., 2020).
Within plants’ microbiome, fungal diversity (mycobiota) is of particular interest in terrestrial ecosystems given that many survival, nutrition, and interaction strategies depend on them. Currently, the international LIFE MycoRestore consortium, focused on Mediterranean forests from Spain, Italy, and Portugal, seeks to utilize mycological biodiversity in order to increase soil and tree health, leading to a greater forest resilience to climate change, drought, forest fires, and pests. However, fungi can also be pathogens responsible for some of the most devastating diseases affecting trees, such as chestnut blight caused by Cryphonectria parasitica and ink disease caused by oomycetes of the Phytophthora genus. Metabarcoding studies have shown that the diversity and composition of fungal and oomycete soil communities are associated with the severity of symptoms in declining oak dehesas (Ruiz Gómez et al., 2019). In contrast, soil mycobiota of Castanea sativa trees affected by Phytophthora cambivora have been found to be particularly resilient to the pathogen (Venice et al., 2021). Direct examination of plant material is also of great importance. Root-associated fungi can be affected by pathogens, as shown by the limited diversity of symbiotic ectomycorrhizas found in Quercus ilex forests affected by Phytophthora cinnamomi (Corcobado et al., 2014). Moreover, analysis of internal tissues of trees allows the study of endophytic fungi, which commonly act as antagonists to pathogens and enhancers of plant immunity response (Busby et al., 2016b; Terhonen et al., 2019). Endophytes can increase tree tolerance to abiotic stressors as well, such as drought (Bae et al., 2009; Khan et al., 2016; Ferus et al., 2019), salinity (Rodriguez et al., 2008), and heat (Redman et al., 2002).
Therefore, the aim of the present work is to shed light on the relationship between diversity of plant mycobiota and health condition of Mediterranean forests, represented by four key species: holm oak, cork oak, and chestnut, that are of great economic importance in Iberian Peninsula ecosystems, and pyrenean oak, which is endemic to Spain. To this end, wood and bark from healthy and declined specimens was sampled in locations where insect pests and fungal diseases are known to be prevalent. The mycobiota composition was assessed by means of metabarcoding using the ITS1 region of ribosomal DNA.
2 Materials and methods
2.1 Sampling sites and procedure
Bark and wood samples were extracted from healthy and declining trees of Castanea sativa (chestnut), Quercus suber (cork oak), Quercus ilex (holm oak), and Quercus pyrenaica (pyrenean oak) from Salamanca (Castile and Leon, Spain) forests (Table 1 and Figure 1). Each site sampled corresponded to a single tree species. Sampling was conducted for all four sites in June and July 2020.
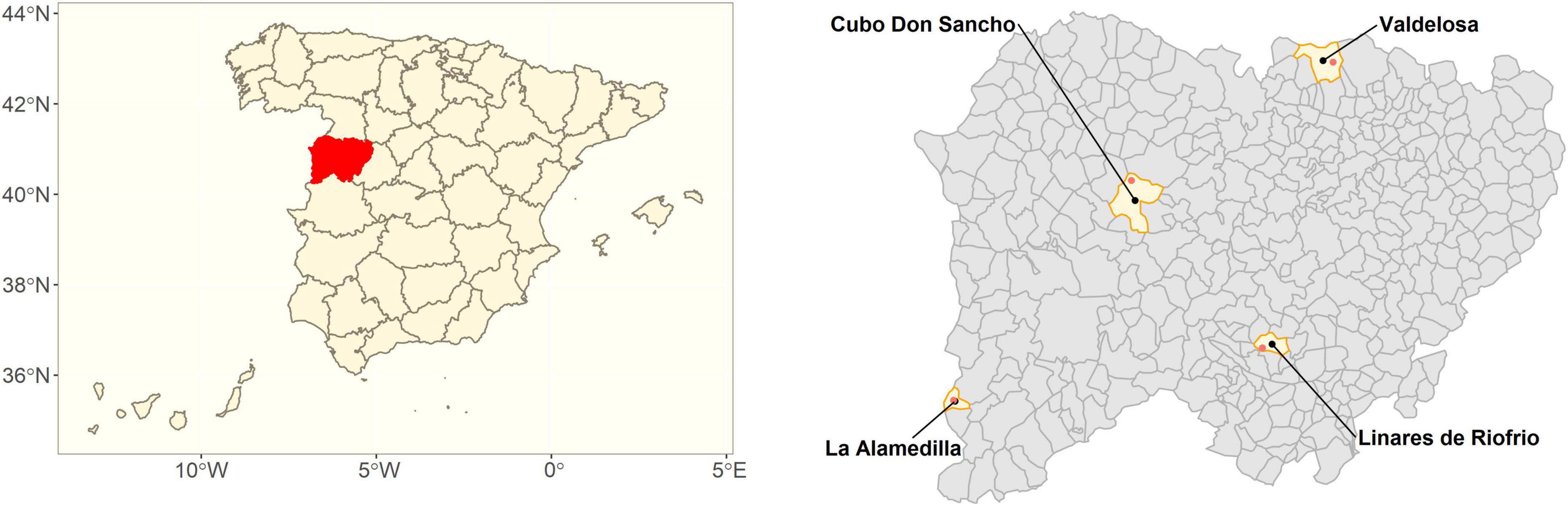
Figure 1. Location of sampling sites. (Left) Map of Spain’s provinces, the sampled Salamanca province is highlighted in red. (Right) Close up of Salamanca province. Sampled municipalities are highlighted in yellow. Red dots correspond to sampling sites.
For all sampling sites, declining patches were defined as areas with high percentage of declining trees (presence of canker wounds or stem bleeds, >70% of trees with severe dieback and foliage wilting). Tree diameter was measured at breast height using calipers. Tree height was measured using a Vertex hypsometer. Crown conditions (% mortality and defoliation) were evaluated according to ICP Forests Manual Part IV “Visual Assessment of Crown Condition and Damaging Agents” (Eichhorn et al., 2016). Each sampling site had been previously characterized by the occurrence of a putative biotic stressor (Table 1), although sampled trees were not specifically tested for their presence. All selected symptomatic trees were alive at the moment of sampling and had variable although comparable degree of decline. Dead or close-to-death trees were not sampled to ensure that detected fungi belonged to microbial communities present in physiologically active trees. Stands of healthy trees were primarily composed of asymptomatic trees free of dieback or crown transparency.
Three plots of healthy trees and three with high degree of decline were selected in each site. Material from five live trees (North orientation) was sampled and pooled per plot. After removing the external bark, one sample was taken per tree from the main trunk at the height of 50 cm over the collar to a depth of 2–3 cm. Only xylem and the internal bark layer (phloem) were considered in the analysis. Six xylem-phloem samples were obtained per tree species (two health conditions × three plots), this totalling 24 samples. Samples were stored at −20°C prior to processing.
2.2 Sample processing
Samples were thawed and washed three times, first with 1 ml of 0.9% NaCl, then with 1 ml of 7% PVP and again with 1 ml of 0.9% NaCl. Following centrifugation for 5 min at 10000 rpm, the supernatant was discarded to remove polyphenols and impurities. Samples were then pulverized by milling during for 5 min at 30 Hz (RETSCH MM 400) with 2 mm tungsten steel beads. DNA was extracted from the wood powder using the EZNA Tissue DNA kit (Omega Bio-tek, Norcross, GA, USA), according to the manufacturer’s instructions. The yield and purity of the DNA was measured by Qubit and then stored at −20°C until use.
2.3 Library preparation and sequencing
Samples were sent for molecular analysis to Biome Makers Inc., (West Sacramento, CA, USA). Region 1 of fungal Internal Transcribed Spacer 1 (ITS1) gene was amplified using WineSeq® custom primers according to Patent WO2017096385 (Becares and Fernandez, 2017). After quality control by gel electrophoresis, each library was pooled in equimolar amount and subsequently sequenced on an Illumina MiSeq instrument (Illumina, San Diego, CA, USA) using 2 × 301 paired-end reads. Sequencing data was analyzed through a QIIME-based custom and in-house bioinformatics pipeline (Caporaso et al., 2010; Becares and Fernandez, 2017). Illumina adapters and chimeras were removed (Edgar et al., 2011) and reads were quality-trimmed. ITS1 sequences were clustered into non-singleton operational taxonomic units (OTUs) at a 97% sequence similarity level. Taxonomy assignment and abundance estimation were performed comparing OTUs against UNITE database version 7.2 (Nilsson et al., 2019). Rarefaction curves were used to evaluate the relationship between sequencing depth and the number of OTUs.
2.4 Statistical analysis
Prior to the analysis, taking into consideration that only ITS1 marker was used, OTUs’ raw reads were aggregated at genus level to have better confidence, and to avoid misidentification of closely-related species (Yang et al., 2018).
Differences in height, diameter, crown mortality, and crown defoliation were assessed using Wilcoxon test (p-value < 0.05 for statistical significance). P-values were adjusted for multiple comparisons by means of Bonferroni correction. Differences between mycobiome communities were evaluated in terms of alpha and beta diversity. Alpha diversity was assessed using Hill diversity indexes according to the equation.
Where D is diversity, S is the number of taxa, pi is the proportion of all individuals that belong to taxa i, ri is the rarity of taxa i, defined as 1/pi, and l is the exponent that determines the rarity scale and corresponds to richness (l = 1) or equivalence-corrected versions of Shannon (l = 0) and Simpson indexes (l = −1) (Roswell et al., 2021). Differences between health conditions per tree species at l = [−1, 0, 1] were contrasted using Wilcoxon’s rank test.
Beta diversity was evaluated in terms of differential abundance taking into account that high-throughput sequencing counts should be considered compositional data (Gloor et al., 2017). Analyses followed anova-like differential expression analysis (ALDEx) technique, which has been shown to produce results very similar to the intersection of multiple independent tools (Soneson and Delorenzi, 2013; Wallen, 2021). Reads were transformed to centered log-ratio (clr) values and per-genus technical variation within each sample was estimated using 1,000 Monte-Carlo instances drawn from the Dirichlet distribution, which returns the posterior probability of observing the counts given the data collected (Fernandes et al., 2013). Effect size was then calculated as the median standardized difference of clr values between groups. A genus was deemed as differentially abundant if the estimated effect was bigger than 1 (absolute value) and the 95% confidence interval (95%CI) didn’t include 0.
All fungal genera were included in the functional analysis. Each genus was assigned a functional guild according to the “primary lifestyle” column obtained from FungalTraits database V1.2 (Põlme et al., 2020). Raw read numbers of each guild were summed per tree species and health condition and expressed as log2 (guild abundance/total abundance).
All analyses were performed in R environment 4.1.3 (R Core Team, 2022). Hill diversity analysis was performed using the package MeanRarity (Roswell and Dushoff, 2022). Compositional analysis was performed using the packages ALDEx2 (Fernandes et al., 2014; Gloor et al., 2016), CoDaSeq (Gloor and Reid, 2016), and zCompositions (Palarea-Albaladejo and Martin-Fernandez, 2015). Plotting and data cleaning and formatting were performed using the packages dplyr (Wickham et al., 2022), doParallel (Microsoft Corporation and Weston, 2022), ggplot2 (Wickham, 2016), ggplotify (Guangchuang, 2021), ggpubr (Kassambara, 2020), ggVennDiagram (Gao, 2021), openxlsx (Schauberger and Walker, 2021), and tidyr (Wickham and Girlich, 2022).
3 Results
3.1 Characterization of decline
No differences in height and diameter were observed between healthy and declined trees (Figure 2). Crown mortality and defoliation were higher in declined samples of cork oak and holm oak, while chestnut showed differences only in crown mortality. Pyrenean oaks showed comparable levels of crown mortality and defoliation regardless of health status.
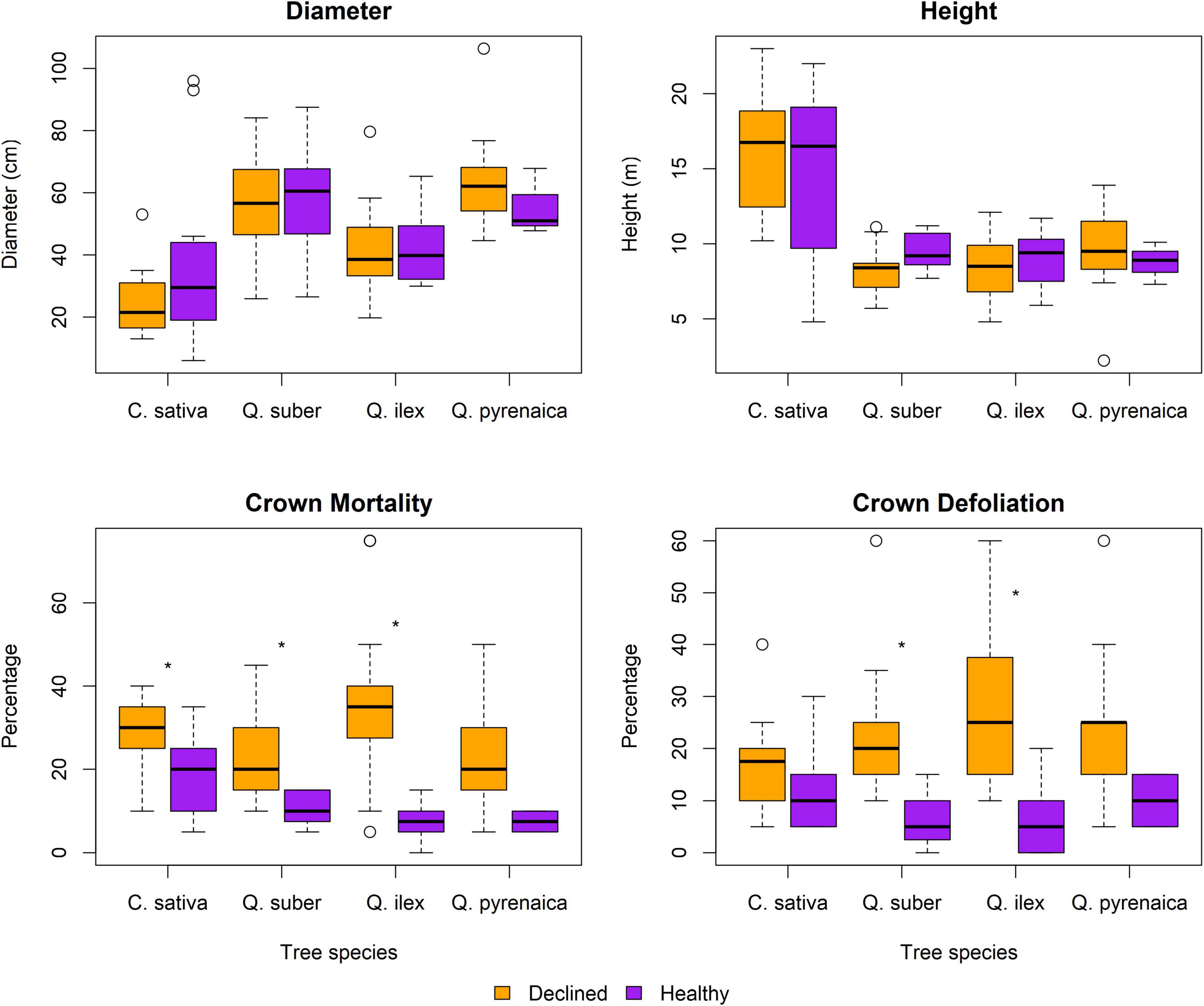
Figure 2. Dendrometric measurements. Boxplots correspond to 15 symptomatic and 15 asymptomatic individuals per tree species, later on pooled into 3 replicates of n = 5 (per health status) for metabarcoding analysis. Asterisks mark statistically significant differences (Wilcoxon tests, p < 0.05 after Bonferroni correction).
3.2 Fungal community description
Sequencing after quality control yielded 38,421 (25,817–66,879) reads on average (median and P25–75) and a total of 483 OTUs clustered at genus level (97% similarity) (Supplementary Tables 1, 2). In general, all samples had sufficient reads to capture most of the diversity, as shown by the plateauing rarefaction curves (Supplementary Figure 1). The most common phyla corresponded to Ascomycota (∼74%), Basidiomycota (∼19%), and Mucoromycota (∼6%) (Figure 3). The same pattern was observed regardless of health condition and ecosystem (Supplementary Figure 2).
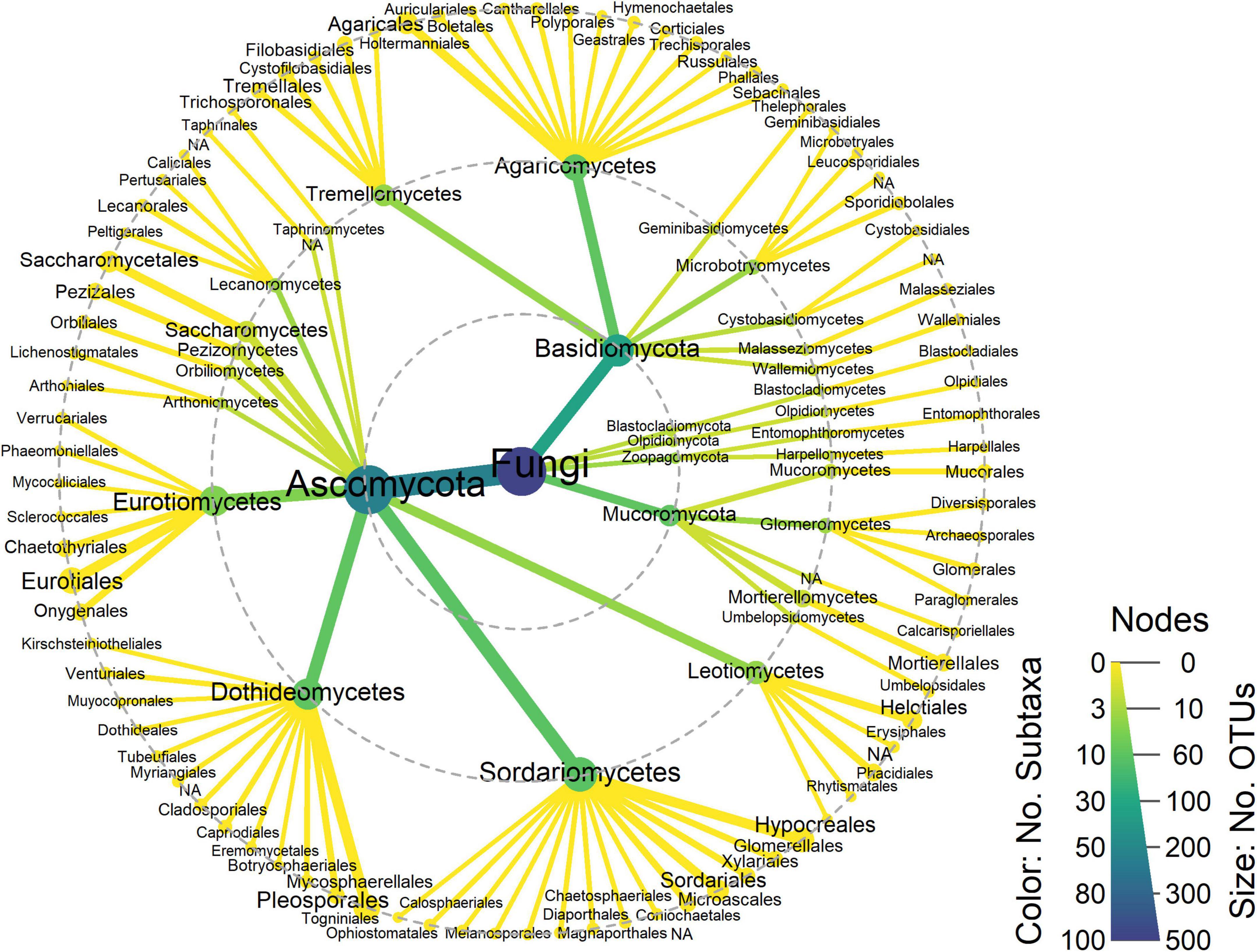
Figure 3. Taxonomic heat tree. Node size represents the number of OTUs and color scale represents the number of subtaxa. Dashed gray circles indicate the following taxonomic levels: phylum (inner), class (middle), order (outer). NA, not assigned.
3.3 Global comparison by health condition
Presence/absence of fungal genera was assessed regardless of tree species as a first approach to find commonalities associated with health status. Some genera were exclusively present in healthy (n = 8, ∼21.1%) or diseased (n = 4, ∼10.5%) samples, although most genera were common to both (n = 26, ∼68.4%) (Figure 4A). Dimensionality reduction analysis showed no signs of clustering in terms of health condition or tree species (Figure 4B).
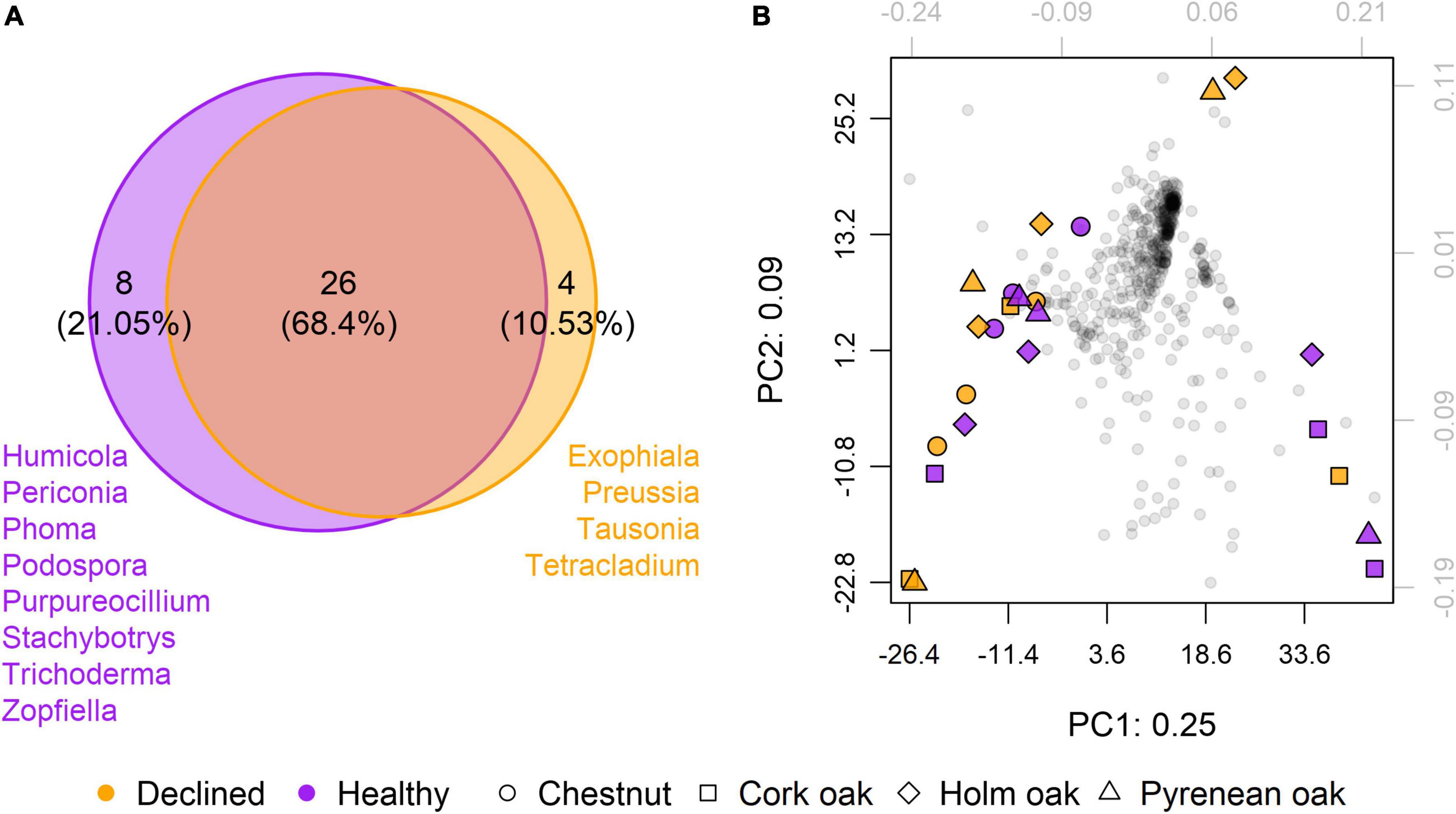
Figure 4. Overall effect of health status on mycobiota. (A) Venn’s diagram showing the number of common and exclusive genera between samples from healthy (n = 12) and diseased (n = 12) trees. Only genera present in at least 10 out of 12 samples per condition are included. (B) Principal components analysis (PCA) of presence/absence based on clr-transformed abundances. Gray dots correspond to genera. Colored dots correspond to samples. Total variance explained by the first two dimensions is 35%.
3.4 Biodiversity analysis
Effect of health conditions on the biodiversity of fungal communities was assessed separately per tree species. No significant differences were found in terms of alpha diversity (Figure 5). On average, healthy and diseased samples had comparable estimators for richness, Hill–Shannon and Hill–Simpson indexes. All samples had uneven, poorly distributed fungal communities and were dominated by a few genera (usually 1 to 3, corresponding with Hill = −1). High variability was found particularly in the richness estimation, as shown by the widening of intervals as Hill’s index approaches 1 (with the exception of holm oak samples).
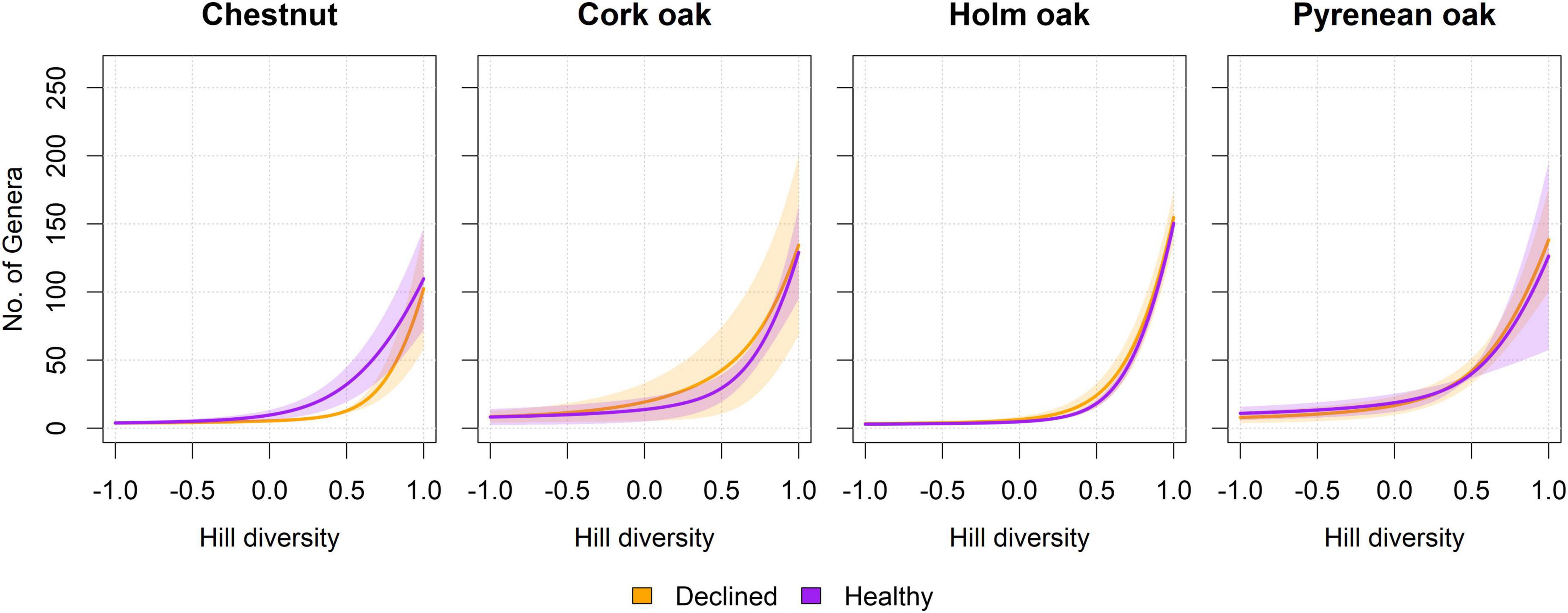
Figure 5. Diversity profiles per tree species and health status. Curves represent the average diversity in samples from healthy and declined trees, in terms of an imaginary assemblage with that same diversity, but in which all species are equally abundant (Jost, 2006; Roswell et al., 2021). The horizontal axis represents the exponent l of Hill diversity, which can be interpreted as equivalence-corrected versions for richness (l = 1), Shannon (l = 0), and Simpson (l = –1) diversity estimators. Shadowed intervals correspond to standard error. No significant differences were found at l = [1, 0, –1] (Wilcoxon test, n = 3 per tree species and health condition, p > 0.05). Raw curves prior to averaging can be found in Supplementary Figure 3.
Beta diversity was evaluated in terms of differences in fungal composition using log ratio-transformed values of raw reads. Differentially abundant (DA) genera between healthy and diseased samples were found in chestnut (15), holm oak (6), and pyrenean oak (4) trees, but not in cork oak (Figure 6). Identities of DA genera, alongside the corresponding effect estimator and 95%CI are shown in Figure 7. DA genera were more prominently found in samples from declined trees of chestnut and pyrenean oak, and in samples from healthy trees of holm oak.
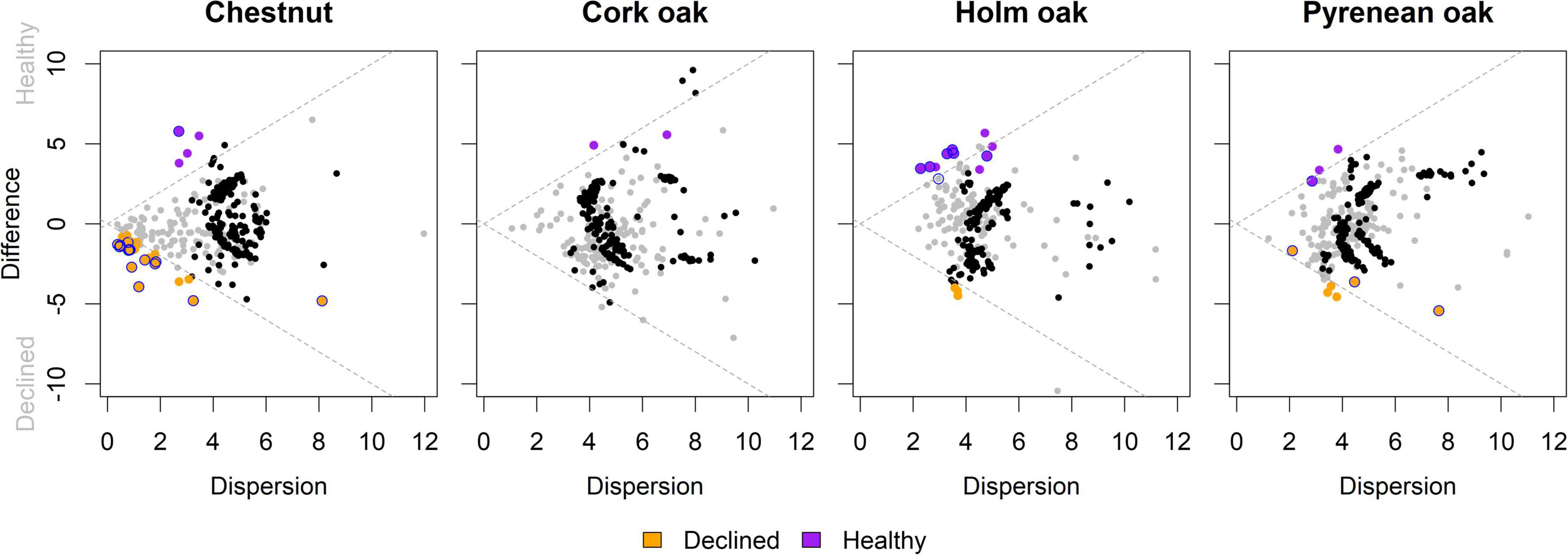
Figure 6. Effect plot of ALDEx differential abundance comparisons. Colored dots represent genera with effect size >1 (purple if more abundant in samples from healthy trees and orange if more abundant in samples from declined trees); blue outline indicates differential abundance (zero not included in 95% CI); rare (clr-transformed abundance < 0) non significant genera are shown in black and abundant (clr-transformed abundance > 0) in grey, as represented in the Bland–Altmann plots in Supplementary Figure 4. Dotted gray lines show the effect = 1 isopleth. Vertical and horizontal axes represent Median Log2 transformations of abundances’ difference (between samples variability) and dispersion (within samples variability), respectively.
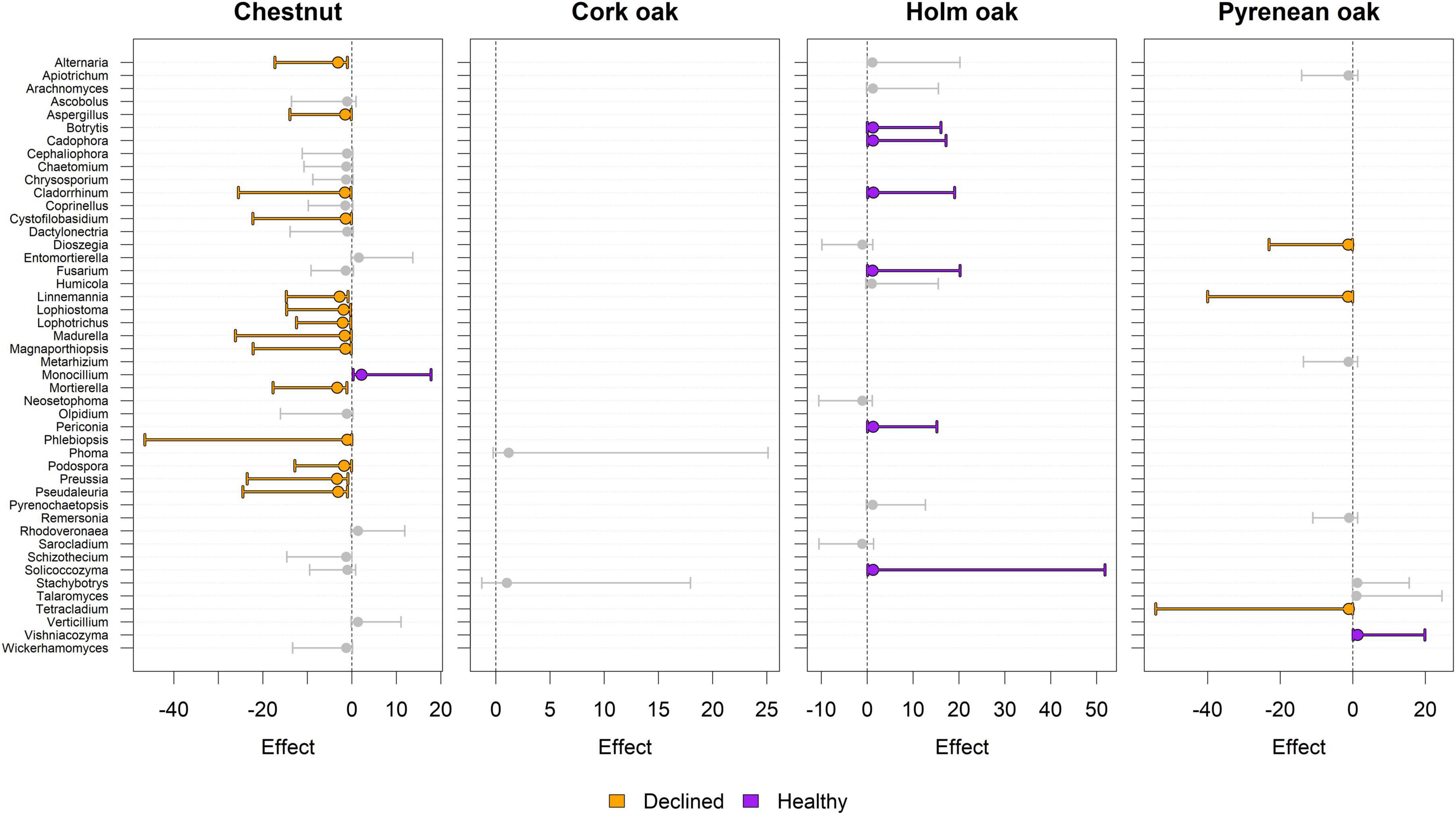
Figure 7. Differentially abundant (DA) genera detected by ALDEx analysis. Effect size point estimators and 95%CI are shown. Vertical gray lines indicate no effect. Colored dots and intervals indicate differential abundance (zero line not crossed) for a given tree species. Only genera with effect >1 (absolute value) in at least one tree species are shown to facilitate visual comparisons. Absence of a genus in a plot indicates that its effect was <1 (absolute value). Positive effect size favors healthy status and vice versa. Size of confidence intervals can be interpreted as a proxy of the effect size estimation robustness (the smaller the better).
3.5 Functional profiles
Assignment of fungal genera to their primary lifestyle was performed per tree species and health status (Figure 8). Although plant pathogens and saprotrophs were the most abundant guilds in all cases, there was no consistent relation with health status. Plant pathogens were more abundant in declined samples of holm oak and healthy samples of chestnut and pyrenean oak, whereas the difference was non-existent in cork oaks. Functional guilds with the biggest differences in abundance per tree species were nectar/tap saprotroph in chestnut, ectomycorrhizas in cork oak, animal parasite in holm oak and wood saprotroph in pyrenean oak. Some guilds couldn’t be found in both healthy and declined trees, such as sooty molds (absent from healthy chestnuts, holm oaks and pyrenean oaks, and declined cork oaks), and animal endosymbionts (absent from declined chestnuts, holm oaks and pyrenean oaks, and healthy cork oaks).
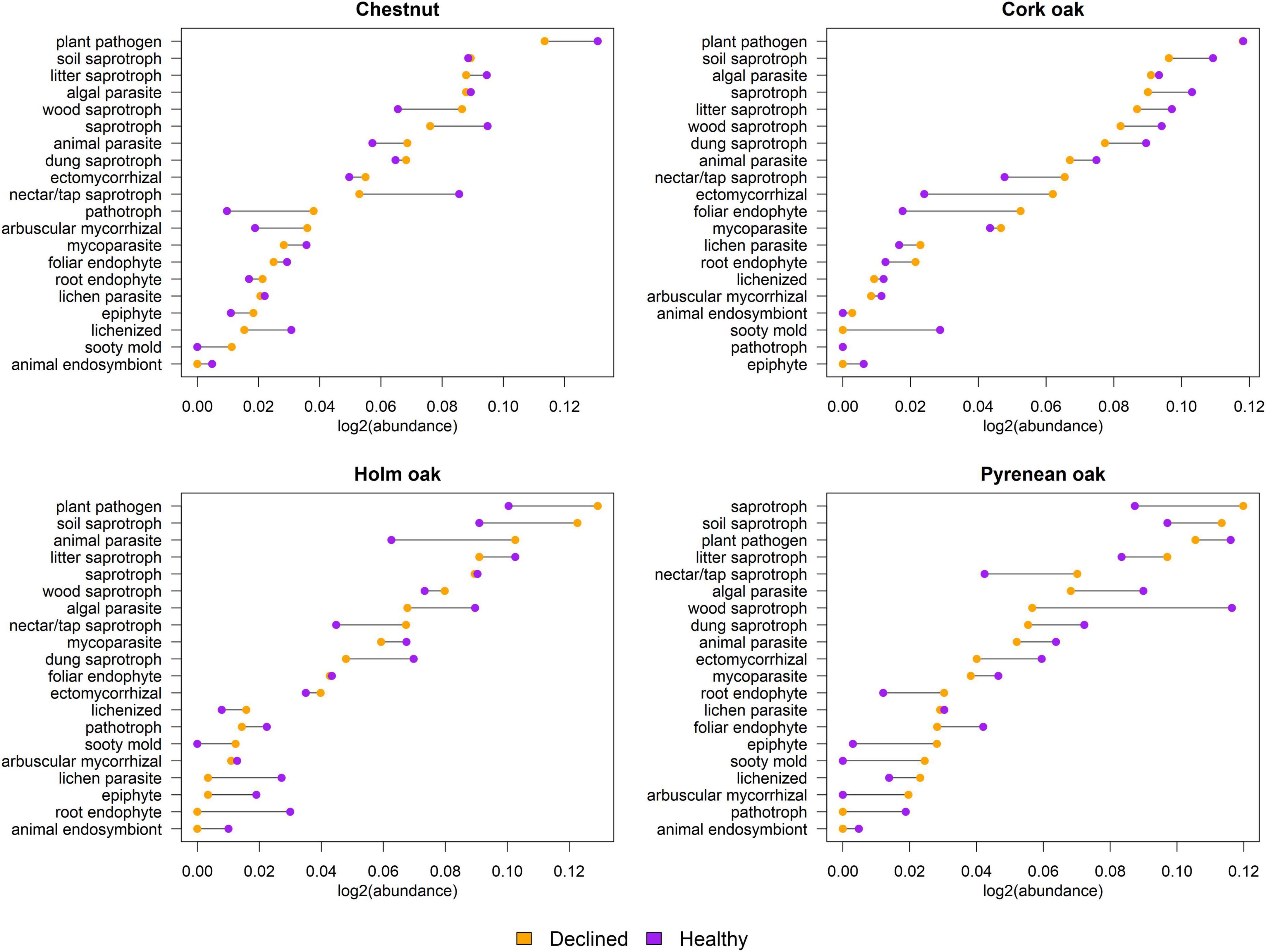
Figure 8. Comparison of functional guilds between declined and healthy samples. Horizontal axis represents sum of abundances expressed as log2 (guild abundance/total abundance). Connecting lines between two dots correspond to the difference between declined and healthy samples.
4 Discussion
Human action and climate crisis contribute to forest dieback and increase the vulnerability of the surviving plants. That way forests are led into a spiral of decline in which trees are weakened and become susceptible to other abiotic and biotic stressors, which ultimately places the health and existence of the whole ecosystem at risk. Mycobiota of trees affected by recent wildfires show increases in genetic diversity and shifts in community structure and taxonomic composition (Huang et al., 2016). Imbalances in microbiome diversity have been related to severity of decline, as essential functions of the holobiont might not be guaranteed, reducing its fitness (Moricca et al., 2012; Bettenfeld et al., 2020; Lyu et al., 2021). Therefore, biodiversity acts as a buffer that makes forests more resilient, minimizing the damages caused by disturbances.
However, a majority of metabarcoding studies focus on commercial crops and soil composition, making the studies on tree health in forest ecosystems very scarce. Even fewer studies have dealt with above-ground microbiota and its composition in tissues from declined and healthy trees. For example, it has been shown that foliar fungi can decrease disease severity in Populus trichocarpa and make the host more resistant against the rust disease (Busby et al., 2016a). In addition, to the best of our knowledge, only a handful of studies have attempted to characterize the microbiota of ecosystems as sensitive to climate change as Mediterranean forests are (Lasa et al., 2019; Maghnia et al., 2019; Ruiz Gómez et al., 2019; Venice et al., 2021; Gómez-Aparicio et al., 2022). These studies, however, only focused on a single tree species or pathosystem, i.e., Quercus suber (Maghnia et al., 2019; Gómez-Aparicio et al., 2022), Quercus pyrenaica (Lasa et al., 2019), Quercus ilex (Ruiz Gómez et al., 2019), and Castanea sativa (Venice et al., 2021), and none of them dealt with above-ground tissues. Thus, the present work supplements prior studies by describing mycobiota in above-ground tissues of declined and healthy trees, belonging to key species in Mediterranean ecosystems in Spain.
In our study no significant differences between the alpha diversity of declined and healthy trees were found. Contrary to this result, more diverse fungal communities have been found in needles and sapwood of declining Picea abies and Pinus sylvestris trees, respectively, as compared to tissues from healthy specimens (Giordano et al., 2009; Millberg et al., 2015). Opposite effect was found in ficus trees where a brown root rot fungus decreased their microbial diversity (Liu et al., 2022). Water stress can also reduce diversity of endophytic mycobiota in cork oak (Linaldeddu et al., 2011). Nonetheless, in some cases the correlation between alpha diversity and plant health status has been found to be weak or non-existent as well (Lebreton et al., 2019; Solís-García et al., 2021; Romeralo et al., 2022).
Some genera were only found in either declined or healthy samples and could be identified as indicator genera. The number of indicator genera was slightly higher in healthy trees than in declined trees, as found in other studies (Hossain et al., 2021). On the one hand, regarding genera exclusive to healthy samples, Phoma and Periconia are known to have a plant-pathogenic lifestyle. Other genera are considered to be primarily saprotrophic, such as Stachybotrys, Humicola, Zopfiella, and Podospora. Parasitic genera such as Trichoderma (mycoparasite) and Purpureocillium (animal parasite) were also only found in healthy samples and have been linked to improved in plant health and resilience (Gong et al., 2017; Dutta et al., 2022). On the other hand, genera exclusive to declined samples were saprotrophs (Tetracladium, Preussia, Tausonia) or animal parasites (Exophiala), although none of them have been described as detrimental to plant health so far.
Within the genera present in both declined and healthy plants, many were differentially abundant, with few genera having significant differences. The chestnut ecosystem had the highest amount of differentially abundant genera. The healthy chestnuts had 4 differentially abundant genera; one of them, Monocillium, was significantly different compared to declined chestnuts and is known for having a saprotrophic lifestyle, with some species being nematode parasites (Ashrafi et al., 2017). Some genera that were more abundant in declined chestnuts also have a saprotrophic lifestyle (Cystofilobasidium, Cladorrhinum, Lophiostoma, Lophotrichus), and a few are well known as plant pathogens. For example, Alternaria and Aspergillus have several species that are either obligate or facultative pathogens. In cork oak, no significant differences were found between declined and healthy plants, neither in the alpha diversity nor in the differential abundance. In other host species, fewer genera were differentially abundant according to health condition.
Functional profiles revealed a predominance of genera with pathogenic and saprobic primary lifestyles in all tree species. This is in line with previous studies that showed high percentage of endophytes with pathogenic potential in twigs from declining Quercus species (Ragazzi et al., 2003). It has been long hypothesized that weak pathogens that have an endophytic state as part of their life cycle might play a role in the rapid decline of stressed oak trees (Petrini and Fisher, 1990; Kehr and Wulf, 1993; Ragazzi et al., 1995). Environmental adversities such as drought can induce switches in the lifestyle of an endophyte from neutral to pathogenic (Gonthier et al., 2006; Moricca and Ragazzi, 2008; Porras-Alfaro and Bayman, 2011). Type and abundance of functional guilds are also coincidental with recent studies performed on soil mycobiota of declining dehesas (Ruiz Gómez et al., 2019). This may be indicative of an increased trafficking of soil-borne fungi to above-ground tissues, taking into account that stressed trees show higher colonization frequencies in comparison to healthy trees (Ragazzi et al., 2003). However, differences in abundance of functional guilds according to health status were not uniform among tree species. It should also be noted that fungi might have different lifestyles when inhabiting tree wood than when observed in soil, which would add to the variability caused by host species and by the different abiotic stressors affecting each sampling site more severely.
There are some limitations to the present work that should be taken into consideration when interpreting the results. First, the study was performed after extracting total DNA and hence it is not possible to check if the microbial communities detected were living or dead. Pathogens are not likely to affect non-active or dead communities present in the ecosystems, and therefore, an RNA sequencing study (for example, studying rRNA instead of rRNA genes) could help to understand how pathogens affect active communities. Second, lack of samples from other latitudes and environmental conditions make it difficult to consider the potential environmental and demographic effects on mycobiomes. It remains to be explored how a combination of biotic and abiotic stress could affect the mycobiome composition. Moreover, although the ITS regions of ribosomal DNA are considered useful DNA barcodes for fungi, in some genera, such as Fusarium, these regions are very conserved across species, complicating OTU identification at species level. We tried to avoid this problem by using data up to the genus level to have better confidence. In turn, this hinders inferences about the functional roles of fungi based on their primary lifestyle because different species within a genus can have very different roles. Nonetheless, the same species can change its role depending on the host, making in silico predictions of functional guilds noisy at any taxonomic resolution level. Moreover, databases still offer partial collections of functional traits, rendering functional analysis incomplete at best. Finally, comparison between different ecosystems should be taken with caution, given the limited sample size.
As a conclusion, in this work we show the resilience of above-ground fungal mycobiota in four tree species located in declining Mediterranean forests. Our study indicates that beta diversity but not alpha diversity of fungal communities was significantly affected by health status of the host trees. Some indicator genera that were only present in either diseased or healthy plants were also identified. These genera could be valuable for assessing the plant’s health status and could be used as predictors of the onset of declines.
Data availability statement
Data and scripts used in this study are available in the following GitHub repository: https://github.com/serbiodh/2022_FrontiersForests. Raw sequencing data are available at NCBI SRA under BioProject PRJNA885270.
Author contributions
AB and LE collected the data. SD-H and FA analyzed the data. SD-H, FA, JN-S, EH, WM, and JD designed the sampling and wrote the manuscript. All authors contributed to the article and approved the submitted version.
Funding
This work was supported by LIFE project MycoRestore “Innovative use of mycological resources for resilient and productive Mediterranean forests threatened by climate change, LIFE18 CCA/ES/001110”, and projects PID2019-110459RB-I00 and PLEC2021-008076 funded by MICINN (Spain) as well as the project VA208P20 funded by JCYL (Spain), both co-financed by FEDER (UE) budget. JN-S was also supported by the European Union’s Horizon Europe Research and Innovation Programme under the MSCA agreement No. 101068728.
Acknowledgments
The data analysis has been carried out using the resources from “Centro de Supercomputación de Castilla y León” (SCAYLE) under the valuable technical support of Carmen Calvo and Jesús Lorenzana. We would also like to thank the reviewers for their insightful comments and suggestions, which have helped improving not only the clarity of the manuscript, but also our understanding of the results presented here.
Conflict of interest
The authors declare that the research was conducted in the absence of any commercial or financial relationships that could be construed as a potential conflict of interest.
Publisher’s note
All claims expressed in this article are solely those of the authors and do not necessarily represent those of their affiliated organizations, or those of the publisher, the editors and the reviewers. Any product that may be evaluated in this article, or claim that may be made by its manufacturer, is not guaranteed or endorsed by the publisher.
Supplementary material
The Supplementary Material for this article can be found online at: https://www.frontiersin.org/articles/10.3389/ffgc.2022.1056980/full#supplementary-material
References
Amoroso, M. M., Rodríguez-Catón, M., Villalba, R., and Daniels, L. D. (2017). “Forest decline in Northern Patagonia: the role of climatic variability,” in Dendroecology. Ecological Studies, Vol. 231, eds M. Amoroso, L. Daniels, P. Baker, and J. Camarero (Cham: Springer).
Ashrafi, S., Stadler, M., Dababat, A. A., Richert-Pöggeler, K. R., Finckh, M. R., and Maier, W. (2017). Monocillium gamsii sp. nov. and Monocillium bulbillosum: two nematode-associated fungi parasitising the eggs of Heterodera filipjevi. MycoKeys 27, 21–38. doi: 10.3897/mycokeys.27.21254
Bae, H., Sicher, R. C., Kim, M. S., Kim, S. H., Strem, M. D., Melnick, R. L., et al. (2009). The beneficial endophyte Trichoderma hamatum isolate DIS 219b promotes growth and delays the onset of the drought response in Theobroma cacao. J. Exp. Bot. 60, 3279–3295. doi: 10.1093/jxb/erp165
Batllori, E., De Cáceres, M., Brotons, L., Ackerly, D. D., Moritz, M. A., and Lloret, F. (2017). Cumulative effects of fire and drought in Mediterranean ecosystems. Ecosphere 8:e01906. doi: 10.1002/ecs2.1906
Becares, A. A., and Fernandez, A. F. (2017). Microbiome Based Identification, Monitoring and Enhancement of Fermentation Processes and Products. French Patents: WO2017096385A1. West Sacramento, CA: Biome Makers Inc.
Bettenfeld, P., Fontaine, F., Trouvelot, S., Fernandez, O., and Courty, P. E. (2020). Woody plant declines. What’s wrong with the microbiome? Trends Plant Sci. 25, 381–394. doi: 10.1016/j.tplants.2019.12.024
Bowd, E. J., Banks, S. C., Bissett, A., May, T. W., and Lindenmayer, D. B. (2022). Disturbance alters the forest soil microbiome. Mol. Ecol. 31, 419–447. doi: 10.1111/mec.16242
Busby, P. E., Peay, K. G., and Newcombe, G. (2016a). Common foliar fungi of Populus trichocarpa modify Melampsora rust disease severity. New Phytol. 209, 1681–1692. doi: 10.1111/nph.13742
Busby, P. E., Ridout, M., and Newcombe, G. (2016b). Fungal endophytes: modifiers of plant disease. Plant Mol. Biol. 90, 645–655. doi: 10.1007/s11103-015-0412-0
Camia, A., and Amatulli, G. (2009). “Weather factors and fire danger in the mediterranean,” in Earth Observation of Wildland Fires in Mediterranean Ecosystems, ed. E. Chuvieco (Berlin: Springer).
Caporaso, J. G., Kuczynski, J., Stombaugh, J., Bittinger, K., Bushman, F. D., Costello, E. K., et al. (2010). QIIME allows analysis of high-throughput community sequencing data. Nat. Methods 7, 335–336. doi: 10.1038/nmeth.f.303
Corcobado, T., Vivas, M., Moreno, G., and Solla, A. (2014). Ectomycorrhizal symbiosis in declining and non-declining Quercus ilex trees infected with or free of Phytophthora cinnamomi. For. Ecol. Manag. 324, 72–80. doi: 10.1016/j.foreco.2014.03.040
Dutta, P., Deb, L., and Pandey, A. K. (2022). Trichoderma-From lab bench to field application: looking back over 50 years. Front. Agron. 91:932839. doi: 10.3389/fagro.2022.932839
Edgar, R. C., Haas, B. J., Clemente, J. C., Quince, C., and Knight, R. (2011). UCHIME improves sensitivity and speed of chimera detection. Bioinformatics 27, 2194–2200. doi: 10.1093/bioinformatics/btr381
Eichhorn, J., Roskams, P., Potočić, N., Timmermann, V., Ferretti, M., Mues, V., et al. (2016). “Part IV: visual assessment of crown condition and damaging agents,” in Manual on Methods and Criteria for Harmonized Sampling, Assessment, Monitoring and Analysis of the Effects of Air Pollution on Forests, ed. UNECE ICP Forests Programme Coordinating Centre (Eberswalde: Thünen Institute of Forest Ecosystems).
Fernandes, D. A., Macklaim, J. M., Linn, T. G., Reid, G., and Gloor, G. B. (2013). ANOVA-like differential expression (ALDEx) analysis for mixed population RNA-Seq. PLoS One 8:e67019. doi: 10.1371/journal.pone.0067019
Fernandes, D. A., Reid, J., Macklaim, M. J., McMurrough, T. A., Edgell, D. R., and Gloor, B. G. (2014). Unifying the analysis of high-throughput sequencing datasets: characterizing RNA-seq, 16S rRNA gene sequencing and selective growth experiments by compositional data analysis. Microbiome 2:15. doi: 10.1186/2049-2618-2-15
Ferus, P., Barta, M., and Konôpková, J. (2019). Endophytic fungus Beauveria bassiana can enhance drought tolerance in red oak seedlings. Trees 33, 1179–1186. doi: 10.1007/s00468-019-01854-1
Ghazoul, J., Burivalova, Z., Garcia-Ulloa, J., and King, L. A. (2015). Conceptualizing forest degradation. Trends Ecol. Evol. 30, 622–632. doi: 10.1016/j.tree.2015.08.001
Giordano, L., Gonthier, P., Varese, G. C., Miserere, L., and Nicolotti, G. (2009). Mycobiota inhabiting sapwood of healthy and declining Scots pine (Pinus sylvestris L.) trees in the Alps. Fungal Divers. 38:e83.
Gloor, B. G., and Reid, G. (2016). Compositional analysis: a valid approach to analyze microbiome high-throughput sequencing data. Can. J. Microbiol. 26, 322–329. doi: 10.1139/cjm-2015-0821
Gloor, G. B., Macklaim, J. M., and Fernandes, A. D. (2016). Displaying variation in large datasets: a visual summary of effect sizes. J. Comput. Graph. Stat. 25, 971–979. doi: 10.1080/10618600.2015.1131161
Gloor, G. B., Macklaim, J. M., Pawlowsky-Glahn, V., and Egozcue, J. J. (2017). Microbiome datasets are compositional: and this is not optional. Front. Microbiol. 8:2224. doi: 10.3389/fmicb.2017.02224
Gómez-Aparicio, L., Domínguez-Begines, J., Villa-Sanabria, E., García, L. V., and Muñoz-Pajares, A. J. (2022). Tree decline and mortality following pathogen invasion alters the diversity, composition and network structure of the soil microbiome. Soil Biol. Biochem. 166:108560. doi: 10.1016/j.soilbio.2022.108560
Gong, B., Liu, G., Liao, R., Song, J., and Zhang, H. (2017). Endophytic fungus Purpureocillium sp. A5 protect mangrove plant Kandelia candel under copper stress. Brazil. J. Microbiol. 48, 530–536. doi: 10.1016/j.bjm.2016.10.027
Gonthier, P., Gennaro, M., and Nicolotti, G. (2006). Effects of water stress on the endophytic mycota of Quercus robur. Fungal Divers. 21:e80.
Gordon, J., Knowlton, N., Relman, D. A., Rohwer, F., and Youle, M. (2013). Superorganisms and holobionts. Microbe. 8, 152–153. doi: 10.1128/microbe.8.152.1
Guangchuang, Y. (2021). ggplotify: Convert Plot to ‘grob’ or ‘ggplot’ Object. R package version 0.1.0.
Hossain, Z., Hubbard, M., Gan, Y., and Bainard, L. D. (2021). Root rot alters the root-associated microbiome of field pea in commercial crop production systems. Plant Soil 460, 593–607. doi: 10.1007/s11104-020-04779-8
Houston, D. R. (1981). Stress Triggered Tree Diseases: The Diebacks and Declines. Washington, DC: US Department of Agriculture, Forest Service.
Huang, Y. L., Devan, M. M., U’Ren, J. M., Furr, S. H., and Arnold, A. E. (2016). Pervasive effects of wildfire on foliar endophyte communities in montane forest trees. Microb. Ecol. 71, 452–468. doi: 10.1007/s00248-015-0664-x
Jones, M. L., Rivett, D. W., Pascual-García, A., and Bell, T. (2021). Relationships between community composition, productivity and invasion resistance in semi-natural bacterial microcosms. eLife 10:e71811. doi: 10.7554/eLife.71811.sa2
Kehr, R. D., and Wulf, A. (1993). Fungi associated with above-ground portions of declining oaks (Quercus robur) in Germany. Eur. J. For. Pathol. 23, 18–27. doi: 10.1111/j.1439-0329.1993.tb00803.x
Khan, Z., Rho, H., Firrincieli, A., Hung, S. H., Luna, V., Masciarelli, O., et al. (2016). Growth enhancement and drought tolerance of hybrid poplar upon inoculation with endophyte consortia. Curr. Plant Biol. 6, 38–47. doi: 10.1016/j.cpb.2016.08.001
Lasa, A. V., Fernández-González, A. J., Villadas, P. J., Toro, N., and Fernández-López, M. (2019). Metabarcoding reveals that rhizospheric microbiota of Quercus pyrenaica is composed by a relatively small number of bacterial taxa highly abundant. Sci. Rep. 9, 1–13. doi: 10.1038/s41598-018-38123-z
Lebreton, L., Guillerm-Erckelboudt, A. Y., Gazengel, K., Linglin, J., Ourry, M., Glory, P., et al. (2019). Temporal dynamics of bacterial and fungal communities during the infection of Brassica rapa roots by the protist Plasmodiophora brassicae. PLoS One 14:e0204195. doi: 10.1371/journal.pone.0204195
Linaldeddu, B. T., Sirca, C., Spano, D., and Franceschini, A. (2011). Variation of endophytic cork oak-associated fungal communities in relation to plant health and water stress. For. Pathol. 41, 193–201. doi: 10.1111/j.1439-0329.2010.00652.x
Liu, T. Y., Chen, C. H., Yang, Y. L., Tsai, I. J., Ho, Y. N., and Chung, C. L. (2022). The brown root rot fungus Phellinus noxius affects microbial communities in different root-associated niches of Ficus trees. Environ. Microbiol. 24, 276–297. doi: 10.1111/1462-2920.15862
Lyu, D., Zajonc, J., Pagé, A., Tanney, C. A. S., Shah, A., Monjezi, N., et al. (2021). Plant holobiont theory: the phytomicrobiome plays a central role in evolution and success. Microorganisms 9:675. doi: 10.3390/microorganisms9040675
Maghnia, F. Z., Abbas, Y., Mahé, F., Prin, Y., El Ghachtouli, N., Duponnois, R., et al. (2019). The rhizosphere microbiome: a key component of sustainable cork oak forests in trouble. For. Ecol. Manag. 434, 29–39. doi: 10.1016/j.foreco.2018.12.002
Manion, P. D. (2003). Evolution of concepts in forest pathology. Phytopathology 93, 1052–1055. doi: 10.1094/PHYTO.2003.93.8.1052
McDowell, N., Pockman, W. T., Allen, C. D., Breshears, D. D., Cobb, N., Kolb, T., et al. (2008). Mechanisms of plant survival and mortality during drought: why do some plants survive while others succumb to drought? New Phytol. 178, 719–739. doi: 10.1111/j.1469-8137.2008.02436.x
Microsoft Corporation, and Weston, S. (2022). doParallel: Foreach Parallel Adaptor for the ‘parallel’ Package. R package version 1.0.17.
Millberg, H., Boberg, J., and Stenlid, J. (2015). Changes in fungal community of Scots pine (Pinus sylvestris) needles along a latitudinal gradient in Sweden. Fung. Ecol. 17, 126–139. doi: 10.1016/j.funeco.2015.05.012
Morán-Ordóñez, A., Duane, A., Gil-Tena, A., De Cáceres, M., Aquilué, N., Guerra, C. A., et al. (2020). Future impact of climate extremes in the Mediterranean: soil erosion projections when fire and extreme rainfall meet. Land Degradat. Dev. 31, 3040–3054. doi: 10.1002/ldr.3694
Moricca, S., Ginetti, B., and Ragazzi, A. (2012). Species-and organ-specificity in endophytes colonizing healthy and declining Mediterranean oaks. Phytopathol. Mediterranea 51, 587–598.
Moricca, S., and Ragazzi, A. (2008). Fungal endophytes in Mediterranean oak forests: a lesson from Discula quercina. Phytopathology 98, 380–386. doi: 10.1094/PHYTO-98-4-0380
Newbold, T., Oppenheimer, P., Etard, A., and Williams, J. J. (2020). Tropical and Mediterranean biodiversity is disproportionately sensitive to land-use and climate change. Nat. Ecol. Evol. 4, 1630–1638. doi: 10.1038/s41559-020-01303-0
Nilsson, R. H., Larsson, K. H., Taylor, A. F. S., Bengtsson-Palme, J., Jeppesen, T. S., Schigel, D., et al. (2019). The UNITE database for molecular identification of fungi: handling dark taxa and parallel taxonomic classifications. Nucleic Acids Res. 47, D259–D264. doi: 10.1093/nar/gky1022
Ochoa-Hueso, R., Munzi, S., Alonso, R., Arróniz-Crespo, M., Avila, A., Bermejo, V., et al. (2017). Ecological impacts of atmospheric pollution and interactions with climate change in terrestrial ecosystems of the Mediterranean Basin: current research and future directions. Environ. Pollut. 227, 194–206. doi: 10.1016/j.envpol.2017.04.062
Palarea-Albaladejo, J., and Martin-Fernandez, J. A. (2015). zCompositions-R package for multivariate imputation of left-censored data under a compositional approach. Chemom. Intell. Lab. Syst. 143, 85–96. doi: 10.1016/j.chemolab.2015.02.019
Panagos, P., Ballabio, C., Borrelli, P., Meusburger, K., Klik, A., Rousseva, S., et al. (2015). Rainfall erosivity in Europe. Sci. Total Environ. 511, 801–814. doi: 10.1016/j.scitotenv.2015.01.008
Petrini, O., and Fisher, P. J. (1990). Occurrence of fungal endophytes in twigs of Salix fragilis and Quercus robur. Mycol. Res. 94, 1077–1080. doi: 10.1016/S0953-7562(09)81336-1
Põlme, S., Abarenkov, K., Henrik Nilsson, R., Lindahl, B. D., Clemmensen, K. E., Kauserud, H., et al. (2020). FungalTraits: a user-friendly traits database of fungi and fungus-like stramenopiles. Fung. Divers. 105, 1–16. doi: 10.1007/s13225-020-00466-2
Porras-Alfaro, A., and Bayman, P. (2011). Hidden fungi, emergent properties: endophytes and microbiomes. Annu. Rev. Phytopathol. 49, 291–315. doi: 10.1146/annurev-phyto-080508-081831
R Core Team (2022). R: A Language and Environment for Statistical Computing. Vienna: R Foundation for Statistical Computing.
Ragazzi, A., Moricca, S., Capretti, P., Dellavalle, I., and Turco, E. (2003). Differences in composition of endophytic mycobiota in twigs and leaves of healthy and declining Quercus species in Italy. For. Pathol. 33, 31–38. doi: 10.1046/j.1439-0329.2003.3062003.x
Ragazzi, A., Vagniluca, S., Moricca, S., and Vigniluca, S. (1995). European expansion of oak decline: involved microrganisms and methodological approaches. Phytopathol. Mediterranea 34, 207–226.
Redman, R. S., Sheehan, K. B., Stout, R. G., Rodriguez, R. J., and Henson, J. M. (2002). Thermotolerance generated by plant/fungal symbiosis. Science 298, 1581–1581. doi: 10.1126/science.1078055
Rodriguez, R. J., Henson, J., Van Volkenburgh, E., Hoy, M., Wright, L., Beckwith, F., et al. (2008). Stress tolerance in plants via habitat-adapted symbiosis. ISME J. 2, 404–416. doi: 10.1038/ismej.2007.106
Romeralo, C., Martín-García, J., Martínez-Álvarez, P., Muñoz-Adalia, E. J., Gonçalves, D. R., Torres, E., et al. (2022). Pine species determine fungal microbiome composition in a common garden experiment. Fung. Ecol. 56:101137. doi: 10.1016/j.funeco.2021.101137
Roswell, M., and Dushoff, J. (2022). MeanRarity: Hill Diversity Estimation and Visualisation R package version 0.0.1.0004.
Roswell, M., Dushoff, J., and Winfree, R. (2021). A conceptual guide to measuring species diversity. Oikos 130, 321–338. doi: 10.1111/oik.07202
Ruiz Gómez, F. J., Navarro-Cerrillo, R. M., Pérez-de-Luque, A., Oβwald, W., Vannini, A., and Morales-Rodríguez, C. (2019). Assessment of functional and structural changes of soil fungal and oomycete communities in holm oak declined dehesas through metabarcoding analysis. Sci. Rep. 9, 1–16. doi: 10.1038/s41598-019-41804-y
Schauberger, P., and Walker, A. (2021). Openxlsx: Read, Write and Edit xlsx Files. R package version 4.2.5.
Shakesby, R. A. (2011). Post-wildfire soil erosion in the Mediterranean: review and future research directions. Earth Sci. Rev. 105, 71–100. doi: 10.1016/j.earscirev.2011.01.001
Solís-García, I. A., Ceballos-Luna, O., Cortazar-Murillo, E. M., Desgarennes, D., Garay-Serrano, E., Patiño-Conde, V., et al. (2021). Phytophthora root rot modifies the composition of the avocado rhizosphere microbiome and increases the abundance of opportunistic fungal pathogens. Front. Microbiol. 11:574110. doi: 10.3389/fmicb.2020.574110
Soneson, C., and Delorenzi, M. (2013). A comparison of methods for differential expression analysis of RNA-seq Data. BMC Bioinform. 14:91. doi: 10.1186/1471-2105-14-91
Terhonen, E., Blumenstein, K., Kovalchuk, A., and Asiegbu, F. O. (2019). Forest tree microbiomes and associated fungal endophytes: functional roles and impact on forest health. Forests 10:42. doi: 10.3390/f10010042
Tiberi, R., Branco, M., Bracalini, M., Croci, F., and Panzavolta, T. (2016). Cork oak pests: a review of insect damage and management. Ann. For. Sci. 73, 219–232. doi: 10.1007/s13595-015-0534-1
Timm, C. M., Carter, K. R., Carrell, A. A., Jun, S. R., Jawdy, S. S., Vélez, J. M., et al. (2018). Abiotic stresses shift belowground Populus-associated bacteria toward a core stress microbiome. mSystems 3:e00070-17. doi: 10.1128/mSystems.00070-17
Torres-Vila, L. M., Sánchez-González, Á, Merino-Martínez, J., Ponce-Escudero, F., Conejo-Rodríguez, Y., Martín-Vertedor, D., et al. (2013). Mark–recapture of C erambyx welensii in dehesa woodlands: dispersal behaviour, population density, and mass trapping efficiency with low trap densities. Entomol. Exp. Appl. 149, 273–281. doi: 10.1111/eea.12133
Trumbore, S., Brando, P., and Hartmann, H. (2015). Forest health and global change. Science 349, 814–818. doi: 10.1126/science.aac6759
Turco, M., von Hardenberg, J., AghaKouchak, A., Llasat, M. C., Provenzale, A., and Trigo, R. M. (2017). On the key role of droughts in the dynamics of summer fires in Mediterranean Europe. Sci. Rep. 7, 1–10. doi: 10.1038/s41598-017-00116-9
Vásquez-Grandón, A., Donoso, P. J., and Gerding, V. (2018). Forest degradation: when is a forest degraded? Forests 9:726. doi: 10.3390/f9110726
Venice, F., Vizzini, A., Frascella, A., Emiliani, G., Danti, R., Della Rocca, G., et al. (2021). Localized reshaping of the fungal community in response to a forest fungal pathogen reveals resilience of Mediterranean mycobiota. Sci. Total Environ. 800:149582. doi: 10.1016/j.scitotenv.2021.149582
Wallen, Z. D. (2021). Comparison study of differential abundance testing methods using two large Parkinson disease gut microbiome datasets derived from 16S amplicon sequencing. BMC Bioinform. 22:265. doi: 10.1186/s12859-021-04193-6
Wang, W., Peng, C., Kneeshaw, D. D., Larocque, G. R., and Luo, Z. (2012). Drought-induced tree mortality: Ecological consequences, causes, and modeling. Environ. Rev. 20, 109–121. doi: 10.1139/a2012-004
Wei, Z., Hu, J., Yin, S., Xu, Y., Jousset, A., Shen, Q., et al. (2018). Ralstonia solanacearum pathogen disrupts bacterial rhizosphere microbiome during an invasion. Soil Biol. Biochem. 118, 8–17. doi: 10.1016/j.soilbio.2017.11.012
Wickham, H., François, R., Henry, L., and Müller, K. (2022). dplyr: A Grammar of Data Manipulation. R Package Version 1.0.9.
Yang, R. H., Su, J. H., Shang, J. J., Wu, Y. Y., Li, Y., Bao, D. P., et al. (2018). Evaluation of the ribosomal DNA internal transcribed spacer (ITS), specifically ITS1 and ITS2, for the analysis of fungal diversity by deep sequencing. PLoS One 13:e0206428. doi: 10.1371/journal.pone.0206428
Keywords: metabarcoding, biodiversity, forest pathology, Quercus, Castanea
Citation: Diez-Hermano S, Ahmad F, Niño-Sanchez J, Benito A, Hidalgo E, Escudero LM, Morel WA and Diez JJ (2022) Health condition and mycobiome diversity in Mediterranean tree species. Front. For. Glob. Change 5:1056980. doi: 10.3389/ffgc.2022.1056980
Received: 29 September 2022; Accepted: 06 December 2022;
Published: 22 December 2022.
Edited by:
Ari Mikko Hietala, The Norwegian Institute of Bioeconomy Research (NIBIO), NorwayReviewed by:
Juan A. Martin, Universidad Politécnica de Madrid, SpainTessa Camenzind, Freie Universität Berlin, Germany
Copyright © 2022 Diez-Hermano, Ahmad, Niño-Sanchez, Benito, Hidalgo, Escudero, Morel and Diez. This is an open-access article distributed under the terms of the Creative Commons Attribution License (CC BY). The use, distribution or reproduction in other forums is permitted, provided the original author(s) and the copyright owner(s) are credited and that the original publication in this journal is cited, in accordance with accepted academic practice. No use, distribution or reproduction is permitted which does not comply with these terms.
*Correspondence: Julio Javier Diez, ✉ JulioJavier.Diez@uva.es; Sergio Diez-Hermano, ✉ sergio.diez.hermano@uva.es