- 1Department of Biology and Ecology Center, Utah State University, Logan, UT, United States
- 2Département des Sciences Biologiques, Centre for Forest Research, Université du Québec à Montréal, Montreal, QC, Canada
- 3Department of Natural Resources, Center for Forest Research (CEF), Institute of Temperate Forest Sciences, Université du Québec en Outaouais, Ripon, QC, Canada
- 4Center d’Étude de la Forêt, Faculté de Foresterie, de Géographie et de Géomatique, Université Laval, Québec, QC, Canada
The formation and turnover of soil organic carbon (C), the largest terrestrial C pool, is strongly impacted by the ultimate source of that C: leaves, wood, roots, and root exudates. The quantity and quality of these inputs is determined by the identity of the plants involved. Yet substantial uncertainty surrounds the complex relationships among plant traits and soil C, precluding efforts to maximize whole-ecosystem C uptake in nature-based climate mitigation scenarios. In this study, we leveraged a biodiversity-ecosystem function experiment with trees (IDENT) to explore the effects of interspecific variation in plant traits on soil C dynamics in the very early stages of stand development (9 years since planting). Mineral soil C stocks to 5 cm depth were quantified in monospecific plots of 19 tree species planted on a former agricultural field, and analyzed in relation to tree growth and functional traits. We found that tree species identity affected soil bulk density and, to a lesser extent, the carbon content of the topsoil, and thereby total C pools. Among species and across plots, mineral soil C stocks were positively correlated with rates of tree growth and were significantly larger beneath broadleaf trees with “fast” functional traits vs. conifers with more conservative leaf traits, when comparisons were made over equivalent soil depth increments. Thus, plant functional traits mediate interspecific differences in productivity, which in turn influence the magnitude of belowground C stocks. These results highlight important linkages between above- and belowground carbon cycles in the earliest stages of afforestation.
Introduction
Ecologists have long observed that plant species can have a strong impact on the chemical properties of the soils below them (Binkley and Giardina, 1998). These plant-soil interactions influence patterns of element cycling (Waring et al., 2015), mediate species coexistence, and ultimately determine how plant communities develop over the course of succession (Kardol et al., 2013; Van der Putten et al., 2013). Recently, however, there has been a surge of interest in plant control over one particular ecosystem process: soil carbon (C) sequestration. Because soils constitute the largest and longest-lived terrestrial reservoir of organic C, even small increases in soil C over large spatial scales might have measurable impacts on atmospheric CO2 concentrations. Soil C stocks can be augmented by increasing soil C inputs (i.e., plant production), decreasing soil C losses (slowing decomposition), or both. Plant traits that vary strongly among species, such as growth rate or litter chemistry, exert direct influence over soil C inputs and outputs. Yet we still lack the ability to identify the species or functional groups which have the strongest positive impacts on soil C stocks, and a single plant may display a constellation of different traits with conflicting impacts on soil C cycling (De Deyn et al., 2008).
This knowledge gap has been thrown into sharp relief in recent years, as “natural climate solutions,” i.e., enhanced C capture in plants, soil and sediments, will likely be a necessary component of global efforts to stabilize the climate. It is well-documented that reforestation and afforestation–among the most promising natural climate solutions (Griscom et al., 2017)–enhance ecosystem C stocks in soils as well as plant biomass, augmenting the CO2 sink capacity of forest ecosystems (Laganière et al., 2010). But which species have the largest positive effect on soil C stocks, and are these the same species that exhibit the greatest capacity to sequester C in wood? Perhaps even more importantly, are there some tree species that trigger soil C losses when planted on former pastures or other forms of highly productive grassland, as some evidence seems to suggest [e.g., (Paul et al., 2002)]? Finally, reforestation projects need to consider interspecific differences in C cycling dynamics not only at stand maturity, but also in the very earliest stages of growth. This is because any CO2 sequestered in biomass and soils over the next three decades will decrease peak warming, assuming anthropogenic emissions are also declining over the same timeframe (Matthews et al., 2022). From this perspective, it is extremely important to minimize soil C losses in the earliest stages of afforestation.
The plant traits which mediate species-specific impacts on soil C cycling fall into three general categories: traits related to plant productivity (C input quantity), tissue chemistry (C input quality), and plant-microbe interactions. First and foremost, the size of the soil C pool is determined by the balance between autotroph-derived C inputs and C losses due to mineralization or leaching. Therefore, all else being equal, increases in plant productivity should enhance soil C stocks, and this assumption is embedded in the ecosystem models that are used to predict terrestrial C cycling. Positive relationships between C inputs and soil C stocks have been empirically observed in many cases (Xu et al., 2013; Lajtha et al., 2014; Cusack et al., 2018), yet in quite a few long-term studies, increasing plant inputs does not translate to gains in soil C (Lajtha et al., 2018). Moreover, at the global scale, elevated CO2 tends to increase plant biomass but decrease soil C, or vice versa (Terrer et al., 2021), implying that higher plant production can actually lead to losses of belowground C.
Changes in C inputs may be decoupled from soil C stocks if chemical traits of plant litter alter microbial metabolism, and thereby the balance between C stabilization and loss (Liang et al., 2017). On the one hand, plant tissue traits which enhance microbial growth efficiency should promote retention of plant-derived C belowground, reducing the respiratory C losses associated with decomposition (Cotrufo et al., 2013). Multiple studies have linked the quality of soil C inputs with the formation of persistent mineral C pools (Bradford et al., 2013; Cotrufo et al., 2015). However, high-quality litter can also stimulate the decomposition of older, more recalcitrant organic matter, a phenomenon known as the “priming effect” (Blagodatskaya and Kuzyakov, 2008). Both empirical (Van Groenigen et al., 2014; Craig et al., 2022) and model-based studies (Guenet et al., 2018) suggest that priming effects can attenuate increases in soil C under higher rates of organic matter input.
Finally, plants may also influence soil C cycling indirectly through their association with root symbionts, such as the ubiquitous mycorrhizal fungi that form associations with most terrestrial plants. Most species associate with either ectomycorrhizal or arbuscular mycorrhizal fungi, two functional groups with strongly divergent evolutionary histories, physiological traits, and life history strategies. The different nutrient acquisition mechanisms of ectomycorrhizal vs. arbuscular mycorrhizal fungi may have implications for soil C cycling: by directly competing with free-living decomposer microorganisms for nutrients, ectomycorrhizae could suppress rates of soil C turnover, leading to accumulation of organic matter (Averill and Hawkes, 2016; Fernandez and Kennedy, 2016). Meanwhile, arbuscular mycorrhizae may actually promote C turnover and loss by releasing exudates that stimulate decomposer activity (Cheng et al., 2012).
To predict tree species impacts on soil C, we must be able to tease apart the effects of productivity, tissue chemistry, and symbiont type on soil C, and assess the relative importance of these drivers. Because edaphic characteristics and plant traits are frequently confounded in natural ecosystems, tree common garden experiments are our best experimental tool to identify such directional relationships between plant traits and soil C stocks. In temperate and boreal forests, the majority of these experiments have shown that tree species identity strongly influences that mass of C held in the forest floor (Vesterdal et al., 2013). However, tree species influence on mineral soil C stocks–which are larger, more persistent, and yet slower to respond to environmental change–is much less certain. Some studies show interspecific differences in mineral soil C stocks (Mareschal et al., 2010; Gurmesa et al., 2013); yet in many comparable experiments, tree species identity does not have statistically significant effects on mineral soil organic matter, even three decades or more after trees were planted (Mueller et al., 2012). However, most tree common garden studies have focused on only a handful of species, strongly limiting statistical power to draw meaningful inference from these investigations. Because more productive tree species often have nutrient rich litter too, it can be difficult to identify the primary driver of soil C responses to afforestation: litter quantity or quality. Moreover, with insufficient cross-species comparisons, it is not possible to assess the generality of biogeochemical patterns within and among plant functional groups (broadleaf vs. conifer, arbuscular mycorrhizal vs. ectomycorrhizal).
Here, we leverage a biodiversity-ecosystem function experiment, the International Diversity Experiment Network with Trees (IDENT), to examine the accumulation of plant-derived C in mineral soils in relation to tree species identity and traits in the earliest stages of afforestation. We focused on the IDENT Montreal site, where nineteen tree species were planted in 2009 on a former agricultural field (Tobner et al., 2014). The species vary widely in their growth rates, leaf and root traits (Tobner et al., 2014; Jewell et al., 2017; Archambault et al., 2019), and mycorrhizal status. We measured soil bulk density and C content across tree species treatments to evaluate two hypotheses regarding the role of plant traits in mediating belowground C pools: Hypothesis (1) Tree species productivity and tissue quality should be positively correlated with soil C accumulation, such that fast-growing broadleaves will be associated with larger soil C stocks than slower-growing conifers. Hypothesis (2) The presence of ectomycorrhizal fungi should slow soil C turnover and allow soil C accumulation in plots dominated by ectomycorrhizal trees.
Materials and methods
Site description
The IDENT Montreal experiment was established in 2009 to examine the effects of taxonomic and functional biodiversity on ecosystem function. The experiment consists of 216 plots (16 m2, 64 trees per plot) randomly assigned to one of four taxonomic richness treatments: 1, 2, 4, or 12 tree species per plot, selected from a pool of 19 species (Table 1). All of our analyses focus on the 76 monospecific plots; these are allocated among four blocks, with one replicate of each of the 19 single-species treatments in each block. All trees were planted on a site (45.5°N, 73.9°W, MAT 6.2°C, MAP 963 mm) formerly managed by McGill University for high-intensity agriculture and crop research, with maize as the dominant crop (alfalfa and soy were planted in some years; M. Samoisette pers. comm.). Soils are coarse-textured, with 91% sand (Archambault et al., 2019), and prior to tree planting, soils had an organic matter content of 4.5% (total C was not directly measured) and a pH of 6.4.
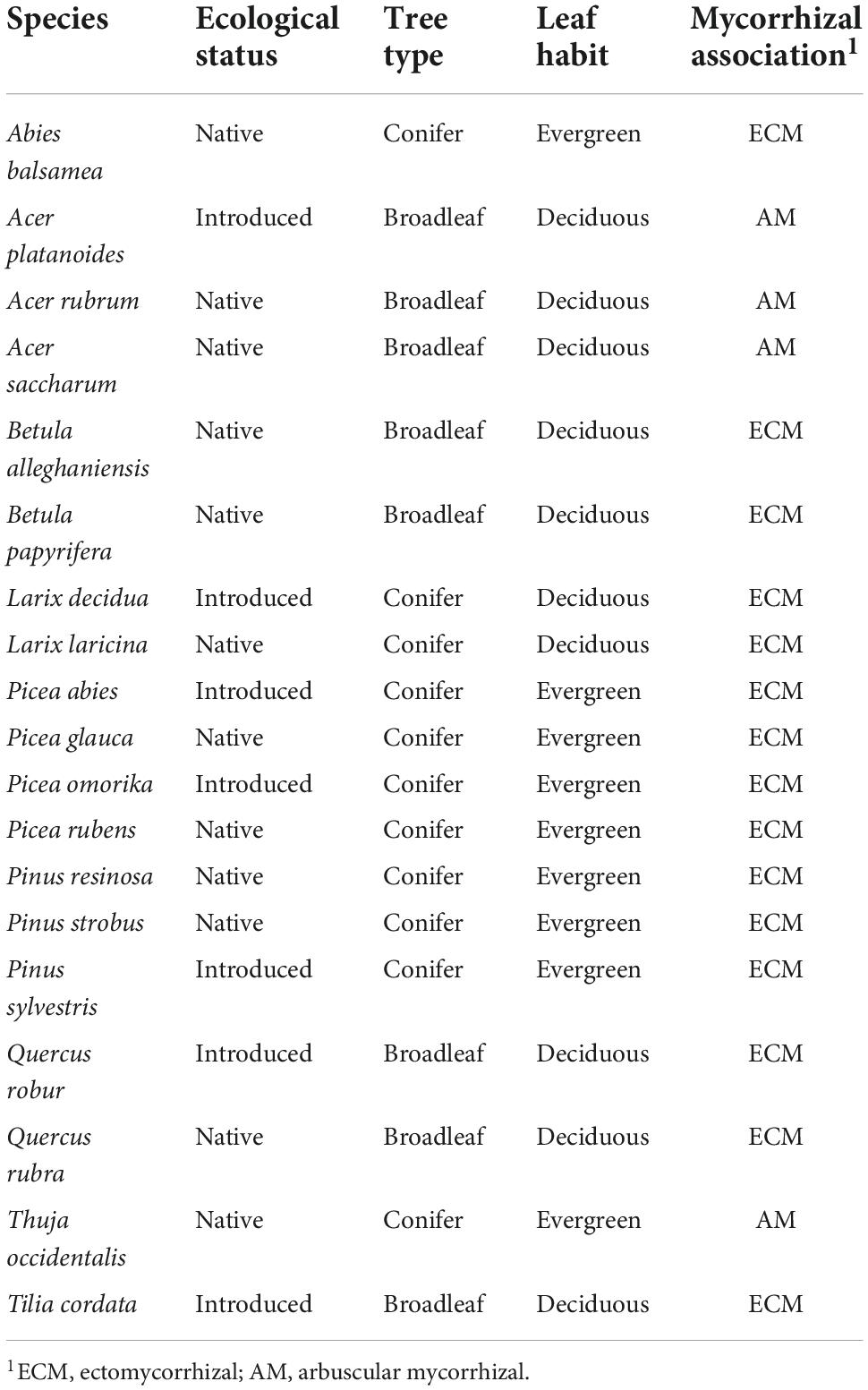
Table 1. Tree species planted in the monospecific plots at the Montreal biodiversity ecosystem function experiment with trees (IDENT) experiment.
Soil sampling and analysis
Soils were sampled from the monospecific plots in August 2017 (i.e., during the 9th growing season) to quantify soil C and N. Eight samples of mineral soil were obtained from each plot in a transect running across the midline, for a total of 608 unique samples, following manual removal of the litter layer (O horizon). Because shallow soil layers are most responsive to reforestation (Richter et al., 1999; Nave et al., 2013), cores were taken to a depth of 5 cm. Samples were immediately shipped to Utah State University where they were analyzed to determine total organic C and N.
To quantify soil C content, soils were sieved to 2 mm and ground prior to removal of inorganic C with sulfurous acid. Because we removed inorganic C from the samples, all of our measurements reflect organic C only; we henceforth use “C stock” interchangeably with “soil organic C stocks.” Samples were analyzed on a Costech 4010 Elemental Analyzer to quantify total organic C and N. We calculated the arithmetic mean of soil C values from the eight separate soil cores within a plot to obtain plot-level organic C content. We converted these C contents to stocks by multiplying by plot-specific soil bulk density, measured in each of the 76 monospecific plots in July 2020. For this we used a volumetric soil corer (volume 338 cm3) and took one core in the center of each plot, which was subsequently dried and weighed to calculate bulk density.
We analyzed soil data in relation to indices of plant productivity and plant tissue traits, measured by other collaborators in the same plots. Basal diameters of each tree in every plot were measured annually and used to calculate aboveground productivity. We determined stem basal area growth rates (cm2 y–1) over the 9-year study for each individual tree, excluding individuals that had died by the 2017 census. The average growth rate of individual stems within a plot was thereafter used as an estimate of aboveground stem growth rates at the plot scale. These rates were positively correlated with aboveground biomass measured in each plot (calculated by summing basal diameters of all living trees censused in 2017) (R2 = 0.425, P < 0.001), suggesting that rates of stem growth capture differences in total aboveground biomass production from planting to the time when soils were sampled in 2017. Leaf functional traits were taken from measurements conducted in the IDENT plots in 2012 by Jewell et al. (2017). These traits were wood density, specific leaf area, leaf lifespan, carbon and nitrogen content of litter (determined with an elemental analyzer), and litter decomposition rate (measured using litterbags deployed in situ for 184 days). Note that these functional trait data were collected only for the 12 native species included in the IDENT experiment (Table 1).
Statistical analyses
We used ANCOVAs to model bulk density, C content, and C stocks (i.e., the product of bulk densities and C content) as a function of species identity, plant functional type (ectomycorrhizal vs. arbuscular mycorrhizal; needle leaf [conifer] vs. broadleaf), and plot-specific stem growth rates. In addition to exploring variation in soil C pools among plots, we were also interested in exploring potential drivers of interspecific variation in C pools. For these species-level analyses, we calculated mean total C stocks for each species across all replicate plots. We used Spearman’s correlations to explore relationships among these species mean values and selected plant traits for the 12 native species: tissue C and N content of leaf litter; decomposition rate of species-specific litter; and specific leaf area, leaf lifespan, and wood density.
Finally, we used path model analysis to explore relationships among plant functional traits, litter traits, tree productivity, and soil C pools among species. To include plant functional traits in these models, we included species’ first principal component scores from a PCA conducted on wood density, specific leaf area, and leaf lifespan. The first principal component of this trait matrix (79.8% of variation) was positively associated with specific leaf area and negatively associated with leaf lifespan, capturing a common life-history trade-off along the leaf economic spectrum. To include litter traits in the path analysis, we conducted a PCA on leaf litter decomposition rate and litter C and N content; the first axis of this ordination (58.8% of variance) was positively correlated with litter C content and negatively correlated with decay rate. The final model included paths to reflect direct impacts of plant functional traits, litter traits, and productivity on soil C stocks, as well as a correlation between functional traits and productivity. All statistical analyses were conducted in R version 3.3.2; path analyses were carried out using the lavaan package (Rosseel, 2012).
Results
Rates of stem growth varied six-fold among species (F18,57 = 15.46, P < 0.001) and were 30% higher among broadleaves vs. conifers (t = 3.05, P = 0.003), while ectomycorrhizal and arbuscular mycorrhizal tree species did not exhibit different rates of growth (t = 0.16, P = 0.872). Soil bulk density varied 40% among species (F16,56 = 1.70, P = 0.075), from 1.43 g cm–3 under Quercus rubra to 1.02 g cm–3 in Picea rubens plots. Mineral soil bulk density was greater under broadleaves vs. conifers (F1,56 = 28.40, P < 0.001) and under arbuscular mycorrhizal vs. ectomycorrhizal trees (F1,56 = 6.90, P = 0.011) (Figures 1A,B). Soil C content also exhibited substantial though non-statistically significant variation among species (F16,56 = 1.18, P = 0.312) (Figure 2), but did not vary between plant functional types (P > 0.05) (Figures 1C,D).
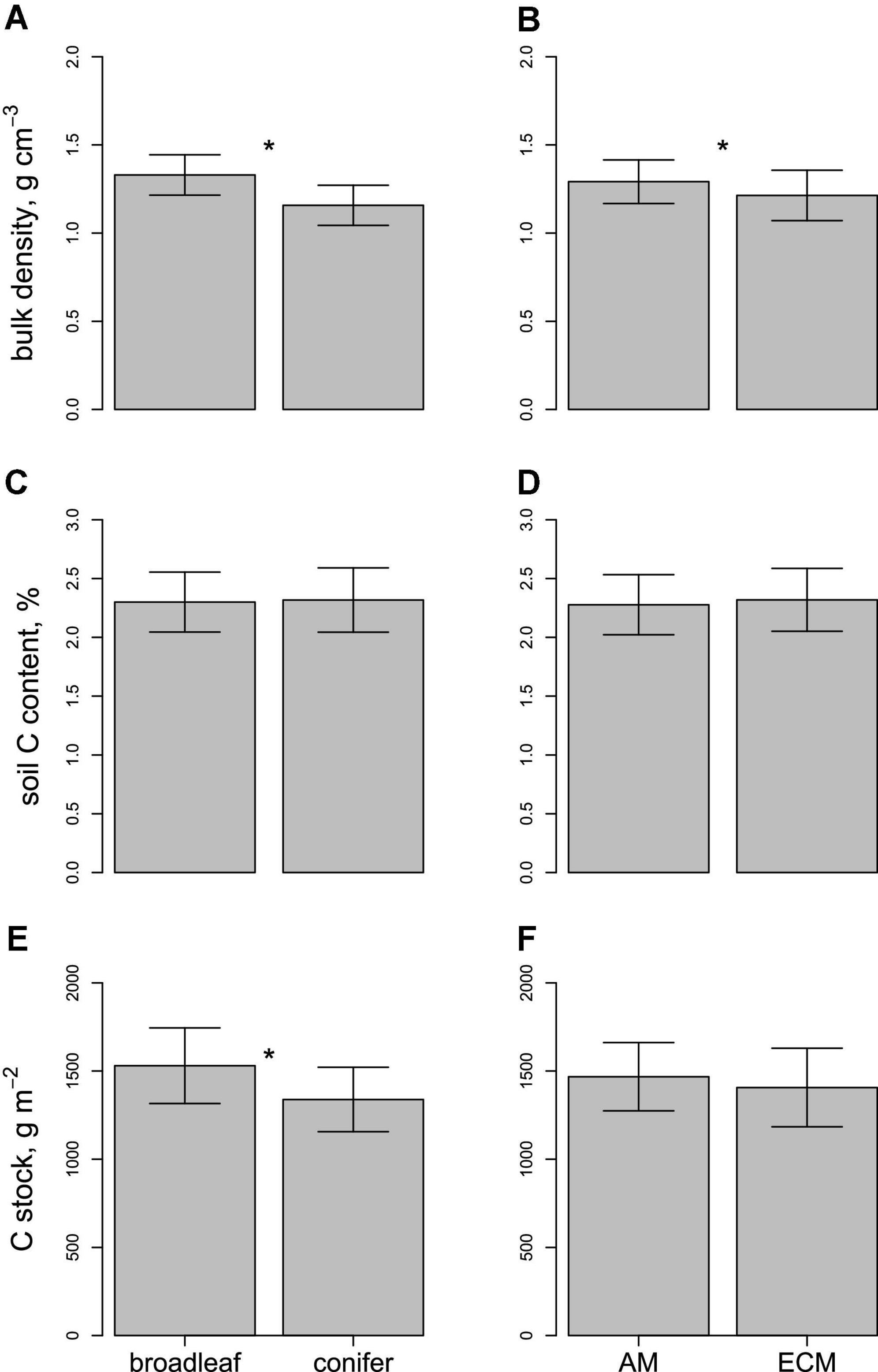
Figure 1. Variation in soil properties (bulk density, carbon content, and carbon stocks) beneath different plant functional groups (Panels A,C,E): broadleaves (N = 32 observations) vs. conifers (N = 44), (Panels B,D,F): arbuscular mycorrhizal trees (N = 16) vs. ectomycorrhizal trees (N = 60). Asterisks indicate significant differences among groups in the ANCOVA models. All soils were sampled to 5 cm depth. Error bars represent ± 1 SD.
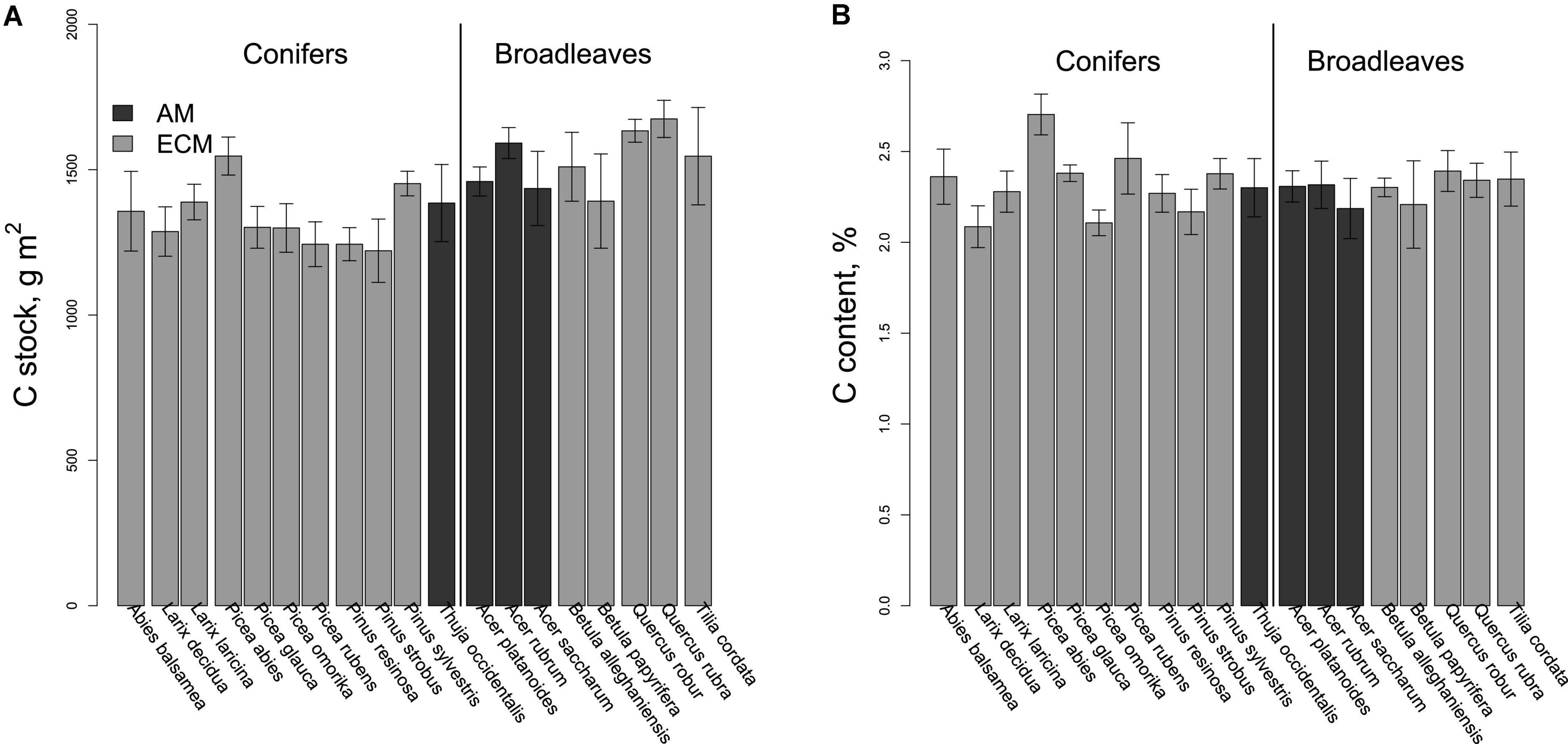
Figure 2. (A) Soil C stocks and (B). C content (5 cm depth) beneath the 19 species studied in the Montreal biodiversity ecosystem function experiment with trees (IDENT) experiment. Note that bars are grouped by genus. Error bars represent ± 1 SE (N = 4 plots per species).
Soil C stocks (i.e., the product of bulk density and C content) varied strongly among species, from 1.21 kg m–2 under Pinus strobus to 1.67 kg m–2 under Quercus rubra (Figure 2). Soil C stocks were positively correlated with rates of tree growth (Figure 3; Table 2) and were significantly higher beneath broadleaves vs. conifers (Figure 1E; Table 2), but did not vary between different mycorrhizal types (Figure 1F; Table 2). After controlling for plant leaf type, mycorrhizal association and growth rate, species identity did not have an impact on soil C pools (Table 2).
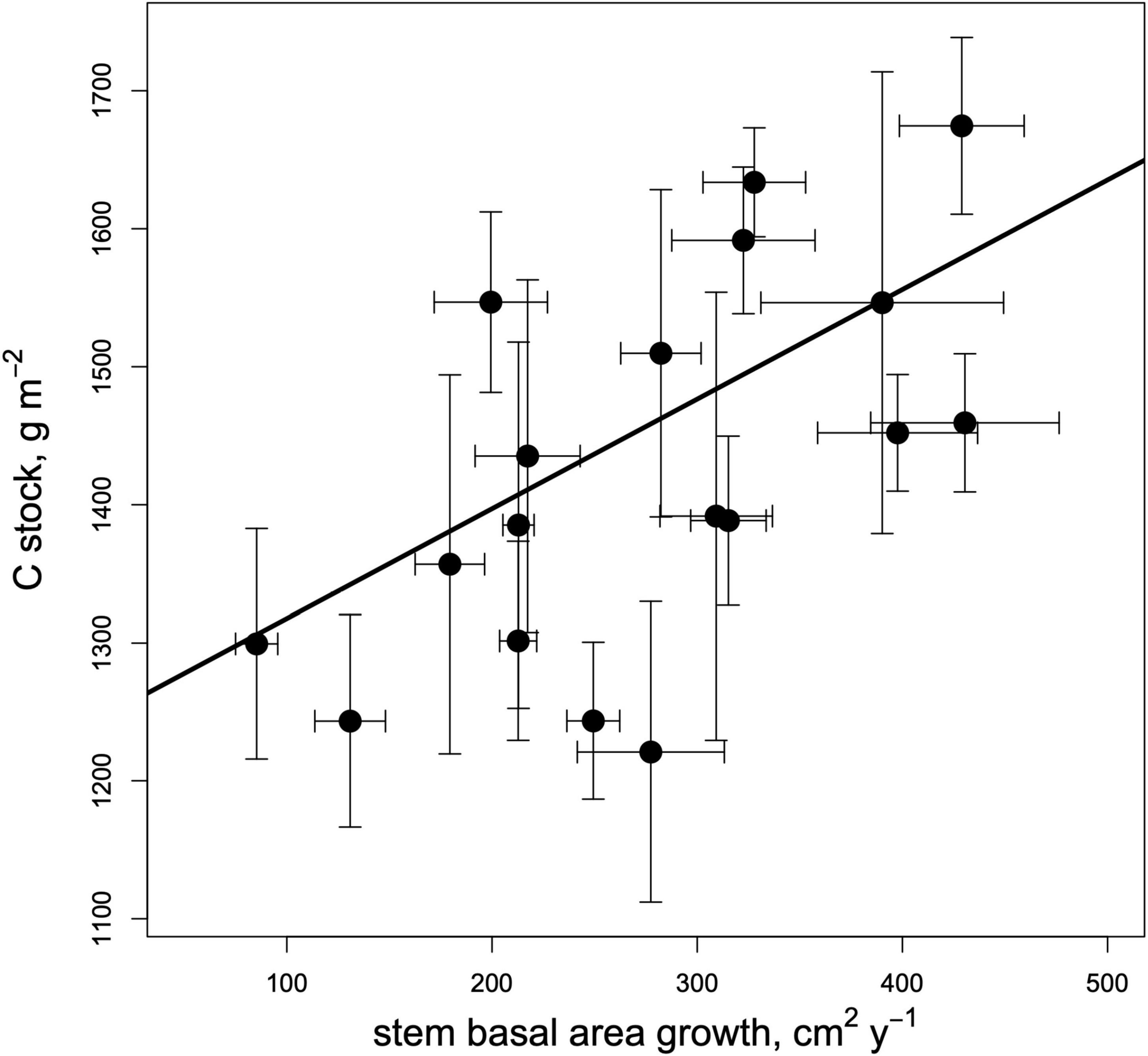
Figure 3. Relationship between species-mean soil C stocks (to 5 cm depth) and productivity (annual stem basal area increase) across the 19 trees species in the biodiversity ecosystem function experiment with trees (IDENT) Montreal common garden. Error bars (x and y) represent ± 1 SE (N = 4 plots per species).
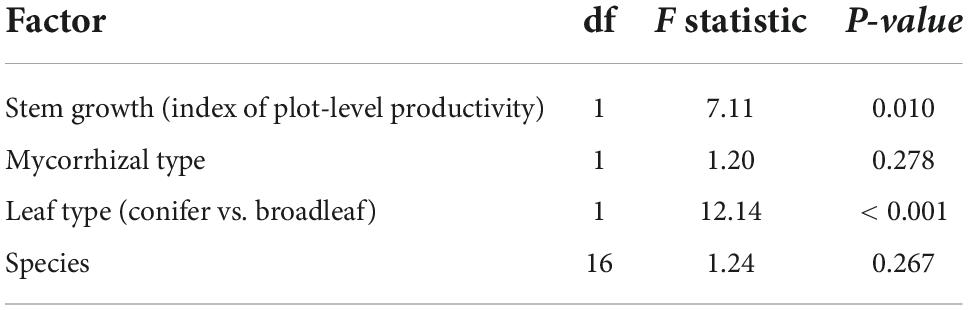
Table 2. Results of a ANCOVA model examining the effect of productivity, tree species identity, and tree functional type on soil C stocks.
Correlation analysis demonstrated that the tree species which exhibited the fastest rates of growth had functional traits on the “fast” end of the leaf economic spectrum including high specific leaf area, greater wood density and a shorter leaf lifespan (Table 3). However, productivity and leaf economic traits were largely unrelated to the C:N ratio of leaf litter and its rate of decay, indices which capture the chemical quality of organic matter inputs to soil (Table 3). Therefore, across the 12 species for which we had functional trait data, productivity and litter quality were not tightly coupled. Soil C stocks increased with stem growth rate as well as leaf litter decay rate (Table 3). When we explored these relationships using a path model (N = 12 observations, χ2 = 1.938, P = 0.164, CFI = 0.966, RMSEA = 0.292, AIC = 107.3), we found that plant functional traits had no direct impact on soil C stocks, but strongly shaped interspecific variation in productivity (Figure 4). In turn, soil C stocks were positively influenced by productivity and negatively influenced by litter decomposition resistance (in other words, leaf tissue that decomposed more slowly was associated with smaller soil C pools) (Figure 4).
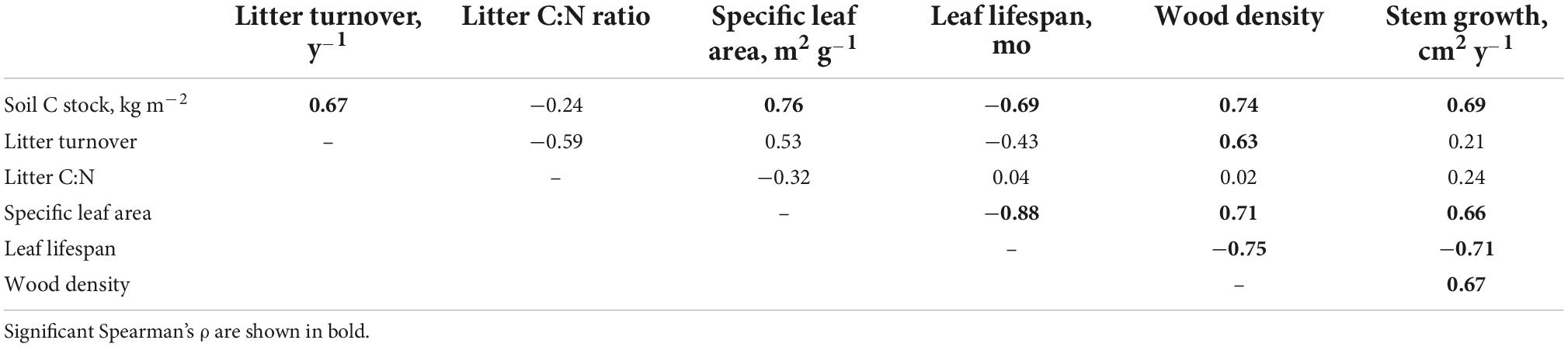
Table 3. Spearman’s correlations among species-specific soil C stocks, leaf functional traits, and litter characteristics across the 12 native tree species for which functional trait data were collected.
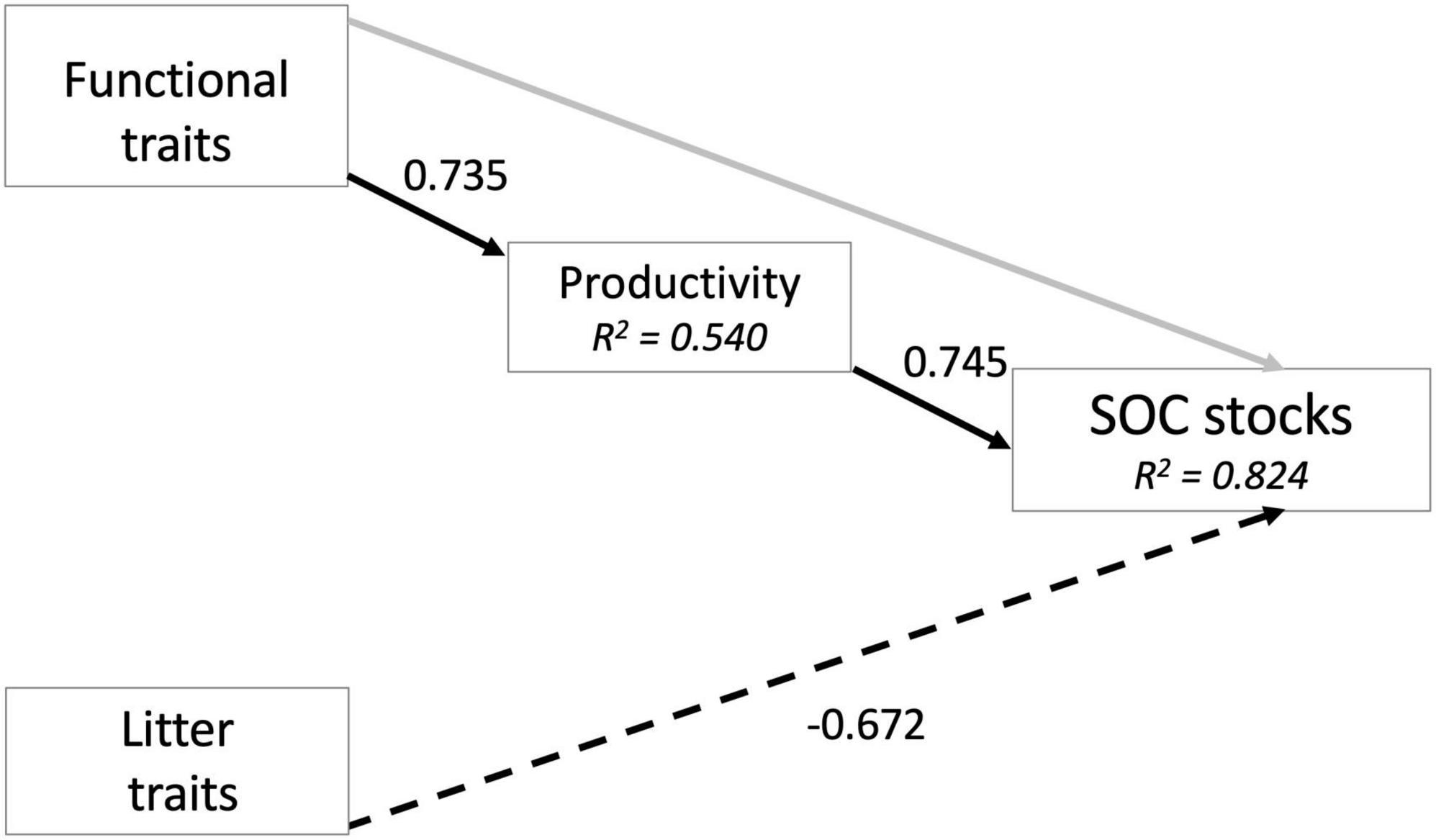
Figure 4. Path models demonstrating relationships among plant and litter traits, tree productivity, and species-specific soil organic C (SOC) stocks. Significant paths are shown in black, and non-significant paths in gray; dotted lines indicate negative relationships. Numbers beside each line are correlation coefficients.
Discussion
We leveraged a large tree common garden experiment to test two hypotheses regarding plant control over soil C stocks: that faster-growing tree species with high-quality tissues promote mineral C accumulation (Hypothesis 1); and that ectomycorrhizal tree species are associated with larger soil C stocks because they slow decomposition in the topsoil (Hypothesis 2). Below, we discuss the experimental evidence for each of our hypotheses.
Are changes in soil C detectable shortly after canopy closure?
Prior to assessing our hypotheses regarding the effects of species identity on soil C stocks, we must evaluate the power of our experimental design to detect any changes in soil C pools, if present. In a large-scale review of afforestation experiments, Paul et al. (2002) found that mineral soil C stocks frequently decrease in the first decade following planting, reflecting site preparation-induced disturbance; subsequent C gains are not reliably detectable for 30 years (Paul et al., 2002; Nave et al., 2013) or longer (Ashwood et al., 2019). Yet these temporal dynamics vary strongly among previous land uses and different plant functional groups (Paul et al., 2002), suggesting that the specific species chosen may influence our capacity to detect soil C responses to afforestation.
Here, we used a rigorous soil sampling campaign (> 600 cores in total) to identify changes in shallow mineral soil C stocks in young tree stands, and found that C pools varied 37% across 19 different species. These patterns in soil C stocks emerged from strong interspecific differences in soil bulk density and pronounced (although not statistically significant) cross-species variation in soil C content. These two parameters were not correlated with one another (P = 0.434) but exhibited similar magnitudes of interspecific variation, and so contributed jointly to the variation in soil C stocks across the 76 plots we surveyed. However, it is important to note that C stock patterns related to plant functional type (broadleaf vs. conifer) were driven entirely by differences in bulk density rather than C content, which did not vary between groups. Differences in soil bulk density may be due to bioturbation by earthworms (Lang and Russell, 2020), which readily respond to differences in tree species identity (Reich et al., 2005), or due to the effects of growing tree roots on soil pore structure (Helliwell et al., 2017). A previous study at our site found greater root biomass in evergreen vs. deciduous stands (Archambault et al., 2019), which may explain why soils were less dense below conifers. Other studies have also identified large changes in soil bulk density and soil structure among stands of different tree species (Morazzo et al., 2021) or following afforestation of cultivated land (Podrázský et al., 2015). Meanwhile, variation in C content is likely driven by plant litter quantity and quality, as discussed further below. Because we did not quantify total soil depth, vertical variation in mineral soil C content, or any changes in litter mass, we cannot definitively conclude that tree species have altered total belowground C stocks after 9 years. We also do not know whether there were pre-existing differences in soil abiotic properties (e.g., clay content) that may have influenced spatial patterns in soil C. However, our results demonstrate that tree species can influence shallow mineral C pools, even in the first decade of their growth, via effects on bulk density and C content.
Do plant functional traits affect soil C stocks?
We found that differences in soil C stocks were to some extent predictable based on tree traits. To maximize belowground C storage in young forest stands, we must identify the mechanisms that link plant traits and changes in soil C storage, and subsequently the correlations among different plant functional traits within and among species. Theory predicts that the species which exhibit the highest rates of growth are morphologically and physiologically adapted to maximize resource acquisition (Reich, 2014). In other words, the tree species which produce more biomass in a given time interval (larger quantity of soil C inputs) also have nutrient-rich leaves and roots (higher C input quality). If these parameters have opposing impacts on soil C accumulation, then the net impact of a fast-growing tree species on soil C stocks becomes difficult to predict.
We found evidence that faster-growing tree species (as indexed by stem growth rate) are associated with higher mineral soil C stocks in the earliest stages of afforestation, supporting our first hypothesis. This pattern was evident at multiple levels: between plant functional groups (productive broadleaves vs. slow-growing conifers), among species (as shown in the correlation analysis), and even across plots. Specifically, we explored the relationship between plot-specific stem growth rates and soil C stocks, independent of plant species identity, and found a weak but significant correlation (R2 = 0.061, P = 0.018). This finding is striking given that our index of plant growth rate is by no means a perfect metric of soil C inputs. For example, basal area increment may not be tightly linked to the production of fine roots, which are often the primary source of most organic soil C (Jackson et al., 2017). Moreover, since the stands we studied are young, the magnitude of aboveground C inputs may be more related to leaf habit than total aboveground biomass, because only one or a few cohorts of evergreen leaves may have senesced by the time we sampled soils.
Across the 12 tree species for which we had trait data, stem growth and functional traits were tightly correlated. The tree species which grew fastest had a high specific leaf area, dense wood, and a shorter leaf lifespan. The path analysis provides support for a strong indirect influence of plant functional traits on soil C stocks via their control over interspecific variation in productivity. Interestingly, however, leaf and wood economic traits were not tightly correlated with the C:N ratio or decomposition rate of leaf litter, suggesting that plant tissue quality (as perceived by microbial decomposers) is independent of species’ positions on the leaf economic spectrum. This observation is also supported by the path analysis, which found that that aboveground productivity (which was correlated with economic traits) and litter chemistry had equally strong, but opposing effects on soil C stocks. The C pool tended to be higher beneath species with fast-decaying litter, consistent with growing evidence that such litters are more efficiently converted into mineral-associated soil organic matter (Haddix et al., 2016; Sokol et al., 2019), but see Craig et al. (2022). More “decomposable” litter is often associated with greater bioturbation by litter fauna, accelerating organic matter movement into the mineral soil (Mareschal et al., 2010).
In the context of this experiment, we suggest that plant species identity could have influenced mineral soil C via three pathways which operate somewhat independently. First, faster-growing tree species enhance C stocks by increasing organic matter inputs. Second, species with more decomposable litter likely promote the conversion of plant tissue into the microbial by-products that are preferentially stabilized in mineral soils. Third, differences in soil bulk density between broadleaves and conifers contributed to the larger C stocks observed in the former group. We focused specifically on shallow soils because very young trees are not expected to have much impact on soil properties at depth. However, the higher soil C stocks under broadleaves may reflect a redistribution of mass across the soil profile, rather than a true gain in total belowground carbon. Our results are consistent with other reviews showing that broadleaf trees are associated with larger mineral soil C pools than conifers (Laganière et al., 2010; Mayer et al., 2020). Due to the young age of the stands and the high within-species variability in soil C content in mineral topsoils, additional observations will be necessary to confirm whether these patterns persist as the forest matures, and whether they occur over the entire soil profile.
Do plant-microbe interactions impact mineral soil C stocks early in stand development?
At the global scale, the presence of ectomycorrhizal-associated tree species is associated with increases in soil C storage relative to soil N content (Averill et al., 2014). This pattern may be driven by competition between ectomycorrhizal fungi and free-living decomposers, resulting in a suppression of soil C turnover and resulting soil C accumulation–i.e., the “Gadgil effect” (Gadgil and Gadgil, 1971; Fernandez and Kennedy, 2016). However, site-specific and modeling studies suggest that ectomycorrhizal trees may actually enhance soil C turnover via rhizosphere priming, as their exudation rates tend to be higher than those of arbuscular mycorrhizal trees (Brzostek et al., 2015; Sulman et al., 2017).
Contrary to our second hypothesis, we found no evidence that the presence of ectomycorrhizal trees systematically affected soil C pools, at least in the mineral topsoils examined here. It is possible that our sampling strategy failed to capture the influence of tree mycorrhizal type on accumulation of C in the litter layer, where ectomycorrhizal-induced suppression of decomposition may be most pronounced. Studies of mixed stands have shown that mycorrhizal type does not have a strong influence on the total amount of C in the soil profile, but rather its distribution, with more carbon in the topsoil beneath ectomycorrhizal species (Craig et al., 2018). Nonetheless, our data clearly show that variability in mineral C pools within mycorrhizal type is greater than that between groups, suggesting minimal impacts of mycorrhizae on mineral soils.
Strengths and limitations of the experimental design
The IDENT-Montreal tree common garden experiment examines a larger array of plant species than most comparable studies, enhancing our power to detect soil variation within and among plant functional groups, and unravel underlying mechanisms. Our study was intentionally designed to explore patterns in soil C stocks in the mineral soil (which is less responsive to land use change than the litter layer) and in the first decade following tree planting (when ecosystem C dynamics are not at steady state). The interspecific patterns we observed may change through stand development, reflecting both altered biomass allocation in maturing trees and changes in soil biogeochemistry as stands age, e.g., (Lajtha, 2020). Based on the data reported here, it would be premature to conclude that specific species are inherently more suitable for promoting belowground C accumulation. However, our results do show that even young trees can alter soil bulk density and C content. This is highly relevant for natural climate solutions that are intended to have an effect in the next few decades (Matthews et al., 2022), suggesting that changes in C cycling can occur very quickly, but that care must be taken to design sampling schemes which appropriately quantify the impacts on the total belowground C stock.
Although the IDENT-Montreal experiment was established to test specific ecological theories regarding the relationship between biodiversity and ecosystem processes, the plots studied here are quite representative of regenerating temperate and boreal forests. The density of tree seedlings planted (40,000 ha–1) mimics the extremely high proliferation of seedlings in naturally regenerating woodlands at the boreal/temperate forest ecotone [13,000–80,000 stems ha–1; (Brissette, 1996; Brissette et al., 1999; Bose et al., 2016)], and the species planted are all typical of the ecoregion. We do note that plots are relatively small, and movement of litter or fine roots across plot boundaries could weaken our ability to detect treatment effects. The same processes of litter movement must frequently occur in naturally occurring mixed temperate forests. Interestingly, the effects of individual plant species on soil properties is strong in forests worldwide, even in exceedingly diverse tropical forests where dozens of species co-occur at small spatial grains (Waring et al., 2015). At IDENT-Montreal, as in naturally occurring forests, it is likely that the dominant organic matter inputs to soils in any particular sampling location reflect the identity of the tree species immediately above them.
Conclusion
Examining species-specific impacts on soil C across a range of functionally distinct tree species can shed light on the mechanisms that link plant traits with belowground carbon storage. Here we show that faster-growing species, usually broadleaf trees with high-quality litter, are associated with larger soil C stocks in the earliest stages of afforestation. These patterns are driven by changes in bulk density and soil C content, although the latter did not vary significantly among species. Therefore, for the 19 species studied here, the tree species which sequester the most C in wood also tend to have beneficial effects on soil C stocks, at least when soils are compared over equal depths. These patterns are consistent with analyses of soil C dynamics following reforestation/afforestation at global scale, but contradict findings of other tree common garden experiments which fail to identify interspecific differences in soil C stocks or content after several decades. Additional research is urgently needed to confirm the generality of our findings, and assess how plants impact soil biogeochemistry throughout the course of stand development and across all soil horizons.
Data availability statement
The original contributions presented in this study are included in the article/supplementary material, further inquiries can be directed to the corresponding author.
Author contributions
BW, CM, and AP conceived the study. CM and AP provided access to the IDENT-Montreal experiment. KS, RK, and AM gathered and processed the data. MB contributed to the path analyses. BW analyzed the data and wrote the manuscript with input from KS, MB, RK, CM, AM, and AP. All authors contributed to the article and approved the submitted version.
Conflict of interest
The authors declare that the research was conducted in the absence of any commercial or financial relationships that could be construed as a potential conflict of interest.
Publisher’s note
All claims expressed in this article are solely those of the authors and do not necessarily represent those of their affiliated organizations, or those of the publisher, the editors and the reviewers. Any product that may be evaluated in this article, or claim that may be made by its manufacturer, is not guaranteed or endorsed by the publisher.
References
Archambault, C., Paquette, A., Messier, C., Khlifa, R., Munson, A. D., and Handa, I. T. (2019). Evergreenness influences fine root growth more than tree diversity in a common garden experiment. Oecologia 189, 1027–1039. doi: 10.1007/s00442-019-04373-5
Ashwood, F., Watts, K., Park, K., Fuentes-Montemayor, E., organic carbomBenham, S., and Vanguelova, E. I. (2019). Woodland restoration on agricultural land: Long-term impacts on soil quality. Restor. Ecol. 27, 1381–1392. doi: 10.1111/rec.13003
Averill, C., and Hawkes, C. V. (2016). Ectomycorrhizal fungi slow soil carbon cycling. Ecol. Lett. 19, 937–947. doi: 10.1111/ele.12631
Averill, C., Turner, B. L., and Finzi, A. C. (2014). Mycorrhiza-mediated competition between plants and decomposers drives soil carbon storage. Nature 505, 543–545. doi: 10.1038/nature12901
Binkley, D., and Giardina, C. (1998). Why do tree species affect soils? The warp and woof of tree-soil interactions. Biogeochemistry 42, 89–106. doi: 10.1023/A:1005948126251
Blagodatskaya, E., and Kuzyakov, Y. (2008). Mechanisms of real and apparent priming effects and their dependence on soil microbial biomass and community structure: Critical review. Biol. Fertil. Soils 45, 115–131. doi: 10.1007/s00374-008-0334-y
Bose, A. K., Weiskittel, A., Wagner, R. G., and Kuehne, C. (2016). Assessing the factors influencing natural regeneration patterns in the diverse, multi-cohort, and managed forests of Maine, USA. J. Veg. Sci. 27, 1140–1150. doi: 10.1111/jvs.12433
Bradford, M. A., Keiser, A. D., Davies, C. A., Mersmann, C. A., and Strickland, M. S. (2013). Empirical evidence that soil carbon formation from plant inputs is positively related to microbial growth. Biogeochemistry 113, 271–281. doi: 10.1007/s10533-012-9822-0
Brissette, J. C. (1996). Effects of intensity and frequency of harvesting on abundance, stocking and composition of naturally regeneration in the Acadian Forest of Eastern North America. Silva Fenn. 30, 301–314.
Brissette, J. C., Frank, R. M., Stone, T. L., and Skratt, T. A. (1999). Precommercial thinning in a northern conifer stand: 18-year results. For. Chron. 75, 967–972. doi: 10.5558/tfc75967-6
Brzostek, E. R., Dragoni, D., Brown, Z. A., and Phillips, R. P. (2015). Mycorrhizal type determines the magnitude and direction of root-induced changes in decomposition in a temperate forest. New Phytol. 206, 1274–1282. doi: 10.1111/nph.13303
Cheng, L., Booker, F. L., Tu, C., Burkey, K. O., Zhou, L., Shew, H. D., et al. (2012). Arbuscular mycorrhizal fungi increase organic carbon decomposition under elevated CO2. Science 337, 1084–1087. doi: 10.1126/science.1224304
Cotrufo, M. F., Soong, J. L., Horton, A. J., Campbell, E. E., Haddix, M. L., Wall, D. H., et al. (2015). Formation of soil organic matter via biochemical and physical pathways of litter mass loss. Nat. Geosci. 8, 776–779. doi: 10.1038/ngeo2520
Cotrufo, M. F., Wallenstein, M. D., Boot, C. M., Denef, K., and Paul, E. (2013). The microbial efficiency-matrix stabilization (MEMS) framework integrates plant litter decomposition with soil organic matter stabilization: Do labile plant inputs form stable soil organic matter? Glob. Change Biol. 19, 988–995. doi: 10.1111/gcb.12113
Craig, M. E., Geyer, K. M., Beidler, K. V., Brzostek, E. R., Frey, S. D., Stuart Grandy, A., et al. (2022). Fast-decaying plant litter enhances soil carbon in temperate forests but not through microbial physiological traits. Nat. Commun. 13:1229. doi: 10.1038/s41467-022-28715-9
Craig, M. E., Turner, B. L., Liang, C., Clay, K., Johnson, D. J., and Phillips, R. P. (2018). Tree mycorrhizal type predicts within-site variability in the storage and distribution of soil organic matter. Glob. Change Biol. 24, 3317–3330. doi: 10.1111/gcb.14132
Cusack, D. F., Halterman, S. M., Tanner, E. V. J., Wright, S. J., Hockaday, W., Dietterich, L. H., et al. (2018). Decadal-scale litter manipulation alters the biochemical and physical character of tropical forest soil carbon. Soil Biol. Biochem. 124, 199–209. doi: 10.1016/j.soilbio.2018.06.005
De Deyn, G. B., Cornelissen, J. H. C., and Bardgett, R. D. (2008). Plant functional traits and soil carbon sequestration in contrasting biomes. Ecol. Lett. 11, 516–531. doi: 10.1111/j.1461-0248.2008.01164.x
Fernandez, C. W., and Kennedy, P. G. (2016). Revisiting the “Gadgil effect”: Do interguild fungal interactions control carbon cycling in forest soils? New Phytol. 209, 1382–1394. doi: 10.1111/nph.13648
Gadgil, R. L., and Gadgil, P. D. (1971). Mycorrhiza and litter decomposition. Nature 233:133. doi: 10.1038/233133a0
Griscom, B. W., Adams, J., Ellis, P. W., Houghton, R. A., Lomax, G., Miteva, D. A., et al. (2017). Natural climate solutions. Proc. Natl. Acad. Sci. U.S.A. 114, 11645–11650. doi: 10.1073/pnas.1710465114
Guenet, B., Camino-Serrano, M., Ciais, P., Tifafi, M., Maignan, F., Soong, J. L., et al. (2018). Impact of priming on global soil carbon stocks. Glob. Change Biol. 24, 1873–1883. doi: 10.1111/gcb.14069
Gurmesa, G. A., Schmidt, I. K., Gundersen, P., and Vesterdal, L. (2013). Soil carbon accumulation and nitrogen retention traits of four tree species grown in common gardens. For. Ecol. Manag. 309, 47–57. doi: 10.1016/j.foreco.2013.02.015
Haddix, M. L., Paul, E. A., and Cotrufo, M. F. (2016). Dual, differential isotope labeling shows the preferential movement of labile plant constituents into mineral-bonded soil organic matter. Glob. Change Biol. 22, 2301–2312. doi: 10.1111/gcb.13237
Helliwell, J. R., Sturrock, C. J., Mairhofer, S., Craigon, J., Ashton, R. W., Miller, A. J., et al. (2017). The emergent rhizosphere: Imaging the development of the porous architecture at the root-soil interface. Sci. Rep. 7:14875. doi: 10.1038/s41598-017-14904-w
Jackson, R. B., Lajtha, K., Crow, S. E., Hugelius, G., Kramer, M. G., and Piñeiro, G. (2017). The ecology of soil carbon: Pools, vulnerabilities, and biotic and abiotic controls. Annu. Rev. Ecol. Evol. Syst. 48, 419–445. doi: 10.1146/annurev-ecolsys-112414-054234
Jewell, M. D., Shipley, B., Low-Décarie, E., Tobner, C. M., Paquette, A., Messier, C., et al. (2017). Partitioning the effect of composition and diversity of tree communities on leaf litter decomposition and soil respiration. Oikos 126, 959–971. doi: 10.1111/oik.03868
Kardol, P., De Deyn, G. B., Laliberté, E., Mariotte, P., and Hawkes, C. V. (2013). Biotic plant-soil feedbacks across temporal scales. J. Ecol. 101, 309–315. doi: 10.1111/1365-2745.12046
Laganière, J., Angers, D. A., and Paré, D. (2010). Carbon accumulation in agricultural soils after afforestation: A meta-analysis. Glob. Change Biol. 16, 439–453. doi: 10.1111/j.1365-2486.2009.01930.x
Lajtha, K. (2020). Nutrient retention and loss during ecosystem succession: Revisiting a classic model. Ecology 101:e02896. doi: 10.1002/ecy.2896
Lajtha, K., Bowden, R. D., Crow, S., Fekete, I., Kotroczó, Z., Plante, A., et al. (2018). The detrital input and removal treatment (DIRT) network: Insights into soil carbon stabilization. Sci. Total Environ. 64, 1112–1120. doi: 10.1016/j.scitotenv.2018.05.388
Lajtha, K., Townsend, K. L., Kramer, M. G., Swanston, C., Bowden, R. D., and Nadelhoffer, K. (2014). Changes to particulate versus mineral-associated soil carbon after 50 years of litter manipulation in forest and prairie experimental ecosystems. Biogeochemistry 119, 341–360. doi: 10.1007/s10533-014-9970-5
Lang, B., and Russell, D. J. (2020). Effects of earthworms on bulk density: A meta-analysis. Eur. J. Soil Sci. 71, 80–83. doi: 10.1111/ejss.12846
Liang, C., Schimel, J. P., and Jastrow, J. D. (2017). The importance of anabolism in microbial control over soil carbon storage. Nat. Microbiol. 2:17105. doi: 10.1038/nmicrobiol.2017.105
Mareschal, L., Bonnaud, P., Turpault, M. P., and Ranger, J. (2010). Impact of common European tree species on the chemical and physicochemical properties of fine earth: An unusual pattern. Eur. J. Soil Sci. 61, 14–23. doi: 10.1111/j.1365-2389.2009.01206.x
Matthews, H. D., Zickfeld, K., Dickau, M., MacIsaac, A. J., Mathesius, S., Nzotungicimpaye, C.-M., et al. (2022). Temporary nature-based carbon removal can lower peak warming in a well-below 2 °C scenario. Commun. Earth Environ. 3:65. doi: 10.1038/s43247-022-00391-z
Mayer, M., Prescott, C. E., Abaker, W. E. A., Augusto, L., Cécillon, L., Ferreira, G. W. D., et al. (2020). Influence of forest management activities on soil organic carbon stocks: A knowledge synthesis. For. Ecol. Manag. 466:118127. doi: 10.1016/j.foreco.2020.118127
Morazzo, G., Riestra, D. R., Leizica, E., Álvarez, L., and Noellemeyer, E. (2021). Afforestation with different tree species causes a divergent evolution of soil profiles and properties. Front. For. Glob. Change 4:685827. doi: 10.3389/ffgc.2021.685827
Mueller, K. E., Eissenstat, D. M., Hobbie, S. E., Oleksyn, J., Jagodzinski, A. M., Reich, P. B., et al. (2012). Tree species effects on coupled cycles of carbon, nitrogen, and acidity in mineral soils at a common garden experiment. Biogeochemistry 111, 601–614. doi: 10.1007/s10533-011-9695-7
Nave, L. E., Swanston, C. W., Mishra, U., and Nadelhoffer, K. J. (2013). Afforestation effects on soil carbon storage in the United States: A synthesis. Soil Sci. Soc. Am. J. 77, 1035–1047. doi: 10.2136/sssaj2012.0236
Paul, K. I., Polglase, P. J., Nyakuengama, J. G., and Khanna, P. K. (2002). Afforesttion and soil carbon. For. Ecol. Manag. 168, 241–257.
Podrázský, V., Holubík, O., Vopravil, J., Khel, T., Moser, W. K., and Prknová, H. (2015). Effects of afforestation on soil structure formation in two climatic regions of the Czech Republic. J. For. Sci. 61, 225–234. doi: 10.17221/6/2015-JFS
Reich, P. B. (2014). The world-wide “fast-slow” plant economics spectrum: A traits manifesto. J. Ecol. 102, 275–301. doi: 10.1111/1365-2745.12211
Reich, P. B., Oleksyn, J., Modrzynski, J., Mrozinski, P., Hobbie, S. E., Eissenstat, D. M., et al. (2005). Linking litter calcium, earthworms and soil properties: A common garden test with 14 tree species. Ecol. Lett. 8, 811–818. doi: 10.1111/j.1461-0248.2005.00779.x
Richter, D. D., Markewitz, D., Trumbore, S. E., and Wells, C. G. (1999). Rapid accumulation and turnover of soil carbon in a re-establishing forest. Nature 400, 56–57.
Rosseel, Y. (2012). lavaan: An R package for structural equation modeling. J. Stat. Softw. 48, 1–36.
Sokol, N. W., Kuebbing, S. E., Karlsen-Ayala, E., and Bradford, M. A. (2019). Evidence for the primacy of living root inputs, not root or shoot litter, in forming soil organic carbon. New Phytol. 221, 233–246. doi: 10.1111/nph.15361
Sulman, B. N., Brzostek, E. R., Medici, C., Shevliakova, E., Menge, D. N. L., and Phillips, R. P. (2017). Feedbacks between plant N demand and rhizosphere priming depend on type of mycorrhizal association. Ecol. Lett. 20, 1043–1053. doi: 10.1111/ele.12802
Terrer, C., Phillips, R. P., Hungate, B. A., Rosende, J., Pett-Ridge, J., Craig, M. E., et al. (2021). A trade-off between plant and soil carbon storage under elevated CO2. Nature 591, 599–603. doi: 10.1038/s41586-021-03306-8
Tobner, C. M., Paquette, A., Reich, P. B., Gravel, D., and Messier, C. (2014). Advancing biodiversity-ecosystem functioning science using high-density tree-based experiments over functional diversity gradients. Oecologia 174, 609–621. doi: 10.1007/s00442-013-2815-4
Van der Putten, W. H., Bardgett, R. D., Bever, J. D., Bezemer, T. M., Casper, B. B., Fukami, T., et al. (2013). Plant-soil feedbacks: The past, the present and future challenges. J. Ecol. 101, 265–276. doi: 10.1111/1365-2745.12054
Van Groenigen, K. J., Qi, X., Osenberg, C. W., Luo, Y., and Hungate, B. A. (2014). Faster decomposition under increased atmospheric CO2 limits soil carbon storage. Science 344, 508–509. doi: 10.1126/science.1249534
Vesterdal, L., Clarke, N., Sigurdsson, B. D., and Gundersen, P. (2013). Do tree species influence soil carbon stocks in temperate and boreal forests? For. Ecol. Manag. 309, 4–18. doi: 10.1016/j.foreco.2013.01.017
Waring, B. G., Álvarez-Cansino, L., Barry, K. E., Becklund, K. K., Dale, S., Gei, M. G., et al. (2015). Pervasive and strong effects of plants on soil chemistry: A meta-analysis of individual plant ‘zinke’ effects. Proc. R. Soc. B 282:20151001. doi: 10.1098/rspb.2015.1001
Keywords: biodiversity-ecosystem function experiments, functional traits, IDENT, soil carbon, tree plantation
Citation: Waring BG, Smith KR, Belluau M, Khlifa R, Messier C, Munson A and Paquette A (2022) Soil carbon pools are affected by species identity and productivity in a tree common garden experiment. Front. For. Glob. Change 5:1032321. doi: 10.3389/ffgc.2022.1032321
Received: 30 August 2022; Accepted: 08 November 2022;
Published: 24 November 2022.
Edited by:
Andreas Schindlbacher, Austrian Research Center for Forests (BFW), AustriaReviewed by:
Ingo Schöning, Max Planck Institute for Biogeochemistry, GermanyMelanie Brunn, University of Koblenz and Landau, Germany
Copyright © 2022 Waring, Smith, Belluau, Khlifa, Messier, Munson and Paquette. This is an open-access article distributed under the terms of the Creative Commons Attribution License (CC BY). The use, distribution or reproduction in other forums is permitted, provided the original author(s) and the copyright owner(s) are credited and that the original publication in this journal is cited, in accordance with accepted academic practice. No use, distribution or reproduction is permitted which does not comply with these terms.
*Correspondence: Bonnie G. Waring, bonnie.waring@gmail.com
†Present Address: Bonnie G. Waring, Department of Life Sciences, Grantham Institute on Climate Change and the Environment, Imperial College London, London, United Kingdom