- 1Research Institute of Forest Resource Information Techniques, Chinese Academy of Forestry, Beijing, China
- 2Key Laboratory of Forest Management and Growth Modeling, National Forestry and Grassland Administration, Beijing, China
- 3Industry Development and Planning Institute, National Forestry and Grassland Administration, Beijing, China
- 4Experimental Center of Tropical Forestry, Chinese Academy of Forestry, Pingxiang, China
Forest carbon and nitrogen storage significantly affect forest ecosystems and global carbon and nitrogen cycles. Forest management can achieve sustainable development by regulating stand structure. Therefore, the relationship between stand structure and soil carbon (SOCD) and nitrogen storage (SOND) needs in depth study. In this study, experiments were carried out in mixed and pure Pinus massoniana forests to analyze the effects of stand density, stand age, and their interaction on the change trends in SOCD and SOND in different soil layers. The results showed that, in upper (0–20 cm), middle (20–40 cm), and lower (40–60 cm) soil layers, with increased stand density, the SOCD of pure P. massoniana stands first increased and then decreased, while SOND increased monotonically; in mixed P. massoniana stands, SOCD and SOND both increased monotonically. In different development stages (young, middle-aged, and near-mature), the average SOCD of pure P. massoniana stands were 91.31, 88.56, and 85.98 t/ha, respectively, while the average SOCD of mixed P. massoniana stands were 55.92, 48.61, and 55.05 t/ha. The SOCD of pure P. massoniana stands was significantly higher than mixed P. massoniana stands at all growth and development stages. In pure P. massoniana stands, with increasing stand density, the SOCD of young, middle-aged, and near-mature stands first increased and then decreased, while the SOND increased monotonically. In the mixed P. massoniana stands, with increasing stand density, the SOCD of young, middle-aged, and near-mature stands increased monotonically, while the SOND of young stands increased initially and then decreased, while those of middle-aged and near-mature stands increased monotonically. These results emphasized that the artificial regulation of stand density at the appropriate development stage can maximize the carbon and nitrogen fixation potential of forest soil.
Introduction
The soil carbon and nitrogen pools of forest ecosystems are massive reservoirs that represent more than 70% of global carbon and nitrogen storage (Liu et al., 2007; Pan et al., 2011). Controlling the dynamic changes of soil carbon and nitrogen reserves would be indispensable for alleviating global warming and the sustainable development of forest ecosystems (Li M. M. et al., 2013). Forest soil carbon is preserved in many forms, mainly through soil respiration and carbon and nitrogen cycle exchange with aboveground stand and atmosphere. Therefore, a change in forest status will alter forest soil carbon and nitrogen storage [soil carbon (SOCD) and storage (SOND)] (Xu and Shang, 2016). The relationship between forest SOCD and SOND and stand structural characteristics must be understood to effectively manage forest ecosystems. Forest management can be an important driving force in regulating forest ecosystems (Hanan et al., 2018). Indeed, through the proper regulation of stand structure factors, such as density, tree species composition, and age structure, the soil carbon and nitrogen sequestration processes can be controlled to a certain extent (Nordmeyer et al., 2007; Vesterdal et al., 2013; Truax et al., 2018).
In recent years, studies have shown that stand structure has a significant effect on SOCD and SOND. Stand density is the main stand factor that forest management regulate stands because it affects soil temperature, humidity, and soil respiration rate (Cheng et al., 2015; Lei et al., 2018; Zhang et al., 2018). Stand density is often adjusted via thinning, which decreases stand canopy density, leading to increased stand surface runoff, aggravated soil erosion, and obvious changes in SOCD and SOND (Jurgensen et al., 2012; Dang et al., 2018). Thinning can also alter the local plant community, so forest management often implements artificial replanting, which forms mixed forests (Ming et al., 2018). Artificial replanting and natural regeneration will directly alter the composition of tree species, making a managed forest significantly different from a natural forest. Tree species composition is another important index of stand structure that affects the content of SOCD and SOND (Wang et al., 2010). In some cases, rapid growth by individual trees in pure forests can compensate for the initial SOCD and SOND losses due to thinning (Augusto et al., 2014). Studies have shown that mixed forest diversity is increased by both naturally regenerated trees and artificially planted trees, which can maintain the exchange balance between underground soil carbon and nitrogen and the aboveground stand (Hulvey et al., 2013; Jucker et al., 2014). In addition, mixed forests with diverse compositions can have enriched litter composition and enhanced litter decomposition rates, both of which affect the soil carbon cycle (Sun et al., 2016; Li et al., 2020).
The contents of SOCD and SOND can be significantly different in different stages of forest growth and development (Wu et al., 2020). In younger stages, the consumption of water and nutrients for tree growth is low, as is the intake of SOCD and SOND from the soil. With increasing age, the consumption of nutrients by trees increases, and the soil carbon and nitrogen from decomposed litter are also utilized more. The SOCD and SOND of stands also change dynamically with increasing stand age. In addition, this dynamic may be closely related to changes in stand structure, and may be affected by factors such as tree species composition and stand density (Na et al., 2021; Xia et al., 2021). With the growth of trees, the expansion of tree roots in soil increases correspondingly. With increased soil depth, the carbon and nitrogen in the soil exist in more stable forms that are not easily used by trees and are less affected by trees. Therefore, the influence of stand structure on SOCD and SOND is different at different depths (Sun et al., 2019). Indeed, topsoil has been seen to be more sensitive to changes in stand structure. The carbon and nitrogen input from litter decomposition and forest root precipitation complete the biochemical process in the surface soil. As it is processed, small amounts of carbon and nitrogen are transported into the deep soil in various forms. The stored carbon and nitrogen in the deep soil mainly comes from the soil itself (Yu et al., 2014; Witing et al., 2019). However, some studies have found that stand structure does not have different effects on deep soil and surface soil, and the specific respiration rate of deep soil can store unstable carbon and nitrogen in a progressive process, which is a key pathway by which carbon and nitrogen are input into deep soil (Guenet et al., 2012; Heitkötter et al., 2017). Based on the above research, this study explored the relationship between SOCD, SOND, and stand structure in the upper (0–20 cm), middle (20–40 cm), and lower (40–60 cm) soil layers.
Pinus massoniana is the main tree species used for afforestation in barren mountainous areas in subtropical areas. Forest management can improve the P. massoniana stand quality by adjusting stand structure (Zhang et al., 2020). Changes in stand structure will affect the exchange between soil and aboveground forest biomass, affect soil respiration rates, and subsequently affect SOCD and SOND. The relationship between the dynamic changes in forest SOCD and SOND and stand structure is of fundamental importance to forest management. Therefore, this study explored the relationship between forest SOCD and SOND and stand structure, primarily to guide and optimize the effectiveness of human intervention and forest management practices to achieve sustainable forest development.
Materials and methods
Study site
The study was conducted in the Tropical Forestry Experimental Center of Chinese Academy of Forestry, Pingxiang City, Guangxi Province, within latitudes 21°57′47″–22°19′27″ N and longitudes 106°39′50″–106°59′30″ E. The study area has a subtropical semi-humid-humid climate with distinct dry and wet seasons and abundant light, water, and heat resources. Historically, the mean annual rainfall for the area ranges between 1,200–1,500 mm and the annual evaporation ranges between 1,261–1,388 mm. The geomorphology is mainly mountainous and hilly, with an altitude range of 130–1,045.9 m, but most areas fall between 500–800 m. The soil-forming parent rocks are mainly argillaceous sandstone, gravelly limestone, granite, and limestone, and the soils are mainly red soil and latosol.
Experimental design
In order to study the relationship between stand structure and SOCD and SOND, selection of P. massoniana evenaged plantation of Tropical Forestry Experimental Center, initial density for 2,500 trees/ha. The P. massoniana plantation had been managed completely according to the rotation period management mode. We selected 90 standard sample P. massoniana plantation plots, including 45 pure P. massoniana plots and 45 mixed P. massoniana plots. The sample plots were circular plots of 400 m2. The mixed forest broad-leaved tree species mainly include Eucalyptus, Mytilaria laosensis, Castanopsis hystrix, etc. P. massoniana pure forest sample plots are those tree sample plots with more than 65% of the total stock of P. massoniana, and P. massoniana mixed forest sample plots are those tree sample plots with 35–65% of the total stock of P. massoniana (Meng, 2006). According to the established requirements of the experiment, the plots were selected so that there was no significant difference between stand factors (i.e., DBH and tree height) and site factors (i.e., altitude, slope, etc.) (Table 1). According to the growth characteristics of P. massoniana, the pure and mixed plots could be divided into different age classes: young forest (Mean stand age is 1–10 years), middle-aged forest (Mean stand age is 11–20 years), and near-mature forest (Mean stand age is more than 21 years), with 15 plots in each age group (Meng, 2006).
Field survey and soil sampling
In sample plots where trees have DBH ≥ 5 cm and young trees have heights > 30 cm and DBH < 5 cm, the DBH and tree height can be measured using the DBH ruler and ultrasonic tree altimeter. For each plot, the tree species names were recorded, the DBH and tree heights were measured, and the shrub species, numbers, and heights were recorded. Soil samples were collected according to the plum blossom method, and 1 kg of the upper, middle, and lower soils were collected according at 20 cm intervals in the east, west, south, north, and center of each plot area. The upper layer of soil was 0–20 cm, the middle layer of soil was 20–40 cm, and the lower layer of soil was 40–60 cm. The samples were air-dried and crushed, and then the fine roots of the plants were removed and samples were sealed for storage. Soil organic carbon was determined using the potassium dichromate method; and available nitrogen was determined via alkaline hydrolysis distillation (Qiu et al., 2019).
Data processing
We calculated the SOCD as follows (Kong et al., 2019):
where SOCD is soil organic carbon storage (t/ha); SOND is soil nitrogen storage (t/ha); SOC is soil organic carbon content (g/kg); N1 is soil organic nitrogen content (g/kg); h is soil thickness (h = 0.2 m); and ρ is soil bulk density (g/cm3).
First, a multi-factor variance analysis was conducted to analyze whether stand density, age group, and soil layer had significant effects on SOCD and SOND. Second, a linear mixed model was adopted to consider the effects of age group, soil layer, and their interaction on SOCD and SOND. The expressions were as follows (Hernández et al., 2016):
where a0 and a1 are the fixed parameters of the model; μj and μk are the random effects; εij, εik, and εijk are error term; Yij is the logarithmic conversion value of SOCD or SOND at ith sample of the jth age group; Yik is the logarithmic conversion value of SOCD or SOND at ith sample of the kth soil layer; and Yijk is the logarithmic conversion value of SOCD or SOND at ith sample of the kth soil layer and the jth age group. Nij is the stand density at ith sample of the jth age group; Nik is the stand density at ith sample of the kth soil layer; Nijk is the stand density at ith sample point of the kth soil layer and the jth age group. Age group, soil layer, and stand density are nested structure, such as in the same age group, several soil layers are nested in the same age group; in the same age group and soil layer, several stand density levels are nested in the same age group and soil layer.
Since the defined domain of the independent variable (stand density) in this study was small, a logarithmic conversion of soil carbon and nitrogen reserves was carried out, and the logarithmic conversion values of the soil carbon and nitrogen reserves were used to eliminate any autocorrelation or heteroscedasticity in the data. Data analysis and illustrations were completed in R 4.0.3 and the OriginPro software, respectively.
Results
Factors affecting soil carbon and storage
The multivariate analysis of variance (Table 2) showed that stand density and soil depth had significant effects on SOCD and SOND (P < 0.001). The interactions between stand age and soil depth and between stand density and soil depth significantly affected SOCD and SOND. Stand density and soil depth both significantly affected SOCD and SOND, the former above ground and the later underground, and the interaction between them was more sensitive than the interaction between stand age and soil depth.
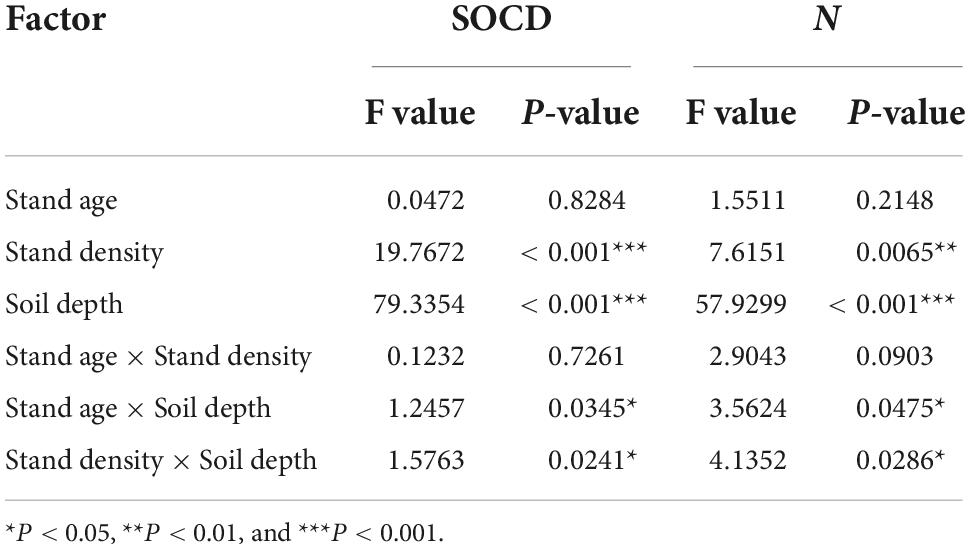
Table 2. Analysis results affecting soil carbon and N. In the table, asterisk represents statistical significance.
Soil carbon and storage at different depths in mixed and pure P. massoniana stands of different densities
The linear regression was able to accurately describe the effect of stand density on SOCD at different depths (Figures 1A,B). The change trends of SOCD in the upper, middle, and lower layers were similar to the trend with decreasing stand density. In the pure P. massoniana stands, with increased stand density, the SOCD in the upper, middle, and lower layers increased at first and then decreased. When the stand density was less than 1,500 trees/ha, the growth rate of SOCD in the upper layer was significantly higher than those in the middle and lower layers. When the stand density was 1,500–2,000 trees/ha, upper layer, the SOCD in lower layer and middle layer reached the peak, respectively. When stand density exceeded 1,500 trees/ha, the SOCD in upper, middle, and lower layers decreased with increasing stand density, and the rate of SOCD decrease in the upper layer was the fastest. In mixed P. massoniana stands, the SOCD of the upper, middle, and lower layers increased uniformly with increasing stand density. When the stand density increased to 2,500 trees/ha, the increases in SOCD in the upper, middle, and lower layers were 94.07, 53.07, and 29.83 t/ha, respectively.
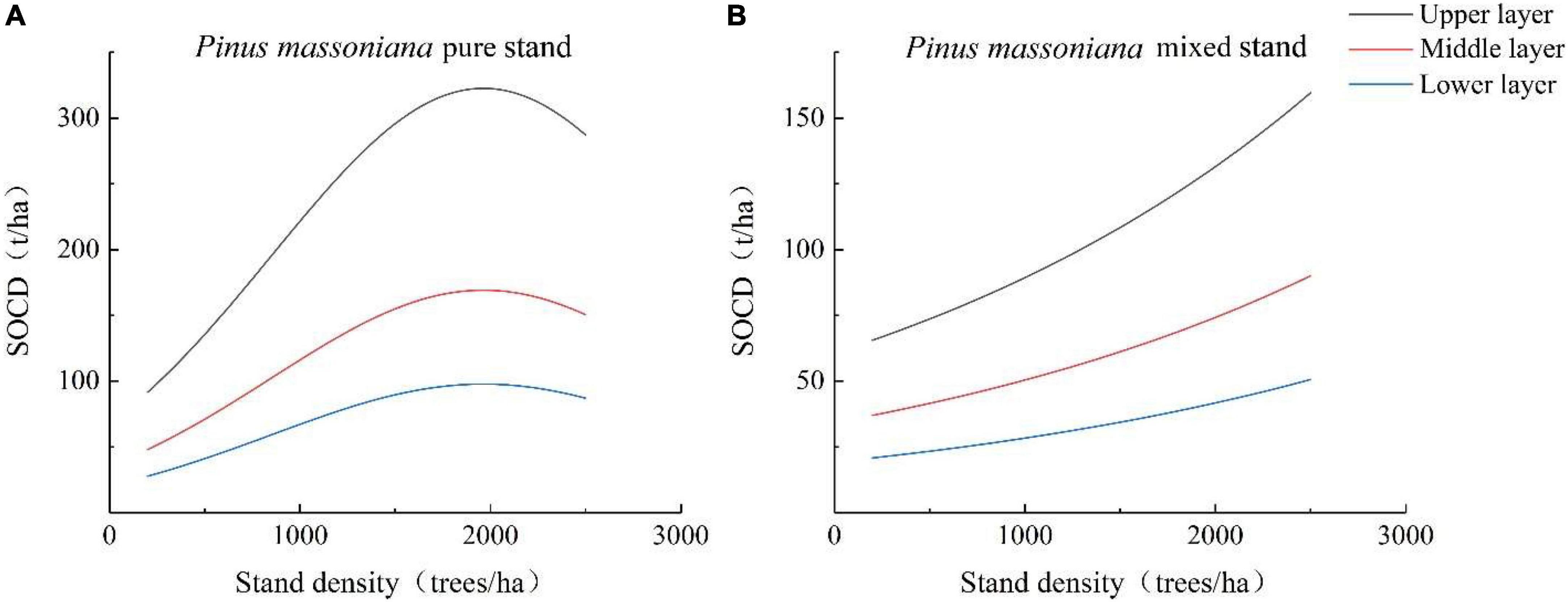
Figure 1. Soil carbon (SOCD) at different depths and stand densities. (A) SOCD at different depths and different stand densities in pure P. massoniana stands; (B) SOCD at different depths and different stand densities in mixed P. massoniana stand.
The SOND of upper, middle, and lower layers showed a “J” curve, and the SOND increased with increasing stand density (Figures 2A,B). The N contents of upper and middle layers were higher in pure P. massoniana stands than in mixed P. massoniana stands, while the SOND content of the lower layer was lower in pure P. massoniana stands than in mixed P. massoniana stands. With increasing stand density, the difference in the SOND contents of the upper and middle layers of the pure and mixed P. massoniana stands became larger, while the SOND content in the lower layer of the pure P. massoniana stand gradually increased and eventually surpassed that in the mixed stand. When the stand density increased to 2,500 trees/ha, the SOND contents in the upper, middle, and lower layers of the pure P. massoniana stand increased by 2.86, 2.15, and 1.33 g/kg, respectively, while those of the mixed P. massoniana stands increased by 1.63, 1.13, and 0.91 g/kg.
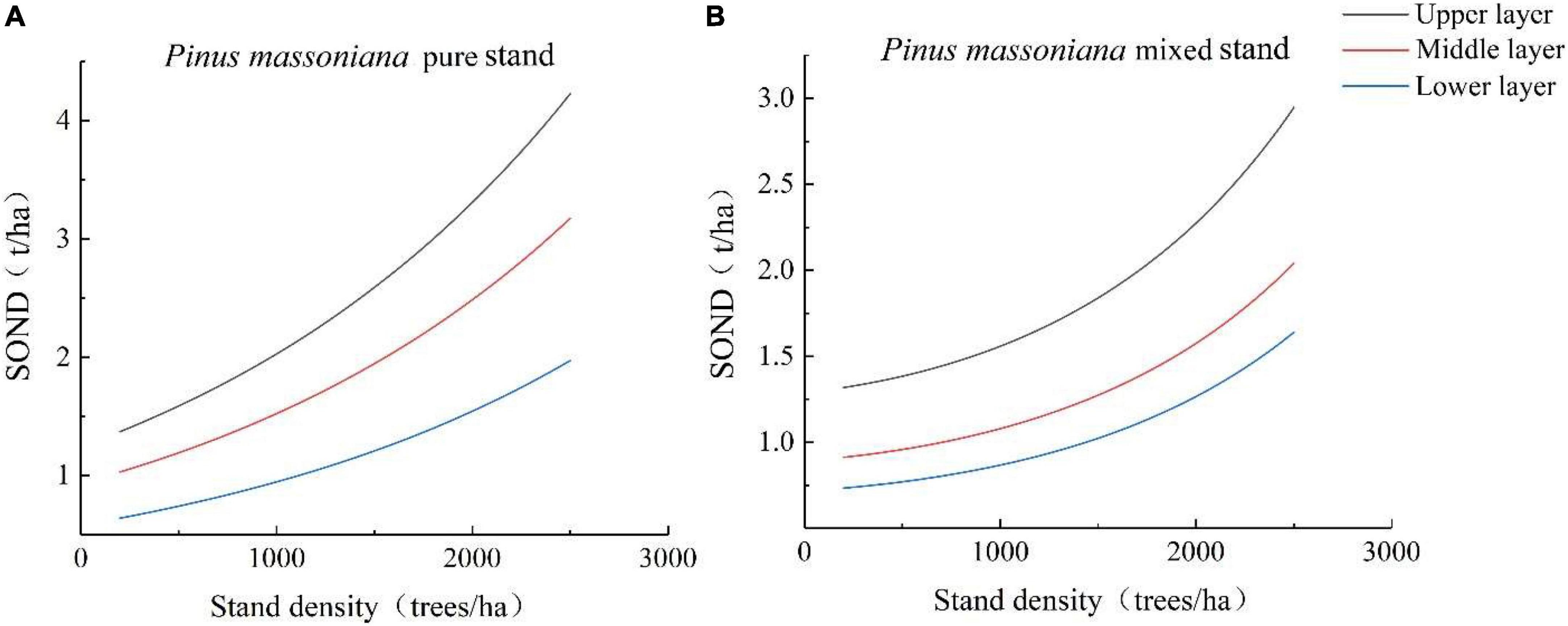
Figure 2. Storage (SOND) at different depths and stand densities. (A) SOND at different depths and stand densities in pure P. massoniana stands; (B) SOND at different depths and different stand densities in mixed P. massoniana stands.
Effects of age group on soil carbon and storage at different depths in pure and mixed stands of P. massoniana
According to the analysis of variance (Table 3; Figures 3A–D), there were significant differences in SOCD among the different soil layers in different age groups of pure and mixed P. massoniana stands. The SOCD of the upper, middle, and lower layers of the pure P. massoniana stands were significantly higher than those of the mixed P. massoniana stands. The SOND contents in the upper layers of both pure and mixed P. massoniana stands were significantly different from those of the middle and lower layers, and there were no significant differences between middle and lower layers. There were no significant differences in SOND content between the upper, middle, and lower layers of either pure or mixed P. massoniana stands.
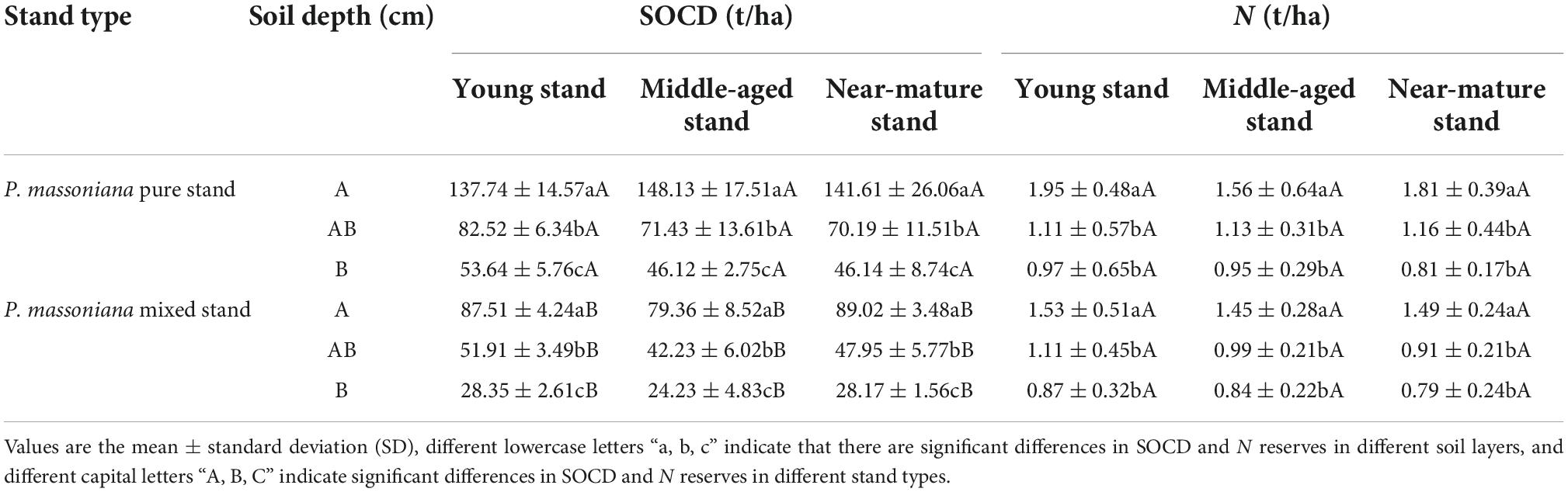
Table 3. Analysis results of soil carbon and N of different age groups and different depths in pure and mixed stands of P. massoniana.
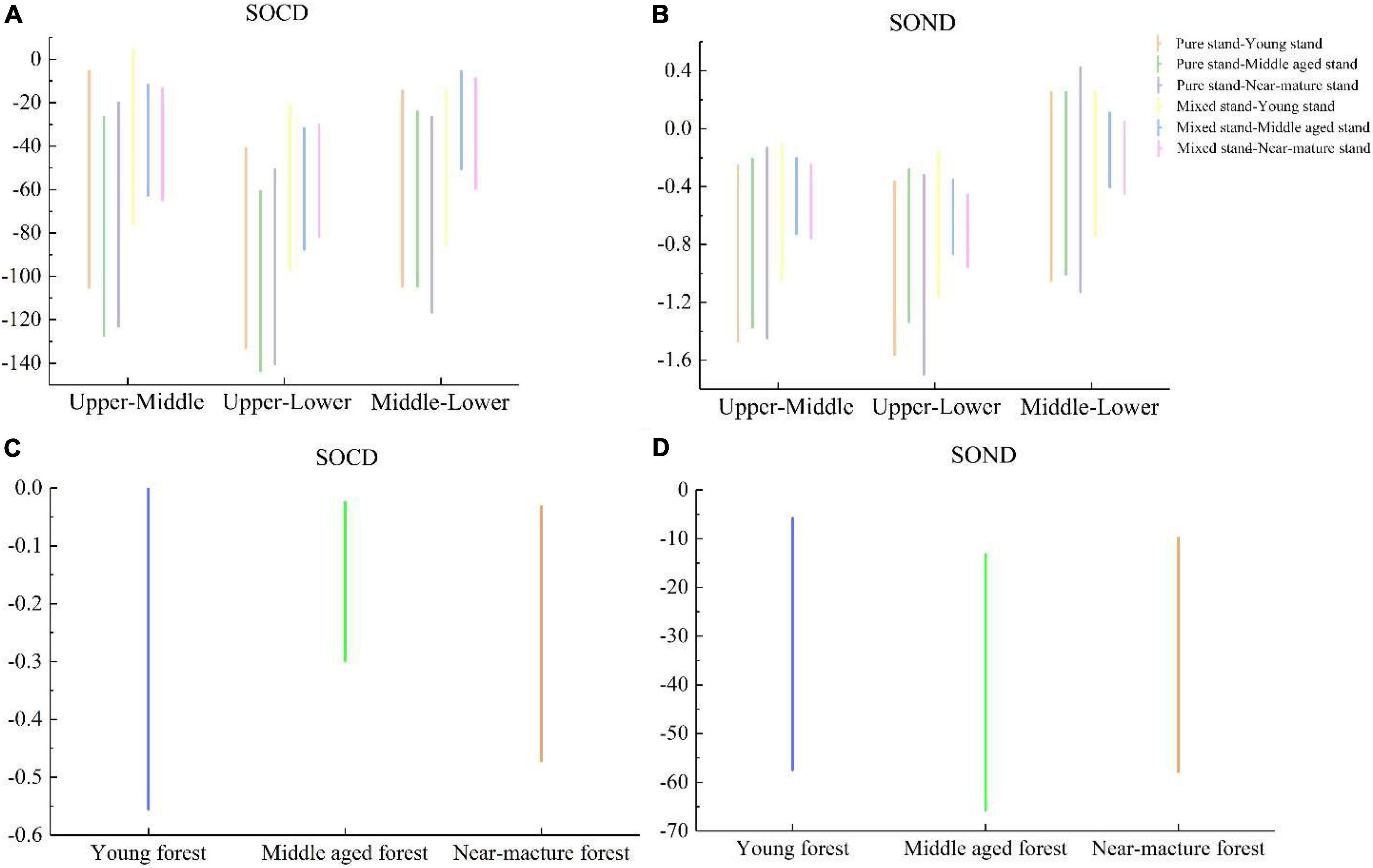
Figure 3. Analysis of variance results. (A) 95% family-wise confidence level for SOCD in different age groups in different soil layers; (B) 95% family-wise confidence level of SOND in different age groups; (C) 95% family-wise confidence level of SOCD in mixed and pure P. massoniana stands; (D) 95% family-wise confidence level of SOND in mixed and pure P. massoniana stands.
The SOCD decreased with increasing soil depth in all different age groups of both pure and mixed P. massoniana stands, albeit at different rates (Figures 4A–C). The changes in SOCD of different age groups in the upper, middle, and lower layers were consistent with the trends observed with changes in stand density. When the stand density was 1,000–1,500 trees/ha, the SOCD of the middle-aged P. massoniana pure stand peaked. At stand densities of 1,500–2,000 and 2,000–2,500 trees/ha, the SOCD of the near-mature and young stands peaked, respectively. Compared with the initial SOCD, the SOCD peaks of the middle-aged, young, and near-mature pure P. massoniana stands increased by 3.5, 1.7, and 4.1 times, respectively. When the stand density increased to 2,500 trees/ha, the SOCD of middle-aged, young, and near-mature mixed P. massoniana stands increased by 0.85, 1.59, and 2.69 times, respectively.
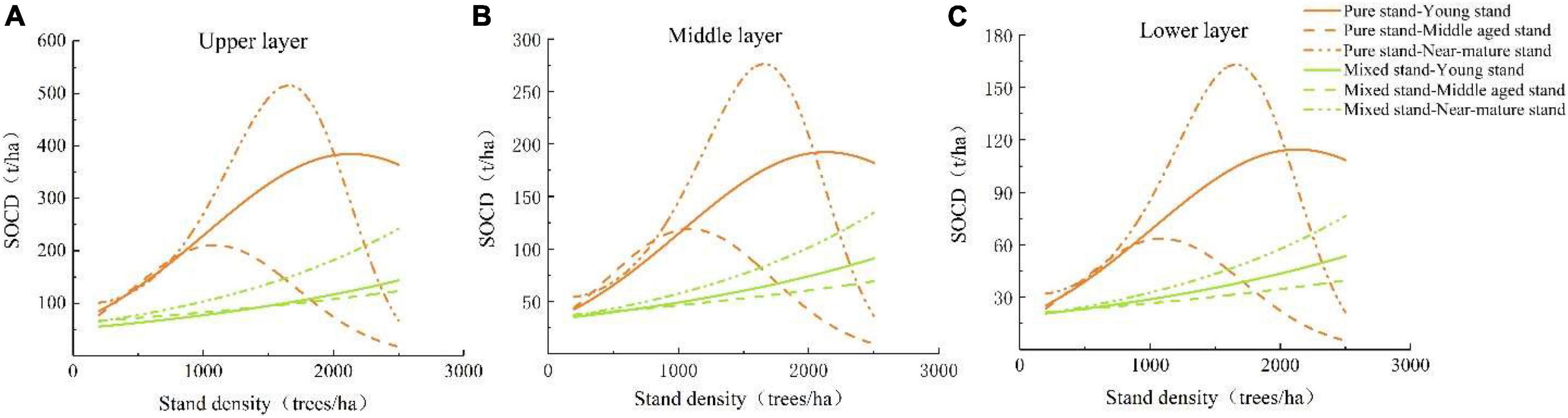
Figure 4. Soil carbon (SOCD) with different stand densities and different age groups at different depths in pure and mixed P. massoniana stands. (A) SOCD in the upper soil layer with different stand densities and different age groups in mixed and pure P. massoniana stands; (B) SOCD in the middle soil layer with different stand densities and different age groups in mixed and pure P. massoniana stands; (C) SOCD in the lower soil layer with different stand densities and different age groups in mixed and pure P. massoniana stands.
The SOND contents of different age groups of pure and mixed P. massoniana stands decreased with increasing soil depth (Figures 5A–C). Overall, in the upper, middle, and lower layers, the SOND contents of different age groups of the pure P. massoniana stands increased with increasing stand density. The young mixed P. massoniana stands increased initially, but then decreased with increasing stand density, while the middle-aged and near-mature stands increased continuously. With increasing stand density, the SOND contents of young pure P. massoniana stands were always higher those that of the mixed P. massoniana stands the in upper and middle layers, but at densities between 500–1,200 trees/ha, the SOND content of the middle layers of mixed P. massoniana stands were briefly higher than the middle layer of the young pure P. massoniana stands.
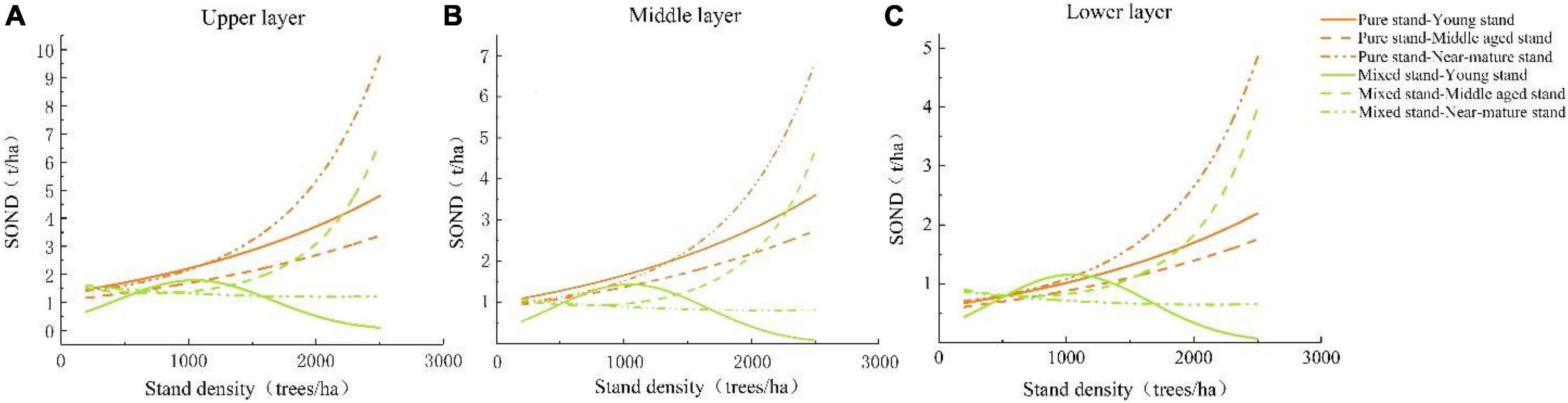
Figure 5. Storage (SOND) with different stand densities and different age groups at different depths in pure and mixed P. massoniana stands. (A) SOND in the upper soil layer with different stand densities and different age groups in mixed and pure P. massoniana stands; (B) SOND in the middle soil layer with different stand densities and different age groups in mixed and pure P. massoniana stands; (C) SOND in the lower soil layer with different stand densities and different age groups in mixed and pure P. massoniana stands.
Discussion
Effect of stand density on soil carbon and storage of pure and mixed P. massoniana stands
Forest type, stand density, and age group structure do not provide a sufficiently comprehensive assessment for effective forest management, so other important influencing factors like the relationships between soil carbon and nitrogen and stand structure must be considered (Ali et al., 2019). The SOCD of pure P. massoniana stands and SOND of mixed P. massoniana stands of different densities exhibited significant differences. The change trends of SOCD in pure and mixed P. massoniana stands with different densities were different, while the change trends of SOND were roughly similar across different stand densities. With increases in stand density, the SOCD of pure P. massoniana stands initially increased, but then decreased, while the SOCD of mixed P. massoniana stands increased monotonically. As for the interpretation rate of biological characteristics, the interpretation rate of SOCD was higher and tended to be more stable at higher stand densities. Most research to date has shown that stand density has a significant effect on SOCD, but the effect of stand density on SOCD is indirect, acting through its direct effect on soil respiration (Kuzyakov and Gavrichkova, 2010; Olajuyigbe et al., 2012; Bujalskı et al., 2014). Soil respiration is an important soil carbon loss pathway and it is significantly affected by climate characteristics such as soil temperature and humidity. The regulation of stand density will produce obvious changes in local microclimates, therefore soil respiration will be significantly enhanced or weakened. When the stand density is too high or too low, soil respiration can be restricted. Therefore, through soil respiration, stand density will indirectly affect soil carbon storage. As stand density increases, soil respiration will increase until peaking, at which point the soil carbon storage will also peak; continued increases in stand density will then lead to decreases in respiration and soil carbon storage, resulting in an “S” curve change trend (Colman and Schimel, 2013; Shao et al., 2013; Liu et al., 2021). The difference of soil aggregation size distribution indicates that plant root input and litter decomposition play a key role in the change of SOCD (Oades, 1993). Macro-aggregates can absorb carbon quickly, while micro-aggregates absorb carbon slowly, but the storage form of carbon will be more stable (Li Y. L. et al., 2013). The pure forest has a single tree species, and most of the soil is dominated by macro-aggregates, while mixed forest has larger soil diversity and soil pore space, which makes it easier for soil to form micro poly collective. With the increase of stand density, the macro-aggregates in pure P. massoniana forest soil quickly absorb the carbon decomposed by litter, which leads to the increase of SOCD. However, coniferous species mainly produce low-quality litter, and there are many substances that are difficult to decompose (such as lignin, cellulose, etc.) (Shi et al., 2018). A small amount of micro poly collectively absorb carbon more slowly, and the soil carbon absorbed by macro-aggregates is unstable and gradually loses, which leads to the decrease of SOCD. In the mixed forest, fast-growing broad-leaved tree species such as Eucalyptus, Mytilaria laosensis, Castanopsis hystrix, etc., mainly produce high-quality litter. The litter accumulated by the increase of stand density is slowly decomposed and absorbed by a large number of micro-aggregates, forming stable soil carbon (Cotrufo et al., 2019; Lavallee et al., 2020), and the SOCD increases monotonically.
The SOCD and SOND of mixed P. massoniana stands increased with increasing stand density, which was consistent with the study by Laganiere et al. (2017) of the soil carbon cycle balance, which observed a specific “threshold” at which capacity limitations were reached at the input and output ends of the carbon and nitrogen cycles. According to the afforestation principle of mixed forests, with increased or decreased stand density, the complementary tree species in mixed stands will show relative increases or decreases, which is the theoretical equivalent to enlarging or reducing the specific “threshold” of soil carbon and nitrogen cycles according to a specific multiplier, while the SOCD and SOND showed relative exponential growth, or “J”, curves (Son et al., 1999; Kuzyakov and Larionova, 2005). With increasing stand density, mixed P. massoniana stands became predominantly composed of small-diameter trees, which accounted for ∼56%. In the mixed P. massoniana stands, most of the naturally regenerated tree species that met the standards of imported wood were tree species considered complementary for afforestation. As the undergrowth seedlings increasingly meet the standards for imported wood, the carbon fixation and nitrogen fixation in forest ecosystem will increase, and the SOCD and SOND reserves will also naturally increase. Mixed P. massoniana stands have a stronger resilience to climate change disturbance because of their tree species diversity and stable stand structures. Local climate changes caused by the regulation of stand density can promote the cyclic conversion between understory vegetation and soil carbon, thus contributing to the long-term preservation of SOCD (Silva et al., 2015).
Differences in soil carbon and storage between pure and mixed P. massoniana stands
The SOCD of the pure P. massoniana stands was significantly higher than that of the mixed P. massoniana stands, while the difference in SOND was not significant. This conclusion was different from previously published reports (e.g., Guckland et al., 2009; Schleuß et al., 2014). Specifically, comparing pure to mixed P. massoniana stands, it could be seen that artificially replanted and naturally renewed broad-leaved tree species grew slower than P. massoniana, which is a fast-growing tree species. Therefore, the pure stands could form a main forest layer more quickly, thereby reducing light penetration to the understory and inhibiting the growth of herbs and shrubs, which is matched by corresponding increases in litter and SOCD (Dawud et al., 2017; Terrer et al., 2021). However, as broad-leaved species of mixed stand gradually grow into the main forest layer, the canopy density gradually increases and exceeds that of pure stands, and the SOCD of the mixed stand will also increase. In this study, while the soil in the upper layer was strongly affected by surface runoff, the differences in the SOCD of the pure and mixed stands in the middle and lower layers decreased with increasing age, from 30.61 to 22.24 and from 25.29 to 17.97. This conclusion was supported by previous studies which have shown that the characteristics of species or existing functional groups significantly impact soil carbon storage, with coniferous species having a stronger adaptability to environmental change than broad-leaved species, and coniferous species also having a more positive impact on root biomass (soil carbon input), which can promote increases in SOCD (Dawud et al., 2016; Finér et al., 2017; Ratcliffe et al., 2017).
Effect of stand age on soil carbon and storage in pure and mixed stands of P. massoniana
In this paper, the differences in SOCD and SOND between pure and mixed stands of P. massoniana in different age groups and their change trends with changing stand density were studied. The SOCD of different age groups of P. massoniana pure stand increased initially and then decreased with increasing stand density, and the SOCD of different age groups of mixed P. massoniana stands increased with increasing stand density. Among the peaks of SOCD of each age group of the pure P. massoniana stands, that of the near-mature stand was highest, possibly because there was obvious difference in the number of large-diameter trees among different age groups. However, with increasing age, the litter layer will form a thicker layer of coarse humus and the abundance of strong enzymatically-active microorganisms will be higher, which will enhance the soil carbon cycle and increase the soil carbon content (Prieto et al., 2012; Stevenson et al., 2014; Xu et al., 2021). The N of young mixed P. massoniana stands increased initially and then decreased with increasing stand density. In the young stands the canopy was in the developing stage, this meant that the canopy density was low and the shading effect in the upper story was poor, so the water and light resources in the young stands were relatively abundant. The SOND content increased gradually as soil ammoniation and nitrification were promoted. With increasing stand density, tree crown growth, canopy density, and upper shading effect gradually increased, but soil ammoniation and nitrification slowed as resources became limited, thus N decreased. Deep soil nitrogen exists in a more stable form, and its sensitivity to aboveground stand changes gradually decreases with depth (Mayer et al., 2020).
Conclusion
Through qualitative analysis of linear mixed models, it was found that stand density had a significant impact on SOCD and SOND in pure and mixed stands of P. massoniana. With increasing stand density, SOCD and SOND in pure P. massoniana stands first increased and then decreased, while SOCD and SOND in mixed P. massoniana stands increased monotonically. During the growth and development of trees, the SOCD and SOND trends in young, middle-aged, and near-mature pure and mixed P. massoniana stands were consistent with the increases in stand density. For SOND content, that of young mixed P. massoniana stands first increased and then decreased, while those of middle-aged and near-mature stands increased monotonically. Taking corresponding management measures in the appropriate growth and development stages and regulating stand density can therefore effectively realize the maximum carbon and nitrogen fixation potential of the stand, thus maximizing the SOCD and SOND of the stand. There were significant differences in SOCD of different stand types. This paper showed that the SOCD of pure P. massoniana stands in each growth and development stage was significantly higher than that of mixed stands. Under the rotation management scheme, pure P. massoniana stand has higher storage potential, which further shows that regulating stand density can directly and effectively affect SOCD.
Data availability statement
The raw data supporting the conclusions of this article will be made available by the authors, without undue reservation.
Author contributions
KZ and XL: conceptualization, data curation, and writing—review and editing. KZ and HG: methodology. KZ: software, formal analysis, writing—original draft preparation, and visualization. JZ and DG: resources. XL and HG: supervision. XL: funding acquisition. All authors have read and agreed to the published version of the manuscript.
Funding
This research was funded by the Fundamental Research Funds of CAF (CAFYBB2019ZB005) and the China National Key R&D Program during the 13th Five-year Plan Period (2016YFD060020501).
Conflict of interest
The authors declare that the research was conducted in the absence of any commercial or financial relationships that could be construed as a potential conflict of interest.
The reviewer XZ declared a shared affiliation with the authors to the handling editor at the time of review.
Publisher’s note
All claims expressed in this article are solely those of the authors and do not necessarily represent those of their affiliated organizations, or those of the publisher, the editors and the reviewers. Any product that may be evaluated in this article, or claim that may be made by its manufacturer, is not guaranteed or endorsed by the publisher.
References
Ali, A., Dong, D., Akhtar, K., Teng, M., Yan, Z., Urbina-Cardona, N., et al. (2019). Response of understory vegetation, tree regeneration, and soil quality to manipulated stand density in a Pinus massoniana plantation. Glob. Ecol. Conserv. 20:e00775. doi: 10.1016/j.gecco.2019.e00775
Augusto, L., Davies, T., Delzon, S., and Schrijver, A. (2014). The enigma of the rise of angiosperms: Can we untie the knot? Ecol. Lett. 17, 1326–1338. doi: 10.1111/ele.12323
Bujalskı, L., Kaneda, S., Dvorsck, P., and Frouz, J. (2014). In situ soil respiration at reclaimed and unreclaimed post-mining sites: Responses to temperature and reclamation treatment. Ecol. Eng. 68, 53–59. doi: 10.1016/j.ecoleng.2014.03.048
Cheng, X. Q., Kang, F. F., Han, H. R., Liu, H. W., and Zhang, Y. L. (2015). Effect of thinning on partitioned soil respiration in a young Pinus tabulaeformis plantation during growing season. Agric. For. Meteorol. 214-215, 473–482. doi: 10.1016/j.agrformet.2015.09.016
Colman, B. P., and Schimel, J. P. (2013). Drivers of microbial respiration and net N mineralization at the continental scale. Soil Biol. Biochem. 60, 65–76. doi: 10.1016/j.soilbio.2013.01.003
Cotrufo, M. F., Ranalli, M. G., Haddix, M. L., Six, J., and Lugato, E. (2019). Soil carbon storage informed by particulate and mineral-associated organic matter. Nat. Geosci. 12, 989–994. doi: 10.1038/s41561-019-0484-6
Dang, P., Gao, Y., Liu, J. L., Yu, S. C., and Zhao, Z. (2018). Effects of thinning intensity on understory vegetation and soil microbial communities of a mature Chinese pine plantation in the Loess Plateau. Sci. Total Environ. 630, 171–180. doi: 10.1016/j.scitotenv.2018.02.197
Dawud, S. M., Raulund-Rasmussen, K., Domisch, T., Finér, L., Jaroszewicz, B., and Vesterdal, L. (2016). Is tree species diversity or species identity the more important driver of soil carbon stocks, C/N ratio, and pH? Ecosystems 19, 645–660. doi: 10.1007/s10021-016-9958-1
Dawud, S. M., Raulund-Rasmussen, K., Ratcliffe, S., Domisch, T., Finér, L., Joly, F. X., et al. (2017). Tree species functional group is a more important driver of soil properties than tree species diversity across major European forest types. Funct. Ecol. 31, 1153–1162. doi: 10.1111/1365-2435.12821
Finér, L., Domisch, T., Dawud, S. M., Raulund-Rasmussen, K., Vesterdal, L., Bouriaud, O., et al. (2017). Conifer proportion explains fine root biomass more than tree species diversity and site factors in major European forest types. For. Ecol. Manag. 406, 330–350. doi: 10.1016/j.foreco.2017.09.017
Guckland, A., Jacob, M., Flessa, H., Thomas, F. M., and Leuschner, C. (2009). Acidity, nutrient stocks, and organic-matter content in soils of a temperate deciduous forest with different abundance of European beech (Fagus sylvatica L.). J. Plant Nutr. Soil Sci. 172, 500–511. doi: 10.1002/jpln.200800072
Guenet, B., Juarez, S., Bardoux, G., Abbadie, L., and Chenu, C. (2012). Evidence that stable C is as vulnerable to priming effect as is more labile C in soil. Soil Biol. Biochem. 52, 43–48. doi: 10.1016/j.soilbio.2012.04.001
Hanan, E. J., Tague, C., Choate, J., Liu, M., Kolden, C., and Adam, J. (2018). Accounting for disturbance history in models :using remote sensing to constrain carbon and nitrogen pool spin-up. Ecol. Appl. 28, 1197–1214. doi: 10.1002/eap.1718
Heitkötter, J., Niebuhr, J., Heinze, S., and Marschner, B. (2017). Patterns of nitrogen and citric acid induced changes in C-turnover and enzyme activities are different in topsoil and subsoils of a sandy Cambisol. Geoderma 292, 111–117. doi: 10.1016/j.geoderma.2017.01.017
Hernández, J., Pino, A., Vance, E. D., Califra, Á, Del Giorgio, F., Martínez, L., et al. (2016). Eucalyptus and Pinus stand density effects on soil carbon sequestration. For. Ecol. Manag. 368, 28–38. doi: 10.1016/j.foreco.2016.03.007
Hulvey, K. B., Hobbs, R. J., Standish, R. J., Lindenmayer, D. B., Lach, L., and Perring, M. P. (2013). Benefits of tree mixes in carbon plantings. Nat. Climate Change 3, 869–874. doi: 10.1038/nclimate1862
Jucker, T., Bouriaud, O., Avacaritei, D., Coomes, D. A., and Knops, J. (2014). Stabilizing effects of diversity on aboveground wood production in forest ecosystems: Linking patterns and processes. Ecol. Lett. 17, 1560–1569. doi: 10.1111/ele.12382
Jurgensen, M., Tarpey, R., Picjens, J., Kolka, R., and Palik, B. (2012). Long-term effect of silvicultural thinnings on soil carbon and nitrogen pools. Soil Sci. Soc. Am. J. 76, 1418–1425. doi: 10.2136/sssaj2011.0257
Kong, J. Q., Du, Z. Y., Yang, R., and Su, Y. Z. (2019). Evolutionary characteristics of soil organic carbon storage in soil plough layer under a crop- land reclamation process in desert oasis. Chinese J. Appl. Ecol. 30, 180–188. doi: 10.13287/j.1001-9332.201901.037
Kuzyakov, Y., and Gavrichkova, O. (2010). REVIEW: Time lag between photosynthesis and carbon dioxide efflux from soil: A review of mechanisms and controls Y. Glob. Change Biol. 16, 3386–3406. doi: 10.1111/j.1365-2486.2010.02179.x
Kuzyakov, Y., and Larionova, A. A. (2005). Root and rhizomicrobial respiration: A review of approaches to estimate respiration by autotrophic and heterotrophic organisms in soil. J. Plant Nutr. Soil Sci. 168, 503–520. doi: 10.1002/jpln.200421703
Laganiere, J., Pare, D., Thiffault, E., and Bernier, P. Y. (2017). Range and uncertainties in estimating delays in greenhouse gas mitigation potential of forest bioenergy sourced from Canadian forests. GCB Bioenergy 9, 358–369. doi: 10.1111/gcbb.12327
Lavallee, J. M., Soong, J. L., and Cotrufo, M. F. (2020). Conceptualizing soil organic matter into particulate and mineral-associated forms to address global change in the 21st century. Glob. Change Biol. 26, 261–273. doi: 10.1111/gcb.14859
Lei, L., Xiao, W. F., Zeng, L. X., Zhu, J. H., Huang, Z. L., Cheng, R. M., et al. (2018). Thinning but not understory removal increased heterotrophic respiration and total soil respiration in Pinus massoniana stands. Sci. Total Environ. 621, 1360–1369. doi: 10.1016/j.scitotenv.2017.10.092
Li, M. M., Zhang, X. C., Pang, G. W., and Han, F. P. (2013). The estimation of soil organic carbon distribution and storage in a small catchment area of the Loess Plateau. Catena 101, 11–16. doi: 10.1016/j.catena.2012.09.012
Li, Y., Liu, X. J., Xu, W. B., Bongers, F., Bao, W. K., Chen, G. K., et al. (2020). Effects of diversity, climate and litter on soil organic carbon storage in subtropical forests. For. Ecol. Manag. 476:118479. doi: 10.1016/j.foreco.2020.118479
Li, Y. L., Yang, F. F., Ou, Y. X., Zhang, D. Q., Liu, J. X., Chu, G. W., et al. (2013). Changes in forest soil properties in different successional stages in lower tropical China. PLoS One 8:e81359. doi: 10.1371/journal.pone.0081359
Liu, M. Q., Hu, F., and Chen, X. Y. (2007). A review on mechanisms of soil organic carbon stabilization. Acta Ecol. Sinica 27, 2642–2650.
Liu, T. R., Tan, Z. J., Guo, J. P., and Zhang, Y. X. (2021). Effects of stand density on soil respiration and labile organic carbon in different aged Larix principis-rupprechtii plantations. Ecol. Process. 10:44. doi: 10.1186/s13717-021-00301-9
Mayer, M., Prescott, C. E., Abaker, W. E. A., Augusto, L., Cecillon, L., Ferreira, G. W. D., et al. (2020). Tamm review: Influence of forest management activities on soil organic carbon stocks: A knowledge synthesis. For. Ecol. Manag. 466:118127. doi: 10.1016/j.foreco.2020.118127
Ming, A. G., Yang, Y. J., Liu, S. R., Wang, H., Li, Y. F., Li, H., et al. (2018). Effects of near natural forest management on soil greenhouse gas flux in pinus massoniana (lamb.) and cunninghamia lanceolata (lamb.) hook. plantations. Forests 9:229. doi: 10.3390/f9050229
Na, M., Sun, X. Y., Zhang, Y. D., Sun, Z. H., and Rousk, J. (2021). Higher stand densities can promote soil carbon storage after conversion of temperate mixed natural forests to larch plantations. Eur. J. For. Res. 140, 373–386. doi: 10.1007/s10342-020-01346-9
Nordmeyer, A., Henley, D., Watt, M., and Davis, M. (2007). Ecosystem carbon accretion 10 years after afforestation of depleted subhumid grassland planted with three densities of Pinus nigra. Glob. Change Biol. 13, 1414–1422. doi: 10.1111/j.1365-2486.2007.01372.x
Oades, J. M. (1993). The role of biology in the formation, stabilization and degradation of soil structure. Geoderma 56, 377–400. doi: 10.1016/B978-0-444-81490-6.50033-9
Olajuyigbe, S., Tobin, B., Saunders, M., and Nieuwenhuis, M. (2012). Forest thinning and soil respiration in a Sitka spruce forest in Ireland. Agric. For. Meteorol. 157, 86–95. doi: 10.1016/j.agrformet.2012.01.016
Pan, Y. D., Birdsey, R. A., Fang, J. Y., Houghton, R., Kauppi, P. E., Kurz, W. A., et al. (2011). large and persistent carbon sink in the world’s forests. Science 333, 988–993. doi: 10.1126/science.1201609
Prieto, I., Armas, C., and Pugnaire, F. I. (2012). Water release through plant roots: New insights into its consequences at the plant and ecosystem level. N. Phytol. 193, 830–841. doi: 10.1111/j.1469-8137.2011.04039.x
Qiu, X. C., Peng, D. L., Wang, H. B., Wang, Z. Y., and Cheng, S. (2019). Minimum data set for evaluation of stand density effects on soil quality in Larix principis-rupprechtii plantations in North China. Ecol. Indic. 103, 236–247. doi: 10.1016/j.ecolind.2019.04.010
Ratcliffe, S., Wirth, C., Jucker, T., Plas, F., Scherer-Lorenzen, M., Verheyen, K., et al. (2017). Biodiversity and ecosystem functioning relations in European forests depend on environmental context. Ecol. Lett. 20, 1414–1426. doi: 10.1111/ele.12849
Schleuß, P. M., Heitkamp, F., Leuschner, C., Fender, A. C., and Jungkunst, H. F. (2014). Higher subsoil carbon storage in species-rich than species-poor temperate forests. Environ. Res. Lett. 9:014007. doi: 10.1088/1748-9326/9/1/014007
Shao, P., Zeng, X. B., Moore, D. J. P., and Zeng, X. D. (2013). Soil microbial respiration from observations and Earth system models. Environ. Res. Lett. 8:034034. doi: 10.1088/1748-9326/8/3/034034
Shi, F. J., Huang, Z. Y., Li, T., Wei, Q. M., and Yang, M. (2018). Vertical Distribution and correlation analysis of soil microbial biomass carbon and nitrogen in wangtian tree natural forest. For. Environ. Sci. 34:5. doi: 10.3969/j.issn.1006-4427.2018.06.012
Silva, P. M., Rammer, W., and Seidl, R. (2015). Tree species diversity mitigates disturbance impacts on the forest carbon cycle. Oecologia 177, 619–630. doi: 10.1007/s00442-014-3150-0
Son, Y. H., Lee, W. K., Lee, S. E., and Ryu, S. R. (1999). Effects of thinning on soil nitrogen mineralization in a Japanese larch plantation. Commun. Soil Sci. Plant Anal. 30, 2539–2550. doi: 10.1080/00103629909370393
Stevenson, B. A., Hunter, D. W., and Rhodes, P. L. (2014). Temporal and seasonal change in microbial community structure of an undisturbed, disturbed, and carbon-amended pasture soil. Soil Biol. Biochem. 75, 175–185. doi: 10.1016/j.soilbio.2014.04.010
Sun, X. D., Wang, W. N., Razaq, M., and Sun, H. L. (2019). Effects of stand density on soil organic carbon storage in the top and deep soil layers of Fraxinus mandshurica plantations. Aaust. J. For. Sci. 136, 27–44.
Sun, X. L., Zhao, J., You, Y. M., and Sun, J. X. (2016). Soil microbial responses to forest floor litter manipulation and nitrogen addition in a mixed-wood forest of northern China. Sci. Rep. 6:19536. doi: 10.1038/srep19536
Terrer, C., Phillips, R. P., Hungate, B. A., Rosende, J., Pett-Ridge, J., Craig, M. E., et al. (2021). trade-off between plant and soil carbon storage under elevated CO2. Nature 591, 599–603. doi: 10.1038/s41586-021-03306-8
Truax, B., Fortier, J., Gagnon, D., and Lambert, F. (2018). Planting density and site effects on stem dimensions, stand productivity, biomass partitioning, carbon stocks and soil nutrient supply in hybrid poplar plantations. Forests 9:293. doi: 10.3390/f9060293
Vesterdal, L., Clarke, N., Sigurdsson, B. D., and Gundersen, P. (2013). Do tree species influence soil carbon stocks in temperate and boreal forests? For. Ecol. Manag. 309, 4–18. doi: 10.1016/j.foreco.2013.01.017
Wang, H., Huang, Y., Wang, S. L., and Zou, D. S. (2010). Carbon and nitrogen storage under different forest ecosystems in mid-subtropical regions. Chinese J. Eco-Agric. 18, 576–580. doi: 10.3724/SP.J.1011.2010.00576
Witing, F., Gebel, M., Kurzer, H. J., Friese, H., and Franko, U. (2019). Large-scale integrated assessment of soil carbon and organic matter-related nitrogen fluxes in Saxony (Germany). J. Environ. Manag. 237, 272–280. doi: 10.1016/j.jenvman.2019.02.036
Wu, H. L., Xiang, W. H., Ouyang, S., Xiao, W. F., Li, S. G., Chen, L., et al. (2020). Tree growth rate and soil nutrient status determine the shift in nutrient-use strategy of Chinese fir plantations along a chronosequence. For. Ecol. Manag. 460:117896. doi: 10.1016/j.foreco.2020.117896
Xia, Q., Chen, L., Xiang, W. H., Ouyang, S., Wu, H. L., Lei, P. F., et al. (2021). Increase of soil nitrogen availability and recycling with stand age of Chinese fir plantations. For. Ecol. Manag. 480:118643. doi: 10.1016/j.foreco.2020.118643
Xu, C. H., Xu, X., Ju, C. H., Chen, H. Y. H., Wilsey, B. J., Luo, Y. Q., et al. (2021). Long-term, amplified responses of soil organic carbon to nitrogen addition worldwide. Glob. Change Biol. 27, 1170–1180. doi: 10.1111/gcb.15489
Xu, M., and Shang, H. (2016). Contribution of soil respiration to the global carbon equation. J. Plant Physiol. 203, 16–28. doi: 10.1016/j.jplph.2016.08.007
Yu, S. B., Wang, D., Dai, W., and Li, P. (2014). Soil carbon budget in different-aged Chinese fir plantations in south China(Article). J. For. Res. 25, 621–626. doi: 10.1007/s11676-014-0500-z
Zhang, K. X., Liu, X. Z., Lei, X. D., Jia, H. Y., Tang, J. X., and Guo, H. (2020). Short-term economic benefit evaluation of different management modes of Pinus massoniana plantation. J. Beijing For. Univ. 44, 43–54.
Keywords: Pinus massoniana, forest structure, stand density, stand age, pure forest, mixed forest, soil carbon storage, soil nitrogen storage
Citation: Zhang K, Gao D, Guo H, Zeng J and Liu X (2022) Forest structure characteristics on soil carbon and nitrogen storage of Pinus massoniana plantations in southern subtropic region. Front. For. Glob. Change 5:1022221. doi: 10.3389/ffgc.2022.1022221
Received: 18 August 2022; Accepted: 17 October 2022;
Published: 31 October 2022.
Edited by:
Huilin Gao, Shenyang Agricultural University, ChinaReviewed by:
Xiongqing Zhang, Chinese Academy of Forestry, ChinaYixiang Wang, Zhejiang Agriculture and Forestry University, China
Guangyu Zhu, Central South University Forestry and Technology, China
Copyright © 2022 Zhang, Gao, Guo, Zeng and Liu. This is an open-access article distributed under the terms of the Creative Commons Attribution License (CC BY). The use, distribution or reproduction in other forums is permitted, provided the original author(s) and the copyright owner(s) are credited and that the original publication in this journal is cited, in accordance with accepted academic practice. No use, distribution or reproduction is permitted which does not comply with these terms.
*Correspondence: Xianzhao Liu, lxz9179@163.com