- 1Forest Research, Roslin, United Kingdom
- 2Forestry Research Institute of Sweden (Skogforsk), Uppsala, Sweden
- 3ICIFOR-INIA, CSIC, Madrid, Spain
- 4INRAE, ONF, BioForA, Orleans, France
- 5Natural Resources Institute Finland (Luke), Savonlinna, Finland
- 6INRAE, Univ. Bordeaux, BIOGECO, Cestas, France
Deciding how to establish woodland in forest restoration is not straightforward as different outcomes may be obtained from different establishment approaches, each with cost implications and degree of success limitations attached. Planning restoration requires knowledge of site conditions, including how sites are likely to respond under climate change. For objectives of production and high timber quality it is likely that ground preparation will be used, and planting with forest reproductive material (FRM) of known traits, such as: high survival and growth in establishment, drought tolerance adequate for climate projections, good resistance to pests and pathogens. For objectives associated with biodiversity, carbon sequestration, water supply protection, soil protection, natural regeneration could be a less costly solution with a limited amount of assisted translocation of selected FRM to improve resilience. If objectives are for rewilding forest areas, a degree of natural colonisation perhaps with translocation of some FRM could be a solution. Ignoring site conditions and suitability of available sources of FRM for forest restoration is likely to provide unexpected results with a mix of open ground, scrub and scattered trees resulting from climate, herbivore, and browsing impacts. The recent B4EST EU Horizon 2020 project examined progress in novel rapid approaches for testing the quality of FRM from existing genetic trials. Here we review the work of B4EST to show the opportunities from transformative tree breeding in forest restoration schemes, including: new climate projection ensembles at high temporal and spatial resolution to develop norms of reaction and transfer models with genetic components; multi-environment genotype-phenotype associations and multi-locus genotype-environment associations in identifying drivers of local adaptation; techniques for genomic selection using single nucleotide polymorphism (SNP) arrays to derive functional traits from polygenic associations; work on seed orchard site and climate specific FRM and zones for deployment; and work on some of the forest ecosystem service benefits derived at a landscape scale. We conclude that tree-breeding will provide robust forest restoration for planting, and rewilding (assisted natural regeneration), and if not “ignoring” but instead assisting natural colonisation processes – tree breeding may improve long-term forest resilience under environmental change.
Introduction
Many studies of the impacts of climate change on forests have recommended selecting tree species suited to site conditions (Broadmeadow et al., 2005; Meason and Mason, 2014; Hof et al., 2021; Reynolds et al., 2021) to restore, adapt and manage forests to maintain forest resilience. Tree-breeders also work with and select forest material within species, for example a particular provenance (Benito-Garzón et al., 2018; MCPFE, 2020) that shows evidence of improved resilience under climate change. Other studies provide evidence of species diversity through the restoration of mixed forests as a pre-requisite for future forest resilience (Jactel et al., 2017) and ecosystem service provision (Gamfeldt et al., 2013). Furthermore, there is evidence that natural regeneration provides a cheaper and more reliable mechanism for forest restoration, biodiversity, and the provision of ecosystem services (Rey Benayas et al., 2009; Crouzeilles et al., 2017), particularly in the tropics and on land that was previously forested. All these concepts are primarily concerned with different restoration mechanisms to promote the long-term continuity of forest ecosystem function in restoring forests under climate change (Hof et al., 2021).
The idea that species diversity only is important for forest resilience, ignores or underestimates the role of intra-specific genetic diversity (Alberto et al., 2013; Ennos et al., 2019) phenotypic plasticity (Vizcaíno-Palomar et al., 2020) and the evolutionary potential of trees (Lefèvre et al., 2013) which help maintain fitness under environmental change. These genetic factors play a major role in maintaining tree, stand health and vigour under climatic stress. For some species, provenance, progeny, and clonal forest reproductive material (FRM) have been tested and phenotyped for different traits (Vizcaíno-Palomar et al., 2020), including the resistance to abiotic and biotic threats (Hurel et al., 2021) in common garden genetic trials across an environmental range. For some species tested material has been incorporated into seed orchards, to provide FRM with known traits, including the resistance and avoidance of biotic and abiotic threats (Haapanen and Ruotsalainen, 2021). Currently, many managers of plantation forests look only to translocate species to sites based on the match between climate analogues and future projections (Reynolds et al., 2021). Tree breeders, on the other hand, link the evolutionary potential of tree species using knowledge of genetic diversity and adapted phenotypes with acclimation potential, based on provenance (and other genetic) trials within species (Benito-Garzón et al., 2019).
The question of whether to “plant, rewild or ignore”, assuming “plant” for production forests, “rewild” using planting and natural regeneration and open land for nature, and “ignore” (as stated in the topic of the special issue) is a reference to natural colonisation, is likely to be answered differently by societal groups with different frames on forestry objectives (Burton et al., 2019). Outcomes will vary depending upon whether restoration is within wooded [natural regeneration, assisted natural regeneration (ANR)] or unwooded (natural colonisation and assisted colonisation) landscapes. Colonisation methods are gaining prominence in policy and practice in some countries and may be preferred with respect to requiring little or no intervention. For forest and woodland restoration, natural regeneration is often argued as an efficient cost-effective approach (Crouzeilles et al., 2020), as it favours phenotypes and genotypes suited to the current environment (Chazdon and Guariguata, 2016) with less risk to forest biodiversity (Ennos et al., 2019). This is the case in Sweden for example, Scots pine on nutritionally poor sites of low site index in areas of heavy moose browsing are often left to regenerate naturally. However, a natural colonisation approach may be uncertain, particularly when the need for intervention is ignored, as deer browsing damage (Duncker et al., 2012) and a long time-lag (Watts et al., 2020) for species colonisation may compromise expected outcomes of medium- to long-term ecological trajectories and ecosystem service provision. This can be exacerbated by the longer time to canopy closure, described by Elliott et al. (2003) as an “important milestone in forest restoration, creating cooler, shadier and moister conditions on the forest floor and the accumulation of leaf litter that should suppress weeds and encourage establishment of forest tree seedlings.”
A natural colonisation approach aims to restore forest (and other habitats) on open ground where initially few trees exist or in landscapes where forest has been cleared for a long time (over 50 years – Burton et al., 2018). In such circumstances the potential regenerative capacity back to a wooded landscape is uncertain. The method used in tropical forests which has been widely promoted (Anon, 2005; Lamb and Gilmour, 2005; Elliott et al., 2013), differs from a land abandonment (natural colonisation) approach. For example, Forest Landscape Restoration (FLR; Elliott et al., 2013) is an ANR approach which introduces framework tree species that are fast growing and produce fruit and seed quickly, the framework attracts birds and animals that encourage seed dispersal into the restoration site. This approach is quite different to an “ignore” approach to natural colonisation. The difference is that FLR or any ANR approach is more likely to quickly develop a forest ecosystem, whereas natural colonisation on agricultural areas previously devoid of trees for a long period of time may develop woodland more slowly, with accompanying scrub and semi open habitat (Silva et al., 2018).
Planting is favoured by foresters and the processing industry. This is because the planting and tending of closely spaced forest trees and their periodic thinning, usually reduces the time to canopy closure (Waterworth et al., 2012), promotes the growth of improved quality timber (MacDonald and Hubert, 2002; Mason, 2015) compared to natural regeneration, and allows forest managers to utilise the gains achieved by tree breeding programs. This approach, although expensive compared to natural regeneration, provides improved timber quality and value to the forest manager (MacDonald et al., 2010), with faster growth and quicker provision of timber and products to society. It is often the economic benefits from planted production forests that provide the opportunity for forest owners to restore areas (by rewilding and natural colonisation) for a range of ecosystem services (Guadaño et al., 2016; Ahtikoski et al., 2020a,b) by managing less intensively for biodiversity, recreation, and other benefits.
The European Horizon 2020 project “Adaptive Breeding for productive, sustainable and resilient forests under climate change” (B4EST; grant agreement No. 773383) recognised that environmental change is a major driver of forest ecosystem vulnerability, increasing the likelihood of damage and disease, leading to reduced productivity and economic value. In any forest restoration project, we assume a scheme would visualise a successful outcome that is robust in establishment and resilient to environmental change. We believe forest expansion in Europe will require multiple approaches to forest restoration, including the deployment of improved FRM to sustain resilient and high-quality material for the European bioeconomy. The increased economic value of improved FRM will stimulate forest expansion and restoration for a range of ecosystem services, including halting biodiversity decline and increasing carbon sequestration. Profits from production forestry will help fund less intensive forestry practice. For example, Burton et al. (2019) referring to Scotland’s Land Use Strategy (2021–2026) (Scottish Government, 2021) reasoned that forest restoration opportunities should be blended with other land use priorities. We believe this reasoning is also applicable to stands within forests to promote a range of forest objectives. Furthermore, we suggest a portion of forest restoration and expansion should include FRM from novel and flexible adaptive tree breeding strategies and tools that promote the use of tree genotypes of high adaptive potential and high economic value. We hypothesise that tree breeders will need to rapidly identify and select important phenotypes showing heritable traits useful under abiotic and biotic stress, while maintaining genetic diversity and key ecological functions. Furthermore, we make the link to stand and regional scale planning tools for forest restoration, that account for site variability under climate change. Here we review the work of the B4EST project research outputs to show how new tree breeding techniques can improve the resilience of forest restoration to climate change and provide goods and services for society and the developing bioeconomy.
B4EST overview
The B4EST project was divided into five science areas and a communications and dissemination area. Each component undertook collaborative research on one or more of eight case study tree species to illustrate the range of novel tree breeding approaches developed by the European partners in the B4EST consortium. The case study species were: Norway spruce (Picea abies); Scots pine (Pinus sylvestris); maritime pine (Pinus pinaster); stone pine (Pinus pinea); Douglas-fir (Pseudotsuga menziesii); Eucalyptus globulus (clones); poplar (clones); and common ash (Fraxinus excelsior). The schematic of the project structure (Figure 1) shows the interlinkages among the five science areas (work packages) and communication (WP6). One extra work package (WP7, in Figure 1) concerned consortium coordination. Much of the science knowledge was developed in Areas 1 and 2, the technology and methods of applying the science were developed in Areas 3 and 4. In Area 5, this science and its application were considered at the regional and landscape scale to provide a meaningful focus for tree breeders, nurseries, forest managers the forest industry and forest policy, and disseminated in Area 6.
Description of science areas 1–5
Area 1 (WP1) focussed on the re-analysis of different phenotypic traits recorded in various genetic trials in the light of climate data from a new portal (ClimateDT1) to develop norms of reaction (West-Eberhard, 2003) of tested phenotypes to climatic variation, and to measure the genetic components of phenotypic variation and plasticity and the role each may have in maintaining robust forests under climate change. In this area we review the methods to measure the tolerance and acclimation potential to a range of disturbances – both climatic and biotic. Area 2 (WP2) measured the trade-offs among phenotypic traits (e.g., increased growth – poor form – early flushing, resistance to disease – reduced growth) to understand the risks of selecting alternative phenotypes for one objective among others and testing the selection of improved material under uncertainty and specific challenges. Here the partners undertook studies to evaluate both multi-environment genotype-phenotype associations and multi-locus genotype-environment associations in identifying drivers of local adaptation and their genomic signatures. This key feature links to Area 3 (WP3) and provides an important novel step in reducing the time for selection and breeding and selecting better suited material more accurately for given environments and biotic threats. In Area 3 new selection approaches were developed, based on the use of genomic evaluation, and involving assessment using genetic tools for species challenged by environmental change. Here the consideration of new functional traits and gene-to-gene interactions which could be relevant for species undergoing clonal selection and deployment were assessed. This area illustrates a “tour de force” of the different improvements that genomic tools bring to forest tree breeding relevant to forest restoration objectives. Area 4 (WP4) developed stand level models for deployment of material to deliver specific objectives and ecosystem services to society, using improved matching of phenotypes under climate uncertainty and bioeconomic demands. Area 5 (WP5) turned to understanding the benefits, costs, and risks under climate change at the landscape and regional scale. Here the focus of research was the selection among different phenotypes, both temporally and spatially, to improve forest resilience and demonstrate how careful selection of inter- and intra-species diversity in FRM to future climate and soils will help maintain forest diversity and provide valuable timber material and products from European forests.
Climate change and climate data
In considering whether to “replant, rewild, or ignore” to restore forest, a critical appraisal of the impacts of present and projected climate change scenarios should be considered to choose a restoration approach with evidence of robust establishment success, and which is likely to be resilient under climate threats. The UKCP18 Regional (12 km resolution) and Global (60 km resolution) projections (Murphy et al., 2019) offer a monthly time series into both RCP2.6 and RCP 8.5 futures, for low and high impacts scenarios. Each RCP scenario consists of an ensemble to provide information regarding the likelihood of impact of climate extremes on phenotypic traits. A re-analysis of historic experiments and trials used historic data from the Climate Research Unit time series (CRU-TS; Harris et al., 2020). This was downscaled using the method of ClimateEU (Marchi et al., 2020) to produce scale-free climate data linking CRU and UKCP18 for overlapping years using a standardisation data set between 1981 and 2010 from CHELSA (Karger et al., 2017) and producing climate data to the same 12 km resolution as UKCP18. The resulting online ClimateDT climate data portal can downscale data for any location from the 189-year historic and projected period 1901–2089, providing a large range of climate variables and indices at monthly, seasonal, annual, and normal period time scales.
Extreme events
Recent literature has described the importance of extreme events (Hanlon et al., 2021), such as climatic extremes and biotic disturbances (Seidl et al., 2017), in shaping the distribution and components of forests among tree species (Zimmermann et al., 2009). In particular, Barriopedro et al. (2020) showed the importance of extreme event analysis. They compared European summer maximum temperatures to a summer maximum data set for 1981–2010 and demonstrated that five of the summers between 2000 and 2020 had a mean summer temperature anomaly of more than 1.2°C above the warm end of the 1981–2010 distribution. However, less is known about the effects of extreme climate disturbance selection pressure within tree species (Gutschick and BassiriRad, 2003). We used climate projections based on shorter temporal time steps than 30-year averages. The UKCP18 Regional projections (Murphy et al., 2019) provided a monthly time series to better understand the effects of climate extremes on phenotypic traits. Of particular interest to the forestry sector is the impact of extreme summer heat and drought on abiotic damage to stands (Williams et al., 2013; Petr et al., 2014; Allen et al., 2015), and the subsequent biotic damage that is caused by climatic stress (Jactel et al., 2012; Meason and Mason, 2014; Hlásny et al., 2021).
Using the 12 km resolution climate data we examined historic and projected droughts across Europe. The standardised precipitation and evaporation index (SPEI) drought index (Beguería et al., 2014) was calculated for September each year for 3-, 6-, and 12-month antecedent periods. Resulting maps showed relationships with reported heat and drought impacts in the literature. For example, the extreme 2018 heat and drought events across Europe stimulated an increase of damaging populations of the European spruce bark beetle (Ips typographus) in Central, Western and Nordic countries of Europe. We show examples of the extreme droughts in Atlantic and continental regions of Europe in 2003 (Figure 2A) and additionally in Nordic countries in 2018 (Figure 2B), both of which were associated with extreme temperature anomalies.
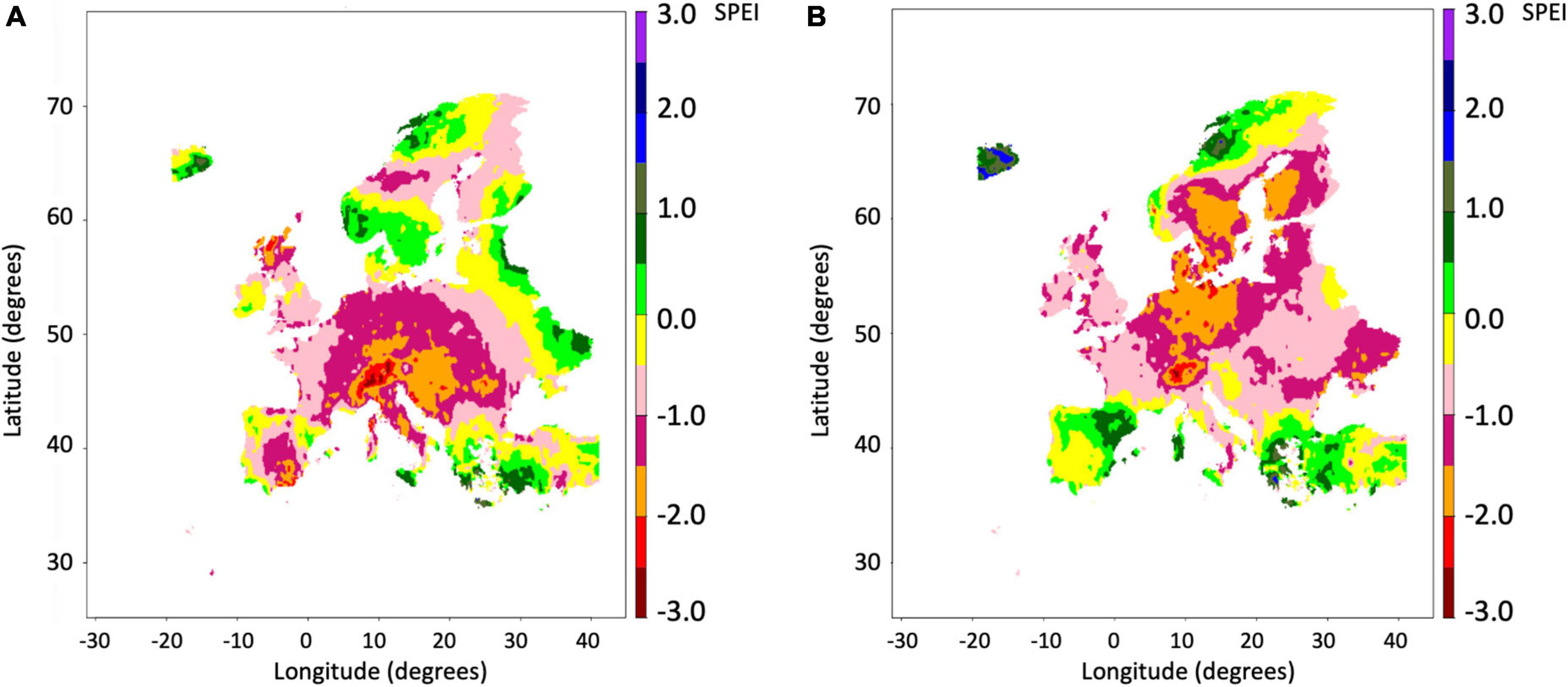
Figure 2. The Standardised Precipitation and Evapotranspiration Index (SPEI) drought index across Europe based on the 6-month period April–September in (A) 2003, and (B) 2018; using CRU-TS climate data (Harris et al., 2020) downscaled to 12 km resolution. SPEI +3, extremely wet/SPEI –3, extremely dry.
Late spring frost is also a serious problem in forestry and under climate change is likely to become more serious. A study of early flushing and late spring frost damage was investigated in 18 Norway spruce provenances from Sweden, the Baltic States, Poland, and Belarus (Svystun et al., 2021). The temperature sum requirement to drive flushing in each provenance was used to model changes in spring flushing under climate change. In Sweden, the results showed a reduction in frost events under future climate scenarios, but an increase in frost events after spring flushing. Results highlight the need for improvements in selection of provenances for deployment in Sweden that avoid late spring frost damage.
Extreme climatic events were found to be the drivers of within population genetic variation (Archambeau et al., 2021) in maritime pine revealing a mechanism of how heritable variation in complex phenotypic traits is maintained in populations. They showed that for height growth, genetic variation is lower in populations exposed to severe cold extreme events. This finding was also reported by Skrøppa and Steffenrem (2021) in a study involving Nordic, Central, and Eastern European provenances of Norway spruce. Archambeau et al. (2021) additionally showed that genetic variation from populations of genetically mixed provenances was not influenced by gene flow that might be thought to increase genetic variation. This B4EST study lends support to the debate on levels of genetic variation, providing evidence for the role natural selection plays in reducing genetic variation.
Uncertainty
Climate uncertainty is a critical concern for foresters, forestry and particularly within the topic of forest restoration. Whether restoration involves natural regeneration or planting, with or without management intervention, if the restoration objective is to achieve robust establishment to develop forest habitat – then a clear understanding of the impacts of climate change are essential. This includes the need for a better understanding of the severity and frequency of extreme events that until recently were not available from climate projection portals.
As an example, B4EST studied the growth potential of Scots pine provenances at the northern and southern edges of the species range (Hallingbäck et al., 2021). In Nordic countries, at the northern edge, photoperiod (latitude) and temperature sum (TS – day degrees >5°C) were critical drivers of growth; at the southern edge the summer heat moisture index (SHM – C/m) was the driver of growth. Photoperiod is a fixed parameter unaffected by climate change, but TS and SHM will continue to be influenced by the changing climate. Consequently, these parameters were used to test the adaptation potential of several provenances of Scots pine under an ensemble of climate projections. Models of the Nordic country provenances predicted a growth increase as very likely in each, but with considerable uncertainty in the magnitude involved. For the Spanish provenances, growth under climate change varied spatially from very unlikely to very likely, but there was greater certainty in the predictions. These results demonstrate the need to be aware of the adaptation potential of material considered for forest restoration, particularly when key traits associated with restoration objectives – like growth and fitness – are considered essential.
Reaction norms and transfer models
A reaction norm describes the variation in a phenotypic trait to changing environmental conditions. It is a way in which tree breeders can observe and characterise the phenotypic plasticity in a genotype by measuring the Genotype × Environment interaction. These types of studies therefore need to relate climatic and site parameters to the trait variation across different genotypes. Usually this is performed across a large environmental gradient in genetic trials (common garden trials), in which all genotypes are tested in all environments under investigation.
Climate data were used to re-analyse past and existing genetic common garden trials in which provenances, progeny and clonal material had been tested. Climate change projections over north-west Europe suggest warmer and drier summers and wetter and milder winters. This trend is accentuated in northern latitudes in the summer and particularly in winter (Jacob et al., 2018) leading to greater risk of frost damage and drought damage in Nordic countries. A study by Hayatgheibi et al. (2021) conducted in Sweden and Finland throughout 2018 during a summer period of severe heat and drought across northern and central Europe, showed significant phenotypic variation to drought in full- and half-sib progenies of 6–9-year-old Norway spruce. The strong heritability of drought resistant phenotypes showed the importance of this trait for the future breeding material of Norway spruce.
Climatic and genomic data to model tree adaptive trait variation
The B4EST project developed a set of six single nucleotide polymorphism (SNP) 50k arrays for Scots pine (PySy50k; Kastally et al., 2021), Norway spruce (Bernhardsson et al., 2021), and the new “4TREES” SNP array (Guilbaud et al., 2020) which can be used to explore the genome of four species (common ash, stone pine, poplar, and maritime pine). This development was designed to improve the speed and economy of selection to identify FRM with useful phenotypic traits through genomic selection (GS), thus reducing the cost of selection from large scale trials. In general, conifers have massive genomes that are relatively little studied. The SNP arrays have been designed to facilitate GS models and genome-wide association studies (GWAS) – linking trait variation to genetic polymorphisms that form the basis of gene expression across the genome.
Each SNP array is based on regions of the genome where a single nucleotide (one of the building blocks of DNA) varies between individuals of the same species. Except for stone pine, a species with extremely low levels of genetic variation, all the SNPs come from existing genomic resources. Having obtained hundreds of thousands of SNPs per species, the initial step for “4TREES” was to produce a screening array, on which approximately 137K SNPs per species (36K SNPs for stone pine) were tested on a representative group of individuals of each species. Following screening, 13.5K (6K SNPs for stone pine) highly informative SNPs were selected. The most important criterion for selection was to incorporate SNPs known to be in candidate genes or regions linked to traits of interest for breeders, such as resistance to ash dieback disease (Hymenoscyphus fraxineus) that ravages ash populations. A good coverage of the genome was also important, as it has been shown to improve predictions (Romero Navarro et al., 2017; Kainer et al., 2018; Norman et al., 2018). The 4TREE array is a highly valuable genome-assisted breeding tool for cost effective genotyping for important tree species in Europe, and the tool demonstrates possibilities for other important tree species.
Genomic selection models predict the breeding value of genotypes in a validation dataset using training data from phenotyping, and these data sets are linked in a genomic prediction equation. Breeding values are calculated from the equation and have shown promising results from a few thousand SNPs (Pegard et al., 2020). It is much more cost effective to undertake GS in tree breeding, than earlier methods of testing FRM in genetic common garden trials using pedigree information. The approach offers the potential to increase genetic gain very quickly using genetic estimated breeding values (GEBV) without the need to plan and finance a long breeding cycle to test potential FRM candidates with useful traits.
The second advantage of genomic studies using an SNP array arises from the opportunity to detect loci that control trait variation in genotypes, such as GWAS studies – an approach to improve our understanding of the genetic architecture controlling the biology and phenology of genotypes. Note, however, that many traits are thought to be controlled by numerous polymorphisms with small effects at single nucleotides on the genome, making the trait polygenic – from many nucleotides (de Miguel et al., 2022). Other uses of SNP arrays include monitoring genetic diversity, tracing the origin of material, and estimating population genetic structure.
A third approach to explore the genetic basis of adaptive traits correlates loci with allele frequency changes in geographical space with changes in environmental variables (Rellstab et al., 2015; López-Hernández and Cortés, 2019) known as genome-environment associations (GEA). The method identifies the loci linked to adaptive constraints and loci linked to ecological variables responsible for selective constraints. Evidence of selection acting on SNPs involved with the response to bioclimatic variables has been shown in different plant species, e.g., Populus spp. (Ingvarsson and Bernhardsson, 2020), Norway spruce (Di Pierro et al., 2017), maritime pine (Jaramillo-Correa et al., 2015), Aleppo pine (Ruiz Daniels et al., 2018), beech (Postolache et al., 2021). Similar methods have been used in the B4EST project to identify candidate SNPs for local adaptation in maritime pine using both univariate and multivariate GEA methods (Archambeau et al., 2022) that can predict adaptation of tree species to future climate change.
Clonal traits
An important objective of B4EST was identifying FRM resilient to changes in climate and at the same time resistant to pests and pathogens. For example, Marchi et al. (2022) examined standard and improved poplar clones (for short rotation agroforestry) under climate change projections across Italy using reaction norms and linear mixed-effects models of growth. This study showed that improved clones were more resilient to a warmer drier climate and more productive than standard clones and have been shown to resist rusts and leaf spot fungus. Poplars are vigorous fast-growing trees and produce valuable timber for plywood and furniture with a long carbon life cycle, filling an important role in the timber production industry.
Reaction Norm, Transfer function, Response function, and the “Universal” version of these models, where the response of different groups of FRM are pooled in a single model, are all components of the modelling work in B4EST. Climate change uncertainty is also a feature of the modelling work. Marchi et al. (2022) used climate uncertainty within the representative concentration pathways (RCP) of greenhouse gas emissions using RCP2.6 and RCP8.5 as low and high emissions scenarios, respectively; and in addition, used contrasting variants of the UKCP18 climate projections ensemble.
The link between genomics and phenotypic traits has also been tested in B4EST. Heuclin et al. (2021) used a novel Bayesian varying coefficient model to identify the genetic markers involved in the variability of phenotypic traits and estimate their effects. This is a key publication for the future development of GWAS in tree breeding.
Genetic architecture controlling phenotypic traits
A novel B4EST study on maritime pine explored the genetic basis for heritable traits in a common garden trial using clones from a sample of trees in 36 populations from the complete natural distribution of the species (Hurel et al., 2021). Assessments of height, spring flushing, susceptibility to pathogens Diplodia sapinea and Armillaria ostoyae and the insect pest pine processionary moth (Thaumetopoea pityocampa) were made across the trial. Results showed significant broad sense heritability for height and spring phenology, and pathogen susceptibility to Diplodia, but no differences in the susceptibility of T. pityocampai incidence was observed. Populations from warmer drier environments were less susceptible to Diplodia but more susceptible to Armillaria, and taller trees were more affected by D. sapinea and shorter trees showed more damage by A. ostoyae. A genomic analysis associated several loci with these phenotypic traits, revealing five SNPs associated with height growth and 27 SNPs associated with spring flushing date and duration. An important result is that many adaptive and growth traits are polygenic (de Miguel et al., 2022), that is important for breeding in GS and for the adaptability of trees with large and complex genomes, such as conifers.
The study of the genetic control of phenotypic traits was an important B4EST topic to assess provenances suitable for future environmental conditions. This provides a genetic improvement to species distribution models (Thuiller, 2003) that ignore intraspecific genetic effects in predicting suitability. A comparison of twelve statistical models were used to predict adaptive trait variation across environmental gradients in the maritime pine clonal common garden trial (Archambeau et al., 2022). In particular, the separation of the effects of genetic variation and phenotypic plasticity among clonal populations was key in improving model fit. Plasticity accounted for around twice the explained variance in height growth, compared to the genetic component, and was driven by environmental factors.
Phenotypic plasticity, genetic diversity, and trade-offs
In B4EST, a study was completed on three species of pine to better understand how phenotypic plasticity and local adaptation underpin variation in the response of key traits. The height growth of maritime pine, Corsican pine (Pinus nigra ssp. laricio) and stone pine across a network of 38 common garden trials in the Mediterranean basin were analysed (Vizcaíno-Palomar et al., 2020). A phenotypic plasticity index, based on the ratio of the difference of the highest phenotypic value (of height) measured for a local population and compared to the value for the climatic range of the species was measured. Also, the coefficient of variation measuring phenotypic variation across the range of the trials was calculated. These indices were calculated separately for very young trees (up to 4 years), young trees (4–8 years), and moderately young trees (8–16 years). Results showed variation in plasticity among the species and within species among age classes, with stone pine exhibiting the highest phenotypic plasticity and least genetic diversity, followed by maritime pine and black pine with a lower plasticity index but higher genetic diversity. For all species, very young pine trees had a greater ability to respond to changes in climate than older trees. Conclusions showed different mechanisms of an adaptive response with stone pine relying primarily on phenotypic plasticity and showing little genetic variation within or among populations. Maritime pine showed high phenotypic plasticity and high genetic diversity within and between populations, with potential for a shorter-term plastic response to rapid climate change and a longer-term adaptive response through natural selection.
The measurement of phenotypic plasticity of FRM is an important factor used in the estimation of whether material is suitable across an environmental range. Often the norm of reaction response of different genotypes across environmental gradients are not parallel, demonstrating the presence of an interaction between genotype and the environment. This can be a problem to tree breeders wanting to breed material that exhibits a useful stable trait (such as growth performance) across a wide range of environmental conditions. Such assessments are useful in examining potential trade-offs among different traits to estimate whether FRM selection can be justified. Results using different common garden experiments (Ramírez-Valiente et al., 2021) indicates that high levels of population genetic differentiation in functional traits and differences in fitness are common in Mediterranean species driven, at least in part, by the adaptation to contrasting temperature and precipitation regimes. This study failed to detect a trade-off between source-use strategies within species, calling for future studies to investigate further whether intraspecific evolution of stress tolerance occurs at the expense of growth potential in Mediterranean environments or not.
Seed sourcing
Different seed sourcing methods (local, predictive, climate-predictive, climate-adjusted, composite, and admixture) can be applicable under different climate change scenarios. In Spain, these methods have been tested using a Scots pine multi-site provenance test (Notivol et al., 2020). Despite the theoretical expectations, for Scots pine, a forest tree species at the southern edge of its distribution, seed-sourcing methods based on climate-matching or a combination of seed sources do not perform better than traditional local or predictive methods or they are not feasible because of the lack of future climate-matched populations.
In breeding programs, other seed sourcing methods can be applied. Seed orchards provide nurseries with the plant material for forest restoration, assuming the approach is to plant, rather than rewild or ignore. The seed orchard function is to produce large amounts of material that has been tested and trialled to confer suitability for the planting site and the potential for particular traits.
In Nordic countries, seed orchard material is widely available for Norway spruce and Scots pine. In B4EST the results from common garden trials of a range of provenances of Scots pine were analysed to provide a method to inform seed orchard choice under uncertainty. An approach developed by Haasnoot et al. (2013) and adapted for planning in forestry by Petr et al. (2015) was used to demonstrate the time frame in which a species choice meets forestry objectives, and when the benefits of those objectives begin to disappear due to climate change. At which point adaptation management is required to reset the state of a forest stand on a different trajectory, chosen from a range of possible actions.
Currently productive seed orchards in Sweden (including the one and a half generation orchards) have been established according to the “current climate” situation which means that climate change has not explicitly been accounted for. The method examined the potential performance of seed orchards using an ensemble of six 30 arc-second GCM climate projections from WorldClim (Hijmans et al., 2005) for the periods 2041–2060 (2050s) and 2061–2080 (2070s) 30-year normal periods for RCP4.5 and 8.5 scenarios. Growth models using projections for the 2050s describe how plants from current seed orchards planted today will perform over shorter-term planning horizons. Growth models using projections for the 2070s indicate the situation facing crops from the next generation (second generation) seed orchards, whose establishment currently are in the development phase. The analysis demonstrated trade-offs between growth and survival in second generation orchards, as models predicted improved survival under warmer conditions at the expense of improved growth. Planning based on the current climatic conditions could lead to orchards becoming maladapted to their intended core area but better in other areas.
Deployment and ecosystem services from forest reproductive material
The development and outcomes of forest stands depends not only on the selection of FRM but also on silviculture and the interaction between them. A demo-genetic modelling approach, where forest growth and demography are coupled with individual genetic diversity and inheritance processes can be used to predict how silvicultural interventions affect genetic diversity and trait development implemented in the LUBERON2 simulation tool (Oddou-Muratorio et al., 2020). They used an open software development platform CAPSIS to improve and update the LUBERON2 model with new features, parameterised for Douglas-fir. Specifically, they introduced: (i) tree growth models; (ii) a demographic model; (iii) quantitative genetic models for multiple traits; (iv) a disturbance generator (including effects of drought); and (v) tools to introduce silvicultural measures. These tools and models simulate four Douglas-fir silvicultural scenarios and analyse the impacts on the evolution of genetic means and variances of two fitness-related traits. Their results (Oddou-Muratorio et al., 2020) demonstrate the opportunities to use adaptive forest management to manage genetic diversity and increase fitness in a future warmer climate.
The potential gains from using improved FRM for future climatic conditions are only realised when the FRM is deployed and used by foresters. B4EST work analysed the potential of new FRM to increase financial returns (Serrano-León et al., 2021). Although their research suggests a change of seed sources, new seed sources, increased use, and even introduction of exotic species, forest practitioners must adhere to current country-based legislation as well maintain an awareness of the perceptions of a wide variety of stakeholders. B4EST summarised stakeholder views by combining results from a GenTree survey on “adaptive tree breeding for productive, sustainable and resilient forests under climate change” with a complementary B4EST survey and published reports (Konnert et al., 2015). The work showed a wide range of national/international regulations affecting the use of FRM in Europe but that national/regional policies often promote the use of native/local FRM and discourage the use of non-local and exotic FRM such as assisted migration. B4EST therefore encourages common regulations which instead promote the use of improved FRM across administrative borders.
With changing climatic conditions, increased risks to tree stands, and greater demand for desirable FRM traits, decision making in the reforestation phase will become increasingly complex. Forest owners and managers will need tools and guidelines to handle these issues through decision support tools. The Planter’s guide (Berlin, 2019) produced by B4EST consists of different web-based support tools for the selection of optimal seed sources of Scots pine and Norway spruce in Sweden. In 2019 the Planter’s guide was extended to be a joint tool between Sweden and Finland with common deployment recommendations (Berlin et al., 2019). B4EST work produced new transfer effect models for growth and survival valid for the entire Fennoscandia (i.e., Sweden, Finland, and Norway), implemented in a beta-version of the Planter’s guide. This approach opens the possibility for joint deployment recommendations in this region, but national stakeholders and formal authorities must play an integral part in co-developing these decision support tools.
The Planter’s guide also illustrates the uncertainties arising from climate change affecting the predicted performance of currently available seedlings from Scots pine seed orchards. Drawing from the modelling approach developed in Hallingbäck et al. (2021), methods to illustrate the variability in spatial distribution of seed orchard performance at different climate warming levels were developed for the Planter’s guide. This allowed a set of different “uncertainty” maps to be generated for all currently available and planned future Scots pine seed orchards in Sweden. A core group of end-users of the Planter’s guide (Forest managers at both large forest companies and forest owner associations) and stakeholders (the Swedish Forestry board) provided feedback on the different methods to handle/illustrate this uncertainty with the aim of implementing this knowledge in a user-friendly way.
Landscape scale restoration
Four landscape scale case studies to estimate the potential use of FRM in forest restoration in different regions of Europe were commissioned in B4EST. Case studies examined: (1) boreal forestry in Nordic countries representing approximately 30% of the European forest area; (2) improved Scots pine material in Scotland and the potential to use native Caledonian pine forest material to increase growth and reduce red band needle blight susceptibility; (3) productive pine and Eucalyptus plantations in France, Portugal, and Spain and the potential for breeding pest and disease resistant material; and (4) the benefits, costs, and risks related to the use of improved poplar clonal FRM in alluvial regions of central and southern Europe.
Nordic case study
Nearly 20% of the growing stock of European forests occurs in Finland, Sweden, and Norway (MCPFE, 2020) and Sweden and Finland produce 30% of sawn timber and 60% of pulp for paper and paperboard in the EU. Climate change has already affected the growth dynamics of forests in Nordic countries. Over a 50-year period (1970–2020) the growing stock in Finland has increased from 1,520 million m3 to 2,506 million m3 driven largely by the temperature sum (day degrees over 5°C) increase.
Norway spruce and Scots pine are the predominant conifer species and birch the main broadleaved species. Milder winters with fewer frost days in the spring have brought about an earlier start to the growing season, but occasional late spring frosts now cause more damage. The summer climate has become warmer and drier, and in recent years has caused major outbreaks of bark beetles (Ips typographus) and the fungal root disease Heterobasidion spp. has become more widespread. These two biotic threats are considered by stakeholders to be a focus of the breeding programmes of Norway spruce and Scots pine. To adapt to climate change, stakeholders consider three important forest management strategies: (1) diversification of tree species, which is a common strategy to distribute risk, (2) artificial regeneration with improved FRM tested and recommended for adaptation to climate change, and (3) enrichment of natural regeneration with FRM better adapted to future climate changes.
The case study analysed models of tree and stand growth under climate change for National Forest Inventory stands in Sweden and Finland for “business as usual,” “conifer production” from a tree breeding programme, and “combined conifer and broadleaved production” where conifer is from improved FRM. The analyses showed the potential to maintain production targets using improved conifer FRM from the breeding programmes (Ahtikoski et al., 2020a,b). In the combined conifer and broadleaved production scenario, production could be maintained from improved conifer FRM while mixing with broadleaved species for resilience and biodiversity, in which birch is unimproved and is from natural regeneration.
Scotland case study
The Scots pine breeding programme in Scotland was terminated in 2002, with the establishment of improved seed orchards. Scots pine is the only native productive conifer in Scotland and the UK, with approximately 154,000 ha managed in plantations covering 14% of Scottish forests and is second only to Sitka spruce occupying 507,000 ha of forest land in Scotland (Forest Research, 2021). Approximately 90% of the Scots pine is of plantation origin and managed for multi-purpose forestry including timber production and forms the north-western Atlantic edge of the Scots pine native range in Europe. Eighty-four fragmented populations of the native Caledonian pine wood retain high levels of genetic diversity with considerable genetic differentiation between western and eastern populations. The Scottish climate shows a distinct rainfall gradient between the wetter west (precipitation ∼3,000 mm) and drier east (precipitation ∼700 mm) of the country. In the west winters are relatively mild and much cooler in the east with a gradient of growing days (over 5°C) of ∼300 days in the west to ∼100 days in the east.
Climate change projections for Scotland suggest the east of Scotland will become drier and the west wetter with a particular seasonal shift in precipitation from summer to winter seasons (Murphy et al., 2019). The likelihood is that Sitka spruce planted in the east of Scotland will therefore be less suited to the drier climate. Recent dry years in Scotland have shown Sitka spruce to be very susceptible to drought crack (Green et al., 2008), and consequently the potential for changing spruce plantations to the less drought sensitive Scots pine is recommended. This is already occurring with a significant increase in the number of Scots pine seedlings planted in recent years – 8.7 and 7.8 million seedlings sold in 2018–2019 and 2019–2020, respectively, compared to an annual average of 2.9 million between 2005–2006 and 2009–2010 (Forest Research, 2021).
Another driver for a switch to Scots pine comes from Perry et al. (2016). They showed that provenances of Scots pine from the west coast of Scotland have greater resistance to Dothistroma septosporum, which causes Dothistroma needle blight in many pine species (including Scots pine), and that west coast provenances also show improved height growth. These findings suggest a growth improvement, resistance to Dothistroma needle blight and provide evidence for re-opening the Scots pine breeding programme to improve Scottish Scots pine seed orchards with material from native Caledonian pinewood provenances in the west of Scotland.
A questionnaire to stakeholders asking about the priorities for tree breeding in Scotland and the UK achieving a 45% response rate, revealed the acceptance of the importance for conserving Scots pine populations, based on the recognition of the multiple benefits of Scots pine across sectors. When asked to rank the most important species for tree improvement research, respondents from the private sector ranked Sitka spruce as their first option and respondents from the public sector ranked Scots pine as their first option. There was general agreement that the Scots pine breeding programme was terminated too soon, and that Scots pine will be increasingly important under climate change. The case study also explored the economic case for Scots pine breeding with a sensitivity analysis of expected gains in growth and disease resistance showing a potential increase in annuity payments to growers of £9–£30 ha–1 year–1, even the low end of this range would repay the investment in re-opening a Scots pine breeding programme.
Restoration and expansion of the fragmented Caledonian pinewoods is an objective of several environmental organisations. These groups were under-represented in the survey and the preferred means of expanding these woodlands is natural regeneration rather than planting (Summers and Cavers, 2021). Given the general appetite for developing Scots pine FRM under climate change, a range of options are available for taking forward a modular programme of research, as it appears in the public interest to do so, and there appears to be no perceived conflict among management objectives in the use of FRM.
Atlantic edge and Southern Europe case study
A case study of the benefits, costs, and risks related to the use of improved FRM for plantation forestry in the Atlantic region of Southern Europe has revealed a disturbing future perspective for forest in the Mediterranean region. In the transition to a bio-based economy ever more reliance on plantation forests is needed to satisfy an increasing demand for wood-based products and non-timber forest products (Gardiner and Moore, 2014). Climate change presents many challenges and threats for forests in southern Europe, notably drought stress (Allen et al., 2015), pest and pathogen occurrence (Jactel et al., 2020) and fire (Silva et al., 2018) all threaten the continued supply of forest ecosystem services in Southern Europe.
Silva et al. (2018) recommended that the 20th century practice of even-aged monoculture forests must adapt to changing conditions and social values of the 21st century, moving towards a mix of species and age classes at the stand and landscape scales. In addition, they argued that scrub on abandoned forest land must be brought back into management to reduce the fuel load in a region so vulnerable to mega-fires. Even in continuous-cover and close-to-nature management, a risk has been expressed of increasing maladaptation as the rate of climate change and climate extremes exceeds the rate at which natural selection can act to drive the adaptation/acclimation of trees and forests to changing climatic conditions (Fréjaville et al., 2019). Consequently, the case study report recommends the use of selected FRM better adapted to climate extremes and introduced into close-to-nature silvicultural systems.
An analysis of stakeholder demands in southern Europe shows a shift from productivity focused FRM to material that has better resilience to biotic disturbances. An exponential increase in diseases and pests has been reported in pine plantations (Jactel et al., 2020) with pine wilt nematode (Bursaphelenchus xylophilus) causing pine wilt disease (PWD), pitch pine canker caused by Fusarium circinatum, tip blight (Diplodia pinea) and red band needle blight (Dothistroma septosporum and Dothistroma pini). These problems are being exacerbated by increasing drought. The development of new tree breeding programmes to rapidly select material more resistant to drought and biotic problems is underway (Menéndez-Gutiérrez et al., 2018; Díaz Vázquez et al., 2020).
A potential solution to the trailing edge problems of maritime pine is the expansion of stone pine that might offer a profitable alternative in Atlantic coastal areas, offering a high-value non-wood forest product of gourmet pine nut kernels that exceed 70–100€.kg–1 in retail value (Mutke et al., 2019). Moreover, this species is more tolerant of drought and less susceptible to the prevailing risks of PWD and F. circinatum (Pimentel et al., 2017). Profitable stone pine cultivation allows for the maintenance of low-density plantations without understory, which is an effective fire-resistant landscape scale adaptive silvicultural system (Guadaño et al., 2016).
Alluvial regions of Central and Southern Europe case study
Poplar is a fast-growing tree and is an economically important forest type, globally covering about 31 million ha (Marchi et al., 2022). In Europe it is commonly planted across the plains of Spain, France, Belgium, Italy, Hungary, and Romania, amounting to over 900,000 ha in the EU. Typical growth rates are 15–20 m3 ha–1 year–1 from improved poplar clones (Chiarabaglio et al., 2020).
Common garden trials have shown that improved clones have increased survival, health, resilience as well as productivity under climate change (Marchi et al., 2022). The case study assembled the evidence that new GS tools more rapidly identify the material suited to projected climate futures of specific site types.
Discussion
The perception of tree breeding in Europe’s forest-based sector expects improvements in a range of species, a diversification of species used in forest restoration and expansion, new plantations that include stands of improved material better adapted to climate change extremes and biotic threats. The expectation of a Scottish “Green Gold” scenario (Burton et al., 2019) in other areas of Europe, defined as the juxtaposition of sustainably managed productive plantation stands, continuous-cover managed stands, stands of native woodland, recolonisation and open areas, must focus on the need for guideline recommendations to enrich natural regeneration with better adapted FRM (Table 1). In a B4EST questionnaire of tree breeding needs, respondents identified the resilience of forest to the effects of climate change as paramount, using material selected for growth, improved wood quality, and resistance to abiotic and biotic threats. Showing that the management of the risk of forest damage from abiotic and biotic threats is becoming critical for the industry exploring the potential of a developing bio-based economy in Europe.
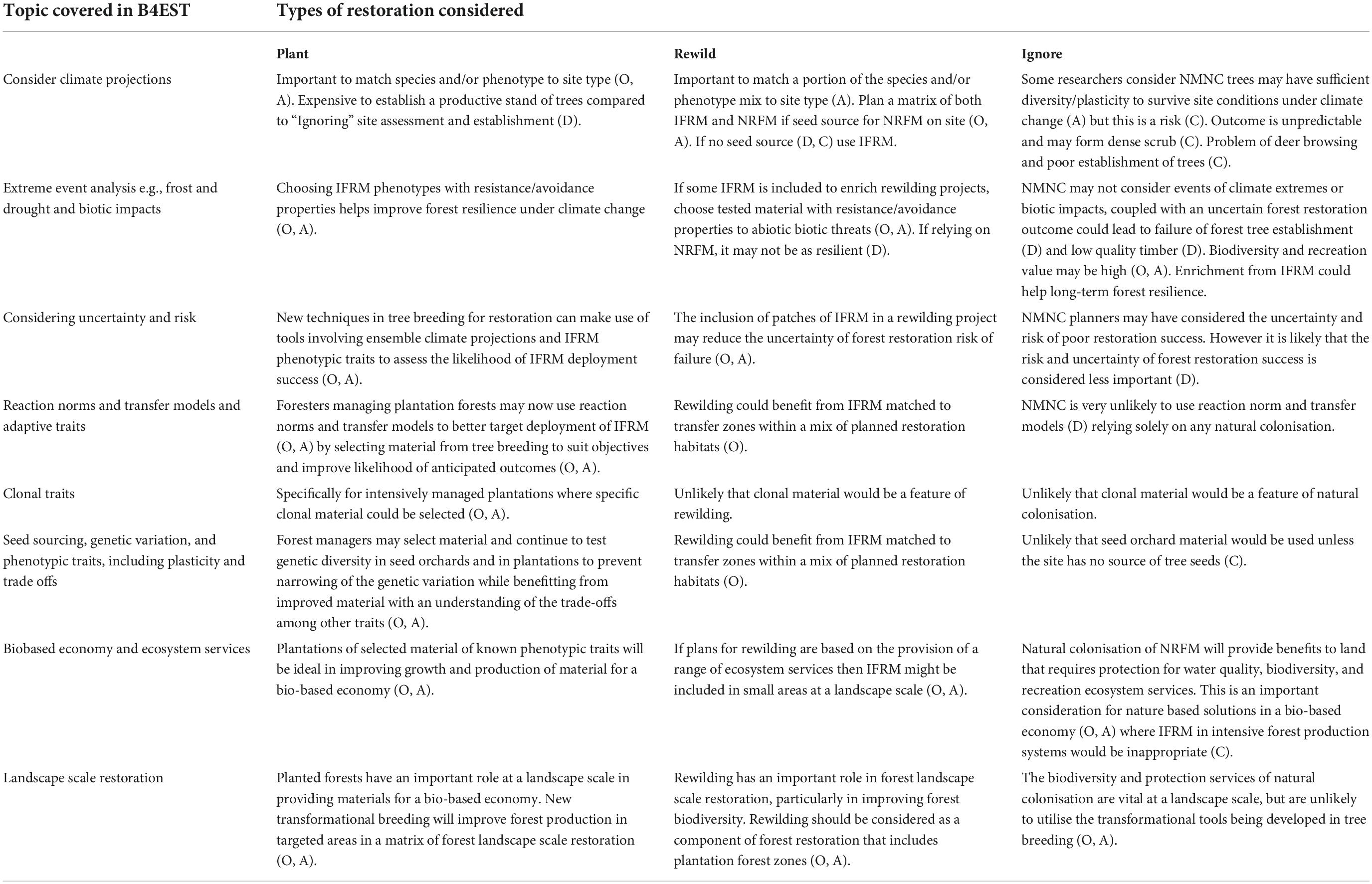
Table 1. Opportunities (O), advantages (A), disadvantages (D), and constraints (C) of new transformational approaches in tree breeding to “Plant, Rewild or Ignore” methods of forest restoration in which improved forest reproductive material (IFRM) and or material from natural regenerating forest material (NRFM) might be managed, or no management natural colonisation (NMNC) is considered important.
Outside the forestry sector, and in the societal and political sphere, forest and woodland restoration and expansion has moved to the centre of attention due to losses from land-use change, fire, pests, and pathogens; but also through potential gains from restoration (Elliott et al., 2013) expansion and the concomitant benefits of wood product substitution in society (Morison et al., 2012). For this reason, forests have become an important contributory mechanism through which economies can offset fossil carbon use through investing in forestry sequestration schemes (Yousefpour et al., 2012; Sing et al., 2018). Importantly, the twin issues of climate change and biodiversity loss are being addressed through forest restoration and expansion projects with the consideration of the effect of time-lags (Watts et al., 2020) and hysteresis affecting the recolonisation of forest specialists in species-area abundance and richness relationships.
We need a mix of forestry objectives into an uncertain future – proportions of natural regeneration, plantation woodlands, as well as a more diverse range of tested genotypes for use in new environmental conditions under climate change. Tree breeding has a contribution to make in delivering these multiple objectives. The European Commission Survey for a European Union Forest Strategy (EC, 2021) showed awareness and concern over the erosion of biodiversity resulting from intensive plantation forestry, with increased risks from pests and diseases under climate change, and the erosion of landscape quality. Novel techniques in tree breeding such as high throughput GS and the development of molecular techniques for assessing genetic diversity, can help improve forest resilience, maintain genetic diversity for future generations, and provide forest material suited to the expanding needs of a bio-based economy. We see this innovation as fulfilling a small proportion of the forestry sector but importantly delivering mutual benefits of conservation and products to industry and society, thereby allowing more extensive forest restoration and expansion projects to deliver other ecosystem services of biodiversity, water quality, soil protection and forest recreation. In many European countries, forest owners manage productive high value stands of trees that provide the income and the opportunity to diversify forestry systems. The range of forestry systems, and particularly systems with low management intensity, provides a wide range of ecosystem services (Duncker et al., 2012; Ray et al., 2016) as well as high forest biodiversity associated with natural regeneration and continuous cover forestry.
The benefits of selecting FRM with tested trait resilience will deliver transformative changes in adaptive forest management, in particular benefits in resisting damage and mortality from biotic impacts. This would happen through selective planning of forest ecosystem service delivery at a landscape scale. Here the benefits from resilient forest genotypes in selected stands will grow in a matrix with more extensively managed forest, where the pressure for single-species single-age plantation silviculture is replaced with a greater proportion of mixed species mixed aged stands, managed on a continuous cover silvicultural system. The economic benefits of breeding improved forest material will offset any reduced income from products in extensively managed stands. For this to work efficiently the selection of FRM at provenance, progeny or at the clonal level must need to assess the trade-offs of traits among phenotypes.
Tree-breeding is considered a lever to meet socio-economic challenges in forestry. The development of tools and knowledge in tree breeding have made available information about the degree of genetic variation and plasticity among and within different tree species. Breeders can therefore select material (genotypes) with a known degree of phenotypic plasticity across environmental gradients and continue to review the genetic diversity of seed orchard material, offering ways to maintain and improve the diversity of the pool. Breeders have climate and site data to assess the suitability and the risk of deployment of different phenotypes under a changing climate while keeping a check on the economic value (Ahtikoski et al., 2020a,b) of material deployed across suitable sites within the range of timber processing facilities in the bioeconomy.
We arrive back at the question: to plant, rewild, or ignore? As tree breeders we believe a contribution is required across the range of these forest restoration options (Table 1). “Planting” and assisted migration of selected and tested FRM will be important to help secure increased resilience under climate change of intensively managed productive stands for the timber and wood products markets. “Rewilding” for biodiversity conservation will provide ecosystem services protecting soil and water and in the longer-term support forest with high carbon above and below ground stocks. “Ignoring” land is likely to have an uncertain trajectory that may not revert to forest. Relying solely on natural colonisation without interventions will lead to a long time-lag for specialist and sensitive species to re-colonise. Without top predators, the likelihood would be large areas of scrub woodland with extremely high densities of deer (Spake et al., 2020).
Rewilding and ignoring could be important and interesting components of forest restoration and expansion for biodiversity and carbon storage in the longer-term. However, society and national economies are heavily reliant on timber and wood products, and the support and benefits offered by tree breeders in the selection of material for planting is key to building and maintaining timber and wood processing in the bioeconomy.
Conclusion
B4EST has explored and tested transformative changes in tree breeding. These include:
1. Tree responses to climate drivers using reaction norm and transfer models, to help locate FRM for deployment under climate change
2. The adoption of linear mixed effects models to account for the components of genetic diversity and phenotypic plasticity in material tested in genetic common garden trials, to help improve our understanding of the changes in the spatial suitability of material under climate change
3. The development of methods to assess the trade-offs among traits within a species, to better understand the changes in other traits when choosing FRM based on a single trait
4. The development and deployment of SNP arrays to map the important areas of multiple loci that control genes affecting traits in forest species, to help map loci that control gene expression without the need to test expression in trials
5. A single “4-Tree” SNP array (Guilbaud et al., 2020) with the novel functionality for probing the genome of four different tree species: common ash, stone pine, poplar, and maritime pine
6. An improved understanding of the links among the omics to improve precision in GS
7. Growth models of the future growth uncertainty of provenances of Scots pine at the leading edge and trailing edge of the species range
8. Calculating the economic benefits of tree breeding approaches in four different regions of Europe: Nordic spruce and Scots pine forests, Scotland’s pine forest, Mediterranean pine forests, and poplar plantations in France and Italy.
These developments bode well for the improvement of tree breeding to stimulate transformative change in the way FRM may be deployed to produce more resilient forest for products in Europe’s developing bioeconomy and, in so doing, provide opportunities for less intensive mixed species forest stands in forest restoration projects, using close to nature approaches to deliver a suite of other ecosystem services to society.
Author contributions
DR, MB, and RA: manuscript writing. SG-M and CB: discussion ideas. JH: case study ideas. RA and LS: quality checking. All authors conceived the study and its objectives.
Funding
This study was funded by the European Project H2020 “Adaptive breeding for productive, sustainable and resilient forests under climate change” (B4EST; grant agreement No. 773383).
Acknowledgments
We are grateful for the very helpful comments of two reviewers and the handling editor.
Conflict of interest
The authors declare that the research was conducted in the absence of any commercial or financial relationships that could be construed as a potential conflict of interest.
Publisher’s note
All claims expressed in this article are solely those of the authors and do not necessarily represent those of their affiliated organizations, or those of the publisher, the editors and the reviewers. Any product that may be evaluated in this article, or claim that may be made by its manufacturer, is not guaranteed or endorsed by the publisher.
Footnotes
References
Ahtikoski, A., Ahtikoski, R., Haapanen, M., Hynynen, J., and Kärkkäinen, K. (2020a). Economic performance of genetically improved reforestation material in joint production of timber and carbon sequestration: a case study from Finland. Forests 11:847. doi: 10.3390/F11080847
Ahtikoski, A., Karhu, J., Ahtikoski, R., Haapanen, M., Hynynen, J., and Kärkkäinen, K. (2020b). Financial assessment of alternative breeding goals using stand-level optimization and data envelopment analysis. Scand. J. Forest Res. 35, 262–273. doi: 10.1080/02827581.2020.1795241
Alberto, F. J., Aitken, S. N., Alia, R., Gonzalez-Martinez, S. C., Hanninen, H., Kremer, A., et al. (2013). Potential for evolutionary responses to climate change - evidence from tree populations. Global Change Biol. 19, 1645–1661. doi: 10.1111/gcb.12181
Allen, C. D., Breshears, D. D., and McDowell, N. G. (2015). On underestimation of global vulnerability to tree mortality and forest die-off from hotter drought in the Anthropocene. Ecosphere 6, 1–55. doi: 10.1890/ES15-00203.1
Anon (2005). Restoring forest Landscapes: An introduction to the art and science of forest landscape restoration. ITTO Technical Series 23. Yokohama: International Tropical Timber Organisation.
Archambeau, J., Garzón, M. B., Barraquand, F., de Miguel, M., Plomion, C., and González-Martínez, S. C. (2022). Combining climatic and genomic data improves range-wide tree height growth prediction in a forest tree. Am. Nat. 200, E141–E159. doi: 10.1086/720619
Archambeau, J., Garzón, M. B., de Vega, M. M., Brachi, B., Barraquand, F., and González-Martínez, S. C. (2021). Extreme climatic events but not environmental heterogeneity shape within-population genetic variation in maritime pine. bioRxiv [preprint]. doi: 10.1101/2021.08.17.456636
Barriopedro, D., Sousa, P. M., Trigo, R. M., Garcia-Herrera, R., and Ramos, A. M. (2020). The exceptional Iberian heatwave of summer 2018. Bull. Am. Meteorol. Soc. 101, S29–S34.
Beguería, S., Vicente-Serrano, S. M., Reig, F., and Latorre, B. (2014). Standardized precipitation evapotranspiration index (SPEI) revisited: parameter fitting, evapotranspiration models, tools, datasets and drought monitoring. Int. J. Climatol. 34, 3001–3023. doi: 10.1002/joc.3887
Benito-Garzón, M., Alía, R., Robson, T. M., and Zavala, M. A. (2011). Intra-specific variability and plasticity influence potential tree species distributions under climate change. Global Ecol. Biogeography 20, 766–778. doi: 10.1111/j.1466-8238.2010.00646.x
Benito-Garzón, M., Fady, B., Davi, H., Vizcaíno-Palomar, N., and Fernández-Manjarrés, J. (2018). Trees on the move: Using decision theory to compensate for climate change at the regional scale in forest social-ecological systems. Reg. Environ. Change 18, 1427–1437. doi: 10.1007/s10113-018-1277-y
Benito-Garzón, M., Robson, T. M., and Hampe, A. (2019). ΔTraitSDM: species distribution models that account for local adaptation and phenotypic plasticity. New Phytol. 222, 1757–1765. doi: 10.1111/nph.15716
Berlin, M. (2019). Planter’s Guide - New Tool for Choosing Scots Pine Plant Material in Sweden and Finland. Sweden: Skogforsk.
Berlin, M., Almqvist, C., Haapanen, M., and Högberg, K. (2019). Common Scots Pine Deployment Recommendations for Sweden and Finland. Sweden: Skogforsk.
Bernhardsson, C., Zan, Y., Chen, Z., Ingvarsson, P. K., and Wu, H. X. (2021). Development of a highly efficient 50K single nucleotide polymorphism genotyping array for the large and complex genome of Norway spruce (Picea abies L. Karst) by whole genome resequencing and its transferability to other spruce species. Mol. Ecol. Resources 21, 880–896. doi: 10.1111/1755-0998.13292
Broadmeadow, M. S. J., Ray, D., and Samuel, C. J. A. (2005). Climate change and the future for broadleaved tree species in Britain. Forestry 78, 145–161. doi: 10.1093/forestry/cpi014
Burton, V., Metzger, M. J., Brown, C., and Moseley, D. (2019). Green Gold to Wild Woodlands: understanding stakeholder visions for woodland expansion in Scotland. Landscape Ecol. 34, 1693–1713. doi: 10.1007/s10980-018-0674-4
Burton, V., Moseley, D., Brown, C., Metzger, M. J., and Bellamy, P. (2018). Reviewing the evidence base for the effects of woodland expansion on biodiversity and ecosystem services in the United Kingdom. For. Ecol. Manage. 430, 366–379.
Chazdon, R. L., and Guariguata, M. R. (2016). Natural regeneration as a tool for large-scale forest restoration in the tropics: prospects and challenges. Biotropica 48, 716–730. doi: 10.1111/btp.12381
Chiarabaglio, P. M., Deidda, A., Bergante, S., Castro, G., Faciotto, G., Giorcelli, A., et al. (2020). Life Cycle Assessment (LCA): new poplar clones allow an environmentally sustainable cultivation. Ann. Silvicultural Res. 45, 76–82.
Crouzeilles, R., Ferreira, M. S., Chazdon, R. L., Lindenmayer, D. B., Sansevero, J. B. B., Monteiro, L., et al. (2017). Ecological restoration success is higher for natural regeneration than for active restoration in tropical forests. Sci. Adv. 3:e1701345. doi: 10.1126/sciadv.1701345
Crouzeilles, R., Beyer, H. L., Monteiro, L. M., Feltran-Barbieri, R., Pessôa, A. C. M., Barros, F. S. M., et al. (2020). Achieving cost-effective landscape-scale forest restoration through targeted natural regeneration. Conserv. Lett. 13, 1–9. doi: 10.1111/conl.12709
de Miguel, M., Rodríguez-Quilón, I., Heuertz, M., Hurel, A., Grivet, D., Jaramillo-Correa, J. P., et al. (2022). Polygenic adaptation and negative selection across traits, years and environments in a long-lived plant species (Pinus pinaster Ait.). Mol. Ecol. 31, 2089–2105. doi: 10.1111/mec.16367
Di Pierro, E. A., Mosca, E., Gonzalez-Martinez, S. C., Binelli, G., Neale, D. B., and La Porta, N. (2017). Adaptive variation in natural Alpine populations of Norway spruce (Picea abies [L.] Karst) at regional scale: landscape features and altitudinal gradient effects. Forest Ecol. Manag. 405, 350–359.
Díaz Vázquez, R., Menéndez, M., and Prada, E. (2020). La mejora genética como herramienta de control frente al nematodo del pino. Primeros pinos tolerantes catalogados en España. Foresta 78, 84–88.
Duncker, P. S., Barreiro, S. M., Hengeveld, G. M., Lind, T., Mason, W. L., Ambrozy, S., et al. (2012). Classification of forest management approaches: a new conceptual framework and its applicability to european forestry. Ecol. Soc. 17:51.
EC (2021). New EU forest strategy for 2030, communication from the commission to the european parliament, the council, the european economic and social committee and the committee of the regions, COM (2021) 572 final. Brussels: European Commission.
Elliott, S., Navakitbumrung, P., Kuarak, C., Zangkum, S., Anusarnsunthorn, V., and Blakesley, D. (2003). Selecting framework tree species for restoring seasonally dry tropical forests in northern Thailand based on field performance. Forest Ecol. Manag. 184, 177–191. doi: 10.1016/S0378-1127(03)00211-1
Elliott, S. D., Blakesley, D., and Hardwick, K. (2013). Restoring Tropical Forests, A Practical Guide. London: Royal Botanic Gardens Kew.
Ennos, R., Cottrell, J., Hall, J. E., and O’Brien, D. (2019). Is the introduction of novel exotic forest tree species a rational response to rapid environmental change? – a British perspective. Forest Ecol. Manag. 432, 718–728. doi: 10.1016/j.foreco.2018.10.018
Fréjaville, T., Vizcaíno-Palomar, N., Fady, B., Kremer, A., and Benito-Garzón, M. (2019). Range margin populations show high climate adaptation lags in European trees. Global Change Biol. 26, 484–495. doi: 10.1111/gcb.14881
Gamfeldt, L., Snäll, T., Bagchi, R., Jonsson, M., Gustafsson, L., Kjellander, P., et al. (2013). Higher levels of multiple ecosystem services are found in forests with more tree species. Nat. Commun. 4:1340.
Gardiner, B., and Moore, J. (2014). “Creating the wood supply of the future,” in Challenges and Opportunities for the World’s Forests in the 21st Century. Forestry Sciences, ed. T. Fenning (Dordrecht: Springer).
Green, S., Hendry, S. J., and Redfern, D. B. (2008). Drought damage to pole-stage Sitka spruce and other conifers in north-east Scotland. Scott. For. 62, 10–18.
Guadaño, C., Iglesias, S., Leon, D., Arribas, S., Gordo, J., Gil, L., et al. (2016). Establecimiento de Plantaciones Clonales de Pinus Pinea Para la Producción de Piñón Mediterráneo, Serie Forestal 28. Madrid: INIA.
Guilbaud, R., Biselli, C., Buiteveld, J., Copini, P., Dowkiw, A., et al. (2020). “Development of a new tool (4TREE) for adapted genome selection in European tree species,” in Proceedings of the Gentree Symposium, (Avignon).
Gutschick, V. P., and BassiriRad, H. (2003). Extreme events as shaping physiology, ecology, and evolution of plants: toward a unified definition and evaluation of their consequences. New Phytol. 160, 21–42. doi: 10.1046/j.1469-8137.2003.00866.x
Haapanen, M., and Ruotsalainen, S. (2021). Adaptive performance of genetically improved and unimproved seedlings of scots pine. Silva Fennica 55, 1–14. doi: 10.14214/SF.10534
Hallingbäck, H. R., Burton, V., Vizcaíno-Palomar, N., Trotter, F., Liziniewicz, M., Marchi, M., et al. (2021). Managing uncertainty in Scots pine range-wide adaptation under climate change. Front. Ecol. Evol. 9:724051. doi: 10.3389/fevo.2021.724051
Hanlon, H. M., Bernie, D., Carigi, G., and Lowe, J. A. (2021). Future changes to high impact weather in the UK. Climatic Change 166, 1–23. doi: 10.1007/s10584-021-03100-5
Harris, I., Osborn, T. J., Jones, P., and Lister, D. (2020). Version 4 of the CRU TS monthly high-resolution gridded multivariate climate dataset. Sci. Data 7, 1–18. doi: 10.1038/s41597-020-0453-3
Haasnoot, M., Kwakkel, J. H., and Walker, W. E. (2013). Dynamic adaptive policy pathways?: A method for crafting robust decisions for a deeply uncertain world. Glob. Environ. Change 23, 485–498. doi: 10.1016/j.gloenvcha.2012.12.006
Hayatgheibi, H., Haapanen, M., Lundströmer, J., Berlin, M., Kärkkäinen, K., and Helmersson, A. (2021). The impact of drought stress on the height growth of young norway spruce full-sib and half-sib clonal trials in Sweden and Finland. Forests 12, 1–15. doi: 10.3390/f12040498
Heuclin, B., Mortier, F., Trottier, C., and Denis, M. (2021). Bayesian varying coefficient model with selection: An application to functional mapping. J. R. Stat. Soc. Ser. C 70, 24–50. doi: 10.1111/rssc.12447
Hijmans, R. J., Cameron, S. E., Parra, J. L., Jones, P. J., and Jarvis, A. (2005). Very high resolution interpolated climate surfaces for global land areas. Int. J. Climatol. 25, 1965–1978.
Hlásny, T., Zimová, S., Merganičová, K., Štēpánek, P., Modlinger, R., and Turčáni, M. (2021). Devastating outbreak of bark beetles in the Czech Republic: drivers, impacts, and management implications. Forest Ecol. Manag. 490:119075. doi: 10.1016/j.foreco.2021.119075
Hof, A. R., Zachrisson, A., and Polvi, L. E. (2021). Forest restoration: do site selection and restoration practices follow ecological criteria? a case study in Sweden. Forests 12:988. doi: 10.3390/f12080988
Hurel, A., de Miguel, M., Dutech, C., Desprez_Loustau, M.-L., Plomion, C., Rodríguez-Quilón, I., et al. (2021). Genetic basis of growth, spring phenology, and susceptibility to biotic stressors in maritime pine. Evol. Appl. 14, 2750–2772. doi: 10.1111/eva.13309
Ingvarsson, P. K., and Bernhardsson, C. (2020). Genome-wide signatures of environmental adaptation in European aspen (Populus tremula) under current and future climate conditions. Evol. Appl. 13, 132–142. doi: 10.1111/eva.12792
Jacob, D., Kotova, L., Teichmann, C., Sobolowski, S. S., Vautard, R., Donnely, C., et al. (2018). Climate impacts in Europe under +1.5°C global warming. Earth’s Future 6, 264–285.
Jactel, H., Bauhus, J., Boberg, J., Bonal, D., Castagneyrol, B., Gardiner, B., et al. (2017). Tree diversity drives forest stand resistance to natural disturbances. Curr. Forestry Rep. 3, 223–243. doi: 10.1007/s40725-017-0064-1
Jactel, H., Branco, M., Duncker, P., Gardiner, B., Grodzki, W., Langstrom, B., et al. (2012). A multicriteria risk analysis to evaluate impacts of forest management alternatives on forest health in Europe. Ecol. Soc. 17:52.
Jactel, H., Desprez-Loustau, M.-L., Battisti, A., Brockerhoff, E., Santini, A., Stenlid, J., et al. (2020). Pathologists and entomologists must join forces against forest pest and pathogen invasions. NeoBiota 58, 107–127.
Jaramillo-Correa, J. P., Rodríguez-Quilón, I., Grivet, D., Lepoittevin, C., Sabastiana, F., Heuertz, M., et al. (2015). Molecular proxies for climate maladaptation in a long-lived tree (Pinus pinaster Aiton. Pinaceae). Genetics 199, 793–807. doi: 10.1534/genetics.114.173252
Kainer, D., Stone, E. A., Padovan, A., Foley, W. J., and Külheim, K. (2018). Accuracy of genomic prediction for foliar terpene traits in Eucalyptus polybractea. G3: Genes| Genomes| Genetics 8, 2573–2583. doi: 10.1534/g3.118.200443
Karger, D. N., Conrad, O., Böhner, J., Kawohl, T., Kreft, H., Soria-Auza, R. W., et al. (2017). Climatologies at high resolution for the earth’s land surface areas. Sci. Data 4, 1–20. doi: 10.1038/sdata.2017.122
Kastally, C., Niskanen, A. K., Perry, A., Kujala, S. T., Avia, K., Cervantes, S., et al. (2021). Taming the massive genome of Scots pine with PiSy50k, a new genotyping array for conifer research. Plant J. 109, 1337–1350. doi: 10.1111/tpj.15628
Konnert, M., Fady, B., Gömöry, D., A’Hara, S., Wolter, F., Ducci, F., et al. (2015). Use and Transfer of Forest Reproductive Material in Europe in the Context of Climate Change. Rome: European Forest Genetic Resources Programme (EUFORGEN), Bioversity International.
Lamb, D., and Gilmour, D. (2005). Rehabilitation and restoration of degraded forests. Restoration Ecol. 13, 578–579. doi: 10.1111/j.1526-100X.2005.00073.x
Lefèvre, F., Koskela, J., Hubert, J., Kraigher, H., Longauer, R., Olrik, D. C., et al. (2013). Dynamic conservation of forest genetic resources in 33 european countries. Conserv. Biol. 27, 373–384. doi: 10.1111/j.1523-1739.2012.01961.x
López-Hernández, F., and Cortés, A. J. (2019). Last-generation genome-environment associations reveal the genetic basis of heat tolerance in common bean (Phaseolus vulgaris L.). Front. Genet. 10:954. doi: 10.3389/fgene.2019.00954
MacDonald, E., Gardiner, B., and Mason, W. (2010). The effects of transformation of even-aged stands to continuous cover forestry on conifer log quality and wood properties in the UK. Forestry 83, 1–16. doi: 10.1093/forestry/cpp023
MacDonald, E., and Hubert, J. (2002). A review of the effects of silviculture on timber quality of Sitka spruce. Forestry 75, 107–138. doi: 10.1093/forestry/75.2.107
Marchi, M., Bergante, S., Ray, D., Barbetti, R., Facciotto, G., Chiarabaglio, P.-M., et al. (2022). Universal reaction norms for the sustainable cultivation of hybrid poplar clones under climate change in Italy. iForest - Biogeosci. Forestry 15, 47–55.
Marchi, M., Castellanos-Acuña, D., Hamann, A., Wang, T., Ray, D., and Menzel, A. (2020). ClimateEU: scale-free climate normals, historical time series, and future projections for Europe. Nat. Sci. Data 7:228. doi: 10.1038/s41597-020-00763-0
Mason, W. L. (2015). Implementing continuous cover forestry in planted forests: experience with sitka spruce (Picea Sitchensis) in the British Isles. Forests 6, 879–902. doi: 10.3390/f6040879
MCPFE (2020). “State of Europe’s Forests: Summary for policymakers,” in Proceedings of the ministerial conference on the protection of forests in europe (Zvolen: Forest Europe, Liaison Unit Bratislava).
Meason, D. F., and Mason, W. L. (2014). Evaluating the deployment of alternative species in planted conifer forests as a means of adaptation to climate change - case studies in New Zealand and Scotland. Ann. Forest Sci. 71, 239–253. doi: 10.1007/s13595-013-0300-1
Menéndez-Gutiérrez, M., Alonso, M., Toval, G., and Díaz, R. (2018). Testing of selected Pinus pinaster half-sib families for tolerance to pinewood nematode (Bursaphelenchus xylophilus). Forestry 91, 38–48.
Morison, J., Matthews, R., Miller, G., Perks, M., Randle, T., Vanguelova, E., et al. (2012). Understanding the Carbon and Greenhouse Gas Balance of Forests in Britain. Edinburgh: Research Report, Forestry Commission.
Murphy, J. M., Harris, G. R., Sexton, D. M. H., Kendon, E. J., Bett, P. E., Clark, R. T., et al. (2019). UKCP18 Land Projections. Exeter: Science Report.
Mutke, S., Vendramin, G. G., Fady, B., Bagnoli, F., and González-Martínez, S. C. (2019). “Molecular and quantitative genetics of stone pine (Pinus pinea),” in Genetic Diversity in Horticultural Plants, Sustainable Development and Biodiversity, ed. D. Nandwani (Berlin: Springer International Publishing), 61–84.
Norman, A., Taylor, J., Edwards, J., and Kuchel, H. (2018). Optimising genomic selection in wheat: effect of marker density, population size and population structure on prediction accuracy. G3 8, 2889–2899. doi: 10.1534/g3.118.200311
Notivol, E., Santos, del Blanco, L., Chambel, R., Climent, J., and Alía, R. (2020). Seed sourcing strategies considering climate change forecasts: a practical test in Scots pine. Forests 11:1222. doi: 10.3390/f11111222
Oddou-Muratorio, S., Davi, H., and Lefevre, F. (2020). Integrating evolutionary, demographic and ecophysiological processes to predict the adaptive dynamics of forest tree populations under global change. Tree Genetics Genomes 16:67. doi: 10.1007/s11295-020-01451-1
Pegard, M., Segura, V., Munoz, F., Bastien, C., Jorge, V., and Sanchez, L. (2020). Favorable conditions for genomic evaluation to outperform classical pedigree evaluation highlighted by a proof-of-concept study in poplar. Front. Plant Sci. 11:581954. doi: 10.3389/fpls.2020.581954
Perry, A., Brown, A. V., Cavers, S., Cottrell, J. E., and Ennos, R. A. (2016). Has Scots pine (Pinus sylvestris) co-evolved with Dothistroma septosporum in Scotland? Evidence for spatial heterogeneity in the susceptibility of native provenances. Evol. Appl. 9, 982–993. doi: 10.111/eva.12395
Petr, M., Boerboom, L. G. J., Ray, D., and Van Der Veen, A. (2015). Adapting Scotland’s forests to climate change using an action expiration chart. Environ. Res. Lett. 10:105005. doi: 10.1088/1748-9326/10/10/105005
Petr, M., Boerboom, L. G. J., Van der Veen, A., and Ray, D. (2014). A spatial and temporal drought risk assessment of three major tree species in britain using probabilistic climate change projections. Climatic Change 124, 791–803. doi: 10.1007/s10584-014-1122-3
Pimentel, C. S., Gonçalves, E. V., Firmino, P. N., Calvão, T., Fonseca, L., Abrantes, I., et al. (2017). Differences in constitutive and inducible defences in pine species determining susceptibility to pinewood nematode. Plant Pathol. 66, 131–139.
Postolache, D., Oddou-Muratorio, S., Vaja, E., Bagnoli, F., Guichoux, E., Hampe, A., et al. (2021). Genetic signatures of divergent selection in European beech (Fagus sylvatica L.) are associated with the variation in temperature and precipitation across its distribution range. Mol. Ecol. 30, 5029–5047. doi: 10.1111/mec.16115
Ramírez-Valiente, J. A., Santos, del Blanco, L., Alía, R., Robledo-Arnuncio, J. J., and Climent, J. (2021). Adaptation of Mediterranean forest species to climate: lessons from common garden experiments. J. Ecol. 10, 1022–1042.
Ray, D., Sing, L., and Nicoll, B. (2016). Agriculture & forestry report card paper 9?: Forest ecosystem services and climate change. London: UKRI. doi: 10.13140/RG.2.1.1382.8087
Rellstab, C., Gugerli, F., Eckert, A. J., Hancock, A. M., and Holderegger, R. (2015). A practical guide to environmental association analysis in landscape genomics. Mol. Ecol. 24, 4348–4370.
Rey Benayas, J. M., Newton, A. C., Diaz, A., and Bullock, J. M. (2009). Enhancement of biodiversity and ecosystem services by ecological restoration: a meta-analysis. Science 325, 1121–1124. doi: 10.1126/science.1172460
Reynolds, C., Jinks, R., Kerr, G., Parratt, M., and Mason, B. (2021). Providing the evidence base to diversify Britain’s forests: initial results from a new generation of species trials. Quarterly J. Forestry 115, 26–37.
Romero Navarro, J. A., Phillips-Mora, W., Arciniegas-Leal, A., Mata-Quirós, A., Haiminen, N., Mustiga, G., et al. (2017). Application of genome wide association_and genomic prediction for improvement of cacao productivity and resistance to black and frosty pod diseases. Front. Plant Sci. 8:1905. doi: 10.3389/fpls.2017.01905
Ruiz Daniels, R., Taylor, R. S., Serra-Varela, M. J., Vendramin, G. G., Gozález-Martínez, S. C., and Grivet, D. (2018). Inferring selection in instances of long-range colonization: the Aleppo pine (Pinus halepensis) in the Mediterranean Basin. Mol. Ecol. 27, 3331–3345. doi: 10.1111/mec.14786
Scottish Government (2021). Scotland’s Third Land Use Strategy 2021-2026: Getting the best from our Land, Paper SG/2021/81. Edinburgh: Scottish Government.
Seidl, R., Thom, D., Kautz, M., Martin-Benito, D., Ascoli, D., Petr, M., et al. (2017). Forest disturbances under climate change. Nat. Climate Change 7, 395–402. doi: 10.1038/nclimate3303
Serrano-León, H., Ahtikoski, A., Sonesson, J., Fady, B., and Lindner, M. (2021). From genetic gain to economic gain: simulated growth and financial performance of genetically improved Pinus sylvestris and Pinus pinaster planted stands in France. Finland and Sweden. Forestry 94, 512–525. doi: 10.1093/forestry/cpab004
Silva, C., Holmberg, G., Turok, J., Stover, D., and Horst, A. (2018). EIP-AGRI Forest Practices & Climate Change -Minipaper 4. Brussels: Climate smart silviculture & genetic resources.
Sing, L., Metzger, M. J., Paterson, J. S., and Ray, D. (2018). A review of the effects of forest management intensity on ecosystem services for northern European temperate forests with a focus on the UK. Forestry 91, 151–164. doi: 10.1093/forestry/cpx042
Skrøppa, T., and Steffenrem, A. (2021). Performance and phenotypic stability of Norway spruce provenances, families, and clones growing under diverse climatic conditions in four Nordic countries. Forests 12, 1–15. doi: 10.3390/f12020230
Spake, R., Bellamy, C., Gill, R., Watts, K., Wilson, T., Ditchburn, B., et al. (2020). Forest damage by deer depends on cross-scale interactions between climate, deer density and landscape structure. J. Appl. Ecol. 57, 1376–1390. doi: 10.1111/1365-2664.13622
Summers, R., and Cavers, S. (2021). The past, present and uncertain future for caledonian pinewoods. Scottish Forestry 75, 19–28.
Svystun, T., Lundströmer, J., Berlin, M., Westin, J., and Jönsson, A. M. (2021). Model analysis of temperature impact on the Norway spruce provenance specific bud burst and associated risk of frost damage. Forest Ecol. Manag. 493:119252. doi: 10.1016/j.foreco.2021.119252
Thuiller, W. (2003). BIOMOD - optimizing predictions of species distributions and projecting potential future shifts under global change. Global Change Biol. 9, 1353–1362. doi: 10.1111/gcb.12728
Vizcaíno-Palomar, N., Fady, B., Alía, R., Raffin, A., Mutke, S., and Benito-Garzón, M. (2020). The legacy of climate variability over the last century on populations’ phenotypic variation in tree height. Sci. Total Environ. 749:2022. doi: 10.1016/j.scitotenv.2020.141454
Waterworth, R. M., Richards, G. P., Brack, C. L., and Evans, D. M. W. (2012). A generalised hybrid process-empirical model for predicting plantation forest growth. Forest Ecol. Manag. 238, 231–243. doi: 10.1186/1471-2164-9-57
Watts, K., Whytock, R. C., Park, K. J., Fuentes-Montemayor, E., Macgregor, N. A., Duffield, S., et al. (2020). Ecological time lags and the journey towards conservation success. Nat. Ecol. Evol. 4, 304–311. doi: 10.1038/s41559-019-1087-8
West-Eberhard, M. J. (2003). Developmental Plasticity and Evolution. Oxford: Oxford University Press.
Williams, A. P., Allen, C. D., Macalady, A. K., Griffin, D., Woodhouse, C. A., Meko, D. M., et al. (2013). Temperature as a potent driver of regional forest drought stress and tree mortality. Nat. Climate Change 3, 292–297. doi: 10.1038/nclimate1693
Yousefpour, R., Jacobsen, J., Thorsen, B. L., Meilby, H., Hanewinkel, M., and Oehler, K. (2012). A review of decision-making approaches to handle uncertainty and risk in adaptive forest management under climate change. Ann. Forest Sci. 69, 1–15. doi: 10.1007/s13595-011-0153-4
Keywords: assisted translocation, natural colonisation, forest reproductive material (FRM), climate portal, genomic selection (GS), genome-wide association studies (GWAS), trade-offs
Citation: Ray D, Berlin M, Alia R, Sanchez L, Hynynen J, González-Martinez S and Bastien C (2022) Transformative changes in tree breeding for resilient forest restoration. Front. For. Glob. Change 5:1005761. doi: 10.3389/ffgc.2022.1005761
Received: 28 July 2022; Accepted: 20 September 2022;
Published: 10 October 2022.
Edited by:
Leland Kendall Werden, ETH Zürich, SwitzerlandReviewed by:
Luis Mijangos, University of Canberra, AustraliaCarolyn Pike, USDA Forest Service, United States
Copyright © 2022 Ray, Berlin, Alia, Sanchez, Hynynen, González-Martinez and Bastien. This is an open-access article distributed under the terms of the Creative Commons Attribution License (CC BY). The use, distribution or reproduction in other forums is permitted, provided the original author(s) and the copyright owner(s) are credited and that the original publication in this journal is cited, in accordance with accepted academic practice. No use, distribution or reproduction is permitted which does not comply with these terms.
*Correspondence: Duncan Ray, ZHVuY2FuLnJheUBmb3Jlc3RyZXNlYXJjaC5nb3YudWs=