- 1Department of Physics, Earth Science and Space Systems Engineering, Morehead State University, Morehead, KY, United States
- 2Laboratorio de Geología de Llanuras, Facultad de Ciencia y Tecnología, Universidad Autónoma de Entre Ríos, Sede Diamante, CICyTTP (CONICET-UADER-Prov.ER), Buenos Aires, Argentina
- 3Department of Geography and Environmental Sciences, Northumbria University, Newcastle upon Tyne, United Kingdom
- 4Department of Biology and Chemistry, Morehead State University, Morehead, KY, United States
- 5Craft Academy for Excellence in Science and Mathematics, Morehead State University, Morehead, KY, United States
- 6United States Geological Survey, Reston, VA, United States
- 7Center for Excellence in Palynology, Department of Geology & Geophysics, Museum of Natural Science, Louisiana State University, Baton Rouge, LA, United States
The middle Miocene Climate Optimum (MMCO) was the warmest interval of the last 23 million years and is one of the best analogs for proposed future climate change scenarios. Fungi play a key role in the terrestrial carbon cycle as dominant decomposers of plant debris, and through their interactions with plants and other organisms as symbionts, parasites, and endobionts. Thus, their study in the fossil record, especially during the MMCO, is essential to better understand biodiversity changes and terrestrial carbon cycle dynamics in past analogous environments, as well as to model future ecological and climatic scenarios. The fossil record also offers a unique long-term, large-scale dataset to evaluate fungal assemblage dynamics across long temporal and spatial scales, providing a better understanding of how ecological factors influenced assemblage development through time. In this study, we assessed the fungal diversity and community composition recorded in two geological sections from the middle Miocene from the coal mines of Thailand and Slovakia. We used presence-absence data to quantify the fungal diversity of each locality. Spores and other fungal remains were identified to modern taxa whenever possible; laboratory codes and fossil names were used when this correlation was not possible. This study represents the first of its kind for Thailand, and it expands existing work from Slovakia. Our results indicate a total of 281 morphotaxa. This work will allow us to use modern ecological data to make inferences about ecosystem characteristics and community dynamics for the studied regions. It opens new horizons for the study of past fungal diversity based on modern fungal ecological analyses. It also sheds light on how global variations in fungal species richness and community composition were affected by different climatic conditions and under rapid increases of temperature in the past to make inferences for the near climatic future.
Introduction
Fungi play key roles in the forest environment and are essential drivers of nutrient cycling in peat-producing forested wetlands (Heitman et al., 2018). Very little is known about how forested wetland fungal communities will change in relation to forecasted warming scenarios. Predictions of this type require large-scale, long-term datasets which do not presently exist (Willis (ed.), 2018). While the needed time to produce such datasets is scarce, the paleo-sciences provide a lens with which we can build predictive models using fossil fungal assemblages. These assemblages occur in two major forms (Taylor et al., 2015): macrofossils, which may preserve significant portions of the fungal organism in exquisite detail, and microfossils, often fragmented or representative of a single phase of the life cycle of fungus, typically the spore, including the sporangiospore, conidium, ascospore, and basidiospore, or other structures such as hyphae, conidiophores, rhizoids, etc.
Fungal microfossils are generally studied under the larger discipline of palynology. They are classified as non-pollen palynomorphs (NPPs), which have increasingly been used as paleoecological indicators in both Quaternary and deeper time studies (van Geel and Aptroot, 2006; Nuñez Otaño et al., 2021; Shumilovskikh et al., 2021; among others). Historically, fossil fungi in deep-time have been identified using an artificial classification scheme based on morphological descriptions that have little to no correlation with modern taxa (O’Keefe et al., 2021). This classification makes it impossible to apply the Coexistence Approach to fossil fungi (Utescher et al., 2014; Grimm and Potts, 2016), which is widely used with other palynomorphs to elucidate ancient climates. Recent advances in training and collaborations with modern mycologists are permitting the discipline to move past this limitation and to recognize members of modern families and genera in the fossil record (Nuñez Otaño et al., 2017, 2021; O’Keefe, 2017; Shumilovskikh et al., 2017; Pound et al., 2019; Bianchinotti et al., 2020; Loughlin et al., 2021).
The FiaWW (Fungi in a Warmer World) project is one such collaboration, bringing together palynologists, mycologists, and climate scientists. It has turned its lens upon the middle Miocene Climatic Optimum (MMCO), the warmest interval of the last 23 million years and an internationally recognized possible analog for the Intergovernmental Panel on Climate Change -Representative Concentration Pathway (IPCC-RCP) 4.5–8.5 warming scenarios, both in terms of intensity and rapidity of warming and in terms of latitudinal effects (Intergovernmental Panel on Climate Change [IPCC], 2014, 2021; Steinthorsdottir et al., 2021). Using fungal palynology, we aim to construct the first large-scale, long-term fungal diversity and worldwide distribution dataset of its kind to build a predictive model for fungal assemblage behavior under future warming scenarios.
As an initial test of the feasibility of the project, we examined low-rank coal samples from two regions, west-central Slovakia and northern Thailand, for fungal palynomorph content. Low-rank coals were chosen specifically for this initial test of concept to (1) control for depositional environment (O’Keefe et al., 2013; Dai et al., 2020), (2) minimize the effects of transport, as organic matter preserved in both systems is predominantly autochthonous (produced from standing biomass and preserved in situ) (O’Keefe et al., 2011; Belkin et al., 2013), and (3) minimize the impact of coalification on the fungal material (Dai et al., 2017, 2020). The two regions presently fall into warm-summer and humid continental climate (Slovakia), as well as tropical wet and dry climate (Thailand) classifications. They appear to have similar climatological settings at the onset of middle Miocene warming (Steinthorsdottir et al., 2021), and warmed unevenly, with temperate Slovakia experiencing relatively greater warming than tropical Thailand, as is anticipated for future warming scenarios (Intergovernmental Panel on Climate Change [IPCC], 2014, 2021). Of note, coals from all samples have similar organic and geochemical compositions and are interpreted as having been deposited in a mosaic of topogenous and ombrogenous mire settings (O’Keefe et al., 2011; Belkin et al., 2013). The topogenous regions are dominated by coniferous trees from the family Cupressaceae and aquatic to emergent herbaceous plants, including Haloragaceae, Cyperaceae, and Poaceae, while the ombrogenous regions are a mixture of hardwood and softwood trees and shrubs with an understory of ferns, grasses, and sedges (Songtham et al., 2005; Kučerová, 2010; Sepulchre et al., 2010; Hudáčková et al., 2020).
Preliminary studies of fungal remains preserved in the coals from Slovakia were carried out by O’Keefe and Hower in the period 2007–2011 (O’Keefe et al., 2011). These studies report only 23 morphotypes for the Slovakian material (O’Keefe et al., 2011). For Thailand, there are no previous studies about the fossil fungal assemblages during the middle Miocene. Here, we present a re-study of the Slovakian material and a first study from coals from Thailand to expand the fossil fungi record in both regions. This study was designed to assess fungal communities and paleoenvironments from the middle Miocene between Thailand and Slovakia. We also wanted to test if fungi were more abundant and diverse in the tropics than in the temperate regions during this period (Hyde et al., 2004; Tedersoo et al., 2014; Chaudhary et al., 2018; among others) or if its pattern of distribution differed from those from plants and other organisms (Rudolph et al., 2018; Větrovský et al., 2020). This study will provide a foundation for the main goal of the FiaWW project, which is MMCO climate reconstruction using fungi as reliable proxies.
Materials and Methods
Study Area
We selected a total of 13 samples from the lower to middle Miocene, including the MMCO, five from Thailand and eight from Slovakia. These are part of previous collaborative research with the United States Geological Survey during the periods 2000–2005 and 2010–2015. Samples from Thailand are from multiple mines in the northern part of the country, while samples from Slovakia are from the west-central portion of the country (Figure 1 and Table 1).
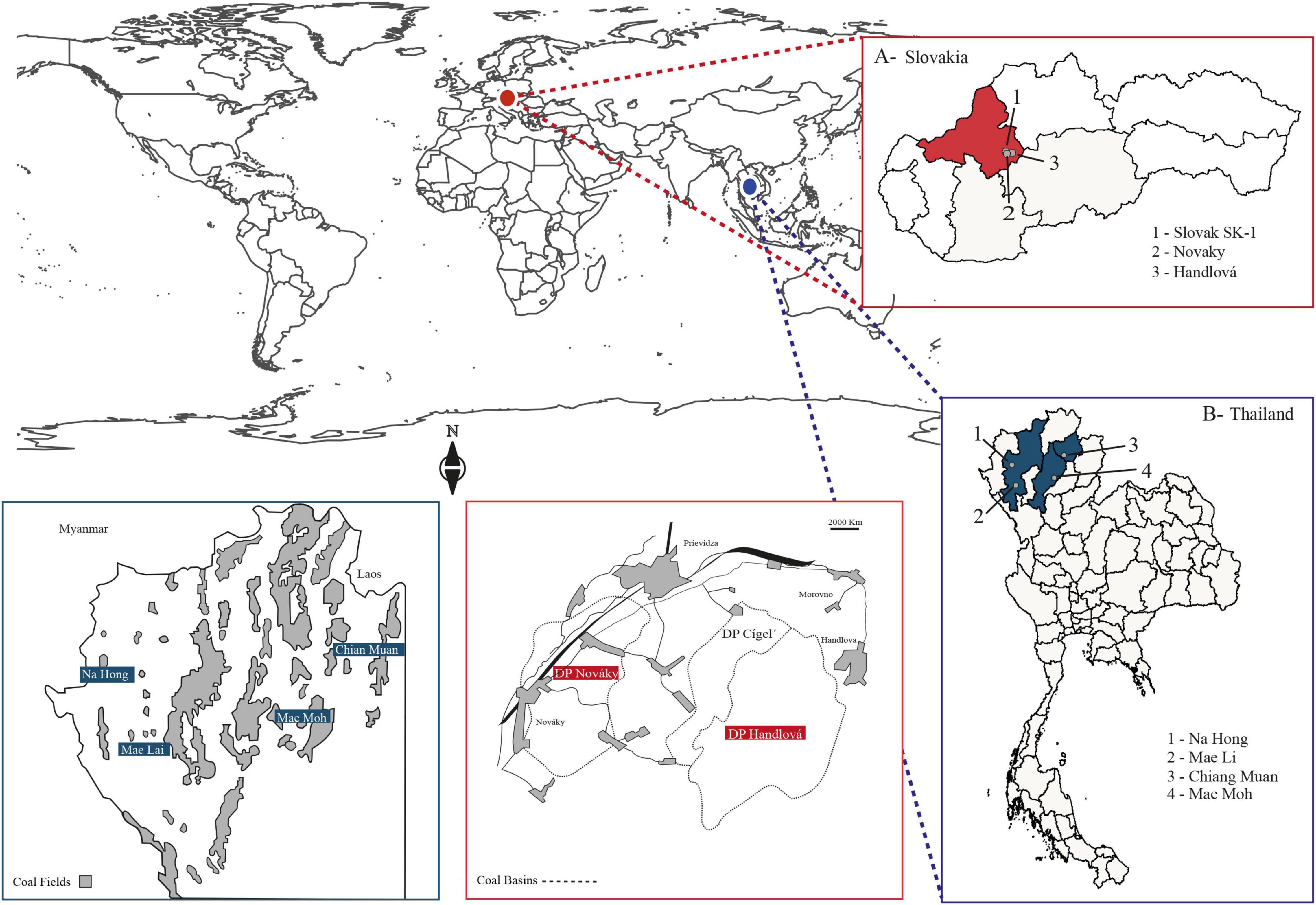
Figure 1. Maps of study areas indicate the regions in Slovakia (red) and Thailand (blue) where the coalfields are located. The gray points indicate the coal basins where the samples for this study were extracted. The regional maps indicating the coal fields and coal basins from Thailand and Slovakia were redrawn and modified from Machajová et al. (2000) and Ratanasthien (2011).
Thailand Stratigraphy
The regional structure of the geology of Thailand is complicated because the basin fill was generally deposited in variably subsiding rift basins and neotectonic overprints (Morley and Racey, 2011). Basin fills represent a mosaic of lowland environments developed atop basement rock and basin margin conglomeratic alluvial fans. Thus, rocks deposited in these environments range from fluvial sandstones with associated finer-grained overbank deposits to lacustrine mudstones and oil shales, and to mire-associated claystones and lignitic coal seams (Morley and Racey, 2011; Friederich et al., 2016). Considering that many of the basins in Thailand are both sources of coal and hydrocarbons and paleontologically important, significant data exists on intra-basin stratigraphy, biostratigraphy, magnetostratigraphy, and plant- and animal-based paleoecology (Benammi et al., 2002, 2010; Ratanasthien, 2002; Songtham et al., 2005; Ratanasthien et al., 2008; Thasod et al., 2008; Coster et al., 2010; Sepulchre et al., 2010; Friederich et al., 2016; Grohé et al., 2020; among others). This makes it possible to organize individual coal seams that occur within the basins by age. The lignites selected for this study were from the coalfields of Mae Moh, Nae Hong, Mae Lai, and Chiang Muan (Figure 1), and were deposited in the lower and middle Miocene (Figure 2; Tewalt et al., 2005; Belkin et al., 2013; World Coal Quality Inventory, 2019).
Slovakia Stratigraphy
Slovakian lignites examined for this study are from the Novaky and Handlova regions of the Upper Nitra Basin in the Carpathians (Figure 1) (Hók et al., 1995; Dill et al., 2008). Coals in this region were deposited during the middle Miocene (Figure 2; Sitár et al., 1987; Planderová, 1991). Like those in Thailand, the coals studied in Slovakia developed in a pull-apart basin produced during extensional tectonics (Hók et al., 1995; Dill et al., 2008). The Upper Nitra Basin is among the smaller basins bounded by normal faults in the western Carpathians (Hók et al., 1995), and has been a target of coal, oil and gas exploration and production. Interestingly, the stratigraphy within the two coal mining regions, which occur in the Kamanec and Nováky Formations, is not well understood (Machajová et al., 2002; Dill et al., 2008). Most authors agree with Hudáček et al. (1982) that the main seam (Hlavný slaj) lignites mined near Nováky and Handlová are probably the same seam, although little evidence is presented to support this, beyond the general stratigraphy of both mining areas. As in Thailand, the seams are developed in a mosaic of terrestrial siliciclastic environments in the Kamenec and Nováky Formations, ranging from fluvial-alluvial to lacustrine in internally-draining grabens with coarse-grained alluvial fans along the margins (Čechovič, 1950). The majority of sediment sources appear to be weathered extrusive igneous rocks from the adjacent proto-Carpathians (Čechovič, 1950; Machajová et al., 2000). The lowermost lignite in the region (Hlboký slaj and Novaký), as noted by Hudáček et al. (1982), is tentatively correlated with the “Alive” member sample SK-1 in our studied set; the majority of samples, however, come from the main seam (Hlavný slaj, Handlová produktívne súvrstvie; Novaký Fm.) or its underlier, the Podlozne slaj (Figure 2; Hudáček et al., 1982; Machajová et al., 2000, 2002). In the Handlova mining area, the main seam has two splits that are most greatly separated (by up to 50 m) in the eastern and northeastern portion of the area by a siliciclastic parting that tapers to nothing in the W and SW portion of the mining area (Machajová et al., 2000). The coal zone is overlain by dark gray fossil plant-bearing clays of the Serravallian-age (late Badenian) Koš Formation (Kučerová, 2010).
Paleoclimate
Plant-based reconstructions from coal basins in northern Thailand have indicated a transition from warm-temperate climates in the early Burgidalian (Chiang Mai lignites) to tropical climates by the late Burgidalian-early Langhian (Hod lignites) that largely persisted through the early middle and upper middle Miocene (Ratanasthien et al., 2008; Morley and Racey, 2011). It has been suggested that these changes coincide with increasing paleotopographic relief and intensification of the SE Asian monsoon (Morley, 2012; Steinthorsdottir et al., 2021).
In west-central Slovakia, Miocene paleobotanical reconstruction indicate that tropical to sub-tropical conditions gave way to highly seasonal warm-temperate to temperate conditions by the late Langhian, with few thermophilus plant taxa retained, even in coal-forming basins (Kvaček et al., 2006). During this time, paleotopographic relief first increased, then decreased slightly in relation to tectonism associated with development of the western Alps and Carpathians (Kvaček, 1994; Steinthorsdottir et al., 2021).
Palynology Methods
We extracted fungal palynomorphs using HF-free protocols outlined for low-rank coal in O’Keefe and Eble (2012); Pound et al. (2021), and Riding (2021). These consisted of crushing the coal to −1 mm, disaggregation in non-phosphatic anionic detergent (Liquinox®), a treatment with hypochlorous acid to de-polymerize lignin, and a treatment with 5% KOH to produce water-soluble organic salts. The salts were then rinsed away before to subject the samples to heavy-density separation using LST® Heavy Liquid (a proprietary lithium-containing heteropolytungstate compound). After this, they were placed in 95% ethanol prior to mounting. We mounted individual coverslips of the palynological residues in polyvinyl alcohol (PVA). We examined the specimens on Leitz and Leica microscopes with variable magnifications from 10 to 1,000×. We obtained z-stack photomicrographs at 1,000× magnification, primarily with Leica ICC50W cameras and using the Leica Application Suite® (LAS) software. We then z-stacked the multiple focal planes using Helicon Focus® software. We imaged a minimum of one fungal palynomorph for each morphotype recorded, and more than five when possible. Fungal palynomorphs were consecutively numbered using the acronym for the laboratory name, OPaL # (O’Keefe Paleoecology Lab).
Fungal Identification
We separated the morphotaxa using the following criteria: shape, ornamentation, color, color variation, number and type of apertures, and/or attachment scars (Hughes, 1953; Pirozynski and Weresub, 1979; Kalgutkar and Jansonius, 2000; Gulis et al., 2005; Seifert et al., 2011; Guarro et al., 2012; Nuñez Otaño et al., 2021). When possible, we identified these taxa to family or genus using the above references and primary literature for each modern taxon (Supplementary Table 1). Ecological information was obtained using available literature about each taxon. FunGuild (a Python-based tool) was used for ecological guild data (Nguyen et al., 2016; Supplementary Table 2) and biogeographic distribution was complemented with Global Fungi database (2021; Větrovský et al., 2020).
Data Analysis
We used presence/absence data to record the diversity observed between Thailand and Slovakia and among samples. We did a boxplot diagram and a Kruskal–Wallis test to compare richness between countries. This test compares two groups without assuming them to follow a normal distribution (McDonald, 2014). The morphotypes were first separated into 10 categories based on shape variation (Seifert and Gams, 2011; Nuñez Otaño et al., 2021). The morphological categories identified were: amerospore, didymospore, dictyospore, phragmospore, bulbil-like spore, epiphyllous structures, clathrate spore, helicospore, staurospore, and indeterminate fungal remains. We calculated the percentage of each morphological category in each sample and plotted them in bar plots to show morphological variation among the samples for both countries.
We used the Shannon–Wiener Index (H′) to calculate α-diversity to examine variation among samples and between sites based on morphological variability. For the indices, we used the morphological categories as taxa and the number of specimens per group in each sample was used as the total number of individuals. We used principal component analysis (PCA) to compare morphology-environment variation (Nuñez Otaño et al., 2021) among samples within localities and between localities. Although the data collected were presence/absence, the analysis was applied to the morphological groups, and PCA was selected because it calculates Euclidean distances between samples (Legendre and Legendre, 2012). We also calculated all the pairwise similarities of our PCA using Euclidean distance to compare the samples. In addition, we completed a separate PCA that excluded amerospores to evaluate how the distances vary in the absence of this group. This is justifiable because the majority of amerospores do not possess sufficient spore traits to permit unambiguous identification in the absence of the rest of the fungus, thus obscures signals in the data in much the same way as widely-disseminated anemophilous pollen (Birks and Birks, 1980). The analyses were carried out in R (R Core Team, 2021).
Pollen-Based Climatological Signals
For a better interpretation of the fossil fungal assemblages found in our samples, we ran an additional analysis using CREST (Climate Reconstruction Software) (Chevalier et al., 2014) to reconstruct terrestrial paleoclimates of the study areas using published palynological records. CREST has been shown to provide suitable quantification of error in middle Miocene terrestrial climate reconstructions and is consistent with Co-existence Approach (Utescher et al., 2014) reconstructions from northwest Europe (Gibson et al., in review). We extracted taxa lists and nearest living relatives from the literature and used as the input for CREST, obtained from Nováky (Kučerová, 2010) and the Novohrad-Nógrád Basin (Hudáčková et al., 2020) for Slovakia and Mae Moh (Songtham et al., 2005) and Chiang Muan (Sepulchre et al., 2010) for Thailand. CREST reconstructions were carried out in R (R Core Team, 2021). Five climate variables were assessed in detail: Mean Annual Temperature (MAT), Mean Temperature of Warmest Quarter (MTWQ), Mean Temperature of Coldest Quarter (MTCQ), Mean Annual Precipitation (MAP), and Precipitation Seasonality (CoV x100). The CREST output provides 50 and 95% (2-σ) uncertainties as well as an optimum. Here we focus on the optima and 95% confidence interval for each variable. All analyses were carried out in R (R Core Team, 2021).
Results
Fossil Fungi
A total of 637 fungal spore specimens were recorded in the samples from Thailand and Slovakia (Supplementary Table 1 and Figures 3–5). These were grouped into 281 morphotaxa, based on detailed morphological comparisons, of which 151 were found only in Thailand, 93 only in Slovakia, and 37 in both Thailand and Slovakia. The fungal diversity of the samples is mostly represented by Ascomycota, but we also found few spores from other phyla, such as Basidiomycota (OPAL 219) and Glomeromycota (OPAL 226) (Figures 3–5). Overall, richness between Thailand and Slovakia is different (Kruskal–Wallis test, P = 0.02), in which the samples from Thailand had a higher number of morphotaxa (mean: 48.8; SD: 16.8) than Slovakia (mean: 21.75, SD: 14.8) (Figure 6). Amerospores and phragmospores were present in all samples, except sample 7 (Figure 7). Reviewing the variation of abundances, amerospores were the dominant group in most of the samples from both countries (Figure 7). In Thailand, the dominance of amero-morphotypes varied from 32% (sample 457; Figure 7A) to 75% (sample 454; Figure 7A), while in Slovakia varied from 45.2% (sample 3; Figure 7B) to 100% in (sample 7; Figure 7B). However, sample 7 was the sample with the lower record of fungi spores, in which only 3 spores count for the 100% of amerospores present in this sample.
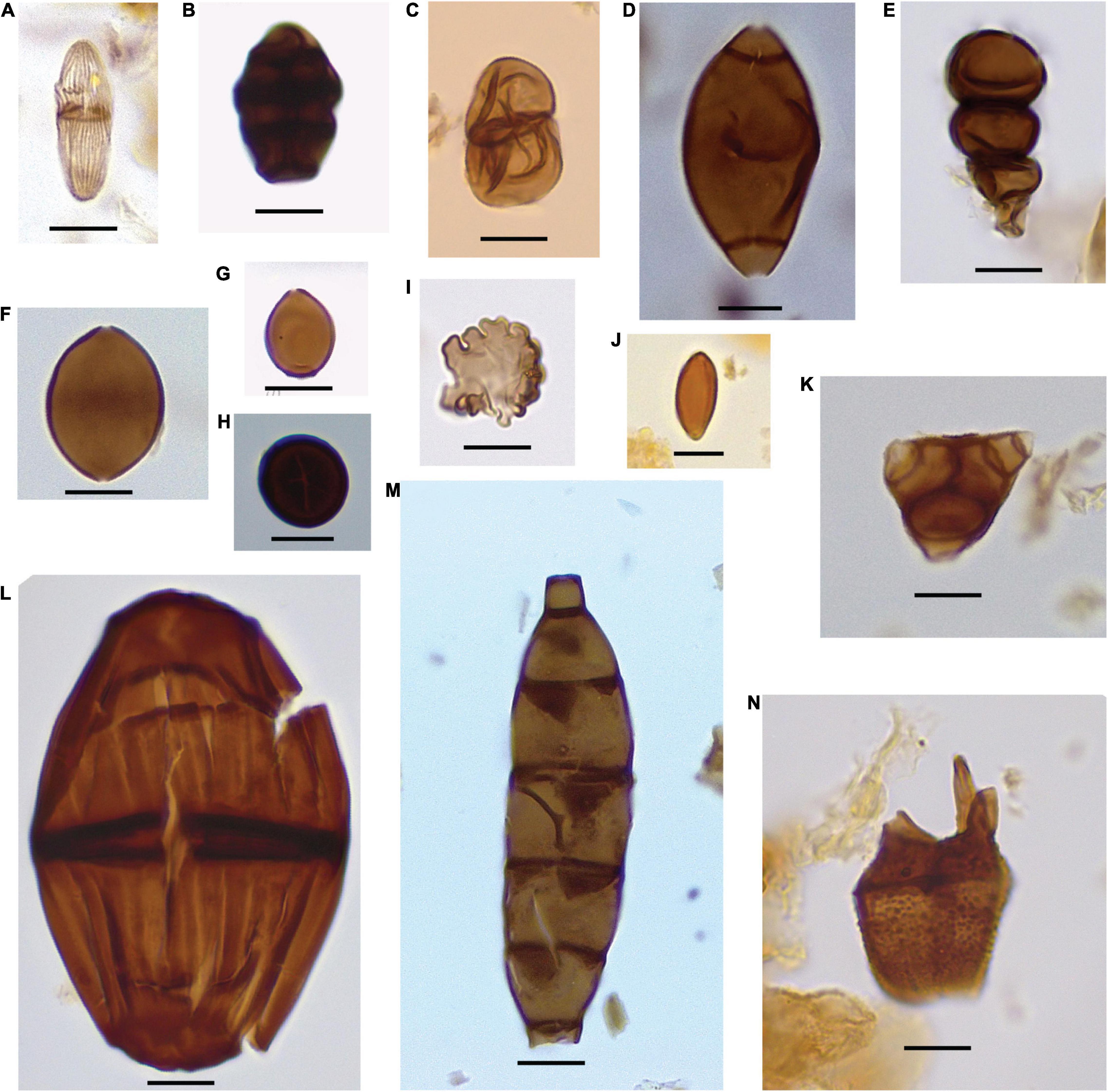
Figure 3. Fungal spores found in Thailand. Each photograph indicates: identification if any, the sample number, morphotype number (OPaL #), and England Finder coordinates (EF). (A) Arecophila sp., sample 457, OPaL 38, EF: N49-3; (B) cf. Canalisporium sp. 2, sample 455, OPaL 140, EF: P26-1; (C) cf. Asterina, samples 453, OPaL 125, EF: Q32-3; (D) Diporotheca, sample 457, OPaL 141, EF: Y35-2; (E) sample 453, OPaL 106, EF: U38-1_T38-3; (F) cf. Chaetomium, sample 457, OPaL 87, EF: N50-2; (G) cf. Cercophora, sample 455, OPaL 92, EF: F24-4; (H) sample 456, OPaL 33, EF: S26; (I) Thyriothecium propagules (Germling), sample 454, OPaL 102, EF: K37-2; (J) sample 453, OPaL 88, EF: G35-2_F35-4; (K) Oncopodiella aff. trigonella, sample 454, OPaL 96, EF: L26-4; (L) Megalohypha aff. aqua-dulces, sample 453, OPaL 97, EF: T37-4; (M) cf. Xylomyces sp., sample 455, OPaL 152, EF: W24-2; (N) Tetraploa, sample454, OPaL 98, EF: AA34. Scale bar: 10 μm. More information of each specimen in Supplementary Table 1.
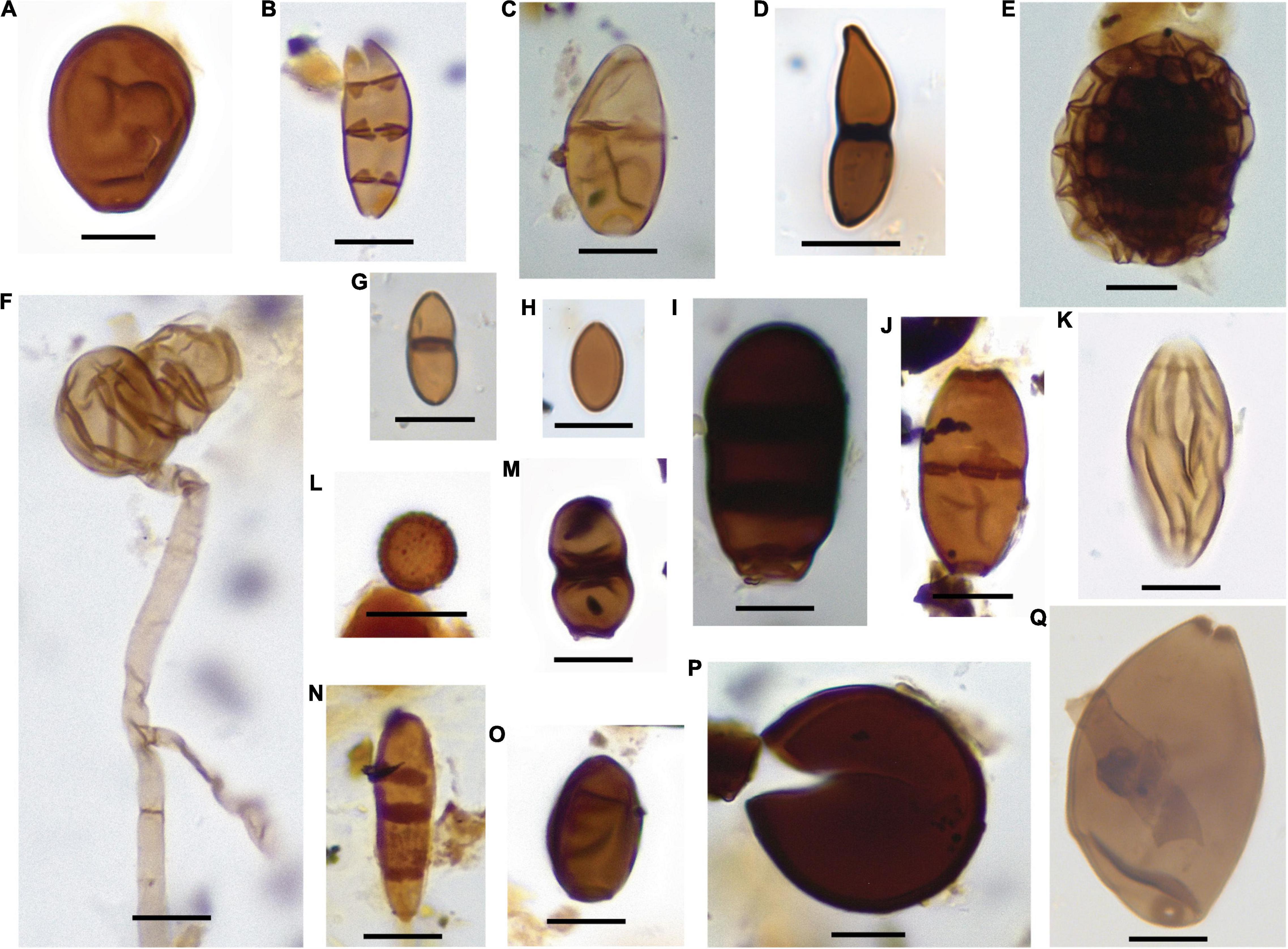
Figure 4. Fungal spores found in Slovakia. Each photograph indicates: identification if any, the sample number, morphotype number (OPaL #), and England Finder coordinates (EF). (A) sample 10, OPaL 77, EF: Q49-2; (B) sample 10, OPaL 227, EF: T39-3; (C) sample 3, OPaL 243, EF: J69-1; (D) Jahnula, sample 4, OPaL 278, EF: F-33; (E) cf. Dictyosporium, sample 4, OPaL 274, EF: O41; (F) Glomeraceae (cf. Glomus), sample 10, OPaL 226, EF: X46-1; (G) cf. Asterina, sample 4, OPaL 229, EF: D34; (H) sample 6, OPaL 78, EF: N70-1; (I) sample 3, OPaL 250, EF E65-4; (J) Ascotaiwania aff. limnetica, sample 10, OPaL 114, EF: N39-3; (K) Diporotheca, sample 5, OPaL 141, EF: M38; (L) cf. Tulostoma, sample 10, OPaL 209, EF: N40-4; (M) sample 10, OPaL 230, EF: 31-4; (N) sample 3, OPaL 233, EF: W42-4; (O) sample 10, OPaL 195, EF: C46-2-4; (P) sample 3, OPaL 250, EF: W41-2; (Q) sample 4, OPaL 194, EF: V32-3. Scale bar: 10 μm. More information of each specimen in Supplementary Table 1.
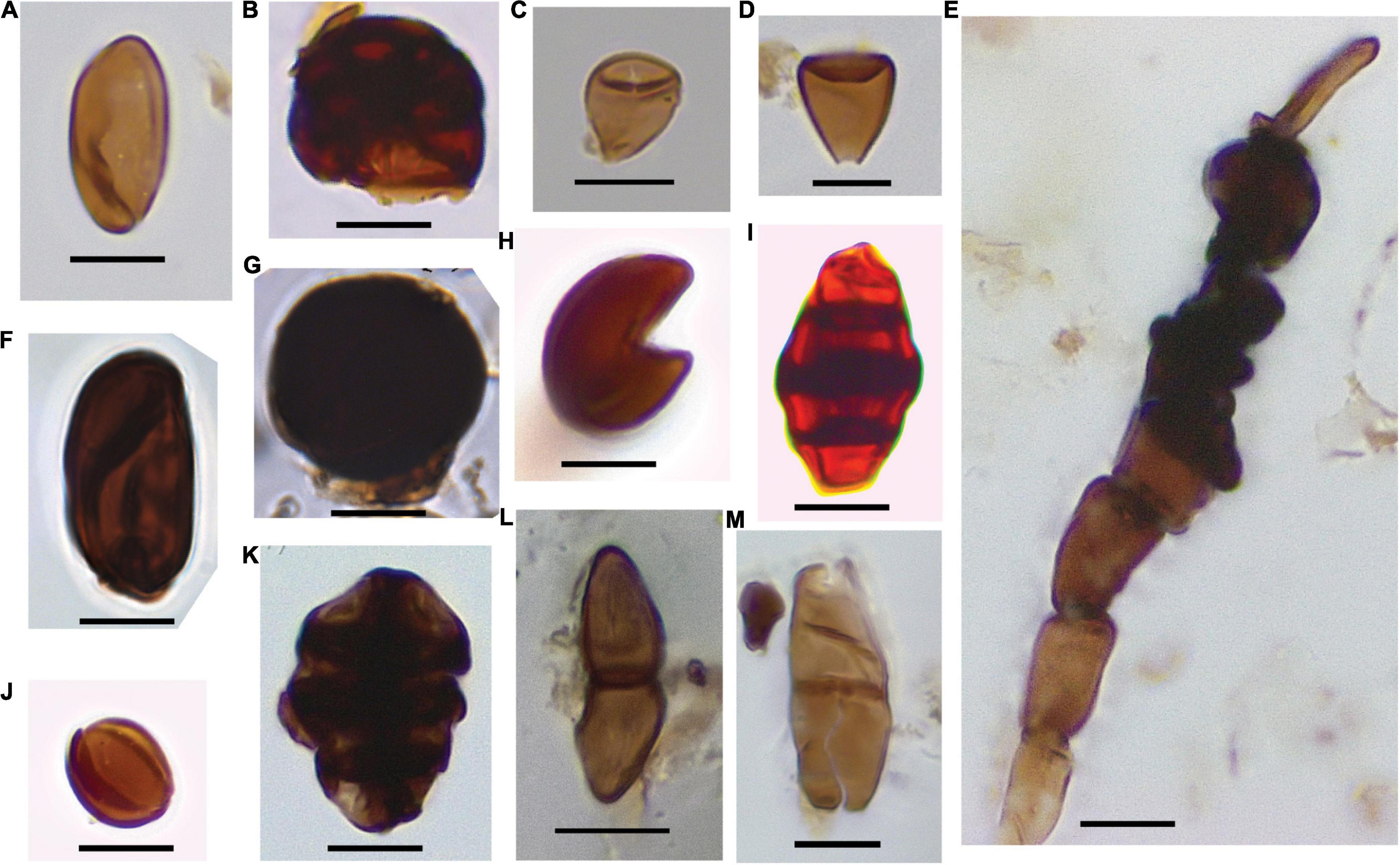
Figure 5. Other fungal spores identified in Thailand and Slovakia. Each photograph indicates: identification if any, the sample number, morphotype number (OPaL #), and England Finder coordinates (EF). (A) cf. Rosellinia, sample 454, OPaL 40, EF: Z29; (B) cf. Berkleasmium, sample 456, OPaL 223, EF: H27; (C) cf. Catenularia sp., sample 454, OPaL 93, EF: F34-1; (D) Catenularia aff. catenulata, sample 454, OPaL 281, EF: Y31-3; (E) Phaeosclera-type, sample 453, OPaL 95, Z48; (F) cf. Ascobolaceae, sample 454, OPaL 39, EF: O26-4; (G) cf. Monotosporella, sample 8, OPaL 18, J24; (H) cf. Arthrinium, sample 455, OPaL 36, EF: R25-2; (I) Phragmocephala, sample 455, OPaL 134, EF: S25-3; (J) Apiosporaceae, sample 455, OPaL 33, V25-4; (K) cf. Canalisporium sp. 1, sample 456, OPaL 140, EF: E26-4; (L) cf. Delitschia, sample 3, OPaL 224, EF: V44-3; (M) Savoryella aff. fusiformis, sample 3, OPaL 124, EF: Q43-2. Scale bar: 10 μm. More information of each specimen in Supplementary Table 1.
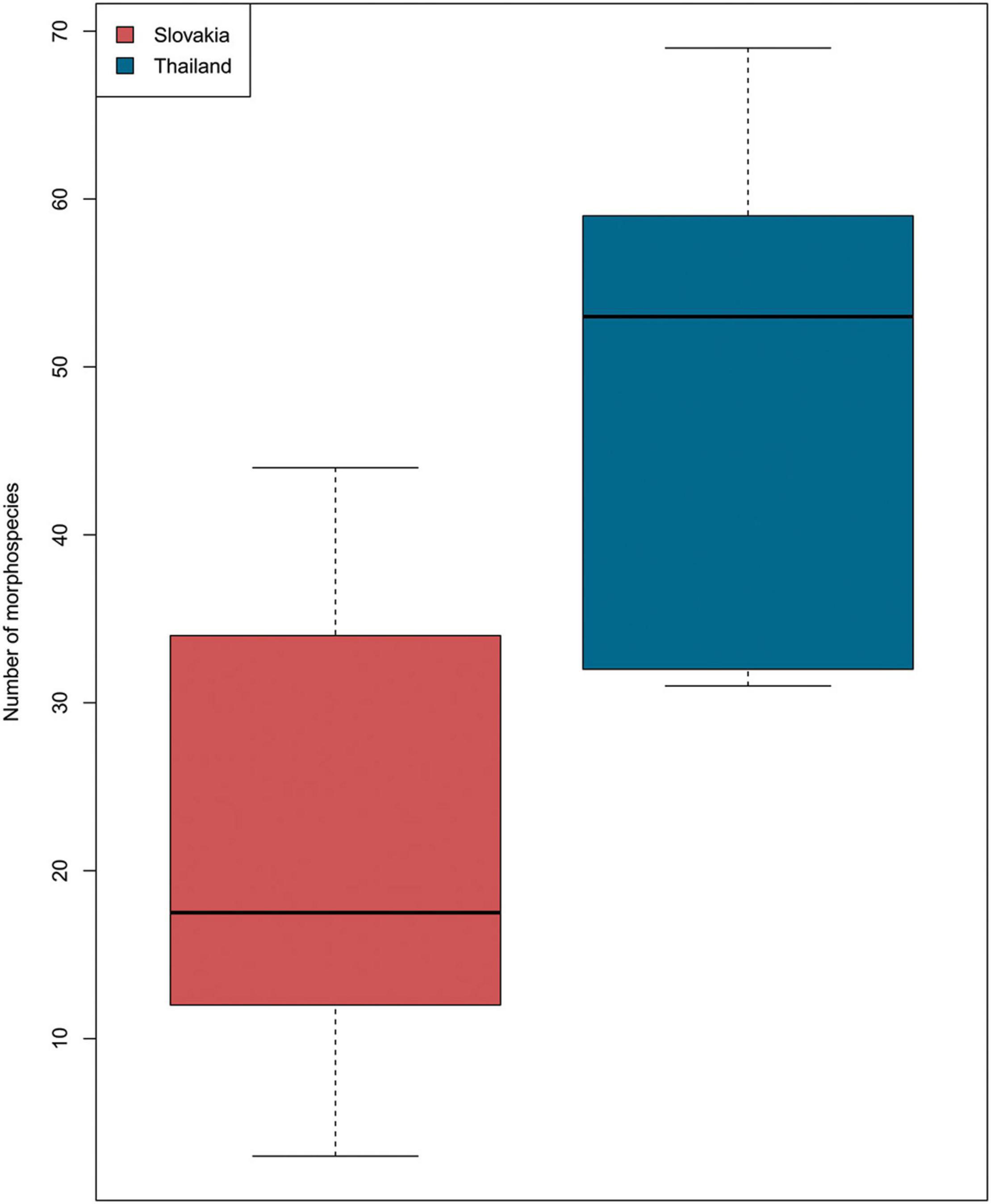
Figure 6. Boxplot indicates the number of morphotaxa for Slovakia (red) and Thailand (blue). Kruskal–Wallis chi-squared = 5.4857, df = 1, p-value = 0.01917.
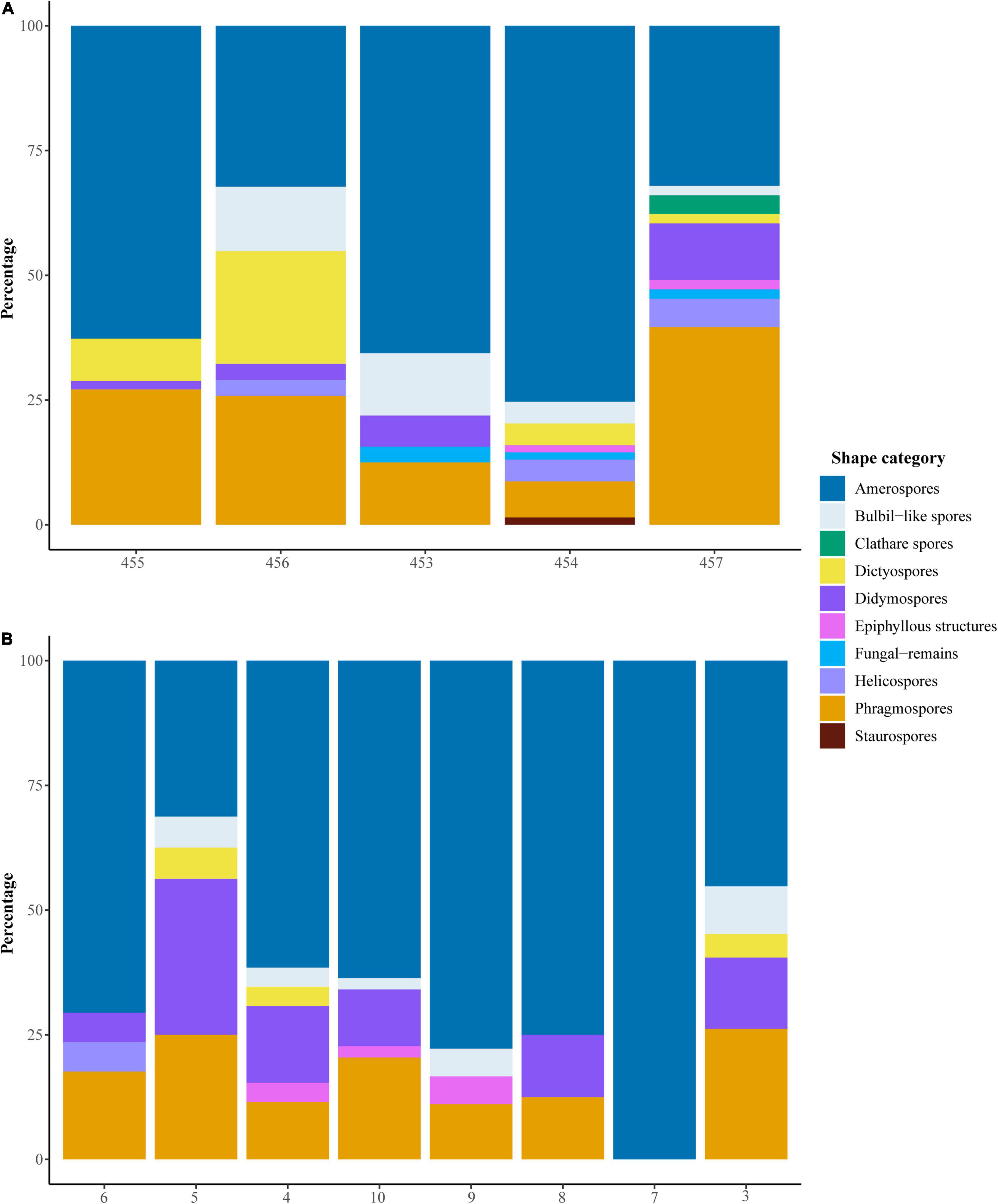
Figure 7. Bar plots show the percentage of each morphological group per sample. The samples are organized in chronological order from young (left) to old (right), refer to Figure 2. (A) Samples from Thailand, (B) Samples from Slovakia.
In the PCA of all morphologies, the two first axes explained 95% of the variation (Figure 8 and Supplementary Table 3). In Axis 1, amerospores drive the variation of the samples, especially sample 454 from Thailand, while in Axis 2, phragmospores drive this variation, especially sample 457 from Thailand (Figure 8). Most of the Slovakian samples were grouped close together, except for samples 3 and 10, while the samples from Thailand were more dispersed (Figure 8).
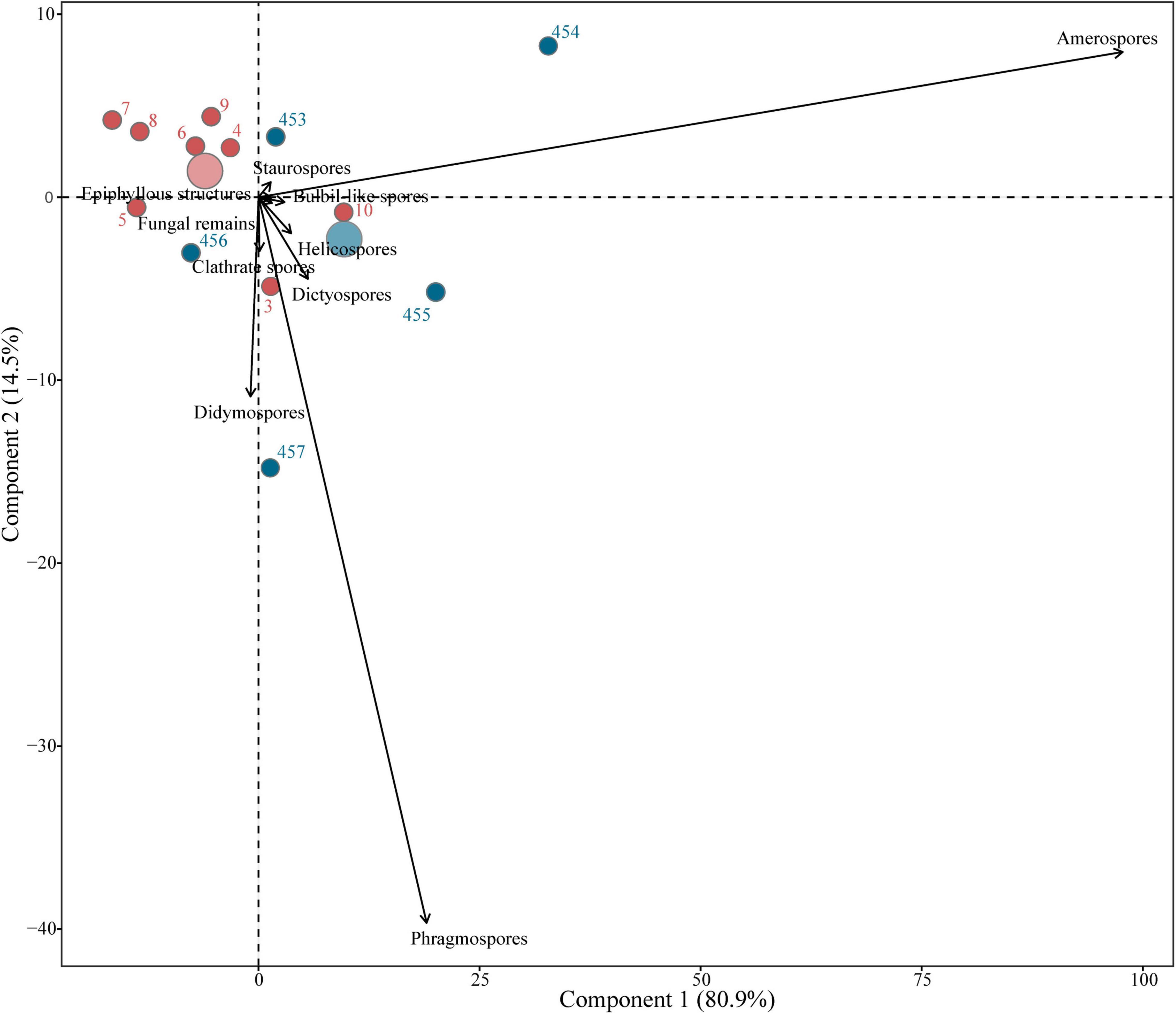
Figure 8. Principal component analysis (PCA), including all morphological groups, compares the variation between and within samples from Thailand and Slovakia. Big circles indicate the midpoint of each group of samples, separating Thailand (blue) and Slovakia (red).
When amerospores were excluded from the PCA, there was a clear separation of the samples by country and within samples depending on the fungal community composition and based on morphotypes and fungal spores identified (Figure 9 and Supplementary Table 2). We also observed that the samples were grouped in a temporal scale, in which 457, from the lower Miocene from Thailand, was different from most of the samples (Figure 2 and Supplementary Table 3). Samples 453 (13.5–16 My) and 456 (13.3–13.1 My), from Thailand, clustered together in the PCA (Figure 9). These samples are temporally close, and their fungal composition suggest similar environments (Figure 2 and Supplementary Table 2). All samples from Slovakia were grouped together (Figure 9), and in the temporal scale these samples are in the same time interval (middle Miocene, Figure 2) and have also similar fungal composition (Supplementary Table 2). At the same time, the Slovakian samples were clustered with Thailand 455, located in the middle Miocene and post MMCO (Figures 2, 9).
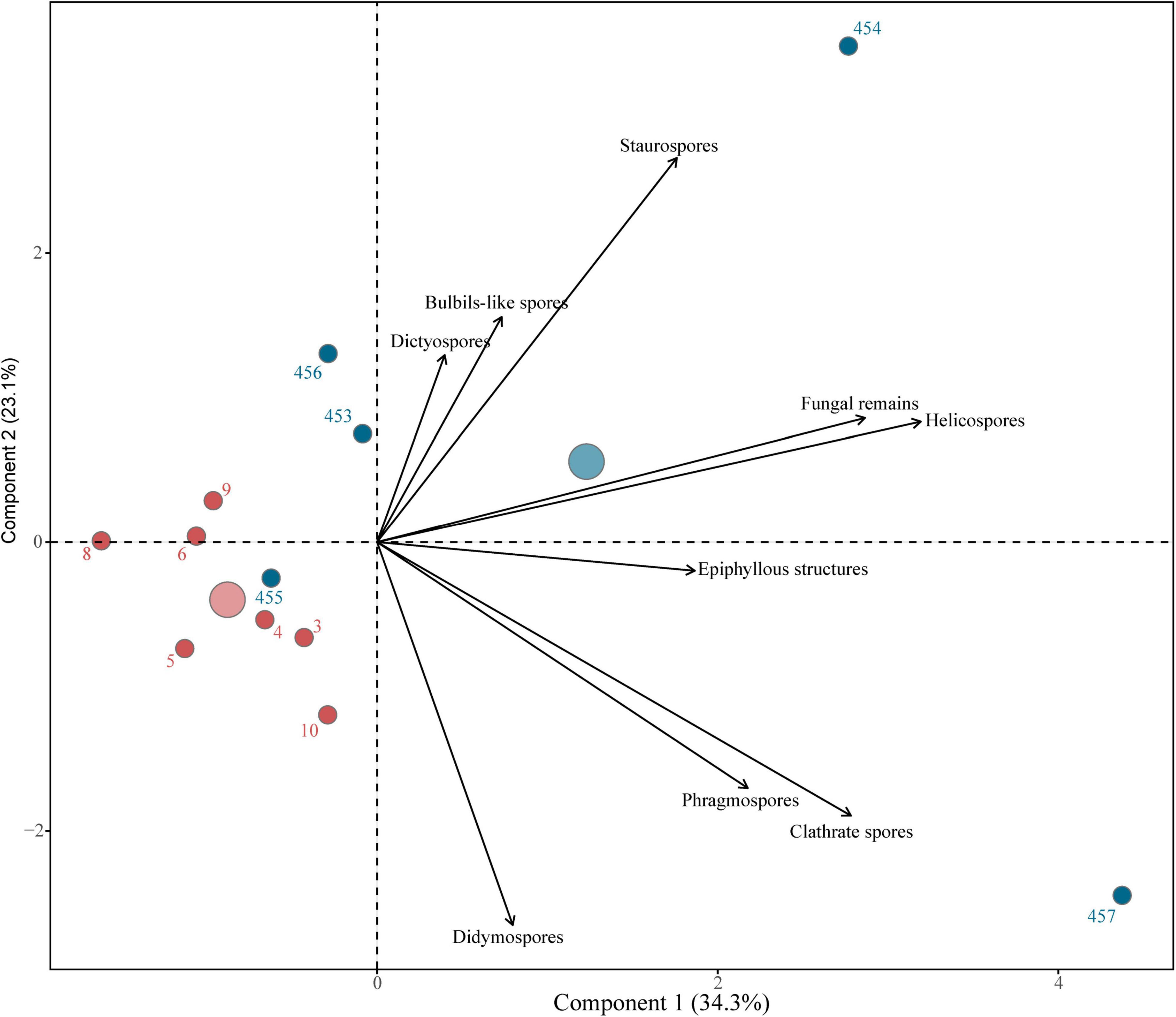
Figure 9. Principal component analysis, without including amerospores, compares the variation between and within samples from Thailand and Slovakia. Big circles indicate the midpoint of each group of samples, separating Thailand (blue) and Slovakia (red).
Based on the shape variation of the spores, we found that the samples from Thailand had a higher morphological richness than Slovakia (Figure 6). Among the samples from Thailand, α diversity was higher in samples 457 (H′: 1.56; lower Miocene) and 456 (H′: 1.53; middle Miocene). Among those from Slovakia, α diversity was higher in samples 3 (H′: 1.36; MMCO) and 5 (H′: 1.42; middle Miocene). The sample with the lowest diversity was sample 7 from Slovakia (H′: 0.0; middle Miocene). Sample 457 had the highest morphological variation, containing nine of the 10 morphological groups; it contained no staurospores (Figure 7A). Sample 7 contained only three amerospores (Figure 7B).
Samples 457 (Thailand) and 5 (Slovakia) were the only samples in which amerospores were not dominant (Figure 7). In sample 457, phragmospores were the dominant morphology (39.6%) followed by amerospores (32.1%, Figure 7A). In sample 5, didymospores were the most abundant (31.3%), followed by phragmospores (25%) and amerospores (21.3%) (Figure 7B). For Thailand, some of the spores identified in 457 were: Catenularia aff. catenulata and Phaeosclera-type (Figure 5 and Supplementary Tables 2, 3). Sample 454 followed sample 457 in morphological variation with spores from eight morphological groups (Figure 7A). In this sample, amerospores represented 75% of the variation, while the other morphologies accounted for the remainder (Figure 7A). Some of the spores identified in 454 were cf. Ascobolaceae, cf. Catenularia sp., Oncopodiella aff. trigonella and Tetraploa (Figures 3, 5 and Supplementary Table 2). Samples 453 and 456 have representatives of five and six morphological groups, respectively (Figure 7A). Spores found only in 453 include Megalohypha aff. aqua-dulces, while in 456 we found cf. Berkleasmium (Figures 3, 5 and Supplementary Tables 2, 3). From Thailand, sample 455 had the lowest morphological variability with only four morphological groups and contained identifiable spores of cf. Arthrinium, Ascotaiwania aff. limnetica, and Monosporites sp. (Figures 3, 5, 7A and Supplementary Table 2).
In Slovakia, sample 4 had the highest morphological variations with six groups, and we observed spores of Jahnula and cf. Cercophora (Figures 4, 7B and Supplementary Table 2). Samples 3, 5, and 10, each had five morphological groups (Figure 7B). Samples 6 and 9 each had 4 groups (Figure 7B), while sample 8 had only three morphological groups (Figure 7B). Spores unique to these samples include Janhula in sample 4 and cf. Monotosporella in sample 8 (Figures 4, 5 and Supplementary Table 2).
Among the samples from Thailand, spores identified in more than one sample were Arecophila sp., cf. Canalisporium, and Phragmocephala (Figures 3, 5 and Supplementary Table 2). In Slovakia, we found cf. Dictyosporium, Glomeraceae, cf. Delitschia, and cf. Tulostoma in several samples (Figures 4, 5 and Supplementary Table 2). Several spores also occurred in both localities, including cf. Rosellinia, cf. Canalisporium, Thyriothecium propagules, cf. Asterina, and Diporotheca (Figures 3–5 and Supplementary Table 2).
Pollen-Based Miocene Climate of Thailand and Slovakia
For the Thailand sites (Table 2), CREST reconstructs a mean annual temperature of 26.3°C (19.2–27.9°C) for Mae Moh with a cooler MAT reconstruction for Chiang Muan of 18.7°C (12.7–27.3°C) (Table 2 and Supplementary Figure 1). Summer temperatures at both sites are comparable with the MTWQ reconstructed at 26.9°C (25.9–29.8°C) for Mae Moh and 26.4°C (20.0–30.8°C) for Chiang Muan (Table 2 and Supplementary Figure 2). For winter temperatures, MTCQ is reconstructed at 24.6°C (16.3–28.1°C) for Mae Moh but considerably cooler at 10.9°C (3.8–24.6°C) for Chiang Muan (Table 2). While MAP at Mae Moh [2,332 mm (796–4,323 mm)] is approximately double Chiang Muan [967 mm (455–3,299 mm)] (Table 2), but precipitation seasonality is very similar, reconstructed at 56.4 (20.5–112.8%) for Mae Moh and 61.5 (17.1–114.5%) for Chiang Muan (Table 2).
For the Slovakia sites (Table 2), CREST reconstructs a MAT of 10.5°C (6.2–19.7°C) for both Nováky and the Novohrad-Nógrád Basin (Supplementary Figure 1 and Table 2). Summer and winter temperatures at the two sites are comparable with a MTWQ reconstructed at 19.0°C (14.5–27.3°C) for Nováky and 18.0°C (14.1–26.9°C) for the Novohrad-Nógrád Basin (Supplementary Figure 2 and Table 2), and a MTCQ reconstructed at 3.2°C (−5.7–12.1°C) and 3.8°C (−5.1–12.1°C) respectively (Table 2). The MAP for both sites is the same at 967 mm (398–2,105 mm) (Table 2), and precipitation seasonality for Nováky [967 mm (455–2,105 mm)] is lower than for the Novohrad-Nógrád Basin [20.5 (10.3–87.1%)] (Table 2).
Discussion
This study tried to describe fossil fungal communities from temperate and tropical regions following a trait-based approach to fungal community. Our results showed, after PCA analyses using morphotaxa, that middle Miocene fungal communities from Thailand and Slovakia may have had similar ecological characteristics and/or responses to those past environments (Figures 8, 9). Spore morphology may be related to ecological requirements due to selective pressures, affecting release pathways, transport, survival rate and deposition (Nguyen et al., 2016; Nuñez Otaño et al., 2021). Similarities between geographically distant regions, as in our study and taking the morphology of spores into account, would suggest those locations shared similar paleoclimate settings and probably were affected by similar spatial processes (see section “Paleoenvironments of Thailand and Slovakia Based on Fungal Composition”; Shearer et al., 2007; Tedersoo et al., 2014; Duarte et al., 2016).
Most of our samples presented amerospores as an important component of the total fungal richness along with phragmospores (Figure 7). Amerospores or rounder spores may indicate a humid environment with structurally closed vegetation and are more related to saprophytic fungi as litter specialist, having better air dispersal pathways and reaching longer distances (Roper et al., 2008; Calhim et al., 2018; Crandall et al., 2020). Otherwise, larger and more allantoid spores could be found frequently on wood and herbs, probably in sites with drier vegetation types (Calhim et al., 2018; Crandall et al., 2020) having more affinity to water dispersal (Magyar et al., 2016). The presence of aero-aquatic fungi recognized throughout spore shapes, such as staurospores, helicospores and bulbil-like spores, allow us to add biotic and abiotic characteristics from freshwater habitats to our paleoenvironmental reconstructions in Thailand and Slovakia in the Miocene (Ingold, 1975; Nuñez Otaño et al., 2021). These results suggest a freshwater setting with high rainfall rates for our study sites and coincided with the CREST paleoclimatic reconstructions based on paleobotanical records (Table 2). These results also suggest the presence of a more structured forest with herbaceous layers, aquatic plants, and hypoxic conditions (Tetraploa), among others (Supplementary Table 2 and Supplementary Figures 1, 2; Schoenlein-Crusius and Piccolo Grandi, 2003; Zhao et al., 2007; Chauvet et al., 2016; Magyar et al., 2021; Nuñez Otaño et al., 2021). Modern epiphyllous fungi have been found in warm, humid subtropical to tropical regions around the world with high annual rainfall and air moisture and in paleontological research works these findings are correlated with warm temperate to subtropical and moist mixed angiosperm forest (Dilcher, 1965; Selkirk, 1975; Johnson and Sutton, 2000; Reynolds and Gilbert, 2005; Limaye et al., 2007; Hofmann, 2010; Piepenbring et al., 2011; Bannister et al., 2016; Bianchinotti et al., 2020).
Recent mycological researchers are trying to describe fungal spore diversity related to fungal community structure and vegetation patterns (Pringle et al., 2015; Glassman et al., 2016; Magyar et al., 2016, 2021; Crandall et al., 2020; Nuñez Otaño et al., 2021; among others). In this study our results show that spore morphology can give us more information about paleo-fungal assemblages than previously thought. And after adding identified fungal species, we found a clearer pattern of fungal distribution related with local biotic and abiotic characteristics (see section “Paleoenvironments of Thailand and Slovakia Based on Fungal Composition”).
Paleoenvironments of Thailand and Slovakia Based on Fungal Composition
Based on fungal composition and morphological variation, the environments for the Burdigalian of Thailand and Langhian and Serravallian of Thailand and Slovakia were:
In the Burdigalian of Na Hong Thailand (sample 457), the fungal community is composed by clathrate spores, bulbil-like spores, helicospores, and epiphyllous structures (Figure 7A), usually associated to local humid and warm conditions and freshwater habitats (Ingold, 1975; Zhao et al., 2007). Meanwhile, the presence of genera, such as Catenularia aff. catenulata, Arecophila sp. and Diporotheca (Supplementary Tables 2, 3), suggests a temperate to tropical environment with freshwater habitats (Umali et al., 1999; Hawksworth and Lücking, 2017; Réblova et al., 2021). Previous paleobotanical and paleoecological studies indicated similar paleoenvironments for the lower Miocene of Na Hong with warm-temperate forest and Taxodium swamp with fern-sedge communities (Songtham et al., 2005; Sepulchre et al., 2010).
In the Langhian MMCO, the fungal composition of Mae Lai Thailand (sample 454) indicates tropical to subtropical environments with freshwater habitats and local humid conditions, based on the presence of helicospores, bulbil-like spores, staurospores, and epiphyllous structures such as Thyriothecium propagules (Figure 7A), and the presence of Tetraploa, Oncopodiella aff. trigonella, and cf. Catenularia sp. (Supplementary Tables 2, 3; Field and Webster, 1985; Bärlocher, 2009; Chauvet et al., 2016). In Slovakia, during the same period (sample 3), the presence of bulbil-like spores, and spores of cf. Canalisporum, cf. Delitschia, and Savoryella aff. fusiformis indicates a tropical to subtropical environment with low energy wetlands (i.e., swamp or lake, Figure 7B). The CREST models for Slovakia indicate similar paleoclimatic conditions with warm-temperate climates MAT of 10.5°C (6.2–19.7°C at 95% confidence), and high precipitation MAP of 967mm (398–2,105 mm) (Supplementary Table 2 and Supplementary Figures 1, 2). Thus, our fungal records and paleoclimatic reconstructions support previous paleobotanical works that indicated that during the early and middle Miocene Thailand (Mae Lai) was a paratropical forest with warm and humid conditions (Yabe, 2002; Kunimatsu et al., 2005), and Slovakia had warmer and wetter conditions that present day (Kvaček et al., 2006; Hudáčková et al., 2020).
During the Langhian and post MMCO, the fungal composition of Thailand (Chiang Muan sample 453) suggests a tropical to subtropical environment and freshwater habitats with the presence of Megalohypha aff. aqua-dulces, cf. Asterina, cf. Xylomyces sp., cf. Dictyosporium and cf. Sordaria (Figure 7A and Supplementary Tables 2, 3) (Goh et al., 1997; Hyde and Goh, 1997; Ferrer et al., 2007; Hosagoudar and Riju, 2013; Hofmann and Piepenbring, 2014; Shumilovskikh et al., 2017; Yang et al., 2018). The CREST models indicated similar climatic conditions for Chiang Muan with a MAT of 18.7°C (12.7–27.3°C at 95% confidence) and a MAP of 967 mm (455–3,299 mm at 95% confidence), suggesting a subtropical and modestly humid climate (Supplementary Table 2 and Supplementary Figures 1, 2). Studies in this region have indicated tropical woodlands and peat swamp forests with fern-sedge communities (Songtham et al., 2005; Sepulchre et al., 2010). For Slovakia (sample 7), we were not able to get paleoecological inferences for the same period because the sample was fungal palynomorph-poor and contained only three amerospore types (Figure 7B and Supplementary Table 1). Based on previous published organic petrography and geochemistry, sample 7 likely represents an area of root-dominated peat produced by plants in an ombrogenous setting (O’Keefe et al., 2011). Previous paleoreconstructions for the late Langhian of Slovakia have indicated environments with highly seasonal warm-temperate to temperate conditions by the late Langhian for Nováky and the Novohrad-Nógrád Basin (Kvaček et al., 2006; Hudáčková et al., 2020).
In the early Serravallian from Thailand (sample 456 from Mae Moh), the presence of dictyospores, bulbil-like spores, helicospores and didymospores, as well as the record of cf. Canalisporium, Phragmocephala, and cf. Berkleasmium (Supplementary Table 2 and Figures 3, 5, 7A) suggest a tropical environment and humid local conditions, with forests and freshwater habitats (Holubová-Jechová, 1987; Yip, 1988; Chen and Tzean, 2010; Zhao et al., 2012; Hüseyin et al., 2014; Su et al., 2015). These results concur with the paleoclimatic reconstructions for Mae Moh that indicate tropical and wet environments during the Serravallian [MAT: 26.3°C (19.2–27.9°C at 95% confidence) and a MTCQ of 24.6°C (16.3–28.1°C at 95% confidence); MAP: 2,332 mm (796–4,323 mm at 95% confidence)] (Supplementary Table 2 and Supplementary Figures 1, 2). Other studies have indicated a warm tropical peat swamp with fern-sedge vegetation, and in the uplands a subtropical warm temperate mixed forest with Pinus and grasses (Songtham et al., 2005; Sepulchre et al., 2010).
Some of the fungi identified in Slovakian samples that were deposited in the Serravallian, in Hlavny slaj (samples 4–6 and 8–10, Figure 2) were cf. Monotosporella, Jahnula, cf. Tulostoma, cf. Dictyosporium, and Thyriothecium propagules, among others (Figures 4, 5 and Supplementary Table 2). The modern ecology of these spores indicates tropical to temperate environments with humid conditions and freshwater habitats (Goh et al., 1999; Sri-indrasutdhi et al., 2010; Cortez et al., 2011; Sadowski et al., 2012; Jeppson et al., 2017; Yang et al., 2018). The Paleoclimatic reconstructions for Slovakia using CREST suggest a warm-temperate climate with high precipitation [MAT: 10.5°C (6.2–19.7°C); MAP: 967 mm (398–2,105 mm)] (Supplementary Table 2 and Supplementary Figures 1, 2). Preliminary studies for the Serravallian of Slovakia have indicated warm-temperate climates persisted through this time in the upper Horna Nitra Basin (Hudáčková et al., 2020). Additionally, samples 4, 6, and 10 are from the base and roof of the seams, where ombrogenous conditions had yet to develop or were giving way as peat production ceased (Hudáček et al., 1982; Machajová et al., 2000, 2002; Dai et al., 2020).
The fungal composition of sample 455 from Mae Moh, Thailand includes Arecophila sp., cf. Arthrinium, cf. Canalisporium, Savoryella aff. fusiformis, cf. Rosellinia, and Ascotaiwania aff. limnetica (Figures 3, 5 and Supplementary Table 2). The modern ecology of these genera suggests a tropical to temperate environment with freshwater habitats similar to the paleoenvironments of the Serravallian samples from Slovakia (Umali et al., 1999; Petrini, 2003, 2013; Songtham et al., 2005; Zhao et al., 2007; Sepulchre et al., 2010; Chauvet et al., 2016). This also explains the clustering of this sample with the Slovakian samples in the PCA (Figure 9). These results are also supported by the paleoclimatic reconstructions made for Thailand and Slovakia during the middle Miocene, suggesting a tropical to warm-temperate environments with humid conditions (Supplementary Table 2 and Supplementary Figures 1, 2).
Paleoenvironments and Paleoclimate During the Middle Miocene
The composition and diversity of fungal communities seems to be influenced by climate, affecting ecosystem functioning (Větrovský et al., 2020). This has been observed in several studies of modern fungal biogeographic distributions (Tedersoo et al., 2014; Bahram et al., 2016; Daws et al., 2020). Our results seem to reflect similar fungal community composition under similar environments in different countries (Figures 8, 9), showing an apparent correlation with the paleoclimate. The paleoclimatic reconstructions made for the middle Miocene of Slovakia and Thailand, using paleobotanical records indicate that Slovakia was seasonal warm temperate (Nováky and the Novohrad-Nógrád Basin), while Thailand was tropical with increasing seasonality during the late Langhian and early Serravallian (Chiang Muan), and ever warm tropical later in the Serravalian (Mae Moh) (Table 2 and Supplementary Figures 1, 2). These results complement our paleoenvironmental reconstructions for the Miocene of Thailand and Slovakia based on the overall fungal community composition using a spore traits-based approach, in which the environments from both countries vary from temperate, subtropical to tropical with local humid conditions. In the fossil record, it appears that the fungal community composition is tightly linked with local floral diversity and microhabitat conditions, but this could be driven by environmental factors at a regional scale, as observed in modern ecosystems (Crandall et al., 2020).
Even though preliminary, the results of this study reflect the presence of uncommon taxa in each country, however, we need to deepen our samples analysis to understand those species distribution and presence at a regional scale in the fossil record. Despite the age differences among the samples, amerospores were common, which could suggest that they either share better dispersal pathways, i.e., air, favoring long dispersal distances or have a wider host range (Halbwachs and Bässler, 2015; Magyar et al., 2016; Calhim et al., 2018). Applying current knowledge about plant-fungal interactions to fossil taxa will help to identify regional fungal distribution and to elucidate ecosystem-level changes of fungal communities through time (Amend et al., 2010; Talbot et al., 2014; Davison et al., 2015).
Conclusion
The FiaWW project is just beginning its exploration of Burdigalian-Serravallian global fungal assemblage distributions. Our assessment of differences at fungal community level between temperate and tropical regions in the middle Miocene hints at greater species richness in Thailand (p < 0.02) and variations in the diversity in both countries within the samples.
This study has resulted in the identification of 281 unique fungal morphotaxa, of which 29 have been identified. More fungi need to be identified to the family or genus level from a larger geographical area and with better age control, especially in Europe. Likewise, more identifications are needed to explore fossil fungal distributions.
This study shows that morphological spore traits could be used in the fossil record as first instance, and when identifications are scarce, to start working at fungal community levels, to describe patterns of fungal diversity and paleoenvironments in the fossil record, and to compare different assemblages at large spatial and temporal scales.
Based on fossil fungal composition and paleoclimatic reconstructions, the paleoenvironments for Thailand and Slovakia were similar during the middle Miocene, with tropical to subtropical environments during the MMCO, but more seasonal warm temperate and tropical with increasing seasonality post MMCO.
Identifying fossil fungi based on its modern analogs and following current nomenclature rules is helpful to redefine fungi as reliable proxies to complement paleontological research works.
These results will be integrated in a larger study with three working hypotheses that underpin the FiaWW project: (1) Overall fungal diversity was higher during the MMCO; (2) MMCO fungal assemblages globally showed a greater species richness and abundance of forest-associated ascomycetes; and (3) Fungal diversity during the MMCO was greatest in areas of warm-unseasonal climates.
Data Availability Statement
The original contributions presented in the study are included in the article/Supplementary Material, further inquiries can be directed to the corresponding authors.
Author Contributions
JO’K and MP conceived the idea for and designed the study. HB supplied samples and background information. IR, TS, CF, SJ, and LT primarily generated data with supporting data generated by MG and JO’K. NN, IR, and JO’K made taxonomic identifications and determined ecological tolerances. IR, NN, JO’K, MG, and MP completed data analyses. IR and NN drafted figures. IR, NN, and JO’K equally led initial writing with contributions from MG, MP, and SW. IR, NN, and JO’K led manuscript editing with supporting editing by all other authors. All authors contributed to the article and approved the submitted version.
Conflict of Interest
The authors declare that the research was conducted in the absence of any commercial or financial relationships that could be construed as a potential conflict of interest.
Publisher’s Note
All claims expressed in this article are solely those of the authors and do not necessarily represent those of their affiliated organizations, or those of the publisher, the editors and the reviewers. Any product that may be evaluated in this article, or claim that may be made by its manufacturer, is not guaranteed or endorsed by the publisher.
Funding
The Fungi in a Warmer World (FiaWW) project was jointly funded by NSF/Geo and NERC under NSF award #2015813 to JO’K and NERC award identifier NE/V01501X/1 to MP.
Acknowledgments
The efforts of IR, NN, SW, MP, MG, JO’K, LT, and TS were supported by FiaWW. TS was also supported by an Undergraduate Research Fellowship awarded by the Department of Physics, Earth Science and Space Systems Engineering at Morehead State University. Samples from Thailand and Slovakia were provided to JO’K under the USGS NCRDS project via James Hower, Robert Finkelman, and HB, and are in the public domain per project chief Joseph A. East. Remaining samples are part of the Harvey Belkin Collection in the Minerals Section of the Smithsonian Institution; slides will be archived at the Smithsonian Institution following project completion.
Supplementary Material
The Supplementary Material for this article can be found online at: https://www.frontiersin.org/articles/10.3389/ffgc.2021.768405/full#supplementary-material
References
Amend, A. S., Seifert, K. A., Samson, R., and Bruns, T. D. (2010). Indoor fungal composition is geographically patterned and more diverse in temperate zones than in the tropics. Proc. Natl. Acad. Sci. U. S. A. 107, 13748–13753. doi: 10.1073/pnas.1000454107
Bahram, M., Kohout, P., Anslan, S., Harend, H., Abarenkov, K., and Tedersoo, L. (2016). Stochastic distribution of small soil eukaryotes resulting from high dispersal and drift in a local environment. ISME J. 10, 885–896. doi: 10.1038/ismej.2015.164
Bannister, J. M., Conran, J. G., and Lee, D. E. (2016). Life on the phylloplane: eocene epiphyllous fungi from Pikopiko Fossil Forest, Southland, New Zealand. N. Z. J. Bot. 54, 412–432. doi: 10.1080/0028825x.2016.1208252
Bärlocher, F. (2009). Reproduction and dispersal in aquatic hyphomycetes. Mycoscience 50, 3–8. doi: 10.1371/journal.pone.0159396
Belkin, H. E., Hower, J. C., O’Keefe, J., Garrison, T., and Johnston, M. (2013). Cenozoic Coals Of Southeast Asia (Malaysia, Philippines, And Thailand): Petrology And Geochemistry. Sosnowiec: The Society for Organic Petrology.
Benammi, M., Chaimanee, Y., and Urrutia Fucugauchi, and Jean-Jacques, J. (2010). Magnetostratigraphic Study of the Continental Sedimentary Sequence of the Chiang Muan Basin, Northern Thailand: Implications for the Age of the First Miocene Hominoids from Thailand. Int. Geol. 7, 646–654. doi: 10.2747/0020-6814.46.7.646
Benammi, M., Urrutia-Fucugauchi, J., Alva-Valdivia, L. M., Chaimanee, Y., Triamwichanon, S., and Jaeger, J.-J. (2002). Magnetostratigraphy of the middle Miocene continental sedimentary sequences of the Mae Moh Basin in northern Thailand: evidence for counterclockwise block rotation. Earth Planet. Sci. Lett. 204, 373–383. doi: 10.1016/s0012-821x(02)01002-6
Bianchinotti, M. V., Martínez, M. A., and Cornou, M. E. (2020). The utility of Desmidiospora: a paradigm shift based on Paleogene fungal remains from the Ñirihuau Basin, Argentina. Palynology 44, 587–596. doi: 10.1080/01916122.2019.1657514
Birks, H. J., and Birks, H. H. (1980). Quaternary Palaeoecology. London: Arnold Publishers Limited, 289.
Calhim, S., Halme, P., Petersen, J. H., Læssøe, T., Bässler, C., and Heilman-Clausen, J. (2018). Fungal spore diversity relects substrate-specific deposition challenges. Sci. Rep. 8:5356. doi: 10.1038/s41598-018-23292-8
Čechovič, V. (1950). Geologické pomery handlovskej a nováckej uhol’nej panvy. Geologickı Zborník Slovenskej Akademie vied a umení 1.
Chaudhary, V. B., Cuenca, G., and Johnson, N. C. (2018). Tropical–temperate comparison of landscape-scale arbuscular mycorrhizal fungal species distributions. Divers. Distrib. 24, 116–128. doi: 10.1111/ddi.12664
Chauvet, E., Cornut, J., Sridhar, K. R., Selosse, M. A., and Bärlocher, F. (2016). Beyond the water column: aquatic hyphomycetes outside their preferred habitat. Fungal Ecol. 19, 112–127. doi: 10.1016/j.funeco.2015.05.014
Chen, J. L., and Tzean, S. S. (2010). Hyphomycetes from Taiwan - Endophragmia and Allied Species. Taiwania 55, 37–42.
Chevalier, M., Cheddadi, R., and Chase, B. M. (2014). CREST (Climate REconstruction SofTware): a probability density function (PDF)-based quantitative climate reconstruction method. Clim. Past 10, 2081–2098. doi: 10.5194/cp-10-2081-2014
R Core Team (2021). R: A Language And Environment For Statistical Computing. Vienna: R Foundation for Statistical Computing.
Cortez, V. G., Baseia, I. G., and Silveira, R. M. B. (2011). –Gasteroid mycobiota of Rio Grande do Sul, Brazil: boletales. J. Yeast Fungal Res. 2, 44–52.
Coster, P., Benammi, M., Chaimanee, Y., Yamee, C., Chavasseau, O., Emonet, E., et al. (2010). A complete magnetic-polarity stratigraphy of the Miocene continental deposits of Mae Moh Basin, northern Thailand, and a reassessment of the age of hominoid-bearing localities in northern Thailand. Geol. Soc. Am. Bull. 122, 1180–1191. doi: 10.1130/b26568.1
Crandall, S. G., Saarman, N., and Gilbert, G. S. (2020). Fungal spore diversity, community structure, and traits across a vegetation mosaic. Fungal Ecol. 45:100920. doi: 10.1016/j.funeco.2020.100920
Dai, S., Bartley, R., Bartley, S., Valentim, B., Guedes, A., O’Keefe, J. M. K., et al. (2017). Organic geochemistry of Funginite (Miocene, Eel River, Mendocino County, California, USA) and Macrinite (Cretaceous, Inner Mongolia, China). Int. J. Coal Geol. 179, 60–71. doi: 10.1016/j.coal.2017.05.015
Dai, S., Bechtel, A., Eble, C. F., Flores, R. M., French, D., Graham, I. T., et al. (2020). Recognition of peat depositional environments in coal: a review. Int. J. Coal Geol. 219:103383. doi: 10.1016/j.coal.2019.103383
Davison, J., Moora, M., Öpik, M., Adholeya, A., Ainsaar, L., Bâ, A., et al. (2015). Global assessment of arbuscular mycorrhizal fungus diversity reveals very low endemism. Science 349, 970–973. doi: 10.1126/science.aab1161
Daws, S. C., Cline, L. A., Rotenberry, J., Sadowsky, M. J., Staley, C., Dalzell, B., et al. (2020). Do shared traits create the same fates? Examining the link between morphological type and the biogeography of fungal and bacterial communities. Fungal Ecol. 46:100948. doi: 10.1016/j.funeco.2020.100948
Dilcher, D. L. (1965). Epiphyllous fungi from Eocene deposits in Western Tennessee, USA. Paleontographica 116, 1–54. doi: 10.1155/1955/76727
Dill, H. G., Sachsenhofer, R. F., Grecula, P., Sasvári, L. A., Palinkaš, L. A., Borojević-Šoštarić, S., et al. (2008). “Fossil fuels, ore and industrial minerals,” in The Geology of Central Europe: Mesozoic and Cenozoic, ed. T. McCann (London: Special Publication), 1341–1449. doi: 10.1144/cev2p.9
Duarte, S., Bärlocher, F., Pascoal, C., and Cássio, F. (2016). Biogeography of aquatic hyphomycetes: current knowledge and future perspectives. Fungal Ecol. 19, 169–181. doi: 10.1016/j.funeco.2015.06.002
Ferrer, A., Sivichai, S., and Shearer, C. A. (2007). Megalohypha, a new genus in the Jahnulales from aquatic habitats in the tropics. Mycologia 99, 456–460. doi: 10.3852/mycologia.99.3.456
Field, J. I., and Webster, J. (1985). Effects of sulphide on survival of aero-aquatic and aquatic hyphomycetes. Trans. Br. Mycol. Soc. 85, 193–199.
Friederich, M. C., Moore, T. A., and Flores, R. M. (2016). A regional review and new insights into SE Asian Cenozoic coal-bearing sediments: why does Indonesia have such extensive coal deposits? Int. J. Coal Geol. 166, 2–35. doi: 10.1016/j.coal.2016.06.013
Gibson, M. E., McCoy, J., O’Keefe, J. M. K., Nuñez Otaño, N. B., Warny, S., and Pound, J. M. The development of UK Neogene Climate: a comparison of the co-existence approach, Bayesian and probability reconstruction techniques. Paleoceanogr. Paleoclimatol. (in review)
Glassman, S. I., Levine, C. R., DiRocco, A. M., Battles, J. J., and Bruns, T. D. (2016). Ectomycorrhizal fungal spore bank recovery after a severe forest fire: some like it hot. ISME J. 10, 389–399. doi: 10.1038/ismej.2015.182
Goh, T. K., Ho, W. H., Hyde, K. D., and Umali, T. E. (1997). New records and species of Sporoschisma and Sporoschismopsis from submerged wood in the tropics. Mycol. Res. 101, 1295–1307. doi: 10.1017/s0953756297003973
Goh, T. K., Hyde, K. D., Ho, W. H., and Yanna. (1999). A revision of the genus Dictyosporium, with descriptions of three new species. Fungal Divers. 2, 65–100.
Grimm, G. W., and Potts, A. J. (2016). Fallacies and fantasies: the theoretical underpinnings of the Coexistence Approach for palaeoclimate reconstruction. Clim. Past 12, 611–622. doi: 10.5194/cp-12-611-2016
Grohé, C., de Bonis, L., Chaimanee, Y., Chavasseau, O., Rugbumrung, M., Yamee, C., et al. (2020). The late middle Miocene Mae Moh Basin of northern Thailand: the richest Neogene assemblage of Carnivora from Southeast Asia and a paleobiogeographic analysis of Miocene Asian carnivorans. Am. Mus. Novit. 3952, 1–57. doi: 10.1206/3952.1
Guarro, J., Gené, A. M., Stchigel, A. M., and Figueras, M. J. (2012). Atlas Of Soil Ascomycetes. Utrecht: CBS-KNAW Fungal Biodiversity Centre.
Gulis, V., Marvanová, L., and Descals, E. (2005). “An illustrated key to the common temperate species of aquatic hyphomycetes,” in Methods to study litter decomposition: A practical guide, eds M. A. S. Graça, F. Bärlocher, and M. O. Gessner (Netherlands: Springer), 153–167. doi: 10.1007/1-4020-3466-0_21
Halbwachs, H., and Bässler, C. (2015). Gone with the wind–a review on basidiospores of lamellate agarics. Mycosphere 6, 78–112. doi: 10.5943/mycosphere/6/1/10
Hawksworth, D. L., and Lücking, R. (2017). Fungal diversity revisited: 2.2 to 3.8 million species. Microbiol. Spectr. 5:4. doi: 10.1128/microbiolspec.FUNK-0052-2016
Heitman, J., Crous, P. W., Gow, N. A., Howlett, B. J., James, T. Y., and Stukenbrock, E. H. (2018). The Fungal Kingdom. Washington: ASM press.
Hofmann, T. A. (2010). Plant parasitic Asterinaceae and Microthyriaceae from the Neotropics (Panama). Frankfurt: Johann Wolfgang Goethe-University.
Hofmann, T. A., and Piepenbring, M. (2014). New records of plant parasitic Asterinaceae (Dothideomycetes, Ascomycota) with intercalary appressoria from Central America and Panama. Trop. Plant Pathol. 39, 419–427. doi: 10.1590/s1982-56762014000600001
Hók, J., Šimon, L., Kováč, P., Elečko, M., Vass, D., Halmo, J., et al. (1995). Tectonics of the Nononitrianska Kotlina Depression in the Neogene. Geol. Carpath. 46, 191–196.
Holubová-Jechová, V. (1987). Studies on Hyphomycetes from Cuba VI. New and rare species with tretic and phialidic conidiogenous cells. Èeská Mykologie 41, 107–114.
Hosagoudar, V. B., and Riju, M. C. (2013). Foliicolous fungi of Silent Valley National Park, Kerala, India. J. Threat. Taxa 5, 3701–3788. doi: 10.11609/jott.o3302.01-88
Hudáček, J., Brodňanová, E., and Blaško, D. (1982). Geologické problémy a prognózy uhol’ných ložisk hononitrianskej uhol’nej panvy. Miner. Slovaca 14, 209–220.
Hudáčková, N., Holcová, K., Halásová, E., Kováčová, M., Doláková, N., Trubač, J., et al. (2020). The Pannonian Basin System northern margin paleogeography, climate, and depositional environments in the time range during. Palaeontol. Electronica 23:a50. doi: 10.26879/1067
Hughes, S. J. (1953). Conidiophores, conidia and classification. Can. J. Bot. 31, 577–580. doi: 10.1139/b53-046
Hüseyin, E., Selçuk, F., and Churakov, B. P. (2014). A new species of Berkleasmium from Ulyanovsk, Russia. Mycosphere 5, 462–466. doi: 10.5943/mycosphere/5/3/8
Hyde, K. D., and Goh, T. K. (1997). Fungi on submerged wood in a small stream on Mt Lewis. North Queensland, Australia. Muelleria 10, 145–157.
Hyde, K. D., Jeewon, R., Bahl, J., Bhilabut, B., Bussaban, B., Cai, L., et al. (2004). “Fungal studies at University of Hong Kong,” in Annual Meeting of Mycological Society of America, (Canada: Mycological Society of America).
Ingold, C. T. (1975). An illustrated guide to aquatic and water-borne Hyphomycetes (Fungi Imperfecti) with notes on their biology. J. Appl. Ecol. 12:989. doi: 10.2307/2402112
Intergovernmental Panel on Climate Change [IPCC] (2014). “Climate Change 2014: Synthesis Report,” in Contribution of Working Groups I, II and III to the Fifth Assessment Report of the Intergovernmental Panel on Climate Change, eds R. K. Pachauri and L. A. Meyer (Geneva: IPCC), 151.
Intergovernmental Panel on Climate Change [IPCC] (2021). “Climate Change 2021: The Physical Science Basis,” in. Contribution of Working Group I to the Sixth Assessment Report of the Intergovernmental Panel on Climate Change, eds V. P. Zhai, A. Pirani, S. L. Connors, C. Péan, S. Berger, and N. Caud (Cambridge: Cambridge University Press).
Jeppson, M., Altes, A., Moreno, G., Nilsson, R. H., Loarce, Y., de Bustos, A., et al. (2017). Unexpected high species diversity among European stalked puffballs—a contribution to the phylogeny and taxonomy of the genus Tulostoma (Agaricales). MycoKeys 21, 33–88.
Johnson, E. M., and Sutton, T. B. (2000). Response of two fungi in the apple sooty blotch complex to temperature and relative humidity. Phytopathology 90, 362–367. doi: 10.1094/PHYTO.2000.90.4.362
Kalgutkar, R. M., and Jansonius, J. (2000). Synopsis of Fossil Fungal Spores, Mycelia and Fructifications. Dallas: American Association of Stratigraphic Palynologists Foundation, 39.
Kučerová, J. (2010). The Badenian flora and the palaeoclimate interpretation of the locality Nováky, Slovak Republic. Journal of the National Museum (Prague). Nat. Hist. Ser. 179, 127–130.
Kunimatsu, Y., Ratanasthien, B., Nakaya, H., Saegusa, H., Nagaoka, S., Suganuma, Y., et al. (2005). An additional specimen of a large-bodied Miocene hominoid from Chiang Muan, northern Thailand. Primates 46, 65–69.
Kvaček, Z. (1994). “Connecting links between the Arctic Paleogene and European Tertiary floras,” in Cenozoic Plants And Climates Of The Arctic. NATO ASI Series (Series I: Global Environmental Change), vol 27, ed. M. C. Boulter and H. V. Fisher (Berlin: Springer).
Kvaček, Z., Kováč, M., Kovar-Eder, J., Doláková, N., Jechorek, H., Parashiv, V., et al. (2006). Miocene evolution of landscape and vegetation in the Central Paratethys. Geol. Carpath. 57, 295–310.
Limaye, R. B., Kumaran, K. P. N., Nair, K. M., and Padmalal, D. (2007). Non-pollen palynomorphs as potential palaeoenvironmental indicators in the Late Quaternary sediments of the west coast of India. Curr. Sci. 92, 1370–1382.
Loughlin, N. J. D., Mayle, F. E., Nuñez Otaño, N. B., O’Keefe, J. M. K., Duncan, N. A., Walker, J. H., et al. (2021). Insights into past land-use and vegetation change in the Llanos de Moxos (Bolivia) using fungal non-pollen palynomorphs. J. Archaeol. Sci. 130:105382.
Machajová, Z., Verbich, F., and Sýkorová, I. (2000). Geologická a petrografická charakteristika ložiska Handlová. Acta Montan. Slovaca Roènik 5, 261–264.
Machajová, Z., Verbich, F., and Sýkorová, I. (2002). The geology, petrography and mineralogy composition of coal from the Nováky deposit. Acta Montan. Slovaca 7, 28–33.
Magyar, D., Van Stan, J. T. II, and Sridhar, K. R. (2021). Hypothesis and Theory: fungal spores in stemflow and potential bark sources. Front. For. Glob. Chang. 4:623758. doi: 10.3389/ffgc.2021.623758
Magyar, D., Vass, M., and Li, D.-W. (2016). “Dispersal strategies of microfungi,” in Biology of Microfungi, ed. LiDe-Wei (Berlin: Springer), 315–371. doi: 10.1007/978-3-319-29137-6_14
McDonald, J. H. (2014). Handbook of Biological Statistics, 3rd Edn. Baltimore: Sparky House Publishing, 157–164.
Morley, C. K., and Racey, A. (2011). “Tertiary stratigraphy,” in The Geology Of Thailand, eds M. F. Ridd, A. J. Barber, and M. J. Crow (London: Geological Society of London), 223–271.
Morley, R. J. (2012). “A review of the Cenozoic palaeoclimate history of Southeast Asia,” in Biotic Evolution and Environmental Change in Southeast Asia, eds D. J. Gower, K. G. Johnson, J. E. Richardson, B. R. Rosen, L. Rüber, and S. T. Williams (Cambridge: Cambridge University Press), 79–114. doi: 10.1017/cbo9780511735882.006
Nguyen, N. H., Song, Z., Bates, S. T., Branco, S., Tedersoo, L., Menke, J., et al. (2016). FUNGuild: an open annotation tool for parsing fungal community datasets by ecological guild. Fungal Ecol. 20, 241–248. doi: 10.1016/j.funeco.2015.06.006
Nuñez Otaño, N., Bianchinotti, M. V., and Saparrat, M. C. N. (2021). “Palaeomycology: a modern mycological view of fungal palynomorphs,” in Applications of non-pollen palynomorphs: from Palaeoenvironmental Reconstructions to Biostratigraphy, eds F. Marret, J. O’Keefe, P. Osterloff, and M. Pound, and Shumilovskikh (London: Geological Society), 511. doi: 10.1144/SP511-2020-47
Nuñez Otaño, N., di Pasquo, M., and Bianchinotti, M. V. (2017). The occurrence of Potamomyces palmarensis sp. nov. in the Late Holocene of El Palmar National Park (Colón, Entre Ríos, Argentina) and transfer of fossil species of Mediaverrunites to Potamomyces. Palynology 41, 267–277. doi: 10.1080/01916122.2016.1146174
O’Keefe, J. M. K. (2017). Fungal palynomorphs from the Miocene Heath Formation, Tumbes Province, Perú. Palynology 41, 309–326. doi: 10.1080/01916122.2017.1366193
O’Keefe, J. M. K., and Eble, C. F. (2012). A comparison of HF-based and non-HF-based palynology processing techniques in clay-rich lignites from the Claiborne Group, upper Mississippi Embayment, United States. Palynology 36, 116–130. doi: 10.1080/01916122.2011.642484
O’Keefe, J. M. K., Hower, J. C., Bechtel, A., Christanis, K., Dai, S., DiMichele, W., et al. (2013). On the fundamental difference between coal rank and type. Int. J. Coal Geol. 118, 58–87. doi: 10.1016/j.coal.2013.08.007
O’Keefe, J. M. K., Hower, J. C., Finkelman, R. F., Drew, J. W., and Stucker, J. D. (2011). Petrographic, geochemical, and mycological aspects of Miocene coals from the Nováky and Handlová mining districts, Slovakia. Int. J. Coal Geol. 87, 268–281. doi: 10.1016/j.coal.2011.06.019
O’Keefe, J. M. K., Nuñez Otaño, N. B., Bianchinotti, M. V., Marret, F., O’Keefe, J., and Osterloff, P., et al. (eds) (2021). “Nomenclature: how do we designate NPP taxa?,” in Applications of Non-Pollen Palynomorphs: from Palaeoenvironmental Reconstructions to Biostratigraphy, (London: Geological Society London), 511. doi: 10.1144/SP511-2020-119
Petrini, L. E. (2003). Rosellinia and related genera in New Zealand. N. Z. J. Bot. 41, 71–138. doi: 10.1080/0028825x.2003.9512833
Piepenbring, M., Hofmann, T. A., Kirschner, R., Mangelsdorff, R., and Perdomo, O. (2011). Diversity patterns of Neotropical plant parasitic microfungi. Ecotropica 17, 27–40.
Pirozynski, K. A., and Weresub, L. K. (1979). “The classification and nomenclature of fossil fungi,” in The whole fungus, the sexual–asexual synthesis. Proceedings of the 2nd International Mycological Conference, University of Calgary, Kananaskis, Alberta, ed. B. Kendrick (Ottawa: National Museum of Natural Sciences, National Museums of Canada and the Kananaskis Foundation), 653–688.
Planderová, E. (1991). Miocene Microflora Of Slovak Central Paratehys And It Biostratigraphical Significance. Bratislava: Dionýìz Stuìr Institute of Geology.
Pound, M. J., O’Keefe, J. M. K., and Marret, F. (2021). “An overview of techniques applied to the extraction of non-pollen palynomorphs, their known taphonomic issues and recommendations to maximize recovery,” in Applications of Non-Pollen Palynomorphs: from Palaeoenvironmental Reconstructions to Biostratigraphy, eds F. Marret, J. O’Keefe, P. Osterloff, M. Pound, and L. Shumilovskikh (London: Geological Society), 511. doi: 10.1144/SP511-2020-40
Pound, M. J., O’Keefe, J. M. K., Nuñez Otaño, N. B., and Riding, J. B. (2019). Three new Miocene fungal palynomorphs from the Brassington Formation, Derbyshire, UK. Palynology 43, 596–607. doi: 10.1080/01916122.2018.1473300
Pringle, A., Vellinga, E., and Peay, K. (2015). The shape of fungal ecology: does spore morphology give clues to a species’ niche? Fungal Ecol. 17, 213–216. doi: 10.1016/j.funeco.2015.04.005
Ratanasthien, B. (2002). Problems of Neogene biostratigraphic correlation in Thailand and surrounding areas. Rev. Mex. Cienc. Geol. 19, 235–241.
Ratanasthien, B. (2011). “Coal deposits,” in The Geology of Thailand, eds M. F. Ridd, A. J. Barber, and M. J. Crow (London: The Geological Society), 393–414.
Ratanasthien, B., Takashima, I., and Matsubaya, O. (2008). Paleaogeography and climatic change recorded on Viviparidae carbon and oxygen isotope in Mae Moh coal mine, northern Thailand. Bull. Geol. Surv. Jpn 59, 327–338. doi: 10.9795/bullgsj.59.327
Réblova, M., Nekvindova, J., and Miller, A. N. (2021). Phylogeny and taxonomy of Catenularia and similar fungi with catenate conidia. MycoKeys 81, 1–44. doi: 10.3897/mycokeys.81.67785
Reynolds, D. R., and Gilbert, G. S. (2005). Epifoliar fungi from Queensland, Australia. Aust. Syst. Bot. 18, 265–289. doi: 10.1890/05-1170
Riding, J. B. (2021). A guide to preparation protocols in palynology. Palynology 45, 1–110. doi: 10.1080/01916122.2021.1878305
Roper, M., Pepper, R. E., Brenner, M. P., and Pringle, A. (2008). Explosively launched spores of ascomycete fungi have drag-minimizing shapes. Proc. Natl. Acad. Sci. U. S. A. 105, 20583–8. doi: 10.1073/pnas.0805017105
Rudolph, S., Schleuning, M., and Piepenbring, M. (2018). Temporal variation of fungal diversity in a mosaic landscape in Germany. Stud. Mycol. 89, 95–104. doi: 10.1016/j.simyco.2018.01.001
Sadowski, E. M., Beimforde, C., Gube, M., Rikkinen, J., Singh, H., Seyfullah, L. J., et al. (2012). The anamorphic genus Monotosporella (Ascomycota) from Eocene amber and from modern Agathis resin. Fungal Biol. 116, 1099–1110. doi: 10.1016/j.funbio.2012.08.003
Schoenlein-Crusius, I. H., and Piccolo Grandi, R. A. (2003). The diversity of aquatic hyphomycetes in South America. Braz. J. Microbiol. 34, 183–193. doi: 10.1590/S1517-83822003000300001
Seifert, K. A., and Gams, W. (2011). The genera of Hyphomycetes - 2011 update. Persoonia 27, 119–129. doi: 10.3767/003158511X617435
Seifer, K., Morgan-Jones, G., Gams, W., and Kendrick, B. (2011). The Genera of Hyphomycetes. Utrecht, The Netherlands: CBS-KNAW Fungal Diversity Center, p. 997.
Selkirk, D. R. (1975). Tertiary fossil fungi from Kiandra, New South Wales. Proc. Linn. Soc. N. S. W. 100, 70–94.
Sepulchre, P., Jolly, D., Ducrocw, S., Chaimanee, Y., Jaeger, J.-J., and Raillard, A. (2010). Mid-Tertiary paleoenvironments in Thailand: pollen evidence. Clim. Past 6, 461–473. doi: 10.5194/cp-6-461-2010
Shearer, C. A., Descals, E., Kohlmeyer, B., Kohlmeyer, J., Marvanová, L., Padgett, D., et al. (2007). Fungal biodiversity in aquatic habitats. Biodivers. Conserv. 16, 49–67. doi: 10.1007/s10531-006-9120-z
Shumilovskikh, L., O’Keefe, J. M. K., and Marret, F. (2021). “An overview of the taxonomic groups of non-pollen palynomorphs,” in Applications of Non-Pollen Palynomorphs: from Palaeoenvironmental Reconstructions to Biostratigraphy, eds F. Marret, J. O’Keefe, P. Osterloff, M. Pound, and L. Shumilovskikh (London: Geological Society), 511. doi: 10.1144/SP511-2020-65
Shumilovskikh, L. S., Ferrer, A., and Schlütz, F. (2017). Non-pollen palynomorphs notes: 2. Holocene record of Megalohypha aqua-dulces, its relation to the fossil form genus Fusiformisporites and association with lignicolous freshwater fungi. Rev. Palaeobot. Palynol. 246, 167–176. doi: 10.1016/j.revpalbo.2017.07.002
Sitár, V., Planderová, E., and Čierna, E. (1987). Knowledge on fossil flora of the Handlová-Nováky lignite basin obtained from the Vt-D-5 drillhole. Západné Karpaty, sér. Paleontológia 12, 69–80.
Songtham, W., Ralanasthien, B., Watanasa, M., Mildenhalf, D. C., Singhamjwampar, S., and Kandharosa, W. (2005). Tertiary basin evolution in northern Thailand: a palynological point of view. Nat. Hist. Bull. Siam Soc. 53, 17–32.
Sri-indrasutdhi, V., Boonyuen, N., Suetrong, S., Chuaseeharonnachai, C., Sivichai, S., and Gareth Jones, E. B. (2010). Wood-inhabiting freshwater fungi from Thailand: Ascothailandia grenadoidia gen. et sp. nov., Canalisporium grenadoidia sp. nov. with a key to Canalisporium species (Sordariomycetes, Ascomycota). Mycoscience 51, 411–420. doi: 10.1007/S10267-010-0055-6
Steinthorsdottir, M., Coxall, H. K., de Boer, A. M., Huber, M., Barbolini, N., Bradshaw, C. D., et al. (2021). Paleoceanogr. Paleoclimatol. 36:e2020A004037.
Su, R., Kuehn, K. A., and Phipps, S. W. (2015). Fungal contributions to carbon flow and nutrient cycling during decomposition of standing Typha domingensis leaves in a subtropical freshwater marsh. Freshw. Biol. 60, 2100–2112. doi: 10.1111/fwb.12635
Talbot, J. M., Bruns, T. D., Taylor, J. W., Smith, D. P., Branco, S., Glassman, S. I., et al. (2014). Endemism and functional convergence across the North American soil mycobiome. Proc. Natl. Acad. Sci. U. S. A. 111, 6341–6346. doi: 10.1073/pnas.1402584111
Tedersoo, L., Bahram, M., Põlme, S., Kõljalg, U., Yorou, N. S., Wijesundera, R., et al. (2014). Global diversity and geography of soil fungi. Science 346:1256688.
Tewalt, S. J., Willett, J. C., and Finkelman, R. B. (2005). The World Coal Quality Inventory: a status report. Int. J. Coal Geol. 63, 190–194. doi: 10.1016/j.coal.2005.02.013
Thasod, Y., Ratanasthiena, B., Tanakab, S., Saegusac, H., and Nakaya, H. (2008). Fine-fraction Clays from Chiang Muan Mine, Phayao Province, Northern Thailand. Sci. Asia 33, 13–21.
Umali, T. E., Quimio, T. H., and Hyde, K. D. (1999). Endophytic fungi in leaves of Bambusa tuldoides. Fungal Sci. 14, 11–18.
Utescher, T., Bruch, A. A., Erdei, B., François, L., Ivanov, D., Jacques, F. M. B., et al. (2014). The coexistence approach–theoretical background and practical considerations of using plant fossils for climate quantification. Palaeogeogr. Palaeoclimatol. Palaeoecol. 410, 58–73. doi: 10.1016/j.palaeo.2014.05.031
van Geel, B., and Aptroot, A. (2006). Fossil ascomycetes in Quaternary deposits. Nova Hedwigia 82, 313–329. doi: 10.1127/0029-5035/2006/0082-0313
Větrovský, T., Kohout, P., Kopecký, M., et al. (2020). A meta-analysis of global fungal distribution reveals climate-driven patterns. Nat. Commun. 10:5142. doi: 10.1038/s41467-019-13164-8
Willis, K. J. (ed.) (2018). State of the World’s Fungi 2018. Report. Kew: Royal Botanic Gardens, 88.
World Coal Quality Inventory (2019). U.S. Geological Survey Geology, Energy, Minerals Science Center Data and Tools. Available online at: https://www.usgs.gov/centers/gemsc/science/world-coal-quality-inventory?qt-science_center_objects=0#qt-science_center_objects (Accessed August 19, 2021)
Yabe, A. (2002). Paleoclimatic conditions inferred from the Tertiary plant megafossil assemblages from northern Thailand. Primate Res. 18, 143–157. doi: 10.2354/psj.18.143
Yang, J., Liu, J. K., Hyde, K. D., Jones, E. G., and Liu, Z. Y. (2018). New species in Dictyosporium, new combinations in Dictyocheirospora and an updated backbone tree for Dictyosporiaceae. MycoKeys 36:83. doi: 10.3897/mycokeys.36.27051
Yip, H. Y. (1988). Berkleasmium correae sp. novo on leaf hairs of Correa lawrenciana. Australas. Plant Pathol. 17, 31–33. doi: 10.1071/app9880031
Zhao, G. Z., Liu, X. Z., and Wu, W. P. (2007). Helicosporous hyphomycetes from China. Fungal Divers. 26, 313–524.
Keywords: MMCO, Slovakia, Thailand, fossil fungi, lignite, palynology
Citation: Romero IC, Nuñez Otaño NB, Gibson ME, Spears TM, Fairchild CJ, Tarlton L, Jones S, Belkin HE, Warny S, Pound MJ and O’Keefe JMK (2021) First Record of Fungal Diversity in the Tropical and Warm-Temperate Middle Miocene Climate Optimum Forests of Eurasia. Front. For. Glob. Change 4:768405. doi: 10.3389/ffgc.2021.768405
Received: 31 August 2021; Accepted: 08 November 2021;
Published: 10 December 2021.
Edited by:
Adriana Lucia Romero-Olivares, New Mexico State University, United StatesReviewed by:
Lorena Musotto, CONICET Instituto Geológico del Sur (INGEOSUR), ArgentinaJordi Revelles, Institut Català de Paleoecologia Humana i Evolució Social (IPHES), Spain
Mario Carlos Saparrat, National University of La Plata, Argentina
Copyright © 2021 Romero, Nuñez Otaño, Gibson, Spears, Fairchild, Tarlton, Jones, Belkin, Warny, Pound and O’Keefe. This is an open-access article distributed under the terms of the Creative Commons Attribution License (CC BY). The use, distribution or reproduction in other forums is permitted, provided the original author(s) and the copyright owner(s) are credited and that the original publication in this journal is cited, in accordance with accepted academic practice. No use, distribution or reproduction is permitted which does not comply with these terms.
*Correspondence: Jennifer M. K. O’Keefe, ai5va2VlZmVAbW9yZWhlYWRzdGF0ZS5lZHU=; Ingrid C. Romero, aW5jYXJvbWVyb0BnbWFpbC5jb20=
†These authors share first authorship
‡These authors share last authorship