- 1Functional Ecology of Plants, Institute of Biology and Environmental Sciences, Carl von Ossietzky University, Oldenburg, Germany
- 2Smithsonian Tropical Research Institute, Balboa, Panama
- 3Department of Functional Morphology and Biomechanics, Zoological Institute, Kiel University, Kiel, Germany
Plants and animals evolve different attachment structures and strategies for reversible or permanent adhesion to different substrate types. For vascular epiphytes, having the ability to permanently attach to their host plants is essential for establishment and survival. Unlike mistletoe roots, roots of vascular epiphytes do not penetrate the host tissues but instead achieve attachment by growing in close contact to the surface of the substrate. However, the fundamental understanding of the attachment functions of epiphytic roots remains scarce, where majority of studies focused on the general root morphology, their functional properties and the descriptions of associated microbial endophytes. To date, research on attachment strategies in plants is almost entirely limited to climbers. Therefore, this study aims to fill the knowledge gap and elucidate the attachment functions of roots of epiphytic orchids. With the use of histology and high-resolution cryo-scanning electron microscopy (cryo-SEM) technique with freeze fracturing, the intimate root-bark substrate interface of epiphytic orchid Epidendrum nocturnum Jacq was investigated. Results showed a flattened underside of the root upon contact with the substrate surface, and the velamen layer appeared to behave like a soft foam, closely following the contours of the substrate. Root hairs emerged from the outermost velamen layer and entered into the crevices in the substrate, whenever possible. A layer of amorphous substance (glue-like substance) was observed on the surface of the root hairs. Combining the observations from this study and knowledge from previous studies, we hypothesised that epiphytic orchid roots produced a layer of glue-like substance to adhere the root to the substrate. Then root hairs are produced and enter into the voids and crevices of the substrate. This further generates a mechanical interlocking mechanism between root and substrate, thus reinforcing the attachment of the root (and hence the whole plant) to its substrate.
Introduction
Attachment structures and strategies that evolved in animals and plants allow reversible or permanent adhesion under diverse environmental conditions and/or to different substrate types (Gorb, 2008). Attachment is achieved by a diversity of biological structures: hooks and spikes (Darwin, 1875; Rowe and Isnard, 2009), smooth pads (Gorb, 2001, 2007; Drechsler and Federle, 2006; Barnes, 2007), thin hairs (Autumn et al., 2002; Geim et al., 2003; Gao et al., 2005), suction cups (Ditsche and Summers, 2019), byssus plaques (Silverman and Roberto, 2007), or attachment pads on adventitious roots (Groot et al., 2003; Seidelmann et al., 2012). As summarized in the review of Gorb (2008), these structures fulfill different biological functions including: (1) firm anchorage for stability (e.g., Walker, 1981; Flammang, 2006); (2) reversible adhesion for effective locomotion (e.g., Irschick et al., 1996; Federle and Endlein, 2004; Tian et al., 2006); (3) firm grip on prey (e.g., Bauer and Kredler, 1988; Betz and Kölsch, 2004); (4) temporary attachment for copulation (e.g., Aiken and Khan, 1992; Voigt et al., 2017); or (5) attachment to a surface in the context of, e.g., pollination or dispersal (Kiviniemi, 1996; Krenn et al., 2005). Both the first and last function aptly apply to the lifeform of vascular epiphytes since they are permanently attached to their host plants (Zotz, 2016).
Epiphytes are, by definition, non-parasitic plants structurally dependent on trees. This lifeform takes advantage of previously unexploited spatial resources (Lüttge, 2007) such as crotches and branches in a tree canopy. Apart from having to cope with the low and intermittent supply of water and – to a lesser degree – nutrients associated with epiphytism (Zotz et al., 2021b), a major challenge for epiphytes is to stay attached to their hosts. Compared to terrestrial plants that develop deep root systems for stable anchorage (Coutts, 1983; Ennos, 2000), many epiphytes grow on bare bark with little to no substrate. Unlike mistletoe roots, roots of vascular epiphytes do not penetrate host tissue, but typically achieve attachment by growing in close contact to the surface of the substrate. Secured attachment is vital for the survival of epiphytes, because the chances of survival on the ground are low (Matelson et al., 1993) in case of dislodgement by wind or heavy rain (Rodríguez-Robles et al., 1990; Tremblay, 2008). Surprisingly, the fundamental understanding of how these plants achieve attachment to their host via their roots is still lacking. Most of the available studies on epiphytic roots focus on general root morphology (e.g., Benzing et al., 1982; Brighigna et al., 1990; Oliveira and Sajo, 1999; Moreira and Isaias, 2008; Thangavelu and Ayyasamy, 2017), functional properties such as the water and nutrients absorption capacity of the orchid velamen (e.g., Pridgeon, 1987; Zotz and Winkler, 2013; Joca et al., 2017), and descriptions of associated microbial endophytes (e.g., Bernard, 1911; Pereira et al., 2003; Tsavkelova et al., 2007; Sathiyadash et al., 2012).
To date, research on attachment strategies in plants is almost entirely limited to climbers (Isnard and Silk, 2009). Climbing is achieved via different devices or mechanisms, such as twining (e.g., Ipomoea purpurea; Scher et al., 2001), by hooks (e.g., Galium aparine; Bauer et al., 2011), by tendrils (e.g., Parthenocissus tricuspidata; Steinbrecher et al., 2010), or with adventitious pads originating from roots (e.g., Hedera helix; Melzer et al., 2010). Several attachment systems of climbers from various families are well-characterized: for example, Moraceae (Ficus pumila; Groot et al., 2003), Araliaceae (H. helix; Zhang et al., 2008; Melzer et al., 2010, 2011), Vitaceae (Parthenocissus quinquefolia, P. tricuspidata; Scherge and Gorb, 2001; Bowling and Vaughn, 2008; Steinbrecher et al., 2010, respectively), Bignoniaceae (Amphilophium crucigerum; Seidelmann et al., 2012), and Passifloraceae (Passiflora discophora; Bohn et al., 2015). Most recently, the attachment mechanism of seagrass using a combination of mechanical interlocking of subdividing root hairs and glue-like substances was described (Posidonia oceanica; Zenone et al., 2020a,b).
Considering that epiphytes attach via their roots to the host plant, their attachment mechanism may be similar to that of root climbers and seagrasses that adhere to either bare bark or rocks. Hence, their adhesion strategies might shed some light on the attachment mechanism of epiphytes. For example, Melzer et al. (2011) state that the root climber English Ivy H. helix attaches to nearly any surface via natural-forming glue and shape-changing root hairs. On the underside of its stem, ivy produces numerous adventitious roots with root hairs that secret a glue-like substance, allowing the plant to adhere onto surfaces, such as tree bark, rocks or walls. For a permanently secured hold to the substrate, the root hairs apparently take on a spirally cracked shape and interlock themselves within the crevices of the substrate (Melzer et al., 2011). Spirally cracked root hairs were also reported in the climber Syngonium podophyllum (Yang and Deng, 2017), again suggesting a link between these structures and anchorage. Spirally cracked root hairs have also been found in seagrasses P. oceanica (Tomasello et al., 2018). In P. tricuspidata, the attachment pads appear to adapt their surface to the substrate geometry and exude an adhesive fluid that fills any space underneath the pad for adhesion to the substrate (Scherge and Gorb, 2001; Steinbrecher et al., 2011). Finally, Zenone et al. (2020b) found that the root hair tips of seagrasses branch and form a pad-like structure, increasing the contact area with the corrugated rock surface. Additionally, a glue-like substance fills gaps between the pad base and the substrate, which further enhances contact area and increases adhesion. However, further investigations are necessary to validate the functions of the various root hair forms (i.e., branches and spirals): do they also increase nutrients absorption by increasing root hair surface-volume ratio, and do they only enhance root contact to the substrate?
Orchidaceae is one of the largest families of flowering plants (Dressler, 1993), with 75% of all the species in the family being epiphytic, constituting the largest percentage (68%) of all epiphytes (including hemiepiphytes) (Zotz et al., 2021c). Epiphytic orchids produce roots that either remain aerial (i.e., free-hanging and not attached) or attach to a substrate. Several studies noted that attached roots are no longer spherical in shape: roots become hemispherical, with one side flattened to follow the contours of the substrate, with root hairs being restricted to the side in contact with the substrate (e.g., Dycus and Knudson, 1957; Stern and Judd, 1999; Stern, 2014; Ponert et al., 2016; Thangavelu and Ayyasamy, 2017; Balachandar et al., 2019a; Deseo et al., 2020). Root hairs of epiphytic orchids have been reported in older parts of the root as well (Dycus and Knudson, 1957; Almeida et al., 2016), further indicating that the root hairs may have other functions beside nutrient and water uptake. Orchid root hairs also exist in various forms, apart from the usual cylindrical shape: there are branched, spiraled and club-shaped thickened versions of root hairs (Leitgeb, 1865; Balachandar et al., 2019a). Spirally cracked root hairs form mainly via the rupturing of the outer cell wall layer, which subsequently causes the inner wall layer to stretch and break (Bernal et al., 2015). However, the phenomenon may also be an artifact following the forceful detachment of roots from their substrate. Some authors hypothesized that these spiral root hairs aid in capillary water absorption or in improved adherence to the substrate (Bernal et al., 2015; Almeida et al., 2016). The similarities with climbing plants and seagrasses (i.e., root hairs on root surface contact substrate; presence of spiral-shaped root hairs) are good starting points to study the attachment mechanism of epiphytic orchids.
Given the lack of reports demonstrating exactly how orchid roots are attached to their host plant, the present study aimed at filling that knowledge gap and elucidate the attachment functions and mechanism of roots of epiphytic orchid. With the use of histology and a high-resolution cryo-scanning electron microscopy (cryo-SEM) technique combined with freeze fracturing, we directly studied the intimate interface between the root and its substrate. From these images and knowledge from previous publications, we propose a schematic diagram, to summarise our hypothesis of how epiphytic orchid roots (and hence the whole plant) adhere to their substrates.
Materials and Methods
Plant Materials
Fieldwork was conducted in April 2019 in the Barro Colorado Natural Monument on Lake Gatun, Panama. To collect root samples for microscopy analyses, three adult epiphytic orchids, Epidendrum nocturnum Jacq attached to different Annona glabra tree branches were identified (Figure 1A). A. glabra are restricted to the marshes around the coast and islands, and the trees have a short stature, rarely growing to a height of more than 7 m (Croat, 1978) and have a relatively open crown structure. The trees were accessed with a boat for samples collection, therefore the roots were mainly collected from orchids which were maximum 2 m above the boat. For each plant, one root (with 5 cm measured from the root cap discarded) was collected together with the bark substrate still attached. The remaining roots were about 5 cm long and were further divided into three parts, ca. 1.5 cm each, totaling nine pieces of root segments (Figure 1B). To examine if free hanging orchid roots had any root hair development, roots that were not attached to any substrate were also collected from another three independent E. nocturnum plants. The roots were prepared into nine root segments as stated above. These root segments were preserved in individual Eppendorf tubes with 50% ethanol for export to Germany, where further microscopy analyses were carried out.
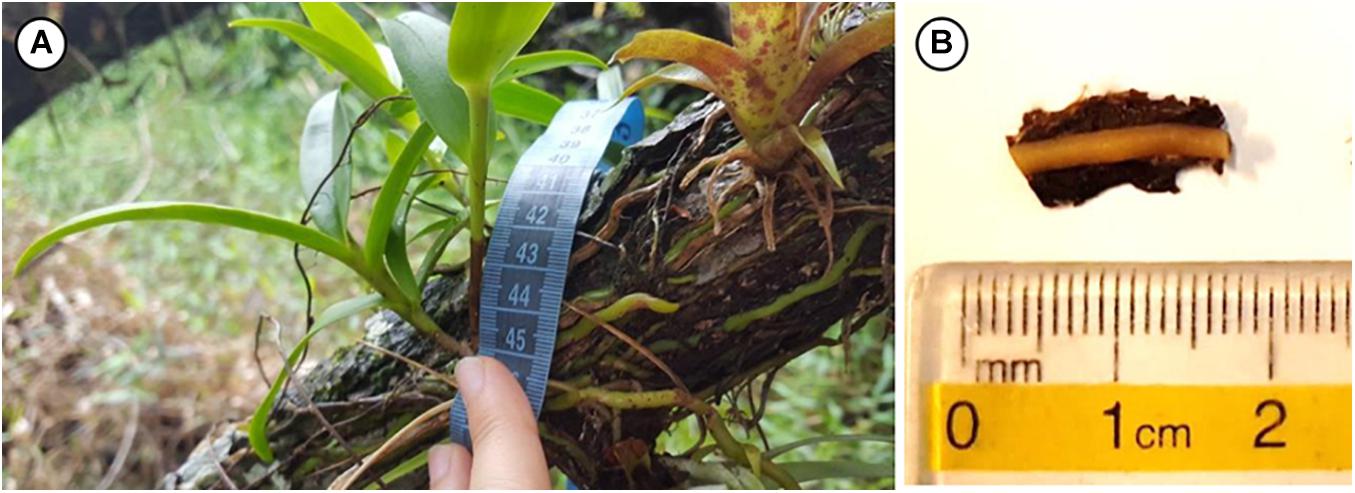
Figure 1. (A) Epidendrum nocturnum in its natural habitat in Panama. The green fleshy roots are growing on the surface of the substrate. (B) An example of a root segment that was collected and preserved in 50% ethanol.
Initial Examination of Roots – Freehand Thin Sections
To examine if there were any of root hairs on the orchid roots, both attached and free hanging root segments were examined. Three root segments from each group were randomly selected to perform the freehand cuts. For the root segments with attached substrate, the substrates were carefully removed to better visualize any root hairs that were growing onto the substrate. Freehand cuts were prepared with a disposable razor blade. The cuts were bleached for 30 min in a solution of 2% NaClO to clear cell contents. Next, the cuts were rinsed three times in distilled water and then stained for 1 min with safranin (0.1 g in 100 ml deionized water) to stain lignified/suberized cell walls red. These cuts were further soaked for 2–3 h in 70% ethanol to remove excess stain. Fifteen cuts from each group (free-hanging and attached roots) were randomly selected and were viewed at 2–8× zoom ratio with a stereo microscope Olympus Type SZX16 (objective lens total magnification 2.1–690×, Olympus, Japan). Pictures were taken with a connected digital camera (Olympus UC30, 3.2 megapixel, Olympus, Japan).
Histology – TECHNOVIT®
To examine the root hair-substrate interface and to understand how the root is attached to the substrate, histological examinations of the root hair-substrate cross-sections were carried out. To prepare the samples for histological examinations, another three root segments of E. nocturnum (with attached substrate) were selected and further sliced into 0.2 cm root sections. This was to ensure that the root sections were as small as possible for effective dehydration and infiltration of the resin into the root and bark cells. During the process of cutting the root segments into smaller sections, some bark substrates were separated from the root. Among those roots that were still attached to a piece of bark substrate, 10 pieces were randomly selected for infiltration and embedding in TECHNOVIT® 7100 (Kulzer GmbH, Wehrheim, Germany). TECHNOVIT® is a polymerization system based on 2-hydroxyethyl methacrylate that remains transparent after polymerization. The root segments were already preserved in 50% ethanol, therefore, further dehydration proceeded in an ascending ethanol concentration series as the following: 70, 90, 96, 96, and 99% EtOH, changed every other hour, last step overnight. Then, the samples were pre-infiltrated with a solution made from equal parts of 99% EtOH and TECHNOVIT® 7100, at 4°C overnight. If samples were floating after the change in ethanol/pre-infiltration solution, they were placed in a vacuum desiccator for at least 10 min (or until samples fall to the bottom of the Eppendorf tube), to remove all moisture within the samples. Lastly, samples were infiltrated with a solution made of TECHNOVIT® 7100 and Hardener 1, at 4°C overnight. This step was repeated to ensure that the bark substrate was fully infiltrated. For polymerization, the infiltrated samples were arranged carefully into the Teflon Histoform embedding cavities (Size: S, Kulzer GmbH, Wehrheim, Germany), and filled with 0.7 ml TECHNOVIT® polymerization solution each. Polymerization was completed in 2 h at room temperature. To release the polymerized blocks from the mold, a Histobloc carrier part was glued to the polymerized blocks with TECHNOVIT 3040 (2-component resin based on methyl-methacrylate) (Kulzer GmbH, Wehrheim, Germany).
The polymerized blocks (total 10 blocks) were clamped in a microtome (Reichert-Jung SUPERCUT 2050, Wetzlar, Germany). Thin sections (5 μm) of the blocks were cut by HISTOBLADES (Kulzer GmbH, Wehrheim, Germany). Firstly, the blocks were sliced at 8 μm to remove any uneven surfaces. Then, thin sections of 5 μm were made. During this process, it was common that thin sections were curling onto themselves and were unusable. Nonetheless, at least 10 undamaged sections of each block were collected in a water bath at 20–25°C. Thin sections were air dried for at least 2 h on microscope slides (VWR®), subsequently stained in 0.05% toluidine blue aq. (toluidine chloride 1272, Merck KGaA, Darmstadt, Germany) for 1 min, washed three times in distilled water for 30 s each, and air dried again overnight. Toluidine blue is a polychromatic dye used to stain carboxylated polysaccharides with pinkish purple; lignin and tannins with greenish blue or bright blue; and nucleic acids with purplish or greenish blue (O’Brien et al., 1964). Lastly, these thin sections were mounted in ENTELLAN® (107961, Merck KGaA, Darmstadt, Germany). Four microtome cuts from each of the 10 undamaged sections made were randomly selected and viewed at 4–25 × magnification (with a 10 × eyepiece magnification) using a light microscope POLYVAR Type 300602 (Reichert-Jung, Wetzlar, Germany). Pictures were taken with a connected camera (Olympus UC30, 3.2 megapixel, Olympus, Japan).
Cryo-Scanning Electron Microscopy
The remaining three root segments of E. nocturnum (with attached substrate) were prepared for cryo-SEM examination. The samples were shock-frozen (−140°C) to study the root-substrate interface in a SEM Hitachi S-4800 (Hitachi High-Technologies Corp., Tokyo, Japan) equipped with a Gatan ALTO 2500 cryo-preparation system (Gatan Inc., Abingdon, United Kingdom). For details of sample preparation and mounting for cryo-SEM, see Gorb and Gorb (2009). Cryo-SEM is useful, where preservation of the natural morphology of cells and tissues is desired. Additionally, it allows visualization of fluids, which might be crucial in the case of root-substrate attachment. Mounted root segments with bits of bark substrate attached were sputter-coated in frozen conditions with gold-palladium (thickness 10 nm) and examined at 3 kV acceleration voltage and temperature of −120°C in the microscope.
To ensure that the results of the cryo-SEM analyses were not artifacts due to the preparative procedures and long storage in ethanol, three 1 cm fresh root samples of another epiphytic orchid, Dendrobium koordersii J.J.Sm, mounted onto Robinia pseudoacacia bark, were collected in January 2021 in the greenhouse in the Botanical Garden Kiel, Germany. All plant names follow The Plant List (2013).
Results
The majority of the freehand cuts of the E. nocturnum roots (with attached substrate) displayed a semi-circular shape, with the underside of the root being flattened out to the substrate surface (Figures 2A,C). This was in comparison to the free-hanging roots that remained spherical in shape, without any flattened sides (Supplementary Figure 1). From the roots with attached substrate, root hairs were clearly present as extensions of the velamen layer, from the part of the root that was attached to the substrate (Figures 2B,D). However, on the free-hanging roots, no root hairs were observed in any of the cuts from the sample (Supplementary Figure 1). Both attached and free-hanging roots did not have a uniform number of velamen layers in the cross-sections (Figures 2A,C and Supplementary Figure 1).
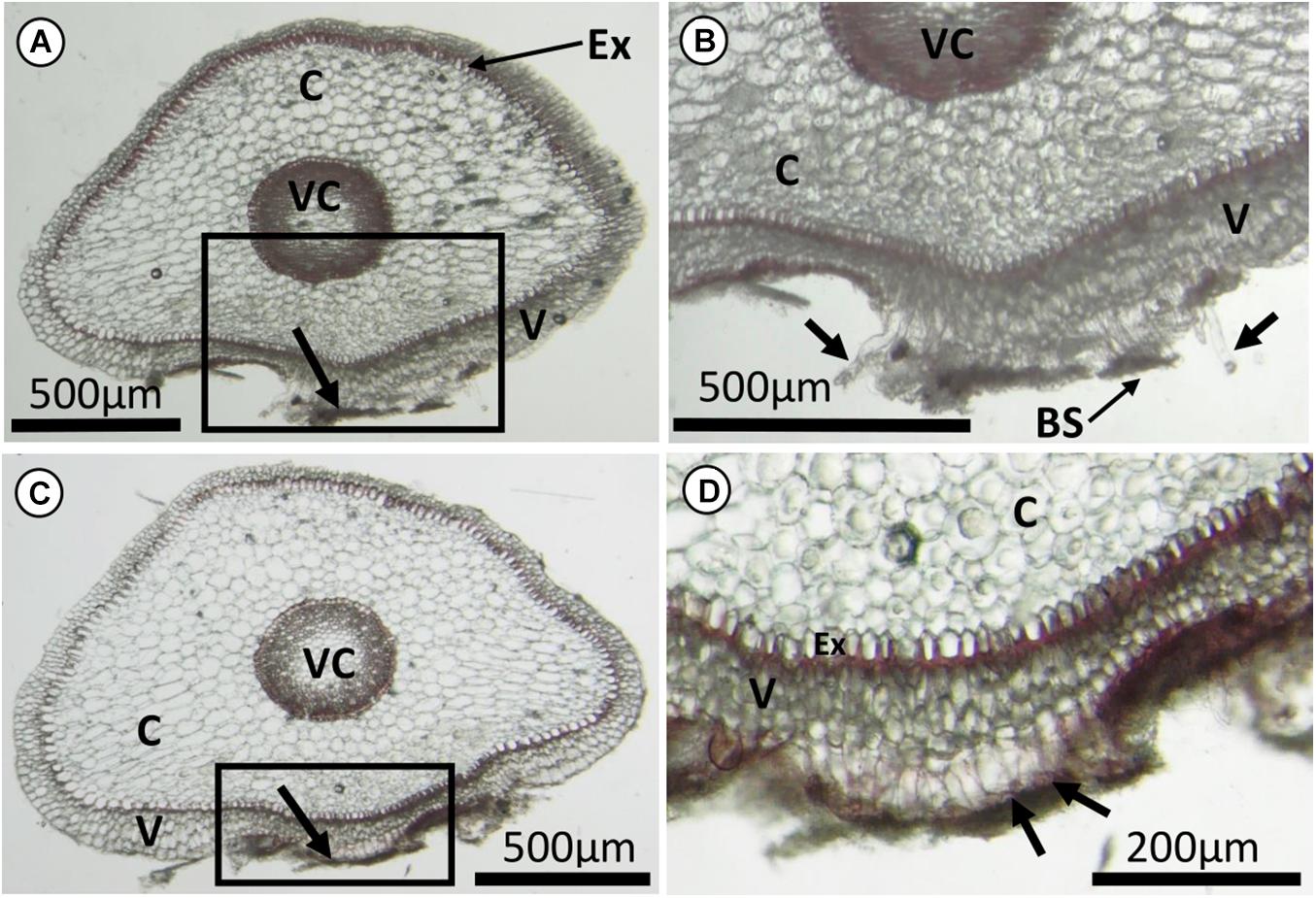
Figure 2. Freehand cuts of roots of Epidendrum nocturnum with most of the substrate removed. (A,C) Non-cylindrical root sections, that were flattened at the underside of the root in contact to the bark substrate (indicated by black arrows). (B,D) Enlarged images corresponding to the boxes in (A,C), respectively. There were extensions of tubular root hairs from the velamen layer. Some of the root hairs tips were probably broken off when the substrate was removed before making the cuts (black arrows in B), and some were still adhering to the substrate (black arrows in D). Ex, exodermis with U-thickened walls; C, cortical parenchyma; V, velamen; VC, vascular cylinder; BS, bark substrate.
To have a better illustration of how the root hairs interacts with the substrate, histological thin sections were examined. In some cases, the root and bark cells were not well infiltrated with Technovit, hence, those thin sections were not successful and there were incomplete cell structures (not shown). For those thin sections with successful infiltration, the root cells also showed a flattened side on the part that was in contact with the substrate (Figures 3A,B). Root hairs were emerging from the outermost velamen layer facing the substrate (Figures 3A,D,F,G), growing into the crevices of the substrate (Figures 3D,F). On the underside of the root, the outermost velamen layer appeared to behave like a soft foam, closely following the contours of the adhered substrate (Figures 2D, 3G).
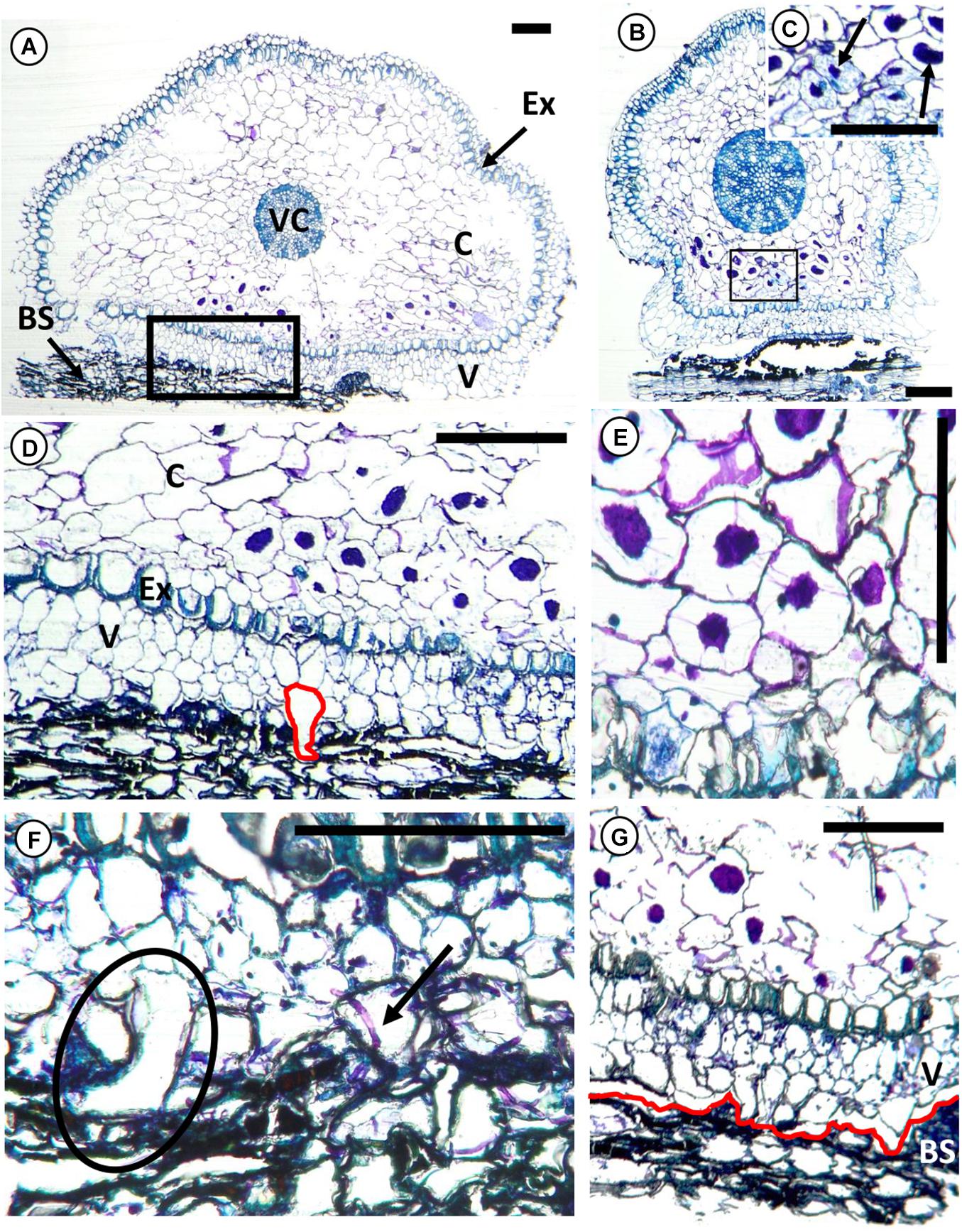
Figure 3. Transverse histological sections of root segment of Epidendrum nocturnum in contact with the bark substrate of the tree Annona glabra, with toluidine blue staining. Only the outermost portion of the phellem, is shown. (A,B) Non-cylindrical root segment with root hairs adhering to the bark substrate. (C) Cortex fungus (in association to the mycorrhizae) forming pelotons (arrows). They are mainly found in cells next to the substrate. (D) Root hairs enter crevices in the bark substrate. The root hair, highlighted in red, has a vertical length of 120 μm. (E) Adjacent cells with pelotons communicated by hyphae. (F) Root hair growing onto bark substrate (circle) and a piece of mycorrhizal hyphae extending from the bark substrate into the epidermal cells (arrow). (G) The outermost velamen layer replicated the contours of the substrate layer (highlighted in red). The root got separated from the bark substrate during the slicing procedure with the microtome. Ex, exodermis with U-thickened walls; C, cortical parenchyma; V, velamen; VC, vascular cylinder; BS, bark substrate. Scale bars, 200 μm.
For the cryo-SEM observations, root segments were examined (Figure 4) and a layer of attached substrate was gently removed to reveal the root hairs underneath (Figures 4B,C). Comparing the preserved and fresh samples, the preserved samples had more micro-organisms observed on the root and substrate surfaces. For examples, many diatoms were seen on the E. nocturnum roots (Figure 4B) that came from Panama but not on the D. koordersii samples from the greenhouse in Kiel. Nonetheless, the samples were similar in terms of having root hairs distributed along the length of the root segments and root hairs interacting with the substrate. Root hairs were growing between the layers of the substrate and in the crevices wherever possible (Figures 4C–E and Supplementary Figures 2C–E). Root hair size and length varied: hairs stop growing and flattened out at the tip once contacting the substrate (Figure 4F and Supplementary Figure 2E). A layer of amorphous substance (“glue-like substance”) was observed to be coated on the surface of the root hair (Figures 4F,G and Supplementary Figures 2F,G) and it appeared to be a very thin layer. On top of this glue-like layer, there were pieces of bark substrate that were either adhered directly on the glue or imprinted on the surface (Figure 4G). Root hairs of D. koordersii were also observed to bend and twist to hook onto smaller particles (Supplementary Figures 2D,E).
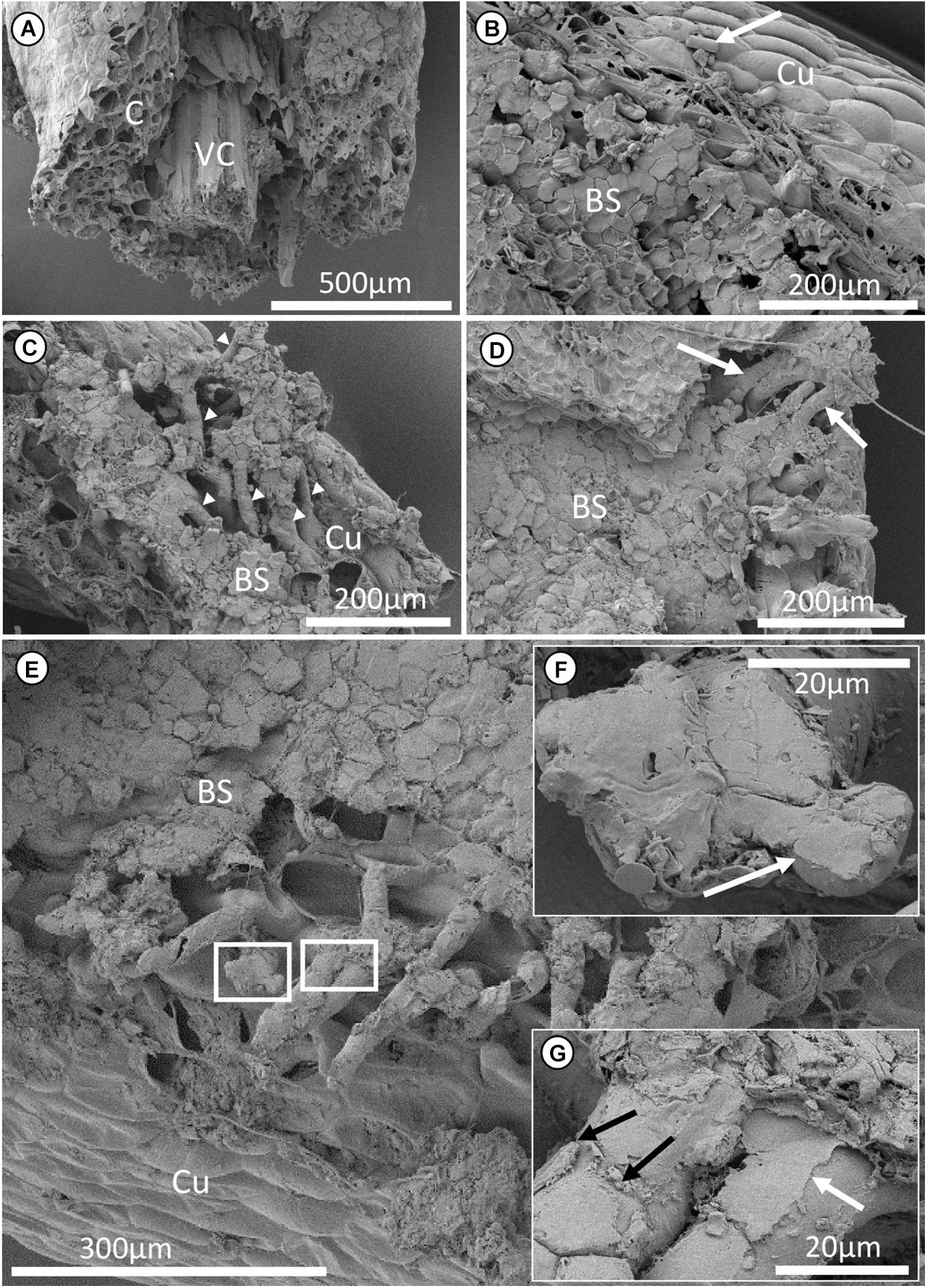
Figure 4. Cryo-scanning electron microscopy micrographs of a segment of Epidendrum nocturnum root attached to bark substrate of Annona glabra. (A) Transverse section of the root segment. (B–E) View of how root hairs are attached to the bark substrate. (B) Bark substrates are attached to the cuticle of the root. Some diatoms (together with other micro-organisms) can also be observed on the root surface. White arrow points at a diatom. (C,D) Root hairs growing between the crevices of the layers of bark substrate (white arrowheads and arrows). Pockets of hollow spaces are present between the root hairs. (F,G) Enlarged images corresponding to the boxes in (E). (F) The end of a single root hair that is flattened due to the extremely thin cell wall of the root hair, probably appressed to the substrate and molded according to the microstructure of the substrate. (G) A layer of amorphous substance (glue) is adhering to the root hair (white arrow, also labeled in F). Pieces of bark substrates were attached or imprinted (black arrows) to the root hairs. C, cortical parenchyma; VC, vascular cylinder; BS, bark substrate; Cu, cuticle.
The cell wall of the root hairs of E. nocturnum and D. koordersii was very thin ca. 1 μm (Figure 5A and Supplementary Figure 2B). This extremely thin cell wall allowed the root hairs to be flattened against the surface of the substrate and molded to the microstructures of the substrate (Figure 4F and Supplementary Figure 2E). A cracked root hair was observed in E. nocturnum (Figure 4E), and a more obvious spirally cracked root hair was observed in D. koordersii when the root hair was ruptured (Supplementary Figure 3). The ruptured area of the root hairs consisted of several broken layers of cell wall material (Figures 5B,C and Supplementary Figure 3).
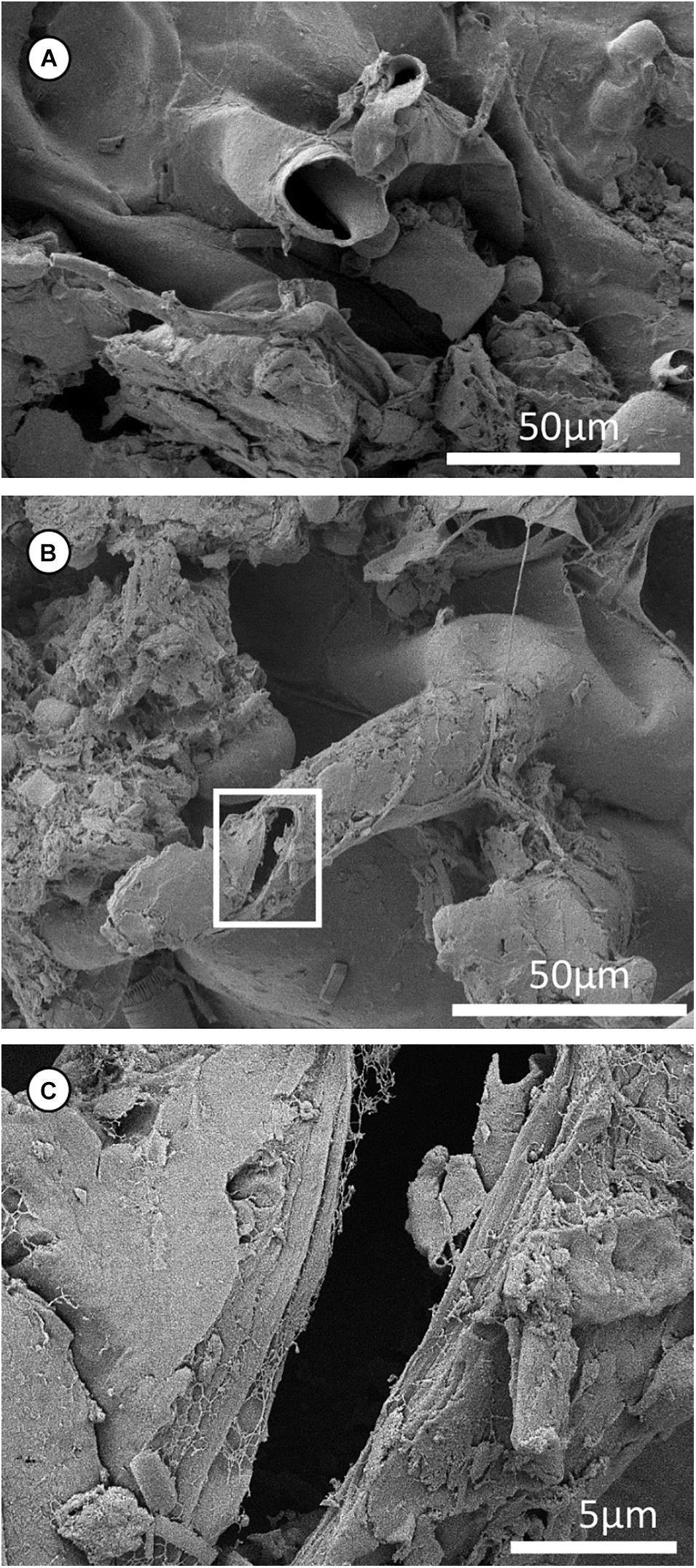
Figure 5. Cryo-scanning electron microscopy micrographs of a segment of Epidendrum nocturnum root hairs. (A) A piece of broken root hair; the cell wall of the root hair is very thin (i.e., <0.5 μm). (B) A single root hair that had a flattened tip and was broken with a crack. (C) Enlarged box in (B). Cracked root hair, with broken layers.
Discussion
In a typical ground-rooted terrestrial plant, root hairs are ephemeral and their growth is restricted to the area directly behind the zone of root elongation (Dickison, 2000; Grierson and Schiefelbein, 2002). The growth of root hairs is typically interpreted as an increase in surface area for nutrient uptake, microbe interactions and anchorage in the soil (Jungk, 2001; Grierson and Schiefelbein, 2002). The number and length of root hairs is largely regulated by the supply of mineral nutrients, in particular phosphate and nitrate (Foehse and Jungk, 1983; Bates and Lynch, 1996). In epiphytic orchids, however, root hairs are not restricted to the distal region of the root apex (Bibikova and Gilroy, 2002). Roots in epiphytic orchids are found along the length of the root, even in the older parts of the roots, which yields a large contact area for adhesion to the substrate. Studies found that contact to substrate is necessary for the development of root hairs since the presence of root hairs are only recorded on root surface facing the substrate (Groom, 1893; Chomicki et al., 2014; Thangavelu and Ayyasamy, 2017; Balachandar et al., 2019b). Free-hanging aerial roots remained spherical/cylindrical in shape without any root hair development (Supplementary Figure 1), while those attached to substrate were flattened on the side that was in contact to the substrate (Figures 2, 3). A flattened surface suggests a maximization of contact area for water and nutrient uptake (Thangavelu and Ayyasamy, 2017). Root hairs emerged from the outermost layer of the immature velamen in this flattened area. This is in agreement with other studies, where root hairs were also observed in the regions with direct contact to the substrate (Almeida et al., 2016; Thangavelu and Ayyasamy, 2017). It suggests that root hairs promote the attachment of orchids to its substrate by increasing the contact area of the root to the substrate.
We observed that there was a very tight contact between root and substrate, where the outermost velamen layer acts like a soft foam, molding closely onto the contours of the substrate for effective adhesion (Figures 2D, 3G). Then, there are root hairs, which have very thin cell walls (i.e., ca. 0.5 μm, measured from Figure 5A). Literature data for comparison are very limited. For example, cell wall thickness of young fresh root hairs of Lyginia barbata is similar with 0.5 μm (Shane et al., 2011), but P. oceanica have root hair cell wall thickness of 1.07 ± 0.15 μm (Zenone et al., 2020a). Given the differences in the type of environments and habitats that these plants grow in, the root hairs also serve different purposes. In the case of epiphytic orchid root hairs with the function of adhesion, having a thin cell wall would allow the root hairs to be readily flattened against the substrate or to protrude into voids, crevices and spaces between the layers of substrate (Figure 4D and Supplementary Figures 2D,E). This most likely promotes attachment as a mechanical interlocking mechanism between root and substrate. In the SEM images, an amorphous substance was observed to coat the surface of the root hairs (Figure 4G and Supplementary Figure 2F). This glue-like substance probably helps to hold the root in place. The presence of glue had also been reported in epiphytic ferns (Moran unpublished observation in Zotz et al., 2021a), in climbing figs, root climbers and in seagrasses (Groot et al., 2003; Melzer et al., 2011; Steinbrecher et al., 2011; Huang et al., 2016; Zenone et al., 2020b). Melzer et al. (2009) stated that English Ivy’s root hairs exude a glue-like substance as roundish excretes. Further analysis is needed to confirm if this is also true for orchid roots. Nonetheless, the repeated observation of glues on root hairs of epiphytic and climbing plants suggests that there may be a “universal” mechanism for attachment of non-parasitic plants to a host.
To remain securely attached to their host plants, epiphytic orchids have to withstand gravity and mechanical disturbances, e.g., wind. Principally, on very rough bark with deep fissures, when the whole root can grow into gaps, effective orchid root attachment to the substrate might be possible without root hairs. However, especially on smooth bark, which lacks larger fissures, the growth of many fine root hairs into microscale crevices on the surface provides reinforcement of attachment by mechanical interlocking, which may be critical for a strong and secure attachment. Root hairs were observed along the length of the root, in between the layers of substrates (Figures 3D,F, 4C), and they were present in different sizes and lengths (Figure 4E and Supplementary Figure 2C). Therefore, this further implies that root hairs were growing into voids and crevices, whenever present, to increase the overall contact of the root to the substrate.
Some studies placed emphasis on the spirally cracked root hairs, stating that the shape change in the root hairs played a role in providing stronger attachment of the plant to their substrate and also facilitating water uptake by capillarity (Melzer et al., 2010; Almeida et al., 2016; Yang and Deng, 2017). However, none of these additional functions has yet been shown experimentally. There is no consensus on how these spirally cracked roots are formed. One possibility is the rupturing of the outer cell wall of the root hair (caused by external force), followed by stretching and breakage of the inner walls (Bernal et al., 2015). Alternatively, it could simply result from the helical arrangement of the cellulose microfibrils in the cell wall, inducing the spiral form when the cells stretch due to growth, by some form of pressure (Frei and Preston, 1961; Lloyd and Chan, 2002; Smyth, 2016), or when root hairs dry out and shrink (Melzer et al., 2010). Yang and Deng (2017) argued that the spiral shaped root hairs in climber plant S. podophyllum have two functions. Firstly, ruptured cell walls of the root hairs release a glue-like substance that eventually helps in the adherence of root and substrate. Secondly, the spirally cracked root hairs act as microsprings for shock absorption when the root is loaded by external forces. For example, when the root is pulled, root hairs extend and crack helically to allow for more flexible elongation of the cell, which helps in dissipating energy to prevent complete detachment of the plant from its substrate. From this study, a cracked root hair was observed in E. nocturnum (Figure 5B) and a slightly spiral shaped root hair was observed in D. koordersii (Supplementary Figure 3). Although none of the observed cracks followed the length of the root hair or were clearly orthogonal to it but the cracks were always angled to the length of the root hair, and this may eventually cause the root hair to have a spiral shape. The spirally cracked root hair seemed to result from the rupturing of several cell layers on different points along the root hair (Supplementary Figure 3A), which may eventually cause the root hair to break completely and twist spirally, as suggested by Bernal et al. (2015). However, the presence of spirally cracked root hairs was only scarcely observed in this study. While the arguments regarding the origin and function of spiral root hairs may also be plausible in the case of epiphytic orchids, a larger sample size and further physiological and ontogenetic studies with orchids are necessary to put these ideas to a rigorous test.
Conclusion
This study focused at the root hair-substrate interface, with the combination of freehand cuts, histological sectioning and cryo-SEM techniques, to elucidate the attachment mechanism of epiphytic orchids to the bark substrate of their host plants. This study provides an in-depth, thorough understanding of how epiphytic orchids attach to their hosts via their roots. A glue-like substance is observed on the surface of the root hairs, and that root hairs enter the crevices of the substrate suggesting a mechanical interlocking mechanism between the root and the substrate for a more secure anchorage. Based on the knowledge from previous studies and the new observations from this study, we suggest a schematic diagram that summarizes our current understanding and our hypothesis on how epiphytic orchids attach to their substrate via their roots. This diagram illustrates only the attachment of orchid roots on relatively smooth bark substrates (without deep fissures) with microscale crevices on the surface (Figure 6):
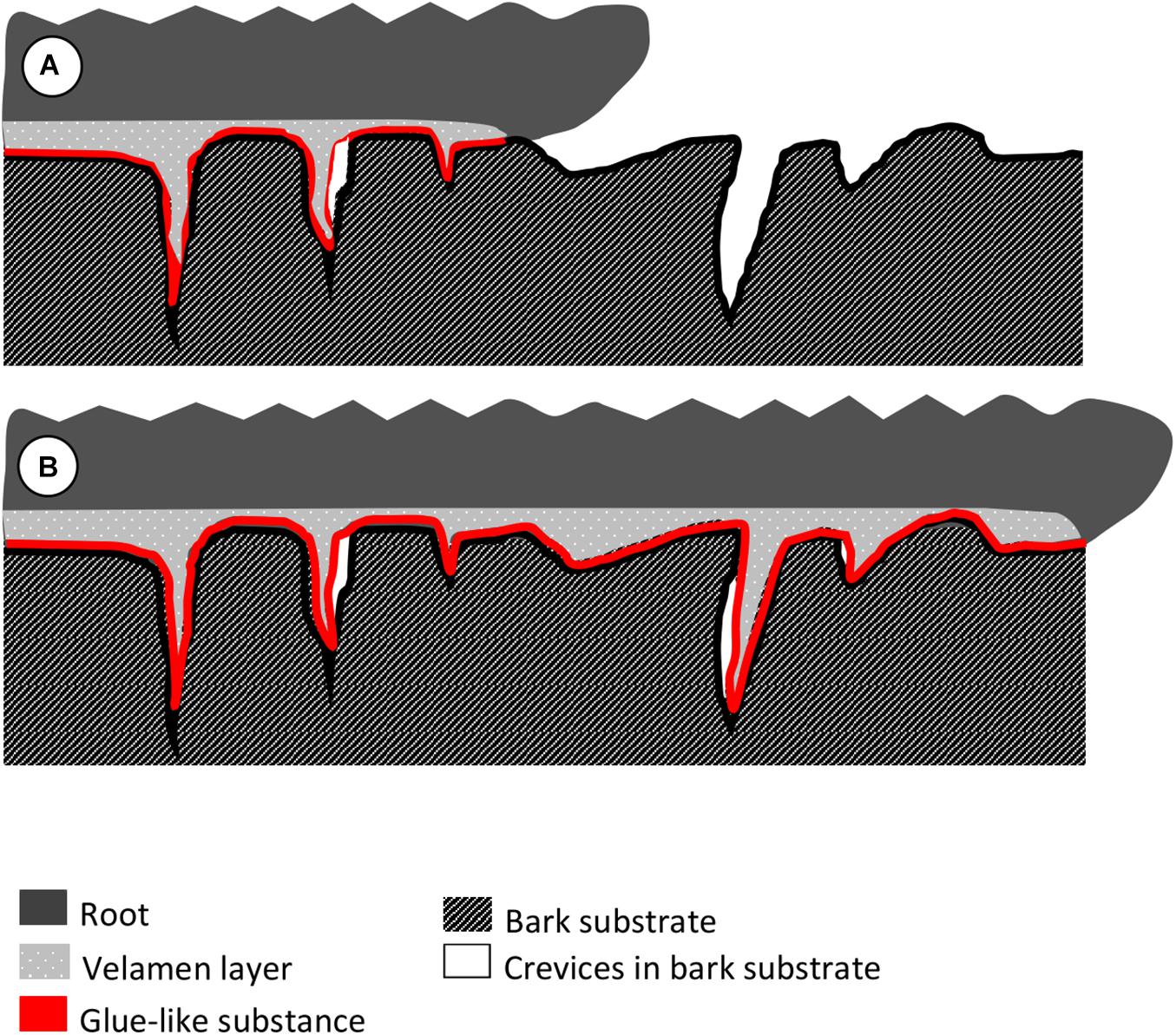
Figure 6. Hypothesis on how epiphytic orchids attach to their substrate via their roots as deduced from the observations. (A) The underside of the root, which is in contact to the substrate, produces root hairs from the outer velamen layer. The outermost velamen layer resembles a foam and molds closely to the microstructures of the substrate. A layer of glue-like substance is in between the root and the substrate, which could help the root adhere to the substrate. (B) With continued root growth along the length of the substrate, root hairs persist in the cavities, generating additional long-lasting mechanical interlocking on top of the adherence via glue.
a) The underside of the root, which is in contact to the substrate, produces root hairs from the outer velamen layer. The outermost velamen layer acts like a foam and molds closely to the microstructures of the substrate. In addition, there is a layer of glue-like substance between root and substrate, which could be an additional help for the root to adhere to the substrate.
b) As the root grows along the substrate, root hairs continue to grow into voids and crevices on the substrate, and generate an additional mechanical interlocking mechanism between root and substrate, further reinforcing the attachment of the root (and the whole plant itself), to the host. Meanwhile, old root hairs maintain attachment along the entire length of the root.
Data Availability Statement
The raw data supporting the conclusions of this article will be made available by the authors, without undue reservation.
Author Contributions
GZ, HE, and JT conceived and designed the research. SG contributed analytical tools. JT conducted the experiments and analysis, analyzed the data, and wrote the manuscript. GZ, SG, and HE revised the manuscript. All authors read and approved the final manuscript.
Funding
This work was supported by a grant from the German Research Foundation (Grant number El 1092/1-1). (https://www.dfg.de).
Conflict of Interest
The authors declare that the research was conducted in the absence of any commercial or financial relationships that could be construed as a potential conflict of interest.
Publisher’s Note
All claims expressed in this article are solely those of the authors and do not necessarily represent those of their affiliated organizations, or those of the publisher, the editors and the reviewers. Any product that may be evaluated in this article, or claim that may be made by its manufacturer, is not guaranteed or endorsed by the publisher.
Acknowledgments
Permission to work in the Barro Colorado Nature Monument was given by the Smithsonian Tropical Research Institute. A permit to export plants was granted by the Panamanian authorities (SEX/P-3-19). We are grateful to Dietrich Ober for access to the Botanical Garden Kiel and making their greenhouse collections available for this study. We thank Esther Appel from the Functional Morphology and Biomechanics group in Kiel for preparing the samples. We also thank the two reviewers for their comments to improve the manuscript.
Supplementary Material
The Supplementary Material for this article can be found online at: https://www.frontiersin.org/articles/10.3389/ffgc.2021.764357/full#supplementary-material
References
Aiken, R., and Khan, A. (1992). The adhesive strength of the palettes of males of a boreal water beetle, Dytiscus alaskanus J. Balfour Browne (Coleoptera: Dytiscidae). Can. J. Zool. 70, 1321–1324. doi: 10.1139/z92-185
Almeida, A. B. R., Smidt, E. C., and Amano, E. (2016). Development and function of root hairs in Acianthera Scheidw. (Orchidaceae: Pleurothallidinae). Aust. J. Basic Appl. Sci. 10, 122–126.
Autumn, K., Sitti, M., Liang, Y. A., Peattie, A. M., Hansen, W. R., Sponberg, S., et al. (2002). Evidence for van der waals adhesion in gecko setae. Proc. Natl. Acad. Sci. U.S.A. 99, 12252–12256. doi: 10.1073/pnas.192252799
Balachandar, M., Ravi, R. K., Nagaraj, K., and Muthukumar, T. (2019a). Vegetative anatomy and mycorrhizal morphology of Schoenorchis nivea (Lindl.) Schltr., (Orchidaceae) and their adaptive significance. Acta Biol. Szegediensis 63, 1–13. doi: 10.14232/abs.2019.1.1-13
Balachandar, M., Ravi, R. K., Ranjithamani, A., and Muthukumar, T. (2019b). Comparative vegetative anatomy and mycorrhizal morphology of three South Indian Luisia species (Orchidaceae) with the note on their epiphytic adaptations. Flora 251, 39–61.
Barnes, W. J. P. (2007). Functional morphology and design constraints of smooth adhesive pads. MRS Bull. 32, 479–485. doi: 10.1557/mrs2007.81
Bates, T., and Lynch, J. P. (1996). Stimulation of root hair elongation in Arabidopsis thaliana by low phosphorus availability. Plant Cell Environ. 19, 529–538. doi: 10.1111/j.1365-3040.1996.tb00386.x
Bauer, G., Klein, M.-C., Gorb, S. N., Speck, T., Voigt, D., and Gallenmüller, F. (2011). Always on the bright side: the climbing mechanism of Galium aparine. Proc. R. Soc B Biol. Sci. 278, 2233–2239. doi: 10.1098/rspb.2010.2038
Bauer, T., and Kredler, M. (1988). Adhesive mouthparts in a ground beetle larva (Coleoptera, Carabidae, Loricera pilicornis F.) and their function during predation. Zool. Anz. 221, 145–166.
Benzing, D., Ott, D., and Friedman, W. (1982). Roots of Sobralia macrantha (Orchidaceae): structure and function of the velamen-exodermis complex. Am. J. Bot. 69, 608–614. doi: 10.2307/2443070
Bernal, A. A., de Camargo Smidt, E., and Bona, C. (2015). Spiral root hairs in Spiranthinae (Cranichideae: Orchidaceae). Braz. J. Bot. 38, 411–415. doi: 10.1007/s40415-015-0141-2
Betz, O., and Kölsch, G. (2004). The role of adhesion in prey capture and predator defence in arthropods. Arthropod Struct. Dev. 33, 3–30. doi: 10.1016/j.asd.2003.10.002
Bibikova, T., and Gilroy, S. (2002). Root hair development. J. Plant Growth Regul. 21, 383–415. doi: 10.1007/s00344-003-0007-x
Bohn, H. F., Günther, F., Fink, S., and Speck, T. (2015). A passionate free climber: structural development and functional morphology of the adhesive tendrils in Passiflora discophora. Int. J. Plant Sci. 176, 294–305. doi: 10.1086/680231
Bowling, A., and Vaughn, K. (2008). Structural and immunocytochemical characterization of the adhesive tendril of Virginia creeper (Parthenocissus quinquefolia [L.] Planch.). Protoplasma 232:153. doi: 10.1007/s00709-008-0287-x
Brighigna, L., Fiordi, A. C., and Palandri, M. (1990). Structural comparison between free and anchored roots in Tillandsia (Bromeliaceae) species. Caryologia 43, 27–42. doi: 10.1080/00087114.1990.10796984
Chomicki, G., Bidel, L. P., and Jay-Allemand, C. (2014). Exodermis structure controls fungal invasion in the leafless epiphytic orchid Dendrophylax lindenii (Lindl.) Benth. ex Rolfe. Flora 209, 88–94.
Coutts, M. (1983). “Root architecture and tree stability,” in Tree Root Systems and Their Mycorrhizas, eds D. Atkinson, K. K. S. Bhat, M. P. Coutts, P. A. Mason, and D. J. Read (Dordrecht: Springer), 171–188.
Deseo, N. B., de Guzman, C. C., Nuevo, P. A., and Torreta, N. K. (2020). Morpho-anatomy of adventitious roots of vanilla (Vanilla planifolia Jacks. ex Andrews) during attachment to support post. Thailand Nat. Hist. Mus. J. 14, 31–36.
Ditsche, P., and Summers, A. (2019). Learning from Northern clingfish (Gobiesox maeandricus): bioinspired suction cups attach to rough surfaces. Philos. Trans. R. Soc. B 374:20190204. doi: 10.1098/rstb.2019.0204
Drechsler, P., and Federle, W. (2006). Biomechanics of smooth adhesive pads in insects: influence of tarsal secretion on attachment performance. J. Comp. Physiol. A 192, 1213–1222. doi: 10.1007/s00359-006-0150-5
Dressler, R. L. (1993). Phylogeny and Classification of the Orchid Family. Cambridge: Cambridge University Press.
Dycus, A. M., and Knudson, L. (1957). The role of the velamen of the aerial roots of orchids. Bot. Gaz. 119, 78–87.
Ennos, A. (2000). The mechanics of root anchorage. Adv. Bot. Res. 33, 133–157. doi: 10.1016/S0065-2296(00)33042-7
Federle, W., and Endlein, T. (2004). Locomotion and adhesion: dynamic control of adhesive surface contact in ants. Arthropod Struct. Dev. 33, 67–75. doi: 10.1016/j.asd.2003.11.001
Flammang, P. (2006). “Adhesive secretions in echinoderms: an overview,” in Biological Adhesives, eds A. M. Smith and J. A. Callow (Berlin: Springer), 183–206.
Foehse, D., and Jungk, A. (1983). Influence of phosphate and nitrate supply on root hair formation of rape, spinach and tomato plants. Plant Soil 74, 359–368.
Frei, E., and Preston, R. D. (1961). Cell wall organization and wall growth in the filamentous green algae Cladophora and Chaetomorpha II. Spiral structure and spiral growth. Proc. R. Soc. London. B Biol. Sci. 155, 55–77. doi: 10.1098/rspb.1961.0057
Gao, H., Wang, X., Yao, H., Gorb, S., and Arzt, E. (2005). Mechanics of hierarchical adhesion structures of geckos. Mech. Mater. 37, 275–285. doi: 10.1016/j.mechmat.2004.03.008
Geim, A. K., Dubonos, S., Grigorieva, I., Novoselov, K., Zhukov, A., and Shapoval, S. Y. (2003). Microfabricated adhesive mimicking gecko foot-hair. Nat. Mater. 2, 461–463. doi: 10.1038/nmat917
Gorb, E. V., and Gorb, S. N. (2009). “Functional surfaces in the pitcher of the carnivorous plant nepenthes alata: a cryo-sem approach,” in Functional Surfaces in Biology: Adhesion Related Phenomena, Vol. 2, ed. S. N. Gorb (Dordrecht: Springer), 205–238.
Gorb, S. N. (2007). Smooth attachment devices in insects: functional morphology and biomechanics. Adv. Insect Physiol. 34, 81–115. doi: 10.1016/S0065-2806(07)34002-2
Gorb, S. N. (2008). Biological attachment devices: exploring nature’s diversity for biomimetics. Philos. Trans. R. Soc. A Math. Phys. Eng. Sci. 366, 1557–1574. doi: 10.1098/rsta.2007.2172
Groot, E. P., Sweeney, E. J., and Rost, T. L. (2003). Development of the adhesive pad on climbing fig (Ficus pumila) stems from clusters of adventitious roots. Plant Soil 248, 85–96. doi: 10.1023/A:1022342025447
Huang, Y., Wang, Y., Tan, L., Sun, L., Petrosino, J., Cui, M.-Z., et al. (2016). Nanospherical arabinogalactan proteins are a key component of the high-strength adhesive secreted by English ivy. Proc. Natl. Acad. Sci. U.S.A. 113, E3193–E3202. doi: 10.1073/pnas.1600406113
Irschick, D. J., Austin, C. C., Petren, K., Fisher, R. N., Losos, J. B., and Ellers, O. (1996). A comparative analysis of clinging ability among pad-bearing lizards. Biol. J. Linn. Soc. 59, 21–35. doi: 10.1111/j.1095-8312.1996.tb01451.x
Isnard, S., and Silk, W. K. (2009). Moving with climbing plants from Charles Darwin’s time into the 21st century. Am. J. Bot. 96, 1205–1221. doi: 10.3732/ajb.0900045
Joca, T. A. C., de Oliveira, D. C., Zotz, G., Winkler, U., and Moreira, A. S. F. P. (2017). The velamen of epiphytic orchids: variation in structure and correlations with nutrient absorption. Flora 230, 66–74. doi: 10.1016/j.flora.2017.03.009
Jungk, A. (2001). Root hairs and the acquisition of plant nutrients from soil. J. Plant Nutr. Soil Sci. 164, 121–129.
Kiviniemi, K. (1996). A study of adhesive seed dispersal of three species under natural conditions. Acta Bot. Neerl. 45, 73–83. doi: 10.1111/j.1438-8677.1996.tb00496.x
Krenn, H. W., Plant, J. D., and Szucsich, N. U. (2005). Mouthparts of flower-visiting insects. Arthropod Struct. Dev. 34, 1–40. doi: 10.1016/j.asd.2004.10.002
Lloyd, C., and Chan, J. (2002). Helical microtubule arrays and spiral growth. Plant Cell 14, 2319–2324. doi: 10.1105/tpc.141030
Matelson, T. J., Nadkarni, N. M., and Longino, J. T. (1993). Longevity of fallen epiphytes in a neotropical montane forest. Ecology 74, 265–269. doi: 10.2307/1939523
Melzer, B., Seidel, R., Steinbrecher, T., and Speck, T. (2011). Structure, attachment properties, and ecological importance of the attachment system of English ivy (Hedera helix). J. Exp. Bot. 63, 191–201. doi: 10.1093/jxb/err260
Melzer, B., Steinbrecher, T., Seidel, R., Kraft, O., Schwaiger, R., and Speck, T. (2009). “Mechanics and structure of the attachment system of English ivy (Hedera helix L.),” in Proceedings of the 6th Plant Biomechanics Conference Cayenne, French Guyana, ed. B. Thibaut (Cayenne: ECOFOG), 205–210.
Melzer, B., Steinbrecher, T., Seidel, R., Kraft, O., Schwaiger, R., and Speck, T. (2010). The attachment strategy of English ivy: a complex mechanism acting on several hierarchical levels. J. R. Soc. Interface 7, 1383–1389. doi: 10.1098/rsif.2010.0140
Moreira, A. S. F. P., and Isaias, R. M. D. S. (2008). Comparative anatomy of the absorption roots of terrestrial and epiphytic orchids. Braz. Arch. Biol. Technol. 51, 83–93. doi: 10.1590/S1516-89132008000100011
O’Brien, T., Feder, N., and McCully, M. E. (1964). Polychromatic staining of plant cell walls by toluidine blue O. Protoplasma 59, 368–373.
Oliveira, V. D. C., and Sajo, M. D. G. (1999). Root anatomy of nine Orchidaceae species. Braz. Arch. Biol. Technol. 42, 0–0. doi: 10.1590/S1516-89131999000400005
Pereira, O. L., Rollemberg, C. L., Borges, A. C., Matsuoka, K., and Kasuya, M. C. (2003). Epulorhiza epiphytica sp. nov. isolated from mycorrhizal roots of epiphytic orchids in Brazil. Mycoscience 44, 0153–0155. doi: 10.1007/s10267-002-0087-7
Ponert, J., Trávníček, P., Vuong, T. B., Rybková, R., and Suda, J. (2016). A new species of Cleisostoma (Orchidaceae) from the Hon Ba nature reserve in Vietnam: a multidisciplinary assessment. PLoS One 11:e0150631. doi: 10.1371/journal.pone.0150631
Pridgeon, A. (1987). “The velamen and exodermis of orchid roots,” in Orchid Biology. Reviews and Perspectives, Vol. 4, ed. J. Arditti (Ithaca: Cornell University Press), 139–192.
Rodríguez-Robles, J. A., Ackerman, J. D., and Meléndez, E. (1990). Host distribution and hurricane damage to an orchid population at Toro Negro Forest, Puerto Rico. Caribb. J. Sci. 26, 163–164.
Rowe, N., and Isnard, S. (2009). Biomechanics of climbing palms and how they climb. Plant Signal. Behav. 4, 875–877. doi: 10.4161/psb.4.9.9426
Sathiyadash, K., Muthukumar, T., Uma, E., and Pandey, R. R. (2012). Mycorrhizal association and morphology in orchids. J. Plant Interact. 7, 238–247. doi: 10.1080/17429145.2012.699105
Scher, J. L., Holbrook, N. M., and Silk, W. K. (2001). Temporal and spatial patterns of twining force and lignification in stems of Ipomoea purpurea. Planta 213, 192–198. doi: 10.1007/s004250000503
Seidelmann, K., Melzer, B., and Speck, T. (2012). The complex leaves of the monkey’s comb (Amphilophium crucigerum, Bignoniaceae): a climbing strategy without glue. Am. J. Bot. 99, 1737–1744. doi: 10.3732/ajb.1200288
Shane, M. W., McCully, M. E., Canny, M. J., Pate, J. S., and Lambers, H. (2011). Development and persistence of sandsheaths of Lyginia barbata (Restionaceae): relation to root structural development and longevity. Ann. Bot. 108, 1307–1322. doi: 10.1093/aob/mcr244
Silverman, H. G., and Roberto, F. F. (2007). Understanding marine mussel adhesion. Mar. Biotechnol. 9, 661–681. doi: 10.1007/s10126-007-9053-x
Smyth, D. R. (2016). Helical growth in plant organs: mechanisms and significance. Development 143, 3272–3282. doi: 10.1242/dev.134064
Steinbrecher, T., Beuchle, G., Melzer, B., Speck, T., Kraft, O., and Schwaiger, R. (2011). Structural development and morphology of the attachment system of Parthenocissus tricuspidata. Int. J. Plant Sci. 172, 1120–1129. doi: 10.1086/662129
Steinbrecher, T., Danninger, E., Harder, D., Speck, T., Kraft, O., and Schwaiger, R. (2010). Quantifying the attachment strength of climbing plants: a new approach. Acta Biomater. 6, 1497–1504. doi: 10.1016/j.actbio.2009.10.003
Stern, W. L. (2014). Anatomy of the Monocotyledons Volume X: Orchidaceae. Oxford: Oxford University Press.
Stern, W. L., and Judd, W. S. (1999). Comparative vegetative anatomy and systematics of Vanilla (Orchidaceae). Bot. J. Linn Soc. 131, 353–382. doi: 10.1006/bojl.1999.0293
Thangavelu, M., and Ayyasamy, K. (2017). Comparative anatomy of aerial and substrate roots of Acampe praemorsa (Rox.) Blatt. & McCann. Flora 226, 17–28. doi: 10.1016/j.flora.2016.11.001
The Plant List (2013). Version 1.1. Online Available online at: http://www.theplantlist.org/ (accessed 28 October, 2021).
Tian, Y., Pesika, N., Zeng, H., Rosenberg, K., Zhao, B., McGuiggan, P., et al. (2006). Adhesion and friction in gecko toe attachment and detachment. Proc. Natl. Acad. Sci. U.S.A. 103, 19320–19325. doi: 10.1073/pnas.0608841103
Tomasello, A., Perrone, R., Colombo, P., Pirrotta, M., and Calvo, S. (2018). Root hair anatomy and morphology in Posidonia oceanica (L.) Delile and substratum typology: first observations of a spiral form. Aquat. Bot. 145, 45–48. doi: 10.1016/j.aquabot.2017.12.001
Tremblay, R. L. (2008). Ecological correlates and short-term effects of relocation of a rare epiphytic orchid after Hurricane Georges. Endanger. Species Res. 5, 83–90. doi: 10.3354/esr00114
Tsavkelova, E. A., Cherdyntseva, T. A., Botina, S. G., and Netrusov, A. I. (2007). Bacteria associated with orchid roots and microbial production of auxin. Microbiol. Res 162, 69–76. doi: 10.1016/j.micres.2006.07.014
Voigt, D., Tsipenyuk, A., and Varenberg, M. (2017). How tight are beetle hugs? Attachment in mating leaf beetles. R. Soc. Open Sci. 4:171108. doi: 10.1098/rsos.171108
Yang, X., and Deng, W. (2017). Morphological and structural characterization of the attachment system in aerial roots of Syngonium podophyllum. Planta 245, 507–521. doi: 10.1007/s00425-016-2621-4
Zenone, A., Alagna, A., D’Anna, G., Kovalev, A., Kreitschitz, A., Badalamenti, F., et al. (2020a). Biological adhesion in seagrasses: The role of substrate roughness in Posidonia oceanica (L.) Delile seedling anchorage via adhesive root hairs. Mar. Environ. Res. 160:105012. doi: 10.1016/j.marenvres.2020.105012
Zenone, A., Filippov, A., Kovalev, A., Badalamenti, F., and Gorb, S. N. (2020b). Root hair adhesion in Posidonia oceanica (L.) Delile seedlings: a numerical modelling approach. Front. Mech. Eng. 6:88. doi: 10.3389/fmech.2020.590894
Zhang, M., Liu, M., Prest, H., and Fischer, S. (2008). Nanoparticles secreted from ivy rootlets for surface climbing. Nano Lett. 8, 1277–1280. doi: 10.1021/nl0725704
Zotz, G. (2016). Plants on Plants: The biology of Vascular Epiphytes. Cham: Springer International Publishing.
Zotz, G., Hietz, P., and Einzmann, H. J. R. (2021b). Functional ecology of vascular epiphytes. Annual Plant Reviews. doi: 10.1002/9781119312994.apr0777
Zotz, G., Weigelt, P., Kessler, M., Kreft, H., and Taylor, A. (2021c). EpiList 1.0: a global checklist of vascular epiphytes. Ecology 102:e03326. doi: 10.1002/ecy.3326
Zotz, G., Almeda, F., Bautista-Bello, A. P., Eskov, A., Giraldo-Cañas, D., Hammel, B., et al. (2021a). Hemiepiphytes revisited. Perspect. Plant Ecol. Evol. Syst. 51:125620. doi: 10.1016/j.ppees.2021.125620
Keywords: adhesion, attachment, epiphytes, root hairs, glue-like substance, attachment strategy
Citation: Tay JYL, Zotz G, Gorb SN and Einzmann HJR (2021) Getting a Grip on the Adhesion Mechanism of Epiphytic Orchids – Evidence From Histology and Cryo-Scanning Electron Microscopy. Front. For. Glob. Change 4:764357. doi: 10.3389/ffgc.2021.764357
Received: 25 August 2021; Accepted: 21 October 2021;
Published: 10 November 2021.
Edited by:
Alexander Shenkin, University of Oxford, United KingdomReviewed by:
Wakana Azuma, Kobe University, JapanKelsey R. Carter, Division of Earth and Environmental Sciences, Los Alamos National Laboratory, United States
Copyright © 2021 Tay, Zotz, Gorb and Einzmann. This is an open-access article distributed under the terms of the Creative Commons Attribution License (CC BY). The use, distribution or reproduction in other forums is permitted, provided the original author(s) and the copyright owner(s) are credited and that the original publication in this journal is cited, in accordance with accepted academic practice. No use, distribution or reproduction is permitted which does not comply with these terms.
*Correspondence: Jessica Y. L. Tay, amVzc2ljYS50YXkueWluZy5saW5nQHVuaS1vbGRlbmJ1cmcuZGU=