- 1Department of Forestry and Natural Resources, Hardwood Tree Improvement and Regeneration Center, Purdue University, West Lafayette, IN, United States
- 2Department of Entomology, Purdue University, West Lafayette, IN, United States
Bark beetles vector symbiotic fungi and the success of these mutualisms may be limited by competition from other microbes. The outcome of fungal competition is strongly influenced by the physical and chemical conditions of the wood they inhabit. These conditions are in turn subject to climatic variation. In particular, wood moisture content (MC) influences fungal competition and, therefore, could help determine environmental suitability for thousand cankers disease (TCD) caused by Geosmithia morbida and its vector Pityophthorus juglandis. We conducted competition experiments in Juglans nigra wood that was naturally or artificially colonized by G. morbida and other fungi over a range of wood MC expected across prevailing United States climatic conditions. G. morbida outcompeted antagonistic fungi Clonostachys and Trichoderma spp. at <5% equilibrium moisture content. Aspergillus spp. outcompeted G. morbida at low moisture in wood from Indiana. We fit a logistic regression model to results of the competition experiments to predict survival of G. morbida across the United States. Expected survival of G. morbida was highest in historical TCD epicenters and accounted for the low incidence and severity of TCD in the eastern United States. Our results also predict that under future climate scenarios, the area impacted by TCD will expand into the native range of J. nigra. Given its role in emergent forest health threats, climate change should be a key consideration in the assessment of risks to hardwood resources.
Introduction
Range expansions of native pests caused by climate change are a major threat to the health and productivity of forest ecosystems (Ramsfield et al., 2016; Pureswaran et al., 2018). In particular, abiotic factors are known to influence dispersal and reproduction of scolytine beetles (Coleoptera: Curculionidae) and primary symbiotic fungi (Wood, 1982; Six and Bentz, 2007). Beetles and fungi mutualistically rely upon one another for successful host colonization and to satisfy metabolic requirements (Hofstetter et al., 2007, 2015; Six and Bentz, 2007; Mitton and Ferrenberg, 2012). Physicochemical conditions in bark and wood, including nutrient availability, temperature, and moisture, determine the outcome of competition between the primary mutualist of a beetle species and other fungi, and consequently, affect reproductive success and dispersal (Rayner and Boddy, 1988; Ranger et al., 2018).
Co-dispersal of the walnut twig beetle (Pityophthorus juglandis Blackman) and its primary mutualist, the pathogenic fungus Geosmithia morbida Kolařík, Freeland, Utley, and Tisserat, to walnut trees (Juglans spp.) is contingent on physicochemical conditions that favor competitive success and sporulation of G. morbida in walnut wood. Many species of scolytine beetles have evolved specialized structures, glands, and behaviors that maximize favorability of growth conditions for the successful colonization of wood by their mutualistic fungi (Francke-Grosmann, 1967; Weed et al., 2015; Nuotclà et al., 2019). Other species, including P. juglandis, lack specialized structures but rather rely on passive co-dispersal of fungi (Bright, 1981). The hydrophobic spores of G. morbida are borne on conidiophores inside beetle galleries (Tisserat et al., 2009; Kolařík et al., 2011) and picked up by static adhesion to the cuticle of adult P. juglandis as they emerge (Seybold et al., 2016).
Geographic variation in severity and impact of thousand cankers disease (TCD) and establishment of P. juglandis and G. morbida across North America (Tisserat et al., 2011; Griffin, 2015; Juzwik et al., 2020) suggest that climatic factors such as temperature and humidity may be important in determining environmental favorability for development and spread of the disease. TCD results from mass attack of P. juglandis that introduce G. morbida to the inner bark of Juglans and Pterocarya spp., which causes necrotic cankers in the phloem and outer sapwood that interfere with the translocation of nutrients and photosynthate from distal branches and leaves to other parts of the plant (Tisserat et al., 2009).
Geosmithia morbida is evolutionarily adapted to the seasonally dry climate of the western United States (Williams and Newcombe, 2017). Population genetics and historical records indicate that both P. juglandis (Seybold et al., 2012; Rugman-Jones et al., 2015) and G. morbida (Hadziabdic et al., 2014; Zerillo et al., 2014) are native to semiarid southwest North America where the range of their ancestral host, Juglans major (Torr.) A. Heller, crosses into the United States from Mexico (Little, 1976). Large-scale tree mortality attributed to TCD has been restricted to other native and introduced Juglans spp. west of the Great Plains (Seybold et al., 2019). G. morbida is thermophilic (Kolařík et al., 2011) and xerotolerant (Williams and Newcombe, 2017), and commonly found sporulating inside P. juglandis galleries in black walnut (Juglans nigra L.) in the western, but not the eastern, United States (D. Hadziabdic, pers. comm.). In eastern states, including Tennessee (TN) in the Appalachian Mountains and piedmont, fungal antagonists such as Trichoderma spp. are frequently found instead of G. morbida in P. juglandis galleries (Gazis et al., 2018). Native populations of J. nigra in the eastern United States have been largely unaffected by TCD despite detections of the beetle or fungus in nine states (Moore et al., 2019; Seybold et al., 2019; Juzwik et al., 2020; Stepanek, 2020), and moribund trees recovered in disease epicenters in Virginia (VA) and Tennessee (TN) (Griffin, 2015).
Climatic differences between the eastern and western United States provide one possible explanation for geographical difference in the prevalence and spread of TCD within the native and non-native range of J. nigra. In particular, intracontinental differences in prevailing climatic conditions may lead to differences in moisture content (MC) of senescent woody tissues around P. juglandis galleries and affect the relative competitive success and sporulation of G. morbida. When wood dries, its equilibrium moisture content (EMC) is determined by air temperature and relative humidity. Moisture content—the amount of moisture in wood—typically falls below 10% in spring, summer, and autumn in locations in the western United States where TCD has been severe (Seybold et al., 2019) but remains above 10% throughout much of the native range of J. nigra (Eckelman, 1998; Simpson, 1998). For example, in Tippecanoe Co., Indiana (IN), in the humid midwestern United States, summer MC of J. nigra wood was much higher, at 14.9 ± 0.20% (n = 156) for air-dried lumber and 20.1 ± 0.29% (n = 180) for retail firewood (Williams and Ginzel, unpublished data), the predominant pathway for the movement of invasive wood-boring pests (Newton and Fowler, 2009; Jacobi et al., 2012). Furthermore, the peak flight of P. juglandis typically occurs in the spring (Sitz et al., 2017; Chen et al., 2020), coinciding with high levels of precipitation in the Midwest.
Geographical variation in TCD incidence and severity could be partly explained by a competitive advantage for G. morbida over other xylotropic fungi in the western United States. However, under higher prevailing MC in the eastern United States (Eckelman, 1998), the colonization and spread of G. morbida may be inhibited by competition with other fungi that may be better adapted to those conditions. G. morbida was recovered after 133 days from wood dried to a MC of 7% and grown on 25% glycerol agar (Williams and Newcombe, 2017) with a water potential of −20 MPa (Ridout et al., 2017). These moisture levels fall below known limits for fungal wood decomposition (−4 MPa; Griffin, 1977), between limits for biological activity in soils (−14 MPa) and surface litter (−36 MPa; Manzoni et al., 2012), and between limits for soil-dwelling Fusarium (−10 MPa) and extremely xerophilic Penicillium spp. (−40 MPa; Harris, 1981). Water potential supporting the growth of G. morbida is similar to those that support Penicillium and Phialocephala from roots of conifers that withstand seasonal droughts in the seasonally dry western United States (Ridout et al., 2017). To our knowledge, the role of temperature and wood MC in determining the outcome of competition between G. morbida and other fungi in walnut wood has not yet been investigated.
Understanding the environmental parameters that favor TCD is essential for risk assessments to help predict the future threat to J. nigra in a changing climate. Wood MC may have historically limited the spread of TCD in the native range of J. nigra. Nevertheless, conditions could still become favorable for TCD in the eastern United States if prevailing temperature, precipitation, and humidity change as predicted by climate models (USGCRP, 2018). We hypothesized that the success of G. morbida and P. juglandis is limited by competition between G. morbida and other native fungi better adapted to the prevailing temperature and humidity regimes in the native range of J. nigra. To test this hypothesis, we first carried out a series of competition experiments in wood that had been naturally or artificial colonized by G. morbida and other fungi. We used lethal and non-lethal heat treatments to create fungal wood microcosms to reduce the abundance of native fungi in the wood and then inoculated it with G. morbida. In another experiment, we characterized competition between G. morbida and other fungi in nontreated wood that was naturally infested. We calibrated the incubation conditions of the experiments to a range of EMC corresponding to climatic conditions across the United States (Eckelman, 1998; Simpson, 1998). We predicted that G. morbida would have a competitive advantage over other fungi that occur in wood that equilibrated to extremely low MC but would be increasingly outcompeted by other fungi in wood that equilibrated to intermediate and high MC. We validated our interpretation of the results from our competition experiments by first extrapolating expected survival of G. morbida across the United States based on historical climate data, and then comparing inferred TCD severity with historical observations. Finally, we extrapolated the model to climate change scenarios based on high- and low-carbon emission to predict the portions of the native range of J. nigra that will be threatened by TCD 10 and 50 years into the future.
Materials and Methods
Collection of Branches and G. morbida Isolates
Branches (6–8-cm diameter) of black walnut (Juglans nigra) were collected in 2018 and 2019, cut into 20–25-cm lengths and brought or shipped to an authorized containment facility at Purdue University (West Lafayette, IN, United States). All branches were cut laterally into disks to a thickness of 4 mm. G. morbida (Gm-10) used in experiments 1–3 was originally isolated from TCD-symptomatic trees in Tennessee (Hadziabdic et al., 2014) and obtained courtesy of the laboratory collection of Dr. Denita Hadziabdic-Guerry (UT-Knoxville). To inoculate wood disks with G. morbida, they were placed on cultures of Gm-10 growing on 1/8-strength potato dextrose agar (4.9 g PDA powder + 13.1 g agar per 1 L H2O) in vented, high-profile dimension polystyrene petri dishes (100-mm diameter 26-mm deep, Thermo Fisher Scientific, Waltham, MA, United States). Cultures of G. morbida were allowed to grow until colony diameter matched the diameter of the wood disks (8–21 days).
Humidity Chambers
Four fungal competition experiments were conducted in the dark. Humidity chambers with different expected equilibrium relative humidity and wood MC were constructed by preparing supersaturated salt solutions in 250-ml beakers and placing one beaker of each solution inside of a tightly sealed six-quart plastic storage container (Simpson, 1973; Greenspan, 1977). Wood disks were placed inside of sterile standard-size (100-mm diameter by 17-mm deep) polystyrene petri dishes, which were placed inside the humidity chambers with the beakers containing the salt solutions. In all experiments, wood disks that were from the same parts of the same branch were distributed equally and randomly among humidity treatments to obtain independent data on survival of fungi from each portion of the branch or branches for each treatment. Temperature was maintained at 30°C by keeping the plastic storage containers containing the petri dishes and beakers with salt solutions inside a Precision 818 low-temperature incubator (Thermo Fisher), or at 23°C by keeping the containers in a laboratory cabinet. Most treatments were conducted at the optimal growth temperature for G. morbida (30°C) to provide conservative inferences on the effect of wood moisture on its competitive success. In Experiments 3 and 4 described below, an additional temperature treatment at 23°C was included to increase the relative humidity and, thereby, increase wood MC and decrease the competitive advantage of G. morbida.
Experiment 1: High-Heat Pretreatment of Wood Disks and Inoculation With G. morbida
Branches were collected from Purdue University Martell Forest in West Lafayette, IN (40°25′60.0″N, 87°02′07.3″W), where neither G. morbida or P. juglandis have been detected, and allowed to dry on a lab bench for approximately 30 days prior to cutting them into disks. Walnut branch disks from Indiana were wrapped in aluminum foil and placed in an oven at 90°C for 48 h to dry and kill most fungi. The heat-treated disks were then transferred to cultures of G. morbida. After 24 h, disks with visible growth of fungi other than G. morbida were discarded, and the remaining inoculated disks were transferred to sterile plastic petri dishes. Discarding disks with substantial growth of other fungi left a slightly unbalanced sample size across treatments: LiCl (n = 11 inoculated wood disks), KOAc (n = 9), MgNO3 (n = 9), and NH4Cl (n = 11). Disks were then allowed to equilibrate and incubate in the chambers at 30°C for 48 days.
At the conclusion of the incubation period, disks were weighed to record wet mass (mi), and fungal growth was examined under a stereomicroscope (e.g., Figures 1B,F). Fungi growing on the surface of each wood disk were categorized into morphospecies while examining them under 400× magnification. Presence and absence of each morphospecies were recorded for each disk (Figure 1). Mycelia or spores from the most frequently encountered and dominant morphospecies were collected directly into tubes with buffer to extract DNA or cultured to obtain voucher samples. If present, white-to-pink penicilliate conidiophores that resembled those of G. morbida (Figures 1A–C) were mounted on slides and examined at 1000× to confirm their identity. G. morbida was also cultured from conidiophores to verify viability of the spores across the range of final abiotic conditions. All wood disks were then dried at 70°C for at least 96 h and weighed again to record dry mass (mf). Final wood MC of each individual wood disk was calculated gravimetrically according to Eckelman (1998): .
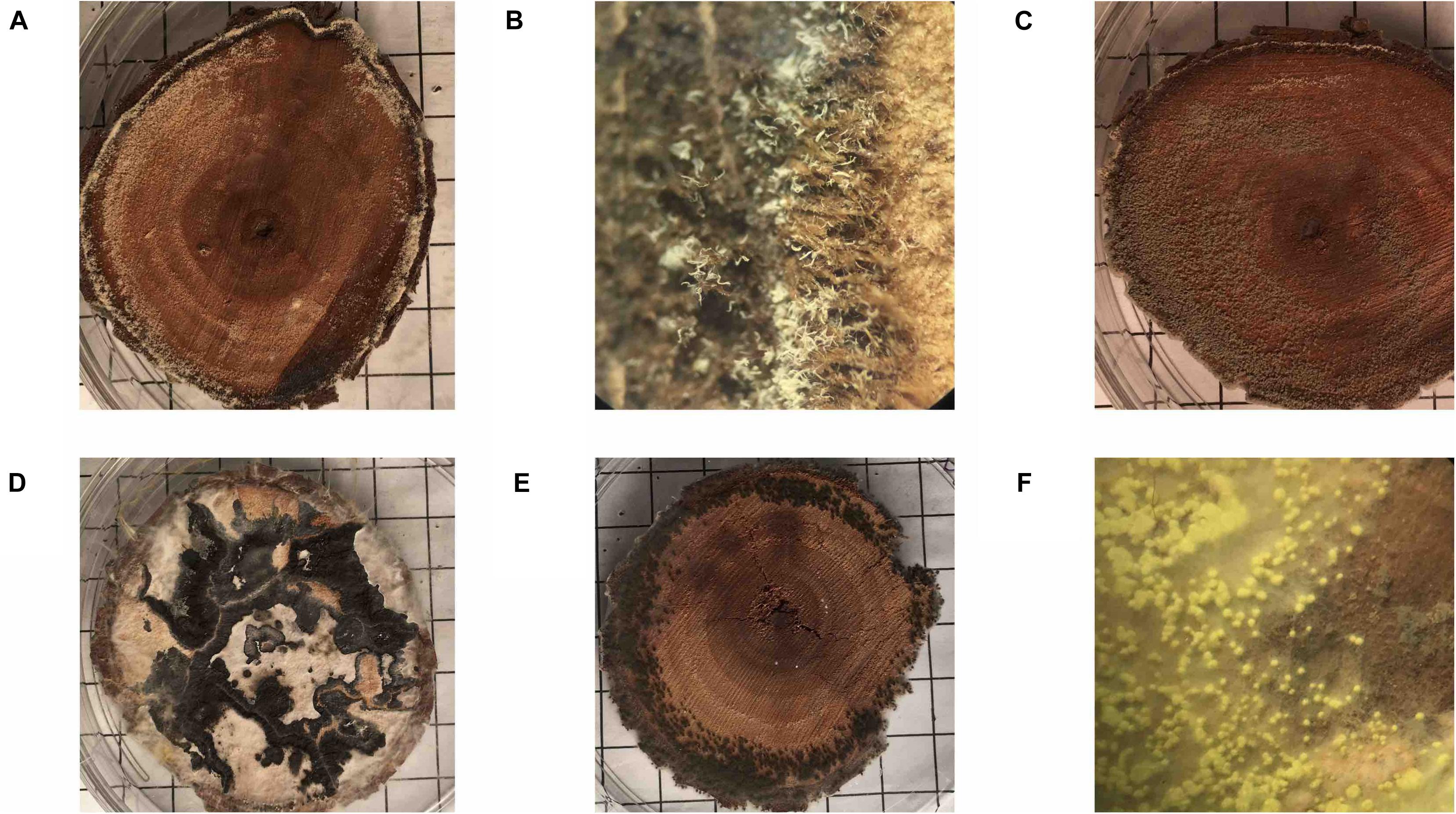
Figure 1. Representative images of wood disks colonized by fungi from Experiments 1 (A,C–E) and 4 (B,F). Wood disk colonized by Geosmithia morbida (A,B), Aspergillus sp. and G. morbida (C), Xylariaceae sp. (D), Trichoderma sp. (E), and Aspergillus (both anamorph and Eurotium sexual stage) sp. and Clonostachys sp. (F) Each grid line is 1 cm2 (A,C–E).
Experiment 2: Low-Heat Pretreatment of Wood Disks and Inoculation With G. morbida
Wood disks were collected, dried, inoculated, and incubated as described for Experiment 1 with the following exceptions: a less intense heat treatment (70°C for 24 h) and longer incubation period in humidity chambers (88 days) was employed to allow more fungal endophytes to persist in the wood prior to being challenged with G. morbida and a longer period to compete in humidity chambers. The final number of disks in each treatment was balanced (n = 8 per saturated salt solution). Fungi were counted and sampled and wood MC was determined gravimetrically as described above.
Experiment 3: Lethal Heat Pretreatment of Wood Disks and Inoculation With G. morbida
A control experiment was designed to demonstrate that fungi capable of growing and competing with G. morbida could be completely removed from the wood at sufficiently high temperature. Growth and survival of G. morbida at all MCs in the absence of other fungi would suggest that the low survival of G. morbida at higher moisture levels in Experiments 1, 2, and 4 was due to competition with other fungi. To ensure full removal of other fungi that had already colonized the wood disks, they were heat-treated at 105°C for 2 days. To ensure colonization by G. morbida, disks were left on cultures for 10 days before being transferred to humidity chambers. Two G. morbida-inoculated disks and four control disks were included in each of the four humidity chamber treatments to verify G. morbida survival and the absence of other fungi. The saturated salt solutions, temperature, and incubation times employed were LiCl, NaCl, or no salt, incubated for 57 days at 30°C. An additional no-salt treatment was incubated at room temperature (23°C), and expected to result in higher relative humidity and final MC. Fungi were sampled and wood MC was determined as described above.
Experiment 4: Fungal Competition in Wood Naturally Colonized by G. morbida
Branches that were already naturally colonized with G. morbida were collected from a TCD disease epicenter. The trees grew on a privately owned plantation of black walnut in Walla Walla, WA, United States. Six branches were collected from six TCD-symptomatic trees and shipped to Purdue University under permit (17-IN-20-007). Two freshly cut disks from each branch were placed directly in sterile petri dishes inside of each of the humidity chambers (n = 18 per treatment). Salt solutions and temperatures employed were the same as described above for Experiment 3. Fully saturated wood disks were allowed to incubate for 111 days to reach their EMC. Fungi were sampled and wood MC determined by drying disks at 100°C for 72 h.
Molecular Identification of Fungi
DNA extraction, polymerase chain reaction (PCR) of the ITS region, and sequence assembly were performed as described previously (Williams and Ginzel, in press). ITS sequences were extracted with ITSX and assigned to genera through Tree-Based Assignment Selector (TBAS; Miller et al., 2015; Carbone et al., 2019) on DeCIFR public high-performance computing clusters (Center for Integrated Fungal Research, North Carolina State University)1. For high-level classification, all sequences were first aligned to a reference tree for all fungi. Isolates assigned to Ascomycetes were then aligned to the tree Pezizomyctonia 2.1, whereas Basidiomycetes were assigned taxonomy with the RDP Bayesian classifier with the Warcup database (Deshpande et al., 2016). Sequences were submitted to GenBank under accession numbers MW584687-MW584698.
Logistic Regressions
To test the hypothesis that the presence or absence of G. morbida and other fungi in each wood disk was significantly correlated to final MC, logistic regression and accompanying analytics were performed in R v 3.6.0 (R Core Team, 2019) using the function glm and tools from the package ROCR (Sing et al., 2005). Because we expected survival rate of each fungus to peak at an optimum MC, we also fit models that included second-order terms for MC. Second-order terms were only retained in the model if they were significant (p < 0.05). Drop-in-deviance χ2 tests and area under the receiver-operating curve (AUC) were used to assess overall regression significance and fit, respectively.
Geographical Prediction of G. morbida Survival
To create maps of expected G. morbida survival across the United States for historical and future prevailing climates, we used climate layers from Multivariate Constructed Analogs (MACA) statistical downscaling method, Version 2 (Abatzoglou, 2013). We derived a map of expected EMC from MACA by first calculating monthly average humidity and temperatures from monthly minimums and maximums for 5 months (i.e., May, June, July, August, and September) between 1995 and 2004. This time period from late spring to early fall corresponds to the time when P. juglandis, the vector of G. morbida, is most active. Next, we inputted monthly averages into Simpson’s (1973) model for wood moisture sorption with parameters estimated by Glass and Zelinka (2010). The Glass and Zelinka (2010) sorption isotherm model used to calculate EMC as a function of ambient air temperature (t, °C) and relative humidity (h, decimal) is given by the following system of equations with constants from Simpson (1973):
For each year between 1995 and 2004, expected G. morbida survival from May to September was calculated from the best logistic model fit to Experiment 4 as a function of EMC from the Glass and Zelinka (2010) model. Expected survival rates for each month (between 0 and 1) were then multiplied across all 5 months to obtain a conservative, cumulative expected probability of survival for each year. Finally, cumulative probabilities of survival for each year were averaged across ten years (1995–2004) for historical analysis. For future climate, we used MACA data generated by the Geophysical Fluids Dynamic Laboratory Earth System Models II (GFDL-ESM2M) under the Representative Concentration Pathway low- (RCP4.5) and high-emission (RCP8.5) scenarios for 10 (2031) and 50 years (2071) into the future. RCP scenarios are coded by the amount of thermal radiation (i.e., 4.5 W/m2 vs. 8.5 W/m2) that is expected to be absorbed and retained by the atmosphere in year 2100. According to the United States National Oceanic and Atmospheric Administration (NOAA), the atmosphere held ∼400 ppm CO2 as of 20192, RCP4.5 projects ∼500 ppm CO2, and RCP8.5 projects ∼800 ppm CO2 in 2071 (IPCC, 2014).
Results
Competition Experiments
Competition with other fungi limited the survival of G. morbida at higher wood moisture levels (Figure 2). G. morbida was released from competition at low wood moisture levels (Experiments 1 and 4; Figures 2A,D) or in the absence of other fungi (Experiment 3; Figure 2C). In general, when other fungi were present in wood disks, G. morbida was most successful at <5% final MC and did not grow or sporulate >30% final MC. Saturated salt solutions were effective at bringing wood disks incubated in the humidity chambers to the target range of MC.
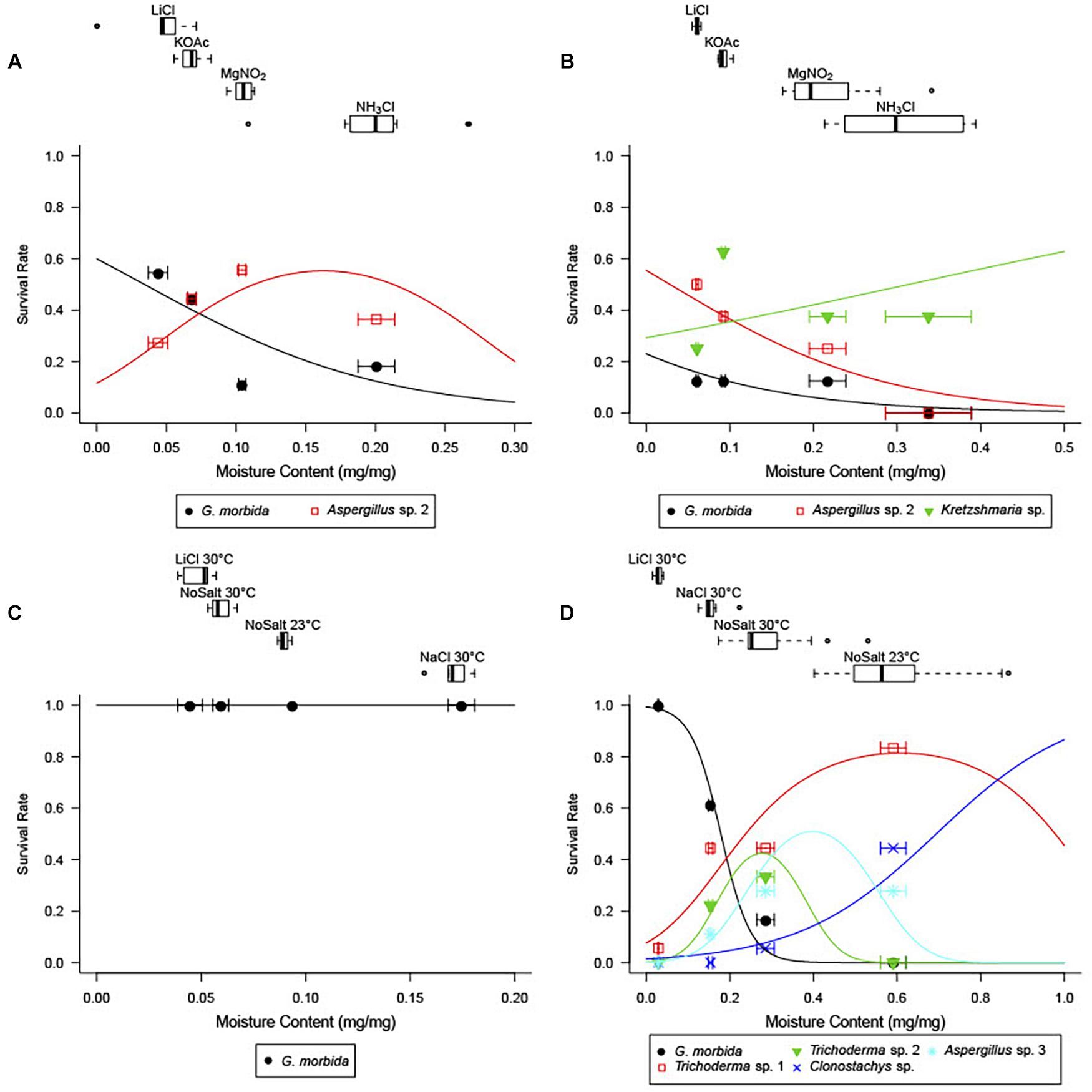
Figure 2. Wood moisture content (MC) by treatment (top panels) and observed and predicted survival of fungal species as a function of wood MC from best-fit logistic regressions (bottom panels) in Experiments 1 (A), 2 (B), 3 (C), and 4 (D). For each saturated salt solution treatment, boxes-and-whiskers show percentiles of final wood MC, and points and error bars in the plotting area give observed mean survival ± SE final MC.
In Experiment 1, when wood from Indiana was pretreated at a high (90°C) non-lethal temperature prior to inoculation with G. morbida, final MC significantly accounted for the survival and sporulation of G. morbida (Figure 2A and Table 1). Control disks that were not inoculated with G. morbida supported growth of other fungi at all final MC levels.
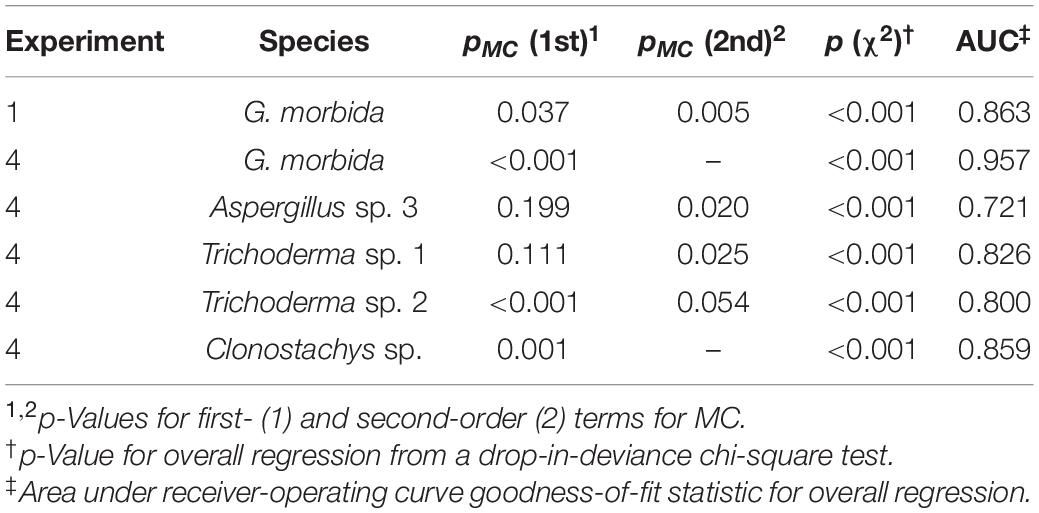
Table 1. Model statistics for logistic regression of survival of fungi on final wood moisture content (MC).
When wood from Indiana was pretreated at a lower (70°C) non-lethal temperature (Experiment 2), final MC did not significantly account for survival of G. morbida (p > 0.05) due to the growth of other fungi at low final MC (Figure 2B). Fungi from Indiana wood samples in Experiments 1 and 2 included Aspergillus sp. (Eurotiomycetes: Eurotiales), Nothophoma sp. (Dothidiomycetes: Pleosporales), and Kretzschmaria sp. (Sordariomycetes: Xylariales) at low final MC, and Diplodia sp. (Dothidiomycetes: Botryosphaeriales) at intermediate final MC (Table 2).
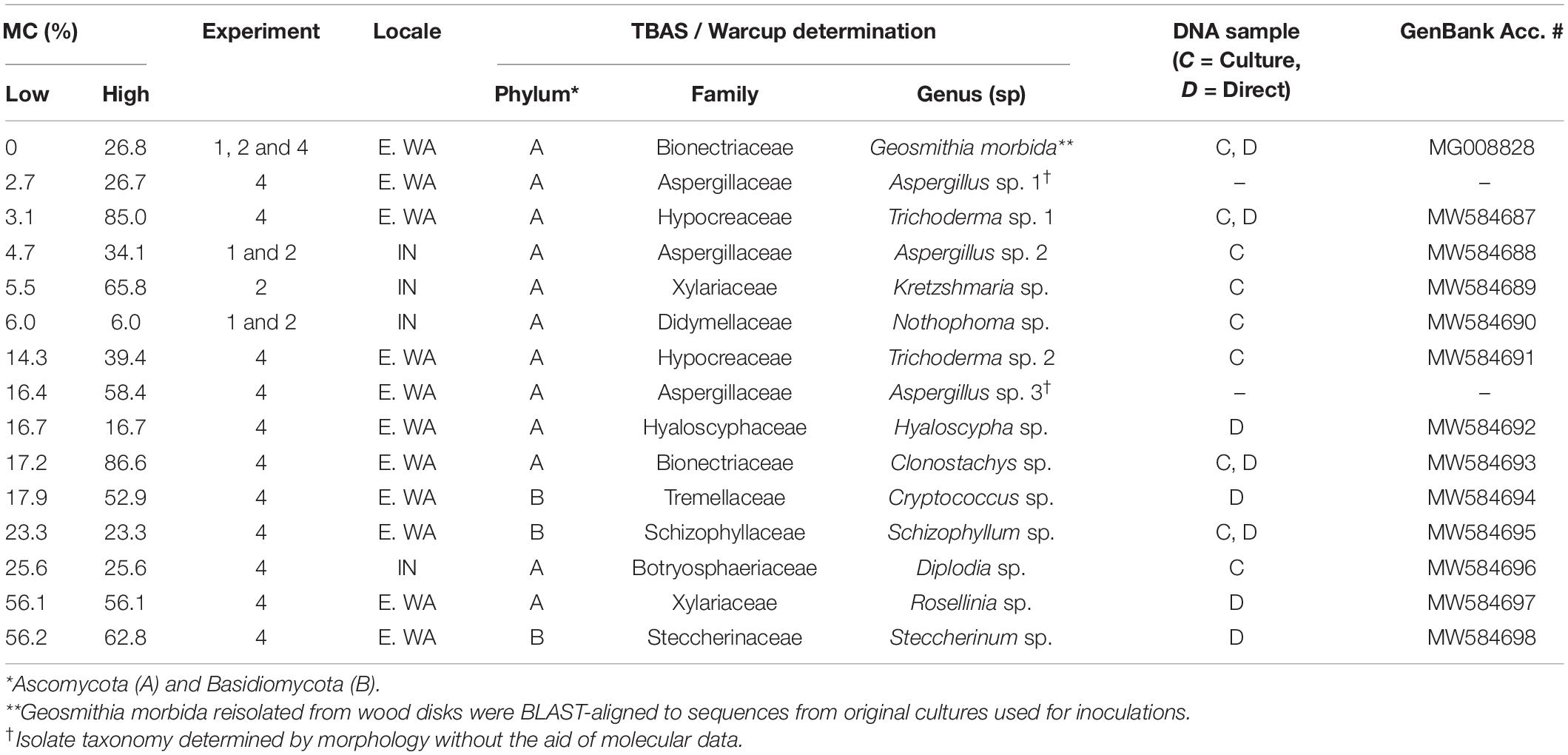
Table 2. Final MC range, molecularly and/or morphologically inferred taxonomy, and GenBank accession numbers for DNA extracted directly (D) and/or from cultures (C) from fungi growing on wood disks in competition experiments.
However, the higher temperature and duration (105°C for 2 days) treatment was sufficient to remove fungi from the wood from Indiana prior to inoculation (Experiment 3). In the absence of other fungi, G. morbida grew and sporulated on all inoculated wood disks regardless of final MC (Figure 2C). Other fungi were not found growing on control or G. morbida-inoculated wood disks (data not shown).
In Experiment 4, final MC accounted for the survival and sporulation of G. morbida in naturally infested wood from TCD-symptomatic trees from Washington (Figure 2D and Table 1). Fungi from Washington wood samples were represented by Aspergillus, Trichoderma, and Clonostachys spp. (Sordariomycetes: Hypocreales) at low and intermediate MC and Rosellinia spp. (Sordariomycetes: Xylariales) and Basidiomycota including Cryptococcus (Tremellomycetes: Tremellales), Schizophyllum, and Steccherinum spp. (Agaricomycetes: Polyporales) at higher final MC (Table 2). The best-fit model for probability of G. morbida survival (P) as a function of final MC was:
Geographical Trends in Expected G. morbida Survival
When predicting survival using the model above from EMC calculated from climate models, survival for the 10-year period from 1995 to 2004 closely followed geographical patterns of TCD severity observed from that time to the present (Figure 3A). West of the Great Plains and east of the Cascades, predicted survival generally ranged from 50 to 80%. Epicenters of TCD in seasonally dry intermountain western and northwestern states such as Washington (WA), Utah (UT), Idaho (ID), and the native range of P. juglandis in arid southwestern states of Arizona (AZ) and New Mexico (NM) exceeded 70% expected G. morbida survival. Elsewhere in the West, TCD epicenters in Oregon (OR), California (CA), and Colorado (CO) were predicted to support ∼60% expected survival of G. morbida.
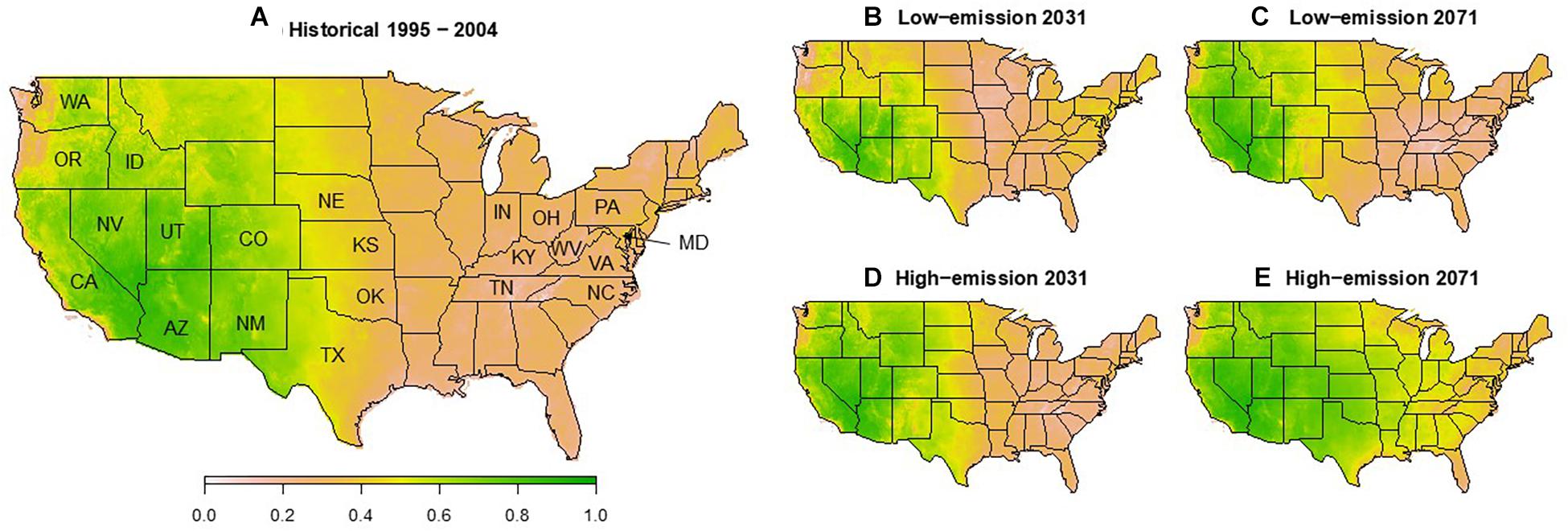
Figure 3. Annotated map of regions and States of the United States referred to in the text and modeled survival of G. morbida as a function of equilibrium moisture content (EMC) for average historical (A) and future low- (B,C, RCP4.5) and high-emission (D,E, RCP8.5) climate scenarios 20 (B,D) and 50 years (C,E) into the future. Survival for each year was obtained by multiplying monthly survival estimates from a logistic regression fit to results from Experiment 4 for May through September. For historical survival (A), yearly survival estimates were averaged across the 10-year timespan. Monthly average EMC was calculated using the model of Glass and Zelinka (2010) as a function of monthly average temperature and humidity averaged from Multivariate Constructed Analogs (MACA) statistical downscaling method, Version 2 (Abatzoglou, 2013) and Geophysical Fluids Dynamic Laboratory Earth System Models II (GFDL-ESM2M) under the Representative Concentration Pathway scenarios (IPCC, 2014).
In the native range of J. nigra, expected survival for G. morbida was generally below 20% (Figure 3A). However, known TCD epicenters along the north Atlantic seaboard in eastern Pennsylvania (PA), Maryland (MD), and VA are located in some of the only pockets in the East where expected G. morbida survival reached ∼50% according to our model. By contrast, in Knox and Polk Cos., TN, where TCD caused a local and transient outbreak, suitability for G. morbida fell to 20% or below.
Under RCP 4.5, the geographical envelope in which G. morbida survival rates exceed 50% in the United States is expected to remain relatively stable from 2021 to 2071 (Figures 3B,C). However, this envelope would expand across the Great Plains and Mississippi Valley into the humid midwestern and southeastern states under RCP 8.5 (Figures 3D,E).
Discussion
Wood Moisture, Fungal Competition, and TCD
Our research demonstrates that fungal competition mediated by abiotic conditions potentially limits the success and dispersal of G. morbida and adds to a growing body of evidence that environmental conditions, including abiotic factors and the host microbiome, account for the lower severity of TCD in the native range of J. nigra (Griffin, 2015; Seybold et al., 2019; Onufrak et al., 2020). There were also notable differences in G. morbida competition and survival between branches from WA and those from IN, where xerophilic-thermotolerant fungi, including Xylariaceae and Aspergillus spp., outcompeted G. morbida at low moisture. These fungi survived temperatures up to 70° and even 90°C (Aspergillus sp.) for 48 h. Prior colonization of the substrate by these fungi could have prevented G. morbida from colonizing wood disks at lower moisture levels. These findings raise the possibility that other fungi in walnut wood inhibit colonization and growth of G. morbida under climatic conditions that would otherwise favor the development of disease and could have important management implications under future climate.
In ambrosia beetle galleries, Aspergillus spp. are known to be parasites of beetle–fungal mutualisms, where they outcompete symbiotic fungi, decrease fecundity, or cause disease of beetles (Moore, 1971, 1973; Nuotclà et al., 2019). This exploitation is more successful when physicochemical conditions favor Aspergillus spp. over ambrosia fungi (Ranger et al., 2018). Aspergillus sp. outcompeted G. morbida in our experiments, suggesting it could also interfere with the mutualism between G. morbida and P. juglandis.
The ability of G. morbida to outcompete Trichoderma spp. and other fast-growing fungi at low wood moisture has potentially important implications for efforts to employ them as biological control agents (Gazis et al., 2018). Both Trichoderma spp. from WA grew well and partly outcompeted G. morbida at 15% MC, which is equivalent to levels in air-dried walnut wood in the Midwest (Williams and Ginzel, unpublished data). However, G. morbida was still observed successfully growing and sporulating more frequently than either Trichoderma spp. on wood disks at 15% MC (cf. Gazis et al., 2018). Our findings support exercising caution when drawing biological inferences from competition assays conducted in standard laboratory media to inform management decisions. When the target organism is a specialist, stress-tolerant, or ruderal species, such laboratory assays are likely to favor competitive, resource-demanding putative antagonists when the target organism would otherwise outcompete them under realistic field conditions (Newcombe, 2011; Fierer, 2017; Whitaker and Bakker, 2019).
Mapping the expected survival of G. morbida across the United States based on EMC reproduced with high fidelity the historical geography of well-known TCD epicenters which have been reviewed elsewhere (Seybold et al., 2019). Our experiments suggest that the higher EMC in walnut wood in the Midwest, Appalachia, and Atlantic United States limits the rates of successful establishment and spread of G. morbida due to competition with other fungi. In parts of MD, VA, PA, Ohio (OH), and IN, expected survival of G. morbida was higher than the rest of the native range of J. nigra, but below 50%. In these locations, P. juglandis and/or G. morbida have been detected transiently or incidentally since the early to mid-2010s without the accompanying widespread decline and mortality of J. nigra, indicating less success in these areas than in the western United States. We infer that areas of transient TCD outbreaks in eastern TN and western North Carolina (NC) that occurred following years of below-average precipitation (Griffin, 2015) have nevertheless been historically unfavorable for G. morbida. However, this prediction that wood moisture and fungal competition limit G. morbida and TCD severity will require future validation through field studies of galleries in branches of mature trees.
Drought and above-average temperatures may have increased host stress and susceptibility, leading to successful attack and colonization by P. juglandis as suggested by Griffin (2015). Even though the expected survival of G. morbida is extremely low in Appalachia, TN and neighboring parts of NC experienced severe and extreme drought starting in March of 2007 that persisted in Knoxville, TN, until November 2008 [Palmer drought severity index, Palmer, 1965; National Oceanic and Atmospheric Administration (NOAA), 2020]. During this time, there was also a drought in CA that persisted into the end of 2009 [National Oceanic and Atmospheric Administration (NOAA), 2020]. These conditions likely led to physiological stress and could have facilitated low bark moisture and heightened susceptibility of Juglans spp. to attack in the years leading up to outbreaks of TCD that were detected in these areas in 2010.
The dependence of G. morbida on low-moisture wood for competitive success over other wood-inhabiting fungi may also partly explain the severity of TCD in urban and periurban forests and plantations (Seybold et al., 2019). Closed canopies provide a cool, humid microclimate, which may favor natural competitors of G. morbida in walnut wood. By comparison, the canopies of trees in plantations are likely to provide a more diminished effect on moisture retention compared to natural forests. In urban areas, relative humidity would be expected to be even lower because trees are even more widely spaced and heat islands generate higher temperatures (Imhoff et al., 2010). Forest diversity, management activities, buildup of other host-specific biotic disturbance agents, or abundance of opportunistic pathogens (Williams and Ginzel, in press) could also partly account for the higher incidence of TCD in plantations and urban forests. Nevertheless, wood MC is not the only abiotic factor likely to limit the potential geographic range of G. morbida and P. juglandis in J. nigra (Kolařík et al., 2011; Sitz et al., 2017). Most importantly, climatic differences may impact the flight activity and survival of P. juglandis (Luna et al., 2013; Hefty et al., 2017, 2018; Chen et al., 2020). Within the native range of black walnut, it is also possible that xerophilic and thermotolerant fungi such as Xylariaceae and Aspergillus spp. from this study might outcompete G. morbida and thereby limit the spread of TCD.
Linking the geographical limitations of the potential range of G. morbida in the native range of J. nigra to the limited reproduction and dispersal of P. juglandis rests on the assumptions that the symbiosis is truly mutualistic. Evidence for a causal link between MC and TCD severity would be further strengthened if this mutualism was found to be obligate. Primary symbiotic fungi not only weaken hosts to facilitate mass attack by bark beetles but also provide nutritional and/or detoxifying functions and/or supplement primary and secondary metabolic processes by producing or providing precursors to bark beetle pheromones and hormones (Six, 2003, 2013). For these reasons, bark beetles typically have greatly decreased fecundity or fail to reproduce without their primary fungal symbiont (Six, 2003). If G. morbida provided such benefits to P. juglandis, the beetle would be expected to have lower establishment success in areas where G. morbida is unable to compete with other locally adapted fungi unless alternative symbionts were available (Six and Bentz, 2007).
Our mapping of expected survival of G. morbida across historic and future climatic conditions also rests on the additional key assumption that MC of inner bark and outer sapwood fully equilibrate to ambient conditions within the timeframe of the P. juglandis lifecycle. Woody tissues lose moisture quickly following successful attacks by bark beetles and other wood-boring insects (Nikolov and Encev, 1967; Pinard and Huffman, 1997; Magnussen and Harrison, 2008; Negi and Joshi, 2009; Lawes et al., 2011). Under constant environmental conditions, EMC of bark also reflects that of the wood (Martin, 1967) across angiosperms and gymnosperms (Reeb and Brown, 2007; Glass and Zelinka, 2010). Compared to other woody tissues, bark is by far the fastest to equilibrate its moisture level to ambient conditions (Negi and Joshi, 2009). Furthermore, humidity is low and vapor pressure deficit is high during spring and summer in the western United States, and senescent bark tissues are therefore likely to dry faster west of the Great Plains.
Natural History and Implications for Forest Health in a Changing Climate
Pityophthorus juglandis and G. morbida have a co-evolved history in native forest ecosystems of the western and southwestern United States, where they would be presumed to be coadapted with their ancestral host, J. major (Seybold et al., 2012; Hadziabdic et al., 2014; Zerillo et al., 2014; Rugman-Jones et al., 2015). An ancestral Juglans sect. Rhysocaryon sp. diverged into J. major, J. nigra, and other species during a climatologically cooler period, 2.6–5.3 Ma (Stone et al., 2009; Mu et al., 2020; Song et al., 2020). J. nigra would then have radiated across humid eastern North America during interglacial periods. Over the last 10,000 years, J. major, P. juglandis, and G. morbida were restricted to moist canyons and cooler montane regions of the arid southwestern United States and Mexico (Little, 1976), and TCD was likely precluded from the current range of J. nigra by a lack of connectivity in host populations as well as an unfavorable moisture regime for the fungus. Across the state of Kansas alone, relative humidity varies twofold from ∼40% near the Colorado border in the West to ∼80% along the Missouri River [National Oceanic and Atmospheric Administration (NOAA), 2021], which corresponds to ∼7.5 and 15.6% EMC (Glass and Zelinka, 2010) at the thermal optimum for G. morbida growth of 30°C (Kolařík et al., 2011).
However, in the far western portion of its range, J. nigra is a strictly riparian species, much like its western relatives including J. microcarpa in Texas (TX) and Oklahoma (OK). This adaptation for riparian areas, along with the activities of humans who used black walnut for food, fiber, and fuel, likely permitted eastern–western connectivity between J. nigra, J. major and J. microcarpa over the last 10,000 years. Evidence that western populations of J. nigra have the greatest resistance to G. morbida provides support for interceding periods of genetic connectivity with J. major and periodic pressure from TCD in western J. nigra (Sitz et al., 2021). Transient periods of connectivity favored by shifting climatic conditions may have facilitated gene flow through hybridization zones and provided a corridor for pest-pathogen complexes such as TCD to reach far-western J. nigra populations in the Great Plains.
During the last 200 years, the introduction of evolutionarily naïve (Ploetz et al., 2013) eastern J. nigra families to regions where environmental conditions remain favorable to the development of TCD may have led to runaway mortality in urban forests and plantations in the western United States (Tisserat et al., 2011; Seybold et al., 2019; Moricca et al., 2020). Moreover, the failure of P. juglandis to successfully establish in the native range of J. nigra despite incidental introductions and high host susceptibility may be due to unfavorable conditions for its symbiont (Utley et al., 2013; Moore et al., 2019), in addition to differences in temperature and seasonal precipitation that may affect beetle flight, dispersal, and survival (Luna et al., 2013; Hefty et al., 2017, 2018; Chen et al., 2020).
Conclusion and Relevance to Other Pathosystems
The geographical and realized host ranges of destructive, native, and non-native forest insects will continue to expand as global temperatures rise and climates shift in future decades (Cullingham et al., 2011; Ramsfield et al., 2016; Pureswaran et al., 2018). Such expansions are strongly determined by the environmental conditions that support the growth and reproduction of beetles and their symbiotic fungi (Six and Bentz, 2007). Based on our findings, these conditions include MC and its influence on competition between G. morbida and secondary fungi in walnut wood.
The effect of climatic conditions on fungal competition and dispersal may be applied to other emergent beetle-fungal disease complexes, but its relevance will depend on the nature of the symbiosis. Other factors determining the relevance of fungal competition in other pathosystems include whether the fungus is obligate for the beetle, availability of alternative fungal symbionts (Six and Bentz, 2007), strength of the pathogen, and the ecophysiology of the fungal symbiont and competing fungi. Geosmithia morbida is highly xerotolerant (Williams and Newcombe, 2017), while other primary fungal symbionts of bark beetles in the Ophiostomatales and Microascales may be less xerotolerant (Kirisits, 2013). These fungi may also vary in their ability to withstand desiccation (Temple et al., 1997) and/or more highly virulent (Caballero et al., 2019) and, thus, less likely to be limited by other fungi or the presence of moderate wood moisture.
Juglans nigra is among the most valuable hardwood species native to the eastern United States (Duval et al., 2013), with an estimated value of over USD 500 billion in merchantable timber alone (Newton and Fowler, 2009). Our study of fungal competition in walnut wood indicates that TCD presents a risk to the long-term sustainability of J. nigra within its native range. In light of these findings, investigations of bark beetle–fungal mutualisms will be critical to build a better understanding of the joint influence that climate and biotic interactions have on the reproductive success of pathogens and their vectors and forest disease epidemiology.
Data Availability Statement
The datasets presented in this study can be found in online repositories. The names of the repository/repositories and accession number(s) can be found below: https://www.ncbi.nlm.nih.gov/genbank/, accession numbers MW584687-MW584698. All data and R scripts are available at: https://github.com/readingradio/WilliamsGinzel.TCD.Xerotolerance.2021.
Author Contributions
GW and MG conceived of the project and wrote the manuscript. GW performed the experiments and analyses. Both the authors contributed to the article and approved the submitted version.
Funding
This project was supported by a Fred M. Van Eck Foundation Memorial Scholarship from Purdue University.
Conflict of Interest
The authors declare that the research was conducted in the absence of any commercial or financial relationships that could be construed as a potential conflict of interest.
Publisher’s Note
All claims expressed in this article are solely those of the authors and do not necessarily represent those of their affiliated organizations, or those of the publisher, the editors and the reviewers. Any product that may be evaluated in this article, or claim that may be made by its manufacturer, is not guaranteed or endorsed by the publisher.
Acknowledgments
We thank Caleb Kell, Holly Wantuch, Felix Coronado, Ashley Howes, and Dan Bollock for technical support. We also thank Bart Nelson, Brian Beheler, Jim McKenna, and Dan Cassens for access to field sites, host material, and assistance. Publication of this article was funded in part by Purdue University Libraries Open Access Publishing Fund. This research was in partial fulfillment of a doctoral degree for GW from Purdue University.
Footnotes
References
Abatzoglou, J. T. (2013). Development of gridded surface meteorological data for ecological applications and modelling. Int. J. Climatol. 33, 121–131. doi: 10.1002/joc.3413
Bright, D. E. (1981). Taxonomic monograph of the genus Pityophthorus eichoff in North and Central Americs (Coleoptera: Scolytidae). Mem. Entomol. Soc. Canada 113, 1–378. doi: 10.4039/entm113118fv
Caballero, J. R. I., Jeon, J., Lee, Y. H., Fraedrich, S., Klopfenstein, N. B., Kim, M. S., et al. (2019). Genomic comparisons of the laurel wilt pathogen, Raffaelea lauricola, and related tree pathogens highlight an arsenal of pathogenicity related genes. Fun. Gen. Biol. 125, 84–92. doi: 10.1016/j.fgb.2019.01.012
Carbone, I., White, J. B., Miadlikowska, J., Arnold, A. E., Miller, M. A., Magain, N., et al. (2019). T-BAS version 2.1: tree-based alignment selector toolkit for evolutionary placement of DNA sequences and viewing alignments and specimen metadata on curated and custom trees. Microbiol. Resour. Announc. 8:e00328-19.
Chen, Y., Aukema, B. H., and Seybold, S. J. (2020). The effects of weather on the flight of an invasive bark beetle, Pityophthorus juglandis. Insects 11, 156. doi: 10.3390/insects11030156
Cullingham, C. I., Cooke, J. E. K., Dang, S., Davis, C. S., Cooke, B. J., and Coltman, D. W. (2011). Mountain pine beetle host-range expansion threatens the boreal forest. Mol. Ecol. 20, 2157–2171. doi: 10.1111/j.1365-294x.2011.05086.x
Deshpande, V., Wang, Q., Greenfield, P., Charleston, M., Porras-Alfaro, A., Kuske, C. R., et al. (2016). Fungal identification using a Bayesian classifier and the Warcup training set of internal transcribed spacer sequences. Mycologia 108, 1–5. doi: 10.3852/14-293
Duval, R. P., McConnell, T. E., and Hix, D. M. (2013). Annual change in Ohio hardwood stumpage prices, 1960 to 2011. For. Prod. J. 64, 19–25. doi: 10.13073/FPJ-D-13-00075
Eckelman, C. A. (1998). The Shrinking and Swelling of Wood and Its Effect on Furniture. Purdue University Cooperative Extension Service. FNR 163. Available online at: https://www.extension.purdue.edu/extmedia/fnr/fnr-163.pdf (accessed August 17, 2021).
Fierer, N. (2017). Embracing the unknown: disentangling the complexities of the soil microbiome. Nat. Rev. Microbiol. 15, 579–590. doi: 10.1038/nrmicro.2017.87
Francke-Grosmann, H. (1967). “Ectosymbiosis in wood-inhabiting insects,” in Symbiosis, ed. S. M. Henry (New York, NY: Academic Press), 141–205. doi: 10.1016/b978-1-4832-2758-0.50010-2
Gazis, R., Poplawski, L., Klingeman, W., Boggess, S. L., Trigiano, R. N., Graves, A. D., et al. (2018). Mycobiota associated with insect galleries in walnut with thousand cankers disease reveals a potential natural enemy against Geosmithia morbida. Fungal Biol. 122, 241–253. doi: 10.1016/j.funbio.2018.01.005
Glass, S. V., and Zelinka, S. L. (2010). “Chapter 4: moisture relations and physical properties of wood,” in Forest Products Laboratory. Wood Handbook: Wood as an Engineering Material, Centennial, Vol. 2010, ed. General Technical Report FPL-GTR-190 (Madison, WI: U.S. Dept. of Agriculture, Forest Service, Forest Products Laboratory), 4.1–4.19.
Greenspan, L. (1977). Humidity fixed points of binary saturated aqueous solutions. J. Res. Natl. Bur. Stand. 81, 89–96. doi: 10.6028/jres.081a.011
Griffin, D. M. (1977). Water potential and wood-decay fungi. Annu. Rev. Phytopathol. 15, 315–326. doi: 10.1146/annurev.py.15.090177.001535
Griffin, G. J. (2015). Status of thousand cankers disease on eastern black walnut in the eastern United States at two locations over 3 years. For. Pathol. 45, 203–214. doi: 10.1111/efp.12154
Hadziabdic, D., Vito, L. M., Windham, M. T., Pscheidt, J. W., Trigiano, R. N., and Kolarik, M. (2014). Genetic differentiation and spatial structure of Geosmithia morbida, the causal agent of thousand cankers disease in black walnut (Juglans nigra). Curr. Genet. 60, 75–87. doi: 10.1007/s00294-013-0414-x
Harris, R. F. (1981). “Effect of water potential on microbial growth and activity,” in Water Potential Relations Soil Microbiology, Vol. 9, eds J. F. Parr, W. R. Gardner, and L. F. Elliot (Madison, WI: Soil Science Society of America), 23–95. doi: 10.2136/sssaspecpub9
Hefty, A. R., Aukema, B. H., Venette, R. C., Coggeshall, M. V., McKenna, J. R., and Seybold, S. J. (2018). Reproduction and potential range expansion of walnut twig beetle across the Juglandaceae. Biol. Invasions 20, 2141–2155. doi: 10.1007/s10530-018-1692-5
Hefty, A. R., Seybold, S. J., Aukema, B. H., and Venette, R. C. (2017). Cold tolerance of Pityophthorus juglandis (Coleoptera: Scolytidae) from northern California. Environ. Entomol. 46, 967–977. doi: 10.1093/ee/nvx090
Hofstetter, R. W., Dempsey, T., Klepzig, K., and Ayres, M. (2007). Temperature-dependent effects on mutualistic, antagonistic, and commensalistic interactions among insects, fungi and mites. Commun. Ecol. 8, 47–56. doi: 10.1556/comec.8.2007.1.7
Hofstetter, R. W., Dinkins-Bookwalter, J., Davis, T. S., and Klepzig, K. D. (2015). “Symbiotic associations of bark beetles,” in Bark Beetles: Biology and Ecology of Native and Invasive Species, eds F. Vega and R. Hofstetter (San Diego, CA: Academic Press), 209–245. doi: 10.1016/b978-0-12-417156-5.00006-x
Imhoff, M. L., Zhang, P., Wolfe, R. E., and Bounoua, L. (2010). Remote sensing of the urban heat island effect across biomes in the continental USA. Remote Sens. Environ. 114, 504–513. doi: 10.1016/j.rse.2009.10.008
IPCC (2014). Climate Change 2014: Synthesis Report. Contribution of Working Groups I, II and III to the Fifth Assessment Report of the Intergovernmental Panel on Climate Change, eds R. K. Pachauri and L. A. Meyer (Geneva: IPCC), 151.
Jacobi, W. R., Hardin, J. G., Goodrich, B. A., and Cleaver, C. M. (2012). Retail firewood can transport live tree pests. J. Econ. Entomol. 105, 1645–1658. doi: 10.1603/ec12069
Juzwik, J., Moore, M., Williams, G., and Ginzel, M. (2020). “Assessment and etiology of thousand cankers disease within the native range of black walnut (Juglans nigra),” in Forest Health Monitoring: National Status, Trends, and Analysis 2019. Gen. Tech. Rep. SRS-250, eds K. M. Potter and B. L. Conkling (Asheville, NC: US Department of Agriculture, Forest Service, Southern Research Station), 169–178.
Kirisits, T. (2013). “12 Dutch Elm disease and other ophiostoma diseases,” in Infectious Forest Diseases, eds P. Gonthier and G. Nicolotti (Wallingford: CAB International), 256. doi: 10.1079/9781780640402.0256
Kolařík, M., Freeland, E., Utley, C., and Tisserat, N. (2011). Geosmithia morbida sp. nov., a new phytopathogenic species living in symbiosis with the walnut twig beetle (Pityophthorus juglandis) on Juglans in USA. Mycologia 103, 325–332. doi: 10.3852/10-124
Lawes, M. J., Richards, A., Dathe, J., and Midgley, J. J. (2011). Bark thickness determines fire resistance of selected tree species from fire-prone tropical savanna in north Australia. Plant Ecol. 212, 2057–2069. doi: 10.1007/s11258-011-9954-7
Little, E. L. (1976). Atlas of United States Trees: Minor Western Hardwoods, Vol. 3. Washington, DC: U.S. Department of Agriculture, Forest Service.
Luna, E. K., Sitz, R. A., Cranshaw, W. S., and Tisserat, N. A. (2013). The effect of temperature on survival of Pityophthorus juglandis (Coleoptera: Curculionidae). Environ. Entomol. 42, 1085–1091. doi: 10.1603/en13151
Magnussen, S., and Harrison, D. (2008). Temporal change in wood quality attributes in standing dead beetle-killed lodgepole pine. For. Chron. 84, 392–400. doi: 10.5558/tfc84392-3
Manzoni, S., Schimel, J. P., and Porporato, A. (2012). Responses of soil microbial communities to water stress: results from a meta-analysis. Ecology 93, 930–938. doi: 10.1890/11-0026.1
Miller, M. A., Schwartz, T., Pickett, B. E., He, S., Klem, E. B., Scheuermann, R. H., et al. (2015). A RESTful API for access to phylogenetic tools via the CIPRES science gateway. Evol. Bioinform. Online 11, 43–48. doi: 10.4137/EBO.S21501
Mitton, J. B., and Ferrenberg, S. M. (2012). Mountain pine beetle develops an unprecedented summer generation in response to climate warming. Am. Nat. 179, E163–E171.
Moore, G. E. (1971). Mortality factors caused by pathogenic bacteria and fungi of the southern pine beetle in North Carolina. J. Invertebr. Pathol. 17, 28–37. doi: 10.1016/0022-2011(71)90121-2
Moore, G. E. (1973). Pathogenicity of three entomogenous fungi to the southern pine beetle at various temperatures and humidities. Environ. Entomol. 2, 54–57. doi: 10.1093/ee/2.1.54
Moore, M., Juzwik, J., Miller, F., Roberts, L., and Ginzel, M. D. (2019). Detection of Geosmithia morbida on numerous insect species in four eastern states. Plant Heal. Prog. 20, 133–139. doi: 10.1094/php-02-19-0016-rs
Moricca, S., Bracalini, M., Benigno, A., Ghelardini, L., Furtado, E. L., Marino, C. L., et al. (2020). Observations on the non-native thousand cankers disease of walnut in Europe’s southernmost outbreak. Glob. Ecol. Conserv. 23:e01159. doi: 10.1016/j.gecco.2020.e01159
Mu, X.-Y., Tong, L., Sun, M., Zhu, Y.-X., Wen, J., Lin, Q.-W., et al. (2020). Phylogeny and divergence time estimation of the walnut family (Juglandaceae) based on nuclear RAD-Seq and chloroplast genome data. Mol. Phylogenet. Evol. 147:106802. doi: 10.1016/j.ympev.2020.106802
National Oceanic and Atmospheric Administration (NOAA) (2020). Historical Palmer Drought Indices. Available online at: https://www.ncdc.noaa.gov/temp-and-precip/drought/historical-palmers/ (accessed February 11, 2020).
National Oceanic and Atmospheric Administration (NOAA) (2021). Climate Data Online. Available online at: https://www.ncdc.noaa.gov/cdo-web/ (accessed July 27, 2021).
Negi, S., and Joshi, V. D. (2009). Role of moisture content in rendering the sal tree component susceptible to the borer (Hoplocerambyx spinicornis) attack. Asian J. Anim. Sci. 3, 190–192.
Newcombe, G. (2011). “Endophytes in forest management: four challenges,” in Endophytes of Forest Trees, eds A. M. Pirttilä and A. C. Frank (Berlin: Springer), 251–262. doi: 10.1007/978-94-007-1599-8_16
Newton, L., and Fowler, G. (2009). Pathway Assessment: Geosmithia sp. and Pityophthorus juglandis Blackman movement from the western into the eastern United States. Available online at: https://www.uaex.edu/environment-nature/ar-invasives/invasive-diseases/docs/Thousand-Cankers%20Disease.pdf (accessed August 17, 2021).
Nuotclà, J. A., Biedermann, P. H. W., and Taborsky, M. (2019). Pathogen defence is a potential driver of social evolution in ambrosia beetles. Proc. R. Soc. B 286:20192332. doi: 10.1098/rspb.2019.2332
Onufrak, A. J., Williams, G. M., Klingeman, W. E., Cregger, M. A., Klingeman, D. M., DeBruyn, J. M., et al. (2020). Regional differences in the structure of Juglans nigra phytobiome reflect geographical differences in thousand cankers disease severity. Phytobiomes J. 4, 388–404. doi: 10.1094/PBIOMES-05-20-0044-R
Palmer, W. C. (1965). Meteorologic Drought. U.S. Weather Bureau, Research Paper No. 45. Washington, DC: U.S. Weather Bureau.
Pinard, M. A., and Huffman, J. (1997). Fire resistance and bark properties of trees in a seasonally dry forest in eastern Bolivia. J. Trop. Ecol. 13, 727–740. doi: 10.1017/S0266467400010890
Ploetz, R. C., Hulcr, J., Wingfield, M. J., and de Beer, Z. W. (2013). Destructive tree diseases associated with ambrosia and bark beetles: black swan events in tree pathology? Plant Dis. 97, 856–872. doi: 10.1094/PDIS-01-13-0056-FE
Pureswaran, D. S., Roques, A., and Battisti, A. (2018). Forest insects and climate change. Curr. For. Rep. 4, 35–50. doi: 10.1007/s40725-018-0075-6
R Core Team (2019). R: A Language and Environment for Statistical Computing. Vienna: R Foundation for Statistical Computing.
Ramsfield, T. D., Bentz, B. J., Faccoli, M., Jactel, H., and Brockerhoff, E. G. (2016). Forest health in a changing world: effects of globalization and climate change on forest insect and pathogen impacts. For. An Int. J. For. Res. 89, 245–252. doi: 10.1093/forestry/cpw018
Ranger, C. M., Biedermann, P. H. W., Phuntumart, V., Beligala, G. U., Ghosh, S., Palmquist, D. E., et al. (2018). Symbiont selection via alcohol benefits fungus farming by ambrosia beetles. Proc. Natl. Acad. Sci. U.S.A. 115:201716852. doi: 10.1073/pnas.1716852115
Rayner, A. D. M., and Boddy, L. (1988). Fungal Decomposition of Wood: Its Biology and Ecology. Hoboken, NJ: Wiley and Sons, doi: 10.1086/416403
Reeb, J., and Brown, T. (2007). Air- and Shed-Drying Lumber. Oregon State University Extension EM 8612-E. Available online at: https://catalog.extension.oregonstate.edu/sites/catalog/files/project/pdf/em8612.pdf (accessed August 17, 2021).
Ridout, M., Houbraken, J., and Newcombe, G. (2017). Xerotolerance of Penicillium and Phialocephala fungi, dominant taxa of fine lateral roots of woody plants in the intermountain Pacific Northwest. USA. Rhizosphere 4, 94–103. doi: 10.1016/j.rhisph.2017.09.004
Rugman-Jones, P. F., Seybold, S. J., Graves, A. D., and Stouthamer, R. (2015). Phylogeography of the walnut twig beetle, Pityophthorus juglandis, the vector of thousand cankers disease in North American walnut trees. PLoS One 10:e0118264. doi: 10.1371/journal.pone.0118264
Seybold, S. J., Coleman, T. W., Dallara, P. L., Dart, N. L., Graves, A. D., Pederson, L. A., et al. (2012). Recent collecting reveals new state records and geographic extremes in the distribution of the walnut twig beetle, Pityophthorus juglandis Blackman (Coleoptera: Scolytidae), in the United States. Pan Pac. Entomol. 88, 277–280. doi: 10.3956/2012-32.1
Seybold, S. J., Klingeman, W. E. III, Hishinuma, S. M., Coleman, T. W., and Graves, A. D. (2019). Status and Impact of Walnut twig beetle in Urban Forest, Orchard, and native forest ecosystems. J. For. 117, 152–163. doi: 10.1093/jofore/fvy081
Seybold, S. J., Penrose, R. L., and Graves, A. D. (2016). “Invasive bark and ambrosia beetles in California Mediterranean forest ecosystems,” in Insects and Diseases of Mediterranean Forest Systems, eds T. D. Paine and F. Lieutier (Berlin: Springer), 583–662. doi: 10.1007/978-3-319-24744-1_21
Simpson, W. T. (1973). Predicting equilibrium moisture content of wood by mathematical models. Wood Fiber Sci. 5, 41–49.
Simpson, W. T. (1998). Equilibrium Moisture Content of Wood in Outdoor Locations in the United States and Worldwide. FPL-RN-0268. Madison, WI: US Dept. of Agriculture, Forest Service.
Sing, T., Sander, O., Beerenwinkel, N., and Lengauer, T. (2005). ROCR: visualizing classifier performance in R. Bioinformatics 21:7881.
Sitz, R., Utley, C., Hall, A., Tisserat, N., and Cranshaw, W. (2017). Trapping the Walnut twig beetle to determine flight patterns in colorado. Southwest. Entomol. 42, 347–356. doi: 10.3958/059.042.0204
Sitz, R. A., Luna, E., Ibarra Caballero, J., Tisserat, N., Cranshaw, W. S., McKenna, J. R., et al. (2021). Eastern black walnut (Juglans nigra L.) originating from native range varies in their response to inoculation with Geosmithia morbida. Front. For. Global Change 4:12. doi: 10.3389/ffgc.2021.627911
Six, D. L. (2003). Bark beetle-fungus symbioses. Insect Symbiosis 1, 97–114. doi: 10.1201/9780203009918.ch7
Six, D. L. (2013). The bark beetle holobiont: why microbes matter. J. Chem. Ecol. 39, 989–1002. doi: 10.1007/s10886-013-0318-8
Six, D. L., and Bentz, B. J. (2007). Temperature determines symbiont abundance in a multipartite bark beetle-fungus ectosymbiosis. Microb. Ecol. 54, 112–118. doi: 10.1007/s00248-006-9178-x
Song, Y.-G., Fragnière, Y., Meng, H.-H., Li, Y., Bétrisey, S., Corrales, A., et al. (2020). Global biogeographic synthesis and priority conservation regions of the relict tree family Juglandaceae. J. Biogeogr. 47, 643–657. doi: 10.1111/jbi.13766
Stepanek, L. (2020). Walnut Twig Beetle & Thousand Cankers Disease. Lincoln, NE. Available online at: https://nfs.unl.edu/publications/walnut-twig-beetle-nebraska (accessed June 13, 2021).
Stone, D. E., Oh, S.-H., Tripp, E. A., and Manos, P. S. (2009). Natural history, distribution, phylogenetic relationships, and conservation of Central American black walnuts (Juglans sect. Rhysocaryon). J. Torrey Bot. Soc. 136, 1–25. doi: 10.3159/08-ra-036r.1
Temple, B., Horgen, P. A., Bernier, L., and Hintz, W. E. (1997). Cerato-ulmin, a hydrophobin secreted by the causal agents of Dutch elm disease, is a parasitic fitness factor. Fun. Gen. Biol. 22, 39–53. doi: 10.1006/fgbi.1997.0991
Tisserat, N., Cranshaw, W., Leatherman, D., Utley, C., and Alexander, K. (2009). Black Walnut Mortality in Colorado caused by the walnut twig beetle and thousand cankers disease. Plant Heal. Prog. 11:10. doi: 10.1094/php-2009-0811-01-rs
Tisserat, N., Cranshaw, W., Putnam, M. L., Pscheidt, J., Leslie, C. A., Murray, M., et al. (2011). Thousand cankers disease is widespread in black Walnut in the Western United States. Plant Heal. Prog. 12:35. doi: 10.1094/PHP-2011-0630-01-BR
USGCRP (2018). “Chapter 24: Northwest,” in Impacts, Risks, and Adaptation in the United States: Fourth National Climate Assessment, Vol. II, eds D. R. Reidmiller, C. W. Avery, D. R. Easterling, K. E. Kunkel, K. L. M. Lewis, T. K. Maycock, et al. (Washington, DC: U.S. Global Change Research Program). doi: 10.7930/NCA4.2018
Utley, C., Nguyen, T., Roubtsova, T., Coggeshall, M., Ford, T. M., Grauke, L. J., et al. (2013). Susceptibility of Walnut and Hickory species to Geosmithia morbida. Plant Dis. 97, 601–607. doi: 10.1094/PDIS-07-12-0636-RE
Weed, A. S., Ayres, M. P., and Bentz, B. J. (2015). “Population dynamics of bark beetles,” in Bark Beetles: Biology and Ecology of Native and Invasive Species, eds F. Vega and R. W. Hofstetter (San Diego, CA: Academic Press), 209–245. doi: 10.1016/B978-0-12-417156-5.00004-6
Whitaker, B. K., and Bakker, M. G. (2019). Bacterial endophyte antagonism toward a fungal pathogen in vitro does not predict protection in live plant tissue. FEMS Microbiol. Ecol. 95:fiy237.
Williams, G. M., and Ginzel, M. D. (2021). Forest and plantation soil microbiomes differ in their capacity to suppress feedback between geosmithia morbida and rhizosphere pathogens of J. nigra seedlings. Phytobiomes J. (in press).
Williams, G. M., and Newcombe, G. (2017). “Xerotolerance of Geosmithia morbida,” in Proceedings of the 2016 Society of American Foresters National Convention, Madison, WI. doi: 10.5849/jof.2016-108
Wood, S. L. (1982). The bark and ambrosia beetles of North and Central America (Coleoptera: Scolytidae), a taxonomic monograph. Great Basin Nat. Mem. 6:11356.
Keywords: climate change, forest health, walnut, wood sorption, fungi, competition, bark beetle, central hardwood region
Citation: Williams GM and Ginzel MD (2021) Competitive Advantage of Geosmithia morbida in Low-Moisture Wood May Explain Historical Outbreaks of Thousand Cankers Disease and Predict the Future Fate of Juglans nigra Within Its Native Range. Front. For. Glob. Change 4:725066. doi: 10.3389/ffgc.2021.725066
Received: 14 June 2021; Accepted: 04 August 2021;
Published: 08 September 2021.
Edited by:
Richard Hamelin, University of British Columbia, CanadaReviewed by:
Pedro Miguel Naves, Instituto Nacional Investigaciao Agraria e Veterinaria (INIAV), PortugalJennifer Gene Klutsch, University of Alberta, Canada
Copyright © 2021 Williams and Ginzel. This is an open-access article distributed under the terms of the Creative Commons Attribution License (CC BY). The use, distribution or reproduction in other forums is permitted, provided the original author(s) and the copyright owner(s) are credited and that the original publication in this journal is cited, in accordance with accepted academic practice. No use, distribution or reproduction is permitted which does not comply with these terms.
*Correspondence: Matthew D. Ginzel, bWdpbnplbEBwdXJkdWUuZWR1