- 1Forest Management Laboratory, National Institute for Amazon Research, Manaus, Brazil
- 2Climate and Ecosystem Sciences Division, Lawrence Berkeley National Laboratory, Berkeley, CA, United States
- 3Center for Distance Education, Federal University of Amazonas, Manaus, Brazil
- 4Center for Tropical Forest Science, Smithsonian Tropical Research Institute, Gamboa, Panama
- 5Environmental and Climate Sciences Department, Brookhaven National Laboratory, Upton, NY, United States
- 6Earth System Analysis and Modeling, Pacific Northwest National Laboratory, Richland, WA, United States
- 7Environmental Sciences Division and Climate Change Science Institute, Oak Ridge National Laboratory, Oak Ridge, TN, United States
Leaf respiration in the dark (Rdark) and light (Rday) is poorly characterized in diverse tropical ecosystems, and little to no information exists on the degree of light suppression in common tree species within the Amazon basin, and their dependences upon plant functional traits and position within the canopy. We quantified Rdark and apparent Rday using the Kok method and measured key leaf traits in 26 tree individuals of different species distributed in three different canopy positions: canopy, lower canopy, and understory. To explore the relationships between the leaf traits we used the standardized major axis (SMA). We found that canopy trees had significantly higher rates of Rdark and Rday than trees in the understory. The difference between Rdark and Rday (the light suppression of respiration) was greatest in the understory (68 ± 9%, 95% CI) and lower canopy (49 ± 9%, 95% CI) when compared to the canopy (37 ± 10%, 95% CI). We also found that Rday was significantly and strongly correlated with Rdark (p < 0.001) for all the canopy positions. Also, leaf mass per area (LMA) and leaf Phosphorus concentration (P) had a significant relationship with Rdark (p < 0.001; p = 0.003), respectively. In addition, a significant relationship was found for LMA in the canopy and lower canopy positions (p = 0.009; p = 0.048) while P was only significant in the canopy (p = 0.044). Finally, no significant relationship was found between Rdark and nitrogen, sugars, and starch. Our results highlight the importance of including representation of the light suppression of leaf respiration in terrestrial biosphere models and also of accounting for vertical gradients within forest canopies and connections with functional traits.
Introduction
Autotrophic respiration is one of the processes that strongly regulates both terrestrial and global carbon balances (Tang et al., 2019). However, large uncertainties remain in the magnitude of biological and environmental controls over tropical autotrophic respiration, largely due to limited observational studies requiring advanced technologies (e.g., portable photosynthesis systems) deployed to logistically challenging field sites. In tropical forests, limited estimates suggested that only 30% of the carbon fixed by photosynthesis is allocated to the formation of new tissues (biomass), with the rest being respired back into the atmosphere (Chambers et al., 2004; Malhi et al., 2014). Previous work has estimated that autotrophic respiration of tropical forests contributes with 20–29 Mg C ha year–1, mostly represented by leaf respiration, with an estimated 32–56% of the total, but this component is still highly uncertain (Chambers et al., 2004; Metcalfe et al., 2010; Malhi, 2012; Malhi et al., 2014). Both field observations and modeling development of autotrophic respiration are substantially less studied than photosynthesis (Huntingford et al., 2017). This might be explained by the difficulty in measuring foliar CO2 release (Meir et al., 2001; Chambers et al., 2004; Heskel, 2018) due to the lower fluxes and higher signal to noise ratio when compared to measurements of photosynthesis (Tcherkez et al., 2017a,b).
Leaf respiration can be substantially inhibited by light (Kok, 1948, 1949) and this metabolism is dynamic and complex, and regulated by changes in enzyme activities and interactions with photosynthesis, photorespiration, and other pathways such as nitrate assimilation (Tcherkez et al., 2017a,b). For example, Heskel and Tang (2018) showed that the net primary productivity (NPP) may be overestimated when the inhibition of the leaf respiration by light is not accounted for in a temperate forest. In addition, current leaf respiration models generally do not take into account changes in the vertical gradient of light availability within the forest, which is known to greatly influence plant metabolism (Weerasinghe et al., 2014; Heskel and Tang, 2018). Respiratory rates have also been associated with morphological and nutrient variation of leaves, such as leaf mass per area (LMA), nitrogen, and phosphorus concentration (Meir et al., 2001; Atkin et al., 2015; Crous et al., 2017; Rowland et al., 2017), and can be regulated by the availability of respiratory substrates, such as non-structural carbohydrates including soluble sugars and starch (Collalti et al., 2019).
The difference between apparent respiration in the light (Rday) and respiration in the dark (Rdark) is known as the light suppression of leaf respiration. The two main approaches used to estimate Rday and consequently light suppression are, the 13CO2 isotopic method (Loreto et al., 1999, 2001; Gong et al., 2018) and the Kok method (Kok, 1948, 1949). The isotopic method assumes that in an atmosphere of 13CO2 leaves will use it for photosynthesis, and all 12CO2 detected is from mitochondrial respiration. This method is based on the time of labeling the 13CO2 and 12CO2 release to separate photosynthesis and respiration, and is considered to be the most accurate method (Loreto et al., 2001; Tcherkez et al., 2017a). The Kok method uses the abrupt change in the initial slope of the light response curve of photosynthesis (i.e., sudden change in the quantum yield of photosynthesis at low light levels) to estimate Rday. A linear regression is adjusted between net photosynthesis and the irradiance points above the Kok “break.” The y-intercept of the fitted line provides an estimate of Rday (Farquhar and Busch, 2017; Tcherkez et al., 2017a,b; Way et al., 2018; Yin et al., 2020).
It is known that the Kok method can underestimate rates of light respiration (Gong et al., 2018; Way et al., 2018; Yin et al., 2020), but on the other hand is the most practical method to apply in logistically challenging field conditions (Tcherkez et al., 2017a). The Kok “effect” has been described as due to not only changes in respiratory rates with light, but also other physiological and biochemical process (Gauthier et al., 2020; Yin et al., 2020). At least four non-mutually exclusive phenomenon could explain the Kok “effect” including a decrease in the photochemical efficiency of photosynthesis, refixation of internal CO2 sources (respiration, photorespiration, etc.) by photosynthesis, and a decrease of mitochondrial respiration due to light inhibition of key enzymes including pyruvate dehydrogenase (Yin et al., 2020). CO2 refixation has been widely discussed, but there are still disagreements regarding its importance in the Kok “effect” (Buckley et al., 2017; Farquhar and Busch, 2017). In addition, interactions of respiratory substrates with other biochemical pathways during the day may also be involved (Tcherkez and Ghashghaie, 2017; Tcherkez et al., 2017a). For example, light-dependent biosynthetic pathways active during the day, including fatty acid biosynthesis (Sauer and Klaus-Peter, 1983).
Light is considered a limiting resource in forests understory varying as a function of height in the canopy (Mulkey et al., 1996). For this reason, trees modify their resource capture and use strategies according to light availability, reflecting changes in their morphological and nutrient composition, due to the high competition for light (Wright et al., 2004). Studies such as those by Kosugi et al. (2012), Weerasinghe et al. (2014), and Heskel and Tang (2018) reported a large effect of leaf position within the canopy on leaf respiration. However, little to no information exists on the magnitudes of leaf respiration in common tree species within the Amazon basin, and their dependence upon position within the canopy. Given the great biodiversity of tropical forests (Cardoso et al., 2017; ter Steege et al., 2020), the scarcity of leaf respiration measurements in the tropics, and the known high sensitivity of leaf respiration to canopy position (Griffin et al., 2002), there is an urgent need to quantify both Rdark and the suppression of respiration that occurs in the light. In particular, we need in situ measurements to know how it varies across common tree species, as a function of height within the forest.
Due to the great importance of the Amazon rainforest in the global climate context, this study aims to quantify the Rdark and Rday from leaf autotrophic respiration, the component that most contributes to total autotrophic respiration, and is the most sensitive to environmental change (Chambers et al., 2004; Malhi et al., 2014; Cavaleri et al., 2017). Thus, the questions that guided this work were: (i) Does the Kok method, which can be easily applied in the field, provide accurate estimates of Rday as same as the 13CO2 labeling method do?; (ii) What are the Rdark and Rday leaf respiration rates of common “Terra-firme” tree species in the Central Amazon, and what is the degree of light suppression?; (iii) Does canopy position influence the variation of leaf respiration rates and light suppression?; and lastly (iv) What are the relationships between respiratory rates and leaf functional traits? In the laboratory we compared estimates of Rdark and Rday, using two methods: the 13CO2 method based on a portable photosynthesis system coupled to a cavity ringdown isotopic analyzer for 13CO2 and 12CO2, and the Kok method. In the field, we used the Kok method to measure Rdark and Rday in 26 trees occupying three different canopy positions including the canopy, lower canopy, and understory where we also measured key leaf traits.
Materials and Methods
Determination of Leaf Dark Adapted RDark and RDay Using the Kok and 13CO2 Methods
For all gas exchange measurements, branches were cut and recut in a bucket with water to restore hydraulic conductivity (Wu et al., 2020). For all leaf gas exchange measurements reported here, the block temperature was set (Tblock) at 31°C, and leaf temperature was measured while the air flow rate through the chamber was maintained at 300 μmol s–1 with reference CO2 concentrations maintained at 400 ppm. For the Kok method, the respiratory CO2 flux of the sampled leaves was measured using a portable photosynthesis system, IRGA (Infrared gas analyzer), model (Li-6400XT, Li-Cor, Lincoln, NE, United States). Following the introduction of a leaf into the chamber with 100 μmol m–2 s–1 of photosynthetically active radiation (PAR), the two IRGAs were matched. Net photosynthetic assimilation values (Anet) were subsequently recorded for PAR values of 100, 90, 80, 70, 60, 50, 40, 30, 20, 15, 10, and 5 μmol m–2 s–1 (with IRGA matching before each recording). Following this, the light source was switched off (0 μmol m–2 s–1) and the leaf was allowed 10 minutes to acclimate in the dark before matching the IRGAs and recording the dark-adapted respiration rate (Rdark). Therefore, for the Kok method, which was applied both in the lab and field, Rdark was obtained from leaves dark adapted for 10 min following a reverse light curve starting at 100 μmol m–2 s–1 PAR photon flux. Due to the interactions that occur in the presence of light, the Kok method (Kok, 1948) was used to estimate the apparent respiratory rates in the light. The Kok method consists of determining Rday at low irradiance, due to the “break” that occurs in the light curve near the light compensation point. In this method, Rday is estimated as the intercept, on the “y” axis, of the net photosynthesis rate as a function of PAR, at low light intensity (Supplementary Figure 1). For each leaf, a linear regression using at least three points between 5 and 20 PAR was performed to estimate Rday. Results for both Rdark and Rday are presented as positive values but are measured as negative net photosynthesis values on the leaf gas exchange system. Data from the dark-adapted measurement of Rdark and the apparent estimate of light respiration (Rday), was used to calculate the light suppression, according to Equation 1:
For the 13CO2 method (Loreto et al., 2001), two modifications were made to the portable photosynthesis system including 1) a 400 ppm atmosphere of 99% 13CO2 (Cambridge Isotopes) was delivered to the leaf chamber and 2) a fraction (50 ml min–1) of the air exiting the leaf chamber was routed to a cavity ringdown isotopic analyzer for CO2 (G2131-i, Picarro) which measured the concentration of 13CO2 and 12CO2 exiting the leaf chamber. This method assumes that the leaf uses the 13CO2 atmosphere for photosynthesis with any 12CO2 detected deriving from leaf respiration (Loreto et al., 2001).
For the 13CO2 method applied only in the lab, the leaf was placed in the Li6400XT leaf chamber in the dark for 10 min and Rdark was obtained from dark adapted leaves. The difference in 12CO2 between the empty chamber and the chamber with the dark-adapted leaf was used to calculate Rdark using the 13CO2 method. Following dark respiration measurement, Rday was estimated at at 100 μmol m–2 s–1 PAR photon flux using the same method as in the dark. The experiments were carried out with a tropical tree species Couepia longipendula Pilg., growing near the laboratory and exposed to natural sunlight for 6–7 h per day. To compare the Kok and 13CO2 methods for determining Rday, Rdark, and the degree of suppression by light (%), both methods were performed on six different leaves from the same individual, but each on a different branch.
Field Study Site
Field data of 26 trees was collected at the Tropical Silviculture Experimental Station (E.E.S.T - ZF2), managed by the National Institute for Amazon Research (INPA), located approximately 60 km NW of Manaus, Brazil. The ZF-2 has an area of approximately 21,000 ha adjacent to extensive areas of undisturbed tropical forest. Data was collected in a permanent plot installed in 1996, known as North–South transect (2°35′40.3″S, 60°12′28.7″W), located at km 33 of the local road, covering an area of 5 ha (20 m × 2,500 m) (Araújo et al., 2020). The predominant vegetation in the reserve is type of Dense Tropical Rainforest of “Terra Firme” with a great diversity of woody and herbaceous species. The North-South transect presents in the plateau areas a predominance of species belonging to the families Lecythidaceae, Sapotaceae, Burseraceae, and Fabaceae. Among these families, the most common species belonged to the genera Eschweilera, Pouteria, Protium, Swartzia, and Inga (Carneiro, 2004).
Tree Species Selection in the Field
We sampled 26 trees (Table 1), all located along the North-South Transect, between 200 and 400 m of the transect length, in plateau areas. Access to the canopy leaves was obtained by climber who scaled trees and with a pole pruner removed the branches. Thus, the trees were selected taking into consideration: (i) ecological aspects, with a focus on highly abundant tree species in the Amazon basin (Ter Steege et al., 2013; Cardoso et al., 2017) and (ii) practical, related to climber access to the individual’s branches. The classification of crown illumination index was performed according to Clark and Clark (1992). In the 26 individuals studied, crowns were distributed in different classes (see Table 1). For the analysis, eight trees were classified in understory, nine in the lower canopy and nine in the canopy group. The ones with low, medium and high lateral light were associated to understory trees, the ones with some overhead light are lower canopy and trees with full overhead light were classified as canopy.
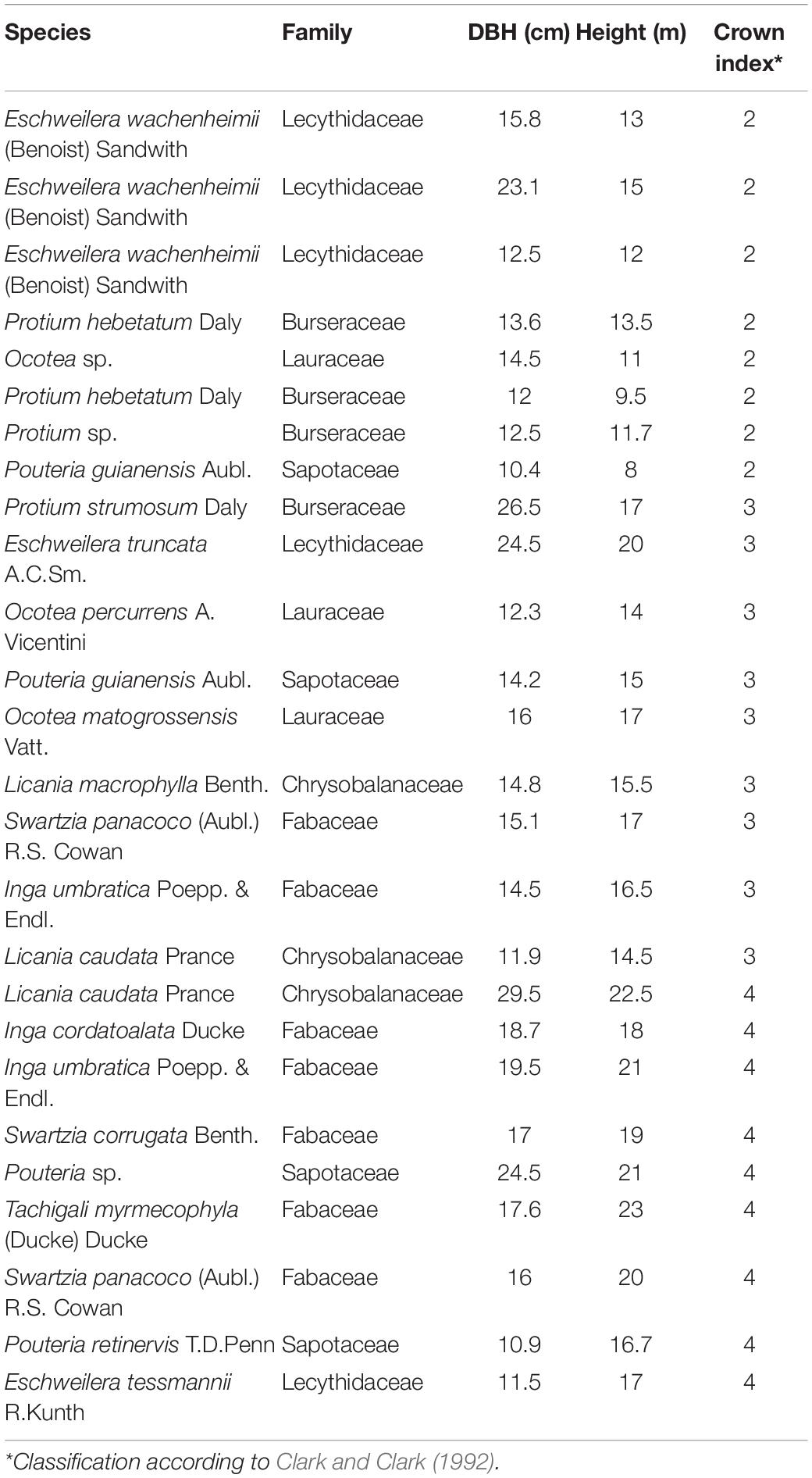
Table 1. List of the 26 individuals studied and their corresponding species, diameter at breast height (DBH), total height and crown illumination index (Clark and Clark, 1992).
Determination of Leaf RDark and RDay Using the Kok Method in the Field
The measurements were made in 2019 during three different field campaigns, in June/July, September and November. Data was collected between 8 am and 2 pm Local Time. The measurements were made using mature, fully expanded leaves with a good visual aspect. A fully expanded leaf per tree was selected for each field campaign. Due to the method of access to the canopy, the orientation for obtaining branches was not standardized and branches were collected on positions North, South, East, and West of the crown.
Leaf Traits
For LMA, three leaves were collected, including the one used for gas exchange measurements. From each leaf, we obtained disks of known diameter (1.7 cm). These were dried in an oven at 65°C for 72 h until constant mass was obtained to calculate the LMA (g m–2). For leaf nitrogen and phosphorus concentration, leaves were collected following the same drying process and subsequently the leaf samples were ground in a Wiley mill. The nitrogen (N) concentration was determined by the Kjeldahl method, and the phosphorus concentration (P) obtained by colorimetry and the absorbance readings made at 660 nm in a spectrophotometer using ammonium molybdate and 3% ascorbic acid. For nonstructural carbohydrates, the leaf samples were collected only in the first campaign (June/July), kept, and transported for 3 h in a box with ice with a temperature between 0 and 4°C to the laboratory in Manaus, Brazil (consistent with guidelines from Landhäusser et al., 2018). In the laboratory, they were placed in microwaves for 90 s and dried in an oven for 72 h at 65°C. The processed leaf samples were ground in a ball mill and sent to the Pacific Northwest National Laboratory in Washington State, United States for the quantification of non-structural carbohydrates including sugars, starch and total carbohydrates (NSC) as previously described (Dickman et al., 2019; Zhang et al., 2021).
Data Analysis
All data reported in the manuscript followed the format for leaf-level gas exchange recently described (Ely et al., 2021). All statistical analysis was performed using R version 3.6.0 (R Core Team, 2019) and IGOR Pro, version 6.37 (WaveMetrics, Inc., United States). To compare the methods used to estimate Rday, Rdark, and the degree of suppression by light (%) obtained from the Kok and 13CO2 methods, were performed a non-parametric test, Wilcoxon, (n = 4–6). In the field study, to verify the effect of canopy position on the studied variables, we performed a Kruskal–Wallis test, considering significant differences if p ≤ 0.05 (n = 3 replicate leaf sampling campaigns across 26 individuals). A Spearman correlation matrix (Supplementary Figure 2) was performed to evaluate the correlations for all the data (n = 77). To access the relationships between Rdark and leaf traits (i.e., Rday, LMA, N, P, sugars, starch, and total NSC concentrations), we applied the Standardized major axis (SMA) regression, using the Standardized Major Axis Tests and Routines (SMATR) package (Warton et al., 2012). For this analysis all the data were log transformed to assume normalize distribution. All the results are presented as mean ± 95% CI.
Results
Comparison Between Kok and 13CO2 Methods for Estimating RDay and the Degree of Light Suppression
In order to validate the Kok method for the determination of Rday in the remote central Amazon field site, an inter-comparison between Kok and 13CO2 methods for estimating Rday and the degree of light suppression was performed at the National Institute for Amazon Research (INPA) campus in Manaus, Brazil by taking advantage of a cavity ringdown CO2 isotope spectrometer that we interfaced to the portable photosynthesis system supplied with 13CO2 (see section “Materials and Methods”). Due to its proximity to the laboratory, we analyzed a tree from Couepia longipendula Pilg. We observed no statistical difference of dark respiration between the methods (p = 0.94, n = 6). The mean values of dark adapted Rdark and 13CO2 methods was 0.67 ± 0.17 and 0.68 ± 0.27 μmol CO2 m–2 s–1 (95% CI), respectively (Figure 1A). Both Kok and 13CO2 methods also observed that estimates of Rday were lower than Rdark (Figure 1B). The mean Rday values, determined by the Kok method was 0.36 ± 0.17 μmol CO2 m–2 s–1 and the 13CO2 method 0.27 ± 0.03 μmol CO2 m–2 s–1. Thus, the difference is not statistically significant for values of Rday observed between the methods (p = 0.73, n = 4). Finally, the percentage of leaf dark respiration suppression by light was also statistically equal between the Kok and 13CO2 methods (p = 0.41, n = 4), with 44 ± 17% (Kok) and 54 ± 17% (13CO2) (Figure 1C).
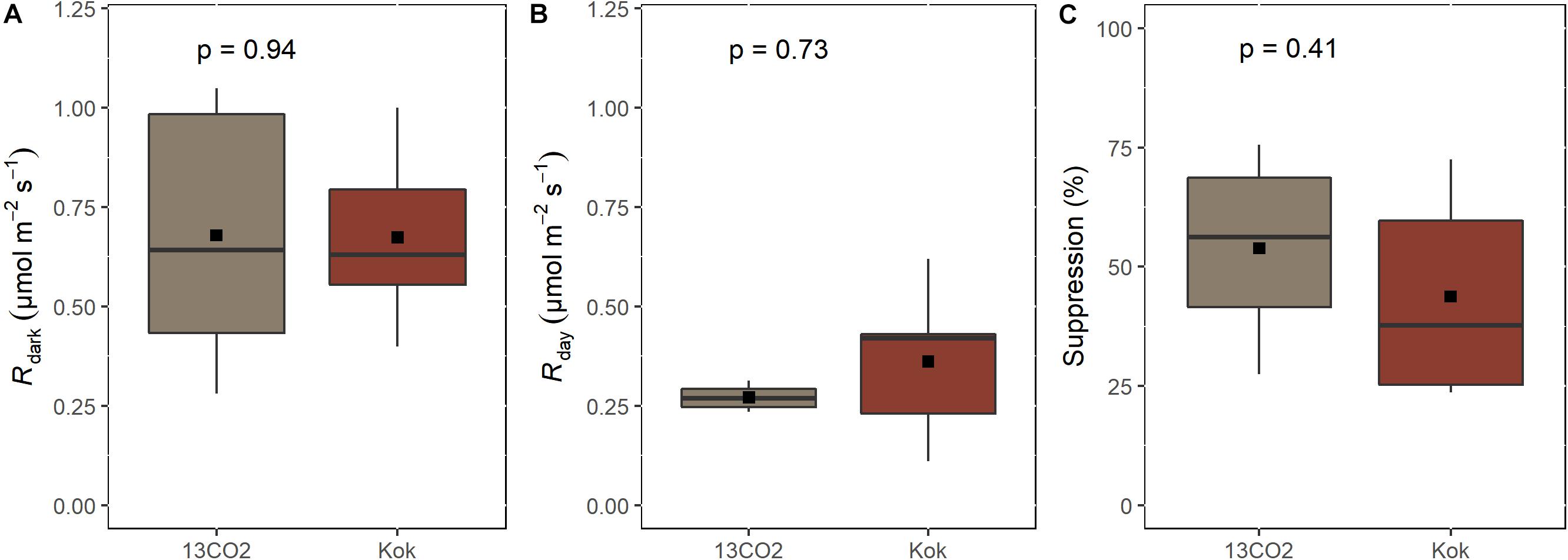
Figure 1. Comparison between Kok and 13CO2 method for estimating leaf respiration rates in the dark (Rdark) (A); in the light (Rday) (B), and the suppression of Rdark (Suppression) (C). The line inside the box indicates the median of the data, the colored bar represents the 50% variation of the data, the vertical lines represent the 90% of the variation, and the square point inside the colored box represents the mean. (n = 4–6) The test used was Wilcoxon-test.
Effect of Crown Position on Respiratory Rates and Degree of Light Suppression in a Central Amazon Forest Transect
Given the favorable sign of comparison between the Kok and 13CO2 methods for estimation of Rday and the light suppression of Rdark, we utilized the Kok method to determine these leaf respiratory rates in an established north–south forest transect with identified species in the central Amazon. Figure 2 summarizes the leaf respiration measurements from 26 individuals across 18 common species in the Amazon Forest, and grouped according to canopy position (understory, lower canopy, and canopy). The mean values of Rdark observed among the 26 individuals ranged from 0.19 ± 0.04 μmol CO2 m–2 s–1 to 0.90 ± 0.26 μmol CO2 m–2 s–1 (95% CI), whereas mean estimated values of Rday using the Kok method showed reduced values ranging from 0.01 ± 0.01 to 0.69 ± 0.27 μmol CO2 m–2 s–1. Thus, the light suppression of Rdark ranged between 17 ± 6% to 95 ± 4%. Moreover, the pattern of reduced Rday relative to Rdark was observed for each of the 26 individuals. Overall, a general pattern emerged that higher rates of Rdark and Rday were associated with lower values of light suppression of Rdark. Moreover, lower rates of Rdark and Rday were associated with higher values of light suppression of Rdark. Relative to canopy species, understory species tended to have lower rates of Rdark and Rday but higher values of light suppression of Rdark.
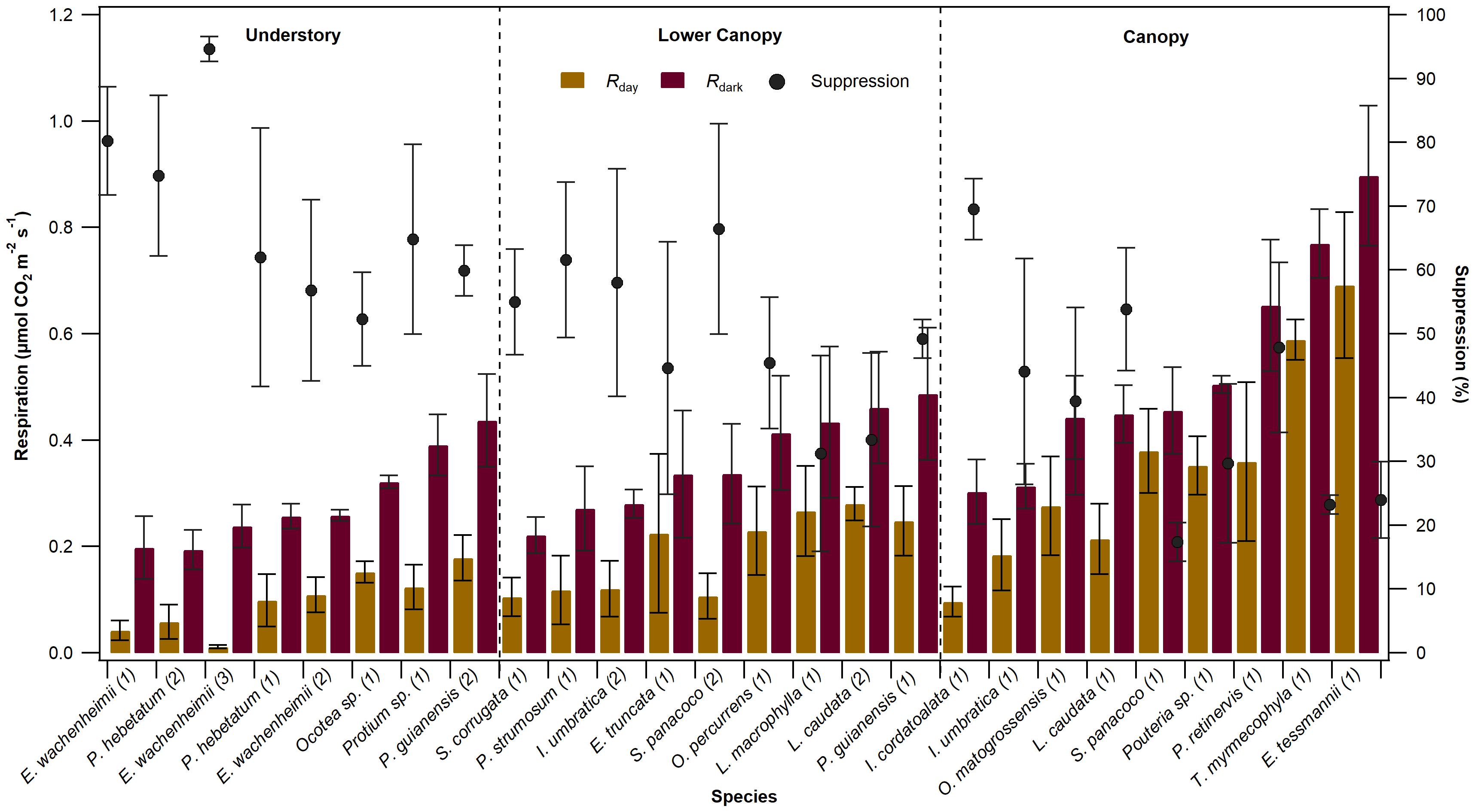
Figure 2. Respiration rate measured in the light (Rday, brown bars) and in dark adapted leaves (purple bar), and light suppression (black point) for each individual studied. The number after the specie name represent the number of individuals per specie studied. Values are mean ± standard error (n = 2–3). Data are organized in function of crown position, understory, lower canopy, and canopy.
Figure 3 shows the mean Anet versus PAR leaf gas exchange response curves for species in the canopy (Figure 3A), lower canopy (Figure 3B), and understory (Figure 3C). All three canopy positions showed the classic Kok effect where a sudden change in the gradient of the initial slope of net photosynthesis (Anet) occurred at low PAR intensities (0–15 μmol m–2 s–1) which corresponded to the light compensation point (1–6 μmol photons m–2 s–1). When the mean rate of Rdark and estimates of Rday and the percent light suppression of Rdark was obtained for each of the three canopy positions, a large effect of canopy position was observed. Rdark was the highest in canopy trees (0.50 ± 0.07 μmol of CO2 m–2 s–1) and lowest in the understory trees (0.25 ± 0.03 μmol of CO2 m–2 s–1) with the lower canopy trees with intermediate values of Rdark (0.36 ± 0.04 μmol of CO2 m–2 s–1) (Figure 3D). A similar pattern was observed for estimates of Rday using the Kok method with canopy trees showing the highest rates (0.32 ± 0.07 μmol of CO2 m–2 s–1), understory trees showing the lowest rates (0.10 ± 0.02 μmol of CO2 m–2 s–1), and intermediate rates in lower canopy trees (0.18 ± 0.03 μmol of CO2 m–2 s–1) (Figure 3E). Thus, there was a decrease in both Rdark and Rday with depth into the canopy. In contrast, the light suppression of Rdark showed a different trend with respect to canopy position with canopy trees showing the lowest values (37 ± 10%), understory trees showing the highest values (68 ± 9 μmol of CO2 m–2 s–1), and lower canopy trees showing intermediate values (49 ± 9 μmol of CO2 m–2 s–1) (Figure 3F). Thus, while Rdark and Rday decreased with depth into the forest canopy, the light suppression of Rdark increased. These trends were found to be statistically significant (p < 0.001, n = 74–77).
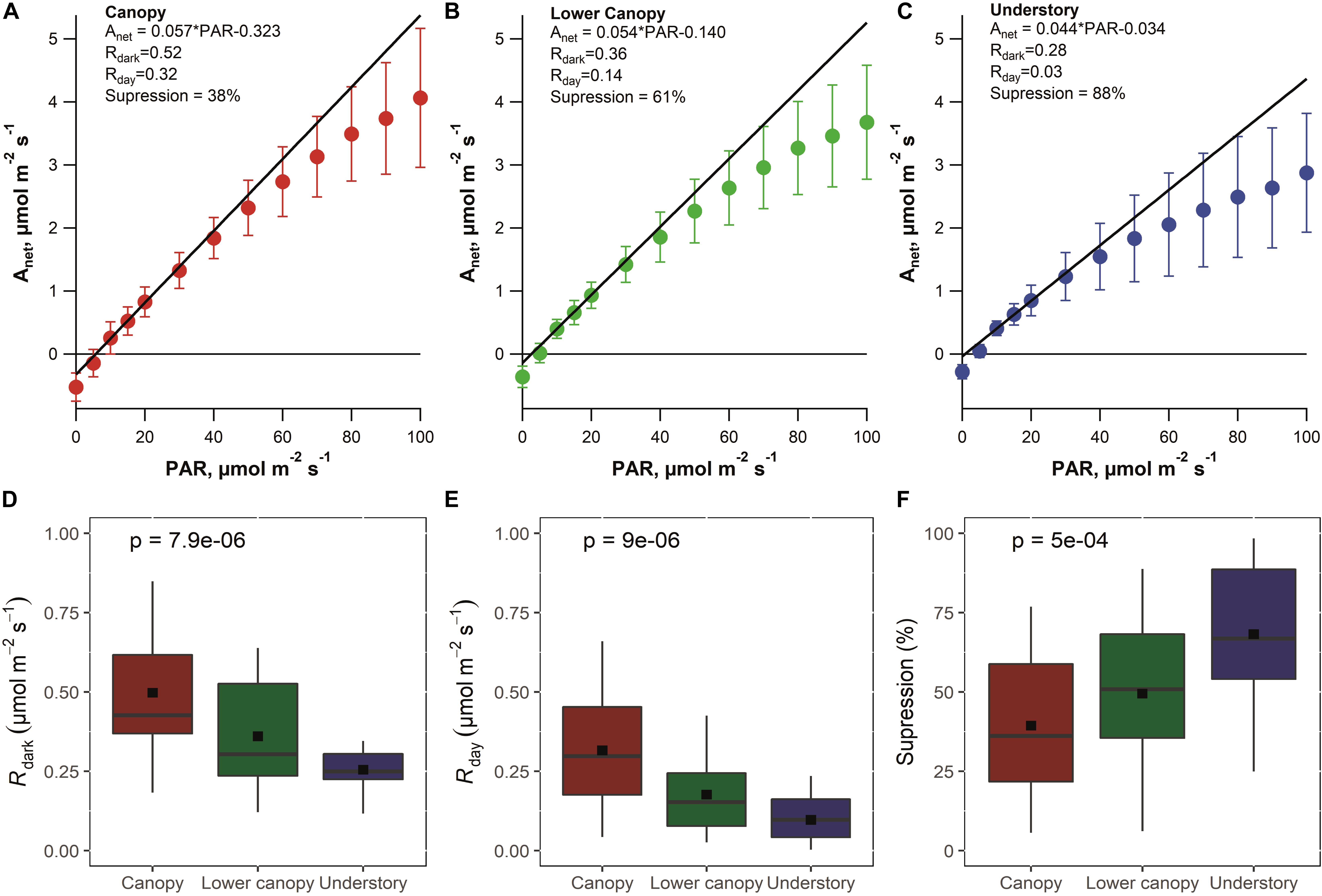
Figure 3. Light response curve showing the Kok effect for each class of crown position: (A) canopy, (B) lower canopy, and (C) understory. The linear regressions for each canopy position were adjusted using values between 10 and 20 of PAR. These values are related to the abrupt change in the quantum yield (QY) of net CO2 assimilation. The y-intercept of each adjusted linear regression was used to obtain apparent light respiration, and was estimated using the Kok method. Points are the average of leaves measured during 2019 in three different campaigns, and the vertical line represents the standard error (understory, n = 24; lower canopy, n = 27; canopy, n = 26). The second part of the figure shows the boxplots containing (D) respiration rates in the dark (Rdark), (E) apparent respiration rates in the light (Rday), and (F) apparent suppression of light respiration relative to the dark. Each colored box represents the variation of 50% of the data, the line inside each box indicates the median, the vertical lines represent the nineteenth percent of the variation, and the square point inside the colored box represents the mean (understory, n = 24; lower canopy, n = 27; canopy, n = 24–26). p values are shown for the non-parametric, Kruskal–Wallis test.
Relationships Between Respiratory Rates and Leaf Traits
The mean values of leaf mass area (LMA) were 99 ± 5 g m–2 in canopy trees, 88 ± 4 g m–2 in lower canopy trees and 86 ± 2 g m–2 in the understory trees. There were no statistically difference (p = 0.069, n = 77) between the groups (Supplementary Figure 3A). For N and P leaf concentration (Supplementary Figures 3B,C), no clear variation with canopy position was observed (p = 0.29; p = 0.28, n = 77). We also observed no statistical difference in concentration of sugars (p = 0.13, n = 26) and total NSC (p = 0.19, n = 26; Supplementary Figures 3D–F). Also, for starch there was no statistical difference between the groups (Supplementary Figure 3E; p = 0.47, n = 26), but the mean values for the canopy (0.68 ± 0.19%) was higher when compared to the lower canopy (0.44 ± 0.11%) and understory (0.40 ± 0.14%).
The SMA analysis showed a strong and positive correlation between Rday and Rdark (Figure 4A) for all the data and for all the canopy positions (Supplementary Table 1). The same pattern was observed for Rday and the percentage (%) of light suppression (Figure 4B). For LMA and Phosphorus, the SMA analysis showed a significant difference for each class of canopy position (Supplementary Table 2). The relationship between Rdark and LMA were not significant for the understory (R2 < 0.001, p = 0.91), while for phosphorus the regression was only significant for the canopy group (R2 = 0.17, p = 0.044). Finally, no significant correlations were observed between Rdark and nitrogen (R2 = 0.012, p = 0.278) or any of the components of NSCs (Figures 5D–F), including sugars (R2 = 0.04, p = 0.32), starch (R2 = 0.02, p = 0.51), and total NSC (R2 = 0.047, p = 0.29).
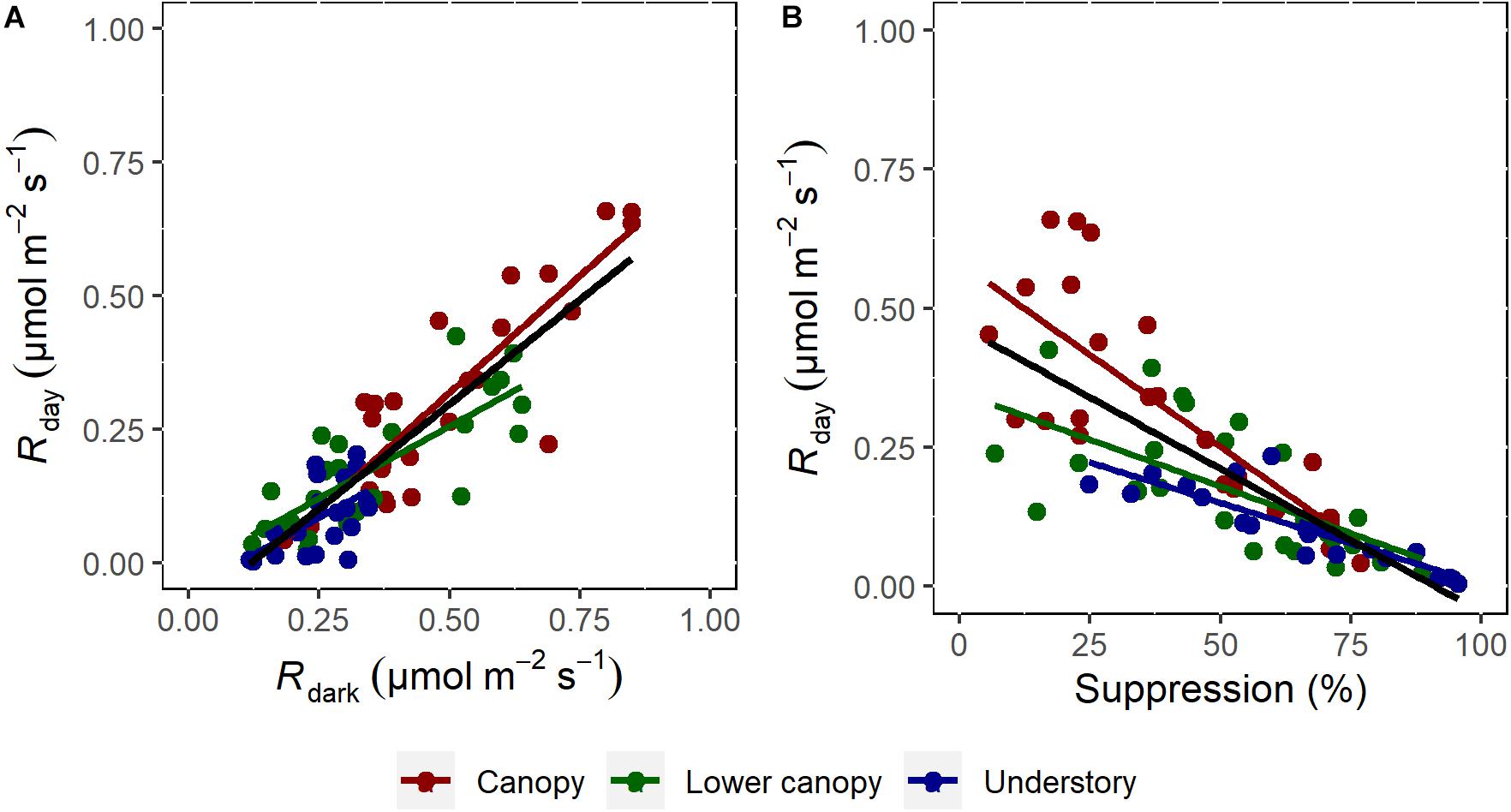
Figure 4. Respiration rates in the light as a function of respiration in the dark (A), and respiration in the light as a function of light suppression (B). The data were obtained from the measurements collected in the understory (blue), lower canopy (green), and canopy trees (red), n = 74–76. Results from the standardized major axis (SMA) regression are presented in Supplementary Table 1. Note that when no significant relationship between the variables was found, the regression line was suppressed.
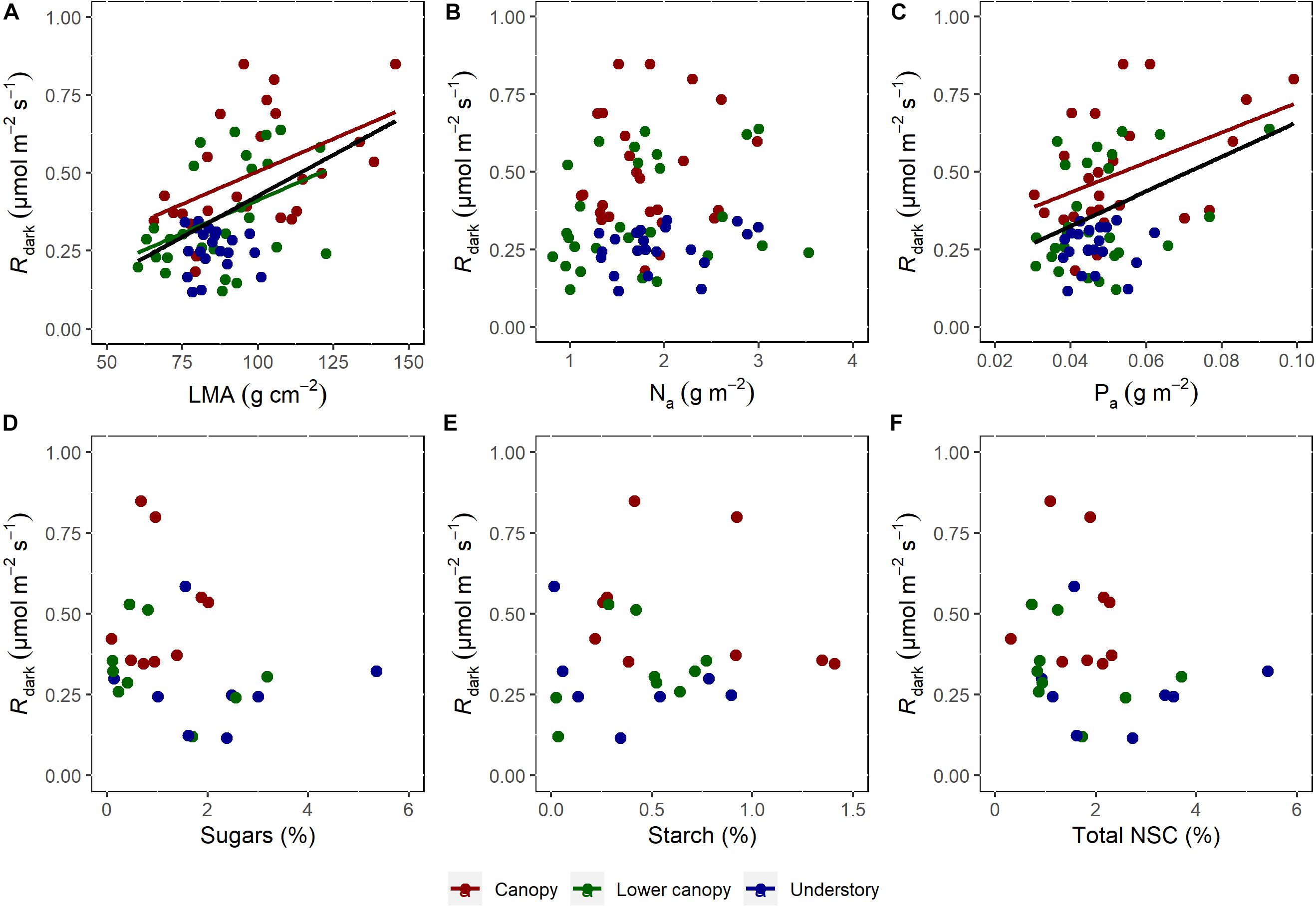
Figure 5. Respiration rates in the dark as a function of LMA (A), nitrogen (B), phosphorus (C), (n = 76). Sugars (D), Starch (E), and Total NSC (F), (n = 26). The data was derived from the measurements collected in understory (blue), lower canopy (green), and canopy (red) trees. Results from the standardized major axis (SMA) regression are presented in Supplementary Table 2. Note that when no significant relationship between the variables was found, the regression line was suppressed.
Discussion
Although a relatively small sampling size was analyzed by this study due to logistical constrains, we found interesting signs using the Kok method, which provided reliable estimates of apparent day respiration, with similar results to the 13CO2 labeling method (Figure 1). Therefore, the Kok method is a good way to estimate day respiration when the isotopic method is not possible to be used. Also, it is important to highlight that both methods do not consider the CO2 refixation by the photosynthesis, and, in this study, we measured the efflux of respiratory CO2. In the central Amazon field work, our results highlight the influence of canopy position on the rates of Rday and Rdark (Figures 3D,E). In addition, light suppression was also influenced by canopy position (Figure 3F), with higher values in the understory and lower values in the canopy. Rdark has a positive relationship with Rday (Figure 4A) and LMA (Figure 5A), which indicates that the higher respiration is associated with higher LMA. Our finding that trees in the canopy have higher LMA than trees in the understory (Supplementary Figure 3A), corroborates findings that LMA from the forest floor to the canopy top increases in every forest where it has been measured, including the tropics (Cavaleri et al., 2010). This pattern may be associated with plant strategies for using light. In this study, no relationship was found between Rdark and N (Figure 5B) and carbohydrates (Figures 5D–F). However, relationships between Rday and P concentration were significant (Figure 5C).
The relationship between higher respiration rates in the upper canopy leaves and the availability of light may be related to a higher demand for energy for possible repairs due to photodamage (Weerasinghe et al., 2014; Dos Santos et al., 2018). Moreover, the finding that upper canopy leaves had higher rates of respiration than understory leaves is consistent with the emerging view that leaf respiration is strongly dependent upon photosynthesis. For example, a literature review suggested that the ratio of respiration to photosynthesis is relatively constant across a wide diversity of plant species (Gifford, 2003). Given that photosynthesis rates of sun exposed upper canopy leaves in mature tropical forests are higher than understory leaves (Weerasinghe et al., 2014; Dos Santos et al., 2018), the pattern of higher canopy leaf respiration relative to understory leaf respiration is expected. These results have important implications for improving the representation of vertical differences in plant communities and associated leaf carbon cycling in dynamic vegetation models such as the Functionally Assembled Terrestrial Ecosystem Simulator (FATES) model (Koven et al., 2020).
Crown Position and the Effect on Leaf Respiration and Light Suppression of RDark
Leaf respiration is affected by the availability of light (Meir et al., 2001; Kosugi et al., 2012; Asao et al., 2015; Araki et al., 2017). The microenvironment along the vertical gradient of the forest can be dramatically different, especially with respect to temperature and light (Mulkey et al., 1996; Marenco et al., 2014; Rey-Sánchez et al., 2016). The need for light varies between species life strategies, directing the investment of their carbon resources, whether for growth, defense, or maintenance of existing tissues (Poorter and Bongers, 2006). Thus, the importance of the vertical gradient in light in influencing the physiological characteristics of leaves has been well documented (Kosugi et al., 2012; Weerasinghe et al., 2014; Heskel and Tang, 2018). The lower leaf respiration rates in the understory indicate that these trees do not use as much energy for growth and maintenance of leaves relative to canopy trees. Although net photosynthesis rates are likely also lower in the understory, this pattern would promote a positive carbon balance under light-limited conditions, a typical characteristic of understory species that invest in survival, waiting for the moment of increased light availability to invest in growth (Poorter and Bongers, 2006; Lambers et al., 2008). In this study, light suppression of Rdark was higher in understory trees relative to canopy trees (Figure 3F). In contrast to our finding, some studies have not observed differences in light suppression when assessing the effect of upper and lower position of the same canopy (Weerasinghe et al., 2014; Heskel and Tang, 2018). This highlights the need for additional field studies characterizing the vertical gradients of the light suppression of leaf respiration throughout diverse tropical forests.
The degree of light suppression of respiration as a function of canopy position had a different behavior when compared to respiratory rates in the light and in the dark. The degree of light suppression was higher for species in the understory and lower for species in the canopy (Figures 3F, 4B). Respiratory activity throughout the day is regulated, in part, by the demand for energy and the production of respiratory substrates during photosynthesis (Lambers et al., 2008; Tcherkez and Ghashghaie, 2017; Collalti et al., 2019). In this study, we found that the degree of light suppression of leaf respiration is lower in species that have higher dark respiration and an overall greater metabolic activity. Higher irradiance is associated with a greater demand for respiratory products, such as ATP, NADH, and carbon skeletons associated with growth and maintenance, reflecting higher rates of respiration (Weerasinghe et al., 2014). In addition, higher leaf respiratory rates in upper canopy leaves may also be related to the much higher diurnal variations in leaf temperature when compared to understory leaves (Way et al., 2018). For example, daytime leaf temperatures in the upper canopy in the central Amazon dry season, regularly exceed 40°C (Jardine et al., 2017). To elucidate which factors influence the variation in light suppression, it is necessary to understand the effect of temperature and irradiance on leaf respiration (Atkin et al., 2000; Lambers et al., 2008; Way et al., 2015, 2018). These environmental sensitivities of the light suppression of leaf respiration by light is poorly characterized in tropical forests. Moreover, the rate of leaf respiratory inhibition by light can vary under conditions of water availability (Crous et al., 2012; Turnbull et al., 2017) and depending on the age of the soils, being higher in old and P-deficient soils (Atkin et al., 2013). In temperate forests, the inhibition of respiration shows a marked seasonal variation (Heskel et al., 2014; Heskel and Tang, 2018). Thus, the rate of leaf respiratory inhibition by light in tropical forests should be not only be evaluated as a function of canopy position and the influence of the light/temperature microclimate, but also soil moisture and fertility. However, it is important to note that the degree of light suppression of leaf respiration may depend on the method of estimating daytime respiration (Way et al., 2018; Keenan et al., 2019).
Studies that estimated autotrophic respiration of the ecosystem in the Amazon rainforest (Chambers et al., 2004; Malhi et al., 2014), considered the inhibition of light respiration through correction factors obtained in studies not developed in the Amazon. Accurate knowledge of the true values of light suppression of leaf respiration is important for inferring the carbon use efficiency (CUE) of tropical forests which has been reported as low as 30% (Chambers et al., 2004). Although the Amazon rainforest is of great importance for the global carbon cycle, the quantification of light suppression of respiration in this biome is still limited. Inhibition of leaf respiration strongly influences the respiration estimates of the ecosystem and when not considered in the models, they cause overestimations in autotrophic respiration, and consequently, underestimate NPP (Kroner and Way, 2016; Wehr et al., 2016; Keenan et al., 2019).
Relationships Between Respiratory Rates and Leaf Traits
Canopy trees, which have a relatively exposed canopy, have higher values of respiration, LMA, and nitrogen rates (Asao et al., 2015; Araki et al., 2017; Rowland et al., 2021). Those individuals in the canopy invest in a smaller leaf area and higher mass (higher LMA) to protect them from damage by excessive irradiance, while those in the understory invest in a lower LMA to enhance light interception (Wright et al., 2004; Poorter and Bongers, 2006; Lambers et al., 2008). Nitrogen and phosphorus assimilation in plants are very energy demanding processes that require a substantial investment of adenosine triphosphate (ATP), the high energy containing molecule produced by respiration (Tcherkez and Ghashghaie, 2017; Tcherkez et al., 2017a). Leaf nitrogen and phosphorus are key elements of central metabolites and biopolymers like nucleic acids, nucleotides, proteins, and phospholipids which are crucial components of both photosynthetic and respiratory metabolism (Tcherkez and Ghashghaie, 2017). Phosphorus and nitrogen limitations can constrain numerous processes related to respiratory metabolism including glycolysis and electron transport rates (Atkin et al., 2015; Rowland et al., 2017; Tcherkez and Ghashghaie, 2017). Thus, knowledge of their leaf concentrations are important for improvements in modeling carbon fluxes and dynamics in forests (Zhu et al., 2019), and forest responses to climate warming (Tang et al., 2018).
Upper canopy foliage outside of the tropics has generally been shown to have a higher total leaf protein content (more photosynthetic and respiratory machinery) than lower canopy foliage and therefore a higher respiratory cost associated with maintaining that machinery (Weerasinghe et al., 2014). However, in this study in the central Amazon, a clear vertical gradient in leaf nitrogen concentration was not observed, while that of Rdark, Rday, and the % suppression by light showed strong vertical patterns. Our observations are similar to previous work in the western Amazon (Meir et al., 2001) that observed that respiration in the dark correlates with leaf concentrations of phosphorus (Figure 5C), but not with nitrogen (Figure 5B). This is a similar result to that obtained when comparing the behavior of leaf dark respiration in the Jaru forest in Rondônia, Brazil (Meir et al., 2001). At the Jaru site, the best predictor of Rdark was LMA and leaf phosphorus concentrations, while leaf nitrogen concentrations showed little influence. Although some studies report a strong relationship between respiratory rates and nitrogen concentration, the characteristics of the study site, such as soil fertility, must be considered (Crous et al., 2017; Rowland et al., 2017). These observations support a previous study that concluded that productivity in Amazonian ecosystems are limed by soil phosphorus (Quesada et al., 2010). When a global data synthesis was conducted, the positive relationship between Rdark and nitrogen and phosphorus concentrations were found to be higher in regions with low soil phosphorus concentrations (Crous et al., 2017; Rowland et al., 2017). These results are relevant to the modeling of the carbon cycle in tropical forests, as they support the hypothesis that nutrient limitations effect photosynthetic and respiratory rates at different scales (Lambers et al., 2008; Peng et al., 2021).
Non-structural carbohydrates (NSCs) are central components of plant metabolism involved in numerous physiological process including growth, reproduction, protection, and defense as they are subunits of structural carbohydrates (e.g., cell walls which make up the majority of plant biomass) and are substrates for respiration (Hartmann and Trumbore, 2016; Landhäusser et al., 2018; Dickman et al., 2019). In this study, the variation of canopy position did not statistically effect sugar, starch or total NSC concentrations (Supplementary Figures 3D–F). However, the average leaf concentration of sugars was slightly higher in the understory relative to the canopy. This is in apparent contrast to previous observations that have shown that leaves more exposed to sun usually have higher levels of soluble sugars when compared to shade leaves (Weerasinghe et al., 2014; Dayer et al., 2021). One explanation for the difference may be because our samples were collected during the early mornings where leaf NSCs may be depleted due to their use as respiratory substrates at night; leaf NSC concentrations tend to peak at midday when biosynthesis from photosynthesis is high (Dickman et al., 2019; Gersony et al., 2020). The lack of clear variation of leaf starch concentrations with canopy position may also be related to the fact that starch is a reserve carbohydrate with a slower turnover rate relative to sugars (Dickman et al., 2019). These findings are consistent with the emerging view that leaf respiration rates under unstressed conditions are uncoupled from carbohydrate supply in leaves (McCutchan and Monson, 2001). For example, Asao and Ryan (2015) reported that changes in carbohydrate levels did not directly affect the responses of photosynthesis and respiration in trees under branch girdling.
Conclusion
In the present study, we found that emission of respiratory CO2 is up to two times higher at the forest canopy than those in the understory. Light suppression of leaf respiration varies according to the degree of canopy position. So, trees from the canopy had higher rates of light and dark respiration than trees from the understory. On the other hand, light suppression is higher in the understory and lower on canopy trees. In addition, leaf respiration shows a positive relationship with LMA and P concentration. These changes in physiology as a function of light availability have a great importance in carbon assimilation and can influence drought responses (Rowland et al., 2021). When the suppression is not considered in the models, it may cause an underestimation of NPP. Furthermore, knowledge about the impact of canopy position on inhibition of respiration will allow the refinement of the parameterization of climate models, as canopy trees have lower suppression and, consequently, have higher exchange of CO2 with the atmosphere than the understory. In the context of climate change, a higher occurrence of extreme events is expected, which will cause an increase in the frequency of natural disturbances in the forest. The sudden increase in light availability and higher temperatures for the surviving understory species as well as new recruits could be exploited to understand how carbon and energy metabolism shifts under the new environmental conditions.
Data Availability Statement
All data presented in the manuscript can be downloaded free of charge from the NGEE-Tropics data archive at http://dx.doi.org/10.15486/ngt/1819364.
Author Contributions
DS, KJ, and JR performed the experiments and analyzed the data. DS and KJ wrote the manuscript. NH and JC provided financial and logistical support. JR, AR, NM, BG, AW, IS-F, NH, and JC improved the manuscript. All authors contributed to the article and approved the submitted version.
Funding
This material is based upon work supported as part of the Next Generation Ecosystem Experiments-Tropics (NGEE-Tropics) funded by the U.S. Department of Energy, Office of Science, Office of Biological and Environmental Research’s Terrestrial Ecosystem Science Program through contracts No. DE-AC02-05CH11231 to LBNL, No. DESC0012704 to BNL, and No. DE-AC05-1008 00OR22725 to ORNL (managed by UT-Battelle, LLC, for the DOE). Additional funding for this research was provided by the Brazilian Conselho Nacional de Desenvolvimento Científico e Tecnológico (CNPq).
Conflict of Interest
The authors declare that the research was conducted in the absence of any commercial or financial relationships that could be construed as a potential conflict of interest.
Publisher’s Note
All claims expressed in this article are solely those of the authors and do not necessarily represent those of their affiliated organizations, or those of the publisher, the editors and the reviewers. Any product that may be evaluated in this article, or claim that may be made by its manufacturer, is not guaranteed or endorsed by the publisher.
Acknowledgments
Logistical and scientific support is acknowledged by the Forest Management laboratory (LMF), Tropical Forest Science (CFT) and Large Scale Biosphere-Atmosphere (LBA) programs at the National Institute for Amazon Research (INPA). The assistance of Sidnei Pereira in the field is acknowledged. We would also like to acknowledge Pacific Northwest National Laboratory for NSC analysis.
Supplementary Material
The Supplementary Material for this article can be found online at: https://www.frontiersin.org/articles/10.3389/ffgc.2021.723539/full#supplementary-material
References
Araki, M. G., Gyokusen, K., and Kajimoto, T. (2017). Vertical and seasonal variations in temperature responses of leaf respiration in a Chamaecyparis obtusa canopy. Tree Physiol. 37, 1269–1284. doi: 10.1093/treephys/tpx012
Araújo, R. F., Chambers, J. Q., Celes, C. H. C., Muller-landau, H. C., Santos, A. P. F., Emmert, F., et al. (2020). Integrating high resolution drone imagery and forest inventory to distinguish canopy and understory trees and quantify their contributions to forest structure and dynamics. PLoS One 15:e0243079. doi: 10.1371/journal.pone.0243079
Asao, S., Bedoya-Arrieta, R., and Ryan, M. G. (2015). Variation in foliar respiration and wood CO2 efflux rates among species and canopy layers in a wet tropical forest. Tree Physiol. 35, 148–159. doi: 10.1093/treephys/tpu107
Asao, S., and Ryan, M. G. (2015). Carbohydrate regulation of photosynthesis and respiration from branch girdling in four species of wet tropical rain forest trees. Tree Physiol. 35, 608–620. doi: 10.1093/treephys/tpv025
Atkin, O. K., Bloomfield, K. J., Reich, P. B., Tjoelker, M. G., Asner, G. P., Bonal, D., et al. (2015). Global variability in leaf respiration among plant functional types in relation to climate and leaf traits. New Phytol. 206, 614–636. doi: 10.1111/nph.13253
Atkin, O. K., Evans, J. R., Ball, M. C., Lambers, H., and Pons, T. L. (2000). Leaf respiration of snow gum in the light and dark. Interactions between temperature and irradiance. Plant Physiol. 122, 915–923. doi: 10.1104/pp.122.3.915
Atkin, O. K., Turnbull, M. H., Zaragoza-Castells, J., Fyllas, N. M., Lloyd, J., Meir, P., et al. (2013). Light inhibition of leaf respiration as soil fertility declines along a post-glacial chronosequence in New Zealand: an analysis using the Kok method. Plant Soil 367, 163–182. doi: 10.1007/s11104-013-1686-0
Buckley, T. N., Vice, H., and Adams, M. A. (2017). The Kok effect in Vicia faba cannot be explained solely by changes in chloroplastic CO 2 concentration. New Phytol. 216, 1064–1071. doi: 10.1111/nph.14775
Cardoso, D., Särkinen, T., Alexander, S., Amorim, A. M., Bittrich, V., Celis, M., et al. (2017). Amazon plant diversity revealed by a taxonomically verified species list. Proc. Natl. Acad. Sci. U.S.A. 114, 10695–10700. doi: 10.1073/pnas.1706756114
Carneiro, V. M. C. (2004). Composição Florística e Análise Estrutural da Floresta Primária de Terra Firme na Bacia do Rio Cuieiras, Manaus-AM. Ph.D. thesis. Manaus: National Institute of Amazonian Research, 77.
Cavaleri, M. A., Coble, A. P., Ryan, M. G., Bauerle, W. L., Loescher, H. W., and Oberbauer, S. F. (2017). Tropical rainforest carbon sink declines during El Niño as a result of reduced photosynthesis and increased respiration rates. New Phytol. 216, 136–149. doi: 10.1111/nph.14724
Cavaleri, M. A., Oberbauer, S. F., Clark, D. B., Clark, D. A., and Ryan, M. G. (2010). Height is more important than light in determining leaf morphology in a tropical forest. Ecology 91, 1730–1739. doi: 10.1890/09-1326.1
Chambers, J. Q., Tribuzy, E. S., Toledo, L. C., Crispim, B. F., Higuchi, N., Dos Santos, J., et al. (2004). Respiration from a tropical forest ecosystem: partitioning of sources and low carbon use efficiency. Ecol. Appl. 14, 72–88. doi: 10.1890/01-6012
Clark, D. A., and Clark, D. B. (1992). Life history diversity of canopy and emergent trees in a neotropical rain forest. Ecol. Monogr. 62, 315–344.
Collalti, A., Tjoelker, M. G., Hoch, G., Mäkelä, A., Guidolotti, G., Heskel, M., et al. (2019). Plant respiration: controlled by photosynthesis or biomass? Glob. Chang. Biol. 26, 1739–1753. doi: 10.1111/gcb.14857
Crous, K. Y., O’Sullivan, O. S., Zaragoza-Castells, J., Bloomfield, K. J., Negrini, A. C. A., Meir, P., et al. (2017). Nitrogen and phosphorus availabilities interact to modulate leaf trait scaling relationships across six plant functional types in a controlled-environment study. New Phytol. 215, 992–1008. doi: 10.1111/nph.14591
Crous, K. Y., Zaragoza-Castells, J., Ellsworth, D. S., Duursma, R. A., Löw, M., Tissue, D. T., et al. (2012). Light inhibition of leaf respiration in field-grown Eucalyptus saligna in whole-tree chambers under elevated atmospheric CO2 and summer drought. Plant Cell Environ. 35, 966–981. doi: 10.1111/j.1365-3040.2011.02465.x
Dayer, S., Murcia, G., Prieto, J. A., Durán, M., Martínez, L., Píccoli, P., et al. (2021). Non-structural carbohydrates and sugar export in grapevine leaves exposed to different light regimes. Physiol. Plant. 171, 728–738. doi: 10.1111/ppl.13258
Dickman, L. T., McDowell, N. G., Grossiord, C., Collins, A. D., Wolfe, B. T., Detto, M., et al. (2019). Homoeostatic maintenance of nonstructural carbohydrates during the 2015–2016 El Niño drought across a tropical forest precipitation gradient. Plant Cell Environ. 42, 1705–1714. doi: 10.1111/pce.13501
Dos Santos, V. A. H. F., Ferreira, M. J., Rodrigues, J. V. F. C., Garcia, M. N., Ceron, J. V. B., Nelson, B. W., et al. (2018). Causes of reduced leaf-level photosynthesis during strong El Niño drought in a Central Amazon forest. Glob. Chang. Biol. 24, 4266–4279. doi: 10.1111/gcb.14293
Ely, K. S., Rogers, A., Agarwal, D. A., Ainsworth, E. A., Albert, L. P., Ali, A., et al. (2021). A reporting format for leaf-level gas exchange data and metadata. Ecol. Inform. 61:101232. doi: 10.1016/j.ecoinf.2021.101232
Farquhar, G. D., and Busch, F. A. (2017). Changes in the chloroplastic CO2 concentration explain much of the observed Kok effect: a model. New Phytol. 214, 570–584. doi: 10.1111/nph.14512
Gauthier, P. P. G., Saenz, N., Griffin, K. L., Way, D., and Tcherkez, G. (2020). Is the Kok effect a respiratory phenomenon? Metabolic insight using 13C labeling in Helianthus annuus leaves. New Phytol. 228, 1243–1255. doi: 10.1111/nph.16756
Gersony, J. T., Hochberg, U., Rockwell, F. E., Park, M., Gauthier, P. P. G., and Holbrook, N. M. (2020). Leaf carbon export and nonstructural carbohydrates in relation to diurnal water dynamics in mature oak trees1[OPEN]. Plant Physiol. 183, 1612–1621. doi: 10.1104/pp.20.00426
Gifford, R. M. (2003). Plant respiration in productivity models: conceptualisation, representation and issues for global terrestrial carbon-cycle research. Funct. Plant Biol. 30, 171–186. doi: 10.1071/FP02083
Gong, X. Y., Tcherkez, G., Wenig, J., Schäufele, R., and Schnyder, H. (2018). Determination of leaf respiration in the light: comparison between an isotopic disequilibrium method and the Laisk method. New Phytol. 218, 1371–1382. doi: 10.1111/nph.15126
Griffin, K. L., Turnbull, M., and Murthy, R. (2002). Canopy position affects the temperature response of leaf respiration in Populus deltoides. New Phytol. 154, 609–619. doi: 10.1046/j.1469-8137.2002.00410.x
Hartmann, H., and Trumbore, S. (2016). Understanding the roles of nonstructural carbohydrates in forest trees–from what we can measure to what we want to know. New Phytol. 211, 386–403. doi: 10.1111/nph.13955
Heskel, M. A. (2018). Small flux, global impact: Integrating the nuances of leaf mitochondrial respiration in estimates of ecosystem carbon exchange. Am. J. Bot. 105, 815–818. doi: 10.1002/ajb2.1079
Heskel, M. A., Bitterman, D., Atkin, O. K., Turnbull, M. H., and Griffin, K. L. (2014). Seasonality of foliar respiration in two dominant plant species from the Arctic tundra: response to long-term warming and short-term temperature variability. Funct. Plant Biol. 41, 287–300. doi: 10.1071/FP13137
Heskel, M. A., and Tang, J. (2018). Environmental controls on light inhibition of respiration and leaf and canopy daytime carbon exchange in a temperate deciduous forest. Tree Physiol. 38, 1886–1902. doi: 10.1093/treephys/tpy103
Huntingford, C., Atkin, O. K., Martinez-De La Torre, A., Mercado, L. M., Heskel, M. A., Harper, A. B., et al. (2017). Implications of improved representations of plant respiration in a changing climate. Nat. Commun. 8:1602. doi: 10.1038/s41467-017-01774-z
Jardine, K. J., Jardine, A. B., Holm, J. A., Lombardozzi, D. L., Negron-Juarez, R. I., Martin, S. T., et al. (2017). Monoterpene ‘thermometer’ of tropical forest-atmosphere response to climate warming. Plant Cell Environ. 40, 441–452. doi: 10.1111/pce.12879
Keenan, T. F., Migliavacca, M., Papale, D., Baldocchi, D., Reichstein, M., Torn, M., et al. (2019). Widespread inhibition of daytime ecosystem respiration. Nat. Ecol. Evol. 3, 407–415. doi: 10.1038/s41559-019-0809-2
Kok, B. (1948). A critical consideration of the quantum yield of Chlorella photosynthesis. Enzymologia 13, 407–415.
Kok, B. (1949). On the interrelation of respiration and photosynthesis in green plants. Biochim. Biophys. Acta 3, 625–631.
Kosugi, Y., Takanashi, S., Yokoyama, N., Philip, E., and Kamakura, M. (2012). Vertical variation in leaf gas exchange parameters for a Southeast Asian tropical rainforest in Peninsular Malaysia. J. Plant Res. 125, 735–748. doi: 10.1007/s10265-012-0495-5
Koven, C. D., Knox, R. G., Fisher, R. A., Fisher, R. A., Chambers, J. Q., Chambers, J. Q., et al. (2020). Benchmarking and parameter sensitivity of physiological and vegetation dynamics using the functionally assembled terrestrial ecosystem simulator (FATES) at Barro Colorado Island, Panama. Biogeosciences 17, 3017–3044. doi: 10.5194/bg-17-3017-2020
Kroner, Y., and Way, D. A. (2016). Carbon fluxes acclimate more strongly to elevated growth temperatures than to elevated CO2 concentrations in a northern conifer. Glob. Chang. Biol. 22, 2913–2928. doi: 10.1111/gcb.13215
Lambers, H., Chapin, S., and Pons, T. (2008). Plant Physiological Ecology. New York, NY: Springer. doi: 10.1007/978-0-387-78341-3
Landhäusser, S. M., Chow, P. S., Dickman, L. T., Furze, M. E., Kuhlman, I., Schmid, S., et al. (2018). Methods paper Standardized protocols and procedures can precisely and accurately quantify non-structural carbohydrates. Tree Physiol. 38, 1764–1778. doi: 10.1093/treephys/tpy118
Loreto, F., Delfine, S., and Di Marco, G. (1999). Estimation of photorespiratory carbon dioxide recycling during photosynthesis. Aust. J. Plant Physiol. 26, 733–736.
Loreto, F., Velikova, V., and Di Marco, G. (2001). Respiration in the light measured by 12CO2 emission in 13CO2 atmosphere in maize leaves. Aust. J. Plant Physiol. 28, 1103–1108. doi: 10.1071/pp01091
Malhi, Y. (2012). The productivity, metabolism and carbon cycle of tropical forest vegetation. J. Ecol. 100, 65–75. doi: 10.1111/j.1365-2745.2011.01916.x
Malhi, Y., Farfán Amézquita, F., Doughty, C. E., Silva-Espejo, J. E., Girardin, C. A. J., Metcalfe, D. B., et al. (2014). The productivity, metabolism and carbon cycle of two lowland tropical forest plots in south-western Amazonia, Peru. Plant Ecol. Divers. 7, 85–105. doi: 10.1080/17550874.2013.820805
Marenco, R. A., Antezana-vera, S. A., Romenya, P., Angelo, M., Camargo, B., De Oliveira, M. F., et al. (2014). Fisiologia de espécies florestais da Amazônia?: fotossíntese, respiração e relações hídricas. Acta Amaz. 61, 786–789.
McCutchan, C. L., and Monson, R. K. (2001). Night-time respiration rate and leaf carbohydrate concentrations are not coupled in two alpine perennial species. New Phytol. 149, 419–430. doi: 10.1046/j.1469-8137.2001.00039.x
Meir, P., Grace, J., and Miranda, A. C. (2001). Leaf respiration in two tropical rainforests: constraints on physiology by phosphorus, nitrogen and temperature. Funct. Ecol. 15, 378–387. doi: 10.1046/j.1365-2435.2001.00534.x
Metcalfe, D. B., Meir, P., Aragão, L. E. O. C., Lobo-do-Vale, R., Galbraith, D., Fisher, R. A., et al. (2010). Shifts in plant respiration and carbon use efficiency at a large-scale drought experiment in the eastern Amazon. New Phytol. 187, 608–621. doi: 10.1111/j.1469-8137.2010.03319.x
Mulkey, S. S., Kitajima, K., and Wright, S. J. (1996). Plant physiological ecology of tropical forest canopies. Trends Ecol. Evol. 11, 436–437. doi: 10.1016/0169-5347(96)10043-4
Peng, Y., Bloomfield, K. J., Cernusak, L. A., Domingues, T. F., and Colin Prentice, I. (2021). Global climate and nutrient controls of photosynthetic capacity. Commun. Biol. 4, 1–9. doi: 10.1038/s42003-021-01985-7
Poorter, L., and Bongers, F. (2006). Leaf traits are good predictors of plant performance across 53 rain forest species. Ecology 87, 1733–1743.
Quesada, C. A., Lloyd, J., Schwarz, M., Patiño, S., Baker, T. R., Czimczik, C., et al. (2010). Variations in chemical and physical properties of Amazon forest soils in relation to their genesis. Biogeosciences 7, 1515–1541. doi: 10.5194/bg-7-1515-2010
R Core Team (2019). R: A Language and Environment for Statistical Computing. Vienna: R Foundation for Statistical Computing. Available online at: https://www.R-project.org/
Rey-Sánchez, A. C., Slot, M., Posada, J. M., and Kitajima, K. (2016). Spatial and seasonal variation in leaf temperature within the canopy of a tropical forest. Clim. Res. 71, 75–89. doi: 10.3354/cr01427
Rowland, L., Antonio, C. L., Oliveira, R. S., Bittencourt, P. R. L., Giles, A. L., Coughlin, I., et al. (2021). The response of carbon assimilation and storage to long-term drought in tropical trees is dependent on light availability. Funct. Ecol. 35, 43–53. doi: 10.1111/1365-2435.13689
Rowland, L., Zaragoza-Castells, J., Bloomfield, K. J., Turnbull, M. H., Bonal, D., Burban, B., et al. (2017). Scaling leaf respiration with nitrogen and phosphorus in tropical forests across two continents. New Phytol. 214, 1064–1077. doi: 10.1111/nph.13992
Sauer, A., and Klaus-Peter, H. (1983). On the light dependency of fatty acid synthesis by isolated spinach chloroplasts. Plant Physiol. 73, 11–15. doi: 10.1016/0304-4211(80)90112-1
Tang, X., Fan, S., Zhang, W., Gao, S., Chen, G., and Shi, L. (2019). Global variability in belowground autotrophic respiration in terrestrial ecosystems. Earth Syst. Sci. Data 11, 1839–1852. doi: 10.5194/essd-11-1839-2019
Tang, Z., Xu, W., Zhou, G., Bai, Y., Li, J., Tang, X., et al. (2018). Patterns of plant carbon, nitrogen, and phosphorus concentration in relation to productivity in China’s terrestrial ecosystems. Proc. Natl. Acad. Sci. U.S.A. 115, E6095–E6096. doi: 10.1073/pnas.1808126115
Tcherkez, G., Gauthier, P., Buckley, T. N., Busch, F. A., Barbour, M. M., Bruhn, D., et al. (2017a). Leaf day respiration: low CO2flux but high significance for metabolism and carbon balance. New Phytol. 216, 986–1001. doi: 10.1111/nph.14816
Tcherkez, G., Gauthier, P., Buckley, T. N., Busch, F. A., Barbour, M. M., Bruhn, D., et al. (2017b). Tracking the origins of the Kok effect, 70 years after its discovery. New Phytol. 214, 506–510. doi: 10.1111/nph.14527
Tcherkez, G., and Ghashghaie, J. (2017). Plant Respiration?: Metabolic Fluxes and Carbon Balance. Cham: Springer. doi: 10.1007/978-3-319-68703-2
Ter Steege, H., Pitman, N. C. A., Sabatier, D., Baraloto, C., Salomão, R. P., Guevara, J. E., et al. (2013). Hyperdominance in the Amazonian tree flora. Science 342:1243092. doi: 10.1126/science.1243092
ter Steege, H., Prado, P. I., de Lima, R. A. F., Pos, E., de Souza Coelho, L., de Andrade Lima Filho, D., et al. (2020). Biased-corrected richness estimates for the Amazonian tree flora. Sci. Rep. 10, 1–13. doi: 10.1038/s41598-020-66686-3
Turnbull, M. H., Ogaya, R., Barbeta, A., Peñuelas, J., Zaragoza-Castells, J., Atkin, O. K., et al. (2017). Light inhibition of foliar respiration in response to soil water availability and seasonal changes in temperature in Mediterranean holm oak (Quercus ilex) forest. Funct. Plant Biol. 44, 1178–1193. doi: 10.1071/FP17032
Warton, D. I., Duursma, R. A., Falster, D. S., and Taskinen, S. (2012). smatr 3- an R package for estimation and inference about allometric lines. Methods Ecol. Evol. 3, 257–259. doi: 10.1111/j.2041-210X.2011.00153.x
Way, D. A., Aspinwall, M. J., Drake, J. E., Crous, K. Y., Campany, C. E., Ghannoum, O., et al. (2018). Responses of respiration in the light to warming in field-grown trees: a comparison of the thermal sensitivity of the Kok and Laisk methods. New Phytol. 222, 132–143. doi: 10.1111/nph.15566
Way, D. A., Holly, C., Bruhn, D., Ball, M. C., and Atkin, O. K. (2015). Diurnal and seasonal variation in light and dark respiration in field-grown Eucalyptus pauciflora. Tree Physiol. 35, 840–849. doi: 10.1093/treephys/tpv065
Weerasinghe, L. K., Creek, D., Crous, K. Y., Xiang, S., Liddell, M. J., Turnbull, M. H., et al. (2014). Canopy position affects the relationships between leaf respiration and associated traits in a tropical rainforest in Far North Queensland. Tree Physiol. 34, 564–584. doi: 10.1093/treephys/tpu016
Wehr, R., Munger, J. W., McManus, J. B., Nelson, D. D., Zahniser, M. S., Davidson, E. A., et al. (2016). Seasonality of temperate forest photosynthesis and daytime respiration. Nature 534, 680–683. doi: 10.1038/nature17966
Wright, I. J., Reich, P. B., Westoby, M., Ackerly, D. D., Baruch, Z., Bongers, F., et al. (2004). The worldwide leaf economics spectrum. Nature 428, 821–827. doi: 10.1038/nature02403
Wu, J., Serbin, S. P., Ely, K. S., Wolfe, B. T., Dickman, L. T., Grossiord, C., et al. (2020). The response of stomatal conductance to seasonal drought in tropical forests. Glob. Chang. Biol. 26, 823–839. doi: 10.1111/gcb.14820
Yin, X., Niu, Y., van der Putten, P. E. L., and Struik, P. C. (2020). The Kok effect revisited. New Phytol. 227, 1764–1775. doi: 10.1111/nph.16638
Zhang, P., Mcdowell, N. G., Zhou, X., Wang, W., Leff, R. T., Pivovaroff, A. L., et al. (2021). Declining carbohydrate content of Sitka-spruce treesdying from seawater exposure research article. Plant Physiol 185, 1682–1696. doi: 10.1093/plphys/kiab002
Keywords: carbon cycle, Kok method, leaf traits, Rday, Rdark, tropical forest
Citation: Souza DC, Jardine KJ, Rodrigues JVFC, Gimenez BO, Rogers A, McDowell N, Walker AP, Higuchi N, Sampaio-Filho IJ and Chambers J (2021) Canopy Position Influences the Degree of Light Suppression of Leaf Respiration in Abundant Tree Genera in the Amazon Forest. Front. For. Glob. Change 4:723539. doi: 10.3389/ffgc.2021.723539
Received: 10 June 2021; Accepted: 02 September 2021;
Published: 30 September 2021.
Edited by:
Tomas Ferreira Domingues, University of São Paulo, BrazilReviewed by:
Hao Ran Lai, University of Canterbury, New ZealandGiuliano Maselli Locosselli, University of São Paulo, Brazil
Copyright © 2021 Souza, Jardine, Rodrigues, Gimenez, Rogers, McDowell, Walker, Higuchi, Sampaio-Filho and Chambers. This is an open-access article distributed under the terms of the Creative Commons Attribution License (CC BY). The use, distribution or reproduction in other forums is permitted, provided the original author(s) and the copyright owner(s) are credited and that the original publication in this journal is cited, in accordance with accepted academic practice. No use, distribution or reproduction is permitted which does not comply with these terms.
*Correspondence: Kolby J. Jardine, a2pqYXJkaW5lQGxibC5nb3Y=