- Department of Ecology and Graduate Program in Ecology, Federal University of Rio de Janeiro, Rio de Janeiro, Brazil
Habitat loss in highly deforested landscapes such as the Brazilian Atlantic Forest has been severely affecting the diversity and survival of palm species. As some species are more sensitive than others, trait responses to the environment, as well as environmental effects on fecundity, growth, and mortality rates, may affect species demography. Considering this context, we studied functional and demographic responses of three palm species (Astrocaryum aculeatissimum, Euterpe edulis, and Geonoma schottiana) to habitat loss in the Atlantic Forest in southeastern Brazil by measuring morpho-physiological traits related to plant growth and light acquisition for photosynthesis. We also tested the response of population fitness to fragment size. Plant survival and growth was subsequently monitored in 2006 and 2007, and population dynamics were summarized in pool matrices for large and small forest fragments in the monitoring periods comprehending one full year between 2005–2006 and 2006–2007. The asymptotic growth rate of populations (defined here as population fitness, λ) in five forest fragments was then calculated. Diameter of individuals of the demography plots (from year 2005 to 2007) was used to calculate the relative diameter growth rate. Later, in 2015, we measured a set of morpho-physiological functional traits in palms in the same plots used in the demographic studies. While A. aculeatissimum populations were stable in both monitoring periods in small and large fragments, E. edulis populations were predicted to decline due to intense predation by monkeys in the large fragment, but were stable in the smaller fragments, and G. schottiana populations were stable in the large fragments in both monitoring periods, but populations in the smaller fragments were predicted to decline in the second period, i.e., with lower fitness in these fragments. In addition, the functional traits analyzed showed that G. schottiana is a forest interior species associated with the shade/understory environment response. E. edulis was also affected by the size of the fragment, but due to a disruptive interaction with a predator and showed intermediate functional traits values. On the other hand, A. aculeatissimum thrived in areas with higher and lower incidence of light and was not demographically affected by forest remnant size. This suggests that E. edulis and A. aculeatissimum are habitat generalists. We concluded that differences in the ecophysiological performance of palms due to distinct morpho-physiological functional traits related to leaf economic spectrum, such as LDMC or specific leaf area (SLA) and to photosynthetic responses to light environment as electron transport rate (ETR) and saturation irradiance (Ik) were linked to the demographic variation observed in forest remnants of different size.
Introduction
Habitat fragmentation and habitat loss imply the ongoing partition of large areas into small and isolated patches or “islands” (Laurance et al., 2002; Liu et al., 2019), as well as changes in habitat configuration (Fahrig, 2003). These processes have profound effects on biodiversity (Fahrig, 2003; Haddad et al., 2015; Wilson et al., 2016). Fragmentation produces changes in population and community dynamics (Laurance et al., 2002; Haddad et al., 2015), however, the severity of impacts generated by fragmentation depends on factors such as fragment area, edge structure, matrix surrounding the fragment, distance, isolation, and habitat availability (Laurance et al., 2002; Fahrig, 2013; Wilson et al., 2016; Liu et al., 2019). Yet, the intensity of impact increases in smaller and more isolated fragments (Haddad et al., 2015; Liu et al., 2019). The response of species to fragmentation depends on life-history traits associated with dispersal, establishment, and persistence (Wilson et al., 2016; Zambrano et al., 2019) and are species-specific, depending on the ecological requirements of species (Ibáñez et al., 2014; Zambrano et al., 2019). Have been observed, however, that populations size of different species in forest remnants fluctuate and are less stable than those in forests that have not been fragmented (Laurance et al., 2002).
The ability of species to respond to changes in environmental conditions and resource availability are related to functional traits, which are defined as “measurable morphological, physiological or phenological features of species that impact their fitness via their effects on demographic features” (Violle et al., 2007). Functional traits also mediate the responses associated with fundamental processes such as species dispersal, establishment, and persistence (Ackerly and Cornwell, 2007; De Bello et al., 2013; Zambrano et al., 2019). The increase in species abundance with traits facilitating colonization and persistence, especially in isolated and/or smaller fragments, is a response that alters the growth and, eventually, the occurrence of some populations in the long term, changing the species composition and dynamics (Dupré and Ehrlén, 2002; May et al., 2013). Species with traits related to more conservative use of resources, such as low specific leaf area (SLA), high leaf dry matter content (LDMC), low leaf nitrogen content, and others are in the lower end of the leaf economic spectrum, showing often low growth rates and are more tolerant to environmental stresses. On the other hand, more acquisitive species are located at the opposite side of the leaf economic spectrum, showing higher growth rates and higher competitive ability in more productive environments (Wright et al., 2004; Messier et al., 2016). Leaf variation patterns are commonly associated with gradients of light, water, and nutrient availability. Plant size (height) is also a vital attribute to plant life cycles (Westoby et al., 2002). The relationship between functional traits related to leaf economics spectrum, and variation in plant life histories and plant demography was recently demonstrated, and may affect the ability of species to persist in fragmented landscapes (Adler et al., 2014; Rüder et al., 2018; Laughlin et al., 2020). In order to unravel the relationship between demography and functional traits, elasticity analysis may be used to investigate relative contributions to fitness components of survival, individual growth, and fecundity to population growth rates (Adler et al., 2014).
Previous studies in the Atlantic Forest in Brazil found that palms are sensitive to decreases in area and forest cover (Benchimol et al., 2016). Palm species dependent on the forest interior are more affected by habitat loss, while palm species that thrive in open areas become more abundant (Benchimol et al., 2016). Despite numerous studies on the effects of habitat loss and fragmentation, few have focused on the role of functional responses and population dynamics together in fragmentation scenarios. In this study, we aimed to verify whether a connection exists between functional traits and demography of different species in response to habitat size. We selected three palm species that occupy different forest strata: Astrocaryum aculeatissimum, Euterpe edulis, and Geonoma schottiana. These species were common in the fragments of different sizes selected for this study in the Atlantic Forest. We estimated population dynamics for each species in each of five fragments and measured seven key morphological functional traits. We also used rapid light curves (RLC) to measure the present state of light absorption in photosynthesis (Cavender-Bares and Bazzaz, 2008; Figueroa et al., 2013). We hypothesize that the understory species (G. schottiana) has traits related to shade habitats and respond negatively in terms of population demography to the loss of habitat. Additionally, species with traits more related to conservative use of resources will show higher elasticities to survival and will be more resistant to habitat loss.
Materials and Methods
Study Sites
This study was carried out in five Atlantic Forest fragments, two of which are federal protected areas (hereafter “large fragments”): Poço das Antas Biological Reserve (∼3,500 ha) and União Biological Reserve (∼7,700 ha). The other three sites (hereafter “small fragments”) are forest fragments located in private properties: Santa Helena (57 ha), Estreito (21 ha), and Afetiva-Jorge (19 ha). All sites are located in southeastern Brazil, in Rio de Janeiro state, in the municipalities of Rio das Ostras, Silva Jardim, and Casimiro de Abreu. These fragments were part of a large, continuous expanse of forest until a century ago (Carvalho, 2005), when fragmentation began following the implementation of coffee production and other agricultural crops.
The habitat in all five sites is classified as Lowland Atlantic Rainforest (“Floresta Ombrófila Densa Submontana” sensu IBGE, 2012). All sites are surrounded by pasture, agricultural fields, and secondary forests. The climate in the area is classified as Walter and Lieth Equatorial type (Walter, 1971), with mean annual rainfall of ca. 2,100 mm (Souza and Martins, 2004). Although there is not a distinct dry season, rainfall from May to August is often lower.
Species Selected for the Study
Astrocaryum aculeatissimum (Schott) Burret is a monoecious, slow-growing palm that has single-stemmed (hereafter “solitary”) or multi-stemmed habit. It is typically 4–8 m in height and 11–15 cm in diameter (Henderson et al., 1995; Lorenzi et al., 2004). It is endemic to the Atlantic Forest and occurs from the state of Bahia, in the northeast, to Santa Catarina, in the south (Henderson et al., 1995; Lorenzi et al., 2004). It is found primarily in the understory of lowland forests, occasionally on flooded sites and in the vegetation matrix surrounding forest fragments.
Euterpe edulis Mart. (i.e., “palmito Juçara”) is a monoecious, solitary, shade-tolerant palm. It is a slow-growing subcanopy palm that can reach 20 m in height and 10–15 cm in diameter. It occurs primarily in forests along the Atlantic coast of Brazil, but can be found inland as far as Argentina and Paraguay, in Seasonal Forests (Henderson et al., 1995). It occupies hill slopes and tops, and sites associated to seasonal flooding up to elevations of 1,000 m (Henderson et al., 1995; Silva-Matos and Watkinson, 1998). This species is harvested for heart-of-palm, one of the most abundant and valuable non-timber forest products in the Atlantic Forest (Fantini and Guries, 2007). It reaches maturity at 8 years of age, therefore harvesting should be done after that to allow the palm to reproduce (Gaiotto et al., 2003). However, intensive harvesting at any age has led to the decline of the species over much of the Atlantic Forest, so many of the surviving populations are small and fragmented (Galetti and Aleixo, 1998; Silva-Matos et al., 1999). Like all solitary palms, E. edulis has a single apical meristem, therefore, harvest the heart-of-palm causes the death of the genetic individual.
Geonoma schottiana Mart. (Ouricana) is a monoecious, solitary or rarely multi-stemmed, shade-tolerant, and slow-growing palm. It is typically 1–4 m in height and grows in the forest understory in lowland forests (Henderson et al., 1995; Lorenzi et al., 2004). It occurs in the Atlantic Forest and in forest formations in Cerrado (Henderson et al., 1995; Lorenzi et al., 2004). In the private property sites selected for this study, the leaves of G. schottiana are harvested for floral arrangements by cutting the leaves or the stem of the plant, which causes death.
Demographic Data
In each fragment, we censused palms in nine 30 m × 30 m plots distributed systematically in three blocks. Each block had three plots 50 m apart, while blocks were 100 m apart. One block was set up in the middle of each fragment and the other two blocks on opposite sides of the first block. In the protected areas, we used existing trails near the center of the fragments. All individuals for all sizes of the three palm species were numbered with an aluminum tag between June and September, 2005. Palm survival was subsequently monitored between June and September of 2006 and 2007. All new plants were also tagged. During each census, each plant was assigned to one of five development classes based on morphological and morphometric analysis: seedling, infant, juvenile, immature, and reproductive (Table 1; Portela et al., 2010).
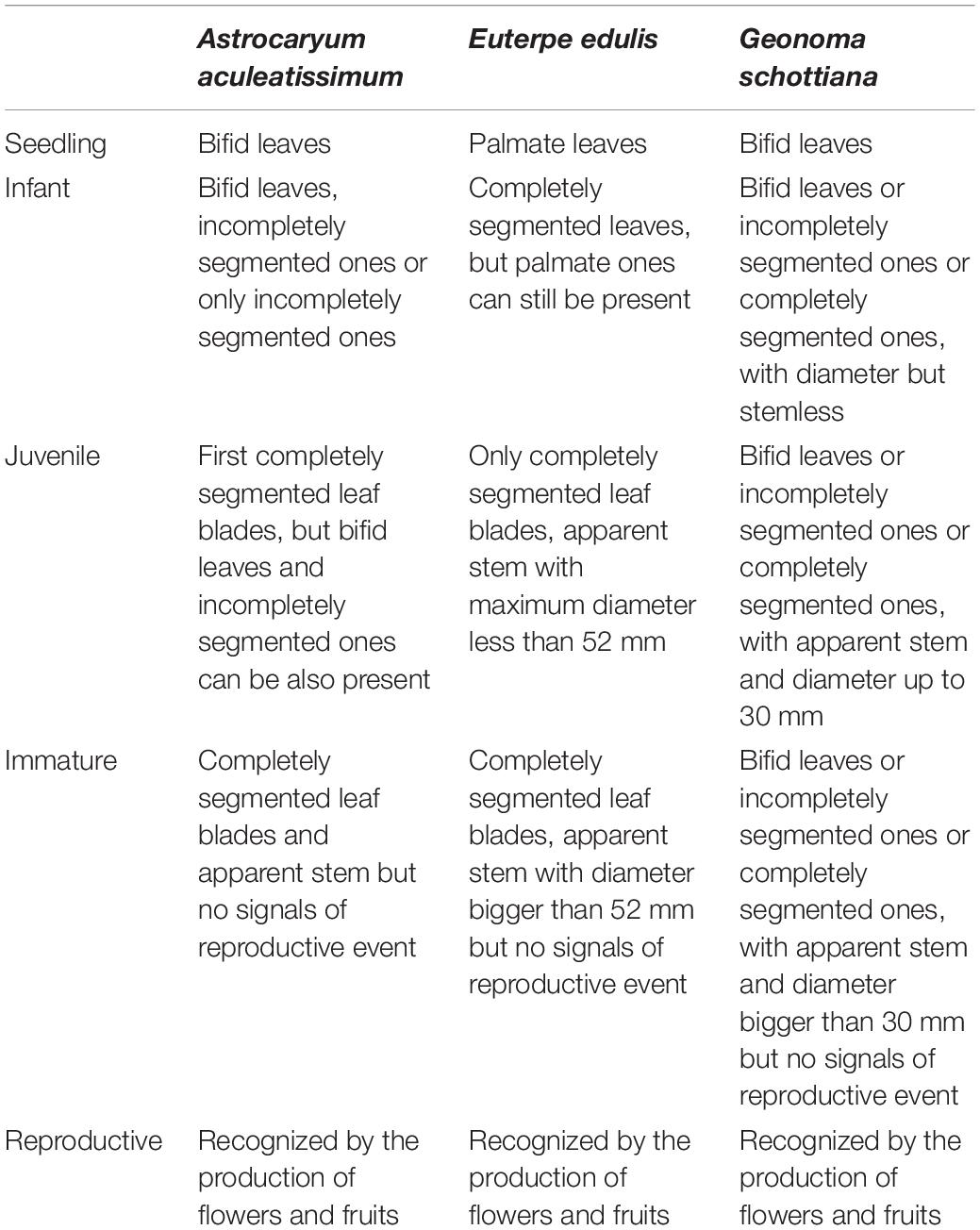
Table 1. Characteristics, based on morphological and morphometrical analysis, of the five ontogenetic stages for Astrocaryum aculeatissimum, Euterpe edulis, and Geonoma schottiana in three small forest fragments and two large forest fragments in the Atlantic Rain Forest.
Demographic Analysis
We developed summary matrices describing the dynamics of the populations in each fragment type (large and small) in each monitoring period (which comprised 1 year each, between 2005–2006 and 2006–2007) by pooling the data from the nine plots in each site type (large and small fragments) to create a “summary matrix.” Summary matrices are the best means of synthesizing the demography of multiple populations because they account for the disproportionate weight that low plant numbers in some size classes in some locations can give to transition probabilities (Horvitz and Schemske, 1995). In our study, using pooled matrices was advantageous because it allowed us to estimate several vital rates not observed in some of the small forest fragments due to low plant density.
From 1 year to the next, plants may grow into the following development class (g = growth), remain in the same stage (s = stasis), shrink into a preceding one (r = regress), or die. For each matrix we used deterministic population matrix models, and we calculated the lower level vital rates (g, s, r, and fecundity), lower level vital rates elasticities and the asymptotic population growth rate (λ) (Caswell, 2001). The standard matrix population model will project population growth if the dominant eigenvalue (λ) of a matrix is >1.0 (implying no resource limitations or competition), or population decline if λ < 1.0 (Caswell, 2001). We concluded that estimates of λ were significantly different from 1.0 if the bias-corrected 95% confidence intervals (CI) failed to include 1.0. Confidence intervals were estimated by bootstrapping; the raw data (individuals) were resampled 2,000 times to obtain 2,000 transition matrices for which we estimated λ. We then used the distribution of these estimates of λ to calculate the upper and lower 95% CI using the procedure detailed in Stubben and Milligan (2007).
All analyses were carried out with the Popbio package (Stubben and Milligan, 2007) in R 2.15.1 software (R Development Core Team, 2015).
Relative Diameter Growth Rate
Diameter of individuals of the demography plots (from year 2005 to 2007) was used to calculate the relative diameter growth rate (RDGR). RDGR = lnDf − lnDi / Tf-Ti. Where Di is the initial diameter value (2005), Df is the final diameter value (2007) and Tf-Ti is the difference between final and initial measurement times, 2 years.
Plant Functional Traits Data
To measure functional traits, we selected randomly 15 plants per species within the nine demographic plots in each fragment, when available, totaling 45 individuals per species in the three small fragments and 30 in the two large ones. Leaflets from the middle part of a whole leaf, pertaining to the mid-crown of each plant, were collected for leaf trait measurements.
The traits measured in the field were height (H, cm), with a Nikon Forestry Pro Laser Rangefinder/Height Meter, basal stem diameter (BSD, mm) just above the roots with a caliper, leaf area (LA, cm2) using a measuring tape and calculated from the area of the ellipse, and chlorophyll concentration in leaves using a non-destructive chlorophyll meter (soil-plant analysis development, Chlorophyll Meter SPAD- 502, Konica Minolta Sensing, Inc.).
For leaf-saturated weight and leaf thickness (TH, mm), small squares of pre-defined area were stored in Ziploc plastic bags with a humid cloth in the dark for 6 h, then weighed on a portable precision balance (Ohaus). Thickness was measured with a caliper (500-784 Mitutoyo IP67 Waterproof Electronic Caliper). The same leaf squares were dried in an oven for 72 h at 60°C and weighed again until constant dry mass values. Leaf saturated weight, dry weight and square area were used to calculate SLA (m2⋅kg–1) and LDMC (mg⋅g–1). Leaf veins were avoided in TH and SPAD readings.
We used RLC to determine the photosynthetic capacity of the different palm specimens in each forest fragment. RLC were measured using a PAM-2500 Portable Chlorophyll Fluorometer (Waltz). RLC provides key parameters such as α (alpha, electrons/photons), which refers to the initial slope of RLC related to the quantum efficiency of photosynthesis, maximum electron transport rate [ETRmax, μmol electrons/(m2⋅s)], and IK [μmol photons/(m2⋅s)], which is the minimum saturating irradiance. The curve represents the relationship between ETR and irradiance (PAR: photosynthetic active radiation) emitted by the PAM fluorometer. The maximum quantum yield in limited light conditions is where alpha intersects the maximum ETR. The saturation irradiance (Ik) value indicates the point where the maximum ETR and alpha intersect, potentially representing the initial saturation point.
Analysis of Plant Functional Traits
As the data did not follow a normal distribution and due to small number of fragments in both category of size, we estimated the mean, size of standard error, standard deviation, and confidence intervals by applying the bootstrap method to each trait per species per fragment size, taking resamples 100,000 times with replacement from the original sample using the package boot in R software (R 3.1.3, R Development Core Team, 2015). We must point out that it is difficult to find well preserved fragments in the studied region. Because of that, fragments were not random but a fixed factor in our analysis.
Results
Deterministic Asymptotic Growth Rate (λ)
Astrocaryum aculeatissimum populations were stable in both monitoring periods, as well as in the small and large fragments (Table 2). E. edulis populations declined at rates of 4.22 and 12.41% per year between 2005–2006 and 2006–2007, respectively, in large fragments. The 95% CI for these estimates were lower than 1.0 in both monitoring periods. In contrast, populations in the small fragments were stable throughout both periods. G. schottiana populations remained stable in the first period of the study in both fragment types, but then, only in the large fragment in the second period. In the second period, the population in the small fragments declined at a rate of 9.18%, with 95% CI for these estimates being lower than 1.0 in both periods.

Table 2. Deterministic asymptotic growth rate (λ) and 95% confidence intervals (95% CI) for Astrocaryum aculeatissimum, Euterpe edulis, and Geonoma schottiana in three small forest fragments and two large forest fragments in the Atlantic Rain Forest.
Lower-Level Vital Rates
The survival rate of A. aculeatissimum, E. edulis, and G. schottiana was high in all development classes and exceeded 75% in post-seedling stages in the large and small fragments (Table 3). A. aculeatissimum and E. edulis had the highest seedling survival rates: 87.16 to 72.37%, whereas G. schottiana had the lowest: 43.82% in 2005–2006 and 22.51% in 2006–2007.
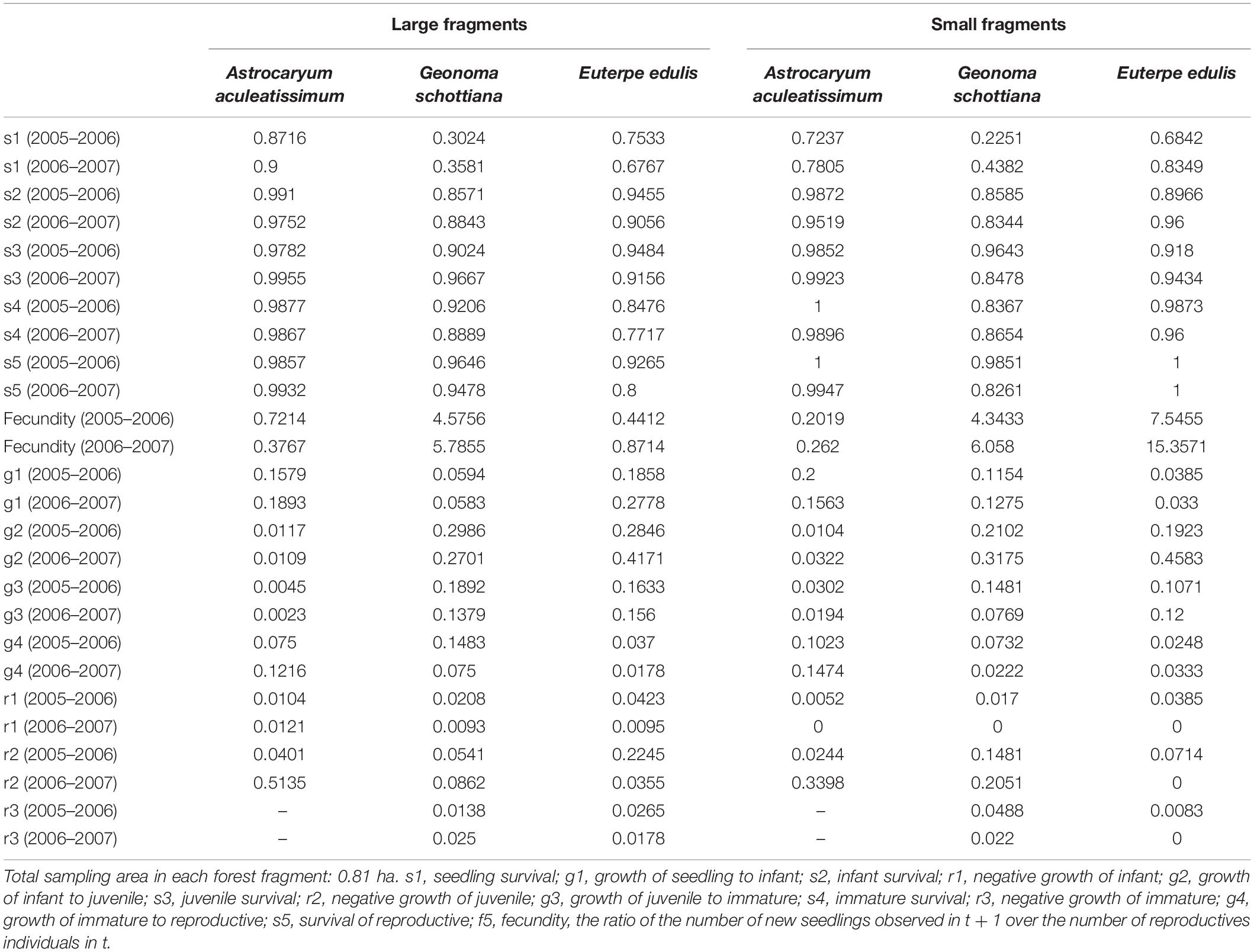
Table 3. Lower-level vital rates for Astrocaryum aculeatissimum, Euterpe edulis, and Geonoma schottiana in three small and two large forest fragments in the Atlantic Rain Forest.
The growth of A. aculeatissimum, E. edulis, and G. schottiana in all stage classes did not vary between years or fragment size (Table 3). However, the development of the seedling into the infant stage was slower in large fragments for G. schottiana and faster for E. edulis when compared with the small fragments, regardless of the monitoring period. The second-period growth of G. schottiana from juvenile to immature and the first-period growth of immature to reproductive were much faster in the large fragments compared with the small fragments, regardless of the period.
A small proportion of palms (less than 10%) receded to a previous stage class after a 1-year period (Table 3). In the case of A. aculeatissimum, 51.35 and 33.98% of juvenile plants receded to the infant stage in the second monitoring period in both small and large fragments, while 22.45% of E. edulis juveniles receded to the infant stage in the first monitoring period in large fragments. The negative growth of G. schottiana juveniles was much higher in small fragments in both transition years.
Euterpe edulis was the palm of highest fecundity in small fragments in both monitoring periods. G. schottiana fecundity was higher, regardless of fragment size and period, compared with the other two species, except with E. edulis in small fragments. E. edulis had a much lower fecundity rate in large fragments in both monitoring periods (Table 3). For the three species and for the two kinds of fragments, the fecundity rate was higher in the second monitoring period for all three species in all fragment sizes, with the exception of A. aculeatissimum in large fragments in the second monitoring period.
Lower-Level Vital Rates Elasticities
For both transition years, elasticity patterns for the three palm species were very similar to each other and for the two kinds of fragments. The highest elasticity values (higher than 0.40) were for survival, especially for the later ontogenetic stages: immature and reproductive (Table 4). Values for growth, regressions and fecundity were generally low. The highest vital rates elasticities for A. aculeatissimum were similar between both transitions years and kind of fragment, with the highest elasticities representing survival of reproductive. For G. schottiana in the second transition year in the small fragments, the elasticity for the reproductive survival was lower when compared with the big fragment and previous year. The highest vital rates elasticities for E. edulis were similar between the two kinds of fragments, but different between years, the elasticities representing survival of reproductive were lower in the second transition year. For the three palms, the survival of reproductives was the most important lower level vital rate for λ and should be the primary targets of management efforts.
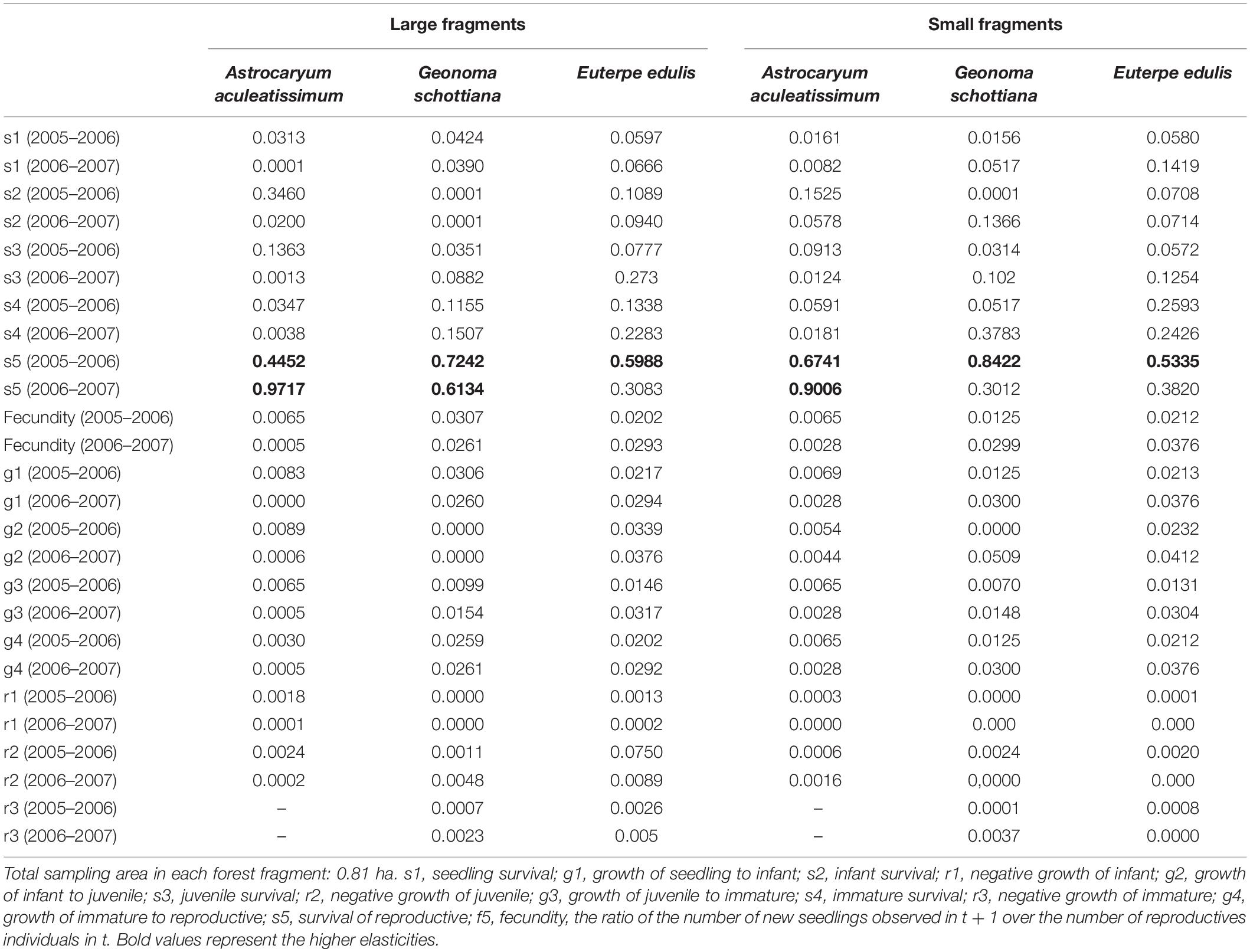
Table 4. Elasticity of lower-level vital rates for Astrocaryum aculeatissimum, Euterpe edulis, and Geonoma schottiana in three small and two large forest fragments in the Atlantic Rain Forest.
Relative Diameter Growth Rate
The three species showed a diameter growth gradient, in the following sequence from the least to the largest: A. aculeatissimum, E. edulis, and G. schottiana (Figure 1). Immature and reproductive individuals of A. aculeatissimum presented a very low RDGR in both fragment types. Immature individuals of E. edulis presented a higher RDGR in large fragments compared with individuals in small fragments. Reproductive individuals of G. schottiana presented a higher RDGR in large fragments compared with individuals in small fragments, but the RDGR of immature individuals was not different between the two types of fragments.
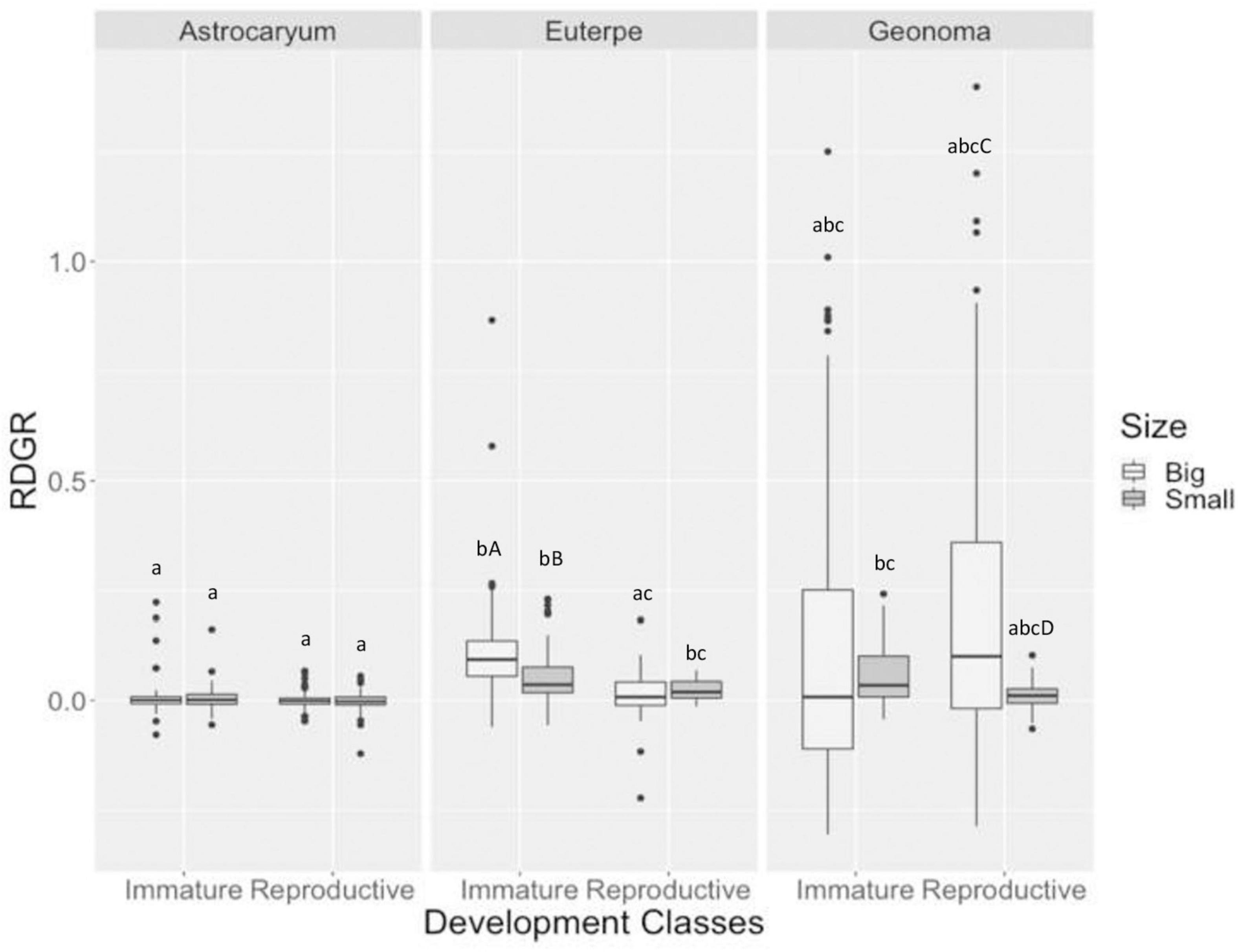
Figure 1. Relative diameter growth rate (RDGR) of individuals of the demography plots (from year 2005 to 2007). The bootstrap confidence intervals were calculated for mean difference, lowercase letters indicate differences in the mean value of the attribute between species, and capital letters indicate differences between fragments.
Functional Traits
The bootstrap confidence intervals were calculated for mean difference (Table 5). Bootstrap and confidence limits for H suggested no differences in the mean H between fragment sizes, but it was between species. A. aculeatissimum and E. edulis were taller, reaching the canopy strata in some areas, while G. schottiana was the smallest, growing in the understory (Figure 2A). E. edulis BSD was larger in the large fragments, while G. schottiana showed the lower values (Figure 2B). Morphological leaf traits differed between species (Figures 2C–F). A. aculeatissimum showed the highest LA, TH, LDMC, and lower SLA, whereas G. schottiana showed the inverse results. Leaf thickness (TH, mm) was the only trait that was lower in small fragments in G. schottiana. Physiological traits related to the photosynthetic response to light showed different trends among species and fragments (Figures 2G–J). Maximum electron transport rate (ETRmax, μmol electrons m–2s–1) was lower in G. schottiana, but did not differ between fragment size (Figure 2G). Minimum Ik [μmol photons/(m2*s)] did not differ between fragments, but it was lower in G. schottiana (Figure 2H). The quantum efficiency of photosynthesis (α, electrons/photons) was similar between species and fragments (Figure 2I). SPAD values were similar between species and fragment size (Figure 2J).
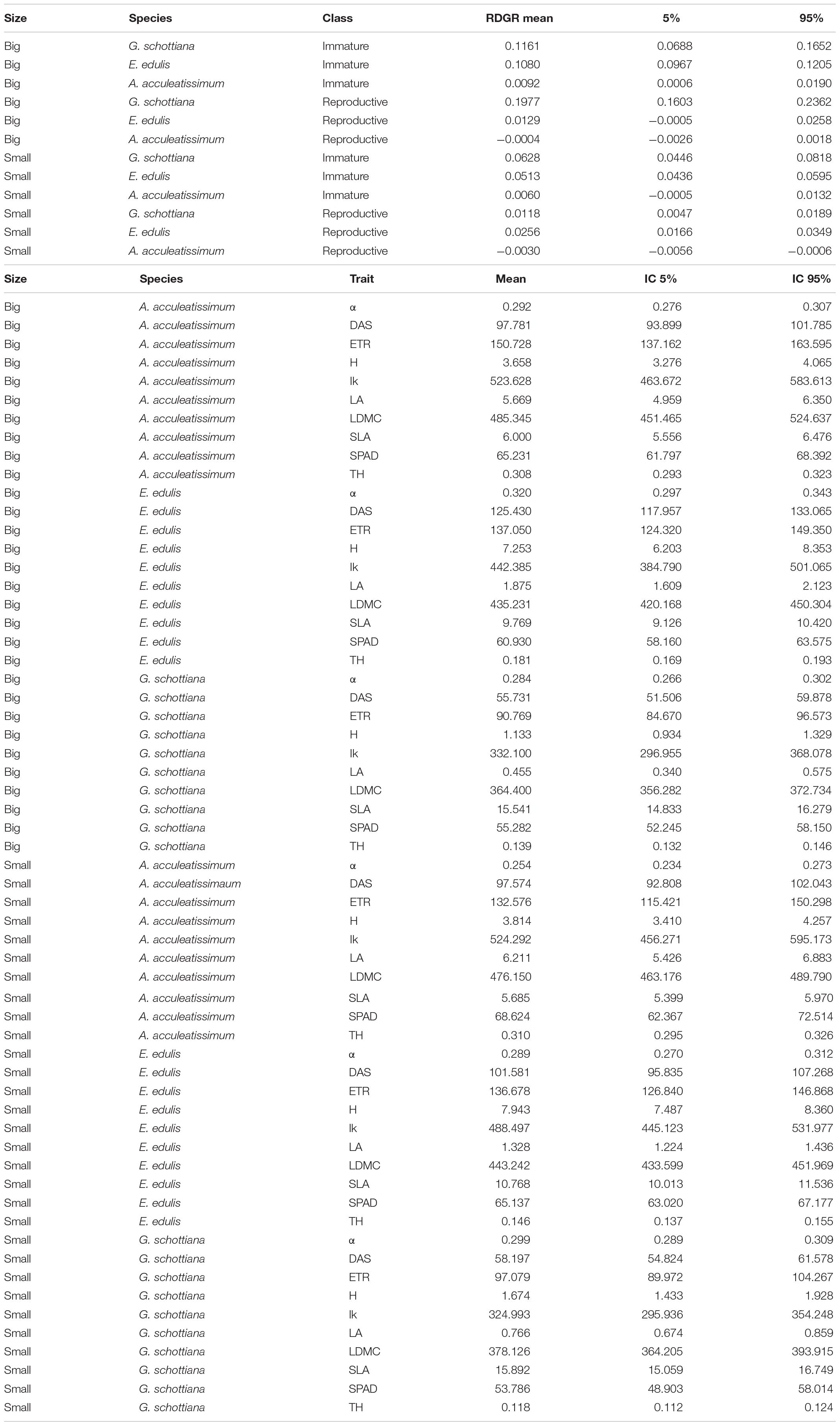
Table 5. The bootstrap confidence intervals for mean of: relative diameter growth rate (RDGR), height (H, cm), basal stem diameter (BSD, mm), leaf area (LA, cm2), leaf thickness (TH, mm), and leaf dry matter content (LDMC, mg⋅g–1), specific leaf area (SLA, m2⋅kg–1), parameter α (alpha, electrons/photons), maximum electron transport rate [ETRmax, μmol electrons/(m2⋅s)], IK, [μmol photons/(m2⋅s)], and chlorophyll concentration (SPAD).
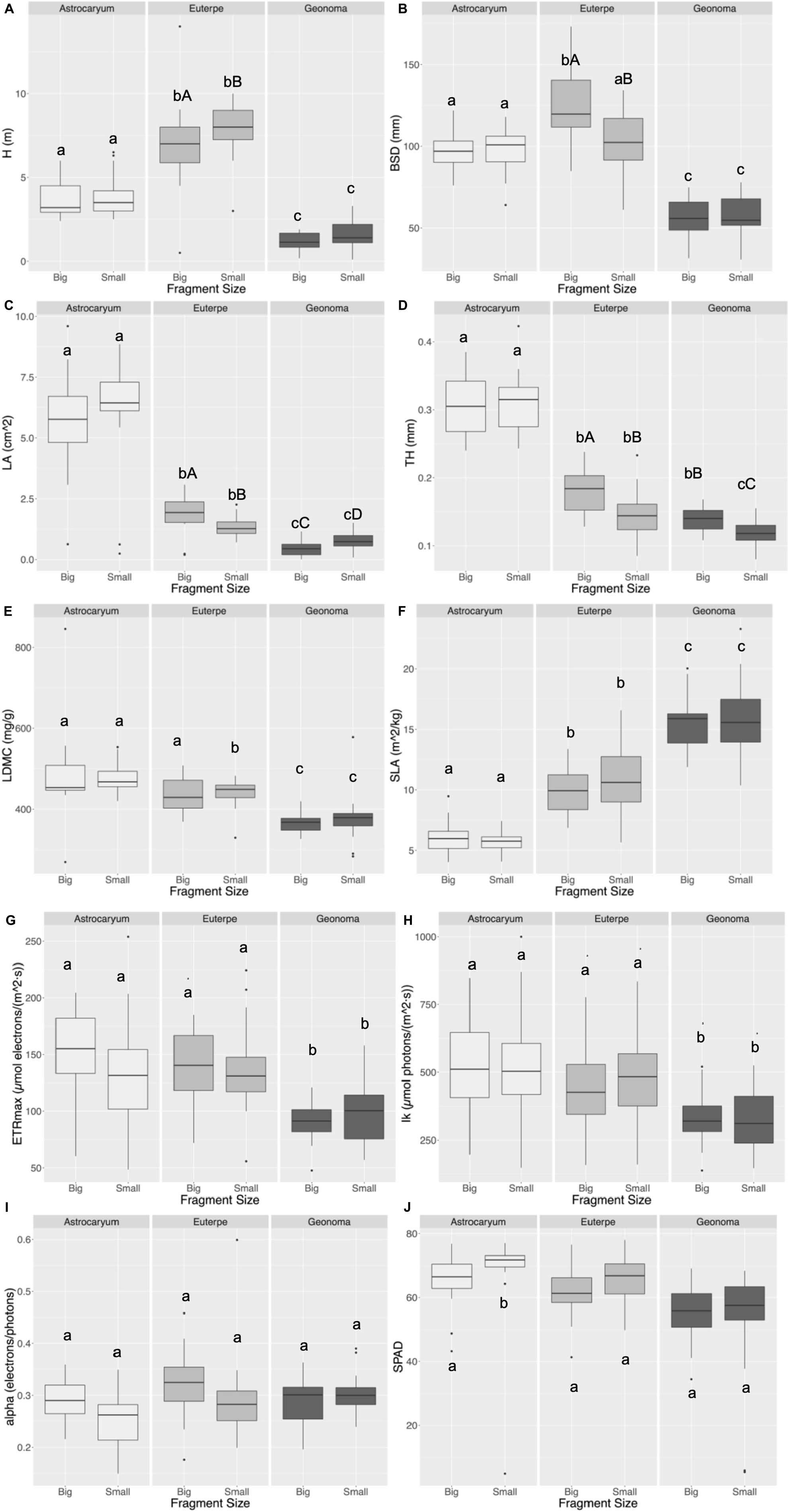
Figure 2. Functional traits measured for each palm species in large and small fragments: height (H, cm – A), basal stem diameter (BSD, mm – B), leaf area (LA, cm2 – C), leaf thickness (TH, mm – D), and leaf dry matter content (LDMC, mg⋅g–1 – E), specific leaf area (SLA, m2⋅kg–1 – F). We used rapid light curves (RLC) to measure the present state of photosynthesis using a PAM-2500 Portable Chlorophyll Fluorometer (Waltz). RLC provide the key parameter α (alpha, electrons/photons – I), which represents the initial slope of RLC related to the quantum efficiency of photosynthesis, maximum electron transport rate [ETRmax, μmol electrons/(m2⋅s) – G], and IK, [μmol photons/(m2⋅s) – H], which refers to the minimum saturating irradiance and chlorophyll concentration (SPAD – J). The bootstrap confidence intervals were calculated for mean difference, lowercase letters indicate differences in the mean value of the attribute between species, and capital letters indicate differences between fragments.
Discussion
The connections between functional traits and demography are not easily demonstrated (Yang et al., 2018). The connections become even more challenging when individuals of different species are subjected to distinct environmental conditions, as those found in fragments of different sizes (Zambrano et al., 2019). We observed, however, that three palm species occurring in the Atlantic Rain Forest differed regarding functional traits related to leaf economic spectrum and also showed differential responses to population growth rates when occurring in fragments of different sizes. As expected, G. schottiana, the only understory species showed morpho-physiological functional traits related to more shady environments, such as lower LA, TH, LDMC, ETR and Ik, and higher SLA. E. edulis, despite been the tallest species showed often intermediary functional trait values, such as LA, TH, and SLA. In contrast, A. aculeatissimum showed traits more related to a conservative use of resources, very low growth rates, and showed population stability in time and by fragment size. Despite all species showed elasticity values more strongly related to survival, G. schottiana and E. edulis showed declines in population growth rates in small and large fragments, respectively. G. schottiana, however, also showed lower vital rates and elasticity for the reproductive survival associated to small fragments and in most cases only in the second period of study. This indicates that this species might be the most negatively affected by fragmentation when in conjunction with drought years.
According to the scientific literature, the three palms analyzed in our study are considered shade-tolerant species (Arroyo-Rodríguez et al., 2007; Gatti et al., 2011), capable of regenerating in the shaded understory of mature forests (Tabarelli et al., 1999). However, species varied in a continuum along the leaf economic spectrum with probable consequences to the observed responses to fragment size. It is important to note, that the causes for population declines to fragmentation may differ between E. edulis and G. schottiana. Different from G. schottiana, E. edulis population was decreasing in the large fragments in both monitoring periods. This was caused by heart-of-palm consumption by a hyper-abundant monkey population (Sapajus nigritus) in these areas. This dramatic population decline was detected in a 10-year plant demographic study in the same area (Portela and Dirzo, 2020). Apart from this disruptive interaction, this palm species seem to have higher capacity to overcome the challenges of high light availability or fluctuating light conditions (Schumann et al., 2017; Li et al., 2019), such as would occur in smaller fragments with more open canopy, sunflecks, or tree-fall gaps. Besides the differences in leaf functional traits, the three studied species presents higher values of sensibility that is characteristic of long-lived and slow-grow species. Species considered to be long-lived and slow-grow commonly have a greater influence of individuals from late (reproductive) classes on λ, as they have a higher survival rate within the population. In short-lived fast-grow species, individuals from early classes (seedling) tend to have the greatest influence on λ, precisely due to the high survival and growth rates of these individuals (Franco and Silvertown, 2004).
A substantial loss of palm stems has been reported in the scientific literature in response to reduced forest cover on a landscape scale, following a non-linear pattern decline, which suggests that Arecaceae is very sensitive to deforestation and habitat loss (Benchimol et al., 2016). The response of palms to reduced forest cover was positive for open-area species and negative for forest-interior species. Some genera of palm species, like Geonoma and Bactris, are already known for reduced population growth in altered environments, and categorized as forest-interior species (Chazdon, 1985; Svenning, 2001; Benchimol et al., 2016). Benchimol et al. (2016) stated that the entire Geonoma genus represents conspicuous elements of Atlantic Forests, found in closed and less disturbed forests. Their assessment may be used as indicator of local levels of forest degradation, particularly related to the structural shrinkage of native forests and increasing levels of canopy openness. Chazdon (1985) shows that lower biomass costs of light interception in adult Geonoma cuneata from a well preserved tropical premontane wet forest enable this species to exploit successfully the most deeply shaded microsites in the rain forest understory. Low light levels required to saturate photosynthesis by means of low Ik, together with leaf traits related to low leaf construction costs, such as lower values of LA, LDMC, and higher SLA are crucial for G. schottiana be able to deal with the light environment of the forest understory (Poorter and Bongers, 2006; Poorter, 2009). In addition, the low photosynthetic potential evidenced by low ETR values may extend the payback time of the investment in leaf construction. In contrast, adult E. edulis palms showed a generalist response in light behavior (Benchimol et al., 2016) and was the species with intermediary functional trait values, but young individuals had low potential for growth and survival in forests with greater canopy openness and light transmission (Gatti et al., 2011; Cerqueira et al., 2021). Therefore, small forest fragments have more open canopy and seem to be unfavorable for the establishment of typical shade-tolerant species as G. schottiana, and even for those with great plasticity and wide geographical distribution as E. edulis. This trend has potentially severe ecological and ecosystem consequences (Cerqueira et al., 2021).
As aforementioned, G. schottiana was more affected demographically by habitat reduction than the other two palms in our study. Seedling survival was much lower compared with the other two species, and negative growth of juveniles was much higher in small fragments in both monitoring periods. The same intense negative growth was observed in small fragments for Heliconia acuminata, a perennial herb native to central Amazonia (Bruna and Oli, 2005). The population of G. schottiana in small fragments was decreasing in size in the second monitoring period, a trend that seemed to be directly related to a reduction in rainfall, which amounted to 3,472 mm in 2005, 2,664 mm in 2006, and 2,271 mm in 2007. The effects of rainfall reductions could be more pronounced in small fragments because it tends to be drier due to edge effects (Laurance et al., 2002). As Braz et al. (2016) stated that Geonoma seeds are sensitive to water scarcity, this might explain the lower seedling survival rate. However, in a climate change scenario, Geonoma may not be endangered in small forest fragments, as an increase in precipitation and temperature is predicted for southeastern Brazil over the coming decades (Vale et al., 2021), along with a probable decrease in the duration of dry spells (Nunes et al., 2018). However, uncertainty on the occurrence of extreme rainfall events may not be ruled out (Zilli et al., 2017).
It seems evident that forest-interior species such as G. schottiana are more affected by habitat loss and forest fragmentation. In contrast, E. edulis, an endangered species, and A. aculeatissimum, an endemic species of the Atlantic Forest, may be favored by their higher capacity to use higher light intensities due to their higher ETR and Ik, which may explain the occurrence of persistent populations (stable lambda) in small forest fragments. The size of the fragments analyzed in our study represents the size of the majority of fragments in the Atlantic Forest (more than 80% of the fragments are <50 ha; Ribeiro et al., 2009). Souza and Prevedello (2020) studied the density and demography of E. edulis and found that protected areas may be crucial for the long-term conservation of overexploited plants. Mendes and Portela (2020) presented empirical data on the importance of small populations in very small forest fragments, even with few adults/ha. Demographic data collected over 15 years (2005–2019) from three small Atlantic Rainforest fragments showed that all E. edulis populations were demographically viable. Volenec and Dobson (2020) synthesized results of existing empirical studies on the contribution of individual small reserves to biodiversity conservation across taxa and ecosystems. They found that small reserves and fragments may provide a significant contribution to maintain matrix quality in the landscape, as they can harbor significant portions of regional biodiversity. As mentioned, small fragments represent the majority of Atlantic Forest habitat left, and may be essential to maintain viable populations in areas of high human disturbance. Small populations scattered in a highly fragmented landscape might constitute a metapopulation that can help maintain viable genetic populations, therefore deserving attention and conservation efforts.
Conclusion
Differences in ecophysiological performance due to distinct morpho-physiological functional traits related to leaf economic spectrum, such as LDMC or SLA and to photosynthetic responses to light environment as ETR and Ik were linked to the demographic variation of palms in forest remnants with different characteristics. G. schottiana was demographically affected by habitat reduction (lower fitness in small fragments). Given the species morphological and physiological traits, it should be classified as a low disturbance forest interior species. E. edulis was also affected by the size of the fragment, but due to a disruptive interaction with a predator and showed intermediate functional traits values. On the other hand, A. aculeatissimum were not demographically affected by forest remnant size, which is probably due to higher photosynthetic capacity as well as other morphological characteristics related to a conservative use of resources in addition to the capacity for shade tolerance. We highlight the importance of considering small Atlantic Forest fragments in private properties for biodiversity conservation efforts, as they contribute to the maintenance of populations with different morpho-physiological functional traits and demographic behavior on a landscape scale. Conserving these small habitats is possible to conserve different life-histories, even for close related species.
Data Availability Statement
The raw data supporting the conclusions of this article will be made available by the authors, without undue reservation.
Author Contributions
RP, SC-T, and EM conceived this study, collected the data, and wrote the manuscript. RP and SC-T analyzed the data. All authors contributed to the article and approved the submitted version.
Funding
Financial support was provided by FAPESP (Proc. No. 2005/60788-4) and CAPES fellowship to RP and SC-T.
Conflict of Interest
The authors declare that the research was conducted in the absence of any commercial or financial relationships that could be construed as a potential conflict of interest.
Publisher’s Note
All claims expressed in this article are solely those of the authors and do not necessarily represent those of their affiliated organizations, or those of the publisher, the editors and the reviewers. Any product that may be evaluated in this article, or claim that may be made by its manufacturer, is not guaranteed or endorsed by the publisher.
Acknowledgments
We thank Eraldo dos Santos Almeida, Adilson Pintor, and Antonio Tavares de Oliveira for help in the field. We are grateful to ICMBIO for permission to work in their protected areas and to private landowners for permission to work in the fragments. We also would like to thanks the reviewers comments that improved the manuscript.
References
Ackerly, D. D., and Cornwell, W. K. (2007). A trait-based approach to community assembly: partitioning of species trait values into within- and among-community components. Ecol. Lett. 10, 135–145. doi: 10.1111/j.1461-0248.2006.01006.x
Adler, P. B., Salguero-Gómez, R., Compagnoni, A., Hsu, J. S., Ray-mukherjee, J., Mbeau-Ache, C., et al. (2014). Functional traits explain variation in plant life history strategies. Proc. Natl. Acad. Sci. U.S.A. 111, 740–745. doi: 10.1073/pnas.1410430111
Arroyo-Rodríguez, V., Aguirre, A., Benítez-Malvido, J., and Mandujano, S. (2007). Impact of rain forest fragmentation on the population size of a structurally important palm species: Astrocaryum mexicanum at Los Tuxtlas, Mexico. Biol. Conserv. 138, 198–206. doi: 10.1016/j.biocon.2007.04.016
Benchimol, M., Talora, D. C., Mariano-Neto, E., Oliveira, T. L. S., Leal, A., Mielke, M. S., et al. (2016). Losing our palms: the influence of landscape-scale deforestation on Arecaceae diversity in the Atlantic forest. For. Ecol. Manag. 384, 314–322. doi: 10.1016/j.foreco.2016.11.014
Braz, M. I. G., Moura, R. M., Portela, R. C. Q., and de Mattos, E. A. (2016). Ample germination ability under wide-ranging environmental conditions in a common understory tropical palm. Plant Species Biol. 31, 211–218. doi: 10.1111/1442-1984.12104
Bruna, E. M., and Oli, M. K. (2005). Demographic effects of habitat fragmentation on a tropical herb: life-table response experiments. Ecology 86, 1816–1824. doi: 10.1890/04-1716
Caswell, H. (2001). Matrix Population Model: Construction, Analysis and Interpretation, 2nd Edn. Sunderland, MA: Sinauer Associates, 722.
Carvalho, F. A. (2005). Efeitos da Fragmentação Florestal na Florística e Estrutura da Mata Atlântica Submontana de Região de Imbaú, Município de Silva Jardim, RJ. Ph.D. thesis. Campos dos Goytacazes, BR: Universidade Estadual no Norte Fluminense Darcy Ribeiro. doi: 10.1590/S0102-33062006000300022
Cavender-Bares, J. A., and Bazzaz, F. (2008). “From leaves to ecosystems: using chlorophyll fluorescence to assess photosynthesis and plant function in ecological studies,” in Chlorophyll a Fluorescence. Advances in Photosynthesis and Respiration, Vol. 19, eds G. Papageorgiou and C. Govindjee (Dordrecht: Springer), 737–755. doi: 10.1007/978-1-4020-3218-9_29
Cerqueira, A. F., Rocha-Santos, L., Benchimol, M., and Mielke, M. S. (2021). Habitat loss and canopy openness mediate leaf trait plasticity of an endangered palm in the Brazilian Atlantic Forest. Oecologia 196, 619–631. doi: 10.1007/s00442-021-04879-x
Chazdon, R. L. (1985). Leaf display, canopy structure, and light interception of two understory palm species. Am. J. Bot. 72, 1493–1502. doi: 10.2307/2443299
De Bello, F., Vandewalle, M., Reitalu, T., Lepš, J., Prentice, H. C., Lavorel, S., et al. (2013). Evidence for scale- and disturbance-dependent trait assembly patterns in dry semi-natural grasslands. J. Ecol. 101, 1237–1244. doi: 10.1111/1365-2745.12139
Dupré, C., and Ehrlén, J. (2002). Habitat configuration, species traits and plant distributions. J. Ecol. 90, 796–805. doi: 10.1046/j.1365-2745.2002.00717.x
Fahrig, L. (2003). Effects of habitat fragmentation on biodiversity. Annu. Rev. Ecol. Evol. Syst. 34, 487–515. doi: 10.1146/annurev.ecolsys.34.011802.132419
Fahrig, L. (2013). Rethinking patch size and isolation effects: the habitat amount hypothesis. J. Biogeogr. 40, 1649–1663. doi: 10.1111/jbi.12130
Fantini, A. C., and Guries, R. (2007). Forest structure and productivity of palmiteiro (Euterpe edulis Martius) in the Brazilian Mata Atlântica. For. Ecol. Manag. 242, 185–194.
Figueroa, F. L., Jerez, C. G., and Korbee, N. (2013). Use of in vivo chlorophyll fluorescence to estimate photosynthetic activity and biomass productivity in microalgae grown in different culture systems. Lat. Am. J. Aquat. Res 41, 801–819. doi: 10.3856/vol41-issue5-fulltext-1
Franco, M., and Silvertown, J. (2004). A comparative demography of plants based upon elasticities of vital rates. Ecology 85, 531–538. doi: 10.1890/02-0651
Galetti, M., and Aleixo, A. (1998). Effects of palm heart harvesting on avian frugivores in the Atlantic rain forest of Brazil. J. Appl. Ecol. 35, 286–293.
Gaiotto, F. A., Grattapaglia, D., and Vencovsky, R. (2003). Genetic structure, mating system and long-distance gene flow in Euterpe edulis mart. J. Hered. 94, 399–406. doi: 10.1093/jhered/esg087
Gatti, M. G., Campanello, P. I., and Goldstein, G. (2011). Growth and leaf production in the tropical palm Euterpe edulis: light conditions versus developmental constraints. Flora Morphol. Distrib. Funct. Ecol. Plants 206, 742–748. doi: 10.1016/j.flora.2011.04.004
Haddad, N. M., Brudvig, L. A., Clobert, J., Davies, K. F., Gonzalez, A., Holt, R. D., et al. (2015). Habitat fragmentation and its lasting impact on Earth’s ecosystems. Sci. Adv. 1:e1500052. doi: 10.1126/sciadv.1500052
Henderson, A., Galeano, G., and Bernal, R. (1995). Field Guide to the Palms of the Americas. Princeton, NJ: Princeton University Press.
Horvitz, C. C., and Schemske, D. W. (1995). Spatiotemporal variation in demographic transitions of a tropical understory herb: projection matrix analysis. Ecol. Monogr. 65:155. doi: 10.2307/2937136
Ibáñez, I., Katz, D. S. W., Peltier, D., Wolf, S. M., and Connor Barrie, B. T. (2014). Assessing the integrated effects of landscape fragmentation on plants and plant communities: the challenge of multiprocess-multiresponse dynamics. J. Ecol. 102, 882–895. doi: 10.1111/1365-2745.12223
IBGE (2012). Manual de Classificação da Vegetação Brasileira. Coordenação de Recursos Naturais e Estudos Ambientais. Série Secundária: Manuais técnicos em geociências. Brasil: IBGE.
Laughlin, D. C., Gremer, J. R., Adler, P. B., Mitchell, R. M., and Moore, M. M. (2020). The net effect of functional traits on fitness. Trends Ecol. Evol. 35, 1037–1047. doi: 10.1016/j.tree.2020.07.010
Laurance, W. F., Lovejoy, T. E., Vasconcelos, H. L., Bruna, E. M., Didham, R. K., Stouffer, P. C., et al. (2002). Ecosystem decay of Amazonian forest fragments: a 22-year investigation. Conserv. Biol. 16, 605–618. doi: 10.1046/j.1523-1739.2002.01025.x
Li, Z. R., Wakao, S., Fischer, B. B., and Niyogi, K. K. (2019). Sensing and responding to excess light. Annu. Rev. Plant Biol. 60, 239–260. doi: 10.1146/annurev.arplant.58.032806.103844
Liu, J., Coomes, D. A., Gibson, L., Hu, G., Liu, J., Luo, Y., et al. (2019). Forest fragmentation in China and its effect on biodiversity. Biol. Rev. 94, 1636–1657. doi: 10.1111/brv.12519
Lorenzi, H., Souza, H. M., Medeiros-Costa, J. T., Cerqueira, L. S. C., and Ferreira, E. (2004). Palmeiras Brasileiras e Exóticas Cultivadas. Nova Odessa: Plantarum.
May, F., Giladi, I., Ristow, M., Ziv, Y., and Jeltsch, F. (2013). Plant functional traits and community assembly along interacting gradients of productivity and fragmentation. Perspect. Plant Ecol. Evol. Syst. 15, 304–318. doi: 10.1016/j.ppees.2013.08.002
Mendes, E. T. B., and Portela, R. C. Q. (2020). Comments on “the importance of protected areas for overexploited plants: evidence from a biodiversity hotspot”. souza and prevedello 2020. Biol. Conserv. 243:108482. doi: 10.1016/j.biocon.2020.108617
Messier, J., McGill, B. J., Enquist, B. J., and Lechowicz, M. J. (2016). Trait variation and integration across scales: Is the leaf economic spectrum present at local scales? Ecography 13, 685–697. doi: 10.1111/ecog.02006
Nunes, L. H., Greco, R., and Marengo, J. A. (2018). Climate Change in Santos Brazil: Projections, Impacts and Adaptation Options. Berlin: Springer. doi: 10.1007/978-3-319-96535-2
Poorter, L. (2009). Leaf traits show different relationships with shade tolerance in moist versus dry tropical forests. New Phytol. 181, 890–900. doi: 10.1111/j.1469-8137.2008.02715.x
Poorter, L., and Bongers, F. (2006). Leaf traits are good predictors of plant performance across 53 rain forest species. Ecology 87, 1733–1743. doi: 10.1890/0012-9658(2006)87[1733:LTAGPO]2.0.CO;2
Portela, R. C. Q., Bruna, E. M., and Santos, F. A. M. (2010). Demography of palm species in Brazil’s Atlantic forest: a comparison of harvested and unharvested species using matrix models. Biodivers. Conserv. 19, 2389–2403. doi: 10.1007/s10531-010-9846-5
Portela, R. C. Q., and Dirzo, R. (2020). Forest fragmentation and defaunation drive an unusual ecological cascade: predation release, monkey population outburst and plant demographic collapse. Biol. Conserv. 252:108852. doi: 10.1016/j.biocon.2020.108852
R Development Core Team (2015). R: A Language and Environment for Statistical Computing. Vienna: R Foundation for Statistical Computing.
Ribeiro, M. C., Metzger, J. P., Martensen, A. C., Ponzoni, F. J., and Hirota, M. M. (2009). The Brazilian Atlantic forest: how much is left, and how is the remaining forest dis- tributed? Implications for conservation. Biol. Conserv. 142, 1141–1153. doi: 10.1016/j.biocon.2009.02.021
Rüder, N., Comita, L. S., Condit, R., Purves, D., Rosenbaum, B., Visser, M. D., et al. (2018). Beyond the fast-slow continuum: demographic dimensions structuring a tropical tree community. Ecol. Lett. 21, 1075–1084. doi: 10.1111/ele.12974
Schumann, T., Paul, S., Melzer, M., Dörmann, P., and Jahns, P. (2017). Plant growth under natural light conditions provides highly flexible short-term acclimation properties toward high light stress. Front. Plant Sci. 8:681. doi: 10.3389/fpls.2017.00681
Silva-Matos, D. M., Freckleton, R. P., and Watkinson, A. R. (1999). The role of density dependence in the population dynamics of a tropical palm. Ecology 80, 2635–2650. doi: 10.1111/nph.15107
Silva-Matos, D. M., and Watkinson, A. R. (1998). The fecundity, seed and seedling ecology of the edible palm Euterpe edulis in south-eastern Brazil. Biotropica 30, 595–603. doi: 10.1111/j.1744-7429.1998.tb00099.x
Souza, A. C., and Prevedello, J. A. (2020). The importance of protected areas for overexploited plants: evidence from a biodiversity hotspot. Biol. Conserv. 243:108482. doi: 10.1016/j.biocon.2020.108482
Souza, A. F., and Martins, F. R. (2004). Population structure and dynamics of a neotropical palm in fire-impacted fragments of the Brazilian Atlantic Forest. Biodivers. Conserv. 13, 1611–1632. doi: 10.1023/B:BIOC.0000029326.44647.7f
Stubben, C. J., and Milligan, B. G. (2007). Estimating and analyzing demographic models using the popbio package in R. J. Stat. Softw. 22, 1–23. doi: 10.18637/jss.v022.i11
Svenning, J.-C. (2001). On the role of microenvironmental heterogeneity in the ecology and diversification of neotropical rain-forest palms (Arecaceae). Bot. Rev. 67, 1–53. doi: 10.1007/BF02857848
Tabarelli, M., Mantovani, W., and Peres, C. A. (1999). Effects of habitat fragmentation on plant guild structure in the montane Atlantic forest of southeastern Brazil. Biol. Conserv. 91, 119–127. doi: 10.1016/S0006-3207(99)00085-3
Vale, M. M., Arias, P. A., Ortega, G., Cardoso, M., Oliveira, B. F. A., Loyola, R., et al. (2021). “Climate change and biodiversity in the Atlantic Forest: best climatic models, predicted changes and impacts, and adaptation options,” in The Atlantic Forest: History, Biodiversity, Threats and Opportunities of the Mega-Diverse Forest, eds M. C. M. Marques and C. E. V. Grelle (Cham: Springer), 253–267. doi: 10.1007/978-3-030-55322-7_12
Violle, C., Navas, M.-L., Vile, D., Kazakou, E., Fortunel, C., Hummel, I., et al. (2007). Let the concept of trait be functional! Oikos 116, 882–892. doi: 10.1111/j.2007.0030-1299.15559.x
Volenec, Z. M., and Dobson, A. P. (2020). Conservation value of small reserves. Conserv. Biol. 34, 66–79. doi: 10.1111/cobi.13308
Westoby, M., Falster, D. S., Moles, A. T., Vesk, P. A., and Wright, I. J. (2002). Plant ecological strategies: some leading dimensions of variation between species. Annu. Rev. Ecol. Syst. 33, 125–159. doi: 10.1146/annurev.ecolsys.33.010802.150452
Wilson, M. C., Chen, X. Y., Corlett, R. T., Didham, R. K., Ding, P., Holt, R. D., et al. (2016). Habitat fragmentation and biodiversity conservation: key findings and future challenges. Landsc. Ecol. 31, 219–227. doi: 10.1007/s10980-015-0312-3
Wright, I. J., Reich, P. B., Westoby, M., Ackerly, D. D., Baruch, Z., Bongers, F., et al. (2004). The worldwide leaf economics spectrum. Nature 428, 821–827. doi: 10.1038/nature02403
Yang, J., Cao, M., and Swenson, N. G. (2018). Why functional traits do not predict tree demographic rates. Trends Ecol. Evol. 33, 326–336.
Zambrano, J., Garzon-Lopez, C. X., Yeager, L., Fortunel, C., Cordeiro, N. J., and Beckman, N. G. (2019). The effects of habitat loss and fragmentation on plant functional traits and functional diversity: what do we know so far? Oecologia 191, 505–518. doi: 10.1007/s00442-019-04505-x
Keywords: Atlantic Forest, conservation, demography, fragmentation, functional traits, palms
Citation: Portela RCQ, Colmenares-Trejos SL and de Mattos EA (2021) Linking Plant Functional Traits to Demography in a Fragmented Landscape. Front. For. Glob. Change 4:717406. doi: 10.3389/ffgc.2021.717406
Received: 30 May 2021; Accepted: 25 October 2021;
Published: 19 November 2021.
Edited by:
Gerardo Avalos, University of Costa Rica, Costa RicaReviewed by:
Daijun Liu, University of Birmingham, United KingdomKelsey R. Carter, Los Alamos National Laboratory, United States
Ned Fetcher, Wilkes University, United States
Copyright © 2021 Portela, Colmenares-Trejos and de Mattos. This is an open-access article distributed under the terms of the Creative Commons Attribution License (CC BY). The use, distribution or reproduction in other forums is permitted, provided the original author(s) and the copyright owner(s) are credited and that the original publication in this journal is cited, in accordance with accepted academic practice. No use, distribution or reproduction is permitted which does not comply with these terms.
*Correspondence: Rita de Cássia Quitete Portela, cml0YXBvcnRlbGFAZ21haWwuY29t