- 1Department of Natural Resources & The Environment, Cornell University, Ithaca, NY, United States
- 2United States Department of Agriculture Forest Service, Northern Research Station, St. Paul, MN, United States
- 3Cary Institute of Ecosystem Studies, Millbrook, NY, United States
- 4The Nature Conservancy, Arlington, VA, United States
- 5Department of Forestry and Natural Resources, Purdue University, West Lafayette, IN, United States
Major efforts are underway to harness the carbon sequestration capacity of forests to combat global climate change. However, tree damage and death associated with insect and disease disturbance can reduce this carbon sequestration capacity. We quantified average annual changes in live tree carbon accumulation associated with insect and disease disturbances utilizing the most recent (2001 – 2019) remeasurement data from National Forest Inventory plots in the contiguous United States. Forest plots recently impacted by insect disturbance sequestered on average 69% less carbon in live trees than plots with no recent disturbance, and plots recently impacted by disease disturbance sequestered on average 28% less carbon in live trees than plots with no recent disturbance. Nationally, we estimate that carbon sequestration by live trees, defined as the estimated average annual rate of above- and belowground carbon accumulation in live trees (diameter at breast height ≥ 2.54 cm) on forest land, has been reduced by 9.33 teragrams carbon per year (95% confidence interval: 7.11 to 11.58) in forests that have experienced recent insect disturbance and 3.49 teragrams carbon per year (95% confidence interval: 1.30 to 5.70) in forests that have experienced recent disease disturbance, for a total reduction of 12.83 teragrams carbon per year (95% confidence interval: 8.41 to 17.28). Strengthened international trade policies and phytosanitary standards as well as improved forest management have the potential to protect forests and their natural capacity to contribute to climate change mitigation.
Introduction
Both reductions in greenhouse gas emissions and increases in carbon (C) sequestration and storage by natural systems are necessary to ameliorate global climate change (Edenhofer et al., 2014). The contribution of natural systems, especially forests, to cost-effective global climate change mitigation has, until recently, been underestimated and could provide as much as 37% of the near-term mitigation necessary to meet the goals of the Paris Climate Agreement (Griscom et al., 2017). In the United States (US), conservation, restoration, and improved land management could provide C sequestration equivalent to an estimated 21% of current net annual emissions (Fargione et al., 2018).
United States (US) forests currently provide an estimated 149 teragrams (Tg) in net live tree C sequestration annually (Domke et al., 2020b). Given the magnitude of the US’s live tree C sink, significant attention has been focused on whether this capacity can be increased through improved forest management (Birdsey et al., 2006), avoided deforestation (Fargione et al., 2018), and/or reforestation (Domke et al., 2020a). The US forest C sink, including soils, standing and downed wood, and live trees, may also be in jeopardy due to increasing levels of disturbance (Anderegg et al., 2015), conversion (Homer et al., 2020), and/or declining sequestration rates in old growth stands (Pugh et al., 2019).
Introduced and native insects and diseases can act solely or collectively with other forest stressors to damage or kill large numbers of trees in short periods of time, reducing a forest’s capacity to sequester C as well as increasing emissions of stored C through decomposition of wood in dead or injured trees (Ellison et al., 2005; Hicke et al., 2012; Lovett et al., 2016). Historically, native and introduced forest insects and diseases have impacted an average of 20.4 million hectares, or approximately 15% of the US’s total forest cover, annually (Dale et al., 2001). An estimated 41% of the live forest biomass in the contiguous US could be impacted by the fifteen most damaging non-native insects and diseases already established in the US (Fei et al., 2019). Both native and non-native insects and diseases are expected to exhibit increased impacts in response to climate change, potentially creating a positive climate feedback loop (Ayres and Lombarderó, 2000). In the face of more frequent and severe forest disturbance, efforts to harness or improve the capacity of forests to reduce atmospheric C levels could be rendered ineffective (Hicke et al., 2012).
Forest stand productivity will typically decline immediately after insect and disease outbreaks and can remain at reduced levels following substantial tree mortality (Romme et al., 1986; Crowley et al., 2016). Tree injury or death increases space and resource availability for surviving plants, with opportunistic plant species typically filling resulting gap openings (Eschtruth et al., 2006). Productivity may eventually recover as plants recolonize, or even increase beyond pre-outbreak levels if replacement species are especially fast-growing (Crowley et al., 2016). Longer-term C sequestration capacity and storage can increase or decrease depending on the productivity and maximum achieved biomass of replacement plant species as well as the soil characteristics they engender (Flower and Gonzalez-Meler, 2015; Crowley et al., 2016). However, when the prevalence of disturbance across a landscape is elevated, such as when forests experience frequent insect and disease outbreaks, standing tree C stocks and sequestration rates can be reduced for extended periods of time (Busby and Canham, 2011). When C emissions from decomposition outweigh C sequestration rates by live plants, forests can even temporarily convert from net C sinks into net C sources (Kurz et al., 2008). Evaluating impacts to both live tree biomass and live tree C sequestration capacity is necessary for understanding the role of disturbance in forest C dynamics.
Here we quantify the extent to which insect and disease disturbances are influencing live tree C sequestration capacity through the analysis of temporal changes in C stocks in aboveground and belowground live tree biomass (hereinafter referred to as “live tree C”) in forests of the contiguous US. We use remeasurement data from US National Forest Inventory (NFI) plots with minimal to no evidence of anthropogenic disturbance (i.e., timber harvest) to test the hypothesis that in forests of the contiguous US, native and non-native insect and disease disturbance is associated with reduced live tree C sequestration capacity over the most recent NFI remeasurement interval. For this analysis, we define C sequestration capacity as the estimated average annual rate of above- and belowground C accumulation in live trees [diameter at breast height [dbh] ≥ 2.54 cm] on forest land, where nearly 97% of the carbon in live tree forest biomass occurs (Domke et al., 2020b). Our assessment is the first to evaluate the impacts of insect and disease disturbance on forest C sequestration capacity by quantifying the resulting average annual change in live tree C accumulation across the contiguous US.
Materials and Methods
Data Sources and Descriptions
The NFI employs a multi-phase inventory. First, current aerial imagery is used in a pre-screening process to classify land use at all plot locations. Next, each plot location is assigned to a stratum using other imagery or thematic products obtained from remote sensors. A stratum is a defined geographic area that includes plots with similar attributes; in many regions, strata are defined by predicted percent canopy cover (Bechtold and Patterson, 2005).
Permanent ground plots are distributed approximately every 2,428 hectares across the 48 contiguous states of the US. Each permanent ground plot comprises a series of four smaller fixed-radius (7.32 m) subplots spaced 36.6 m apart in a triangular arrangement with one subplot in the center. Individual plots can be delineated into separate mapped domains called conditions when site-level attributes differ between subplots. Tree- and site-level attributes such as dbh and tree height are measured at regular temporal intervals on plots that have at least one forested condition defined in the pre-screening process (Bechtold and Patterson, 2005).
The proportion of condition-level disturbance present (e.g., animal, disease, fire, geologic, human, insect, unknown, vegetation, and weather) is recorded for each NFI plot. A disturbance is recorded on NFI plots with at least one forested condition when there is evidence of mortality or damage to 25 percent of the trees or 50 percent of an individual species’ count in the condition since the last measurement, and the disturbance is at least 0.4 ha in size. Three different disturbances may be recorded per condition from most prominent to least prominent. National Forest Inventory plots that do not meet these criteria or that have no to minor observable disturbance at the time of measurement have no condition-level disturbance recorded (Bechtold and Patterson, 2005).
For this study, all forested NFI plots in the contiguous US containing trees having dbh ≥ 2.54 cm over the most recent plot remeasurement interval were considered (N = 91,723). Estimates of average annual net change in live tree C accumulation per hectare were compiled using the two most recent sequential NFI plot measurements. The number of years between measurements varied from 2.6 to 15.3 years (mean = 6.5, se = 1.8), but all measurements were recorded between 2001 and 2019.
National Forest Inventory (NFI) data are widely used to evaluate the impacts of disturbance on forest ecosystems throughout the US (Fei et al., 2019; Coulston et al., 2020; Ward et al., 2020; Burrill et al., 2021; Randolph et al., 2021). We assigned each NFI plot to a dominant disturbance type (i.e., animal, disease, fire, geologic, insect, none, unknown, vegetation, or weather) based on the greatest proportion of condition-level disturbance recorded on the plot at the most recent NFI measurement. If more than one disturbance type was recorded, the higher proportion was used to assign the dominant disturbance type. If the proportions of disturbance were within 5 percentage points of each other (i.e., too close to assign a dominant disturbance type), the plot was excluded from analysis (N = 12). Plots having less than 0.25 proportion of forest (equivalent to less than one NFI subplot) were also excluded from analysis to avoid substantial deviation from the true values of average annual change in live tree C sequestration capacity on forest land. In addition, plots having over 0.25 proportion of a cutting, other, or cutting and other silviculture treatment were excluded to restrict the analysis to the effects of non-anthropogenic disturbance. Lastly, two plots with estimates of annual net C sequestration capacity by live trees which were biologically unrealistic (i.e., over three times greater than the next highest value) were removed from the final analysis. In total, 77,901 unique NFI plots were included in our analysis of average annual change in live tree C sequestration capacity.
By assigning each NFI plot to a dominant disturbance type based on the highest proportion of condition-level disturbance present at the time of measurement, we intentionally consolidated multiple disturbance types when recorded. We note that only 157 NFI plots out of 77,901 included in our analysis had two or three disturbance types simultaneously recorded at the time of measurement, and the majority had one type that was clearly dominant based on the proportion of the plot impacted.
Statistical Analysis
We fit a linear mixed-effects model for NFI plot-level average annual change in live tree C. We first fit the full additive model using ecoprovince, dominant disturbance type assigned to the NFI plot, and amount of live tree C at the beginning of the most recent NFI remeasurement interval as fixed effects. An ecoprovince is a biogeographic unit commonly used to describe an area with similar ecological attributes (McNab et al., 2007). The amount of live tree C at the beginning of the remeasurement interval was included to account for regression of a plot’s average annual change in live tree C sequestration capacity toward the mean. Lastly, we included the Federal Information Processing System (FIPS) state and county that contained the NFI plot as a random effect. At the time of this analysis, NFI plots from the Rocky Mountain States (i.e., Arizona, Colorado, Idaho, Montana, New Mexico, Nevada, Utah, and Wyoming) did not have remeasurement data available and therefore could not be included in our analysis of average annual net change in live tree C accumulation per hectare (N = 13,415).
Starting with the full model, we evaluated the explanatory power of all competing models using Akaike Information Criterion (AIC) and selected the most parsimonious model based on minimum AIC (Burnham and Anderson, 2004). We used R version 3.6.3 (R Core Team, 2021) and the add-on package “lmerTest” (Kuznetsova et al., 2017) for the mixed model. The package “MuMIn” (Bartoń, 2020) was used for model selection.
Upon investigation of whether model assumptions had been met, minor departures from normality were observed. We attribute this, in part, to NFI’s definition of forest land which includes “land that is at least 10 percent stocked by forest trees of any size, or land formerly having such tree cover, and not currently developed for a non-forest use.” Under this definition, an area (and the NFI plots therein) can be classified as forest without having live trees present. This definition increased the number of plots that had no live tree biomass at the beginning and/or end of the remeasurement interval. However, there is no reliable way to differentiate these from plots that had zero biomass due to natural disturbance, and therefore all plots having zero live tree biomass at either the beginning or end of the remeasurement interval (except those removed for reasons previously specified) were included in the analysis. Rerunning the analysis after removing plots having zero live tree biomass at either the first or second NFI measurement had little effect on our model estimates.
To generate an estimate of the annual reduction in live tree C that is associated with insect and disease disturbance for the contiguous US, we required both an estimate of the area of forest land disturbed and the per hectare impact of disturbance on C sequestration by live trees in each ecoprovince. We used the proportion of NFI plots dominantly disturbed by insects and disease at the end of the most recent NFI plot remeasurement interval to estimate the area of forest disturbed by insects and disease in each ecoprovince. Disturbances recorded at the start of the remeasurement interval were used for the Rocky Mountain ecoprovinces since NFI plot remeasurement data were unavailable at the time of this analysis.
We then applied our model’s estimates for the effects of insect and disease disturbance on C sequestration by live trees in each ecoprovince to these estimated forest areas. However, there were some ecoprovinces where our model could not accurately generate estimates and uncertainties for the effects of insect and/or disease disturbance on C sequestration capacity by live trees. Specifically, we could not generate accurate estimates and uncertainties for the ecoprovinces where there were too few insect or disease disturbed NFI plots (N < 30). In these instances, we applied the average estimates by disturbance type for all remeasured plots in the US. These average estimates were also used for the Rocky Mountain ecoprovinces since the lack of NFI remeasurement data for these ecoprovinces precluded assessment of average annual change in live tree C related to disturbance. We summed these impacts across ecoprovinces to estimate the total annual reduction in live tree C associated with insect and disease disturbance across the contiguous US.
Results
Our most parsimonious model for NFI plot-level average annual change in live tree C, based on model selection using maximum likelihood, included the dominant disturbance type assigned to the plot, ecoprovince in which the plot is located, initial live tree C measurement on the plot, and state/county in which the plot is located as predictor variables (AIC = 359690, r2 = 0.14). The ecoprovince in which a plot is located explained the greatest amount of variation in average annual change in live tree C, followed by the state/county in which the plot is located, dominant disturbance type assigned to the plot, and the plot’s initial live tree C measurement (Tables 1,2).

Table 1. Fixed effect analysis of variance results for linear models of average annual change in live tree C sequestration capacity (Mg C ha–1 yr–1) showing predictor variables, numerator, and denominator degrees of freedom, sum of squares, mean squares, F statistics, and unadjusted P-values.
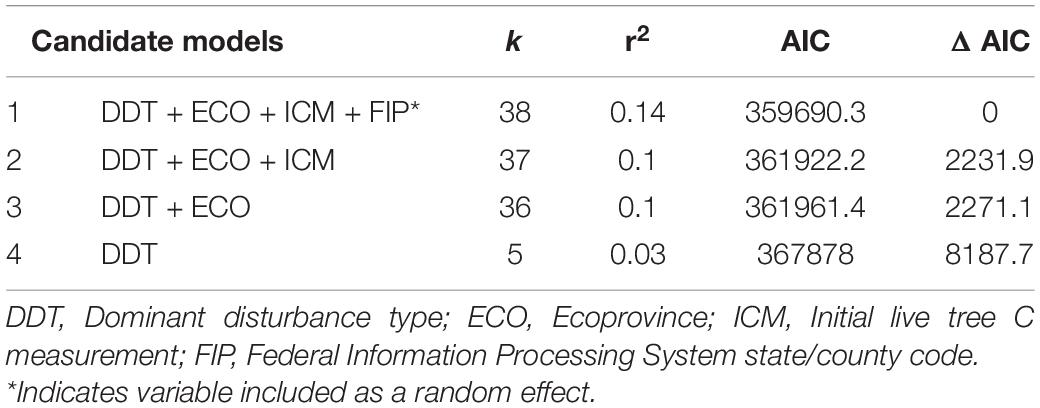
Table 2. Best-ranked linear fixed-effects and mixed-effects models examining insect and disease disturbance on average annual change in live tree C sequestration capacity (Mg C ha–1 yr–1) showing candidate models, number of parameters, coefficients of determination (adjusted r2 for fixed-effects models and conditional r2 for mixed-effects model), AIC values, and change in AIC values.
Live trees in NFI plots with recorded evidence of recent insect or disease disturbance had significantly lower annual C sequestration capacity than live trees in plots with no recent disturbance. Live trees in NFI plots with no recent recorded disturbance sequestered on average 1.44 megagrams C per hectare per year (Mg C ha–1 yr–1) [95% Confidence Interval (CI): 1.29 to 1.59] over the most recent NFI remeasurement interval, whereas live trees in plots with evidence of insect disturbance sequestered less than a third of that rate on average at 0.45 Mg C ha–1 yr–1 (95% CI: 0.36 to 0.54, t = −21.64, p < 0.001). Live trees in plots with evidence of disease disturbance sequestered slightly over two thirds of that rate on average at 1.04 Mg C ha–1 yr–1 (95% CI: 0.95 to 1.13, t = −8.86, p < 0.001).
Evidence of insect disturbance was primarily observed in ecoprovinces spanning the Northeast, Midwest, Rocky Mountains, and Pacific Northwest. Evidence of disease disturbance was primarily observed in ecoprovinces spanning New England, the Great Lakes region, Florida Panhandle, Rocky Mountains, and West Coast (Figure 1 and Supplementary Figure 1). Ecoprovinces with the greatest reductions in average annual live tree C sequestration capacity associated with recent insect disturbance included the Southeastern Mixed Forest, Outer Coastal Plain Mixed Forest, Sierran Steppe, Cascade Mixed Forest, Midwest Broadleaf Forest, and Laurentian Mixed Forest. Ecoprovinces with the greatest reductions in average annual live tree C sequestration capacity associated with recent disease disturbance included the Central Interior Broadleaf Forest, Eastern Broadleaf Forest, and Midwest Broadleaf Forest (Figures 2, 3 and Supplementary Figures 1, 2).
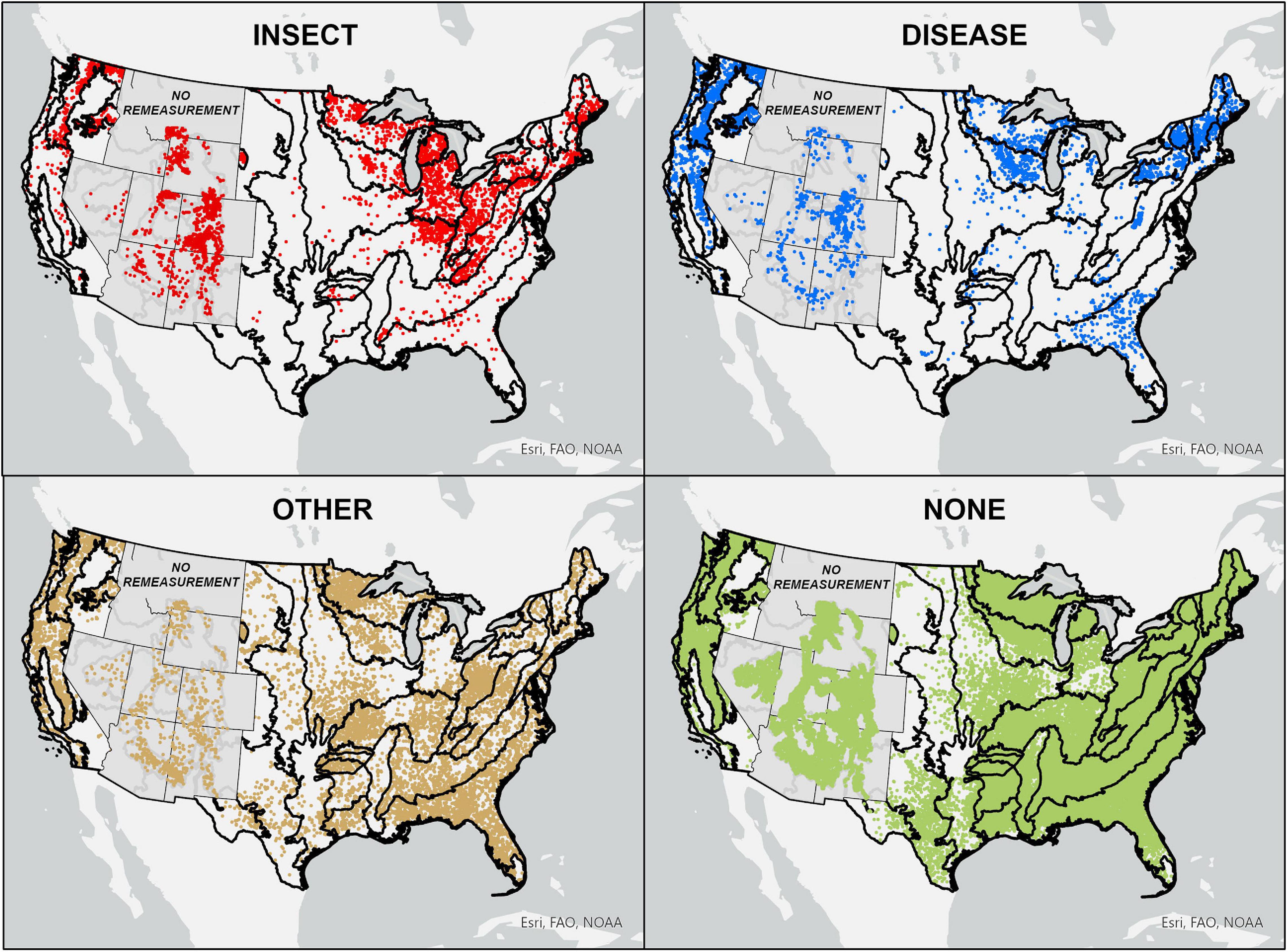
Figure 1. Approximate locations of NFI plots by dominant disturbance type assigned to each NFI plot across contiguous US. Black lines delineate ecoprovince boundaries. Grayed out states (i.e., Rocky Mountain states) contain NFI plots having only one recent live tree C measurement that prohibited their inclusion in our analysis of average annual change in live tree C sequestration capacity.
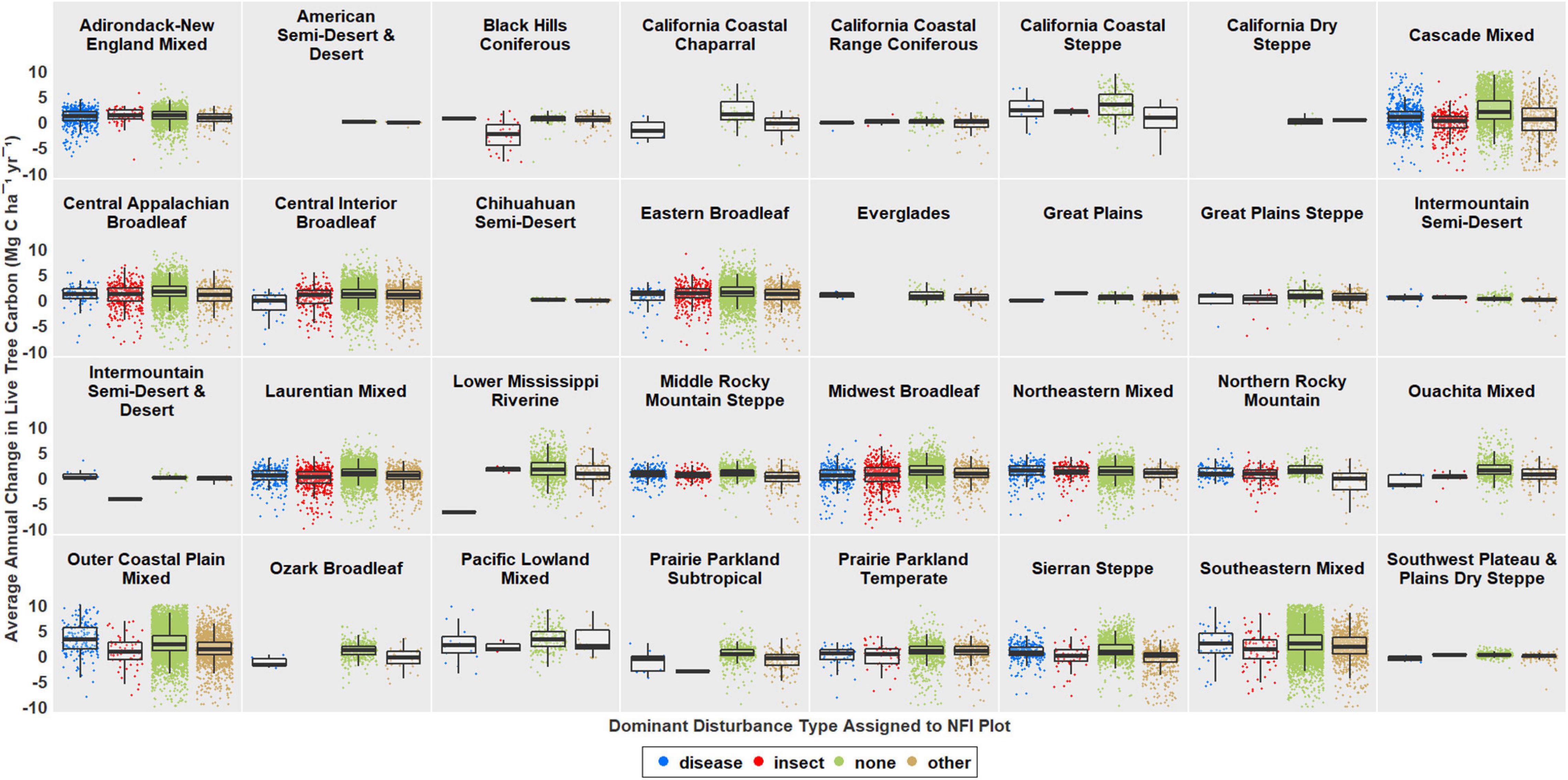
Figure 2. Box and scatter plots of average annual change in live tree C (Mg C ha–1 yr–1) by dominant disturbance type assigned to NFI plots in 32 ecoprovinces of the contiguous US. Scatterplots show average annual change in live tree C for individual NFI plots by dominant disturbance type. Box plots show medians and interquartile ranges by dominant disturbance type with error bars representing 95% confidence intervals. 693 outlier NFI plots (i.e., over three standard deviations from the mean) were removed from this figure in order to facilitate visualization of differences between dominant disturbance types.
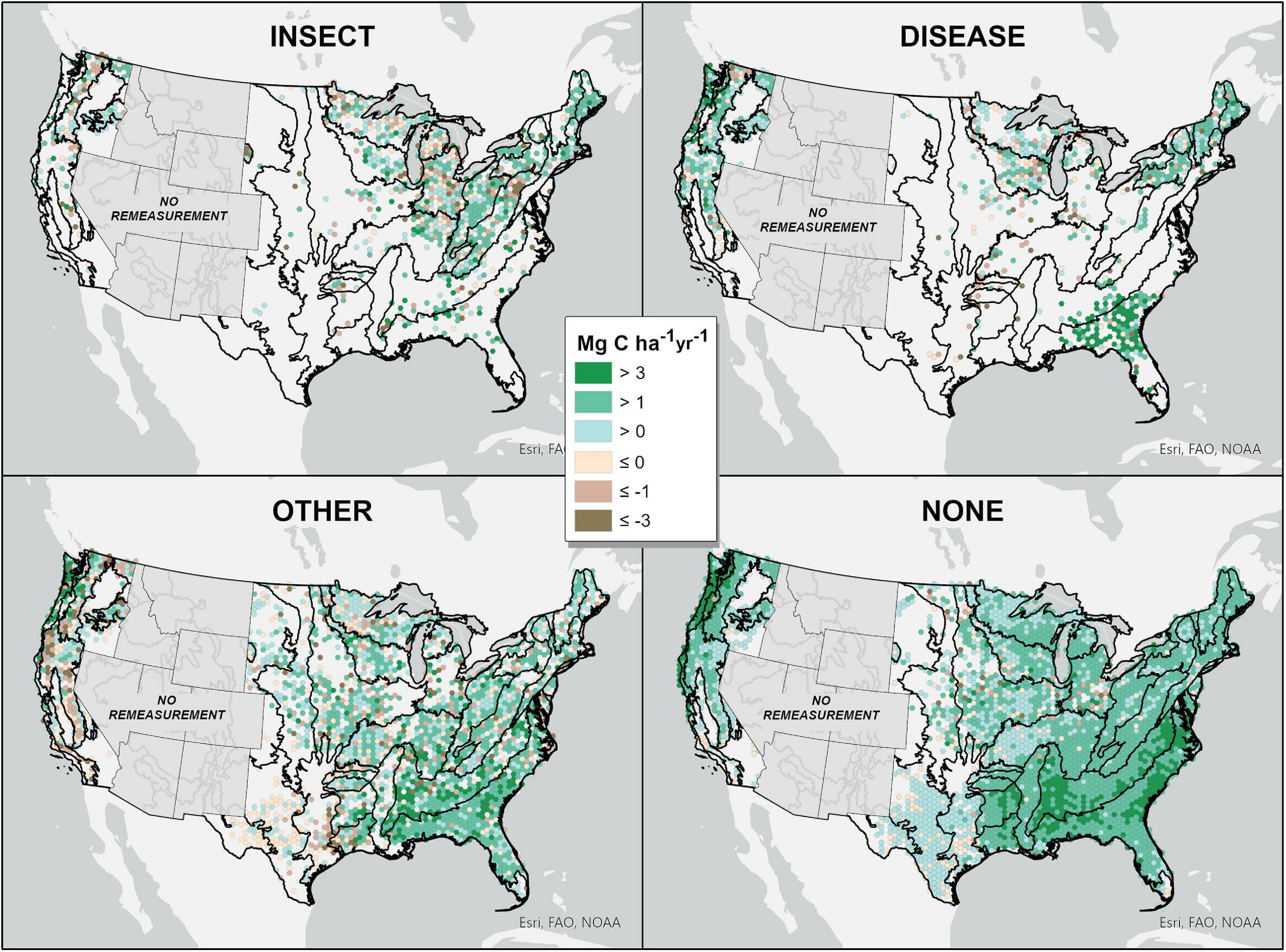
Figure 3. Average annual change in live tree C for NFI plots (aggregated by 144000 ha. hexacell) by dominant disturbance type assigned to each NFI plot across forests of the contiguous US. Map scaling and NFI plot density prohibited visualization of average annual change in live tree C sequestration capacity for individual NFI plots. To visualize trends, NFI plots have been aggregated by 144000 ha. hexacells with average annual change in live tree C values representing the mean of all NFI plots included in each hexacell. Black lines delineate ecoprovince boundaries. Grayed out states (i.e., Rocky Mountain states) contain NFI plots having only one recent live tree C measurement that prohibited their inclusion in our analysis of average annual change in live tree C.
Ecoprovinces with the greatest annual reductions in live tree C associated with insect and disease disturbance included the Southern Rocky Mountain Steppe, Cascade Mixed Forest, Midwest Broadleaf Forest, and Laurentian Mixed Forest. We estimate that live tree C sequestration capacity was reduced by 9.33 teragrams C per year (Tg C yr–1) (95% CI: 7.11 to 11.58) in forests that have experienced recent insect disturbance and 3.49 Tg C yr–1 (95% CI: 1.30 to 5.70) in forests that have experienced recent disease disturbance, for a total reduction of 12.8 Tg C yr–1 (95% CI: 8.41 to 17.28) associated with insect or disease disturbance across forests of the contiguous US over the most recent NFI remeasurement interval (Supplementary Table 1).
Discussion
Our estimated annual reduction in live tree C sequestration capacity associated with forest insect and disease disturbance (12.8 Tg C yr–1) represents approximately 9% of the contiguous US’s total annual forest C sequestration and is equivalent to the carbon dioxide emissions from over 10 million passenger vehicles driven for one year [United States Environmental Protection Agency (US EPA), 2020; Domke et al., 2020b]. Even though our results indicate that the majority of US forests continue to sequester C in live tree biomass, forests with evidence of recent insect and disease disturbance are associated with greatly reduced live tree C sequestration capacity.
These reductions should be considered conservative because the NFI only records disturbance when mortality or damage of 25% of trees or 50% of an individual tree species’ count is present at the time of remeasurement, and the disturbance affects at least 0.4 ha. This qualification of disturbance largely excludes less intense or severe disturbances associated with insects or disease, including those from which trees recovered during the remeasurement interval. For example, impacts from defoliators that do not result in severe tree injury or death are likely not accounted for, as trees will produce new foliage before the NFI plot is remeasured. Less intense or severe disturbances may have negative, neutral, or even positive effects on C sequestration by live trees (Flower and Gonzalez-Meler, 2015). We also lacked NFI remeasurement data for the Rocky Mountain states to include in our analysis of average annual change in live tree C sequestration capacity. It is unclear whether our estimates would have been significantly different if remeasurement data from the Rocky Mountain states were available since these forests are heavily impacted by insect and disease disturbance but relatively slow growing (Birdsey et al., 2019; Stanke et al., 2021).
The NFI does not differentiate between native and non-native insect and disease disturbance. Therefore, the annual reduction in live tree C sequestration capacity that we observed should be considered indicative of both native and non-native insects and diseases. Given the observed reductions in C sequestration by live trees affected by insect and disease disturbance, the introduction of additional non-native insects and diseases coupled with more severe native insect and disease outbreaks could significantly reduce the US’s forest C sequestration capacity. Improvements in preventative policies and forest management strategies could minimize further reductions to live tree C sequestration capacity and help ensure the maximum contribution of US forests to climate change mitigation.
Attempts to contain or eradicate introduced forest insects and diseases have historically been successful only in limited situations (e.g., Anoplophora chinensis) (Tobin et al., 2014). Therefore, stronger preventative regulations and their enforcement within international trade, focusing on solid wood packaging materials (SWPM) (such as pallets and crates), and live plants destined for the nursery trade, are necessary to combat the threat of non-native forest insect and disease introductions associated with increasing trade volumes (Roy et al., 2014; Lovett et al., 2016). The rate of introduction of non-native wood-boring insects could be reduced dramatically by increasing compliance with international regulations for treatment of SWPM, switching to alternative packaging materials, or requiring use of alternative packaging materials by trade partners that have repeatedly shipped infested SWPM (Lovett et al., 2016). The introduction of non-native insects and diseases on live plants could be reduced by further restricting the importation of plants for horticultural use (Roy et al., 2014), especially those that are congeneric with native woody plants in North America, as these congeners are most likely to transmit non-native insects and diseases to native tree species (Lovett et al., 2016).
However, even the most stringent preventative policies and enforcement measures will not eliminate the risk of new non-native forest insect and disease introductions, necessitating increased investment and research into improved methods for inspection of incoming SWPM and live plants, early detection of new outbreaks, and strategic rapid response. Expanded programs that implement and utilize new technologies and approaches such as environmental DNA, sentinel plant networks, crowdsourcing, remote sensing, etc. may improve interdiction success rates (Pontius et al., 2005; Reeser et al., 2011; Kenis et al., 2018; Brown et al., 2020). Advancements in and dedicated funding for classical biological control programs, genetic biocontrol research (i.e., sterile-release, sex ratio approaches, and gene drive), and host tree resistance breeding, are also necessary long-term management tools for non-native insects and diseases that become established and widespread (Sniezko, 2006; Kenis et al., 2017; Teem et al., 2020).
Improved forest management may also reduce impacts of both native and non-native insect and disease disturbance on forest C sequestration capacity, but management outcomes are often context- and species-dependent. Forest management that encourages mixed age and biodiverse stands has the potential to increase forest resilience as well as reduce the risk of catastrophic stand damage and/or mortality and consequent loss of C sequestration capacity (Muzika, 2017). Forest thinning can reduce damage by native bark beetles to conifers by increasing the vigor of remaining trees and enhancing their ability to produce resins, which are used as a defense against the beetles (Fettig et al., 2007; Hood et al., 2016). However, thinning has had little success in controlling non-native pests such as the emerald ash borer, for which trees have little evolved resistance (Muzika, 2017). Pre-emptive harvesting of susceptible species salvages some economic value from trees that will succumb to mortality from insect or disease disturbance but could also harm forest ecosystems directly through impacts of the harvesting operation (Foster and Orwig, 2006) and indirectly as individual trees that may exhibit resistance are removed, reducing the species’ ability to develop resistance over time.
Our results shed light on the potential impacts that insect and disease disturbances are already having on live tree C sequestration capacity, but further research is needed to better characterize these losses over longer time scales, clarify the roles of native versus non-native species, and develop mitigation strategies. Completing remeasurements of NFI plots in the Rocky Mountain states is of particular importance for full assessment of impacts associated with insect and disease disturbances on forest C sequestration across the contiguous US. Focused studies encompassing current and future ranges of native and non-native insects and diseases could clarify the influence of each on forest C dynamics. Well-designed field experiments could reveal whether the impacts of non-native insects and diseases on live tree C sequestration capacity are additive to or interact with those of native species and/or other forest stressors. Modeling exercises could evaluate which prevention and management strategies have the greatest potential for maintaining or increasing the US forest C sink.
There are several important limitations to consider when interpreting these results. First, disturbance types recorded for NFI plots do not account for climate change, some extreme weather events (e.g., high or low temperatures), or changes in atmospheric chemistry (e.g., nitrogen deposition, rising CO2 concentrations) which can greatly affect C cycling in forests. These factors can stimulate forest productivity and C sequestration in some cases or lead to higher mortality and lower productivity in others, potentially confounding the reductions in live tree C sequestration capacity estimated in this analysis (Domke et al., 2018). Second, our analysis solely focused on impacts to changes in live tree C. Extending this research to account for transfers of C from live biomass to dead organic matter, soils, and salvaged or preemptively harvested wood products will be important for holistic assessment of impacts of insect and disease disturbance on forest C stocks and dynamics (Hicke et al., 2012; Dobor et al., 2020). Thirdly, the linear mixed model used in this study assumes a linear response (i.e., increase or decrease) in the predicted rate of carbon sequestration for plots with evidence of forest insect and/or disease disturbance. There may be cases where this assumption does not hold and the relationship between initial carbon stocks and the rate of carbon sequestration is non-linear. Lastly, because remeasurement data from the Rocky Mountain states were not available at the time of this analysis, the dominant disturbance type recorded for each NFI plot within the Rocky Mountain ecoprovinces was based on disturbance recorded at the start of the most recent NFI remeasurement interval. This could have implications for our estimated national annual reduction in live tree C sequestration capacity as historic observations of insect and disease disturbance may or may not be representative of current trends.
Harnessing natural climate change mitigation globally holds great promise for maintaining warming below 2°celsius, but the maximum C sequestration capacity of forests is likely only to be realized if disturbance from both native and non-native insects and diseases is reduced. Countries currently experiencing the greatest accumulation of non-native forest insects and diseases are also trade centers and are more likely to have the necessary resources to implement effective prevention and forest management strategies. Non-native forest insects and diseases are also among the most serious and urgent near-term ecological threats to North America’s forests and trees, virtually eliminating entire species and/or genera from the continent’s forests within a matter of decades (e.g., American chestnut, Castanea dentata, American elm, Ulmus americana, and ash species, Fraxinus spp.) (Ellison et al., 2005; Lovett et al., 2016). While most newly introduced non-native insects and diseases result in limited damage, some have devastating impacts. These are disproportionately wood-boring insects which have been introduced at increasing rates in recent decades, with more expected to arrive in the near future (Aukema et al., 2011; Leung et al., 2014). Therefore, the urgent need to reduce insect and disease disturbance for forest C sequestration purposes will also protect myriad ecological, economic, and societal benefits of forests. Systematically reducing new forest insect and disease introductions in contaminated international trade (both SWPM and live plants) may be one of the most tractable steps society can take to protect forest ecosystems and combat global climate change.
Data Availability Statement
All underlying data used in the analyses in this study are available in multiple formats in the Forest Inventory and Analysis DataMart (https://apps.fs.usda.gov/fia/datamart/).
Author Contributions
BQ led all aspects of this research project from ideation and data analysis to manuscript writing and submission. GD and BW helped conceptualize data utilization approaches, prepared and provided all NFI data included in this analysis, and provided critical insights and writing contributions related to the US Department of Agriculture (USDA) Forest Service’s NFI. GL, LG, and SF provided critical insights, writing contributions, and literature review on implications for forest management and policy. JF provided critical insights, writing contributions, and literature review related to natural climate solutions for the United States. JR and KS-K assisted with project organization and figure creation as well as writing contributions related to future research needs. All authors assisted with project conceptualization as well as manuscript review and editing prior to submission.
Funding
Funding from The Nature Conservancy and USDA Forest Service supported this project. SF’s contributions were partially supported by the National Science Foundation (Grant No. 1638702). GL’s contributions were supported by the Doris Duke Charitable Foundation and the F.M. Kirby Foundation. The USDA Forest Service international programs grant covered the open access costs for this manuscript.
Conflict of Interest
The authors declare that the research was conducted in the absence of any commercial or financial relationships that could be construed as a potential conflict of interest.
Publisher’s Note
All claims expressed in this article are solely those of the authors and do not necessarily represent those of their affiliated organizations, or those of the publisher, the editors and the reviewers. Any product that may be evaluated in this article, or claim that may be made by its manufacturer, is not guaranteed or endorsed by the publisher.
Acknowledgments
We extend special gratitude to Kathleen Freeman (Geospatial Analyst – The Nature Conservancy, Florida Chapter) for her assistance with creating the maps featured in this manuscript. We also wish to thank the USDA Forest Service, Forest Inventory and Analysis Program for continuing to provide a publicly accessible, representative, and statistically robust dataset describing US forests. This research was supported, in part, by the USDA Forest Service. The findings and conclusions in this manuscript are those of the authors and should not be construed to represent any official USDA or US Government determination or policy. Lastly, we wish to thank Charles D. Canham (Forest Ecologist – Cary Institute of Ecosystem Studies) and Erika L. Mudrak (Statistical Consultant – Cornell Statistical Consulting Unit) for their thoughtful critiques and comments on the manuscript and associated analysis prior to submission.
Supplementary Material
The Supplementary Material for this article can be found online at: https://www.frontiersin.org/articles/10.3389/ffgc.2021.716582/full#supplementary-material
Supplementary Figure 1 | Ecoprovince boundaries and codes of the contiguous US. For full descriptions of these ecoprovinces see Supplementary Table 1.
Supplementary Figure 2 | Number of NFI plots within each 144000 ha. hexacell recorded as having insect, disease, other, or no disturbance at the most recent remeasurement across contiguous US. Figure 3 displayed mean average annual change in live tree C sequestration capacity for each disturbance type by 144000 ha. hexacell. Here we display the number of NFI plots included in each of those hexacells for additional information and context. Black lines delineate ecoprovince boundaries. Greyed out states (i.e., Rocky Mountain states) contain NFI plots having only one recent live tree C measurement that prohibited their inclusion in our analysis of average annual change in live tree C sequestration capacity.
Supplementary Table 1 | Forest area and disturbance characteristics of the 36 ecoprovinces of the contiguous US showing ecoprovince codes and names, number of NFI plots recorded as having insect, disease, other, or no disturbance at the most recent measurement, estimated forest area impacted by insect and disease disturbance, model estimates for average annual change in live tree C associated with insect and disease disturbance, and estimated annual reduction in live tree C associated with insect and disease disturbance with 95% confidence intervals.
References
Anderegg, W. R. L., Hicke, J. A., Fisher, R. A., Allen, C. D., Aukema, J., Bentz, B., et al. (2015). Tree mortality from drought, insects, and their interactions in a changing climate. New Phytol. 208, 674–683. doi: 10.1111/nph.13477
Aukema, J. E., Leung, B., Kovacs, K., Chivers, C., Britton, K. O., Englin, J., et al. (2011). Economic impacts of Non-Native forest insects in the continental United States. PLoS One 6:e24587. doi: 10.1371/journal.pone.0024587
Ayres, M. P., and Lombarderó, M. J. (2000). Assessing the consequences of global change for forest disturbance from herbivores and pathogens. Sci. Total Environ. 262, 263–286. doi: 10.1016/s0048-9697(00)00528-3
Bartoń, K. (2020). MuMIn package: Multi-Model Inference. R package version 1.43.17. Available online at: https://CRAN.R-project.org/package=MuMIn (accessed 21 July, 2020).
Bechtold, W. A., and Patterson, P. L. (eds). (2005). The Enhanced Forest Inventory and Analysis Program — National Sampling Design and Estimation Procedures. USDA Gen. Tech. Rep. SRS- 80. Asheville: U.S. Department of Agriculture, Forest Service, Southern Research Station. 85.
Birdsey, R., Dugan, A., Healey, S., Dante-Wood, K., Zhang, F., Chen, J., et al. (2019). Assessment of the Influence of Disturbance, Management Activities, and Environmental Factors on Carbon Stocks of United States National Forests. Fort Collins: U.S. Department of Agriculture, Forest Service, Rocky Mountain Research Station.
Birdsey, R., Pregitzer, K., and Lucier, A. (2006). Forest Carbon Management in the United States. J. Environ. Qual. 35, 1461–1469. doi: 10.2134/jeq2005.0162
Brown, N., Pérez-Sierra, A., Crow, P., and Parnell, S. (2020). The role of passive surveillance and citizen science in plant health. CABI Agric. Biosci. 1:17. doi: 10.1186/s43170-020-00016-5
Burnham, K. P., and Anderson, D. R. (2004). “Information and Likelihood Theory: A basis for Model Selection and Inference,” in Model Selection and Multimodel Inference, eds K. P. Burnham and D. R. Anderson (New York: Springer), 49–96. doi: 10.1007/978-0-387-22456-5_2
Burrill, E. A., DiTommaso, A. M., Turner, J. A., Pugh, S. A., Christensen, G., Perry, C. J., et al. (2021). FIA Database Description and User Guide for Phase 2 (version: 9.0.1). Available online at: <http://fia.fs.fed.us/library/database-documentation/>. (accessed July 21, 2021).
Busby, P. E., and Canham, C. D. (2011). An exotic insect and pathogen disease complex reduces aboveground tree biomass in temperate forests of eastern North America. Can. J. For. Res. 41, 401–411. doi: 10.1139/X10-213
Coulston, J. W., Edgar, C. B., Westfall, J. A., and Taylor, M. E. (2020). Estimation of forest disturbance from retrospective observations in a broad-scale inventory. Forests 11:1298. doi: 10.3390/f11121298
Crowley, K. F., Lovett, G. M., Arthur, M. A., and Weathers, K. C. (2016). Long-term effects of pest-induced tree species change on carbon and nitrogen cycling in northeastern U.S. forests: a modeling analysis. For. Ecol. Manage. 372, 269–290. doi: 10.1016/j.foreco.2016.03.045
Dale, V. H., Joyce, L. A., McNulty, S., Neilson, R. P., Ayres, M. P., Flannigan, M. D., et al. (2001). Climate change and forest disturbances. Bioscience 51, 723–734.
Dobor, L., Hlásny, T., Rammer, W., Zimová, S., Barka, I., and Seidl, R. (2020). Is salvage logging effectively dampening bark beetle outbreaks and preserving forest carbon stocks? J. Appl. Ecol. 57, 67–76. doi: 10.1111/1365-2664.13518
Domke, G., Williams, C. A., Birdsey, R., Coulston, J., Finzi, A., Gough, C., et al. (2018). “Chapter 9: Forests,” in Second State of the Carbon Cycle Report, eds N. Cavallaro, G. Shrestha, R. Birdsey, and M. A. Mayes (Washington: U.S. Global Change Research Program), 365–398.
Domke, G. M., Oswalt, S. N., Walters, B. F., and Morin, R. S. (2020a). Tree planting has the potential to increase carbon sequestration capacity of forests in the United States. Proc. Natl. Acad. Sci. U. S. A. 117, 24649–24651. doi: 10.1073/pnas.2010840117
Domke, G. M., Walters, B. F., Smith, J. E., Nowak, D. J., Ogle, S. M., Coulston, J. W., et al. (2020b). Greenhouse Gas Emissions and Removals from Forest Land, Woodlands, and Urban Trees in the United States, 1990-2018 Appendix 1. – National Scale Estimates for Individual States, 1990-2018. Madison: U.S. Department of Agriculture, Forest Service, Northern Research Station.
Edenhofer, O., Sokona, Y., Minx, J. C., Farahani, E., Kadner, S., Seyboth, K., et al. (2014). Mitigation of Climate Change Working Group III Contribution to the Fifth Assessment Report of the Intergovernmental Panel on Climate Change. Available online at: www.cambridge.org (accessed January 5, 2021).
Ellison, A. M., Bank, M. S., Clinton, B. D., Colburn, E. A., Elliott, K., Ford, C. R., et al. (2005). Loss of foundation species: consequences for the structure and dynamics of forested ecosystems. Front. Ecol. Environ 3, 479–486. doi: 10.2307/3868635
Eschtruth, A. K., Cleavitt, N. L., Battles, J. J., Evans, R. A., and Fahey, T. J. (2006). Vegetation dynamics in declining eastern hemlock stands: 9 years of forest response to hemlock woolly adelgid infestation. Can. J. For. Res. 36:6. doi: 10.1139/X06-050
Fargione, J. E., Bassett, S., Boucher, T., Bridgham, S. D., Conant, R. T., Cook-Patton, S. C., et al. (2018). Natural climate solutions for the United States. Sci. Adv. 4:eaat1869.
Fei, S., Morin, R. S., Oswalt, C. M., and Liebhold, A. M. (2019). Biomass losses resulting from insect and disease invasions in US forests. Proc. Natl. Acad. Sci. U. S. A. 116, 17371–17376. doi: 10.1073/pnas.1820601116
Fettig, C. J., Klepzig, K. D., Billings, R. F., Munson, A. S., Nebeker, T. E., Negrón, J. F., et al. (2007). The effectiveness of vegetation management practices for prevention and control of bark beetle infestations in coniferous forests of the western and southern United States. For. Ecol. Manage. 238, 24–53. doi: 10.1016/j.foreco.2006.10.011
Flower, C. E., and Gonzalez-Meler, M. A. (2015). Responses of temperate forest productivity to insect and pathogen disturbances. Annu. Rev. Plant Biol. 66, 547–569. doi: 10.1146/annurev-arplant-043014-115540
Foster, D. R., and Orwig, D. A. (2006). Preemptive and salvage harvesting of New England forests: when doing nothing is a viable alternative. Conserv. Biol. 20, 959–970. doi: 10.1111/j.1523-1739.2006.00495.x
Griscom, B. W., Adams, J., Ellis, P. W., Houghton, R. A., Lomax, G., Miteva, D. A., et al. (2017). Natural climate solutions. Proc. Natl. Acad. Sci. U. S. A. 114, 11645–11650. doi: 10.1073/pnas.1710465114
Hicke, J. A., Allen, C. D., Desai, A. R., Dietze, M. C., Hall, R. J., Hogg, E. H. T., et al. (2012). Effects of biotic disturbances on forest carbon cycling in the United States and Canada. Glob. Chang. Biol. 18, 7–34. doi: 10.1111/j.1365-2486.2011.02543.x
Homer, C., Dewitz, J., Jin, S., Xian, G., Costello, C., Danielson, P., et al. (2020). Conterminous United States land cover change patterns 2001–2016 from the 2016 National Land Cover Database. ISPRS J. Photogramm. Remote Sens. 162, 184–199. doi: 10.1016/j.isprsjprs.2020.02.019
Hood, S. M., Baker, S., and Sala, A. (2016). Fortifying the forest: thinning and burning increase resistance to a bark beetle outbreak and promote forest resilience. Ecol. Appl. 26, 1984–2000. doi: 10.1002/eap.1363
Kenis, M., Hurley, B. P., Hajek, A. E., and Cock, M. J. W. (2017). Classical biological control of insect pests of trees: facts and figures. Biol. Invasions 19, 3401–3417. doi: 10.1007/s10530-017-1414-4
Kenis, M., Li, H., Fan, J.-T., Courtial, B., Auger-Rozenberg, M.-A., Yart, A., et al. (2018). Sentinel nurseries to assess the phytosanitary risks from insect pests on importations of live plants. Sci. Rep. 8:11217. doi: 10.1038/s41598-018-29551-y
Kurz, W. A., Stinson, G., Rampley, G. J., Dymond, C. C., and Neilson, E. T. (2008). Risk of natural disturbances makes future contribution of Canada’s forests to the global carbon cycle highly uncertain. Proc. Natl. Acad. Sci. U. S. A. 105, 1551–1555. doi: 10.1073/pnas.0708133105
Kuznetsova, A., Brockhoff, P. B., and Christensen, R. H. B. (2017). lmerTest Package: tests in Linear Mixed Effects Models. J. Stat. Softw. 82, 1–26. doi: 10.18637/jss.v082.i13
Leung, B., Springborn, M. R., Turner, J. A., and Brockerhoff, E. G. (2014). Pathway-level risk analysis: the net present value of an invasive species policy in the US. Front. Ecol. Environ. 12:273–279. doi: 10.1890/130311
Lovett, G. M., Weiss, M., Liebhold, A. M., Holmes, T. P., Leung, B., Lambert, K. F., et al. (2016). Nonnative forest insects and pathogens in the United States: impacts and policy options. Ecol. Appl. 26, 1437–1455. doi: 10.1890/15-1176
McNab, C. W., Cleland, D. T., Freeouf, J. A., Keys, J. E., Nowacki, G. J., et al. (2007). United States Department of Agriculture Forest Service Description of “Ecological Subregions: Sections of the Conterminous United States” First Approximation. Washington: USDA Forest Service.
Muzika, R. M. (2017). Opportunities for silviculture in management and restoration of forests affected by invasive species. Biol. Invasions 19, 3419–3435. doi: 10.1007/s10530-017-1549-3
Pontius, J., Hallett, R., and Martin, M. (2005). Using AVIRIS to assess hemlock abundance and early decline in the Catskills, New York. Remote Sens. Environ. 97, 163–173. doi: 10.1016/j.rse.2005.04.011
Pugh, T. A. M. M., Lindeskog, M., Smith, B., Poulter, B., Arneth, A., Haverd, V., et al. (2019). Role of forest regrowth in global carbon sink dynamics. Proc. Natl. Acad. Sci. U. S. A. 116, 4382–4387. doi: 10.1073/pnas.1810512116
R Core Team. (2021). R: A language and environment for statistical computing. Austria: R Foundation for Statistical Computing.
Randolph, K. D. C., Dooley, K., Shaw, J. D., Morin, R. S., Asaro, C., and Palmer, M. M. (2021). Past and present individual-tree damage assessments of the US national forest inventory. Environ. Monit. Assess. 193, 1–18. doi: 10.1007/s10661-020-08796-z
Reeser, P., Sutton, W., and Hansen, E. (2011). Phytophthora species in tanoak trees, canopy-drip, soil, and streams in the sudden oak death epidemic area of south-western Oregon, USA. N. Z. J. For. Sci. 41S, S65–S73. doi: 10.2509/naf2013.008.007
Romme, W. H., Knight, D. H., and Yavitt, J. B. (1986). Mountain Pine Beetle Outbreaks in the Rocky Mountains: regulators of Primary Productivity? Am. Nat 127:4.
Roy, B. A., Alexander, H. M., Davidson, J., Campbell, F. T., Burdon, J. J., Sniezko, R., et al. (2014). Increasing forest loss worldwide from invasive pests requires new trade regulations. Front. Ecol. Environ. 12, 457–465. doi: 10.1890/130240
Sniezko, R. A. (2006). Resistance breeding against nonnative pathogens in forest trees-current successes in North America. Can. J. Plant Pathol. 28, 270–279.
Stanke, H., Finley, A. O., Domke, G. M., Weed, A. S., and MacFarlane, D. W. (2021). Over half of western United States’ most abundant tree species in decline. Nat. Commun. 12:451. doi: 10.1038/s41467-020-20678-z
Teem, J. L., Alphey, L., Descamps, S., Edgington, M. P., Edwards, O., Gemmell, N., et al. (2020). Genetic Biocontrol for Invasive Species. Front. Bioeng. Biotechnol. 8:452. doi: 10.3389/fbioe.2020.00452
Tobin, P. C., Kean, J. M., Suckling, D. M., McCullough, D. G., Herms, D. A., and Stringer, L. D. (2014). Determinants of successful arthropod eradication programs. Biol. Invasions 16, 401–414. doi: 10.1007/s10530-013-0529-5
United States Environmental Protection Agency (US EPA) (2020). Greenhouse Gas Equivalencies Calculator. Available online at: https://www.epa.gov/energy/greenhouse-gas-equivalencies-calculator (accessed January 6, 2021).
Keywords: insects, diseases, climate change, carbon sequestration, forest disturbance
Citation: Quirion BR, Domke GM, Walters BF, Lovett GM, Fargione JE, Greenwood L, Serbesoff-King K, Randall JM and Fei S (2021) Insect and Disease Disturbances Correlate With Reduced Carbon Sequestration in Forests of the Contiguous United States. Front. For. Glob. Change 4:716582. doi: 10.3389/ffgc.2021.716582
Received: 28 May 2021; Accepted: 31 August 2021;
Published: 01 October 2021.
Edited by:
Quinn Thomas, Virginia Tech, United StatesReviewed by:
Christopher Michael Gough, Virginia Commonwealth University, United StatesCelia Faiola, University of California, Irvine, United States
Copyright © 2021 Quirion, Domke, Walters, Lovett, Fargione, Greenwood, Serbesoff-King, Randall and Fei. This is an open-access article distributed under the terms of the Creative Commons Attribution License (CC BY). The use, distribution or reproduction in other forums is permitted, provided the original author(s) and the copyright owner(s) are credited and that the original publication in this journal is cited, in accordance with accepted academic practice. No use, distribution or reproduction is permitted which does not comply with these terms.
*Correspondence: Brendan R. Quirion, brq2@cornell.edu