- 1Laboratoire de Biologie des Ligneux et des Grandes Cultures, INRAE, Université d’Orléans, Orléans, France
- 2INRAE, UR EFNO, Domaine des Barres, Nogent-sur-Vernisson, France
- 3INRAE, URZF, Orléans, France
- 4Institut de Recherche sur la Biologie de l’Insecte, UMR 7261, CNRS – Université de Tours, Tours, France
Global change challenges the adaptive potential of forests. Large-scale alterations of forest canopies have been reported across Europe, and further modifications are expected in response to the predicted changes in drought and windstorm regimes. Since forest canopies are dynamic interfaces between atmosphere and land surface, communities of canopy-dwelling insects are at the forefront of major changes in response to both direct and indirect effects of climate change. First, we briefly introduce the factors shaping arthropod communities in the canopy of temperate forests. Second, we cover the significant impacts of a forest decline on canopy structure and functioning, and more specifically its contrasted effects on insect microhabitats, trophic resources and forest microclimates. Deleterious effects may be expected for several guilds of leaf-dwelling insects. Nonetheless, a forest decline could also lead to transient or long-lasting resource pulses for other canopy-dwelling guilds, especially saproxylic species depending on deadwood substrates and tree-related microhabitats. The novel microclimates may also become more favorable for some particular groups of insects. We pinpoint current knowledge gaps and the technological locks that should be undone to improve our understanding of the canopy biotope and biodiversity in temperate forests. We highlight the need for integrative approaches to reveal the mechanisms at play. We call for cross-scale studies and long-term collaborative research efforts, involving different disciplines such as community and disturbance ecology, plant and insect ecophysiology, and thermal ecology, to better anticipate ongoing functional and conservation issues in temperate forest ecosystems.
Introduction
Forest ecosystems worldwide are currently facing increasing levels of environmental stress, posing severe challenges to their adaptive capacity (Allen et al., 2010; Seidl et al., 2017). Biological invasions (Liebhold et al., 2017), land-use intensification (Seibold et al., 2019), and climate change (Seidl et al., 2017) lead to extended forest diebacks and declines worldwide (Allen et al., 2010). Drought and windstorms have been identified as major drivers of recent forest diebacks and declines in temperate Europe, either through their direct impacts on tree health and survival or by promoting large-scale outbreaks of opportunistic species (Carnicer et al., 2011; Sallé et al., 2014; Seidl et al., 2014, 2017; Biedermann et al., 2019; Senf et al., 2020). The frequency, intensity and spatial extent of both drought and windstorm have been increasing in Europe during the last decades, with a recent acceleration (Gregow et al., 2017; Senf et al., 2020). Damage from these disturbances are predicted to increase even further in temperate areas (Seidl et al., 2014; Samaniego et al., 2018; Spinoni et al., 2018). Consequently, temperate forest ecosystems are expected to undergo major changes in terms of structure and functioning in the near future (Senf et al., 2020).
Depending on disturbance regime, resistance, and resilience level of tree species and/or forest ecosystems, and interactions with other disturbances, the structure and functioning of trees, stands, and forests can be affected to varying degrees of severity (Brodribb et al., 2020). One of the fastest and most conspicuous modifications during forest declines and diebacks is the degradation of tree crown condition (Figure 1; Ishii et al., 2004). Droughts and windstorms can directly affect canopy structure by inducing leaf and twig shedding, and provoking branch and stem breakage (Bréda et al., 2006; Mitchell, 2013). They can also have long-lasting effects when they act as inciting factors of forest declines (Manion, 1981). Several long-term forest monitoring studies already highlight large-scale increase in defoliation in the Mediterranean area during the last decades (Carnicer et al., 2011; Senf et al., 2020), and unprecedented modifications of forest canopies will likely occur in the short-term in temperate Europe.
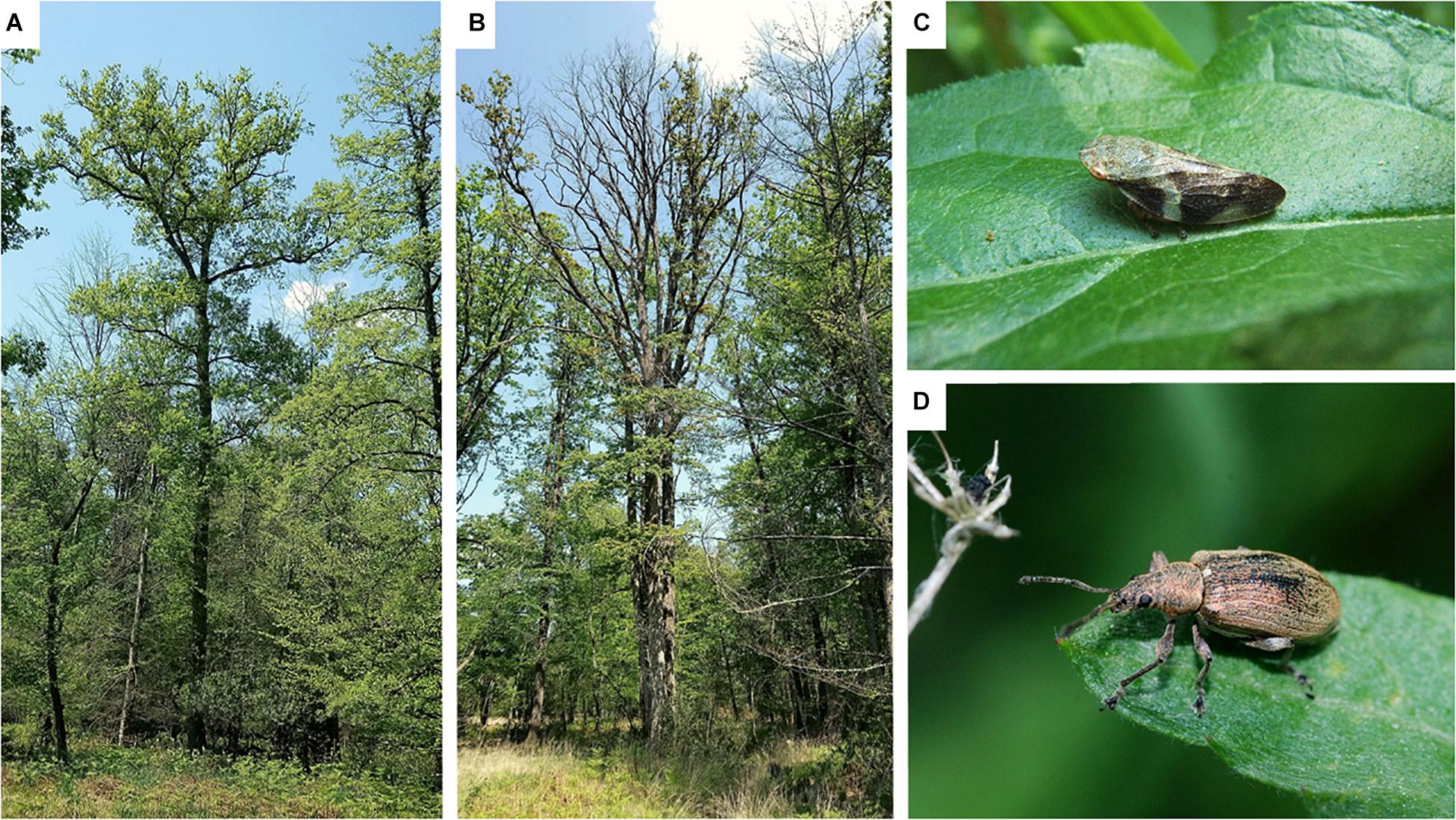
Figure 1. Oaks (Quercus petraea) exhibiting moderate (A) and pronounced (B) symptoms of decline with a reduced foliage density and an accumulation of dead branches. Oak decline will have contrasted effects on canopy-dwelling insects: negative for the sap-feeding Aphrophora alni (C), but positive for generalist leaf-feeder Phyllobius pyri (D). Photo credits: Aurélien Sallé (A,B) and Sébastien Damoiseau (C,D).
The canopy layer of temperate forests is a treasure trove of diverse plant resources, accumulating large amounts of foliage, wood, floral parts, fruits, cones, and seeds (Figure 2). At the interface with atmosphere, it is also the siege of peculiar microclimatic conditions (Leuzinger and Körner, 2007). In addition, the canopy conceals diverse but understudied arthropod communities (Floren and Schmidl, 2008; Ulyshen, 2011). Consequently, decline-driven extended change in canopy structure should dramatically alter the communities of canopy-dwelling arthropods and significantly affect forest biodiversity.
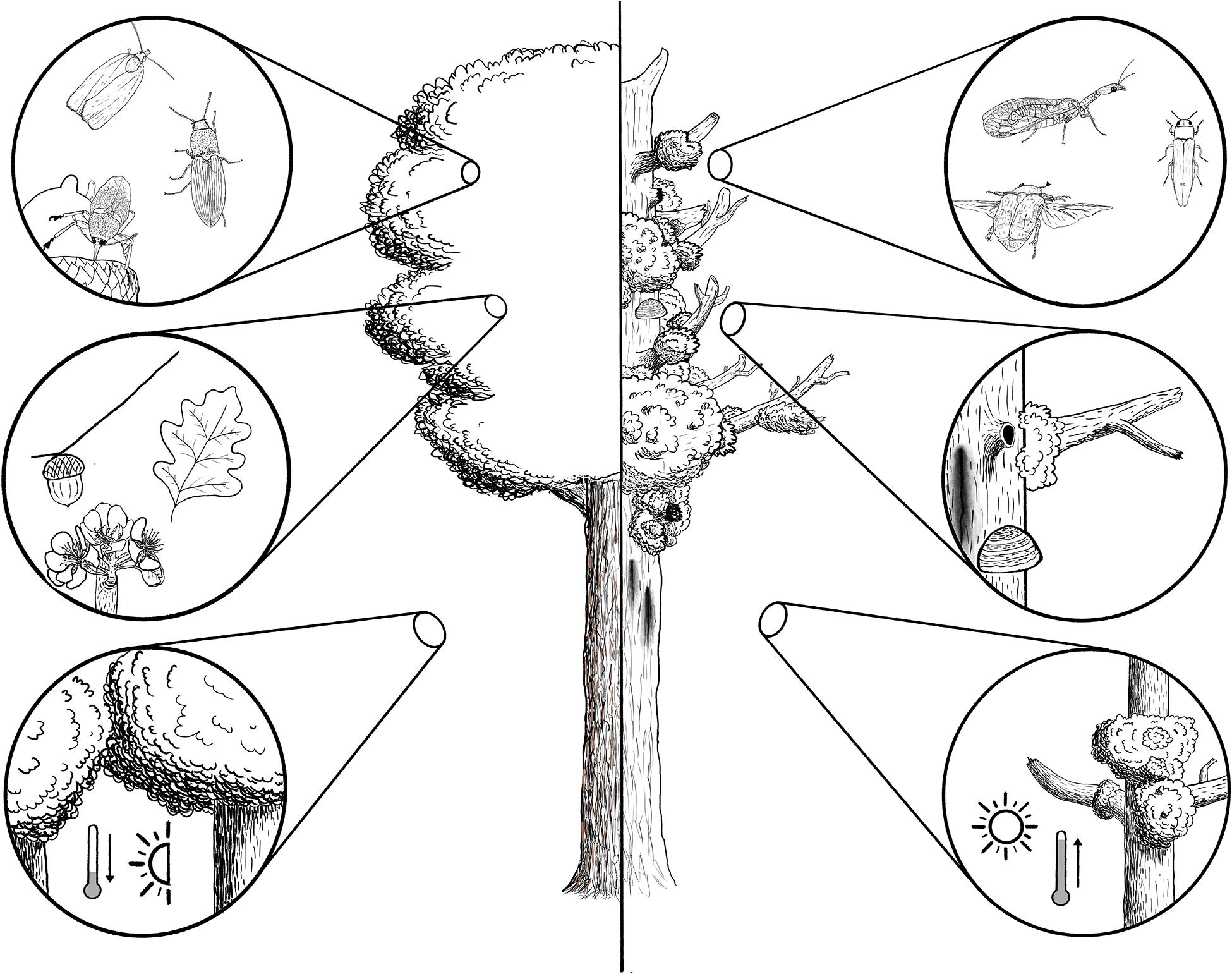
Figure 2. Overview of decline-induced changes in arthropod communities (upper insets), resources and habitats (medium insets), and microclimates (lower insets) in forest canopies. Declining tree crowns (left part) should affect leaf-dwelling species but promote saproxylic species by reducing foliage, flower, and seed amounts but accumulating tree-related microhabitats like perched dead branches, fermented sap exudates, cavities, and fruiting bodies of pathogenic fungi. Reduced canopy cover will also reduce the thermal buffer on lower forest strata but increase the luminosity. Credits: Jérémy Cours.
In this essay, we focus on the relationship between the climate-change-induced physical alterations of the canopy and arthropod communities. We briefly review (i) the factors affecting the composition and distribution of canopy-dwelling arthropods, and (ii) the consequences of forest decline on canopy-related microclimates and resources and how it affects arthropod biodiversity. We bring to the attention that a thorough understanding of the physical structure of altered canopies is needed to infer how climate change will reshape arthropod communities. Our aim is to highlight the gaps in our knowledge on the functional response of temperate forest ecosystems to global environmental change, and to stimulate cross-scale studies involving various disciplines to ameliorate predictions that should feed management plans.
Factors Shaping Arthropod Communities in the Canopy of Temperate Forests
The large amount of plant biomass in the canopy layer can support abundant arthropod populations and promote species richness according to the more-individuals hypothesis (i.e., higher energy availability promotes higher number of individuals, and higher species richness; Müller et al., 2018), or the resource-availability hypothesis (i.e., arthropod distribution reflects the availability of their resources; Wardhaugh, 2014). This large and diverse biomass may also enhance habitat heterogeneity, provide multiple trophic niches and allow the segregation of arthropod species according to the habitat heterogeneity hypothesis (i.e., species richness increases with increasing habitat heterogeneity due to greater niche dimensionality; Tanabe, 2002 but see Müller et al., 2018). The complex three-dimensional structure of tree crowns also provides ecological space of reduced predation for arthropods, known as an escape or enemy-free space (Lawton, 1983; Wardhaugh, 2014). In addition, the canopy provides a unique set of microhabitats and resources, with specific tree-related microhabitats such as mistletoe, suspended soils, epiphytes, perched dead branches, upper trunk cavities, and fruiting bodies of opportunistic fungi (Ulyshen, 2011; Larrieu et al., 2018), allowing the differentiation of dedicated habitat and/or trophic guilds of arthropods. Ultimately, this results in the establishment of complex food webs (Nakamura et al., 2017).
The temperate canopy layer is characterized by particular microclimates. For instance, the leaf surface of trees can be much warmer than local air temperature at the top of the canopy because they intercept a large amount of incoming radiation (Woods et al., 2018; Miller et al., 2021). Leaf temperature depends on the ecophysiological traits of the plant (Pincebourde and Woods, 2012), and is consequently species specific (Leuzinger and Körner, 2007). Canopy microclimates diverge largely from those of the understory. The shape and range of the vertical air temperature profile of temperate canopies depend on several variables including season, time of the day, canopy architecture, and tree species (De Frenne et al., 2021). This gradient contributes to the vertical distribution of arthropod species in the canopy, according to the physiological-efficiency hypothesis (i.e., arthropod distribution reflects their physiological tolerance to abiotic and biotic conditions, Wardhaugh, 2014). Consequently, resource availability, microhabitat richness, and abiotic conditions can be considered as critical factors affecting the abundance of arboreal arthropods (Mottl et al., 2020).
Forest stands also produce a microclimate in the understory that buffers against the atmospheric extremes for temperature and dryness (Figure 2). This buffer effect is present in all forests across latitudes and is relatively independent from tree species (De Frenne et al., 2019), suggesting that this biophysical effect relies almost entirely upon the canopy surface that absorbs or reflects incoming solar radiation. Thus, the amplitude of the buffering effect in the understory is lower when the forest canopy foliage is less dense (Zellweger et al., 2020).
Several studies underlined the vertical stratification of arthropod communities in temperate forests in response to the vertical gradient of resources, microhabitats, and microclimates (e.g., Tanabe, 2002; Bouget et al., 2011; Vodka and Cizek, 2013; Normann et al., 2016; Plewa et al., 2017; Seibold et al., 2018b; Weiss et al., 2019; Urban-Mead et al., 2021). This stratification applies to a diverse range of arthropod taxa and trophic or functional guilds (e.g., saproxylic species, leaf chewers, pollinators, and parasitoids), which results in particular species assemblages and community structure in the canopy layer (Maguire et al., 2014; Weiss et al., 2016; Šigut et al., 2018; Urban-Mead et al., 2021). Stratification patterns can markedly differ between temperate and tropical forests, but they share similar community structures (Weiss et al., 2019). Temperate forests probably shelter less insect taxa specific to the canopy than their tropical counterparts (Floren and Schmidl, 2008; Ulyshen, 2011), but up to 20–40% of saproxylic beetle species can be considered as exclusive to the canopy (Bouget et al., 2011).
In addition to canopy specialists, other species occasionally or temporarily use canopy microhabitats, as a hunting ground or to find mates for instance, and vertical migrations take place from a stratum to another, depending on the season, the stage of the life cycle, or even the time of the day (Ulyshen, 2011). As an example, several recent studies reported that even common species of scorpionflies (Mecoptera) and earwigs (Dermaptera) were quite abundant in the canopy layer while they were previously rather associated with lower forest strata (Vincent et al., 2020; Martínez-Pérez et al., 2021). Similarly, canopy top can be used by aquatic insects during the imago stage, suggesting cross-ecosystem connectivity involving the canopy layer (Le Naour et al., 2019). This underlines the fact that we still do not accurately evaluate the reliance of the overall forest arthropod community on canopy-related resources and processes.
We still have a limited knowledge of the taxonomic and functional diversity of arthropod communities dwelling in the canopy of temperate forests. Many studies have focused on saproxylic beetle communities, as key components of forest ecosystems (e.g., Bouget et al., 2011; Vodka and Cizek, 2013; Plewa et al., 2017; Seibold et al., 2018b). Other taxonomic groups and/or functional guilds have received comparatively little attention (but see Tanabe, 2002; Leksono et al., 2005; Larrivée and Buddle, 2009; Sobek et al., 2009; Maguire et al., 2014; Sallé et al., 2020; Vincent et al., 2020; Urban-Mead et al., 2021). The canopy of temperate forests may then shelter an underestimated pool of patrimonial species (Plewa et al., 2017; Tillier et al., 2020), and can be considered as a biotic frontier deserving special attention (Bouget et al., 2011).
Decline-Driven Modifications in Canopy Habitats and Cascading Effects on Arthropod Communities
Forest declines and diebacks affect key microhabitats or trophic resources for arthropod communities, through reduced foliage density and flower or seed production (Figures 1, 2; Ishii et al., 2004). For routine surveys of forest health, trees are considered in decline when more than half of their ramification is missing and/or more than half of their main branches are dead in the functional part of their canopy (Figure 1; Goudet and Nageleisen, 2019). The dynamic process of crown degradation implies the dieback of branches and twigs, progressing not only vertically from upper to lower levels but also horizontally from outer to inner parts of the tree crown (Drénou et al., 2015; Figures 1, 2). This should affect the structure and composition of arthropod communities since experimental manipulations of branch density and structure can affect the abundance and trophic webs of arboreal arthropods (Halaj et al., 2000). Foliage quality, including biochemical and morphological leaf traits, can be also affected by the loss of tree vigor (Günthardt-Goerg et al., 2013; Hu et al., 2013). Likewise, the greater exposure of leaves in opened canopies can alter their phytochemical profile, and promote the accumulation of defensive metabolites (Yamasaki and Kikuzawa, 2003; Lämke and Unsicker, 2018).
Declines and diebacks can generate novel structures and favor the build-up of features uncommon on healthy trees. They promote tree-related microhabitats associated with reduced tree vigor or decaying trees (e.g., cavities initiated by break-offs of scaffold dead branches, perched dead branches and ascending dead branches emerging from the canopy, stress-induced broom-like mass of twigs, fruiting bodies of opportunistic fungi…) (Figures 1, 2; Ojeda et al., 2007; Larrieu et al., 2018; Cours et al., 2021). Consequently, because forest declines and diebacks promote the accumulation of such biological legacies (Cours et al., 2021), they contribute to increase the structural complexity at multiple scales. The magnitude and sustainability of such changes will depend on disturbance regime on the one hand, and on stand characteristics on the other hand (e.g., Brodribb et al., 2020). In particular, tree characteristics such as resistance and tolerance to disturbance, propensity to accumulate microhabitats and decay rate of woody tissues should strongly influence the resources and habitat availability during the decline process. Foliage loss and accumulation of dead branches are frequently quantified during declines and diebacks as crown condition is generally used as proxy for tree health status (e.g., Goudet and Nageleisen, 2019). However, accurate description and quantification of tree-related microhabitats and foliage quality evolution during the entire process of tree decline is still missing.
The reduction of canopy cover negatively impacts the thermal buffering effect of the understory (Figure 2; Zellweger et al., 2020). Therefore, declines and diebacks would attenuate and even annihilate this buffer effect in the understory but we currently lack surveys to establish this link directly (Thom et al., 2020). In addition, the degradation of tree crown condition is expected to disturb considerably the vertical temperature profile from the ground to the upper canopy layer. The air temperature layers across the vertical gradient of forest canopies reflect the microclimate experienced by relatively large species. Nonetheless, the vast majority of arthropod species has a body size small enough to be influenced primarily by the microclimatic conditions at the plant surface where they live (Pincebourde and Woods, 2020; Pincebourde et al., 2021), and the influence of tree health status on leaf and bark microclimates is unknown.
The profound structural modifications affect habitat opportunities and trophic resources from micro- to macro-scales, with potentially marked cascading effects on communities of canopy-dwelling arthropods. Few studies have directly investigated how dieback or decline-induced change in canopy resources and microclimates can affect the diversity or functional structure of canopy-dwelling arthropod communities. Changes in foliage abundance and quality can markedly influence leaf-dwelling species, with contrasted effects according to their feeding guild. For instance, the abundance of sap-feeding Hemiptera and leaf-mining caterpillars or weevils can be negatively affected by degraded crown conditions (Figure 1; Martel and Mauffette, 1997; Stone et al., 2010; Sallé et al., 2020). However, studies conducted in different declining forests also showed that leaf-chewing beetles, like phyllophagous weevils, could be either promoted (in oak stands; Sallé et al., 2020) or unaffected by degraded crown conditions (in pinyon pine stands; Stone et al., 2010). Likewise leaf-chewing caterpillars were slightly promoted by maple decline (Martel and Mauffette, 1997). The accumulation of biological legacies, like perched deadwood or cavities, generally promotes saproxylic organisms following major disturbances (Beudert et al., 2015; Cours et al., 2021; Kozák et al., 2021). This stands true for canopy-dwelling arthropods as the abundance and species richness of saproxylic species is generally enhanced in the canopy of declining stands (Figure 1; Stone et al., 2010; Sallé et al., 2020; Vincent et al., 2020). These changes in sap-feeding, phyllophagous, saprophagous, and xylophagous species have in turn cascading effects on higher trophic levels and affect the community structure within the canopy (Trotter et al., 2008; Stone et al., 2010; Vincent et al., 2020).
The alteration of canopies can also alter their associated arthropod community through cascading effects on lower vegetation strata. The degree of canopy openness markedly influences the epiphyte communities of temperate forests (Nascimbene et al., 2013), and reduced epiphyte abundance have cascading effects on arboreal arthropod communities (e.g., Miller et al., 2007). Likewise, increased canopy openness markedly changes the understory and herbaceous layers (e.g., Dietz et al., 2020). This promotes floral resources and in turn affects some guilds of canopy-dwelling species, relying on below-canopy resources, such as pollinators (Davis et al., 2020; Mathis et al., 2021; but see Urban-Mead et al., 2021).
Declines and diebacks can consequently promote some particular microhabitat-, trophic-, or functional guilds of canopy-dwelling insects. In this regard, arthropod guilds can be arranged as “winners or losers,” or “victims or perpetrators” of forest declines and diebacks (Sallé and Bouget, 2020; Sire et al., 2021). Depending on disturbance severity and stand characteristics, these biodiversity pulses could be either transient or long-lasting. Nevertheless, whether and how time scale of the decline process has consequences on decline-driven dynamics of canopy-dwelling communities is still poorly known – and we propose that the difficulty to monitor upper levels of canopy forests explains this gap. This will ultimately result in both biological conservation issues and opportunities that should be urgently assessed before forest declines and diebacks further increase in Europe and other parts of the world.
The Need for Multidisciplinary Integrative Approaches Combining Cutting-Edge Tools
Monitoring canopy microhabitats and biodiversity is challenging (Nakamura et al., 2017), but monitoring the canopy of declining trees adds further challenges related to safety issues (e.g., fall of tree limbs) compromising climbing. Their crowns are degrading more or less rapidly jeopardizing the installation of permanent monitoring structures and equipment.
To circumvent these issues, either ground-based or aerial surveys should be selected for canopy access. New processes combining drone imagery, airborne LiDAR, deep learning and modeling should be developed to better assess habitat diversity and abundance in the canopy (Müller et al., 2018; Frey et al., 2020; Santopuoli et al., 2020), and microclimates (Duffy et al., 2021). This would also allow to investigate the outcomes of crown diebacks at larger spatial scales, i.e., from tree to the landscape level, which could be more relevant for stakeholders and conservation managers, but also for the study of local biodiversity (Jackson and Fahrig, 2015; Percel et al., 2019). These approaches could be combined with high throughput metabarcoding that allows to characterize arthropod communities over large spatial scales (e.g., Cai et al., 2021; Sire et al., 2021).
We also need to scale-down the investigations at the plant surfaces and organ scale. Since microclimatic conditions are the most relevant for small-bodied organisms like arthropods (Pincebourde and Woods, 2020; Pincebourde et al., 2021), high resolution surveys of microclimate at plant surfaces (leaves and wood) and within plant matrices (e.g., leaves, wood, and galls) are required to better assess the outcomes of changing microclimates across canopy on forest arthropod communities.
Novel sampling and biomonitoring techniques would help to widen the range of arthropod taxa, and guilds, studied and improve their monitoring. This could be achieved by implementing long-lasting and self-contained ecoacoustic or visual monitoring techniques for canopy-dwelling communities (e.g., Rappaport et al., 2020). Approaches based on next generation sequencing and machine-learning could also be used to infer ecological networks and measure the effect of decline of tree canopies on species interactions (Bohan et al., 2017). Developing environmental DNA surveys in canopy-related microhabitats or matrices like cavities, suspended soils, or perched dead wood would also improve our knowledge of arthropod communities in the canopy.
We call for integrated whole-tree approaches, from forest floor to canopy top and from microhabitats to landscapes, to better understand the functional and biodiversity outcomes of canopy degradation on the whole forest ecosystem. This also claims for more integrated studies linking the physiological status of trees to the physical habitats, trophic resources, and microclimatic conditions they provide and ultimately to the biodiversity they shelter. For this, a better exploration of stress-induced horizontal gradient of inner to outer microhabitats in degraded tree crown, and fine characterization of arthropod community distribution within the canopy gradients, and in the above-tree stratum, would be necessary. Several studies have investigated the impacts of forest diebacks or declines on the diversity of specific taxonomic groups or functional, habitat, or trophic guilds (e.g., Martel and Mauffette, 1997; Trotter et al., 2008; Stone et al., 2010; Beudert et al., 2015; Sallé et al., 2020; Vincent et al., 2020; Cours et al., 2021; Sire et al., 2021). Nonetheless, because of the mixed impacts highlighted by several studies, a multi-taxa and multi-guild approach would be necessary to have a holistic view of the conservation and functional outcomes of ongoing climate changes on forest arthropod communities (Seibold et al., 2018a; Swart et al., 2020). Based on this comprehensive ecological assessment, forest management and biodiversity conservation strategies, including the retention of damaged or declining trees (e.g., Kašák and Foit, 2018), shall be designed in order to take advantage of decline patterns and processes for biodiversity promotion while facilitating the forest regeneration at the same time.
Author Contributions
AS supervised the writing and wrote the first draft of the manuscript. JC and EL contributed to the writing and prepared the figures. CL-V, SP, and CB contributed to the writing. CB co-supervised the research theme with AS. All authors contributed to the article and approved the submitted version.
Funding
This work was supported by the Région Centre-Val de Loire Project no. 2018-00124136 (CANOPEE) coordinated by AS.
Conflict of Interest
The authors declare that the research was conducted in the absence of any commercial or financial relationships that could be construed as a potential conflict of interest.
Publisher’s Note
All claims expressed in this article are solely those of the authors and do not necessarily represent those of their affiliated organizations, or those of the publisher, the editors and the reviewers. Any product that may be evaluated in this article, or claim that may be made by its manufacturer, is not guaranteed or endorsed by the publisher.
References
Allen, C. D., Macalady, A. K., Chenchouni, H., Bachelet, D., McDowell, N., Vennetier, M., et al. (2010). A global overview of drought and heat-induced tree mortality reveals emerging climate change risks for forests. For. Ecol. Manage. 259, 660–684. doi: 10.1016/j.foreco.2009.09.001
Beudert, B., Bässler, C., Thorn, S., Noss, R., Schröder, B., Dieffenbach-Fries, H., et al. (2015). Bark Beetles Increase Biodiversity While Maintaining Drinking Water Quality. Conserv. Lett. 8, 272–281. doi: 10.1111/conl.12153
Biedermann, P. H., Müller, J., Grégoire, J. C., Gruppe, A., Hagge, J., Hammerbacher, A., et al. (2019). Bark beetle population dynamics in the Anthropocene: challenges and solutions. Trends Ecol. Evol. 34, 914–924. doi: 10.1016/j.tree.2019.06.002
Bohan, D. A., Vacher, C., Tamaddoni-Nezhad, A., Raybould, A., Dumbrell, A. J., and Woodward, G. (2017). Next-generation global biomonitoring: large-scale, automated reconstruction of ecological networks. Trends Ecol. Evol. 32, 477–487. doi: 10.1016/j.tree.2017.03.001
Bouget, C., Brin, A., and Brustel, H. (2011). Exploring the “last biotic frontier”: are temperate forest canopies special for saproxylic beetles? For. Ecol. Manage. 261, 211–220. doi: 10.1016/j.foreco.2010.10.007
Bréda, N., Huc, R., Granier, A., and Dreyer, E. (2006). Temperate forest trees and stands under severe drought: a review of ecophysiological responses, adaptation processes and long-term consequences. Ann. For. Sci. 63, 625–644. doi: 10.1051/forest:2006042
Brodribb, T. J., Powers, J., Cochard, H., and Choat, B. (2020). Hanging by a thread? Forests and Drought. Science 368, 261–266. doi: 10.1126/science.aat7631
Cai, W., Yang, C., Wang, X., Wu, C., Larrieu, L., Lopez-Vaamonde, C., et al. (2021). The ecological impact of pest-induced tree dieback on insect biodiversity in Yunnan pine plantations, China. For. Ecol. Manage. 491:119173. doi: 10.1016/j.foreco.2021.119173
Carnicer, J., Coll, M., Ninyerola, M., Pons, X., Sanchez, G., and Penuelas, J. (2011). Widespread crown condition decline, food web disruption, and amplified tree mortality with increased climate change-type drought. Proc. Natl. Acad. Sci. U. S. A. 108, 1474–1478. doi: 10.1073/pnas.1010070108
Cours, J., Larrieu, L., Lopez-Vaamonde, C., Müller, J., Parmain, G., Thorn, S., et al. (2021). Contrasting responses of habitat conditions and insect biodiversity to pest- or climate-induced dieback in coniferous mountain forests. For. Ecol. Manage. 482:118811. doi: 10.1016/j.foreco.2020.118811
Davis, T. S., Rhoades, P. R., Mann, A. J., and Griswold, T. (2020). Bark beetle outbreak enhances biodiversity and foraging habitat of native bees in alpine landscapes of the southern Rocky Mountains. Sci. Rep. 10:16400.
De Frenne, P., Lenoir, J., Luoto, M., Scheffers, B. R., Zellweger, F., Aalto, J., et al. (2021). Forest microclimates and climate change: importance, drivers and future research agenda. Glob. Change Biol. 27, 2279–2297. doi: 10.1111/gcb.15569
De Frenne, P., Zellweger, F., Rodríguez-Sánchez, F., Scheffers, B. R., Hylander, K., Luoto, M., et al. (2019). Global buffering of temperatures under forest canopies. Nat. Ecol. Evol. 3, 744–749. doi: 10.1038/s41559-019-0842-1
Dietz, L., Collet, C., Dupouey, J. L., Lacombe, E., Laurent, L., and Gégout, J. C. (2020). Windstorm-induced canopy openings accelerate temperate forest adaptation to global warming. Global Ecol. Biogeogr. 29, 2067–2077. doi: 10.1111/geb.13177
Drénou, C., Bouvier, M., and Lemaire, J. (2015). The diagnostic method ARCHI applied on declining pedunculate oaks. Arboric. J. 37, 166–179. doi: 10.1080/03071375.2015.1075336
Duffy, J. P., Anderson, K., Fawcett, D., Curtis, R. J., and Maclean, I. M. (2021). Drones provide spatial and volumetric data to deliver new insights into microclimate modelling. Landsc. Ecol. 36, 685–702. doi: 10.1007/s10980-020-01180-9
Floren, A., and Schmidl, J. (2008). Canopy Arthropod Research in Europe: Basic and Applied Studies From The High Frontier. Germany: Bioform Entomology and Equipment.
Frey, J., Asbeck, T., and Bauhus, J. (2020). Predicting tree-related microhabitats by multisensor close-range remote sensing structural parameters for the selection of retention elements. Remote Sens. 12:867. doi: 10.3390/rs12050867
Goudet, M., and Nageleisen, L. M. (2019). Protocole Dépéris: méthode de notation simplifiée de l’aspect du houppier des arbres forestiers dans un contexte de dépérissement. For. Entrepr. 246, 36–40.
Gregow, H., Laaksonen, A., and Alper, M. E. (2017). Increasing large scale windstorm damage in Western, Central and Northern European forests, 1951–2010. Sci. Rep. 7:46397.
Günthardt-Goerg, M. S., Kuster, T. M., Arend, M., and Vollenweider, P. (2013). Foliage response of young central European oaks to air warming, drought and soil type. Plant Biol. 15, 185–197. doi: 10.1111/j.1438-8677.2012.00665.x
Halaj, J., Ross, D. W., and Moldenke, A. R. (2000). Importance of habitat structure to the arthropod food-web in Douglas-fir canopies. Oikos 90, 139–152. doi: 10.1034/j.1600-0706.2000.900114.x
Hu, B., Simon, J., and Rennenberg, H. (2013). Drought and air warming affect the species-specific levels of stress-related foliar metabolites of three oak species on acidic and calcareous soil. Tree Physiol. 33, 489–504. doi: 10.1093/treephys/tpt025
Ishii, H. T., Tanabe, S. I., and Hiura, T. (2004). Exploring the relationships among canopy structure, stand productivity, and biodiversity of temperate forest ecosystems. For. Sci. 50, 342–355.
Jackson, H. B., and Fahrig, L. (2015). Are ecologists conducting research at the optimal scale? Global Ecol. Biogeogr. 24, 52–63. doi: 10.1111/geb.12233
Kašák, J., and Foit, J. (2018). Shortage of declining and damaged sun-exposed trees in European mountain forests limits saproxylic beetles: a case study on the endangered longhorn beetle Ropalopus ungaricus (Coleoptera: cerambycidae). J. Insect Conserv. 22, 171–181. doi: 10.1007/s10841-018-0050-3
Kozák, D., Svitok, M., Wiezik, M., Mikoláš, M., Thorn, S., Buechling, A., et al. (2021). Historical disturbances determine current taxonomic, functional and phylogenetic diversity of saproxylic beetle communities in temperate primary forests. Ecosystems 24, 37–55. doi: 10.1007/s10021-020-00502-x
Lämke, J. S., and Unsicker, S. B. (2018). Phytochemical variation in treetops: causes and consequences for tree-insect herbivore interactions. Oecologia 187, 377–388. doi: 10.1007/s00442-018-4087-5
Larrieu, L., Paillet, Y., Winter, S., Bütler, R., Kraus, D., Krumm, F., et al. (2018). Tree related microhabitats in temperate and Mediterranean European forests: a hierarchical typology for inventory standardization. Ecol. Indic. 84, 194–207. doi: 10.1016/j.ecolind.2017.08.051
Larrivée, M., and Buddle, C. M. (2009). Diversity of canopy and understorey spiders in north-temperate hardwood forests. Agric. For. Entomol. 11, 225–237. doi: 10.1111/j.1461-9563.2008.00421.x
Lawton, J. H. (1983). Plant architecture and the diversity of phytophagous insects. Ann. Rev. Entomol. 28, 23–39. doi: 10.1146/annurev.en.28.010183.000323
Le Naour, A., Baeta, R., Sansault, E., Deville, M., and Pincebourde, S. (2019). Telemetry reveals the habitat selected by immature dragonflies: implications for conservation of the threatened dragonfly Leucorrhinia caudalis (Odonata: Anisoptera). J. Insect Conserv. 23, 147–155. doi: 10.1007/s10841-018-00123-9
Leksono, A. S., Takada, K., Koji, S., Nakagoshi, N., Anggraeni, T., and Nakamura, K. (2005). Vertical and seasonal distribution of flying beetles in a suburban temperate deciduous forest collected by water pan trap. Insect Sci. 12, 199–206. doi: 10.1111/j.1005-295x.2005.00025.x
Leuzinger, S., and Körner, C. (2007). Tree species diversity affects canopy leaf temperatures in a mature temperate forest. Agric. For. Meteorol. 146, 29–37. doi: 10.1016/j.agrformet.2007.05.007
Liebhold, A. M., Brockerhoff, E. G., Kalisz, S., Nuñez, M. A., Wardle, D. A., and Wingfield, M. J. (2017). Biol. Invasions in forest ecosystems. Biol. Invasions 19, 3437–3458. doi: 10.1007/s10530-017-1458-5
Maguire, D. Y., Robert, K., Brochu, K., Larrivée, M., Buddle, C. M., and Wheeler, T. A. (2014). Vertical stratification of beetles (Coleoptera) and flies (Diptera) in temperate forest canopies. Environ. Entomol. 43, 9–17. doi: 10.1603/en13056
Martel, J., and Mauffette, Y. (1997). Lepidopteran communities in temperate deciduous forests affected by forest decline. Oikos 78, 48–56. doi: 10.2307/3545799
Martínez-Pérez, S., Borja, R., García-París, M., Galante, E., and Micó, E. (2021). Ensambles de dermápteros (Dermaptera, Forficulidae) en microhábitats saproxílicos de bosques ibéricos de quercíneas. Bol. Aso. Esp. Entomol. 45, 73–84.
Mathis, C. L., McNeil, D. J. Jr., Lee, M. R., Grozinger, C. M., King, D. I., Otto, C. R., et al. (2021). Pollinator communities vary with vegetation structure and time since management within regenerating timber harvests of the Central Appalachian Mountains. For. Ecol. Manage. 496:119373. doi: 10.1016/j.foreco.2021.119373
Miller, B. D., Carter, K. R., Reed, S. C., Wood, T. E., and Cavaleri, M. A. (2021). Only sun-lit leaves of the uppermost canopy exceed both air temperature and photosynthetic thermal optima in a wet tropical forest. Agric. For. Meteorol. 301:108347. doi: 10.1016/j.agrformet.2021.108347
Miller, K. M., Wagner, R. G., and Woods, S. A. (2007). Effect of gap harvesting on epiphytes and bark-dwelling arthropods in the Acadian forest of central Maine. Can. J. For. Res. 37, 2175–2187. doi: 10.1139/x07-022
Mitchell, S. J. (2013). Wind as a natural disturbance agent in forests: a synthesis. Forestry 86, 147–157. doi: 10.1093/forestry/cps058
Mottl, O., Fibich, P., Klimes, P., Volf, M., Tropek, R., Anderson-Teixeira, K., et al. (2020). Spatial covariance of herbivorous and predatory guilds of forest canopy arthropods along a latitudinal gradient. Ecol. Lett. 23, 1499–1510. doi: 10.1111/ele.13579
Müller, J., Brandl, R., Brändle, M., Förster, B., de Araujo, B. C., Gossner, M. M., et al. (2018). LiDAR-derived canopy structure supports the more-individuals hypothesis for arthropod diversity in temperate forests. Oikos 127, 814–824. doi: 10.1111/oik.04972
Nakamura, A., Kitching, R. L., Cao, M., Creedy, T. J., Fayle, T. M., Freiberg, M., et al. (2017). Forests and their canopies: achievements and horizons in canopy science. Trends Ecol.Evol. 32, 438–451. doi: 10.1016/j.tree.2017.02.020
Nascimbene, J., Thor, G., and Nimis, P. L. (2013). Effects of forest management on epiphytic lichens in temperate deciduous forests of Europe - A review. For. Ecol. Manage. 298, 27–38. doi: 10.1016/j.foreco.2013.03.008
Normann, C., Tscharntke, T., and Scherber, C. (2016). Interacting effects of forest stratum, edge and tree diversity on beetles. For. Ecol. Manage. 361, 421–431. doi: 10.1016/j.foreco.2015.11.002
Ojeda, V. S., Suarez, M. L., and Kitzberger, T. (2007). Crown dieback events as key processes creating cavity habitat for magellanic woodpeckers. Austral Ecol. 32, 436–445. doi: 10.1111/j.1442-9993.2007.01705.x
Percel, G., Laroche, F., and Bouget, C. (2019). The scale of saproxylic beetles response to landscape structure depends on their habitat stability. Landscape Ecol. 34, 1905–1918. doi: 10.1007/s10980-019-00857-0
Pincebourde, S., Dillon, M. E., and Woods, H. A. (2021). Body size determines the thermal coupling between insects and plant surfaces. Funct. Ecol. 35, 1424–1436. doi: 10.1111/1365-2435.13801
Pincebourde, S., and Woods, H. A. (2012). Climate uncertainty on leaf surfaces: the biophysics of leaf microclimates and their consequences for leaf-dwelling organisms. Funct. Ecol. 26, 844–853. doi: 10.1111/j.1365-2435.2012.02013.x
Pincebourde, S., and Woods, H. A. (2020). There is plenty of room at the bottom: microclimates drive insect vulnerability to climate change. Curr. Opin. Insect Sci. 41, 63–70. doi: 10.1016/j.cois.2020.07.001
Plewa, R., Jaworski, T., Hilszczański, J., and Horák, J. (2017). Investigating the biodiversity of the forest strata: the importance of vertical stratification to the activity and development of saproxylic beetles in managed temperate deciduous forests. For. Ecol. Manage. 402, 186–193. doi: 10.1016/j.foreco.2017.07.052
Rappaport, D. I., Royle, J. A., and Morton, D. C. (2020). Acoustic space occupancy: combining ecoacoustics and lidar to model biodiversity variation and detection bias across heterogeneous landscapes. Ecol. Indic. 113:106172. doi: 10.1016/j.ecolind.2020.106172
Sallé, A., and Bouget, C. (2020). Victims or perpetrators: contribution and response of insects to forest diebacks and declines. Ann. For. Sci. 77, 1–3. doi: 10.1515/9781503609600-003
Sallé, A., Nageleisen, L.-M., and Lieutier, F. (2014). Bark and wood boring insects involved in oak declines in Europe: current knowledge and future prospects in a context of climate change. For. Ecol. Manage. 328, 79–93. doi: 10.1016/j.foreco.2014.05.027
Sallé, A., Parmain, G., Nusillard, B., Pineau, X., Brousse, R., Fontaine-Guenel, T., et al. (2020). Forest decline differentially affects trophic guilds of canopy-dwelling beetles. Ann. For. Sci. 77, 1–21.
Samaniego, L., Thober, S., Kumar, R., Wanders, N., Rakovec, O., Pan, M., et al. (2018). Anthropogenic warming exacerbates European soil moisture droughts. Nat. Clim. Change 8, 421–426. doi: 10.1038/s41558-018-0138-5
Santopuoli, G., Di Febbraro, M., Maesano, M., Balsi, M., Marchetti, M., and Lasserre, B. (2020). Machine learning algorithms to predict tree-related microhabitats using airborne laser scanning. Remote Sensing 12, 1–19.
Seibold, S., Cadotte, M. W., MacIvor, J. S., Thorn, S., and Müller, J. (2018a). The necessity of multitrophic approaches in community ecology. Trends Ecol. Evol. 33, 754–764. doi: 10.1016/j.tree.2018.07.001
Seibold, S., Hagge, J., Müller, J., Gruppe, A., Brandl, R., Bässler, C., et al. (2018b). Experiments with dead wood reveal the importance of dead branches in the canopy for saproxylic beetle conservation. For. Ecol. Manage. 409, 564–570. doi: 10.1016/j.foreco.2017.11.052
Seibold, S., Gossner, M. M., Simons, N. K., Blüthgen, N., Müller, J., Ambarlı, D., et al. (2019). Arthropod decline in grasslands and forests is associated with landscape-level drivers. Nature 574, 671–674. doi: 10.1038/s41586-019-1684-3
Seidl, R., Schelhaas, M. J., Rammer, W., and Verkerk, P. J. (2014). Increasing forest disturbances in Europe and their impact on carbon storage. Nat. Clim. Change 4, 806–810. doi: 10.1038/nclimate2318
Seidl, R., Thom, D., Kautz, M., Martin-Benito, D., Peltoniemi, M., Vacchiano, G., et al. (2017). Forest disturbances under climate change. Nat. Clim. Change 7, 395–402. doi: 10.1038/nclimate3303
Senf, C., Buras, A., Zang, C. S., Rammig, A., and Seidl, R. (2020). Excess forest mortality is consistently linked to drought across Europe. Nat. Commun. 11:6200.
Šigut, M., Šigutová, H., Šipoš, J., Pyszko, P., Kotásková, N., and Drozd, P. (2018). Vertical canopy gradient shaping the stratification of leaf-chewer-parasitoid interactions in a temperate forest. Ecol. Evol. 8, 7297–7311. doi: 10.1002/ece3.4194
Sire, L., Yáñez, P. S., Cai, W., Bézier, A., Courtial, B., Cours, J., et al. (2021). Climate-induced forest dieback drives compositional change in insect communities that is concentrated amongst rare species. bioRxiv [Preprint]. doi: 10.1101/2021.04.21.440751
Sobek, S., Gossner, M. M., Scherber, C., Steffan-Dewenter, I., and Tscharntke, T. (2009). Tree diversity drives abundance and spatiotemporal β-diversity of true bugs (Heteroptera). Ecol. Entomol. 34, 772–782. doi: 10.1111/j.1365-2311.2009.01132.x
Spinoni, J., Vogt, J. V., Naumann, G., Barbosa, P., and Dosio, A. (2018). Will drought events become more frequent and severe in Europe? Int. J. Climatol. 38, 1718–1736. doi: 10.1002/joc.5291
Stone, A. C., Gehring, C. A., and Whitham, T. G. (2010). Drought negatively affects communities on a foundation tree: growth rings predict diversity. Oecologia 164, 751–761. doi: 10.1007/s00442-010-1684-3
Swart, R. C., Samways, M. J., and Roets, F. (2020). Tree canopy arthropods have idiosyncratic responses to plant ecophysiological traits in a warm temperate forest complex. Sci. Rep. 10:19905.
Tanabe, S. I. (2002). Between-forest variation in vertical stratification of drosophilid populations. Ecol. Entomol. 27, 720–731. doi: 10.1046/j.1365-2311.2002.00469.x
Thom, D., Sommerfeld, A., Sebald, J., Hagge, J., Müller, J., and Seidl, R. (2020). Effects of disturbance patterns and deadwood on the microclimate in European beech forests. Agric. For. Meteorol. 291:108066. doi: 10.1016/j.agrformet.2020.108066
Tillier, P., Vincent, A., Bouget, C., and Sallé, A. (2020). Découverte d’une nouvelle station française d’une Chrysope rare en Europe: nineta inpunctata (Reuter, 1894) (Neuroptera Chrysopidae). L’Entomologiste 76, 193–196.
Trotter, R. T., Cobb, N. S., and Whitham, T. G. (2008). Arthropod community diversity and trophic structure: a comparison between extremes of plant stress. Ecol. Entomol. 33, 1–11. doi: 10.1111/j.1365-2311.2007.00941.x
Ulyshen, M. D. (2011). Arthropod vertical stratification in temperate deciduous forests: implications for conservation-oriented management. For. Ecol. Manage. 261, 1479–1489. doi: 10.1016/j.foreco.2011.01.033
Urban-Mead, K. R., Muñiz, P., Gillung, J., Espinoza, A., Fordyce, R., and van Dyke, M. (2021). Bees in the trees: diverse spring fauna in temperate forest edge canopies. For. Ecol. Manage. 482:118903. doi: 10.1016/j.foreco.2020.118903
Vincent, A., Tillier, P., Vincent-Barbaroux, C., Bouget, C., and Sallé, A. (2020). Influence of forest decline on the abundance and diversity of Raphidioptera and Mecoptera species dwelling in oak canopies. Eur. J. Entomol. 117, 372–379. doi: 10.14411/eje.2020.041
Vodka, Š, and Cizek, L. (2013). The effects of edge-interior and understorey-canopy gradients on the distribution of saproxylic beetles in a temperate lowland forest. For. Ecol. Manage. 304, 33–41. doi: 10.1016/j.foreco.2013.04.007
Wardhaugh, C. W. (2014). The spatial and temporal distributions of arthropods in forest canopies: uniting disparate patterns with hypotheses for specialisation. Biol. Rev. 89, 1021–1041. doi: 10.1111/brv.12094
Weiss, M., Didham, R. K., Procházka, J., Schlaghamerský, J., Basset, Y., Odegaard, F., et al. (2019). Saproxylic beetles in tropical and temperate forests–A standardized comparison of vertical stratification patterns. For. Ecol. Manage. 444, 50–58. doi: 10.1016/j.foreco.2019.04.021
Weiss, M., Procházka, J., Schlaghamerský, J., and Cizek, L. (2016). Fine-scale vertical stratification and guild composition of saproxylic beetles in lowland and montane forests: similar patterns despite low faunal overlap. PLoS One 11:e0149506. doi: 10.1371/journal.pone.0149506
Woods, H. A., Saudreau, M., and Pincebourde, S. (2018). Structure is more important than physiology for estimating intracanopy distributions of leaf temperatures. Ecol. Evol. 8, 5206–5218. doi: 10.1002/ece3.4046
Yamasaki, M., and Kikuzawa, K. (2003). Temporal and spatial variations in leaf herbivory within a canopy of Fagus crenata. Oecologia 137, 226–232. doi: 10.1007/s00442-003-1337-x
Keywords: biodiversity, forest decline, drought, insect, biological conservation
Citation: Sallé A, Cours J, Le Souchu E, Lopez-Vaamonde C, Pincebourde S and Bouget C (2021) Climate Change Alters Temperate Forest Canopies and Indirectly Reshapes Arthropod Communities. Front. For. Glob. Change 4:710854. doi: 10.3389/ffgc.2021.710854
Received: 17 May 2021; Accepted: 21 July 2021;
Published: 12 August 2021.
Edited by:
Akihiro Nakamura, Xishuangbanna Tropical Botanical Garden (CAS), ChinaReviewed by:
Carl Wardhaugh, New Zealand Forest Research Institute Limited (Scion), New ZealandEstefania Mico, University of Alicante, Spain
Copyright © 2021 Sallé, Cours, Le Souchu, Lopez-Vaamonde, Pincebourde and Bouget. This is an open-access article distributed under the terms of the Creative Commons Attribution License (CC BY). The use, distribution or reproduction in other forums is permitted, provided the original author(s) and the copyright owner(s) are credited and that the original publication in this journal is cited, in accordance with accepted academic practice. No use, distribution or reproduction is permitted which does not comply with these terms.
*Correspondence: Aurélien Sallé, YXVyZWxpZW4uc2FsbGVAdW5pdi1vcmxlYW5zLmZy