- 1Programa de Pós-Graduação em Ecologia, Conservação e Manejo da Vida Silvestre, Universidade Federal de Minas Gerais, Belo Horizonte, Brazil
- 2Maram Projetos Ambientais, Ipatinga, Brazil
- 3Departamento de Biologia Geral, Centro de Ciências Biológicas e da Saúde, Universidade Estadual de Montes Claros, Montes Claros, Brazil
- 4Departamento de Genética, Ecologia e Evolução, Instituto de Ciências Biológicas, Universidade Federal de Minas Gerais, Belo Horizonte, Brazil
Insects make up the bulk of terrestrial diversity and about half of insect species are herbivores that have direct relationships with their host plants and are the basis of the entire food chain, on which wildlife and humanity depend. Some herbivorous insect traits, such as their spatio-temporal distribution, are especially relevant in the current scenario of global changes, which are more pronounced in high elevation areas, helping to improve the effectiveness of conservation actions. Here we evaluated the influence that different spatiotemporal scales have on three free-feeding herbivorous insect guilds (fluid-feeding, leaf-chewing, and xylophagous insects) in montane forest islands immersed in a grassland-dominated matrix (campo rupestre). We assessed whether species turnover or nestedness was the main component determining both spatial and temporal species composition variation (β-diversity) of the herbivorous insect community. We also checked the temporal effect on herbivorous insect guilds composition between vertical strata. We sampled herbivorous insects during two summers and two winters in 14 forest islands of different sizes and shapes in a natural mountainous fragment located in southeastern Brazil. A total of 6597 herbivorous insects representing 557 morphospecies were sampled, 290 of which were fluid-feeding, 147 leaf-chewing and 120 xylophagous insects. We found a main contribution of time scale in the organization of the herbivorous insect composition sampled in this study, mainly by turnover, with small differences among guilds. Additionally, we could see that climate determined the local variation of species, corroborating that we have a highly variable always-green system over space and time where the understory community varies less in comparison to the canopy community. Our findings suggest that long-term ecological research on herbivorous community structure in relation to climatic variation is a key element for future investigations, which can be decisive for the conservation of herbivorous insect communities. We also suggest that the effects of anthropogenic pressures must be monitored in this system, since these forest islands may serve as warming refuges in a fragmented landscape holding an invaluable diversity of species that, without these old-growth forest reservoirs, would be doomed to disappear.
Introduction
Insects make up the bulk of terrestrial diversity (Sabrosky, 1953; Chapman, 2009; Stork, 2018), being considered the most important animals in terrestrial environments (Wilson, 1987). However, recent case studies have shown drastic declines in the total biomass of different insect groups (Schuch et al., 2012; Dirzo et al., 2014; Hallmann et al., 2017; Lister and Garcia, 2018), which can lead to the loss of multiple critical ecosystem services provided by them (e.g., pollination and decomposition) (Ashton et al., 2019). Out of their vast numbers, about half are represented by herbivorous insects that have direct relationships with their host plants (Stork, 2018) and are the basis of the entire food chain, on which wildlife and humanity depend (Cardoso et al., 2020). Many feeding guilds have evolved among herbivorous insects (e.g., fluid-feeding, leaf-chewing and xylophagous insect guilds), with high variability in spatio-temporal distribution (Neves et al., 2014; Kuchenbecker and Fagundes, 2018; Macedo-Reis et al., 2019). Several works report the distinction between some different guilds and the importance of working with each one (see Novais et al., 2018; Monteiro et al., 2020). Changes in habitat characteristics, such as vegetation structure and climatic variations, determine the organization of these guilds in different ways, and changes in the herbivore community have substantial effects on biological interactions throughout the food chain (Leal et al., 2016; Novais et al., 2016, 2019). In addition, some herbivorous insect traits, such as their spatio-temporal distribution (Lewinsohn et al., 2005), are especially relevant in the current scenario of global changes that are more pronounced in high elevation areas (Janzen and Hallwachs, 2019), helping to improve the effectiveness of conservation actions (Cooke et al., 2013; Callisto et al., 2019).
Interpreting the diversity distribution patterns of different herbivorous insect feeding guilds enables us to understand the influence of distinct ecological processes structuring herbivorous insect communities (see Novotny and Weiblen, 2005; Wardhaugh, 2014). Since tropical rainforests are notoriously heterogeneous environments (Basset et al., 2012; Kitching et al., 2013), one of the obvious spatial gradients of species diversity change is related to geographic distance. Insect diversity changes in this horizontal dimension may be driven by multiple factors, including the distance among habitat patches or even the distance of these patches to a continuous forest (e.g., da Silva et al., 2019). In addition, dispersal abilities of herbivorous insects differ among guilds, which may potentially influence them to move in different ways within and among habitats (Tews et al., 2004; Macedo-Reis et al., 2019). Seasonal variability is also common in tropical environments, especially with regard to species composition (Tylianakis et al., 2005), which is affected by resource availability (Shaw, 2004; Inclán et al., 2014) and can be guild-dependent. With a few notable exceptions (see Macedo-Reis et al., 2016, 2019), temporal patterns of insect diversity that reflect seasonal climatic conditions are frequently ignored in tropical rainforests (Didham and Springate, 2003). Particularly, in seasonal environments, both spatial and temporal patterns among herbivorous insects need more attention in order for us to understand these diversity patterns.
Naturally fragmented landscapes are exceptionally adequate for evaluating patterns and mechanisms that determine the distribution of species in environments without confounding effects of human-driven fragmentation and habitat loss (Fahrig, 2017; Haddad et al., 2017). Unlike oceanic islands, which are surrounded by a matrix that is a barrier to most terrestrial fauna, forest islands have more permeable matrices that harbor an important percentage of fauna (Cook et al., 2004; Driscoll, 2005; Yekwayo et al., 2016) and provide additional resources to species (Öckinger et al., 2012). In such systems, that occur immersed in grassland-dominated ecosystems on tropical mountaintops (Streher et al., 2017), vagile organisms like wasps, bees, dung beetles and fruit-feeding butterflies can seek different resources (da Silva et al., 2019; Perillo et al., 2020; Silva et al., 2020), where habitat diversity and configuration of the landscape may elicit different responses among them (Neves et al., 2021). Although the distribution of host plants at a given scale represents food resource availability and physical structure for herbivorous insects to find shelter and breeding sites (Lewinsohn and Roslin, 2008), evidence of this relationship in forests patches at landscape scales and its effects on herbivorous insect communities is still poorly documented (Rossetti et al., 2019).
There is a substantial change in habitat conditions between understory and canopy strata (Shaw, 2004; Basset et al., 2015), and this vertical stratification is often considered to be a key factor promoting the extreme diversity of tropical forests (Oliveira and Scheffers, 2019). This is mainly because vertical stratification is associated with major shifts in abiotic conditions, highlighting the great variety in resource availability that enables greater species coexistence at small scales (Brown, 1981; Scheffers et al., 2017). In fact, herbivorous insect diversity changes in this vertical dimension may be driven by factors such as resource quantity and quality and microclimate conditions (DeVries et al., 1999; Basset et al., 2001, 2003a, 2003b; Brehm, 2007) and they usually show a clear stratification that decreases from the canopy to lower strata (Basset et al., 1992, 2003a, 2003b; Neves et al., 2014). Nevertheless, few studies have investigated whether local patterns of species diversity are related to vertical stratification of different insect feeding guilds, which may be more informative regarding the functional role of arthropods in tropical forests (but see Grimbacher and Stork, 2007; Neves et al., 2014). Furthermore, along with the increasing interest in canopy ecosystems (Nadkarni et al., 2011; Silva J. O. et al., 2017; Swart et al., 2020), here we simultaneously measured herbivorous insect species diversity in both canopy and understory environments through time to contribute to the current knowledge on the subject (DeVries, 1988; Wolda, 1992; Skvarla et al., 2021).
The joint use of multiple scales (α-, β-, and γ-diversities; Whittaker, 1960) as well as a complimentary process-focused approach to decompose β-diversity into turnover (species substitution) and nestedness (a gradient of number of species) is an adequate framework to further advance our knowledge about how communities are structured in space and time, and the underlying mechanisms driving diversity patterns (Baselga, 2010). Indeed, species turnover has consistently been reported as the main component of total β-diversity for a wide range of taxa across different ecosystems (Soininen et al., 2018), and is a recurrent feature among insect communities (Leal et al., 2016; Silva L. F. et al., 2017; Macedo-Reis et al., 2019). However, such studies are still very necessary because distribution patterns vary with the scale of observation, which can range from centimeters to kilometers and from days to years (Allan and Castillo, 2007). Thus, it is important to assess both the horizontal and vertical spatio-temporal β-diversity in order to understand the mechanisms that determine the organization of insect communities, especially in megadiverse environments such as tropical forests.
Since global change would lead to multifaceted ecosystem modifications, including shifts in species distribution ranges and diversity (Chen et al., 2011) and other unpredictable cascading effects at different levels of ecological organization (Peñuelas et al., 2013), here we aimed to explore the spatiotemporal patterns of herbivorous insect diversity (i.e., richness, abundance, and composition) in forest islands in a mountain range in eastern South America (Coelho et al., 2016). Four spatiotemporal scales were considered: three spatial scales (within Malaise traps; between strata of vegetation within forest sites; and among forest islands within sampling dates) and one temporal scale (over 4 sampling dates). We then tested (i) whether different spatiotemporal scales contribute in a different way to the diversity of three herbivorous insect guilds (i.e., fluid-feeding, leaf-chewing, and xylophagous) in the forest islands; (ii) whether the variation of species composition over four seasons (spatial and temporal β-diversity) is determined by substitution (turnover), and (iii) whether the temporal variation of species composition (temporal β-diversity) varies between the vertical strata and among guilds. Specifically, we expect that:
i Spatial scale has the main contribution to the total diversity of herbivorous insects, since the forest islands are of different sizes, shapes and distances to the nearest island or to the continuous forest (Coelho et al., 2018) and the canopy faces higher levels of UV radiation, wind speed and variations in humidity and temperature than the understory (Basset et al., 2003b);
ii Species turnover among sites is more important than nestedness in determining community organization within a region, as nestedness patterns are considerably less common to herbivorous insects in fragmented habitats (Macedo-Reis et al., 2019);
iii Canopy presents the higher variation in species composition (temporal β-diversity) for all three herbivorous insect guilds, as canopy environmental conditions and resource availability vary more than in the understory (Basset et al., 2003b).
Materials and Methods
Study Area
We conducted this study in a natural forest archipelago situated in Serra do Cipó (the southern portion of the Espinhaço Range), Minas Gerais state, southeastern Brazil (19°14′19″S, 43°31′35″W; Figure 1). The Espinhaço formation is an important mountainous formation in Brazil due to its high biodiversity and endemism (Silveira et al., 2016; Miola et al., 2020). It extends almost continuously for 1200 km from northeastern to southeastern Brazil, across two states (Bahia and Minas Gerais), and acting as a humidity barrier between two biomes, the Cerrado (Brazilian savannah) to the west and the Atlantic Forest to the east. Above 900 m. a.s.l. in this region a continuous grassland matrix called campo rupestre is predominant. In the campo rupestre matrix there are some forest islands that merge as natural patches of vegetation from mountaintops. These forest islands occur mainly at 1200 m a.s.l. and their maintenance is dependent on specific climate and soil parameters (see Coelho et al., 2016 for a review). They have a floristic composition similar to seasonal semi-deciduous forests associated with the Atlantic Forest domain (Coelho et al., 2016, 2018). The species composition includes families with wide geographical distributions, although many species are largely endemic and reveal the former isolation of this refuge, which can be called a cloud forest. Compared with lowland forests, these forest islands have a lower canopy and a greater abundance of epiphytes, with a significant representation of Araceae, Orchidaceae, Bromeliaceae, Pteridophyta, and Bryophyta. This elevated representativeness of epiphytes is a consequence of the high humidity in these formations (Whitmore, 1990; Van der Hammen, 1995; Webster, 1995). The plant families with the greatest species richness in these forest islands are Myrtaceae, Lauraceae, and Melastomataceae, followed by Fabaceae, Asteraceae, and Rubiaceae (Coelho et al., 2016). Parameters such as density, diameter, average height, richness and diversity of plant species vary conspicuously between the edge and the nuclear zones of the forest islands. The soil also differs spatially in its physicochemical characteristics and its conditions in nuclear zones are more favorable to the establishment of characteristic species of late stages of succession (Coelho et al., 2016). The climate is mesothermic (Cwb in the Köppen classification), with dry winters and rainy summers, a mean annual rainfall of 1500 mm and average annual temperature ranging from 17.4 to 19.8°C (Ferrari et al., 2016; Silveira et al., 2016).
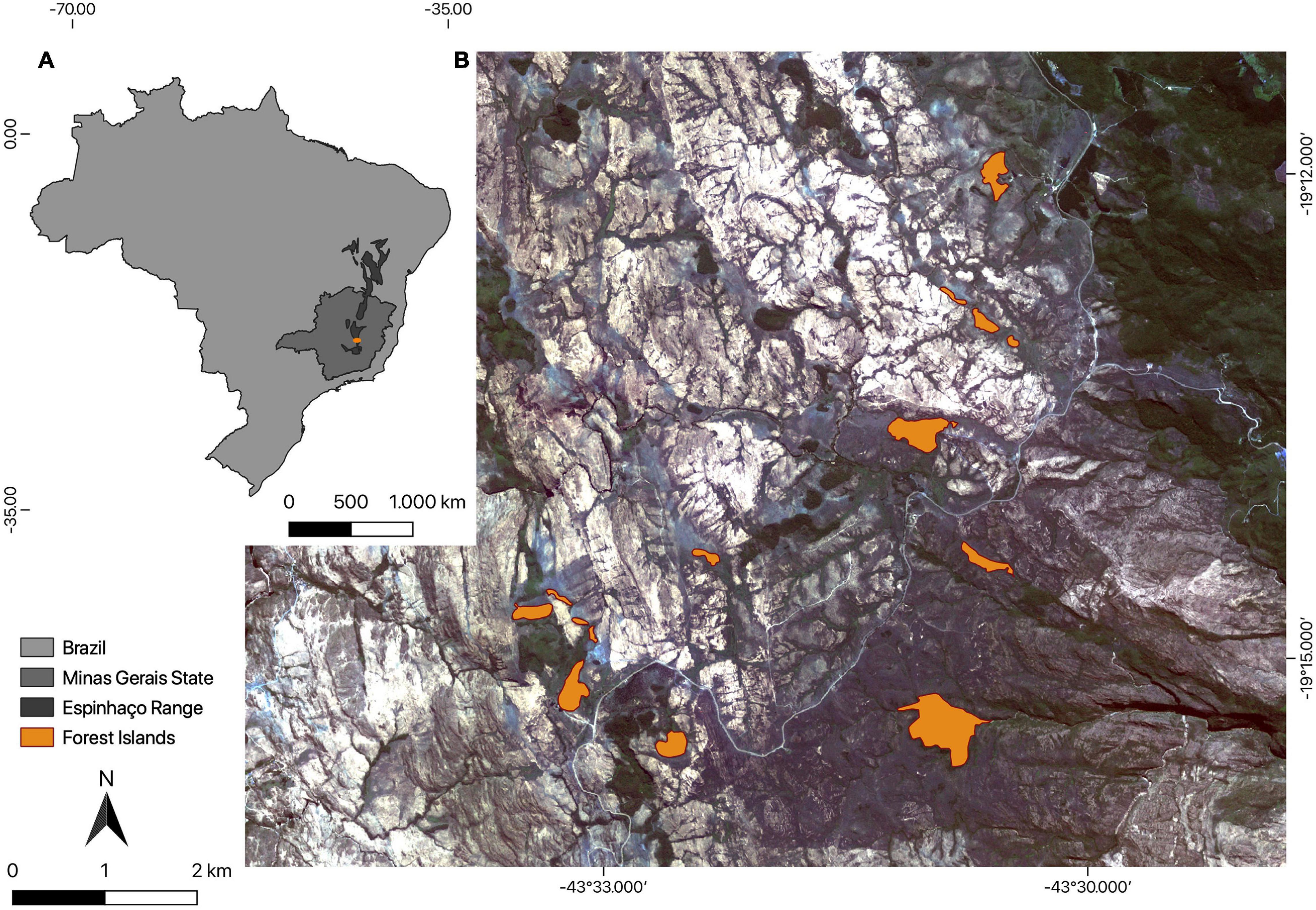
Figure 1. Map showing (A) Espinhaço Range in Brazil and (B) the 14 naturally fragmented forest islands (orange shapes) where we sampled herbivorous insects in a campo rupestre matrix of the Serra do Cipó, southern portion of the Espinhaço Range Biosphere Reserve, Minas Gerais, Brazil.
Sampling Design
A total of 14 forest islands were selected. The chosen areas vary in size (1.21–39.89 ha, mean = 8.21 ha, SD = 10.24 ha) and shape (from circular to elongated limits), and are spaced at variable distances (0.1–1.5 km) (see Supplementary Table 1 for more details). A 20 × 50 m plot was established in the center of each forest patch, where sampling was performed over two consecutive years (2014–2015) with two sampling events in the summer – rainy season (February 9–16, 2014; February 21–28, 2015) and two in the winter – dry season (August 16–23, 2014; August 22–29, 2015). In each sampling period we collected herbivorous insects using two Malaise traps (one soil Malaise trap and one canopy Malaise Window trap – mean height 4,5m ± SE 0,44 – see Supplementary Table 1 to check the heights of each Malaise window traps) located in the center of each plot, which remained in the field for seven days (9408 trap-hours for each type) (Perillo et al., 2020). These types of traps are one of the most widely used non-attractant, static insect traps in surveys of insect diversity (Noyes, 1989; Muirhead-Thomson, 1991; Southwood and Henderson, 2000; also see Skvarla et al., 2021 for a review). Collected herbivorous insects were grouped into one of three guilds according to their feeding habits: fluid-feeding, leaf-chewing or xylophagous insects (wood-associated fauna) (Macedo-Reis et al., 2019). We considered as herbivorous insects every insect belonging to families that directly or indirectly depend on plant resources for survival (Moran and Southwood, 1982; Neves et al., 2014; Macedo-Reis et al., 2019). The insects were identified using taxonomic keys and separated into morphospecies based on external morphological features. All collected insects were stored in the entomological collection of the Laboratory of Insect Ecology at the Federal University of Minas Gerais (Universidade Federal de Minas Gerais – UFMG).
Herbivorous Insect Guilds
We chose to work with exophagous insects, which are free-living organisms that move within and between plant species in search of resources. They present different life-forms (Neves et al., 2010; Araújo, 2013) and can be classified into several trophic guilds according to their feeding habit (Novotny et al., 2010), such as fluid-feeding herbivores, with a modified oral apparatus or stylet to suck plant sap (Ribeiro et al., 1994); chewing herbivores, with well-developed jaws specialized in cutting and grinding plant tissue (Raupp, 1985); and xylophagous herbivores, that breed in inner bark (dead phloem tissue; Raffa et al., 2015) and some feed on symbiotic “ambrosia” fungi that grow in the nutrient poor xylem tissue of woody plants (Gohli et al., 2017). In the case of fluid-feeding herbivores, the stylet needs to overcome the physical protective barriers of plants, such as waxes and trichomes, to access plant tissues. Thus, they are mostly affected by sap nutrient content (Price, 1997; Huberty and Denno, 2006) and tend to specialize more than chewing and xylophagous herbivores by surviving for several generations on a single host plant (Denno and Perfect, 1994; Ødegaard, 2003; Novotny et al., 2010). Chewing herbivores generally have a broad diet of host plant species and are directly influenced by leaf mechanical properties and chemistry (Peeters, 2002; Read and Sanson, 2003). They are considered to comprise the most abundant and species-rich guild (Novotny et al., 2012). Xylophagous herbivores feed on several host families with generally low host specificity (Beaver, 1979; Macedo-Reis et al., 2016) and many of them are capable of quick dispersal and establishment in new habitats (Allen and Humble, 2002; Haack, 2006). The perception of landscape structure by all these insect guilds in different habitats depends on their dispersal ability and foraging range (Weibull et al., 2000; Toivonen et al., 2017; da Silva et al., 2019), which differ among guilds due to several factors, including habitat selection and mortality risks, potentially influencing them to move within forest environments (Tews et al., 2004; Macedo-Reis et al., 2019). Also, these herbivorous insect feeding guilds may respond differently to the same habitat conditions due to their level of specialization and life history constraints (Denno and Roderick, 1991; Vehviläinen et al., 2007; Sobek et al., 2009). Thus, different feeding guilds respond to environmental characteristics differently, especially if we consider different spatial scales (Neves et al., 2013; Lourenço et al., 2015).
Data Analysis
In order to show extrapolated values of species richness to a similar number of individuals we constructed rarefaction curves using the R package “iNEXT” (Chao et al., 2014; Hsieh et al., 2016), available at https://chao.shinyapps.io/iNEXTOnline/. We accounted for every individual collected in the two Malaise traps, in all different seasons, for the three guilds of herbivorous insects. Thus, we estimated the sample completeness for each individual sampled. For extrapolation curves, the number of individuals was up to twice the actual reference size (Chao et al., 2013). We then built rarefaction curves with different sampling intensities, these curves representing the gamma diversity of each stratum per herbivorous insect guild. The sample units (i.e., the 14 forest islands) were used as the independent variable and the richness of each herbivorous insect guild was the dependent variable. Thus, we were able to obtain information about the completeness of herbivorous insect species in relation to each sampling unit. We also assessed the alpha diversity in our smallest sampling unit for each herbivorous insect guild. For this, we used the hill number 0D to show the observed accumulated species richness for our sampling units, with their respective confidence intervals. The non-overlapping of confidence intervals indicates that the insect richness found in the canopy is lower for all three guilds.
To test our hypothesis that spatial scale has a main contribution to the total diversity of herbivorous insects, we partitioned the γ-diversity (i.e., diversity in the 14 islands) into α-diversity (i.e., diversity within Malaise traps) and β-diversity (which was partitioned into β1 – between strata within forest sites; β2 – among forest islands within sampling dates and β3 – over sampling dates). We performed these analyses with the “adipart” (additive diversity partitioning) function, which works with a statistic returned by a function that is assessed according to a nested hierarchical sampling design (our three dimensions), both from the R package “vegan” (Oksanen et al., 2018). We used this function only to describe the species richness per dimension.
We assessed the spatial and temporal variations of the herbivorous species composition (β-diversity) using the Sørensen dissimilarity index in a multiple-spatial and time approach (see Baselga, 2010; Neves et al., 2021). So, we decomposed total spatial and temporal β-diversity among the forest islands (using the Sørensen and Simpson index of similarity), which we partitioned into turnover and nestedness-related components using the “beta.multi” function in the “betapart” R package (Baselga and Orme, 2012). Data were aggregated by forest sites for the spatial partition of β-diversity among sites and aggregated by sampling dates for the partition of β-diversity over sampling dates. The function ‘beta-multi’ includes the functions to compute multiple-site dissimilarities βSOR, βSIM and βNES, called beta.SOR(x), beta.SIM(x), and beta.NES(x), respectively, where x is a data frame in which sites are rows and species are columns. We used the results of this calculation to determine whether species substitution or species loss/gain influenced the general spatial pattern of temporal β-diversity (Baselga, 2010; Neves et al., 2021).
Linear mixed models (LMMs) were used to test the effect of the strata on each herbivore guild (fluid-feeding, leaf-chewing, and xylophagous) temporal β-diversity over seasonal samples, where temporal β-diversity was used as a dependent variable and the strata as an independent variable. The identity of each forest island (14 in total) was used as a random factor in LMMs, to account for spatial pseudoreplication (Bolker et al., 2009). The lme4 package and lmer function were used to perform LMM analyses (Bates et al., 2018). The function Anova of package car, which allows ANOVA in mixed models, was used to deem variables significant or not. All models were subjected to residual analyses to check for model fit and error structure suitability (Crawley, 2013). All statistical analyses were performed using R software v.4.0.
Results
General Patterns
We recorded a total of 6597 herbivorous insects belonging to 41 families, two subfamilies and 557 morphospecies (Table 1). Among these morphospecies, 290 were fluid-feeding, 147 were leaf-chewing and 120 were xylophagous insects. Among fluid-feeding herbivorous insects, Cicadellidae and Psyllidae were the richest in species (28%) and most abundant (72.9%), with the Cicadelidae family alone representing 22.1% and 69.7% of the total richness and abundance, respectively. For leaf-chewing insects, Chrysomelidae and Curculionidae were the richest in species (23.5%) and most abundant (13.4%) while for xylophagous insects, Cerambycidae, and Scolytinae were the richest in species (9.7%) and Cerambycidae and Anobiidae were the most abundant (2.1%), together representing 61.2% of the total richness and 88.4% of the total individuals sampled. There was also a high number of rarely sampled species, comprising 244 singletons (43.8% of the total) and 84 doubletons (15.1% of the total). Of these, 120 singletons and 38 doubletons belonged to fluid-feeding insects guild, 58 singletons and 26 doubletons belonged to leaf-chewing insects guild and 65 singletons and 20 doubletons belonged to xylophagous insects guild. In addition, we had a greater number of unique morphospecies collected in the understory for all guilds. A total of 398 (71.5%) morphospecies were collected exclusively in the understory (224 fluid-feeding insects; 115 leaf-chewing insects; 59 xylophagous insects), while 63 (11.3%) were collected exclusively in the canopy (18 fluid-feeding insects; eight leaf-chewing insects; 37 xylophagous insects) and 96 (17.2%) in both strata (48 fluid-feeding insects; 24 leaf-chewing insects; 24 xylophagous insects).
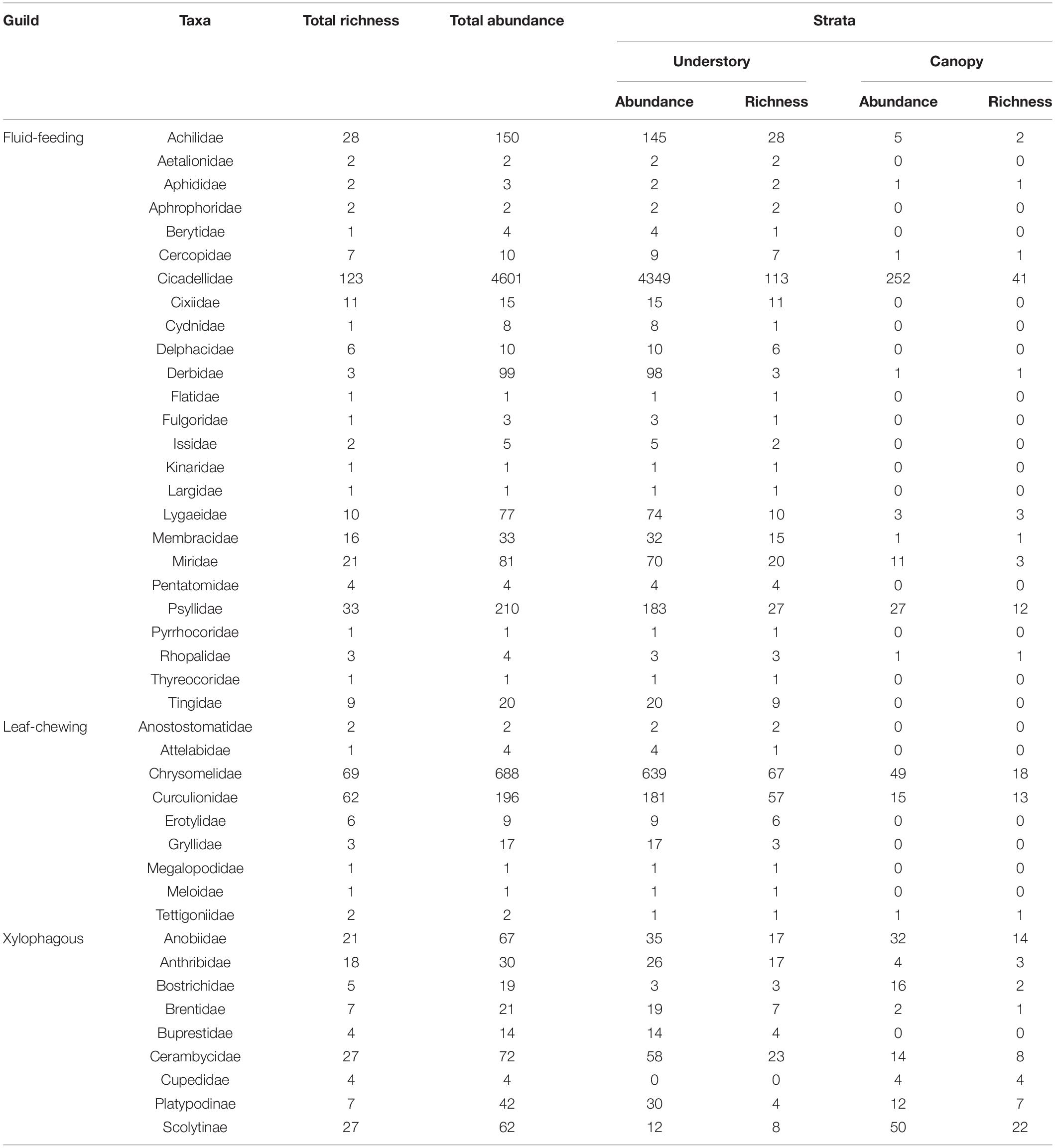
Table 1. Herbivorous insects morphospecies richness and abundance sampled in Serra do Cipó, Minas Gerais, Brazil.
The sampling effort was sufficient to characterize the insect community sampled, with the rarefaction-extrapolation accumulation curves presenting a greater sampling coverage for those carried out in the understory (Supplementary Figure 1), where the observed and extrapolated guilds richness and abundance was higher. The greatest sampling was found for fluid-feeding insects, followed by leaf-chewing insects and xylophagous insects. We observed that the fluid-feeding species richness ranged between 66 and 272 species and its total abundance ranged between 303 and 5043, which corresponds to 87% and 97% of the sampling coverage values, and 91% and 99% of the extrapolated sampling coverage abundance along the canopy and the understory, respectively (extrapolated sample coverage richness = 99 and 357 along the canopy and the understory, respectively). For the leaf-chewing insects, we observed species richness ranging between 32 and 139 species and its total abundance ranged between 65 and 855, which corresponds to 68% and 93% of the sampling coverage values, and 85% and 97% of the extrapolated sampling coverage abundance along the canopy and the understory, respectively (extrapolated sample coverage richness = 46 and 176 along the canopy and the understory, respectively). Finally, we observed that xylophagous species richness ranged between 61 and 83 species and its total abundance ranged between 134 and 197, which corresponds to 74% and 72% of the sampling coverage values, and 86% and 80% of the extrapolated sampling coverage abundance along the canopy and the understory, respectively (extrapolated sample coverage richness = 87 and 129 along the canopy and the understory, respectively). We also found that the diversity of the herbivorous insects sampled in this study was higher in the understory for all three guilds for both observed and extrapolated values (Figure 2).
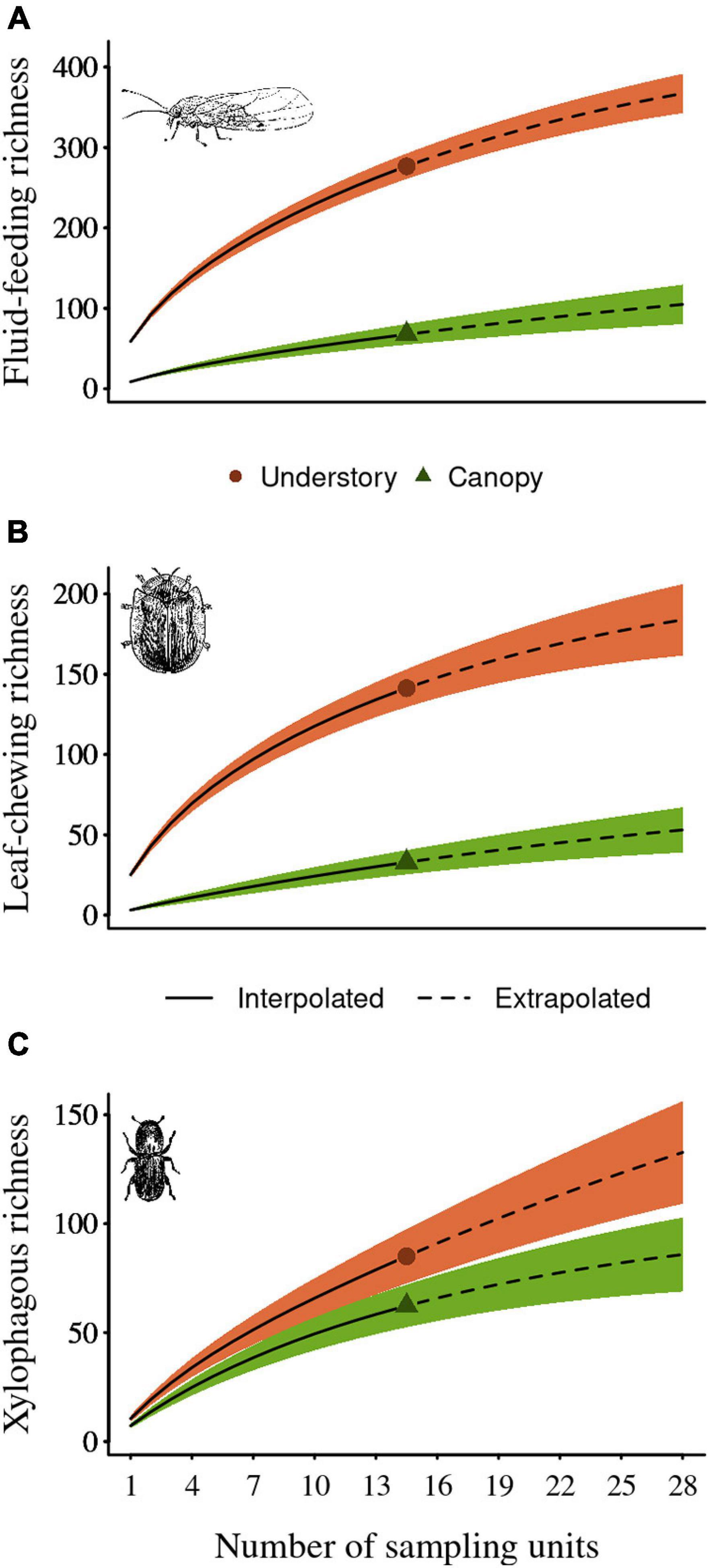
Figure 2. Species diversity curves (0D) for samples (solid lines) and extrapolated samples (dashed lines) as a function of sample size according to the count of incidences of (A) fluid feeding herbivores, (B) leaf chewing herbivores, and (C) xylophagous herbivores in each sampling unit (i.e., forest island). The bar error represents the 95 confidence interval. The red circles in the red line represent the observed samples for understory and the green triangle for the canopy, obtained by a bootstrap method based on 1000 iterations.
Partition of Diversity
We observed a main contribution of temporal scale (β3) to the total diversity when partitioning the total insect species richness for each guild into its additive components [i.e., within Malaise traps (α), between strata within forest sites (β1), among forest islands within sampling dates (β2), and over sampling dates (β3)] (Figure 3). On the other hand, we found a smaller contribution of spatial scale between strata (β1) to the total diversity for each insect guild. The observed β3 over sampling dates was slightly greater for xylophagous insects (86.49%) than for fluid-feeding (79.90%) and leaf-chewing insects (83.43%). In contrast, the contribution of α, β1 and β2 was, respectively, only 7.45%, 5.17%, and 7.48% for fluid-feeding insects, 6.19%, 3.38%, and 7% for leaf-chewing insects and 3.95%, 3.25%, and 6.31% for xylophagous insects. When we decomposed spatial and temporal β-diversity into its turnover and nestedness components, we verified that it was mostly represented by the turnover (βSIM) component in all cases. This component explained more than 75% of the total β-diversity for all guilds and strata (Table 2).
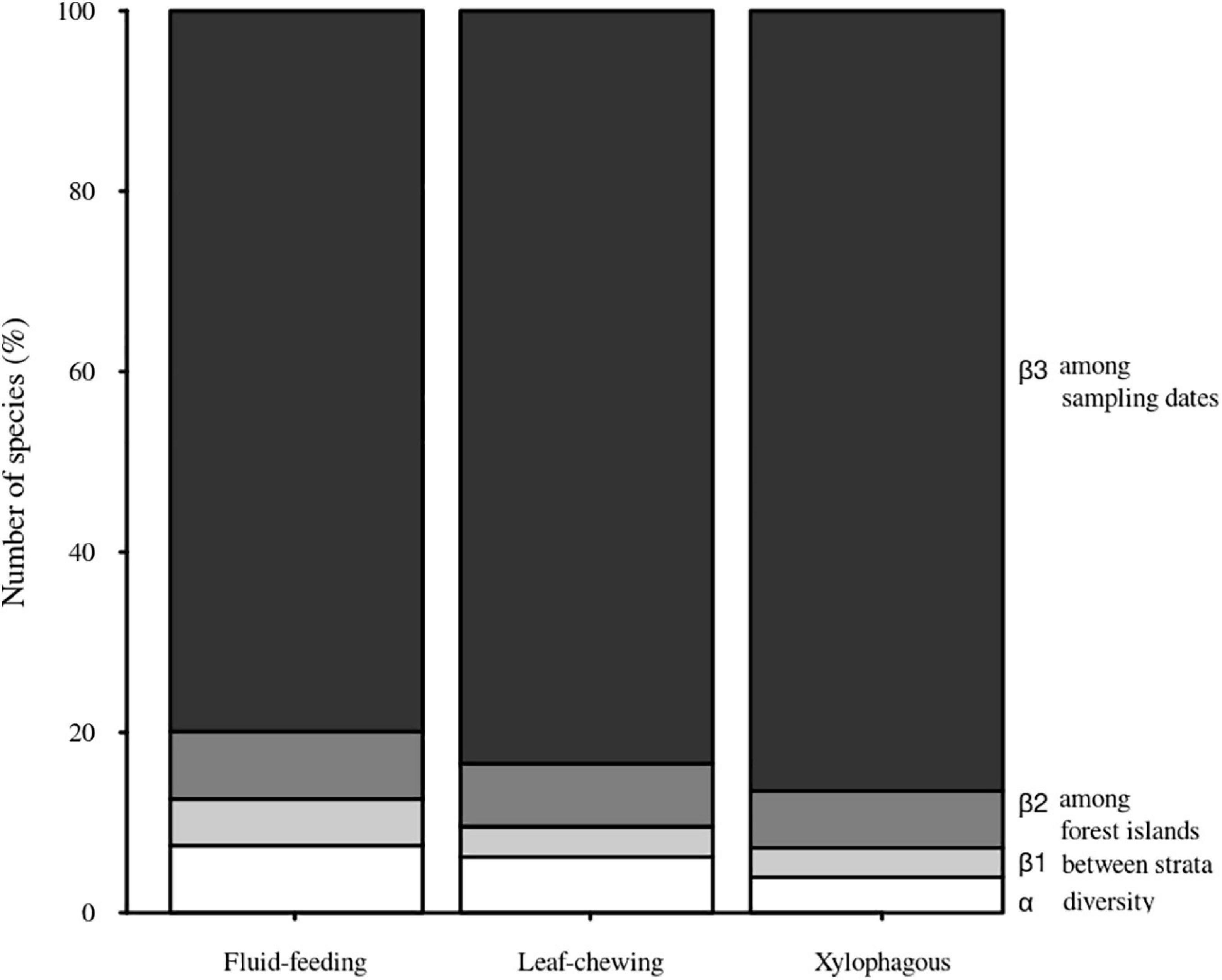
Figure 3. Contribution of the diversity of Malaise traps (α), between strata within forest sites (β1), among forest islands within sampling dates (β2) and over sampling dates (β3) to the total diversity (γ) of herbivorous insects sampled in Serra do Cipó, Minas Gerais, Brazil.

Table 2. β-Diversity using the Sørensen index for herbivorous insects collected in both strata and the importance (%) of turnover (βSIM/βSOR) for each guild at each stratum.
The species composition over time (Temporal β-diversity/βSOR) varied between vegetation strata for fluid-feeding (F = 54.12; Df = 1; p < 0.001) and leaf-chewing insects (F = 20.16; Df = 1; p < 0.001), with higher values for the canopy (Figure 4). Temporal β-diversity of the xylophagous insects did not show variation between strata (F = 1.27; Df = 1; p = 0.27).
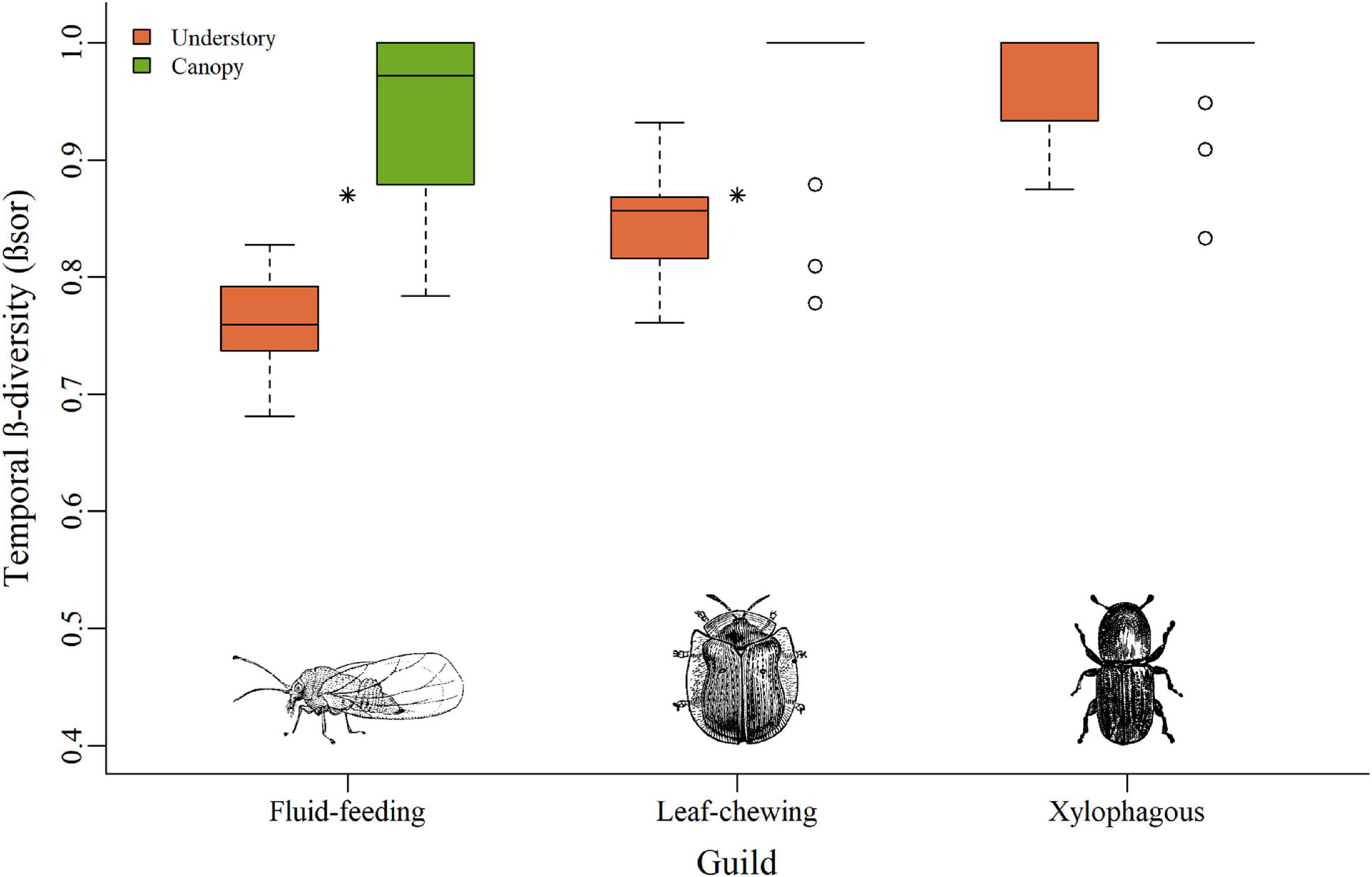
Figure 4. Boxplots of temporal β-diversity relating each forest island to the sampled stratum, for all three guilds of herbivorous insects. Bars represent the interquartile range with the median value. Circles indicate outliers. Stars reflect temporal β-diversity of the two insect guilds that showed relation with the stratum. Dashed lines indicate minimum and maximum values.
Discussion
In this study, we evaluated the influence that different spatiotemporal scales have on three free-feeding herbivorous insect guilds (fluid-feeding, chewing and xylophagous insects) in montane forest islands immersed in a grassland-dominated matrix (campo rupestre). We highlight a main contribution of time scale in the organization of the herbivorous insect community, mainly by turnover, with small differences among guilds. We could see the role of seasonality determining the local variation of species composition, corroborating that we have a highly variable always-green system over space and time, where the understory community varies less compared to the canopy community.
Among the several approaches that have been proposed to study patterns and processes that rule insect diversity across fragmented habitats, spatial metrics, such as patch (e.g., canopy cover, distance to continuous forest) and landscape attributes (e.g., percentage of forest in the landscape, number of patches, patch shape complexity) have been acknowledged to affect diversity patterns and distribution of several groups of insects in mountainous forest islands (da Silva et al., 2019; Perillo et al., 2020). Additionally, studies on temporal issues have drawn attention to help us understand and explain biodiversity dynamics in terrestrial fragmented habitats. Here, we found that the temporal scale exceeded the spatial scale in explaining herbivorous insect diversity, which is the opposite of what was expected for our first hypothesis. Most studies have found a higher predictive power of spatial scale than temporal ones over insect community structure in tropical ecosystems (da Silva et al., 2019; Castro et al., 2020). However, we found a higher importance of seasonal variation on the composition of all three insect guilds sampled in this study. These results indicate that, more than patch attributes, factors such as seasonal variation in temperature and humidity are drivers to temporal variation in resources that directly affect herbivorous insects that inhabit these forest islands. Thus, despite being an evergreen forest, the climatic seasonality of these forest islands is the most important driver for all herbivorous insect guilds sampled in this study and contribute more to their diversity and differences in species composition than spatial factors such as the distance among the forest islands or the vertical stratification (see Macedo-Reis et al., 2016; Novais et al., 2018).
We also found that the vertical stratum was an important factor driving the composition of fluid-feeding and leaf-chewing insect guilds, partially corroborating our third hypothesis. Similarly to our findings, wasps and bees (Perillo et al., 2020) and dung-beetles (da Silva et al., 2019) also have high temporal β-diversity (βsor) in this study system, indicating that the composition of insect communities varies greatly throughout seasons. In fact, all three herbivorous insect guilds, regardless of the strata, showed high temporal β-diversity, corroborating that we do have a highly variable system over space and time. However, fluid-feeding and leaf-chewing insects presented a greater temporal β-diversity for the canopy, which is the opposite of the pattern found for ants in a tropical dry-forest (Neves et al., 2021). The temporal β-diversity of both fluid-feeding and chewing insects being higher in the canopy than in the understory may be linked to the different effects of seasonality on specific microhabitats of these two strata. However, it may also be related to the insect diversity sampled in the understory that was much higher than that sampled in the canopy (which harbors more rare species and therefore has less redundancy) (see Skvarla et al., 2021). Besides, it is important to note that the insect guilds can respond differently to seasonal changes in resource availability between canopy and understory (see also Neves et al., 2014; Macedo-Reis et al., 2019).
Tropical forests have a vertical gradient of microclimatic conditions from the ground level to the canopy, with an increase of temperature and solar radiation, and a decrease in air humidity (Law et al., 2019). In this way, for herbivorous insects associated with forest islands system, it would be reasonable to think that the canopy has more unstable characteristics than the understory. For example, the increase in temperature and solar incidence is directly related to the survival of beetles from the Chrysomelidae and Curculionidae families, since it represents one of the main mortality factors of these insects (Lill and Marquis, 2007). In addition, the greater solar radiation can cause adult individuals of the Cicadellidae family to disperse less or to seek more humid and shaded areas (Ott and Carvalho, 2001; but also see Lessio and Alma, 2004). However, the canopy also has greater turnover of leaves and flowers (Basset et al., 2003b), resources that are especially important for fluid-feeding and chewing insects (Basset et al., 2003a). In this regard, it is known that the life cycle of individuals from the Cicadellidae and Psyllidae family probably follows the phenological pattern of leaf exchange in plants (Novais et al., 2018). Thus, we can expect that an environment with more changes in its conditions that presents, at the same time, more and better resource availability for its associated insects, will present greater temporal β-diversity for fluid-feeding and chewing insects. The same could not be observed for the guild of xylophagous insects, which is similar to the pattern reported among Scolytinae in Malaysian forests (Simon et al., 2003). This pattern could be explained by their close association with the range of suitable wood availability (Grove, 2002; Hulcr et al., 2008), and not leaves. Therefore, wood diameter and air moisture content seem to be better predictors of their diversity (Macedo-Reis et al., 2016), since they feed and spend much of their lives inside the bark, with little interaction with the external environment (Wood, 1982; Zuo et al., 2016). Here, it is important to emphasize that although Lepidoptera like the Symphita larvae are very important representatives of chewing herbivorous insect guild, they were not considered in our results especially due to the limitations of sampling traps (see Schmidt, 2016; Rosa et al., 2019; Skvarla et al., 2021).
When we consider the β-diversity decomposition into turnover and nestedness, we found species turnover as the main driver of β-diversities for the three herbivorous insect guilds in all spatio-temporal scales. This high species replacement supported our second hypothesis and has been documented in similar environments as the most important component of dissimilarity in species over time (Oliver et al., 2016; Nunes et al., 2020; Neves et al., 2021). A greater species turnover implies in high substitution of species, which indicates that each forest island must have a unique community. From this perspective, forest islands can be considered transient environments with great conservation value for many species (Vieira et al., 2008), where their presence can be considered a sample of the dispersers coming from continuous forest and even from open surrounding areas, which, in part, can explain the high rates of rare species (43.8% singletons and 15.1% doubletons) sampled in this study. Similar rates of rare species have been commonly observed in tropical arthropod studies (Coddington et al., 2009), and especially in high-elevation tropical mountains (Perillo et al., 2017). Thus, we argue that species turnover is an important factor for maintaining high diversity in forest islands in tropical mountain areas. Besides, it is important to notice that this system must be linked to metacommunity structure. Preliminary data from a long-term research project (Long term ecological research from campo rupestre – CRSC) also points out to the existence of a dynamic of insect metacommunities among forest islands, where both local (size, shape) and landscape (degree of isolation) characteristics can jointly influence the spatiotemporal dynamics of the structure of metacommunities that inhabit this ecosystem. For example, recent studies carried out with different insect taxa, including ants (Brant et al., 2021), dung beetles (da Silva et al., 2019), fruit-feeding butterflies (Pereira et al., 2017), and wasps and bees (Perillo et al., 2020), have found high species turnover rates among forest islands, showing the importance of each forest island in maintaining the structure and conserving the communities associated with the entire archipelago. Despite the small distance among the forest islands, this provides a valid explanation of spatial and temporal dynamics, driven by habitat heterogeneity and environmental filters, with forest fragments connected by dispersal among habitats.
We presented a diverse array of spatiotemporal distributions among herbivorous insect guilds. Both spatial and temporal factors have mixed contributions in shaping the observed patterns, and the main differences among guilds reflect their use of resources. We showed that in altimontane forest systems there is a great change in the composition of herbivorous insects that are especially shaped by temporal variations in climatic characteristics. Besides, our findings suggest that there are differences between strata, where the understory of this studied system is more diverse and stable than the canopy. Since the montane forest islands are located at a higher elevation with a great propensity to be affected by global changes (Nunes et al., 2020), we suggest that long-term ecological research on herbivorous community structure in relation to climatic variation is a key element for future investigations, which can be decisive for the conservation of herbivorous insect communities. Beyond that, it can work as an advantageous strategy for the development of environmental conservation and monitoring programs, as long-term studies that include multiple sites within a regional species pool enable a thorough assessment of biodiversity change. Additionally, the effects of anthropogenic pressures, such as fire and the constant presence of cattle around this complex forest archipelago system, must be monitored (see Coelho et al., 2018) if we expect to manage and conserve biological diversity in a sustainable manner, since these forest islands may serve as warming refuges in a fragmented landscape holding an invaluable diversity of species that, without these old-growth forest reservoirs, would be doomed to disappear (see Janzen and Hallwachs, 2019). In a changing world, supporting and maintaining monitoring efforts in these systems, including other regions of the Espinhaço Range, will certainly bring an advance in knowledge and will be very useful for our current understanding of the effects of anthropogenic pressures and climate change on communities that are associated to naturally insular systems.
Data Availability Statement
The original contributions presented in the study are included in the article/Supplementary Material, further inquiries can be directed to the corresponding author.
Author Contributions
JK, LM-R, and FN: study design and data analyses. FN: project funding. JK: data collection. JK, LM-R, MF, and FN: writing the manuscript. All authors contributed to the article and approved the submitted version.
Funding
This work was supported by the CNPq for funding the Long-Term Ecological Research “PELD Campos Rupestres da Serra do Cipó” (grant number 441515/2016-9) and FAPEMIG (grant number CRA-APQ-00311-15).
Conflict of Interest
The authors declare that the research was conducted in the absence of any commercial or financial relationships that could be construed as a potential conflict of interest.
Publisher’s Note
All claims expressed in this article are solely those of the authors and do not necessarily represent those of their affiliated organizations, or those of the publisher, the editors and the reviewers. Any product that may be evaluated in this article, or claim that may be made by its manufacturer, is not guaranteed or endorsed by the publisher.
Acknowledgments
We would like to highlight the invaluable help of Lucas Neves Perillo for assistance with field work and Marina Beirão for helping to draw up the map for this study. JK acknowledges a research scholarship from Coordenação de Aperfeiçoamento de Pessoal de Nível Superior (CAPES) grant number (88887.288737/2018-00). FN thanks CNPq (306995/2019-0) for funding. Thank also go to Reserva Vellozia, GSG and Parque Nacional da Serra do Cipó for logistical support. This manuscript is an original research article submitted to journal Frontiers and Global Change in contribution to the research topic Understanding Patterns and Mechanisms of Forest Canopy Diversity and Ecosystem Functions in a Changing World.
Supplementary Material
The Supplementary Material for this article can be found online at: https://www.frontiersin.org/articles/10.3389/ffgc.2021.709403/full#supplementary-material
References
Allan, J. D., and Castillo, M. M. (2007). Stream Ecology: Structure And Function Of Running Waters. Berlin: Springer Science & Business Media.
Allen, E. A., and Humble, L. M. (2002). Nonindigenous species introductions: a threat to Canada’s forests and forest economy1. Can. J. Plant Pathol. 24, 103–110. doi: 10.1080/07060660309506983
Araújo, W. S. (2013). A importância de fatores temporais para a distribuição de insetos herbívoros em sistemas Neotropicais. Rev. Biol. 10, 1–7. doi: 10.7594/revbio.10.01.01
Ashton, L. A., Griffiths, H. M., Parr, C. L., Evans, T. A., Didham, R. K., Hasan, F., et al. (2019). Termites mitigate the effects of drought in tropical rainforest. Science 363, 174–177. doi: 10.1126/science.aau9565
Baselga, A. (2010). Partitioning the turnover and nestedness components of beta diversity. Glob. Ecol. Biogeogr. 19, 134–143. doi: 10.1111/j.1466-8238.200900490.x
Baselga, A., and Orme, C. D. L. (2012). betapart: an R package for the study of beta diversity. Methods Ecol. Evol. 3, 808–812. doi: 10.1111/j.2041-210X.2012.00224.x
Basset, Y., Aberlenc, H. P., Barrios, H., Curletti, G., Bérenger, J. M., Vesco, J. P., et al. (2001). Stratification and diel activity of arthropods in a lowland rainforest in Gabon. Biol. J. Linn. Soc. 72, 585–607. doi: 10.1111/j.1095-8312.2001.tb01340.x
Basset, Y., Aberlenc, H. P., and Delvare, G. (1992). Abundance and stratification of foliage arthropods in a lowland rain forest of Cameroon. Ecol. Entomol. 17, 310–318. doi: 10.1111/j.1365-2311.1992.tb01063.x
Basset, Y., Cizek, L., Cuénoud, P., Didham, R. K., Guilhaumon, F., and Missa, O. (2012). Arthropod diversity in a tropical forest. Science 338, 1481–1484. doi: 10.1126/science.1226727
Basset, Y., Cizek, L., Cuénoud, P., Didham, R. K., Novotny, V., Ødegaard, F., et al. (2015). Arthropod Distribution in a Tropical Rainforest: tackling a Four Dimensional Puzzle. PLoS One 10:e0144110. doi: 10.1371/journal.pone.0144110
Basset, Y., Novotny, V., Barrios, H., Holloway, J. D., and Miller, S. E. (2003a). “Vertical stratification of arthropod assemblages,” in Arthropods of Tropical Forests: Spatio-Temporal Dynamics And Resource Use In The Canopy, eds Y. Basset, V. Novotny, S. E. Miller, and R. L. Kitching (Cambridge: Cambridge University Press), 17–29.
Basset, Y., Novotny, V., Miller, S. E., and Kitching, R. L. (2003b). “Conclusion: arthropods, canopies and interpretable patterns,” in Arthropods of Tropical Forests: Spatio-Temporal Dynamics and Resource Use in The Canopy, eds Y. Basset, V. Novotny, S. E. Miller, and R. L. Kitching (Cambridge: Cambridge University Press), 394–405.
Bates, D., Maechler, M., Bolker, B., Walker, S., Christensen, R. H. B., Singmann, H., et al. (2018). Package ‘lme4’. Version 1:437.
Beaver, R. A. (1979). Host specificity of temperate and tropical animals. Nature 281, 139–141. doi: 10.1038/281139a0
Bolker, B. M., Brooks, M. E., Clark, C. J., Geange, S. W., Poulsen, J. R., Stevens, M. H. H., et al. (2009). Generalized linear mixed models: a practical guide for ecology and evolution. Trends Ecol. Evol. 24, 127–135. doi: 10.1016/j.tree.2008.10.008
Brant, H. S. C., da Silva, P. G., de Castro, F. S., Perillo, L. N., and de Siqueira Neves, F. (2021). Spatiotemporal patterns of ant metacommunity in a montane forest archipelago. Neotrop. Entomol 1–13. doi: 10.1007/s13744-021-00901-2
Brehm, G. (2007). Contrasting patterns of vertical stratification in two moth families in a Costa Rican lowland rain forest. Basic Appl. Ecol. 8, 44–54. doi: 10.1016/j.baae.2006.02.002
Brown, J. H. (1981). Two decades of homage to Santa Rosalia: toward a general theory of diversity. Am. Zool. 21, 877–888. doi: 10.1093/icb/21.4.877
Callisto, M., Solar, R., Silveira, F. A., Saito, V. S., Hughes, R. M., Fernandes, G. W., et al. (2019). A Humboldtian approach to mountain conservation and freshwater ecosystem services. Front. Environ. Sci. 7:195. doi: 10.3389/fenvs.2019.00195
Cardoso, P., Barton, P. S., Birkhofer, K., Chichorro, F., Deacon, C., Fartmann, T., et al. (2020). Scientists’ warning to humanity on insect extinctions. Biol. Conserv. 242:108426. doi: 10.1016/j.biocon.2020.108426
Castro, F. S. D., Da Silva, P. G., Solar, R., Fernandes, G. W., and Neves, F. D. S. (2020). Environmental drivers of taxonomic and functional diversity of ant communities in a tropical mountain. Insect Conserv. Divers. 13, 393–403. doi: 10.1111/icad.12415
Chao, A., Gotelli, N. J., Hsieh, T. C., Sander, E. L., Ma, K. H., Colwell, R. K., et al. (2014). Rarefaction and extrapolation with Hill numbers: a framework for sampling and estimation in species diversity studies. Ecol. Monogr. 84, 45–67. doi: 10.1890/13-0133.1
Chao, A., Wang, Y. T., and Jost, L. (2013). Entropy and the species accumulation curve: a novel entropy estimator via discovery rates of new species. Methods Ecol. Evol. 4, 1091–1100. doi: 10.1111/2041-210X.12108
Chapman, A. D. (2009). Numbers of Living Species in Australia and the World. Toowoomba: Australian Biological Resources Study (ABRS).
Chen, I. C., Hill, J. K., Ohlemüller, R., Roy, D. B., and Thomas, C. D. (2011). Rapid range shifts of species associated with high levels of climate warming. Science 333, 1024–1026. doi: 10.1126/science.1206432
Coddington, J. A., Agnarsson, I., Miller, J. A., Kuntner, M., and Hormiga, G. (2009). Undersampling bias: the null hypothesis for singleton species in tropical arthropod surveys. J. Anim. Ecol. 78, 573–584. doi: 10.1111/j.1365-2656.2009.01525.x
Coelho, M. S., Fernandes, G. W., Pacheco, P., Diniz, V., Meireles, A., Santos, R. M., et al. (2016). “Archipelago of montane forests surrounded by rupestrian grasslands: new insights and perspectives,” in Ecology and Conservation of Mountain-Top Grasslands in Brazil, ed. G. W. Fernandes (New York: Springer), 129–153.
Coelho, M. S., Neves, F. S., Perillo, L. N., Morellato, L. P. C., and Fernandes, G. W. (2018). Forest archipelagos: a natural model of metacommunity under the threat of fire. Flora 238, 244–249. doi: 10.1016/j.flora.2017.03.013
Cook, W. M., Anderson, R. M., and Schweiger, E. W. (2004). Is the matrix really inhospitable? Vole runway distribution in an experimentally fragmented landscape. Oikos 104, 5–14. doi: 10.1111/j.0030-1299.2004.12761.x
Cooke, S. J., Sack, L., Franklin, C. E., Farrell, A. P., Beardall, J., Wikelski, M., et al. (2013). What is conservation physiology? Perspectives on an increasingly integrated and essential science. Conserv. Physiol. 1:cot001. doi: 10.1093/conphys/cot001
da Silva, P. G., Nunes, C. A., Ferreira, L. F., Braga, R. F., Beiroz, W., Perillo, L. N., et al. (2019). Patch and landscape effects on forest-dependent dung beetles are masked by matrix-tolerant dung beetles in a mountaintop rainforest archipelago. Sci. Total Environ. 651, 1321–1331. doi: 10.1016/j.scitotenv.2018.09.195
Denno, R. F., and Perfect, T. J. (1994). Planthoppers: Their Ecology and Management. London: Chapman & Hall.
Denno, R. F., and Roderick, G. K. (1991). “Influence of patch size, vegetation texture, and host plant architecture on the diversity, abundance, and life history styles of sap-feeding herbivores,” in Habitat Structure–The Physical Arrangement of Objects in Space, eds S. S. Bell, E. D. McCoy, and H. R. Mushinsky (United Kingdom: Chapman & Hall), 169–196.
DeVries, P. J. (1988). Stratification of fruit-feeding nymphalid butterflies in a Costa Rican rainforest. J. Res. Lepid. 26, 98–108.
DeVries, P. J., Walla, T., and Greeney, H. F. (1999). Species diversity in spatial and temporal dimensions of fruit-feeding butterflies from two Ecuadorian rainforests. Biol. J. Linn. Soc. 68, 333–353. doi: 10.1111/j.1095-8312.1999.tb01175.x
Didham, R. K., and Springate, N. D. (2003). “Determinants of temporal variation in community structure,” in Arthropods of Tropical Forests: Spatio-Temporal Dynamics and Resource Use in the Canopy, eds Y. Basset, V. Novotny, S. E. Miller, and R. L. Kitching (Cambridge: Cambridge University Press), 28–39.
Dirzo, R., Young, H. S., Galetti, M., Ceballos, G., Isaac, N. J., and Collen, B. (2014). Defaunation in the Anthropocene. Science 345, 401–406. doi: 10.1126/science.1251817
Driscoll, D. A. (2005). Is the matrix a sea? Habitat specificity in a naturally fragmented landscape. Ecol. Entomol. 30, 8–16. doi: 10.1111/j.0307-6946.2005.00666.x
Fahrig, L. (2017). Ecological responses to habitat fragmentation per se. Annu. Rev. Ecol. Evol. Syst. 48, 1–23. doi: 10.1146/annurev-ecolsys-110316-022612
Ferrari, L. T., Schaefer, C. E. G. R., Fernandes, R. B. A., Mendonça, B. A. F., Gjorup, D. F., Corrêa, G. R., et al. (2016). “Thermic and hydric dynamics of ironstone (Canga) and quartzite Rupestrian grasslands in the Quadrilátero Ferrífero: the ecological importance of water,” in Ecology and Conservation of Mountaintop Grasslands in Brazil, ed. G. W. Fernandes (Cham: Springer), 71–85.
Gohli, J., Kirkendall, L. R., Smith, S. M., Cognato, A. I., Hulcr, J., and Jordal, B. H. (2017). Biological factors contributing to bark and ambrosia beetle species diversification. Evolution 71, 1258–1272. doi: 10.1111/evo.13219
Grimbacher, P. S., and Stork, N. E. (2007). Vertical stratification of feeding guilds and body size in beetle assemblages from an Australian tropical rainforest. Austral Ecol. 32, 77–85. doi: 10.1111/j.1442-9993.2007.01735.x
Grove, S. J. (2002). Saproxylic insect ecology and the sustainable management of forests. Annu. Rev. Ecol. Evol. Syst. 33, 1–23. doi: 10.1146/annurev.ecolsys.33.010802.150507
Haack, R. A. (2006). Exotic bark-and wood-boring Coleoptera in the United States: recent establishments and interceptions. Can. J. For. Res. 36, 269–288. doi: 10.1139/x05-249
Haddad, N. M., Gonzalez, A., Brudvig, L. A., Burt, M. A., Levey, D. J., and Damschen, E. I. (2017). Experimental evidence does not support the Habitat Amount Hypothesis. Ecography 40, 48–55. doi: 10.1111/ecog.02535
Hallmann, C. A., Sorg, M., Jongejans, E., Siepel, H., Hofland, N., Schwan, H., et al. (2017). More than 75 percent decline over 27 years in total flying insect biomass in protected areas. PLoS One 12:e0185809. doi: 10.1371/journal.pone.0185809
Hsieh, T. C., Ma, K. H., and Chao, A. (2016). iNEXT: an R package for rarefaction and extrapolation of species diversity (Hill numbers). Methods Ecol.Evol. 7, 1451–1456. doi: 10.1111/2041-210X.12613
Huberty, A. F., and Denno, R. F. (2006). Consequences of nitrogen and phosphorus limitation for the performance of two planthoppers with divergent life-history strategies. Oecologia 149, 444–455. doi: 10.1007/s00442-006-0462-8
Hulcr, J., Beaver, R. A., Puranasakul, W., Dole, S. A., and Sonthichai, S. (2008). A comparison of bark and ambrosia beetle communities in two forest types in northern Thailand (Coleoptera: Curculionidae: Scolytinae and Platypodinae). Environ. Entomol. 37, 1461–1470. doi: 10.1603/0046-225x-37.6.1461
Inclán, D. J., Cerretti, P., and Marini, L. (2014). Interactive effects of area and connectivity on the diversity of tachinid parasitoids in highly fragmented landscapes. Landsc. Ecol 29, 879–889.
Janzen, D. H., and Hallwachs, W. (2019). Perspective: where might be many tropical insects? Biol. Conserv. 233, 102–108. doi: 10.1016/j.biocon.2019.02.030
Kitching, R. L., Ashton, L. A., Nakamura, A., Whitaker, T., and Khey, V. T. (2013). Distance driven species turnover in Bornean rainforests: homogeneity and heterogeneity in primary and post-logging forests. Ecography 36, 675–682. doi: 10.1111/j.1600-0587.2012.00023.x
Kuchenbecker, J., and Fagundes, M. (2018). Diversity of insects associated with two common plants in the Brazilian Cerrado: responses of two guilds of herbivores to bottom-up and top-down forces. Eur. J. Entomol. 115, 354–363. doi: 10.14411/eje.2018.035
Law, S. J., Bishop, T. R., Eggleton, P., Griffiths, H., Ashton, L., and Parr, C. (2019). Darker ants dominate the canopy: testing macroecological hypotheses for patterns in colour along a microclimatic gradient. J. Anim. Ecol. 89, 347–359. doi: 10.1111/1365-2656.13110
Leal, C. R. O., Silva, J. O., Sousa-Souto, L., and de Siqueira Neves, F. (2016). Vegetation structure determines insect herbivore diversity in seasonally dry tropical forests. J. Insect Conserv. 20, 979–988. doi: 10.1007/s10841-016-9930-6
Lessio, F., and Alma, A. (2004). Seasonal and daily movement of Scaphoideus titanus Ball (Homoptera: Cicadellidae). Environ. Entomol. 33, 1689–1694. doi: 10.1603/0046-225X-33.6.1689
Lewinsohn, T. M., Novotny, V., and Basset, Y. (2005). Insects on plants: diversity of herbivore assemblages revisited. Annu. Rev. Ecol. Evol. Syst. 36, 597–620. doi: 10.1146/annurev.ecolsys.36.091704.175520
Lewinsohn, T. M., and Roslin, T. (2008). Four ways towards tropical herbivore megadiversity. Ecol. Lett. 11, 398–416. doi: 10.1111/j.1461-0248.2008.01155.x
Lill, J. T., and Marquis, R. J. (2007). “Microhabitat manipulation: ecosystem engineering by shelter-building insects,” in Ecosystem Engineers: Plants To Protists, eds K. Cuddington, J. E. Byers, A. Hastings, and W. G. Wilson (New York: Academic Press), 107–138.
Lister, B. C., and Garcia, A. (2018). Climate-driven declines in arthropod abundance restructure a rainforest food web. Proc. Natl. Acad. Sci. U. S. A. 115, E10397–E10406. doi: 10.1073/pnas.1722477115
Lourenço, G. M., Campos, R. B., and Ribeiro, S. P. (2015). Spatial distribution of insect guilds in a tropical montane rainforest: effects of canopy structure and numerically dominant ants. Arthropod Plant Interact. 9, 163–174. doi: 10.1007/s11829-015-9359-y
Macedo-Reis, L. E., Novais, S. M. A. D., Monteiro, G. F., Flechtmann, C. A. H., Faria, M. L. D., and Neves, F. D. S. (2016). Spatio-temporal distribution of bark and ambrosia beetles in a Brazilian tropical dry forest. J. Insect Sci. 16:48. doi: 10.1093/jisesa/iew027
Macedo-Reis, L. E., Quesada, M., and de Siqueira Neves, F. (2019). Forest cover drives insect guild diversity at different landscape scales in tropical dry forests. For. Ecol. Manag. 443, 36–42. doi: 10.1016/j.foreco.2019.04.007
Miola, D. T., Ramos, V. D., and Silveira, F. A. (2020). A brief history of research in campo rupestre: identifying research priorities and revisiting the geographical distribution of an ancient, widespread Neotropical biome. Biol. J. Linn. Soc. 133, 464–480. doi: 10.1093/biolinnean/blaa175
Monteiro, G. F., Macedo-Reis, L. E., Dáttilo, W., Fernandes, G. W., Siqueira de Castro, F., and Neves, F. S. (2020). Ecological interactions among insect herbivores, ants and the host plant Baccharis dracunculifolia in a Brazilian mountain ecosystem. Austral Ecol. 45, 158–167. doi: 10.1111/aec.12839
Moran, V. C., and Southwood, T. R. E. (1982). The guild composition of arthropod communities in trees. J. Anim. Ecol. 51, 289–306. doi: 10.2307/4325
Muirhead-Thomson, R. C. (1991). Trap Responses of Flying Insects: The Influence of Trap Design On Capture Efficiency. New York: Academic Press.
Nadkarni, N. M., Parker, G. G., and Lowman, M. D. (2011). Forest canopy studies as an emerging field of science. Ann. For. Sci. 68, 217–224. doi: 10.1007/s13595-011-0046-6
Neves, F. S., Antoniazzi, R., Camarota, F., Pacelhe, F. T., and Powell, S. (2021). Spatiotemporal dynamics of the ant community in a dry forest differ by vertical strata but not by successional stage. Biotropica 53, 372–383. doi: 10.1111/btp.12918
Neves, F. S., Araújo, L. S., Espírito-Santo, M. M., Fagundes, M., Fernandes, G. W., Sanchez-Azofeifa, G. A., et al. (2010). Canopy herbivory and insect herbivore diversity in a dry forest–savanna transition in Brazil. Biotropica 42, 112–118. doi: 10.1111/j.1744-7429.2009.00541.x
Neves, F. S., Silva, J. O., Espírito-Santo, M. M., and Fernandes, G. W. (2014). Insect herbivores and leaf damage along successional and vertical gradients in a tropical dry forest. Biotropica 46, 14–24. doi: 10.1111/btp.12068
Neves, F. S., Sperber, C. F., Campos, R. I., Soares, J. P., and Ribeiro, S. P. (2013). Contrasting effects of sampling scale on insect herbivores distribution in response to canopy structure. Rev. Biol. Trop. 1, 125–137.
Novais, S., Macedo-Reis, L. E., Cristobal-Peréz, E. J., Sánchez-Montoya, G., Janda, M., Neves, F., et al. (2018). Positive effects of the catastrophic Hurricane Patricia on insect communities. Sci. Rep. 8:15042.
Novais, S., Macedo-Reis, L. E., DaRocha, W. D., and Neves, F. S. (2016). Effects of habitat management on different feeding guilds of herbivorous insects in cacao agroforestry systems. Rev. Biol. Trop. 64, 763–777. doi: 10.15517/rbt.v64i2.19100
Novais, S. M. A., Monteiro, G. F., Macedo-Reis, L. E., Leal, C. R. O., and de Siqueira Neves, F. (2019). Changes in the insect herbivore fauna after the first rains in a tropical dry forest. Oecologia Aust. 23, 381–387. doi: 10.4257/oeco.2019.2302.16
Novotny, V., Miller, S. E., Baje, L., Balagawi, S., Basset, Y., Cizek, L., et al. (2010). Guild-specific patterns of species richness and host specialization in plant–herbivore food webs from a tropical forest. J. Anim. Ecol. 79, 1193–1203. doi: 10.1111/j.1365-2656.2010.01728.x
Novotny, V., Miller, S. E., Hrcek, J., Baje, L., Basset, Y., Lewis, O. T., et al. (2012). Insects on plants: explaining the paradox of low diversity within specialist herbivore guilds. Am. Nat. 179, 351–362. doi: 10.5061/dryad.rg155q32
Novotny, V., and Weiblen, G. D. (2005). From communities to continents: beta diversity of herbivorous insects. Ann. Zool. Fenn. 42, 463–475.
Noyes, J. S. (1989). A study of five methods of sampling Hymenoptera (Insecta) in a tropical rainforest, with special reference to the Parasitica. J. Nat. Hist. 23, 285–298. doi: 10.1080/00222938900770181
Nunes, C. A., Castro, F. S., Brant, H. S. C., Powell, S., Solar, R., Fernandes, G. W., et al. (2020). High temporal beta diversity in an ant metacommunity, with increasing temporal functional replacement along the elevational gradient. Front. Ecol. Evol. 8:571439. doi: 10.3389/fevo.2020.571439
Öckinger, E., Lindborg, R., Sjödin, N. E., and Bommarco, R. (2012). Landscape matrix modifies richness of plants and insects in grassland fragments. Ecography 35, 259–267. doi: 10.1111/j.1600-0587.2011.06870.x
Ødegaard, F. (2003). “Taxonomic composition and host specificity of phytophagous beetles in a dry forest in Panama,” in Arthropods of Tropical Forests: Spatio-Temporal Dynamics and Resource Use in the Canopy, eds Y. Basset, V. Novotny, S. E. Miller, and R. L. Kitching (Cambridge: Cambridge University Press), 220–236.
Oksanen, J., Blanchet, F. G., Kindt, R., Legendre, P., Minchin, P. R., Hara, R. B. O., et al. (2018). Package ‘vegan’: Community Ecology Package. R package version 2.5–7.
Oliveira, B. F., and Scheffers, B. R. (2019). Vertical stratification influences global patterns of biodiversity. Ecography 42, 249–249. doi: 10.1111/ecog.03636
Oliver, I., Dorrough, J., Doherty, H., and Andrew, N. R. (2016). Additive and synergistic effects of land cover, land use and climate on insect biodiversity. Landsc. Ecol. 31, 2415–2431.
Ott, A. P., and Carvalho, G. S. (2001). Comunidade de cigarrinhas (Hemiptera: Auchenorrhyncha) de uma área de campo do município de Viamão, Rio Grande do Sul, Brasil. Neotrop. Entomol. 30, 233–243. doi: 10.1590/S1519-566X2001000200005
Peeters, P. J. (2002). Correlations between leaf structural traits and the densities of herbivorous insect guilds. Biol. J. Linn. Soc. 77, 43–65.
Peñuelas, J., Sardans, J., Estiarte, M., Ogaya, R., Carnicer, J., Coll, M., et al. (2013). Evidence of current impact of climate change on life: a walk from genes to the biosphere. Glob. Change Biol. 19, 2303–2338. doi: 10.1111/gcb.12143
Pereira, G. C. N., Coelho, M. S., Beirão, M. D. V., Braga, R. F., and Fernandes, G. W. (2017). Diversity of fruit-feeding butterflies in a mountaintop archipelago of rainforest. PLoS One 12:e0180007. doi: 10.1371/journal.pone.0180007
Perillo, L. N., Barbosa, N. P. U., Solar, R. R. C., and Neves, F. S. (2020). Patterns of diversity in a metacommunity of bees and wasps of relictual mountainous forest fragments. J. Insect Conserv. 24, 17–34. doi: 10.1007/s10841-019-00194-2
Perillo, L. N., Neves, F. S., Antonini, Y., and Martins, R. P. (2017). Compositional changes in bee and wasp communities along neotropical mountain altitudinal gradient. PLoS One 12:e0182054. doi: 10.1371/journal.pone.0182054
Raffa, K. F., Gregoire, J., and Lindgren, B. S. (2015). “Natural history and ecology of bark beetles,” in Bark Beetles: Biology and Ecology of Native and Invasive Species, eds F. E. Vega and R. W. Hofstetter (Cambridge: Academic Press), 1–40. doi: 10.1016/B978-0-12-417156-5.00001-0
Raupp, M. J. (1985). Effects of leaf toughness on mandibular wear of the leaf beetle. Ecol. Entomol. 10, 73–79. doi: 10.1111/j.1365-2311.1985.tb00536.x
Read, J., and Sanson, G. D. (2003). Characterizing sclerophylly: the mechanical properties of a diverse range of leaf types. New Phytol. 160, 81–99. doi: 10.1046/j.1469-8137.2003.00855.x
Ribeiro, S. P., Pimenta, H. R., and Fernandes, G. W. (1994). Herbivory by chewing and sucking insects on Tabebuia ochracea. Biotropica 26, 302–307. doi: 10.2307/2388851
Rosa, A. H. B., Perillo, L. N., Neves, F. S., and Ribeiro, D. B. (2019). Butterflies collected using malaise traps as useful bycatches for ecology and conservation. J. Threat. Taxa 11, 14235–14237. doi: 10.11609/jot.4919.11.9.14235-14237
Rossetti, M. R., Rösch, V., Videla, M., Tscharntke, T., and Batáry, P. (2019). Insect and plant traits drive local and landscape effects on herbivory in grassland fragments. Ecosphere 10:e02717. doi: 10.1002/ecs2.2717
Scheffers, B. R., Shoo, L., Phillips, B., Macdonald, S. L., Anderson, A., VanDerWal, J., et al. (2017). Vertical (arboreality) and horizontal (dispersal) movement increase the resilience of vertebrates to climatic instability. Glob. Ecol. Biogeogr. 26, 787–798.
Schmidt, O. (2016). Records of larentiine moths (Lepidoptera: Geometridae) collected at the Station Linné in Sweden. Biodivers. Data J. 4:e7304. doi: 10.3897/BDJ.4.e7304
Schuch, S., Wesche, K., and Schaefer, M. (2012). Long-term decline in the abundance of leafhoppers and planthoppers (Auchenorrhyncha) in Central European protected dry grasslands. Biol. Conserv. 149, 75–83. doi: 10.1016/j.biocon.2012.02.006
Shaw, D. C. (2004). “Vertical Organization of Canopy Biota,” in Forest Canopies, eds M. D. Lowman and H. B. Rinker (San Diego: Academic Press), 73–101.
Silva, J. O., Leal, C. R. O., Espírito-Santo, M. M., and Morais, H. C. (2017). Seasonal and diel variations in the activity of canopy insect herbivores differ between deciduous and evergreen plant species in a tropical dry forest. J. Insect Conserv. 21, 667–676. doi: 10.1007/s10841-017-0009-9
Silva, L. F., Souza, R. M., Solar, R. R. C., and Neves, F. D. S. (2017). Ant diversity in Brazilian tropical dry forests across multiple vegetation domains. Environ. Res. Lett. 12:035002. doi: 10.1088/1748-9326/aa5f2a
Silva, V. D., Beirão, M. V., and Cardoso, D. C. (2020). Thermal tolerance of fruit-feeding butterflies (Lepidoptera: Nymphalidae) in contrasting mountaintop environments. Insects 11:278.
Silveira, F. A. O., Negreiros, D., Barbosa, N. P. U., Buisson, E., Carmo, F. F., Carstensen, D. W., et al. (2016). Ecology and evolution of plant diversity in the endangered campo rupestre: a neglected conservation priority. Plant Soil 403, 129–152. doi: 10.1007/s11104-015-2637-8
Simon, U., Gossner, M., and Linsenmair, K. E. (2003). “Distribution of ants and bark-beetles in crowns of tropical oaks,” in Arthropods of Tropical Forests: Spatio-Temporal Dynamics and Resource Use in the Canopy, eds Y. Basset, V. Novotny, S. E. Miller, and R. L. Kitching (Cambridge: Cambridge University Press), 59–68.
Skvarla, M. J., Larson, J. L., Fisher, J. R., and Dowling, A. P. (2021). A review of terrestrial and Canopy Malaise Traps. Ann. Entomol. Soc. Am. 114, 27–47. doi: 10.1093/aesa/saaa044
Sobek, S., Scherber, I. C., and Tscharntke, T. (2009). Spatio temporal changes of beetle communities across a tree diversity gradient. Divers. Distrib. 15, 660–670. doi: 10.1111/j.1472-4642.2009.00570.x
Soininen, J., Heino, J., and Wang, J. (2018). A meta-analysis of nestedness and turnover components of beta diversity across organisms and ecosystems. Glob. Ecol. Biogeogr. 27, 96–109. doi: 10.1111/geb.12660
Stork, N. E. (2018). How many species of insects and other terrestrial arthropods are there on Earth? Annu. Rev. Entomol. 63, 31–45. doi: 10.1146/annurev-ento-020117-043348
Streher, A. S., Sobreiro, J. F. F., Morellato, L. P. C., and Silva, T. S. F. (2017). Land surface phenology in the tropics: the role of climate and topography in a snow-free mountain. Ecosystems 20, 1436–1453. doi: 10.1007/s10021-017-0123-2
Swart, R. C., Samways, M. J., and Roets, F. (2020). Tree canopy arthropods have idiosyncratic responses to plant ecophysiological traits in a warm temperate forest complex. Sci. Rep. 10:19905. doi: 10.1038/s41598-020-76868-8
Tews, J., Brose, U., Grimm, V., Tielbörger, K., Wichmann, M. C., Schwager, M., et al. (2004). Animal species diversity driven by habitat heterogeneity/diversity: the importance of keystone structures. J. Biogeogr. 31, 79–92.
Toivonen, M., Peltonen, A., Herzon, I., Heliölä, J., Leikola, N., and Kuussaari, M. (2017). High cover of forest increases the abundance of most grassland butterflies in boreal farmland. Insect Conserv. Divers. 10, 321–330. doi: 10.1111/icad.12226
Tylianakis, J. M., Klein, A. M., and Tscharntke, T. (2005). Spatiotemporal variation in the effects of a tropical habitat gradient on Hymenoptera diversity. Ecology 86, 3296–3302. doi: 10.1890/05-0371
Van der Hammen, T. (1995). “Global change, biodiversity, and conservation of neotropical montane forests,” in Biodiversity and Conservation of Neotropical Montane Forests, eds S. P. Churchill, H. Balslev, E. Forero, and J. L. Luteyn (New York: The New York botanical Garden), 603–607.
Vehviläinen, H., Koricheva, J., and Ruohomäki, K. (2007). Tree species diversity influences herbivore abundance and damage: meta-analysis of long-term forest experiments. Oecologia 152, 287–298. doi: 10.1007/s00442-007-0673-7
Vieira, L., Lopes, F. S., Fernandes, W. D., and Raizer, J. (2008). Comunidade de carabidae (Coleoptera) em manchas florestais no pantanal, Mato Grosso do Sul, Brasil. Iheringia Ser. Zool. 98, 317–324. doi: 10.1590/S0073-47212008000300005
Wardhaugh, C. W. (2014). The spatial and temporal distributions of arthropods in forest canopies: uniting disparate patterns with hypotheses for specialisation. Biol. Rev. 89, 1021–1041. doi: 10.1111/brv.12094
Webster, G. L. (1995). “The panorama of Neotropical Cloud Forests,” in Biodiversity and Conservation of Neotropical Montane Forests: Proceedings of Neotropical Montane Forest Biodiversity and Conservation Symposium, eds S. P. Churchill, H. Balslev, E. Forero, and J. L. Luteyn (New York: The New York Botanical Garden), 57–77.
Weibull, A. C., Bengtsson, J., and Nohlgren, E. (2000). Diversity of butterflies in the agricultural landscape: the role of farming system and landscape heterogeneity. Ecography 23, 743–750. doi: 10.1034/j.1600-0587.2000.230611.x
Whittaker, R. (1960). Vegetation of the Siskiyou Mountains, Oregon and California. Ecol. Monogr. 30, 279–338. doi: 10.2307/1943563
Wilson, E. O. (1987). The little things that run the world (the importance and conservation of invertebrates). Conserv. Biol. 4, 344–346. doi: 10.1111/j.1523-1739.1987.tb00055.x
Wolda, H. (1992). Trends in abundance of tropical forest insects. Oecologia 89, 47–52. doi: 10.1007/BF00319014
Wood, S. L. (1982). The Bark and Ambrosia Beetles of North and Central America (Coleoptera: Scolytidae), A Taxonomic Monograph. Provo: Brigham Young University.
Yekwayo, I., Pryke, J. S., Roets, F., and Samways, M. J. (2016). Surrounding vegetation matters for arthropods of small, natural patches of indigenous forest. Insect Conserv. Divers. 9, 224–235. doi: 10.1111/icad.12160
Keywords: Atlantic Forest, campo rupestre, chewing insects, fluid-feeding insects, xylophagous insects, β-diversity, Malaise trap
Citation: Kuchenbecker J, Macedo-Reis LE, Fagundes M and Neves FS (2021) Spatiotemporal Distribution of Herbivorous Insects Along Always-Green Mountaintop Forest Islands. Front. For. Glob. Change 4:709403. doi: 10.3389/ffgc.2021.709403
Received: 13 May 2021; Accepted: 07 October 2021;
Published: 27 October 2021.
Edited by:
Louise A. Ashton, The University of Hong Kong, Hong Kong, SAR ChinaReviewed by:
Antonio Rodriguez, Swedish University of Agricultural Sciences, SwedenAurélien Sallé, Université d’Orléans, France
Copyright © 2021 Kuchenbecker, Macedo-Reis, Fagundes and Neves. This is an open-access article distributed under the terms of the Creative Commons Attribution License (CC BY). The use, distribution or reproduction in other forums is permitted, provided the original author(s) and the copyright owner(s) are credited and that the original publication in this journal is cited, in accordance with accepted academic practice. No use, distribution or reproduction is permitted which does not comply with these terms.
*Correspondence: Juliana Kuchenbecker, anViZWxpc2FyaW9AZ21haWwuY29t