- 1Rocky Mountain Research Station, USDA Forest Service, Missoula, MT, United States
- 2(Retired) Rocky Mountain Research Station, USDA Forest Service, Missoula, MT, United States
Estimates of dead and down woody material (DWM) biomass are important for nutrient cycling, wildlife habitat assessment, fire effects and climate change science. Most methods used to sample woody material initially assess volume then estimates of wood density are used to convert volume to biomass. To assess initial wood density and decomposition rate, this study examined in situ wood density of lodgepole pine logs at the Tenderfoot Creek Experimental Forest (TCEF), central Montana, United States, 1, 11, and 22 years after felling. Mean wood density decreased from 0.39 to 0.27 g cm–3 over 22 years and the single exponential decay rate was k = 0.012 yr–1 1 and 11-years post-felling and 0.022 yr–1 11 and 22 years post-felling. A common 5-category decay classification system was evaluated for estimating wood density by decay class, which identified significant difference in three of four observed classes.
Introduction
Dead and down woody material (DWM) is an important ecological component in forested systems. Its impacts have been reviewed in previous work, which describe the role of DWM in providing habitat for terrestrial wildlife, nutrient cycling, moisture retention, plant regeneration and aquatic habitats (Maser and Trappe, 1984; Harmon et al., 1986; Maser et al., 1988; Gurnell et al., 1995; Stevens, 1997). DWM often comprises a large proportion of the total above ground biomass in forested systems, potentially 20%, or more (Grier and Logan, 1977; Harmon et al., 1986; Harmon and Hua, 1991). As dead wood decays carbon is transferred to the atmosphere through respiration, thus accounting for DWM is important for climate change science (Currie and Nadelhoffer, 2002; Weedon et al., 2009; Harmon et al., 2020). The importance and relative abundance of DWM necessitate an accurate accounting of its quantity.
Sampling procedures for quantifying the abundance of DWM have been described in earlier work and include planar intersect (Warren and Olsen, 1964; van Wagner, 1968; Brown, 1971), area sampling (Bate et al., 2004), relascope sampling (Ståhl, 1998; Gove et al., 1999), photographic assessments (Fischer, 1981; Ottmar et al., 1998; Keane and Dickinson, 2007) and LiDAR (Marchi et al., 2018; Lopes Queiroz et al., 2020). Most methods measure volume initially, then biomass (mass per unit area) is calculated as the product of volume and wood density. Because biomass is most often used to report the abundance of DWM, accurate estimates of wood density are required.
Factors important for determining wood density include species and decay state (Harmon et al., 1986). Decay state is related to time since death and the rate of decomposition; while rate of decomposition is a function of wood and soil characteristics, site and climate (Maser and Trappe, 1984; Harmon et al., 1986). The density of wood is highest at the time of death and is reduced over time through decomposition – respiration, fragmentation and leaching – until the organic matter that remains is integrated into the soil (Maser and Trappe, 1984; Harmon et al., 1986).
Calculating wood density and decay rate takes time and requires specialized lab equipment, so it may be preferrable for managers and researchers estimating current and future DWM biomass to use locally developed wood density and decay rate values instead of calculating the values themselves. Locally determined values account for factors that introduce variability into wood density and decay rate, especially climate (Harmon et al., 1986). Lodgepole pine wood density and decay rates have not previously been published for high elevation forests central Montana, United States.
The results in this paper are part of a time series study and contain material previously published in Lutes and Hardy (2013). We provide lodgepole pine wood density and decay rate for data collected in three sample years: 1999 (Yr1), 2009 (Yr11) and 2020 (Yr22) at the Tenderfoot Creek Experimental Forest (TCEF) in central Montana. This research addressed four objectives: (1) measure wood density of lodgepole pine (Pinus contorta Douglas ex Loudon var. latifolia Engelm. ex S. Watson) boles that were live and sound when felled; (2) test for significant change in wood density 11 and 22 years after felling; (3) estimate single exponential decay rate (k); and (4) compare wood density across a common 5-category decay classification system (decay classes) (Maser et al., 1979).
Materials and Methods
Study Area
The TCEF is located in central Montana (46.9° N 110.9° W) and is situated at the head of the Tenderfoot Creek drainage. Forest cover is dominated by mixed age-class lodgepole pine with scattered patches of subalpine fir (Abies lasiocarpa) and Engelmann spruce (Picea engelmannii) dominating older stands. Douglas-fir (Pseudotsuga menziesii (Mirb) Franco) is present at the lower elevations of the TCEF. The elevations of sample sites visited in this study ranged between 2,180 and 2,350 m (msl).
Climate
Climate data for the TCEF are available from observations recorded at the Onion Park SNOWTEL site located within the TCEF and were accessed from the National Water and Climate data Center1 and summarized for the period 2002–2019. Average summer (April–September) and winter temperatures (October–March) were 8°C and −4°C, respectively. High temperatures during the warmest parts of summer were typically 19–21°C and often fell below −25°C during the coldest parts of the winter. Average annual water equivalent precipitation was 87 cm – about evenly split between the two seasons.
Sampling
All wood samples in this study were cut from lodgepole pine trees felled when live in 1998. Trees were cut in nine groups located across the TCEF. Group locations had slopes of 0–30% and southwest to northwest aspects. No consideration was made for incorporating microsite conditions in this study, though none of the group locations were in ravines or sites such that there was concern decay rate was influenced by site conditions. These group locations were not selected randomly; rather, they were selected in stands representing a range of forest structures (tree diameter, height and density) representative of the TCEF (Barrett, 1993). Each cutting group was comprised of 7–13 felled trees. We selected trees within each group such that the frequency distribution of diameters at breast height (DBH) of the selected trees would be similar to the diameter distribution of the surrounding stand. These criteria resulted in fewer sample trees in stands with more homogeneous structure. The boles of felled trees were partitioned into 2-m segments (hereafter termed “logs”). Some logs were created by cutting through the entire bole with a chainsaw perpendicular to the central axis, while the remaining boles had the 2-m logs permanently marked with a saw nick. The entire bole was partitioned, thus there was no defined minimum diameter for the logs. Each log was given an identifier consisting of concatenated tree tag number and log number with the logs numbered sequentially starting at the base of the bole. The sample trees yielded 390 logs. After initial samples were collected in 1999, a sampling schedule of the remaining logs was created with random logs selected, without replacement, for sampling at approximately 10-year intervals beginning in 2009.
Log decay class was visually assessed using the classification described in Maser et al. (1979;Table 1). The classification uses a condition criteria assessment of bark, twigs, texture, shape, color of wood, and portion of the log on the ground. An entire log rarely met all the criteria of a class but was assigned the decay class which met most criteria.
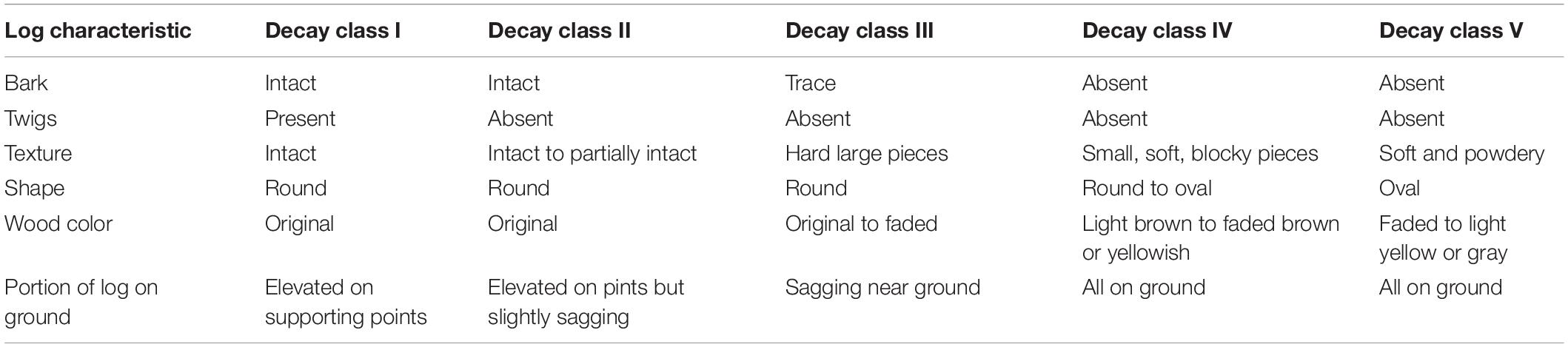
Table 1. The log decay classification characteristics used in this study were reproduced from Maser et al. (1979).
All wood samples were collected by cutting a 5–10-cm thick disk perpendicular to the central axis of the sample log using a chainsaw. The samples were cut from the ends of the logs in Yr1 – less than 10 months after felling – and from the middle of logs in Yr11 and Yr22. The decay class best representing the condition of the log was recorded before removing the sample and piece diameter was measured using a fiexible d-tape after it had been cut from the log. In Yr11 and Yr22 we recorded if the portion of log where the sample was collected was in contact with the ground or suspended above the ground by branches, vegetation, etc. We also recorded if one or both log ends had been completely cut through when initially felled in 1998 (hereafter referred to a “segmented” logs) so we could test if segmentation modified decomposition rate. Sample collection was made more difficult in Yr22 by advanced decay in smaller diameter pieces and portions of the logs in close contact with the forest soil. These pieces broke apart or shredded into small pieces when cut and, despite careful effort, some small, decayed portions were not collected so there is a potential that wood density presented in this study for Yr22 may be a slight overestimate of the actual value. The Yr1 sample included 45 wood samples from the logs of 12 felled tree boles, the Yr11 sample included 25 samples from 16 boles and the Yr22 sample included 29 samples from 25 boles. Samples were stored in sealed plastic bags until lab work began.
Lab Procedure
The same laboratory procedure was used to determine wood density for all samples. All bark was removed as were pieces of dirt, debris, and any loose chips left over from cutting with the chainsaw. The Yr22 samples were wrapped with fine wire to hold all material in place during volume estimation and in the drying oven. Pieces were first weighed and then the volume of the undried samples was determined using water displacement by placing a tub of water on a scale and submersing the piece in the water bath using an apparatus where the submersed portion of the apparatus had a known volume (USDA Forest Products Laboratory, 1956; Williamson and Wiemann, 2010). Piece volume was calculated by assuming 1 g of water displacement was equal to 1 cm3 of wood volume. The sample was weighted again after volume estimation and piece volume was calculated using Eq. 1, which accounted for any water absorbed during the short time the piece was submerged:
where Vn = net volume (cm3), Vg = gross volume (cm3), W1 = piece weight before submersion (g), W2 = piece weight after submersion (g) and Va = volume of the submerged portion of the apparatus (cm3).
All samples were dried at 100°C until weight stabilized - at least 4 days. Wood density was calculated using undried volume and dry weight (Eq. 2).
where D = wood density (g cm–3), W3 = dry piece weight (g) and Vn = net volume (cm3).
Decay rate (k) was determined using the single exponential decay equation described in Olson (1963) but in the form published by Herrmann and Prescott (2008) (Eq. 3).
where k = single exponential decay constant (yr–1), D1 = Wood density at Yr11 or Yr22 (g cm–3), D0 = Wood density at Yr1 (g cm–3) and Yr = time period, in years, between sampling.
To assess how well externally observed log characteristics presented in Maser et al. (1979) predicted wood density we tested the difference in mean wood density across and between decay classes using analysis of variance (ANOVA) and the Tukey– honestly significant difference (HSD).
Results
Histograms of DBH, height, and age for the sample trees are shown in Figure 1. Ages for three of the trees could not be sampled because of heart rot at stump height. Logs sampled at Yr1 displayed no substantial evidence of shrinkage or decay. When the study was initiated (Yr1) the mean sound, bark-free wood density of the 45 samples collected at the TCEF was 0.39 g cm–3.
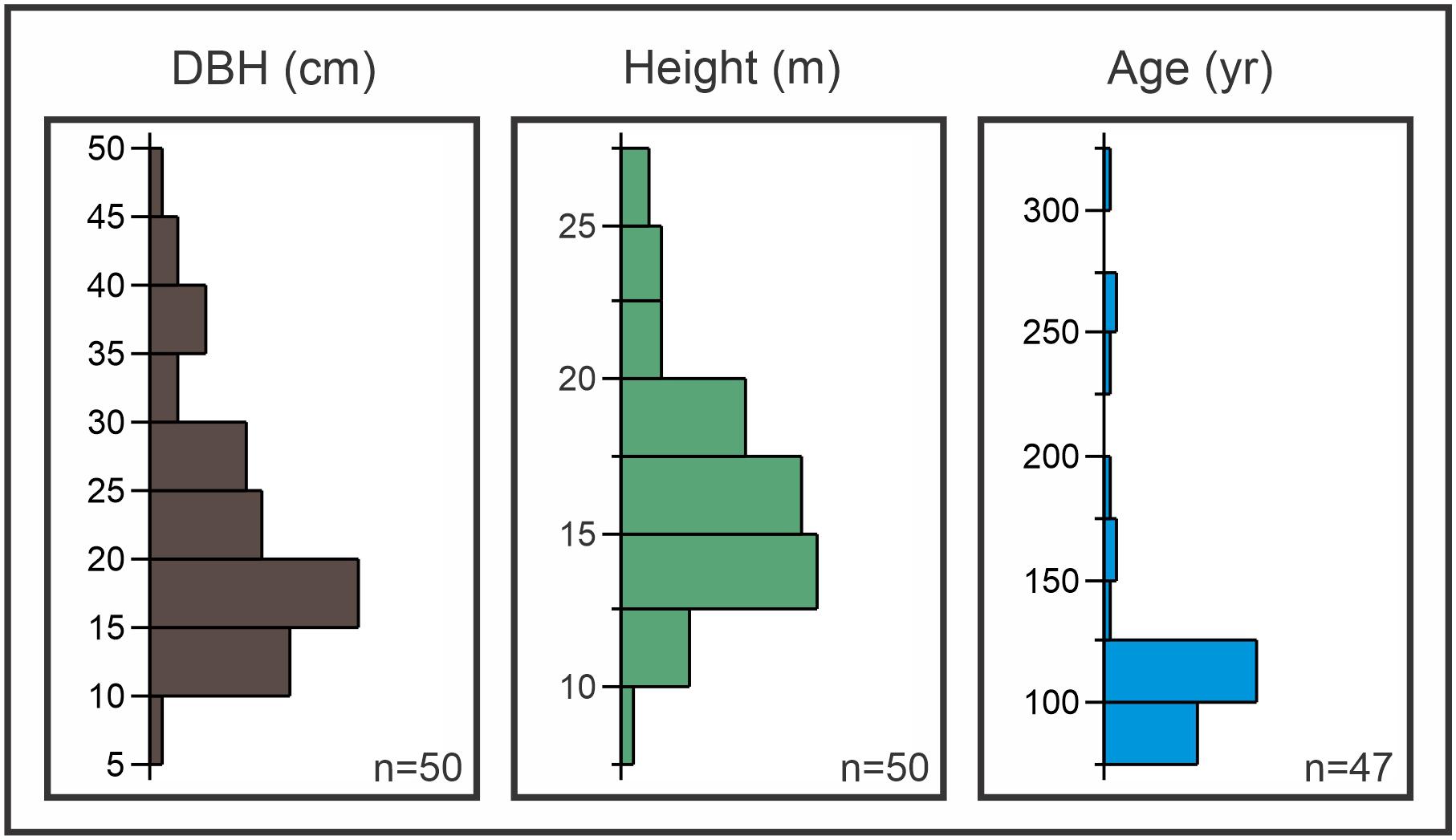
Figure 1. Diameter (DBH), height, and age distribution of the trees selected for this study in 1998. Heart rot prevented aging of three trees.
To limit our estimates of wood density to initially sound wood, we compared the density of samples with heart rot to those without and removed some samples. No samples collected at Yr1 showed evidence of heart rot. Two samples collected in Yr11 had substantial heart rot and the wood density of the pieces was significantly lower than the 23 samples without heart rot [0.24 g cm–3 versus 0.34 g cm–3; t = 6.29; p(t) = < 0.0001] so those two samples were removed from further analysis. Three samples collected in Yr22 from logs >9 m from the stump had minor amounts of heart rot but the mean wood density of the samples was not different than the 26 remaining samples [0.28 g cm–3 versus 0.27 g cm–3; t = 0.40; p(t) = 0.720] so the three samples were retained.
Our 2013 study found 11 samples collected from segmented logs at Yr11 were significantly less dense than logs that were not segmented. Wood density of the 17 samples collected from segmented logs at Yr22 were not significantly different from the 12 samples cut from unsegmented logs [0.267 g cm–3 versus 0.274 g cm–3; t = 0.51, p(t) = 0.615] so the samples cut from segmented logs in Yr22 were retained and, based on the Yr22 finding, we also included the 11 Yr11 samples removed in the 2013 results in the current analysis. The mean diameter of samples from segmented logs and unsegmented logs were not significantly different at Yr11 or Yr22 [F = 0.009, p(F) = 0.925 and F = 2.227, p(F) = 0.147, respectively]. Mean diameter of all samples collected at Yr1, Yr11 and Yr22 were not significantly different [F = 0.07, p(F) = 0.930]. Wood density of the Yr1, Yr11 and Yr22 samples were significantly different [f = 130.75, p(f) = < 0.0001] and significantly different from each other when compared using Tukey HSD (all comparisons p < 0.0001). Wood density and diameter distributions for samples are presented in Table 2. Wood density of samples from logs in contact with the ground collected at Yr11 (n = 14) and the density of samples collected from suspended logs (n = 9) were not significantly different [0.34 g cm–3 versus 0.35 g cm–3, t = 0.64; p(t) = 0.531]. However, at Yr22 wood density of samples collected from logs in contact with the ground (n = 23) were significantly different than the density of samples collected from suspended logs (n = 6) [0.31 g cm–3 versus 0.26 g cm–3; t = 3.91; p(t) = 0.002]. Single exponential decay rate (k) is an indicator of the decomposition rate, where higher k values indicate faster decay. The single exponential decay rate was k = 0.012 yr–1 between Yr1 and Yr11, 0.022 yr–1 between Yr11 and Yr22, and 0.017 yr–1 between Yr1 and Yr22.
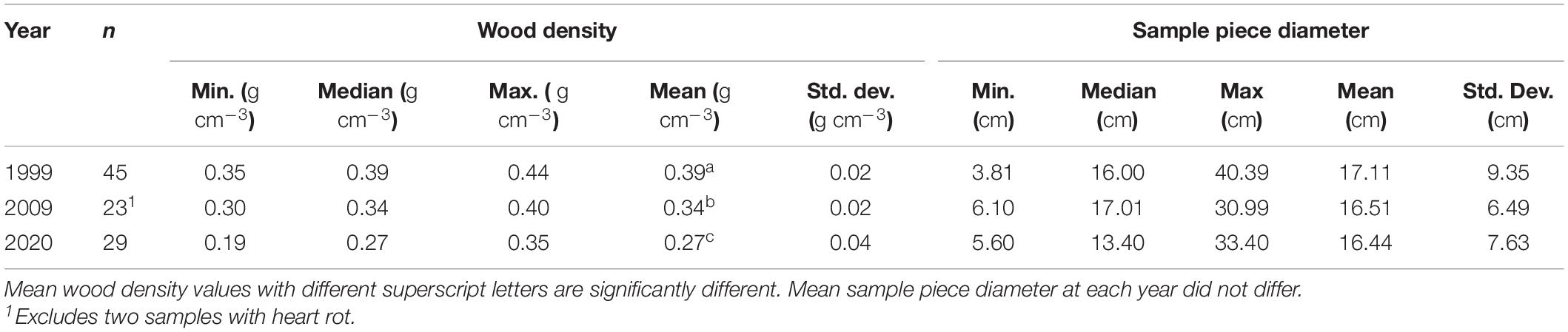
Table 2. Wood density and piece diameter summary statistics for samples collected at Yr1, Yr11, and Yr22.
Wood density was significantly different in three of four observed decay classes [F = 62.25, p(F) < 0.0001]. Mean wood density of samples cut from decay class I and decay class II logs were not significantly different when tested with Tukey HSD (p = 0.566). Wood density of samples cut from decay class III and IV logs were significantly lower than the mean wood density of samples cut from decay class I and II logs and different from each other (p < 0.0001) (Table 3). At Yr1 only decay class I and II logs were observed, at Yr11, only decay class II and III logs were observed and, at Yr22, only decay III and IV logs were observed.
Discussion
Wood density at Yr1 was 0.39 g cm–3, which is within the range of sound lodgepole wood density reported by Maeglin and Wahlgren (1972) for eastern Montana (0.32–0.40 g cm–3) but greater than green lodgepole pine density of 0.38 g cm–3 published in the USDA Forest Service, Wood Handbook (Kretschmann, 2010), and lower than 0.41 g cm–3 published by Koch (1987) for entire stemwood of similar size and age lodgepole pine trees in the same latitudinal range.
After 22 years, we noted confounding effects on wood density of physically segmenting logs into 2-m sections in 1998. Samples collected in Yr11 from segmented logs were significantly less dense than those from unsegmented logs and, in our 2013 paper, we expressed surprise that segmentation would impact wood density 1-m from a cut after just 11 years. At Yr22 we did not find wood density of samples cut from segmented logs to be significantly less than those from un-segmented logs, so we reconsidered the removal of the 11 samples from our analysis in 2013. Wood density and decay rate of downed wood is related to diameter (Harmon et al., 1986; Johnson and Greene, 1991) so we compared mean diameter of the samples collected each year of our study and found no significant difference, consequently we did not consider the difference in wood density to be due to piece size. Herrmann and Prescott (2008) studied mass loss of sections of 20 cm logs in the Kananaskis Valley of Alberta. After 10 years, they found no significant difference in mass loss of center and end sections of lodgepole pine samples but did find a significant difference when making the same comparison of white spruce [Picea glauca (Moench) Voss] and subalpine fir [Abies lasiocarpa (Hook.) Nutt.]. Harmon et al. (1986) and Grier (1978) propose there is lag time before the decay process begins, thus delaying decomposition of recently fallen, broken or cut logs. Given that Herrmann and Prescott (2008) noted insignificant difference of end and center piece lodgepole pine wood density after 10 years, the lag time proposed by Harmon et al. (1986) and Grier (1978), and the insignificant difference in wood density from segmented and unsegmented logs at Yr22, we assumed the wood density difference of segmented logs noted in our 2013 analysis was due to chance and the 11 samples removed from the Yr11 dataset were included in the results presented is this paper. Including the previously excluded Yr11 samples changed the mean and median wood values for Yr11 but did not change the significance of wood density and decay class difference tests.
As Busse (1994) and Wei et al. (1997) have noted, we found that logs in contact with the ground at Yr22 had lower wood density than suspended logs. This was counter to what we observed at Yr11 and may be because the logs sampled at Yr11 were initially supported by vegetation and/or their own branches for some intervening time before decomposition of the supporting structures and snow load pushed the logs to the forest floor (Figure 2). Or the difference may simply be due to chance. At Yr22, sample logs in contact with the ground had a buildup of litter and small woody debris on and adjacent to the log, so they appeared to have been in contact with the surface for some time. We made no attempt to examine decay rates of the two positions of down material individually because we could not determine the duration of log contact with the forest floor. In most cases, when logs were in contact with the ground, the parts of the log nearest the ground were more decayed than other parts. An interesting add-on to this study in the future would examine the wood density in parts close to the forest floor compared to other parts of the log.
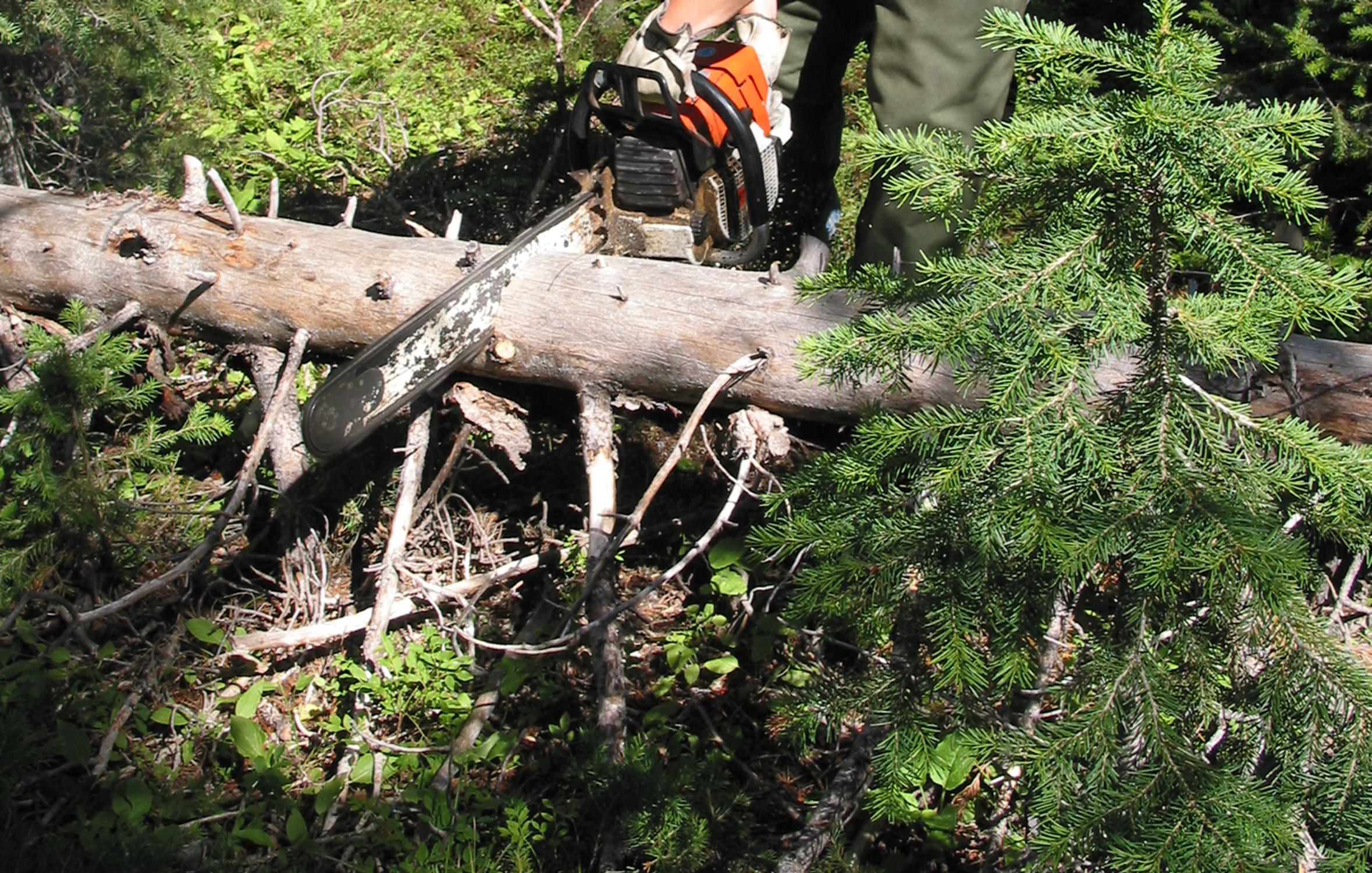
Figure 2. A sample being collected from a log suspended by branches and vegetation in Yr11. Many logs initially suspended were subsequently in contact with the forest floor due to the decomposition of supporting structures and snow load.
Log decay rates between Yr1 and Yr11 and between Yr11 and Yr22 were k = 0.012 yr–1 and k = 0.022 yr–1, respectively. The slower decomposition reported at the first time interval was likely due to the previously mentioned lag period before fungal colonization and associated wood decomposition impacted the density of green wood (Grier, 1978; Harmon et al., 1986). Keane (2008) estimated the 3-year single exponential decay rate of four dead wood components shed from trees at the TCEF: foliage, twigs (0–0.64 cm), branches (0.64–2.54 cm) and large branches (2.54–7.62 cm), to be k = 0.227 yr–1, k = 0.094, k = 0.046 yr–1 and k = 0.045 yr–1, respectively. The decay rates computed for our samples at the TCEF, which were on average greater than the classes reported by Keane (2008), fit the pattern of generally reduced decay rate as piece diameter increases (i.e., surface area to volume ratio decreases) (Harmon et al., 1986). We compared our decay rate estimates against studies conducted in the same general region of North America that included lodgepole pine decay rate for similar time periods. Busse (1994) estimated the time since trees had fallen by dating scars on adjacent trees and calculated decay rates for lodgepole pine logs in central Oregon of k = 0.014 yr–1 and k = 0.022 yr–1 for class I logs aged 0–7 and class II logs aged 10–19 years, respectively. Fahey (1983) studied lodgepole pine boles in Wyoming and calculated mean decay rate of k = 0.0198 yr–1 after 10 years and 0.0174 yr–1 after 15 years. Herrmann and Prescott (2008) collected samples from standing dead or recently fallen trees. They physically removed a 3 cm long segment from the center of 20 cm samples, weighed the center pieces, screwed the entire 20 cm sample back together and placed them on the forest floor. After 10 and 20 years, they reported decay rates of k = 0.073 yr–1 and k = 0.072 yr–1, respectively. In central British Columbia, Wei et al. (1997) studied aboveground 10 – 20 cm diameter woody debris 5–10 years after harvesting and wildfire disturbances, and calculated decay rates of k = 0.021 yr–1 and k = 0.004 yr–1, respectively. They proposed the faster decay rate on the harvested sites was because logs had been in contact with the ground longer. Johnson and Greene (1991) studied “self-thinned” downed boles in five stands in southern Alberta and found the decay rate ranged from k = 0.0171 yr–1 to k = 0.0299 yr–1 for lodgepole pine boles that had been on the ground 15–25 years. Making the direct comparison of decay rate in our results and those from other studies is hard because a variety of methods were used to determine piece age and initial wood density. For instance, it is not clear if any of the samples in the comparison studies were collected from boles that were living immediately before becoming down material, as our samples were. With that in mind, our results were comparable for specific time periods reported in the referenced studies - with the exception of Herrmann and Prescott (2008; Figure 3).
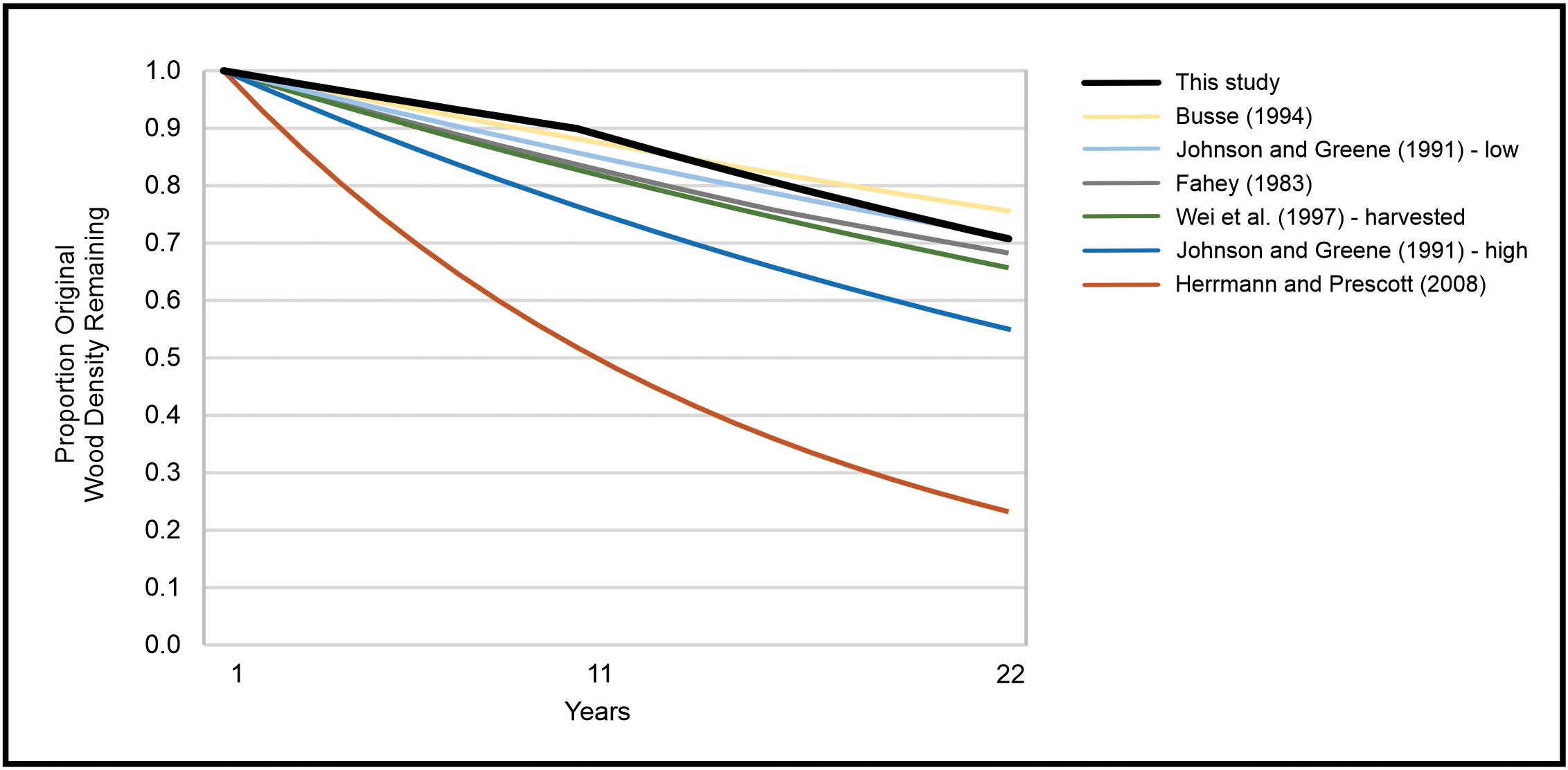
Figure 3. Comparison of the proportion of wood density remaining in this and referenced studies using the published decay rates over a 22-year period. The 11 Yr and 22 Yr decay rates from this study are graphed for the appropriate periods. Johnson and Greene (1991) published high and low rates. The decay rate from Wei et al. (1997) is for material sampled on harvest sites.
There was significant difference in wood density in some decay classes in this study; however, we noted limitations for using decay class as a replacement for determining wood density in the lab. Internal conditions, like heart rot, may lead to significantly lower wood density but will be difficult to detect using external log characteristics. Additionally, we noted a wide range of wood density within decay classes; we found no significant difference in decay class I and II wood density and we collected no decay class V samples. Previous work has noted broad overlap in wood density between classes (Busse, 1994; Pyle and Brown, 1999; Harmon et al., 2008; Larjavaara and Muller-Landau, 2010), thus limiting the usefulness of estimating wood density using decay class; however, Creed et al. (2004) noted that red spruce (Picea rubens Sarg.) and Fraser fir [Abies fraseri (Purch) Poir.] wood density could be reasonably predicted using decay class when studying the species individually.
The sample size for this study was limited by the resources available for field collection and lab analysis. We also were constrained by the total number of samples available – including those to be collected in future work. These limitations precluded us from studying how wood density varied by stand structure and undertaking nutrient analysis. While our sample size was relatively small it was similar to the comparison studies we reference, except for Johnson and Greene (1991) and Busse (1994) who collected over 100 samples.
The influence of slope, slope shape and aspect were not included in any analysis of this study thus any impacts these site characteristics had on the results are unknown. We studied only material that was initially live so we have not attempted to describe decay rate variability due to factors such as log position, location, condition (other than initially sound) or boles the were standing dead before falling and becoming down material.
Future Study
Study logs will be resampled in 2030 to continue examining the relationship of wood density, log position, segmentation of logs and decay class. We surmised that log segmentation had not significantly increased wood decay rate 22 years after felling sample trees but it likely will at some future time so the impact of segmentation will need to be continually assessed and identified to remove bias, as well as to recommend possible protocols for future research. Note that removing wood samples from logs is also a form of segmentation so currently un-segmented logs will be segmented in future sample visits, thus potentially increasing the decay rate of adjacent logs. After just 20 years, we found that some pieces were easily torn apart by the chainsaw, necessitating additional care when cutting and collecting the entire sample. This problem will only become more acute as logs become further decayed and fragmented.
Subsequent sampling will refine decay rate at the TCEF and further assess if the slower decay rate found in the initial 10 years of the study was due to the lag suggested by Harmon et al. (1986) and Grier (1978). The evidence of potential lag time in decomposition suggests alternative loss functions for green wood such as Weibull (Fraver et al., 2013), sigmoid (Freschet et al., 2012) or negative exponential with lag (Harmon et al., 2000) may better estimate decay rate at TCEF and should be tested. As decomposition progresses to create decay class V logs, an additional assessment of the relationship between wood density and decay class can be undertaken. To date, this study has focused only on wood density but, as the study logs decay further, changes in log shape should be assessed using collapse ratio (Fraver et al., 2013) to estimate the change in log volume, thus biomass.
Data Availability Statement
The raw data supporting the conclusions of this article will be made available by the authors, without undue reservation.
Author Contributions
DL and CH designed the research and wrote the manuscript. DL led the collection and analysis of wood samples. Both authors contributed to the article and approved the submitted version.
Funding
Funding was provided by the USDA, Forest Service, Rocky Mountain Research Station, Fire, Fuel and Smoke Science Program and the Forest Service, Office of Fire and Aviation Management.
Conflict of Interest
The authors declare that the research was conducted in the absence of any commercial or financial relationships that could be construed as a potential conflict of interest.
Acknowledgments
We thank Helen Smith and Emily Heyerdahl for helping with data collection, and Jim Reardon for providing lab space and measurement equipment.
Footnotes
References
Barrett, S. W. (1993). Fire History of the Tenderfoot Creek Experimental Forest. Final Report No. INT-92679-RJVA. Missoula, MT: U.S. Department of Agriculture, Forest Service, Rocky Mountain Research Station, Fire Sciences Laboratory.
Bate, L. J., Torgersen, T. R., Wisdom, M. J., and Garton, E. O. (2004). Performance of sampling methods to estimate log characteristics for wildlife. For. Ecol. Manage. 199, 83–102. doi: 10.1016/j.foreco.2004.04.021
Brown, J. K. (1971). A planar intersect method for sampling fuel volume and surface area. For. Sci. 17, 96–102.
Busse, M. D. (1994). Downed bole-wood decomposition in lodgepole pine forests of central oregon. Soil Sci. Soc. Am. J. 58, 221–227. doi: 10.2136/sssaj1994.03615995005800010033x
Creed, I. F., Webster, K. L., and Morrison, D. L. (2004). A comparison of techniques for measuring density and concentrations of carbon and nitrogen in coarse woody debris at different stages of decay. Can. J. For. Res. 34, 744–753. doi: 10.1139/x03-212
Currie, W. S., and Nadelhoffer, K. J. (2002). The imprint of land-use history: patterns of carbon and nitrogen in downed woody debris at the harvard forest. Ecosystems 5, 446–460. doi: 10.1007/s10021-002-1153-x
Fahey, T. J. (1983). Nutrient dynamics of aboveground detritus in Lodgepole pine (Pinus contorta ssp. latifolia) ecosystems, Southeastern Wyoming. Ecol. Monogr. 53, 51–72. doi: 10.2307/1942587
Fischer, W. C. (1981). Photo Guides for Appraising Downed Woody Fuels in Montana Forests: How They Were Made. Research Note No. INT-RN-299. Ogden, UT: USDA, Forest Service, Intermountain Forest and Range Experiment Station, 12.
Fraver, S., Milo, A. M., Bradford, J. B., D’Amato, A. W., Kenefic, L., Palik, B. J., et al. (2013). Woody debris volume depletion through decay: implications for biomass and carbon accounting. Ecosystems 16, 1262–1272. doi: 10.1007/s10021-013-9682-z
Freschet, G. T., Weedon, J. T., Aerts, R., van Hal, J. R., and Cornelissen, J. H. C. (2012). Interspecific differences in wood decay rates: insights from a new short-term method to study long-term wood decomposition. J. Ecol. 100, 161–170. doi: 10.1111/j.1365-2745.2011.01896.x
Gove, J. H., Ringvall, A., Ståhl, G., and Ducey, M. J. (1999). Point relascope sampling of downed coarse woody debris. Can. J. For. Res. 29, 1718–1726. doi: 10.1139/x99-119
Grier, C. C. (1978). A Tsugaheterophylla – Piceasitchensis ecosystem of coastal Oregon: decomposition and nutrient balances of fallen logs. Can. J. For. Res. 8, 198–206. doi: 10.1139/x78-031
Grier, C. C., and Logan, R. S. (1977). Old-growth pseudotsuga menziesii communities of a Western Oregon watershed: biomass distribution and production budgets. Ecol. Monogr. 47, 373–400. doi: 10.2307/1942174
Gurnell, A. M., Gregory, K. J., and Petts, G. E. (1995). The role of coarse woody debris in forest aquatic habitats: implications for management. Aquat. Conserv. 5, 143–166. doi: 10.1002/aqc.3270050206
Harmon, M. E., Fasth, B. G., Yatskov, M., Kastendick, D., Rock, J., and Woodall, C. W. (2020). Release of coarse woody detritus-related carbon: a synthesis across forest biomes. Carbon Balance Manag. 15:1. doi: 10.1186/s13021-019-0136-6
Harmon, M. E., Franklin, J. F., Swanson, F. J., Sollins, P., Gregory, S. V., Lattin, J. D., et al. (1986). Ecology of coarse woody debris in temperate ecosystems. Adv. Ecol. Res. 15, 133–302. doi: 10.1016/s0065-2504(08)60121-x
Harmon, M. E., and Hua, C. (1991). Coarse woody debris dynamics in two old-growth ecosystems. BioScience 41, 604–610. doi: 10.2307/1311697
Harmon, M. E., Krankina, O. N., and Sexton, J. (2000). Decomposition vectors: a new approach to estimating woody detritus decomposition dynamics. Can. J. For. Res. 30, 76–84. doi: 10.1139/x99-187
Harmon, M. E., Woodall, C. W., Fasth, B., and Sexton, J. (2008). Woody Detritus Density and Density Reduction Factors for Tree Species in the United States: A Synthesis. General Technical. Report No. NRS-29. Newton Square, PA: U.S. Department of Agriculture, Forest Service, Northern Research Station. doi: 10.2737/nrs-gtr-29
Herrmann, S., and Prescott, C. E. (2008). Mass loss and nutrient dynamics of coarse woody debris in three Rocky Mountain coniferous forests: 21 year results. Can. J. For. Res. 38, 125–132. doi: 10.1139/x07-144
Johnson, E. A., and Greene, D. F. (1991). A method for studying dead bole dynamics in Pinus contortavar latifolia – picea engelmanniiforests. J. Veg. Sci. 2, 523–530. doi: 10.2307/3236034
Keane, R. E. (2008). Surface Fuel Litterfall and Decomposition in the Northern Rocky Mountains, U. S. A. Research Paper No. RMRS-RP-70. Fort Collins, CO: U.S. Department of Agriculture, Forest Service, Rocky Mountain Research Station. doi: 10.2737/rmrs-rp-70
Keane, R. E., and Dickinson, L. J. (2007). The Photoload Sampling Technique: Estimating Surface Fuel Loadings From Downward-Looking Photographs of Synthetic Fuelbeds. General Technical Report No. RMRS-GTR-190. Fort Collins, CO: U.S. Department of Agriculture, Forest Service, Rocky Mountain Research Station. doi: 10.2737/rmrs-gtr-190
Koch, P. (1987). Gross Characteristics of Lodgepole Pine Trees in North America. General Technical Report No. INT-227. Ogden, UT: U.S. Dept. of Agriculture, Forest Service, Intermountain Research Station. doi: 10.5962/bhl.title.109398
Kretschmann, D. E. (2010). Chapter 5. Mechanical Properties of Wood, in Wood handbook: Wood as an engineering material. General Technical Report No. FPL-GTR-190. Madison, WI: USDA, Forest Service, Forest Products Laboratory.
Larjavaara, M., and Muller-Landau, H. C. (2010). Comparison of decay classification, knife test, and two penetrometers for estimating wood density of coarse woody debris. Can. J. For. Res. 40, 2313–2321. doi: 10.1139/x10-170
Lopes Queiroz, G., McDermid, G., Linke, J., Hopkinson, C., and Kariyeva, J. (2020). Estimating coarse woody debris volume using image analysis and multispectral LiDAR. Forests 11:141. doi: 10.3390/f11020141
Lutes, D. C., and Hardy, C. C. (2013). Lodgepole pine bole wood density 1 and 11 years after felling in Central Montana. West. J. Appl. Forestry 28, 116–120. doi: 10.5849/wjaf.12-033
Maeglin, R. R., and Wahlgren, H. E. (1972). Western Wood Density Survey; Report Number 2. Research Paper No. FPL-RP-183. Madison, WI: U.S. Department of Agriculture, Forest Service, Forest Products Laboratory, 24.
Marchi, N., Pirotti, F., and Lingua, E. (2018). Airborne and terrestrial laser scanning data for the assessment of standing and lying deadwood: current situation and new perspectives. Remote Sens. 10:1356. doi: 10.3390/rs10091356
Maser, C., Anderson, R., Cromack, K. Jr., Williams, J. T., and Martin, R. E. (1979). “Dead and down woody material,” in Wildlife Habitats in Managed Forests, the Blue Mountains of Oregon and Washington: Agricultural. Handbook No. 553, ed. J. W. Thomas (Washington, DC: USDA Forest Service).
Maser, C., Tarrant, R. F., Trappe, J. M., and Franklin, J. F. (1988). From the Forest to the Sea: A Story of Fallen Trees. General Technical Report No. PNW-GTR-229. Portland, OR: U.S. Department of Agriculture, Forest Service, Pacific Northwest Research Station. doi: 10.2737/pnw-gtr-229
Maser, C., and Trappe, J. M. (1984). The Seen and Unseen World of the Fallen Tree. General Technical Report No. PNW-GTR-164. Portland, OR: U.S. Department of Agriculture, Forest Service, Pacific Northwest Research Station. doi: 10.2737/pnw-gtr-164
Olson, J. S. (1963). Energy storage and the balance of producers and decomposers in ecological systems. Ecology 44, 322–331. doi: 10.2307/1932179
Ottmar, R. D., Vihnanek, R. E., and Wright, C. S. (1998). Stereo Photo Series for Quantifying Natural Fuels: Mixed-Conifer With Mortality, weStern Juniper, Sagebrush, and Grassland types in the Interior Pacific Northwest. PMS 830. NFES 2580, Vol. 1. Boise, ID: National Wildfire Coordinating Group, 73.
Pyle, C., and Brown, M. M. (1999). Heterogeneity of wood decay classes within hardwood logs. For. Ecol. Manag. 114, 253–259. doi: 10.1016/s0378-1127(98)00356-9
Ståhl, G. (1998). Transect relascope sampling – a method for the quantification of coarse woody debris. For. Sci. 44, 58–63.
Stevens, V. (1997). The Ecological Role of Coarse Woody Debris, An Overview of the Ecological Importance of CWD in BC forests, Working Paper No. 30. Victoria, BC: Ministry of Forest Research Program.
USDA Forest Products Laboratory (1956). Methods for Determining the Specific Gravity of Wood. Technical Note No. B-14. Madison, WI: USDA, Forest Service, Forest Products Laboratory, 6.
Warren, W. G., and Olsen, P. F. (1964). A line intersect technique for assessing logging waste. For. Sci. 10, 267–276.
Weedon, J. T., Cornwell, W. K., Cornelissen, J. H. C., Zanne, A. E., Wirth, C., and Coomes, D. A. (2009). Global meta-analysis of wood decomposition rates: a role for trait variation among tree species? Ecol. Lett. 12, 45–56. doi: 10.1111/j.1461-0248.2008.01259.x
Wei, X., Kimmins, J. P., Peel, K., and Steen, O. (1997). Mass and nutrients in woody debris in harvested and wildfire-killed lodgepole pine forests in the central interior of British Columbia. Can. J. For. Res. 27, 148–155. doi: 10.1139/x96-169
Keywords: wood density, decay rate, decay class, decomposition, down woody material, lodgepole pine
Citation: Lutes DC and Hardy CC (2021) Lodgepole Pine Bole Wood Density and Decay Rate 1, 11, and 22 Years After Felling in Central Montana, United States. Front. For. Glob. Change 4:687567. doi: 10.3389/ffgc.2021.687567
Received: 29 March 2021; Accepted: 07 June 2021;
Published: 29 June 2021.
Edited by:
Verena C. Griess, ETH Zürich, SwitzerlandReviewed by:
Jarosław Lasota, University of Agriculture in Krakow, PolandYuji Kominami, Forestry and Forest Products Research Institute, Japan
Copyright © 2021 Lutes and Hardy. This is an open-access article distributed under the terms of the Creative Commons Attribution License (CC BY). The use, distribution or reproduction in other forums is permitted, provided the original author(s) and the copyright owner(s) are credited and that the original publication in this journal is cited, in accordance with accepted academic practice. No use, distribution or reproduction is permitted which does not comply with these terms.
*Correspondence: Duncan C. Lutes, ZHVuY2FuLmx1dGVzQHVzZGEuZ292