- 1Facultad de Ciencias Exactas y Naturales, Universidad Nacional de La Pampa, Santa Rosa, Argentina
- 2Facultad de Agronomía, Universidad Nacional de La Pampa, Santa Rosa, Argentina
- 3Consejo Nacional de Investigaciones Científicas y Técnicas (CONICET), Buenos Aires, Argentina
Land-use change and specifically a change in the type of vegetation cover affects soil morphology, chemistry, biology, and nutrient regimes. Numerous studies have documented that in land-use conversions from agricultural land to forest, or from plantations to restored natural savanna most soil attributes and functions undergo changes. The purpose of the present study was to evaluate the changes brought about by afforestation of degraded croplands and to understand the impact of forest vegetation on soil evolution in a semiarid region where soils originally co-evolved with a savanna biotope. We used long-term experiments (>40 years) of five tree species: Pinus halepensis (PH), Pinus halepensis inoculated with ecto-mycorrhiza at planting (PM), Pinus pinea (PP), Eucalyptus spp. (E), and Gleditsia triacanthos (G) and compared these to an agricultural soil (A) at the same site near Santa Rosa, La Pampa in the semiarid center of Argentina. Soil profiles were described, and samples taken for chemical and physical analyses of soil properties [organic matter (OM), pH, cation exchange capacity (CEC) and exchangeable cations, particle size distribution (texture), aggregate stability (MWD), bulk density (BD), porosity (TP), and water holding capacity (WHC)]. We found a strong effect of tree species on soil profile morphology, even taxonomy, and on all studied variables. PM and G had highest OM, CEC, neutral pH, higher TP, WHC, while PH, PP, and E had acid pH, lower base saturation, OM, TP, and WHC. The effect of tree species on the soil profile was noticeable a depth of about 40 cm, comprising the A and AC, but not the C horizons. The results showed that to obtain reasonable results of OM sequestration under forest systems, tree species should be chosen to include legumes to improve C/N stoichiometry for C fixation, or inoculation with mycorrhiza to promote microbial transformation of forest litter.
Introduction
Land-use change and specifically a change in the type of vegetation cover affects soil morphology, chemistry, biology, and nutrient regimes. Numerous studies have documented that in land use conversions from agricultural to forest systems (Lemenih, 2004), or from plantations to restored natural savanna vegetation (Johnson-Maynard et al., 2002) most soil attributes and functions undergo changes. These changes are driven by environmental factors (climate, parent material) and by the forest species and management. Different forest species resulted in a wide span of accrued soil organic carbon levels (Crow et al., 2008; Gurmesa et al., 2013; Prescott and Grayston, 2013; Tang and Li, 2013; Vesterdal et al., 2013). The effect of species also was documented for changes in soil physical and chemical properties such as pH, CEC, and nutrient status (i.e., N and P stocks) (De Vries et al., 2003). A novel concept regarding soil development or soil evolution is defining soils as extended composite phenotypes that co-evolve with the biota associated to each soil in a process of natural selection that provides benefits to those organisms that adapt or “engineer” special habitats or niches within a given soil environment (Phillips, 2009). This concept, based on the notion of soils as “biomantles” or “excited skin” of our planet (Phillips, 2009), is much more dynamic than the traditional view of unidirectional soil development, dependent on the combination of soil forming factors present in a determined location. If soils were indeed extended composite phenotypes, a strong biological change such as caused by the substitution of the dominant vegetation species, should be reflected by significant qualitative changes in soils (in addition to quantitative changes in soil properties) as for instance an effect on soil morphology. Some studies already pointed out that Corsican pine (Pinus nigra) caused incipient podzolization in soils of Tuscany, while at the same time this process was not observed under silver fir (Certini et al., 1998).
In many areas of the world afforestation was promoted to restore degraded agricultural lands (Masera et al., 2003; Mendham et al., 2003; Farley et al., 2004; Niu and Duiker, 2006; Laik et al., 2009; Wei et al., 2013). However, in many semiarid regions of the world original landcover were grasslands or savannas, under which very fertile soils, typically Mollisols, evolved. The Haplustolls, Calciustolls, and Paleustolls of the central Argentinean Dry Pampas developed under savanna vegetation, which consists of a dense grass layer of C3 short bunchgrasses, forbs, and legume trees and shrubs. These soils were converted to croplands by immigrants during the first half of the past century, and like the history of the North American farmlands, prolonged droughts triggered severe soil loss through wind erosion during the 1930s and 1950s. Afforestation was promoted to recover the degraded soils and the most ubiquitous species were Aleppo pine, Stone pine, Black locust, and Eucalypt. A previous study on afforested soil in this region (Riestra et al., 2012) already reported on the effect of these species on some soil chemical and physical properties, concluding that legumes, such as Black locust, or the inoculation with mycorrhiza in Stone pine stands were favorable for carbon sequestration and soil structure.
The present study proposed to deepen our understanding of the changes brought about by afforestation of degraded croplands and to understand the impact of forest vegetation on soil evolution and profile characteristics in a semiarid region where soils originally co-evolved with a savanna biotope. We therefore hypothesized that soils under different forest species would show divergence in their chemical, physical, and biological properties, even in the relatively short time span after this land cover conversion took place. We also wondered whether the 50-year time span was long enough to bring about morphological changes in the soil profiles, and to which profile depth the effect of forest species would reach.
Materials and Methods
Site Description
The study was carried out at the provincial forest nursery at Santa Rosa, La Pampa (Figure 1; Coordinates: 36°34′17″S; 64°16′56″W), on a sandy loam Entic Haplustoll (Usda and Nrcs, 2010). The soils are formed on quaternary aeolian loess deposits forming extensive undulating plains (Zárate and Tripaldi, 2012) in a semiarid climate with a mean annual temperature of 15.5°C and mean annual rainfall of 750 mm (Casagrande et al., 2006). The original vegetation is a dry forest or savanna composed of mainly legume trees of the Prosopis species (e.g., Prosopis Caldenia, P. flexuosa) and a dense grass cover (e.g., Stipa tenuis, Poa ligularis, Piptochaetium napostaensis).
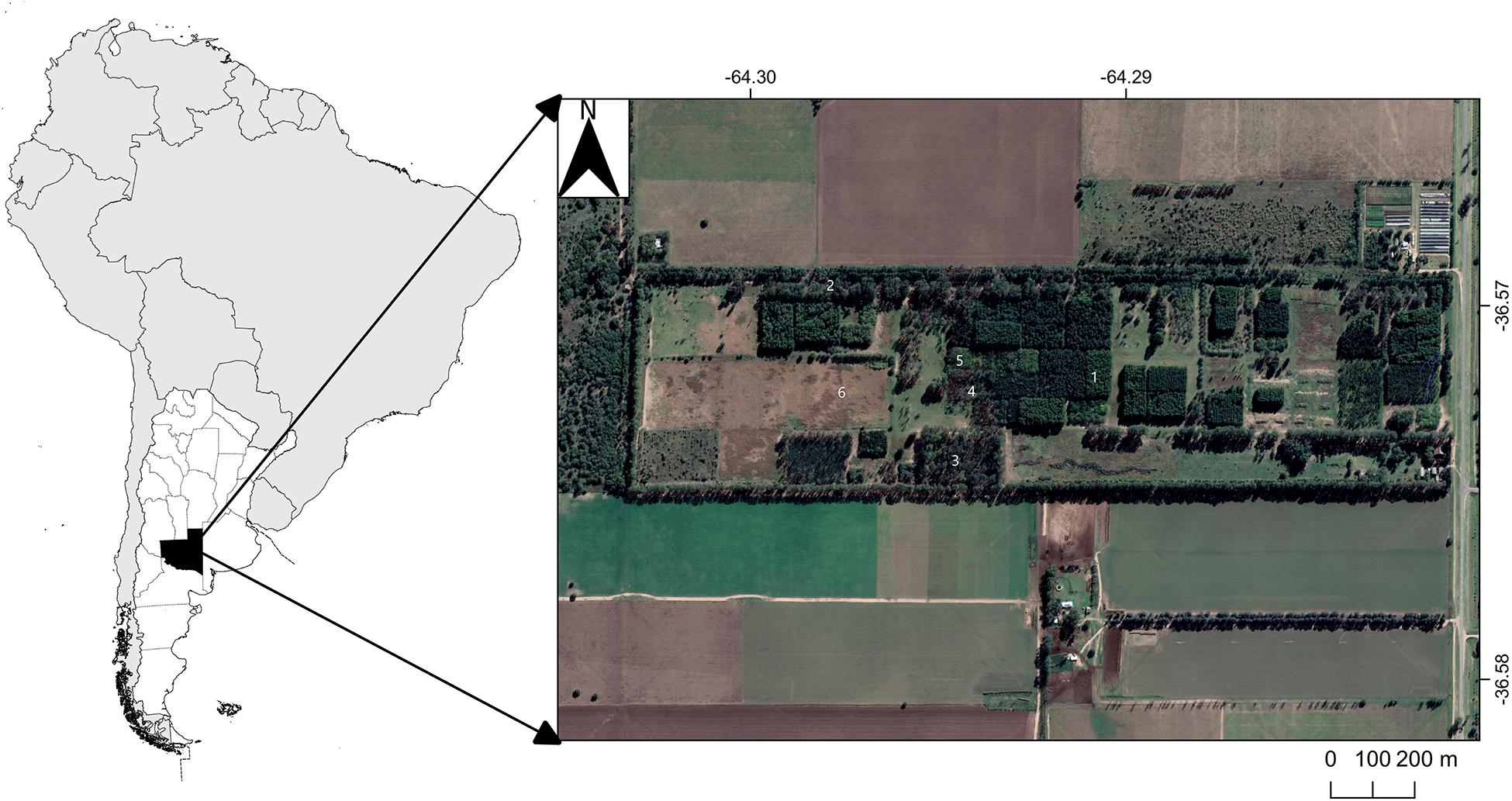
Figure 1. Map of location of the sampling area and aerial view of the afforestation plots near Santa Rosa, La Pampa Argentina. The numbers indicate the treatments: (1) Aleppo pine (PH), (2) Aleppo pine with ectomycorrhiza (PM), (3) Eucalypt (E), (4) Stone pine (PP), (5) Black locust (G), (6) Agriculture (Ag).
The treatments corresponded to four tree species: Aleppo pine, Pinus haleppensis (PH) and Pinus haleppensis inoculated with ecto-mycorrhiza at planting (PM); Stone pine, Pinus pinea (PP); Eucalypt, Eucalyptus spp. (E); and Black locust, Gleditsia triacanthos (G). The inoculated Aleppo pine treatment was included in the forest nursery trials since it was thought that this might improve seedling vigor, seedlings were planted in soil inoculated with mycorrhiza mycelia that were originally brought from Croatia (Poduje, personal communication). It is to be noted that in pine stands that were not grown on inoculated substrate no evidence of fungi can be found. While there most likely exist native ectomycorrhiza in the soils, apparently these do not colonize conifers, perhaps since the native vegetation does not include any coniferous species.
In addition, a control plot which had been cultivated and planted to annual crops for the same time (Ag) was sampled, resulting in six treatments. The size of each forested plot was approximately 3 ha, and the tree distance were 3 by 3 m for PH and PM, 3.5 by 3.5 m for PP, 3 by 3.5 m for E, and 4 by 4 m for G (see Supplementary Material for a map of the forest plots). All stands were more than forty years old at sampling, and had only been pruned, but never cut or thinned. No weed control had been practiced, and the lighter stands had a sparse grass cover.
Soil Sampling and Analytical Procedures
At each treatment site surface samples at two depth layers (0–6 and 6–12 cm) were collected with steel cylinders with a fixed volume of 1,060 cm3. For each treatment, 8 replicate soil samples were taken at random, taking care that in the forest treatments sampling points were equidistant between tree trunks. These samples were used to represent the A-horizon’s chemical properties, considering that changes in land-use are most like to be detected in the uppermost soil layers, and that under soils under native and implanted forest show a strong stratification of SOC and related properties (Noellemeyer et al., 2008, 2006; Gili et al., 2010). In addition, a soil pit was dug in each stand and the soil profile was described according to the soil survey manual (National Soil Survey Center, 2012; Soil Survey Staff, 2014). Soil samples were taken from the center of each horizon for physical-chemical analysis. The sampling was carried out in spring/summer 2008 and refers to the same treatments described by Riestra et al. (2012).
Soil samples were oven-dried at 105°C to constant weight, and the dry weight of each soil core was used to calculated bulk density (BD, Mg m–3) as follows:
Where M is the dry weight of the soil contained in the cylinder, and V is the volume of the cylinder. Soils in this region do not contain stones or gravel, wherefore these are not considered in the determination of BD.
Part of the soil sample was sieved to 2 mm, and roots or any other plant residue particles were excluded from the samples for analytical determinations. Total organic carbon (OC, g kg–1) was determined by wet oxidation with potassium dichromate in sulfuric acid and colorimetric valuation (Skjemstad et al., 2003) and total nitrogen was determined by the semimicro Kjeldahl procedure. Both were made on 0–6 and 6–12 cm samples separately, whereas pooled samples from both depth layers (0–12 cm) were used for all other determinations. Particle size fractions were determined by the Robinson Pipette method and soil texture was obtained with the textural triangle (Gee and Bauder, 1986). Particle density (PD, Mg m–3) analysis was carried out using the standard pycnometer technique (Blake and Hartge, 1986), and total porosity (TP,%) was calculated as:
Field capacity moisture content (FCM,%), and the moisture retention at different tensions were determined using a sandbox and pressure plates (Reynolds, 2007a).
The remaining sample that was not sieved through 2 mm was placed in a battery of sieves with mean diameters of 8, 4, 3, 2, and 1 mm. The soil aggregates that were retained by the largest sieve corresponded to the > 8 mm aggregate class, while the ones that passed the 1 mm sieve were < 1 mm class; both fractions were weighed and then discarded. The remaining aggregate size fractions were: 4–8, 3–4, and 2–3, which were utilized for determining the structural stability of the soil (De Boodt et al., 1967). The structural stability index (SSI) was calculated as the inverse of the mean weight diameter loss of the different aggregate size fractions after wet sieving.
Additional 6 cylinders were extracted (244.1 cm–3) in the A-horizons in order to determine the saturated hydraulic conductivity (K) in the laboratory, using a constant load permeameter in undisturbed samples (Reynolds, 2007a). Infiltration rate was determined using the double ring infiltrometer (Reynolds, 2007b), with a 21.5 cm inner diameter and 30 cm outer diameter cylinder inserted 10 cm into the soil. Four measurements were carried out at each site, on a 20 m transect, with infiltrometers equidistant at 5 m and at equal distances to tree trunks.
Statistical Analysis
Data obtained from soil samples of the horizons described in the soil profiles were not replicated and therefore no statistical analysis was performed on them while the samples taken by the steel cylinder had 8 replicates each and were analyzed by one-way analysis of variance and Tukey test (p < 0.05) to determine significant differences between means. A principal component analysis (PCA) was carried to with the data of pH, CEC, C/N, BD, SSI, and IR from replicated samples to establish the relationships among variables and between these variables and the different treatments. All statistical analyses were carried out with InfoStat/P software (Di Rienzo et al., 2017).
Results
Soil Morphology
Table 1 shows the morphological and chemical characteristics of soil profiles under different forest treatments compared with an agricultural soil as control. Profiles were similar with regards to their total depth (106–140 cm), except for G, which was 200 cm deep, and they also had a similar horizon sequence of A, AC, C, Ck. All forest soils, except G, had organic horizons above the mineral A-horizon, varying in thickness between 2 and 5 cm, while E also had an Oe –horizon. PH and G developed stronger A-horizons (A1, A2), which reached a depth of 30 and 50 cm, respectively, while all other profiles had A-horizons that did not exceed 24 cm depth.
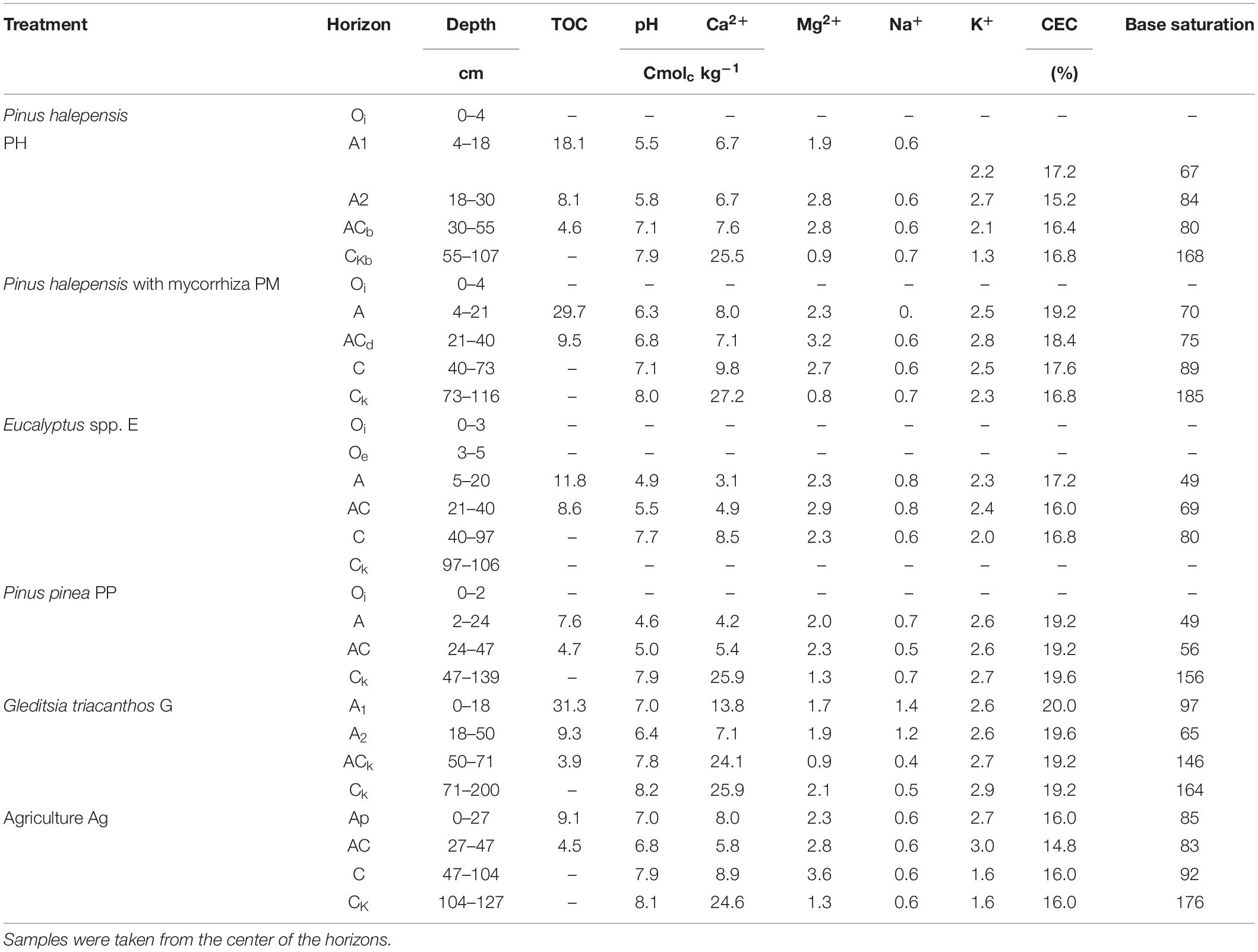
Table 1. Properties of the soil profiles under different forest species (total organic carbon-TOC; cation exchange capacity-CEC).
The color of soil horizons varied between 10 YR 3/2 moist, and 10 YR 3/3 dry in surface soil, and 10 YR 3/4–5/3 moist, and 10 YR 4/4–5/3 dry in C-horizons (data not shown). Aggregate structure followed a gradient from sub-angular blocks in A-horizons to angular blocks in deeper ones. The exception was G where a granular structure was found in the A1 horizon. This treatment also showed most abundant roots in this horizon while these were very scarce in all other profiles.
Soil Chemical Properties
Base saturation (BS) and carbon contents (C) of all A-horizons, except E and PP, were well above the established limits for Mollisols (> 50% BS and ≥ 1% organic matter) (Table 1). The highest value for BS in A-horizons was found in G (97%), followed by Ag (85%) and PH (70%), while the lowest values corresponded to PP (49%) and E (49%), just below the threshold to be classified as a mollic epipedon. These data matched with the pH values, which were highest in Ag and G (both 7.0), whilst all other forest A-horizons had more acidic pH, with lowest values in PP (4.6) and E (4.9). The acidification of these profiles reached a depth of about 30–40 cm, below which all profiles had similar pH values of between 7 and 8. Cation exchange capacity values also were higher in all A-horizons of forest soils, ranging from 17 to 20 Cmolc kg–1, compared to the Ag treatment (16 Cmolc kg–1). Highest exchangeable Ca concentrations were found in the A-horizon of G (13.8 Cmolc kg–1), and lowest ones corresponded to PP and E (4.2 and 3.1 Cmolc kg–1, respectively).
The A-horizons also varied widely in their C contents in the upper 6 cm, ranging from 7.6 g kg–1 in PP to 31.3 g kg–1 in G (Table 1), and all forest treatments except PP, had higher C contents than Ag (9.1 g kg–1).
The differences between forest and agriculture soils, as well as among forest profiles were noticeable down to the AC-horizons, below this depth (C horizons) all profiles showed similar values in BS, C, pH, CEC, and exchangeable Ca. The high Ca values of C-horizons, and correspondingly high BS (>100%) were due to free Ca-carbonate that was not eliminated before exchangeable cation extraction. The presence of free Ca-carbonate in the soil mass varied among profiles from a depth of around 50 cm in PH, PP and G to about 100 cm in Ag and E.
The comparison of OC and N contents in the replicated samples (Table 2) revealed that PM and G had significantly higher carbon (34.0 and 32.7 g kg –1, respectively) and nitrogen (4.4 and 4.8 g kg–1, respectively) contents in the uppermost layer than the other forest treatments (PH 24.3 and 2.8, E 17.9, and 1.6, and PP 13.5, and 1.2 g kg–1 of C and N, respectively) and the agriculture plot (9.3–0.8 g kg–1 for C and N, respectively) (Table 2). The highest C/N ratios were found in E, PP, and Ag (11.2, 11.3, and 11.3, respectively), and the lowest one in G with 6.7, and PM and PH were intermediate with values of 7.1 and 8.7, respectively. Although PM and PH soils had different OC and N contents, their C/N ratio was similar. However, in the 6–12 cm depth layer there were no significant differences among treatments for OC, with values ranging from 10.5 to 11.9 g kg–1, but N contents were significantly higher in PM and G (0.98 and 1.02 g kg–1, respectively), resulting also in lower C/N ratios for these treatments. In all forest soils a strong stratification of OC and N was observed, for instance topsoil OC in PM and G was 3 and 2.7 times higher than OC in the 6–12 cm layer, respectively. This was not the case for Ag (0.9 times higher), and of all forest treatments PP soil was least stratified (1.2 times higher value in 0–6 than in 6–12 cm).
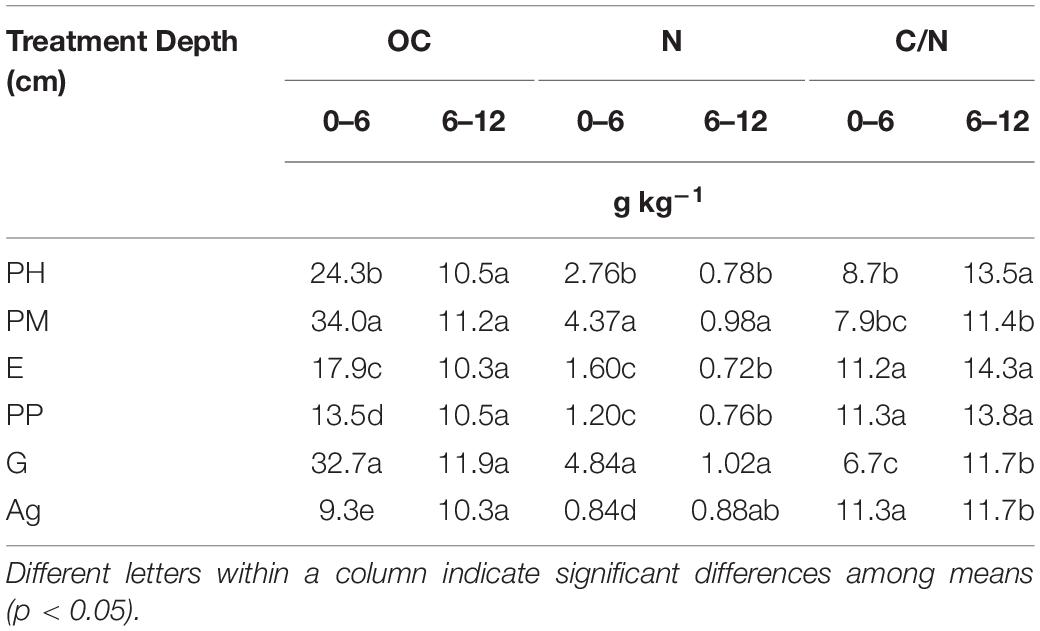
Table 2. Total organic carbon (OC), total nitrogen (N) contents and carbon to nitrogen ratio (C/N) in the 0–6 cm and 6–12 cm depth layers of A-horizons under the five forest treatments (PH, Pinus halepensis; PM, Pinus halepensis with mycorrhiza, E, Eucalyptus spp.; PP, Pinus pinea; G, Gleditsia triacanthos) and the agriculture plot (Ag).
Soil Physical Properties
Soil particle size distribution varied very little for the A horizons of the treatments (Table 3) and all soils had a sandy loam texture throughout their profiles (data not shown). The physical conditions of the A-horizons differed considerably among the treatments (Table 4). The highest bulk density was found, as expected, in the Ag soil, and the lowest values were for the E and G treatments. For the two Aleppo pine treatments, the one with mycorrhiza showed lower BD, than the non-inoculated one, which had a similar value as PP. Particle density was also different among the treatments, although more similarities were found than for BD. Thus, E had the lowest value, followed by PM (1.96 and 2.11 Mg m–3, respectively) while the highest PD was found in G and Ag. Total porosity was highest in the G treatment (59.1%), and the difference to the lowest values in the Ag, PH and PP soils was more than 20% less porosity for the latter. The moisture contents at field capacity were highest in G and PM, whereas PP had an even lower value than the PM and Ag soils (11.9, 13.2, and 13.8%, respectively).
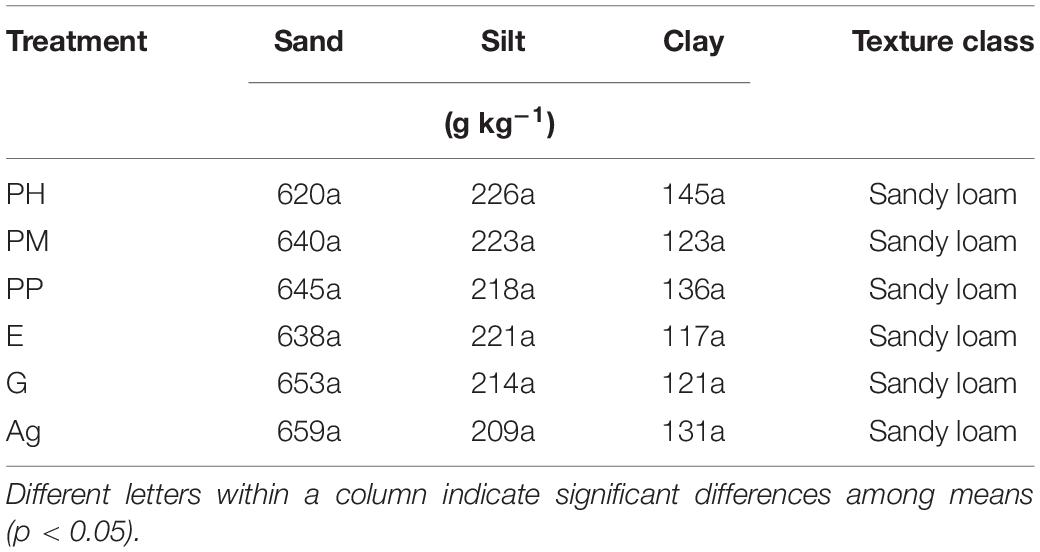
Table 3. Particle size distribution and texture class in the uppermost 0–12 cm of A-horizons under the five forest treatments (PH, Pinus halepensis; PM, Pinus halepensis with mycorrhiza, E, Eucalyptus spp.; PP, Pinus pinea; G, Gleditsia triacanthos) and the agriculture plot (Ag).
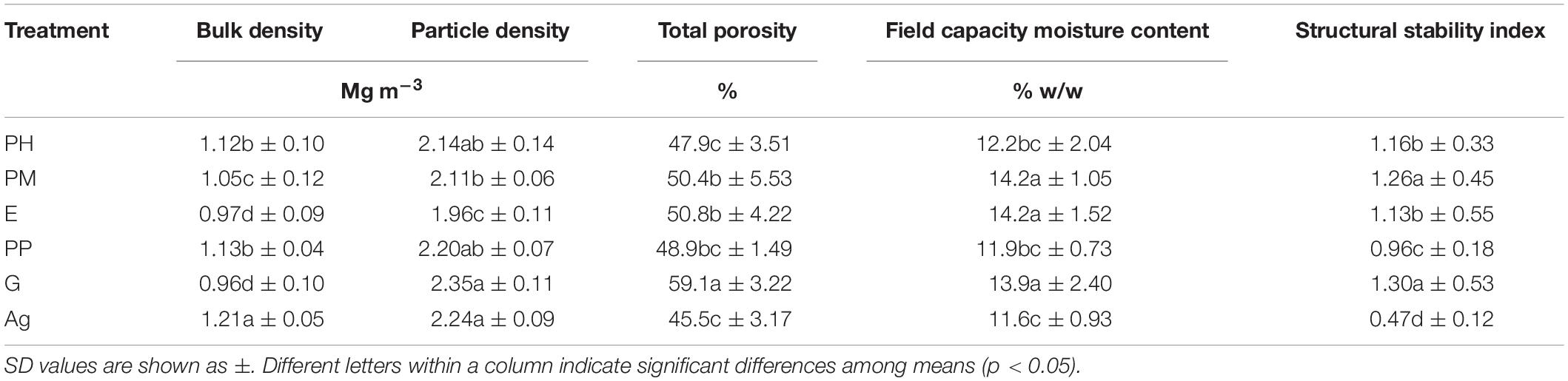
Table 4. Soil physical properties of the upper 6 cm of A-horizons of forest (PH, Pinus halepensis; PM, Pinus halepensis with mycorrhiza, E, Eucalyptus spp.; PP, Pinus pinea; G, Gleditsia triacanthos) and agricultural (Ag) soil profiles at the Santa Rosa site.
Similarly, the SSI was best in PM and G soils (1.26 and 1.30, respectively), PH, E, and PP were intermediate (1.16, 1.13, and 0.96, respectively), while the Ag soil had a much inferior value (0.47). In short, Ag and PP soils had the worst physical condition, while the best soil structure was found in G and PM.
The hydraulic properties (Figure 2) reflected the differences in soil structure and porosity. The best hydraulic conductivity was found in PM and G soils (17.5 and 15.4 cm h–1, respectively), and the lowest conductivity corresponded to E and Ag soils (2.7 and 5.8 cm h–1, respectively). The difference in magnitudes was important since PM had 6.5 times and G 5.7 times the hydraulic conductivity of the soil under eucalypt forest. Similarly, the infiltration rate was lowest in E and PP (111- and 78-mm h–1, respectively), which represented on average 2.7 times less than the best treatments (PH, PM, and G).
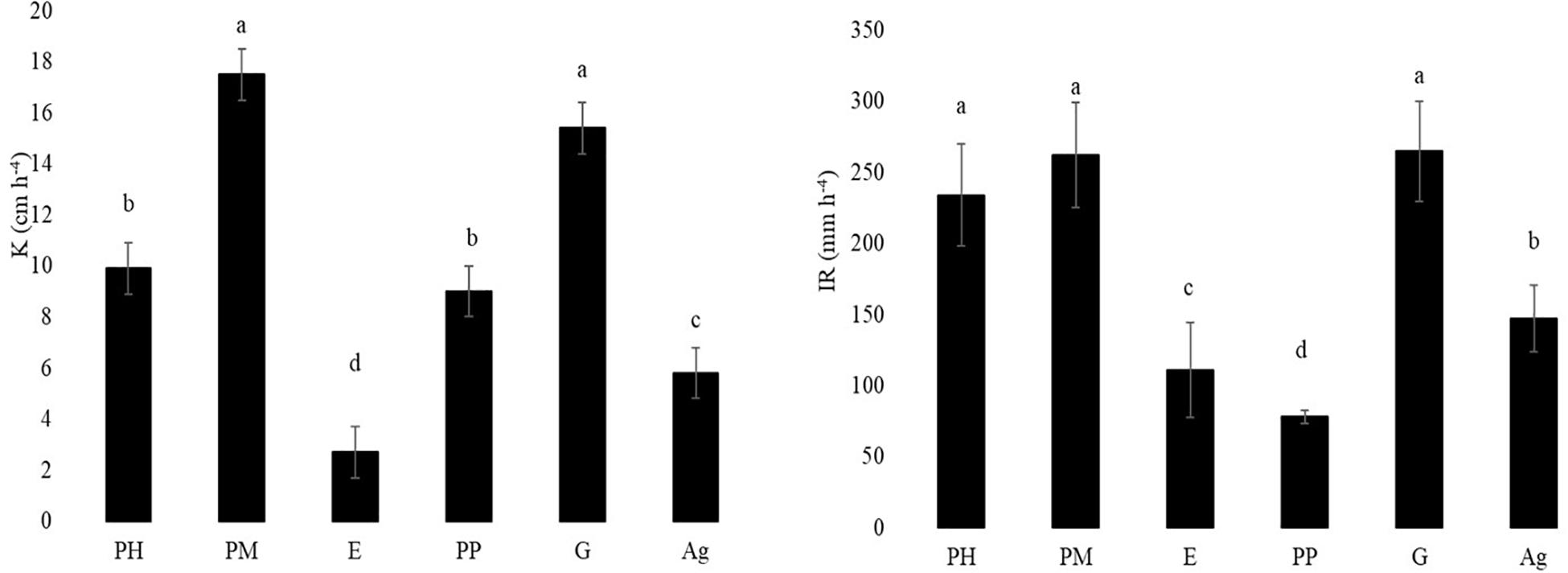
Figure 2. Hydraulic conductivity (K, cm h–1) and infiltration rate (IR, mm h–1) in the five forest treatments (PH- Pinus halepensis, PM- Pinus halepensis with mycorrhiza, E-Eucalyptus spp., PP-Pinus pinea, G-Gleditsia triacanthos) and the agriculture plot. Different letters indicate significant differences among means (p > 0.05), bars indicate SD.
The principal component analysis (Figure 3) explained 90.7% of the variability of the chosen variables (pH, CEC, C/N, SSI, BD, and IR). The first PC was defined positively by C/N and BD, and negatively by CEC, SSI, and IR, while the second PC was primarily defined by pH and IR (Table 5). Among the forest treatments, G and PM were associated with high IR, CEC, and SSI, while on the opposite side PP an E were associated to high C/N and BD. An intermediate position was shown by PH. On the second PC, Ag and to a lesser degree G were different from the other treatments and more related with higher pH while E and PP were in the opposed segment of the bi-plot, indicating lowest pH values and IR. The correlation matrix among the original variables (Table 6) showed that SSI and BD were strongly and negatively related (R2 = –0.93) as was to be expected, like the negative relation between BD and IR (R2 = –0.74). However, these indicators of soil structure were also related to chemical properties such as CEC (BD vs. CEC: R2 = –0.72) and C/N (IR vs. C/N: R2 = –0.94; and C/N vs. BD: R2 = 0.91). This indicated that soil physical, biological, and chemical indicators were interrelated and connected.
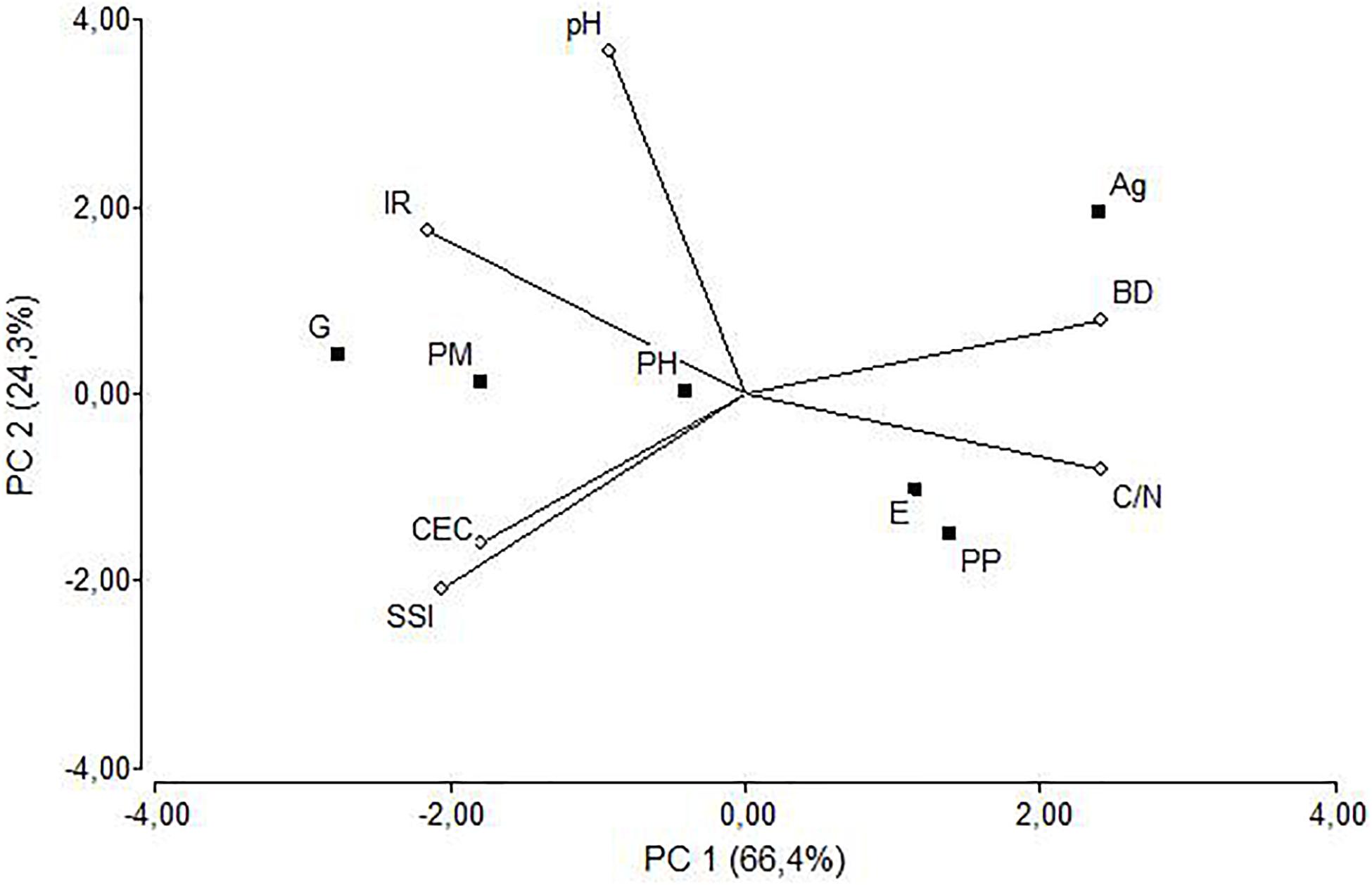
Figure 3. Bi-plot of the principal component analysis for the five forest treatments and the agricultural soil. Variables are pH, cation exchange capacity (CEC), infiltration rate (IR), structural instability index (SSI), bulk density (BD), and carbon to nitrogen ratio (C/N).
Discussion
The fact that soil texture showed no meaningful variation among soil profiles indicated that parent material and its particle size distribution was sufficiently similar as to be able to attribute differences in chemical and physical properties to the effect of land use and forest species. The data obtained showed that the effects of the five forest treatments on the original Haplustoll soil generated significant differences in the morphology of their soil profiles and their chemical and physical attributes.
The most important divergences were seen under E and PP forestations, where acidification of the A and AC horizons resulted in a change in the taxonomic classification, assigning these soils to the Alfisol soil order, due to the fact that with less than 50% base saturation a soil does not classify as a Mollisol (Soil Survey Staff, 2014). The calcium loss and acidification brought about by coniferous forest species has been shown in other climatic and soil conditions, and several mechanisms including an increase in organic acids, acid deposition, and absorption of nutrients have been proposed as the reasons for this (Mareschal et al., 2010). It has also been shown that poor leaf litter quality contributes to the absence of a burrowing earthworm community, which retards leaf litter decomposition and, consequently, leads to forest-floor build-up and soil acidification (Schrijver et al., 2012). Certini et al. (1998) attributed the acidity of stemflow under pine to cause the leaching of cations and organic matter. Both these latter processes most likely occurred in the Eucalypt and Stone pine plots of our experiment. Similar trends of enhanced acidification under Eucalypt plantations have been reported in Ethiopian abandoned farmland soils, where the authors concluded that soil properties deteriorated under Eucalypt (Lemenih, 2004). While this deterioration was strongest in the E and PP soils, PH also had an acid pH and lower base saturation than the G and Ag soils. A general and stronger trend of leaching of base cations and subsequent acidification in forested lands compared to grasslands was the result of a long-term assessment in Canadian soils (Cho et al., 2019). Our results showed that soil acidification will occur even in calcareous soils that were originally covered by grassland steppes or savannas. The scarcity of results on afforestation effects on steppe soils makes is difficult to estimate whether this trend can be generalized. However, Jobbágy and Jackson (2003) also found that afforested grassland soils of the humid Pampas suffered acidification and calcium loss to a depth of between 35 and 60 cm. In our case the only legume among the forest species and the mycorrhiza-inoculated pine were able to retain base cations and conserve a neutral to slightly alkaline pH, similar to the values of the natural grassland Mollisols of this region (INTA et al., 1980). This might be related to differences in other chemical and biological properties the G and PM soils, compared to the E, PP and PH treatments. The former had significantly higher carbon and nitrogen contents in the topsoil compared to the latter, and lower C/N ratios indicating that the litter quality and nitrogen availability were improved in G and PM, while E, PP, and PH had lower quality litter and lower N inputs. Högberg (2007) reported that most boreal and temperate forests are nitrogen limited and respond with higher carbon sequestration to anthropogenic nitrogen inputs. The N-limitation in E, PP and PH might therefore be one of the reasons for their lower carbon contents, due to the restriction this imposes on microbial activity. This has been shown for agricultural soils in this region, where crop sequences without legumes accumulated litter on the soil surface, but had lower topsoil carbon contents (Frasier et al., 2016), similarly Almagro et al. (2021) found that higher N availability in residues promoted C and N stabilization in soil aggregates. In the PM soil, the enhanced decomposing activity due to the presence of ectomycorrhiza facilitated the nutrient cycling and carbon stabilization in the soil, as has been reported for different types of forests (Wallander et al., 2011; Clemmensen et al., 2013; Li et al., 2015). Lin et al. (2017) found that trees which live in symbiosis with ectomycorrhiza usually have greater forest-floor C stocks, but also might accrue more C in the mineral horizons as occurred here in the case of PM compared to its uninoculated counterpart, PH. The authors attribute this to the fact that ectomycorrhiza on one hand have a lower decomposition rate than other soil microorganisms, and on the other hand they can take up organic N compounds from soil organic matter, thus enabling the transformations of low-quality forest litter. This also seems to explain the slightly lower C/N ration in the PM soil compared to PH.
The C/N ratios observed in these forest soils were considerably lower than those reported from a study on European forest soils under different tree species, where black locust forest floor had a ratio of less than 20, while evergreen trees had higher ones (>30). In the mineral topsoil these ratios were lower, for instance an average ratio of 17.6 was found for Aleppo pine (Cools et al., 2014). But these authors also found a strong effect of environmental conditions such as mean annual temperature and precipitation, indicating that warmer and drier climates generally had lower soil C/N ratios than cold and humid regions. Soil type also influenced C/N, with lowest values in Chernozem and Kastanozem A horizons. The soils of the present study would be classified as Kastanozem (IUSS et al., 2015) and under natural savanna vegetation have C/N ratios between 6 and 8 (Noellemeyer et al., 2006).
The amount of carbon stored under Eucalypt and Stone pine forest was lower than under Black locust and ecto-mycorrhiza-inoculated Pine plantations, while the Aleppo pine soil had an intermediate value of OC and showed an intermediate position with regards to other soil properties analyzed by the PCA. The strong correlations between biological (C/N), chemical (CEC, pH), and physical properties (SSI, IR) showed that all three dimensions of soil fertility are interrelated and that the effect of the vegetation to sequester more carbon also results in improved soil structure and chemical properties. The strong feedback between soil physical and biological processes in these soils was already shown by Fernández et al. (2019) in a comparison of natural vegetation and agricultural sites.
Soil physical properties under the different forest species also showed significant divergence. Lower organic C stocks and pH, and consequently less Ca2+ in the soil solution for colloidal stabilization, affected soil structure and pore system (Rawlins et al., 2016; Helliwell et al., 2017). Therefore E, PP, and PH had consistently poorer values for soil physical quality indicators (i.e., higher BD, lower porosity, lower SSI) than G and PM. This reflects the strong interaction between soil pores and soil carbon processes (Kravchenko and Guber, 2017). The deficient hydrological integrity of the soils under E, PP, and PH is clear from their extremely low hydraulic conductivity and infiltration rates, compared to PM and G, indicating high runoff even in rainfall events with low intensity. The low infiltration and hydraulic conductivity are strongly conditioned by the poor soil structure, including pore size distribution and morphology (Fernández et al., 2021). This is probably aggravated by the hydrophobicity of the forest floor under these species. Ellerbrock et al. (2005) found that for soil organic carbon contents <10 g kg–1 wettability increased with OC content, while it decreased for OC contents >10 g kg–1, but they also attributed wettability to organic matter composition, presumably the higher lipid contents of the litter floor under eucalypt and conifers would cause higher water repellency (Mataix-Solera and Doerr, 2004; Bodí et al., 2013). However, the hydrophobicity also depends strongly on the soil moisture content, and stronger water repellency was observed at lower moisture contents (Greiffenhagen et al., 2006), which would be aggravated by the fact that evergreen trees were shown to have a drier soil regime since the throughfall rate of rainwater is lower than in deciduous trees (Augusto et al., 2015).
Thus, in terms of chemical, biological, and physical fertility afforestation with Eucalypt and uninoculated conifers did not result in an improvement. On the contrary, these species deteriorated soil quality, similar to the results of Liu et al. (2021), who concluded that the use of native shrubs and grasses was more effective for restoring soil fertility on degraded croplands than afforestation.
Conclusion
Our results confirmed that Eucalypt and Pine plantations without ectomycorrhiza have a deleterious effect on soil biological, chemical, physical conditions, and on the soil profile morphology, resulting in a different taxonomic order for these soils, due to their low base saturation. On the other hand, the soils under Black locust and Aleppo pine with mycorrhiza showed significantly better physical and hydraulic conditions, which is to be expected given their higher organic matter and nitrogen contents, and lower C/N ratio, indicating that microbial activity was not limited by substrate quality nor by habitat restrictions. The inoculation of pine with ectomycorrhiza and the higher nitrogen availability under the legume species resulted in a positive change of soil biological and physical conditions, enhancing the importance of biological activity for essential soil functions to be preserved. However, considering the scarceness of information of the effects of plantations on steppe and grassland soils, further studies are needed to confirm the proposed processes and effects caused by different forest species on dryland Mollisols.
Data Availability Statement
The original contributions presented in the study are included in the article/Supplementary Material, further inquiries can be directed to the corresponding author/s.
Author Contributions
GM and DR carried out field work and lab analysis. EL and LÁ helped with data analysis and manuscript preparation. EN coordinated the study. All authors contributed to the article and approved the submitted version.
Conflict of Interest
The authors declare that the research was conducted in the absence of any commercial or financial relationships that could be construed as a potential conflict of interest.
Publisher’s Note
All claims expressed in this article are solely those of the authors and do not necessarily represent those of their affiliated organizations, or those of the publisher, the editors and the reviewers. Any product that may be evaluated in this article, or claim that may be made by its manufacturer, is not guaranteed or endorsed by the publisher.
Acknowledgments
We would like to thank two reviewers whose insightful comments greatly helped to improve this manuscript.
Supplementary Material
The Supplementary Material for this article can be found online at: https://www.frontiersin.org/articles/10.3389/ffgc.2021.685827/full#supplementary-material
References
Almagro, M., Ruiz-Navarro, A., Díaz-Pereira, E., Albaladejo, J., and Martínez-Mena, M. (2021). Plant residue chemical quality modulates the soil microbial response related to decomposition and soil organic carbon and nitrogen stabilization in a rainfed Mediterranean agroecosystem. Soil Biol. Biochem. 156:108198. doi: 10.1016/j.soilbio.2021.108198
Augusto, L., De Schrijver, A., Vesterdal, L., Smolander, A., Prescott, C., and Ranger, J. (2015). Influences of evergreen gymnosperm and deciduous angiosperm tree species on the functioning of temperate and boreal forests. Biol. Rev. 90, 444–466. doi: 10.1111/brv.12119
Blake, G. R., and Hartge, K. H. (1986). “Bulk density,” in Methods of Soil Analysis. Part 1. Physical and Minerological Methods, ed. A. Klute (Madison: SSSA), 363–382.
Bodí, M. B., Muñoz-Santa, I., Armero, C., Doerr, S. H., Mataix-Solera, J., and Cerdà, A. (2013). Spatial and temporal variations of water repellency and probability of its occurrence in calcareous Mediterranean rangeland soils affected by fires. CATENA 108, 14–25. doi: 10.1016/j.catena.2012.04.002
Casagrande, G., Vergara, G., and Bellini Saibene, Y. (2006). Cartas agroclímáticas actuales de temperaturas, heladas y lluvia de la provincia de La Pampa (Argentina). Rev. Fac. Agron. 12, 15–22.
Certini, G., Ugolini, F., and Corti, G. (1998). Early stages of podzolization under Corsican pine (Pinus nigra Arn. ssp. laricio). Geoderma 83, 103–125.
Cho, S., Dinwoodie, G., Fu, Y., Abboud, S., and Turchenek, L. (2019). An assessment of long-term soil acidification trends in Alberta, Canada. Ecol. Indic. 98, 712–722. doi: 10.1016/j.ecolind.2018.11.020
Clemmensen, K. E., Bahr, A., Ovaskainen, O., Dahlberg, A., Ekblad, A., Wallander, H., et al. (2013). Roots and associated fungi drive long-term carbon sequestration in boreal forest. Science 339, 1615–1618. doi: 10.1126/science.1231923
Cools, N., Vesterdal, L., De Vos, B., Vanguelova, E., and Hansen, K. (2014). Tree species is the major factor explaining C: N ratios in European forest soils. For. Ecol. Manage. 311, 3–16. doi: 10.1016/j.foreco.2013.06.047
Crow, S. E., Filley, T. R., McCormick, M., Szlávecz, K., Stott, D. E., Gamblin, D., et al. (2008). Earthworms, stand age, and species composition interact to influence particulate organic matter chemistry during forest succession. Biogeochemistry 92, 61–82. doi: 10.1007/s10533-008-9260-1
De Boodt, M., De Leenheer, L., and Frese, H. (1967). West-European Methods For Soil Structure Determination. Ghent: State Faculty of Agricultural Sciences, Ghent.
De Vries, W., Reinds, G. J., and Vel, E. (2003). Intensive monitoring of forest ecosystems in Europe 2: Atmospheric deposition and its impacts on soil solution chemistry. For. Ecol. Manage. 174, 97–115.
Di Rienzo, J. A. A., Casanoves, F., Balzarini, M. G. G., González, L., Tablada, M., Robledo, C. W. W., et al. (2017). Grupo InfoStat. Argentina: FCA, Universidad Nacional de Córdoba.
Ellerbrock, R., Gerke, H., Bachmann, J., and Goebel, M. (2005). Composition of organic matter fractions for explaining wettability of three forest soils. Soil Sci. Soc. Am. J. 69, 57–66.
Farley, K. A., Kelly, E. F., and Hofstede, R. G. M. (2004). Soil organic carbon and water retention after conversion of grasslands to pine plantations in the ecuadorian andes. Ecosystems 7, 729–739. doi: 10.1007/s10021-004-0047-5
Fernández, R., Belmonte, V., Quiroga, A., Lobartini, C., and Noellemeyer, E. (2021). Land-use change affects soil hydro-physical properties in Mollisols of semiarid Central Argentina. Geoderma Reg. 25:e00394. doi: 10.1016/j.geodrs.2021.e00394
Fernández, R., Frasier, I., Quiroga, A., and Noellemeyer, E. (2019). Pore morphology reveals interaction of biological and physical processes for structure formation in soils of the semiarid Argentinean Pampa. Soil Tillage Res. 191, 256–265. doi: 10.1016/j.still.2019.04.011
Frasier, I., Quiroga, A., and Noellemeyer, E. (2016). Effect of different cover crops on C and N cycling in sorghum NT systems. Sci. Total Environ. 562, 628–639. doi: 10.1016/j.scitotenv.2016.04.058
Gee, G. W., and Bauder, J. W. (1986). “Particle-size Analysis,” in Methods of Soil Analysis: Part 1—Physical and Mineralogical Methods, ed. A. Klute (Madison: Soil Science Society of America, American Society of Agronomy), 383–411.
Gili, A., Trucco, R., Niveyro, S., Balzarini, M., Estelrich, D., Quiroga, A., et al. (2010). Soil texture and carbon dynamics in savannah vegetation patches of Central Argentina. Soil Sci. Soc. Am. J. 74:647. doi: 10.2136/sssaj2009.0053
Greiffenhagen, A., Wessolek, G., Facklam, M., Renger, M., and Stoffregen, H. (2006). Hydraulic functions and water repellency of forest floor horizons on sandy soils. Geoderma 132, 182–195. doi: 10.1016/j.geoderma.2005.05.006
Gurmesa, G. A., Schmidt, I. K., Gundersen, P., and Vesterdal, L. (2013). Soil carbon accumulation and nitrogen retention traits of four tree species grown in common gardens. For. Ecol. Manage. 309, 47–57.
Helliwell, J. R., Sturrock, C. J., Mairhofer, S., Craigon, J., Ashton, R. W., Miller, A. J., et al. (2017). The emergent rhizosphere: Imaging the development of the porous architecture at the root-soil interface. Sci. Rep. 7:14875. doi: 10.1038/s41598-017-14904-w
Högberg, P. (2007). Environmental science: nitrogen impacts on forest carbon. Nature 447, 781–782. doi: 10.1038/447781a
INTA, Gobierno de La Pampa, and Universidad Nacional de La Pampa. (1980). Inventario integrado de los recursos naturales de la provincia de La Pampa. Buenos Aires: INTA.
IUSS, WRB, and Working group. (2015). “World Reference Base for Soil Resources 2014, update 2015 International soil classification system for naming soils and creating legends for soil maps,” in World Soil Resources Reports No. 106, ed. World Soil (Rome: FAO).
Jobbágy, E. G., and Jackson, R. B. (2003). Patterns and mechanisms of soil acidification in the conversion of grasslands to forests. Biogeochemistry 64, 205–229. doi: 10.1023/A:1024985629259
Johnson-Maynard, J., Graham, R., Wu, L., and Shouse, P. (2002). Modification of soil structural and hydraulic properties after 50 years of imposed chaparral and pine vegetation. Geoderma 110, 227–240.
Kravchenko, A. N., and Guber, A. K. (2017). Soil pores and their contributions to soil carbon processes. Geoderma 287, 31–39. doi: 10.1016/j.geoderma.2016.06.027
Laik, R., Kumar, K., Das, D. K., and Chaturvedi, O. P. (2009). Labile soil organic matter pools in a calciorthent after 18 years of afforestation by different plantations. Appl. Soil Ecol. 42, 71–78. doi: 10.1016/j.apsoil.2009.02.004
Lemenih, M. (2004). Comparison of soil attributes under Cupressus lusitanica and Eucalyptus saligna established on abandoned farmlands with continuously cropped farmlands and natural forest in Ethiopia. For. Ecol. Manage. 195, 57–67. doi: 10.1016/j.foreco.2004.02.055
Li, A., Fahey, T. J., Pawlowska, T. E., Fisk, M. C., and Burtis, J. (2015). Fine root decomposition, nutrient mobilization and fungal communities in a pine forest ecosystem. Soil Biol. Biochem. 83, 76–83. doi: 10.1016/j.soilbio.2015.01.019
Lin, G., McCormack, M. L., Ma, C., and Guo, D. (2017). Similar below-ground carbon cycling dynamics but contrasting modes of nitrogen cycling between arbuscular mycorrhizal and ectomycorrhizal forests. New Phytol. 213, 1440–1451. doi: 10.1111/nph.14206
Liu, X., Dou, L., Ding, X., Sun, T., and Zhang, H. (2021). Influences of different afforestation systems on the soil properties of limestone mountains in the mid-eastern region of China. CATENA 201:105198. doi: 10.1016/j.catena.2021.105198
Mareschal, L., Bonnaud, P., Turpault, M. P., and Ranger, J. (2010). Impact of common European tree species on the chemical and physicochemical properties of fine earth: an unusual pattern. Eur. J. Soil Sci. 61, 14–23. doi: 10.1111/j.1365-2389.2009.01206.x
Masera, O. R., Garza-Caligaris, J. F., Kanninen, M., Karjalainen, T., Liski, J., Nabuurs, G. J., et al. (2003). Modeling carbon sequestration in afforestation, agroforestry and forest management projects: the CO2FIX V.2 approach. Ecol. Modell. 164, 177–199. doi: 10.1016/S0304-3800(02)00419-2
Mataix-Solera, J., and Doerr, S. (2004). Hydrophobicity and aggregate stability in calcareous topsoils from fire-affected pine forests in southeastern Spain. Geoderma 118, 77–88. doi: 10.1016/S0016-7061(03)00185-X
Mendham, D., Connell, A., and Grove, T. (2003). Change in soil carbon after land clearing or afforestation in highly weathered lateritic and sandy soils of south-western Australia. Agric. Ecosyst. Environ. 95, 143–156.
National Soil Survey Center (2012). Field Book for Describing and Sampling Soils. Washington, D.C.: USDA NRCS.
Niu, X., and Duiker, S. W. (2006). Carbon sequestration potential by afforestation of marginal agricultural land in the Midwestern U.S. For. Ecol. Manage. 223, 415–427. doi: 10.1016/j.foreco.2005.12.044
Noellemeyer, E., Frank, F., Alvarez, C., Morazzo, G., and Quiroga, A. (2008). Carbon contents and aggregation related to soil physical and biological properties under a land-use sequence in the semiarid region of central Argentina. Soil Tillage Res. 99, 179–190. doi: 10.1016/j.still.2008.02.003
Noellemeyer, E., Quiroga, A., and Estelrich, D. (2006). Soil quality in three range soils of the semi-arid Pampa of Argentina. J. Arid Environ. 65, 142–155. doi: 10.1016/j.jaridenv.2005.07.007
Phillips, J. D. (2009). Soils as extended composite phenotypes. Geoderma 149, 143–151. doi: 10.1016/j.geoderma.2008.11.028
Prescott, C. E., and Grayston, S. J. (2013). Tree species influence on microbial communities in litter and soil: Current knowledge and research needs. For. Ecol. Manage. 309, 19–27.
Rawlins, B. G., Wragg, J., Rheinhard, C., Atwood, R. C., Houston, A., Lark, R. M., et al. (2016). Three dimensional soil organic matter distribution, accessibility and microbial respiration in macro-aggregates using osmium staining and synchrotron X-ray CT. Soil Discuss. 0, 1–24. doi: 10.5194/soil-2016-32
Reynolds, W. D. (2007a). “Chapter 75 Saturated hydraulic properties: Laboratory methods,” in Soil Sampling and Methods of Analysis, eds M. R. Carter and E. G. Gregorich (Edmonton: Canadian Society of Soil Science).
Reynolds, W. D. (2007b). “Chapter 77 Saturated hydraulic properties: Ring infiltrometer,” in Soil Sampling and Methods of Analysis, eds M. R. Carter and E. G. Gregorich (Edmonton: Canadian Society of Soil Science).
Riestra, D., Noellemeyer, E., and Quiroga, A. (2012). Soil texture and forest species condition the effect of afforrestation on soil quality parameters. Soil Sci. 177, 279–287.
Schrijver, A., Frenne, P., Staelens, J., Verstraeten, G., Muys, B., Vesterdal, L., et al. (2012). Tree species traits cause divergence in soil acidification during four decades of postagricultural forest development. Glob. Chang. Biol. 18, 1127–1140. doi: 10.1111/j.1365-2486.2011.02572.x
Skjemstad, J. O., Baldock, J. A. A., Skjemstad, J. O., and Baldock, J. A. A. (2003). “Chapter 21 Total and Organic Carbon,” in Soil Sampling and Methods of Analysis, eds M. R. Carter and E. G. Gregorich (Edmonton: Canadian Society of Soil Science).
Tang, G., and Li, K. (2013). Tree species controls on soil carbon sequestration and carbon stability following 20years of afforestation in a valley-type savanna. For. Ecol. Manage. 291, 13–19. doi: 10.1016/j.foreco.2012.12.001
Vesterdal, L., Clarke, N., Sigurdsson, B. D., and Gundersen, P. (2013). Do tree species influence soil carbon stocks in temperate and boreal forests? For. Ecol. Manage. 309, 4–18. doi: 10.1016/j.foreco.2013.01.017
Wallander, H., Ekblad, A., and Bergh, J. (2011). Growth and carbon sequestration by ectomycorrhizal fungi in intensively fertilized Norway spruce forests. For. Ecol. Manage. 262, 999–1007. doi: 10.1016/j.foreco.2011.05.035
Wei, X., Li, Q., Liu, Y., Liu, S., Guo, X., Zhang, L., et al. (2013). Restoring ecosystem carbon sequestration through afforestation: A sub-tropic restoration case study. For. Ecol. Manage. 300, 60–67. doi: 10.1016/j.foreco.2012.06.018
Keywords: semiarid central Argentina, organic matter, base saturation, Mollisols, hydrophobicity
Citation: Morazzo G, Riestra DR, Leizica E, Álvarez L and Noellemeyer E (2021) Afforestation With Different Tree Species Causes a Divergent Evolution of Soil Profiles and Properties. Front. For. Glob. Change 4:685827. doi: 10.3389/ffgc.2021.685827
Received: 25 March 2021; Accepted: 29 July 2021;
Published: 16 August 2021.
Edited by:
Jeff Allen Hatten, Oregon State University, United StatesReviewed by:
Antra Boca, Latvia University of Agriculture, LatviaPer Gundersen, University of Copenhagen, Denmark
Copyright © 2021 Morazzo, Riestra, Leizica, Álvarez and Noellemeyer. This is an open-access article distributed under the terms of the Creative Commons Attribution License (CC BY). The use, distribution or reproduction in other forums is permitted, provided the original author(s) and the copyright owner(s) are credited and that the original publication in this journal is cited, in accordance with accepted academic practice. No use, distribution or reproduction is permitted which does not comply with these terms.
*Correspondence: Elke Noellemeyer, bm9lbGxlbWV5ZXJAYWdyby51bmxwYW0uZWR1LmFy; ZW5vZWxsZW1leWVyQGdtYWlsLmNvbQ==