- 1Research Group on Hydrology in Forest Ecosystem, Department of Environmental Science, Federal University of São Carlos, Sorocaba, Brazil
- 2Applied Mathematics Laboratory, Department of Physics, Chemistry and Mathematics, Federal University of São Carlos, Sorocaba, Brazil
- 3Research group of Polymers from Renewable Sources, Department of Physics, Chemistry and Mathematics, Federal University of São Carlos, Sorocaba, Brazil
- 4Research group on Hydrology in Forest Ecosystem, Department of Environment and Development, Federal University of Amapá, Macapá, Brazil
Few investigations have examined the structural controls of bark on its water storage and influence on stemflow, despite the bark being considered a critical component that determines the time and magnitude of this process. This study seeks to answer the question: Do bark water absorbability and wettability estimates correlate with stemflow yield? We hypothesized that (1) the absorbability and wettability are correlated, that is, greater water absorbability implies greater wettability, and (2) high rates of bark water absorbability and wettability has a strong and negative correlation with stemflow generation. Stemflow yield (Sy) was monitored over 12 months for 31 trees, representing 9 species common to the Brazilian savanna ecosystem known as Cerrado. Bark absorbability, per unit dry weight, changes over time of the water absorbability (BWA - by submersion methodology), bark drying (BWD), bark absorbability rate (BWArate), bark drying rate (BWDrate), and wettability (initial contact angle – CAin and CA rate - CArate) were determined under laboratory conditions. As insoluble lignin may also act to alter bark water storage dynamics, for each species, the bark insoluble lignin content was characterized. Stemflow variability was significant across the study species. Funneling ratios (FR) indicates that all species’ canopies diverted enough rainfall as stemflow to concentrate rainwaters at the surface around their stem bases (FR > 1). Differences in bark water absorbability were notable some of tree species. A decrease in the CA value as a function of time was not observed for all barks, which in association with stemflow yields, allowed a novel classification method of wettability, based on CAin and it’s rate of change: highly wettable (CAin ≤ 75.3° and CArate ≥ 0.26°h–1) and non-wettable (CAin ≥ 93.5° and CArate ≤ 0.13°h–1). So, only from the wettability classification could be observed that the non-wettable bark species presented higher Sy, FR, BWA, and BWArate than highly wettable bark species. The stemflow from species with highly wettable bark had a strong and positive correlation with BWA. On the other hand, non-wettable bark stemflow yield has a strongly and negative correlation with FR, CAin, and BWArate. Thus, bark wettability properties showed to deserves special attention. This novel classification of bark wettability had a substantial effect on stemflow yield comprehension and proved to be an important variable to link laboratory and field investigation for understanding the stemflow yield. These findings will improve our understanding of the stemflow dynamics, water balance and the ecohydrology processes of forest ecosystems.
Introduction
Many interactions between rainfall and forest canopy remain poorly understood. This is problematic because, for rainfall to reach a forest’s soil, it must pass through tree canopies. Some rain droplets fall through canopy gaps, but most will interact with leaves, epiphytes, and/or bark surfaces. A result of these interactions is that a portion of the rainfall is evaporated back to the atmosphere, or intercepted by the canopy (Johnson and Lehmann, 2006; Ahmadi et al., 2013; Liu et al., 2014). The remaining rainwater reaches the forest soil surface as a drip (called throughfall) or as a flow of water down tree stems (stemflow). The stemflow water that reaches the soil are able to create complex spatial patterns, such as infiltration and groundwater recharge. Patterns of rainwater supply to soils via throughfall are highly heterogeneous, with spatial coefficients of variation often exceeding 50% in single events—and > 100% in regions with complex canopies (Van Stan et al., 2020). Although stemflow may be < 5% of the total rainfall across the canopy, its spatial variability is typically higher than observed for throughfall. This occurs because a fraction of rainfall across a single canopy can become highly magnified when locally drained (or “funneled”) to a small area, 10–1 –101 m2 tree–1 (Van Stan and Allen, 2020), next to a tree’s stem base – sometimes resulting in stemflow volumes > 100 times greater than open rainfall (Van Stan and Gordon, 2018). Another tree’s stemflow volume may be so low that open rainfall is many times greater (Van Stan and Gordon, 2018).
Several mechanisms have been proposed to explain the high spatial variability of stemflow volumes within forests (Honda et al., 2015; Spencer and van Meerveld, 2016; Van Stan and Allen, 2020). First, one must consider the size of trees, where bigger canopies tend to capture greater rainfall (Zimmermann et al., 2015). Secondly, the structure of canopies can influence how effectively individual trees drain this capture rainfall, and currently it is hypothesized that there may be an optimum branch inclination angle that most efficiently drains rainwater to the stem (Levia, 2003; Sadeghi et al., 2020). Third, the orientation of canopies within the “tree neighborhood” is believed to impact stemflow generation – some dominant canopies overshadow others (Metzger et al., 2019) or gather wind-driven rainfall (Herwitz and Slye, 1995; Levia and Herwitz, 2005; Van Stan et al., 2011). Finally, bark structure, being the surface over which stemflow must flow, is considered a master variable. Specifically, smoother bark has been generally observed to generate greater stemflow due to lower water storage capacities and fewer flow obstructions (Levia et al., 2010; Van Stan and Levia, 2010; Van Stan et al., 2016).
Of course, all of these factors interact to control stemflow spatial variability (Metzger et al., 2019). However, in forests where tree density is low enough that canopies are rarely in close proximity (like the Cerrado of Brazil) (Honda et al., 2015), the tree neighborhood is unlikely to drive stemflow initiation and total volume per storm event. Rather, in this scenario, the individual canopy and stem bark structural variability is anticipated to drive spatial variability in stemflow across trees. This is because stemflow has persistent contact with the bark during draining while bark water storage capacity must be locally overcome for stemflow to initiate and drain. Thus, the amount of water flowing down the tree stem may depend more on bark properties (Crockford and Richardson, 2000; Levia and Herwitz, 2005) than other meteorological conditions (Voigt and Zwolinski, 1964). Crockford and Richardson (2000) found that bark wettability and water storage capacity are characteristics that greatly influence stemflow production. Moreover, barks may also have chemically heterogeneous compositions that could influence the water absorbability and wettability.
Tree barks contain significant amounts of lignin (sometimes with higher lignin content than their respective woods), a complex phenylpropanoid polymer that has a structural role in plant cell walls while also providing hydrophobicity and protection against infection (Neiva et al., 2020).
Similar as leaf wettability, bark wettability is the amount of water captured, and retained on bark surfaces. Bark has a greater water-holding capacity than foliar surfaces (Herwitz and Slye, 1995; Valová and Bieleszová, 2008) but stemflow generation can begin before the woody frame of a tree is completely wetted (Herwitz, 1987). As well-known, surface wettability is a physical parameter that can be experimentally measured. The liquid, in general, a simple droplet in controlled laboratory conditions, is brought in contact with a solid surface, forming a liquid-solid interface where a droplet shape is created. The shape of that droplet depends on the cohesive interactions present in the molecules of the liquid and the adhesive interactions between the solid and liquid phases of the material (Molnar et al., 2011). When the droplet is brought on the solid surface, a simple physical parameter, the so-called contact angle (CA), can be used to determine the wettability of such a surface. The CA is defined as the angle θ emerging at the contact between the three-phases, which can be measured by the tangent to the liquid-fluid interface and the solid surface. It is measured here counterclockwise, which means it is measured on the liquid side. The origins of this angle probably go back to Galileo, passing through Young and others along the centuries [for a historical introduction and review see Good (1992) and Drelich et al. (2020)].
Hypothetically, the bark water storage capacity and wettability varies substantially between species, but relatively little is known about the dynamics of rainfall interception by the bark of stems and branches and the factors that regulate the process (Valová and Bieleszová, 2008; Ilek et al., 2017a). The differentiation of the bark surface is relatively hard to parametrize and there is little information on the methods of its measurement (Ilek and Jarosław, 2014). Some studies have examined bark water storage capacity (Levia and Frost, 2003; Levia and Wubbena, 2006; Valová and Bieleszová, 2008; Ilek and Jarosław, 2014; Ilek et al., 2015, 2017a,b) or leaf wettability properties (Aryal and Neuner, 2010; Klamerus-Iwan and Błońska, 2018; Papierowska et al., 2018; Klamerus-Iwan et al., 2020a,b,c). Indeed, leaf wettability observations and their relationship with rainfall interception processes are numerous enough to produce multiple critical reviews (e.g., Rosado and Holder, 2013; Holder, 2020), but no study known to the authors has yet evaluated the correlation of bark absorbability and bark wetting properties on stemflow yield. Thus, the focus of this research is to investigate how bark traits correlate to stemflow in an under-researched ecosystem. This investigation is the first to examine multiple factors regarding bark wettability (i.e., initial droplet contact angle and its rate of change with wetting; insoluble lignin content); and the first to discuss how this laboratory investigations of wettability correlated with the stemflow generation in the field.
The main purpose, therefore, of this investigation is to test whether and to what extent bark water absorbability and wettability influences stemflow in trees of Brazilian Cerrado savannah. Cerrado is the second largest biome in South America, as well as the main biome connecting four of the five Brazilian biomes and three important hydrographic basins in South America (Araguaia/Tocantins, São Francisco and Prata) that largely contribute to water recharge in Guarani Aquifer. Thus it has strategic value for several countries, mainly for the ones facing increasing water scarcity (de Leite and Ribeiro, 2018; Pereira et al., 2021). Stemflow variability was monitored over 12 months for 31 trees, representing 9 species common to the Cerrado. These data were used to answer the question: Do bark water absorbability and wettability estimates correlate with stemflow yields? We hypothesized that (1) the bark absorbability and wettability are correlated, that is, greater water absorbability imply greater wettability, and (2) that high rates of bark water absorbability and wettability has a strong and negative correlation with stemflow. Since the Cerrado is considered an arid ecosystem with high biodiversity (grasses, shrubs, and trees) there is a need for more information on how the hydrological processes of this environment are governed, as also as provide parameters for ecohydrological simulations in tropical forests.
Materials and Methods
The experimental site was installed at the Private Natural Heritage Reserve (RPPN) Águas Perenes Forest (Perennial Water Forest), Brotas, São Paulo state, Brazil. The Águas Perenes Forest covers 812 ha and is characterized by secondary Cerrado and Cerradão vegetation (Pereira et al., 2021). The Köppen climate-type of the region is Cwa (Dubreuil et al., 2019), which corresponds to a subtropical climate (C), characterized by warm summers and dry, cool winters (w), such that the annual average temperature is 20°C (a), and the annual average rainfall is 1,337 mm. The predominant soil type is quartzarenicneosol (dos Santos et al., 2018).
Hydrometeorological Data
The study was carried out in sites under 11 years of passive restoration. Three sample units of 400 m2 were installed and the stemflow volume [SF, L tree–1 storm–1], collectors were installed in all trees with DBH > 5cm, totalizing 31 trees distributed in 9 species (Table 1). The accumulated rainfall and stemflow were measured monthly from April 2018 to March 2019. Three The rainfall was measured in an open area without obstructions using three pluviometers made of polyethylene (storage capacity of 1.57 L) installed near the stand, with a maximum distance of 30 m. The pluviometers were installed at a height of 1.20 m. Stemflow collars were constructed by wrapping individual tree stems with a polyurethane gutter, fixed at 1.3 m from the ground (Figure 1). Water running down the stem was captured by these gutters, then drained by a 1.6 mm hose connected to 20L collection tanks. Evaporation from the stemflow collection tanks between storms was assumed to be negligible as the only opening in the tanks was < 2 mm (where the hose was connected). The collar material efficiently captured water. Event stemflow volumes were divided by each tree’s projected canopy area [m2 tree–1] to estimate event stemflow yield [Sy, mm tree–1 storm–1]. Funneling ratio (FR) was computed per specie for mean annual storm and also, it was compared with a normalized stemflow yield (Gordon et al., 2020). While stemflow volume describes total flux to the forest floor, FR describes the efficiency with which individual trees are capable of capturing rainfall and generating stemflow (Siegert and Levia, 2014). Introduced by Herwitz (1986), FR ratio describes the efficiency of each tree to capture rainfall and to generate stemflow, and allows comparing stemflow amounts for plants with different DBH (Siegert and Levia, 2014; Levia and Germer, 2015; Corti et al., 2019). This parameter does not refer to the infiltration area at the soil surface but has the advantage of being related to easily measurable data. FR greater than 1 indicates the contribution of the outlying canopy to stemflow generation. This ratio is expressed by:
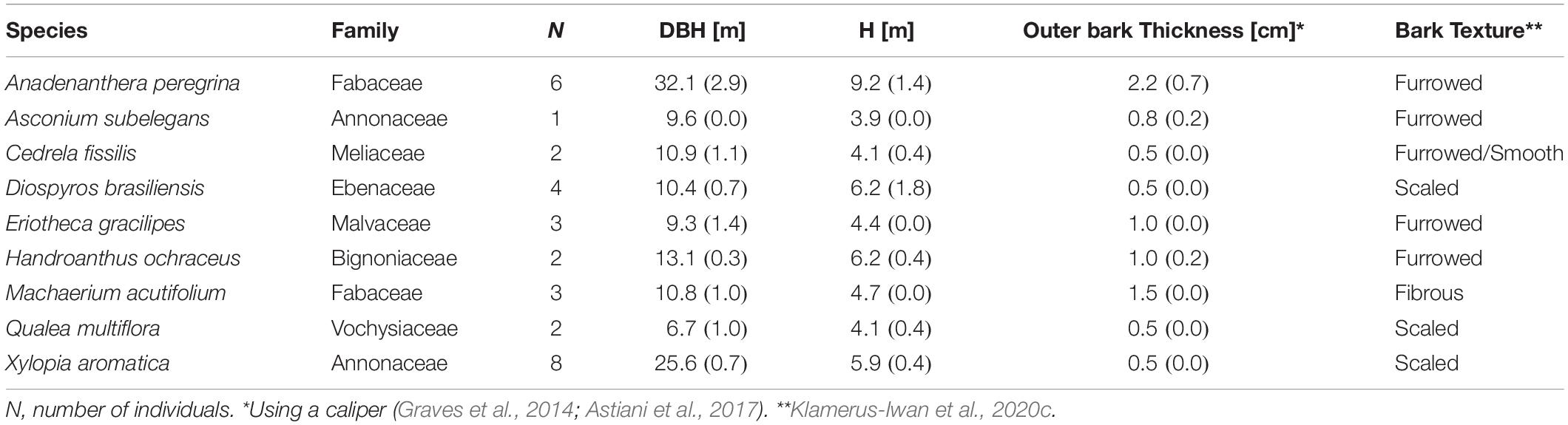
Table 1. Mean (standard errors) of diameter at breast high (DBH), height (H), outer bark thickness and bark texture of 9 Cerrado tree species, Brotas-Brazil.
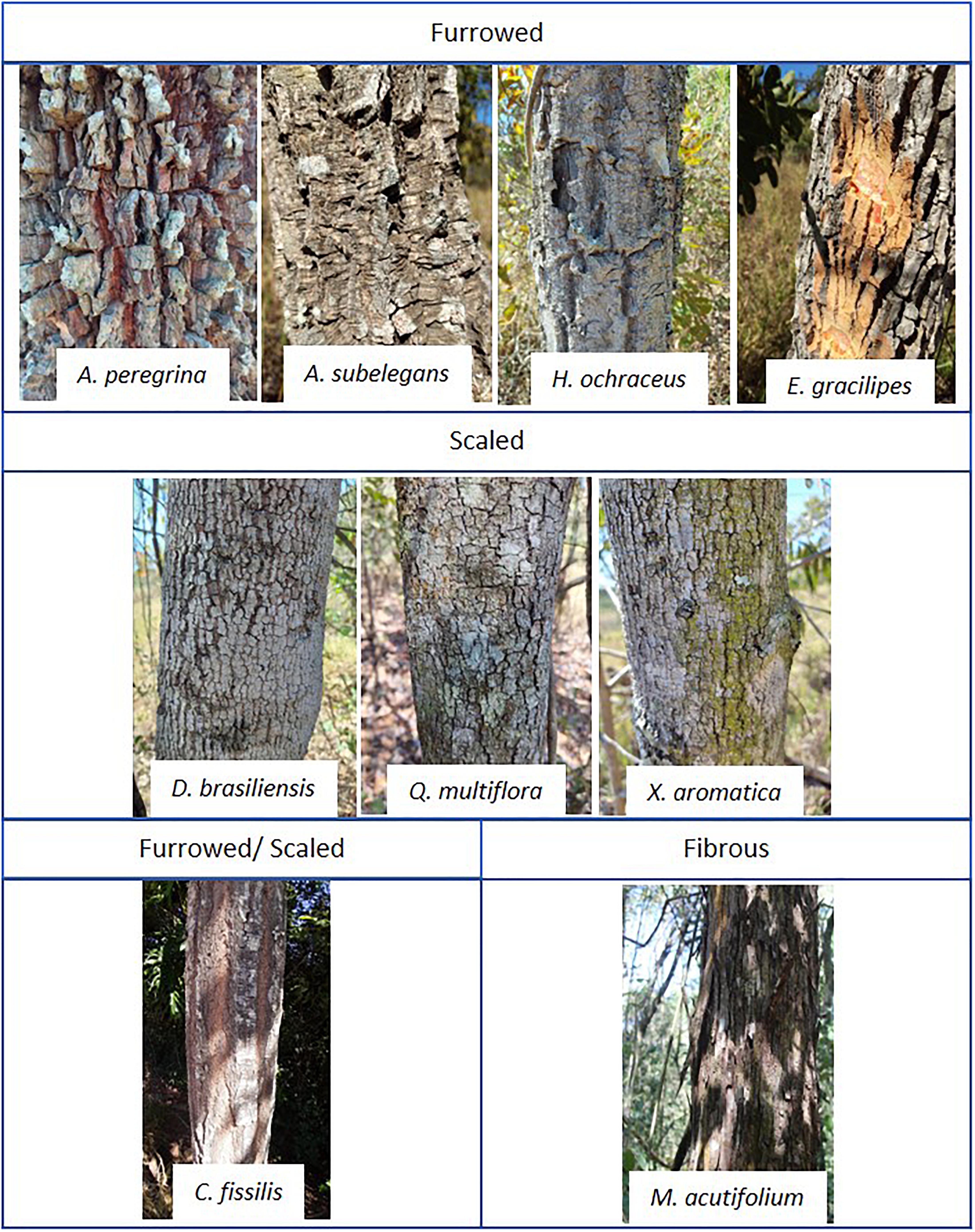
Figure 1. Bark texture for 9 species studied in Brazilian Cerrado. Águas Perenes Forest, Brotas, Brazil.
where, Sy, stemflow yield, is the stemflow volume per tree, in L; P is the precipitation depth, in mm; B is the basal area of the trunk at breast height, in m2.
Bark Water Absorbability (BWA) and Drying Rate (BWD)
By bark absorbability, we mean the ability of bark to absorb water in a given unit of time in full saturation conditions with the assumption that water is absorbed only by the outer bark layer. Analyses performed under laboratory conditions determined the bark water absorbability of species (N) over time. The outer bark samples were collected using a chisel, a saw, and a knife from the stems of similar size at the breast height (1.3 m) by cutting 3-5 rectangular pieces of bark (5 × 5 cm, approximately). Care was taken to ensure that the bark samples were collected from different locations around the stem at breast height. After that, the bark samples were dried at 35°C to constant mass and the weight of samples was the initial weight of the bark [dried mass – Dm, g]. Then, all side surfaces and the inner surface of the samples (surfaces not typically in contact with rainwater) were sealed with silicon prior to soaking, applied in such a way that, during the experiments, water was absorbed only by the outer layer of the bark (Ilek et al., 2017b). Next, the samples were reweighed to determine the dry weight of the insulating layer of individual samples.
Dried bark samples were submerged in deionized water and weighed every hour during the first 12 h, and then, weighed at intervals of 12 h until complete 96 h [saturated mass - Sm, g]. In each weighed, the samples were re-soaked every time. The experimental during time was determined due to no longer resulted in weight gain of individual bark samples. Bark water absorbability of these samples [BWA, %] was determined based on adaptation of a commonly applied submersion method used to obtain the litter water holding capacity (Blow, 1985). The difference in weight between a sample saturated with water and a dry one was related in each case to the weight of the sample in the dry state [equation (2)]. In this way, subsequent values of water absorbability (BWA) were obtained after successive times of immersion in water (BWA1, BWA2).
where BWA is the dried bark water absorbability [%], Sm is the weight of a sample after a subsequent soaking stage [g], and Dm is the weight of a dry sample [g].
The bark water absorbability rate (% h–1) was obtained by equation 3:
where BWAi is the initial bark water absorbability [%], BWAf is the final bark water absorbability [%], and t is the total time of absorbality [h].
Finished 96 h submersion time, the samples were exposed in the same environmental conditions (30°C mean temperature) and weighed as it dried out at intervals of 12, 24, 36, 48, 60, 72, 96, and 144 h. Similar as BWA, subsequent values of bark water drying [BWD,%] were obtained after successive times of drying until no longer resulted in weight loss of individual bark samples The BWD rate [BWDrate,%] was obtained on the same way as BWArate (equation 4):
where BWDi is the initial bark water drying [%], BWDf is the final bark water drying [%], and t is the total time of drying [h].
Contact Angle and Bark Wettability
As aforesaid, the aim of this work is to present a novel methodology to study and measure the surface wettability of the bark in controlled laboratory conditions.
The contact surface can be idealized as homogeneous and smooth, which does not, in general, correspond to the samples measured in the laboratory. The non-idealized samples present a non-homogeneous composition and a non-smooth surface, i.e., it presents some roughness. Figure 2 presents the rough solid surface (left panel) and the ideal surface (right panel). Roughly speaking, a smooth surface is characterized as hydrophilic or hydrophobic according to CA values. For a smooth surface, for θ < 90° is a feature of hydrophilic surfaces while for roughness surfaces, θ > 90°. For a rough surface, the CA characterizing the hydrophilic/hydrophobic behavior is similar to the smooth case (For details and further explanations, please, see Drelich (2019) and references therein).
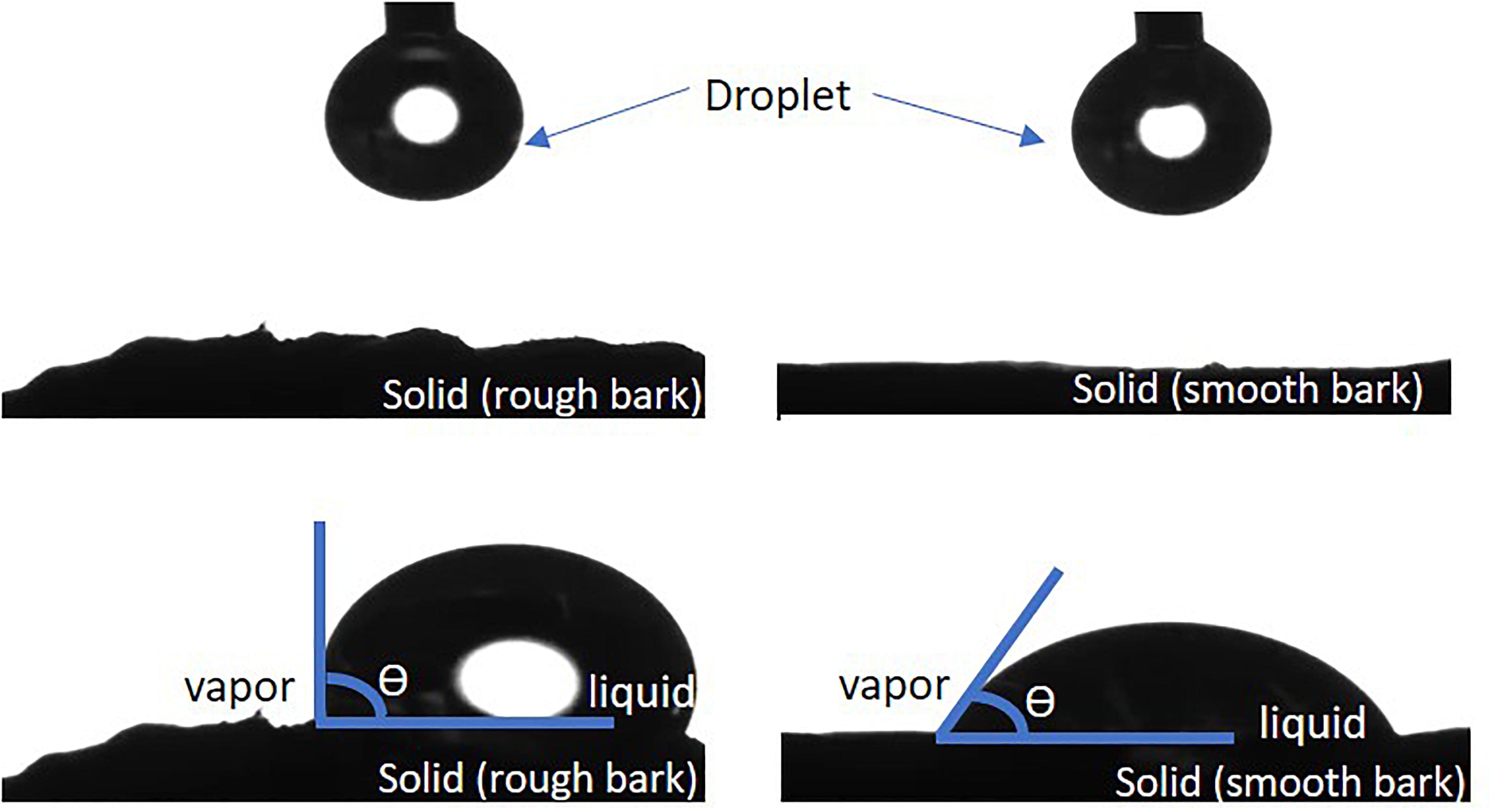
Figure 2. Example for rough and smooth bark surface and the shape of water droplet (θ = contact angle, CA).
In the present work, we assume the evaporation of the droplets can be neglected, which can occur since the observation time scale is much shorter than the expected evaporation time (Butt et al., 2007). Of course, the greater the droplet (in microscopic scale), the greater the evaporation effects. Thus, the main goal here is to study the absorption time of the droplet by the bark surface.
Considering the above explanation, one way to characterize the wettability of a solid surface, or the interaction between a liquid and a solid, is through the contact angle between the two phases. One uses here the well-known sessile droplet method for its simplicity and relative accuracy to measure the CA, in which a droplet of liquid is deposited on a smooth, horizontal surface and the angle is measured between the solid surface and the tangent of the drop profile (Erbil, 2014; Sinderski, 2020).
Measurements of CA were conducted on bark samples in the laboratory at a constant temperature of 21°C. Considering the sessile droplet method, the Ramé-Hart goniometer (Ramé-Hart Instrument Co, Netcong, NJ, United States) was used to measure the CA on the bark surfaces. The instrument was connected to a computer equipped with image recording software, droplet shape analysis, and CA measurement tools. For each species, three bark samples were selected from different individuals. A distilled water droplet (0.1 mL) was placed on external bark sides using a syringe with a 0.13 mm internal diameter needle.
The CA changed with time due to the absorption of the droplet by the solid surface. The shape of the droplet was recorded by the camera starting from second zero (placing the droplet on the bark) with 0.5-s intervals until the droplet was completely absorbed or until it reached the maximum interval of 480s adopted here. The following data were used for further analysis: the initial CA [CAin, t = 0.5s], which represents the maximum of the CA (also known as the advancing CA), the final CA [maximum interval of 480s], representing the minimum of the CA (also known as receding CA), and for CA rate [CArate - difference between the initial and final CA divided per measurement time]. For each droplet, CAs from the right (CAR) and left (CAL) sides of the droplet were obtained, as well as the mean value of both results for every second of measurement. The mean of CAin and CArate was used to characterize the species.
Bark Lignin Content
Different tree species may exhibit different behaviors based on bark constitution. Since lignin tends to be less hydrophilic than cellulose, we tried to determine the lignin content in the outer bark samples and to relate it to the contact angle. The bark lignin was determined according to the TAPPI standards for insoluble lignin (T222 om-02) (TAPPI, 2011). All characterizations were carried out in triplicates and the results correspond to the average values with their standard error.
Data Analyses
Descriptive statistics were compiled for all variables presented and regression analyses were performed to relate bark metrics to hydrologic variables. To characterized stemflow production across species, Analysis of variance (ANOVA) was applied to normal data through Tukey test at 5% probability level to analyze the means of the Sy (mm), BWA, CAin, CArate, and lignin between the tree species. Data that did not meet ANOVA assumptions were subjected to non-parametric Kruskal-Wallis test. Cluster analyses was used to identify similar traits of species between contact angle and stemflow yield. The relation between bark metrics and their effects on stemflow was analyzed by Spearman correlation. All statistical analyses were performed using MinitabV16 (Minitab, Inc., State College, PA, United States).
Results
Stemflow Variability
Total rainfall during the study period was 900 mm and stemflow average was 56.1 ± 13.0 L tree–1. Stemflow volume and monthly precipitation yield had a positive, significant linear association with rainfall for most species (Figure 3 and Supplementary Table 1). Total stemflow during the study period was 63.6 mm. Of the total stemflow, two species contributed with the most yield: 35% was contributed by Q. multiflora (22.2 mm) and 16% (10.4 mm) by H. ochraceus (Figure 4). Funneling ratios indicates that all species captured most of the P and drained as stemflow (three plants’ mean) to the surface around their stem bases (FR > 1) (Figure 5). Mean FR across all plants was 65.0 ± 24.1, however, A. peregrina was 99% lower than that. On the other hand, Q. multiflora and E. gracilipes were consistently high stemflow generators and were the only species that showed above average values.
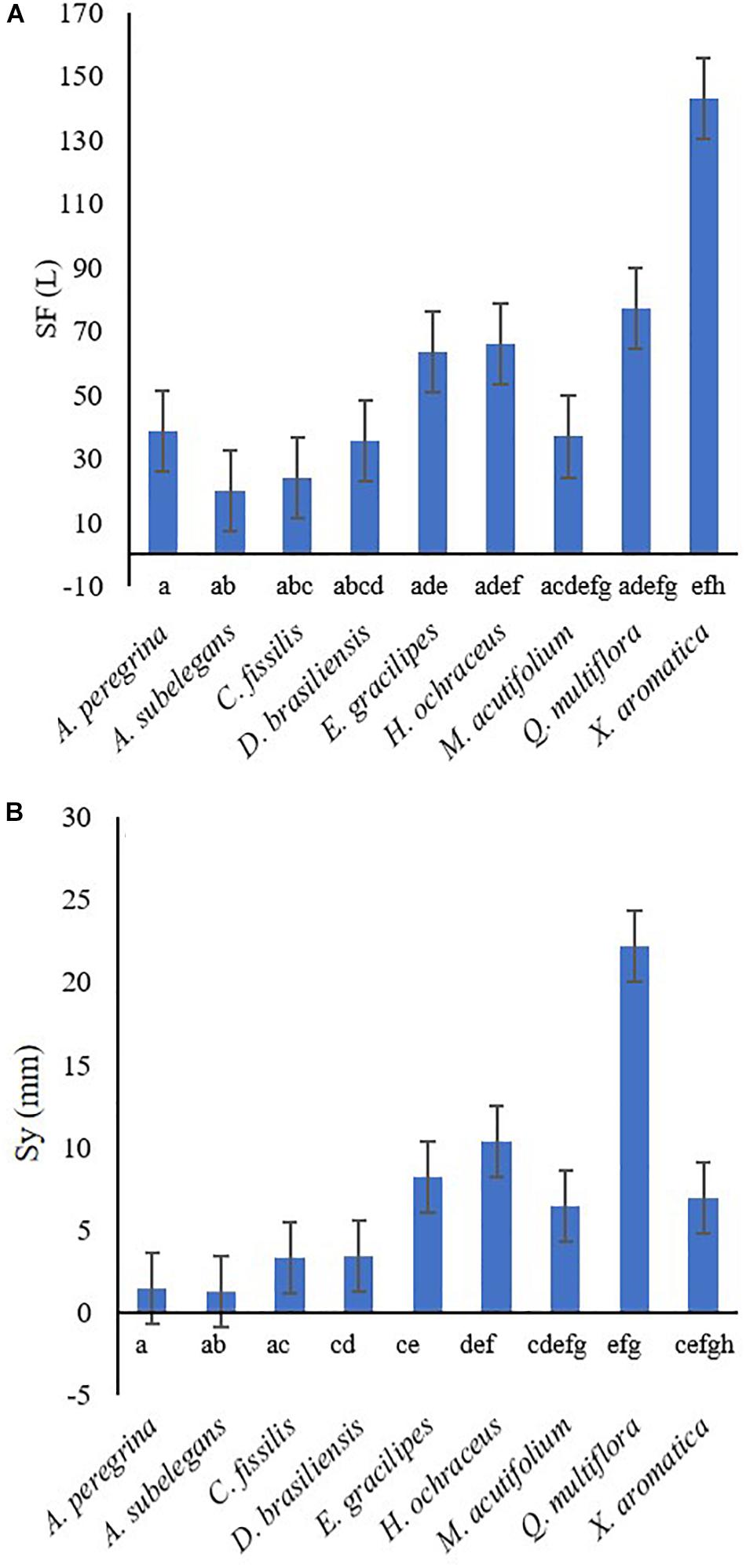
Figure 4. Mean stemflow volume [SF, L] (A) and stemflow yield [Sy, mm] (B) in 9 tree species from April 2018 to March 2019. Águas Perenes Forest, Brotas, Brazil. Different letters indicate significant differences (p < 0.05) among species and vertical bars represent standard errors.
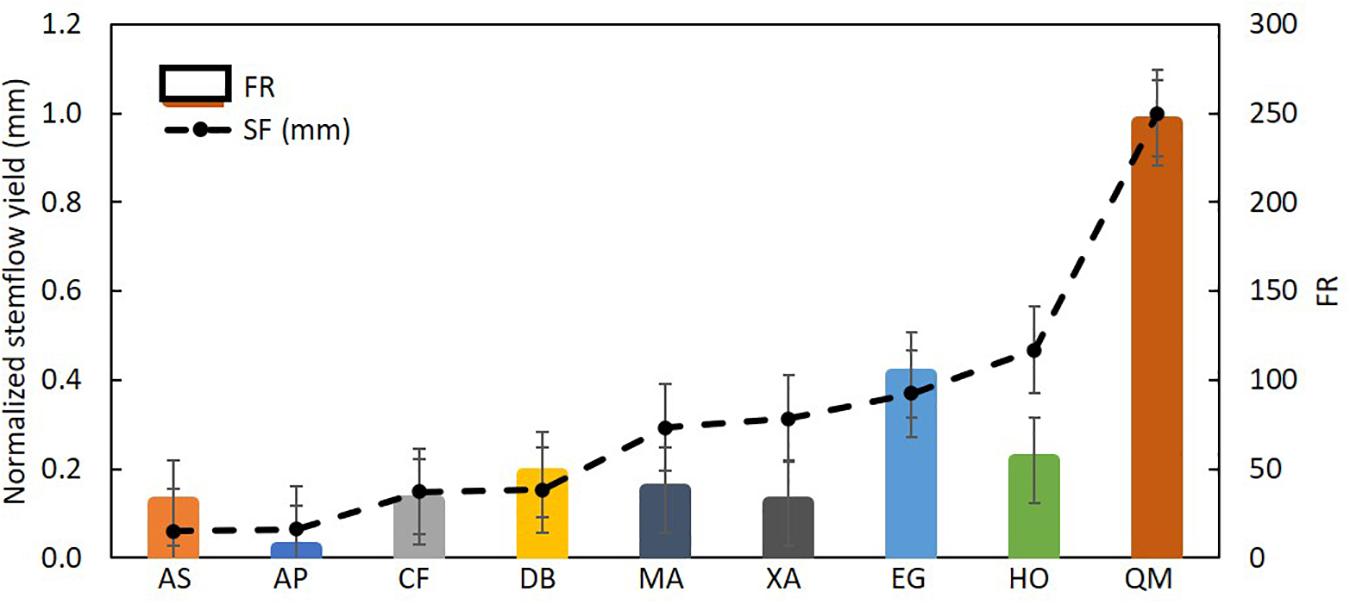
Figure 5. Mean and standard deviation [SD] of normalized stemflow yield per specie and the associated funneling ratio. AS, A. subelegans; AP, A. peregrina; CF, C. fissilis; DB, D. brasiliensis; MA, M. acutifolium; XA, X. aromatica; EG, E. gracilipes; HO, H. ochraceus; QM, Q. multiflora.
Bark Absorbability and Drying
Differences in bark water absorbability (BWA) were noted among the tree species and varied from 70.3% (C. fissilis) to 337.1% (M. acutifolium) (Table 2). At subsequent time intervals, the bark water absorbability of all species increased, and large increments of water absorption were noted in bark samples irrespective of the species. Moreover, Figure 6 shows that the species differ in their dynamics of absorbability (Figure 6A) as also in drying (Figure 6B). After each subsequent immersion time, the absorbability increment decreases. Interspecific differences are revealed in the amount of water absorbability and drying. It is also visible that water absorbability increases but tend to achieve the stability over time. For all species, BWDrate were higher than BWArate. Despite M. acutifolium had the highest BWA, this specie also shown one of the highest BWDrate (1.72% h–1). While Q multiflora and X. aromatica had one of the lowest BWArate, they also had the highest BWDrate (regression analysis for bark water absorbability (BWA) and water drying (BWD) among species are presented in Supplementary Table 2). In general, the relation between BWA and BWD are not related when analyzed with all species together (r = 0.44, p = 0.23). In contrast, the particular analysis by species points out that the BWAis has a indirect and strong relation with BWD (Table 3).
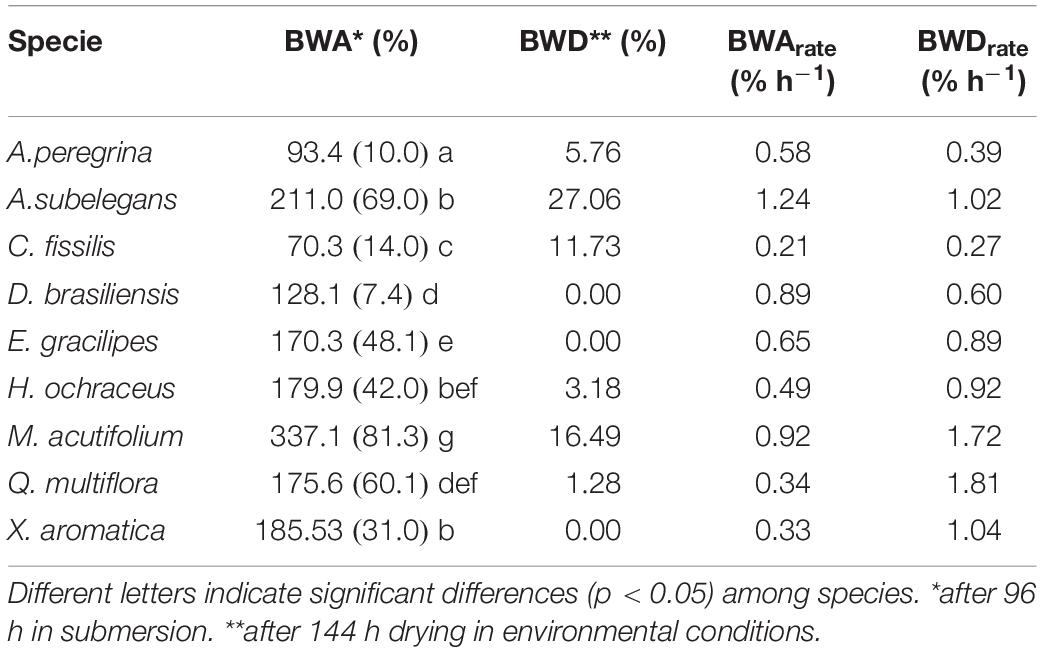
Table 2. Mean (standard error) of bark water absorbability [BWA] and rate [BWArate], and bark water drying [BWD] and rate [BWDrate].
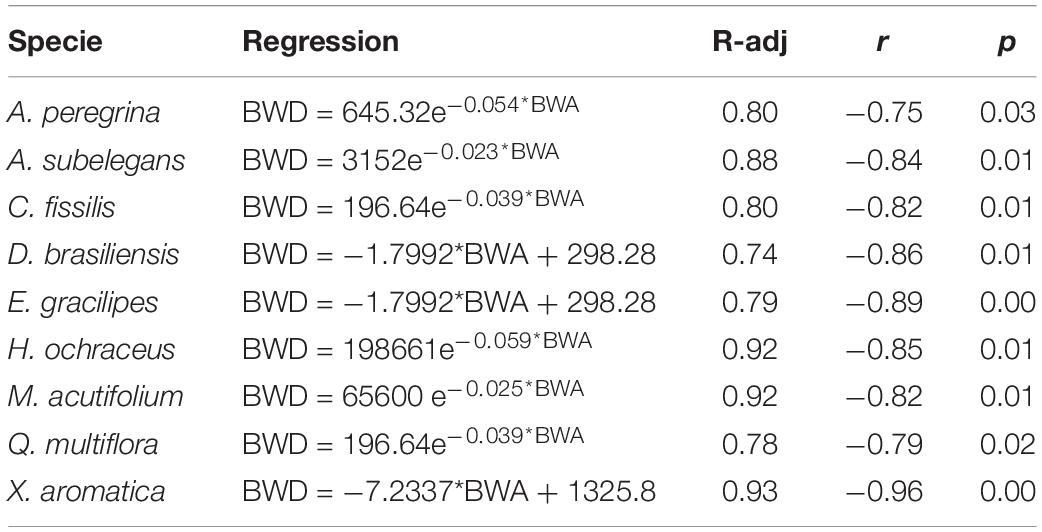
Table 3. Regression analyses for bark water drying (BWD) considering bark water absorbability (BWA).
Bark Wettability and Lignin Content
Table 4 presents the initial CA values along with bark value changes over the period of the experiment duration (CArate). During measurements using the sessile droplet method, a decrease in the CA value as a function of time was not observed for all tested barks (Figures 7 and 8). Overall, the CA behavior could be divided in two groups based on time: (G1) the one in which the droplet was absorbed completely before 480s elapsing (A. peregrina, C. fissilis, D. brasiliensis and X. aromatica), characterized here as highly wettable (CAin ≤ 75.3° and CArate ≥ 0.26° h–1) and, (G2) the other one in which the droplet was not completely absorbed before 480s elapsed (A. subelegans, E. gracilipes, H. ochraceus, M. acutifolium and Q. multiflora), it means, non-wettable (CAin ≥ 93.5° and CArate ≤ 0.13° h–1). These groups were reinforced by cluster analysis of CAin × SF (mm) presented in Figure 9. In general, the analysis of the bark lignin content has shown that the species with the lowest CAin had the lowest insoluble lignin content. A. peregrina was an exception and showed the highest lignin content in the bark samples, but for this species, lignin did not necessarily imply the greatest CAin.
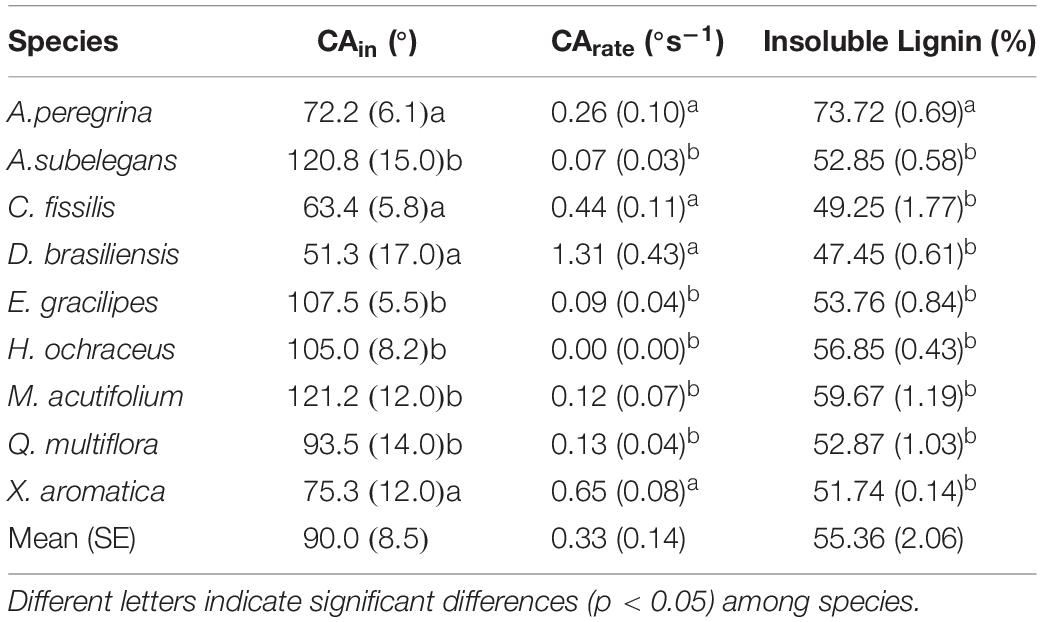
Table 4. Mean (standard error) of initial contact angle [CAin,°], CA rate [CArate,° s–1], and insoluble lignin [%] among species.
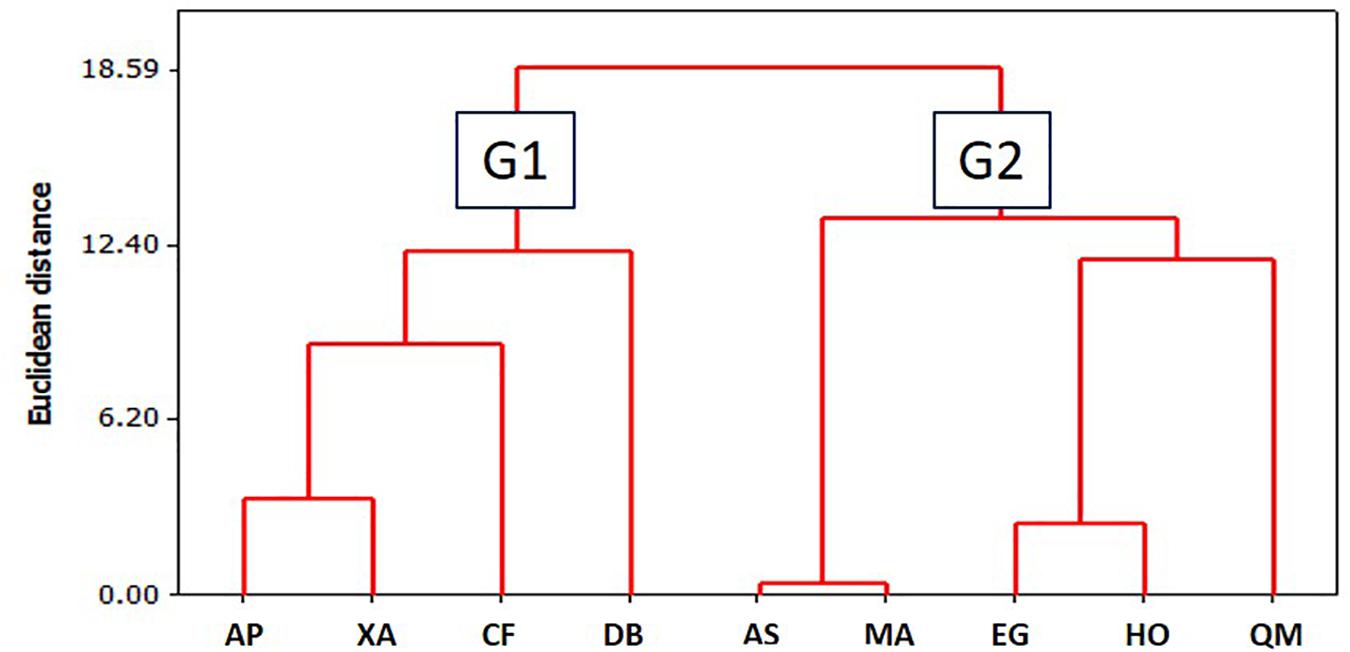
Figure 9. Cluster analysis for CAin x Sy (mm) groups. AP, A. peregrina; XA, X. aromatica; CF, C. fissilis; DB, D. brasiliensis; AS, A. subelegans; MA, M. acutifolium; EG, E. gracilipes; HO, H. ochraceus; QM, Q. multiflora; G1, highly wettable; G2, non-wettable.
Bark Traits by Contact Angle and Stemflow Correlations
To evaluate possible bark influences on stemflow variability, various directly measured metrics were compared. Few visible or strong statistical correlations or correspondences were found between bark structural variables and stemflow when the species were analyzed together (Table 5): a strong correlation was observed between CAin × BWA and moderate for BWArate × SF (L) and Lignin × SF (mm).
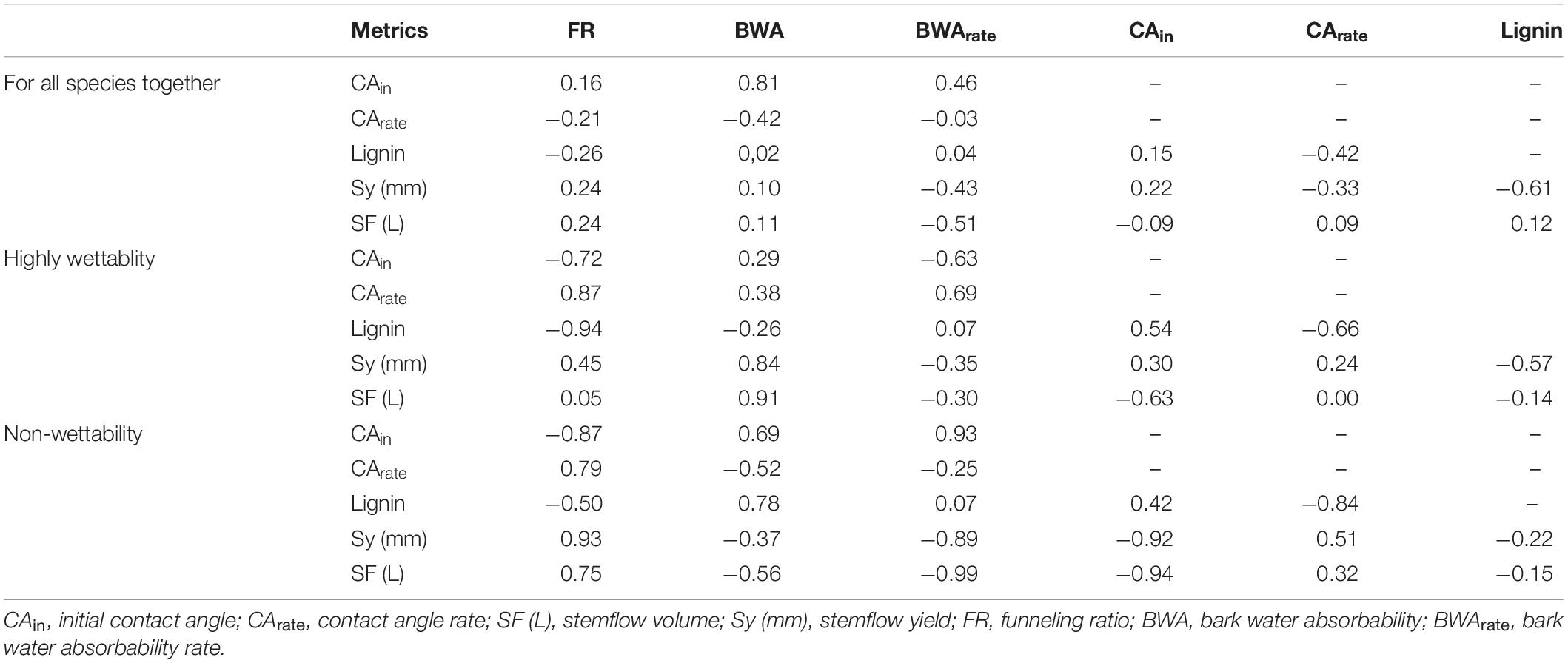
Table 5. Spearman correlations between bark properties and stemflow for all species together and per wettability groups (highly and non-wettability).
However, the analysis from the wettability groups (section “Bark Wettability and Lignin Content”) (highly wettable and non-wettable), showed that CA rate, lignin and SF (L) were approximately 700, 1, and 14% higher in highly wettable bark than in non-wettable bark. On the other hand, Sy, FR, BWA, and BWArate were, respectively, 158, 329, 80, and 46% higher in non-wettable bark (Figure 10). BWA showed a strong and positive correlation (direct relation) with stemflow (volume and depth) for highly wettable bark, whereas in non-wettable bark the correlation was weak/moderate and negative. BWArate and the classification by CAin allowed a strong relation to estimate stemflow (volume and yield) in non-wettable bark, indicating a negative correlation. FR correlated strongly with CA and stemflow variables in non-wettable bark, indicating that with increase of CAin, SF increases with FR. Finally, for highly wettable barks, lignin showed a strong and negative influence to FR, as well strong and negative correlation with CArate for non-wettable barks.
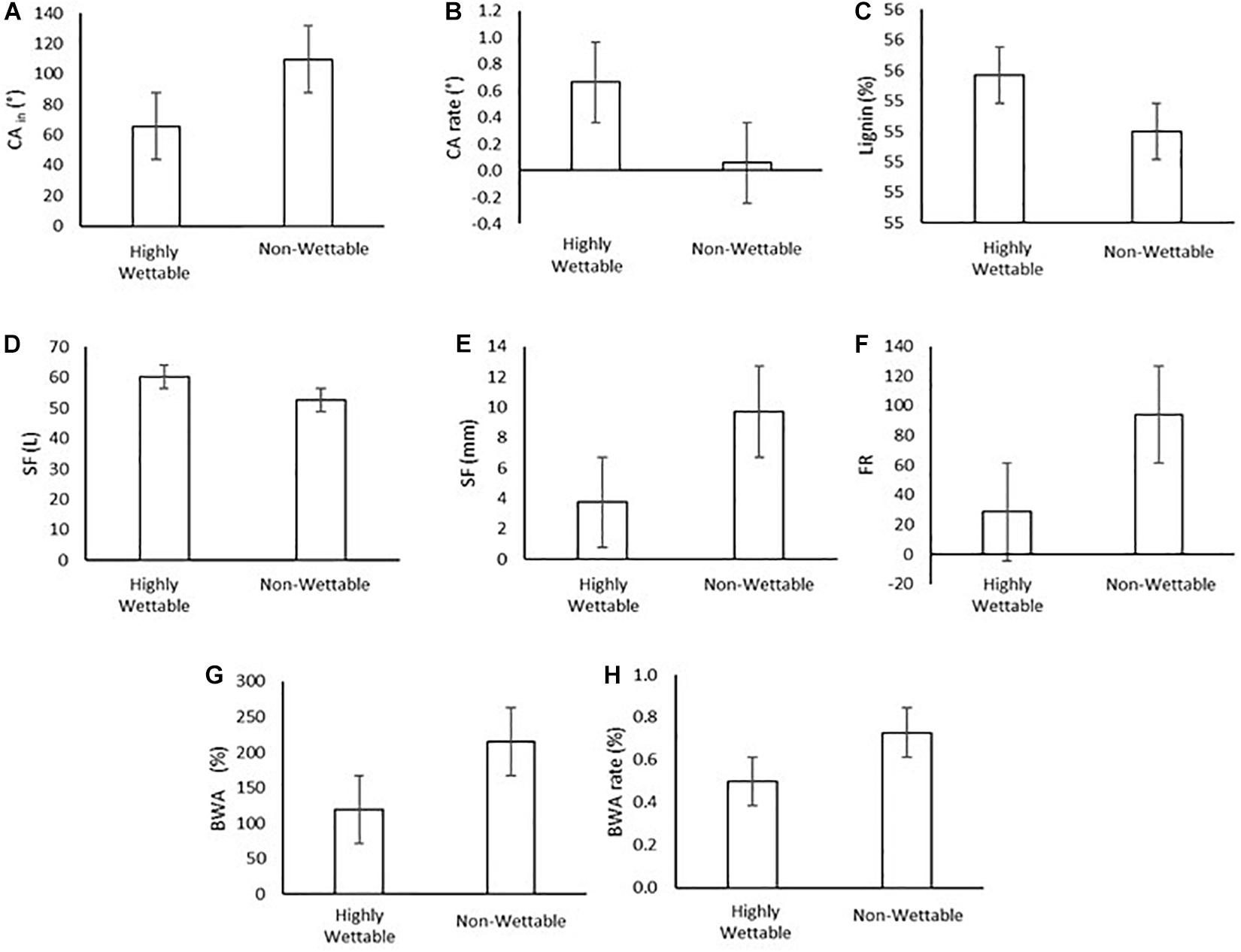
Figure 10. Mean values of initial contact angle [CAin] (A), rate contact angle [CArate] (B), lignin (C), stemflow volume, SF (L) (D), stemflow yield, Sy (mm) (E), funneling ratio, FR (F), bark water absorbability, BWA (G), bark water absorbability rate, BWArate (H).
Discussion
The knowledge of bark absorbability and wettability as well as the understanding of water retention processes on bark surfaces are particularly important and complex. Both bark water absorbability and bark water drying are dynamic processes, and they largely depend on the time during which samples are submersed in the water or exposed to the environment conditions. Considering the bark samples tested in the same way (i.e., in a controlled, systematic experiment), the features of bark water absorbability depended on the species. Apparently in this study, most of furrowed bark tends to absorb more water, although the drying behavior is independent of the bark texture. In terms of absorbability, the first 12 h of the experiment proved to be crucial for the analyzed bark samples, and a significant quantity of absorbed water was noted in the bark for all species during the experiment. After 60 h of the experiment, the bark samples showed an equilibrium tendency. In this case, only M. acutifolium was an exception, that despite presented a greater absorbability, it also presented, together with the Q. multiflora, a higher drying rate. Thus, some species can retain a big amount of water in their barks (> BWA) but they cannot store it for a longer time (< BWDrate), e.g., M. acutifolium and Q. multiflora. Furthermore, some species can retain a small amount of water in their barks (< BWA) but they can store it for a longer time (< BWDrate), e.g., C.fissilis and A. peregrina (Table 2). A similar pattern was observed in woody species bark in the Czech Republic (Valová and Bieleszová, 2008). This difference in the water absorption capacity of the bark between the species may be related to porosity and density. This variables were not measured in this study, but the literature shows that the bark with higher density and lower porosity, while having the same moisture, usually contains more water than the bark with lower density (Ilek et al., 2017a). Although the knowledge about bark absorbability is important to understand the bark and stemflow interaction, in this study these variables did not show a great correlation when we considered all species together (Table 5), and so, we could conclude that isolated BWA by submersion methodology could not be enough to relate to stemflow yield regardless of species. When the bark water storage was being studied, the main idea was that the initial condition necessary for water flow down the stem is the saturation of its bark with water (Kozlowski et al., 2010). In fact, in the bark submersion methodology, all bark surface is permanently exposed to water for a long period of time, forcing the bark to absorb water which does not happen in the field conditions. Nevertheless, in the field conditions, for the bark to absorb water as it drains down the stem, firstly, the amount of water must overcome the bark surface tension. But the field, the bark is not submerged in water. This fact could justify the strong correlations between BWA and SF for highly wettable barks, which showed a lower bark surface tension. In this way, the laboratory method seems to increase the bark saturation up to non-realistic values which cannot be found in natural conditions. Therefore, the wetting properties (rather than the lab-derived submersion estimates) could be crucial to improve the knowledge of stemflow and bark relations in the field conditions.
There is a good correlation between CA and penetration of coatings (solventborne alkyds and drying oils, for example) (Meijer, 2004), implying the wood should have a higher surface free energy than the coating (Nogalska et al., 2019). Thus, one can suppose the same occurs for the penetration of water in the bark. Different wetting processes need different approaches (Young, Wenzel or Cassie-Baxter equations) (Whyman et al., 2008). Thus, different barks may need different approaches and the evaluation of the processes that govern the stemflow generation from the CA in the controlled laboratory conditions seems to be more faithful to the field conditions. In general, small CA (<< 90°) implies a high wettability and high CA (> > 90°) corresponds to low wettability systems (Yuan and Lee, 2013; Prakash et al., 2017; Sinderski, 2020). It is important to consider that not only the initial CA value is relevant when assessing wettability, but also the time evolution of the drop (Papierowska et al., 2018). A non-wettable surface on which the droplet does not dissipate but retains its shape contributes to increasing stemflow yield more quickly, as could be observed by the inverse correlation between BWArate and SF/Sy in non-wettable barks. In turn, in highly wettable barks, the increase in stemflow is positively associated with bark water storage. It should be emphasized that this is the first study known to the authors to document the relation among bark wettability, absorbability and stemflow.
The investigation of stemflow process from bark wetting pointed to a different dynamic from that presented by other authors (Crockford and Richardson, 2000; Levia and Frost, 2003; Levia and Germer, 2015): the bark wettability groups were not formed by species with a single bark texture and it means that other bark properties must to be investigated. For highly wettable bark species there were two scaled bark species (D. brasiliensis and X. aromatic), one mixed (C. fissilis) and another with furrowed bark (A. peregrina – the thickest among those studied and with the highest lignin content), whereas in non-wettable bark group there were four furrowed and one scaled (Q. multiflora). One example of that is M. acutifolium that showed the highest BWA, and so, should have the lowest SF/Sy, but had the highest, as well as the second largest BWDrate and highest CAin. Thus, our results shown that stemflow yields are not a consequence of thick absorptive barks. Additionally, not only smooth and easily wetted bark has the potential for high stemflow yields. Although BWA was in the majority, higher for furrowed bark species, the evaluation by CAin and CArate pointed out that there is greater water repellency through the bark of these same species (non-wettability), which reflected in weak correlations between BWA vs. SF. This pattern in non-wettable bark species was reinforced by the strong and positive correlations with FR, that is, the potential to generate stemflow. On the other hand, BWA for highly wettable bark species could be a good physical parameter to understand the SF yields, since they presented strong/positive correlations (Table 5).
The tree species did not differ in terms of bark lignin content - except A. peregrina. There exists the long-held belief that one of the functions of lignin in the wood cell wall is to provide waterproofing to aid in water transport (Notley and Norgren, 2010), so, this fact could justify a non-influence of lignin in the process of bark water absorbability, wetting, and stemflow. Evidence of lignin’s importance relative to the wettability of wood comes from observations of the effects of weathering. Weathered wood tends to become depleted of lignin near to its surface, which is attributable to photodegradation of the aromatic structures (Hubbe et al., 2015). Indeed, the depletion of lignin in the surface layers of wood exposed to outdoor sunlight has been shown to result in increased wettability by water (Feist, 1993; Huang et al., 2012; Hubbe et al., 2015). Despite that, the association of stemflow and lignin showed that the best analyses were when we considered all species, independent of their wettability properties. In this situation, the lignin was associated with a lower stemflow yield. For highly wettable bark, the lignin had a moderate and inverse correlation with SF(L), and, thus, for these species lower lignin implies increases in stemflow generation. In contrast, for non-wettable bark species, the increment of lignin is associated with decreased of CArate, that on the other hand, is also implicated in the increment of bark water absorbability.
The dynamics of stemflow are a very important element of water balance in the environment. Knowledge gained from this study may augment our understanding in several aspects. Knowing the bark properties and stemflow dynamics for different tree species can help better understand and predict the role of forests in rainfall interception and water balance. Moreover, could be potentially used as a trait to determine plants that could be used for restoration projects, for example. Regarding the physiological ecology of forests, once bark provides a diverse substrate for a variety of these communities (Levia and Germer, 2015), the proposed method may have important implications for understanding the distribution and diversity of epiphytic lichens, bryophytes (Mitchell et al., 2005; Levia and Wubbena, 2006; Valová and Bieleszová, 2008; Van Stan and Pypker, 2015), fungal (Magyar et al., 2021) and metazoans (Ptatscheck et al., 2018), which, in turn, has a detectable and significant influence on stemflow chemistry (Pypker et al., 2011; Levia and Germer, 2015; Van Stan and Pypker, 2015) and, consequently, to the forest floor.
Conclusion
The present study shows how the tree bark traits is related to stemflow in 9 species commonly found in Cerrado. We investigated the association of bark absorbability, wettability, lignin content and stemflow yields. The results obtained here confirm that bark absorbability and wetting depend on the tree species and reject the long-held paradigm that bark surface structure (smooth vs rough) is a major determinant of stemflow (at least in the Cerrado). We obtain important information on factors conditioning the stemflow yield, confirming how difficult is to consider the bark traits individually. The insoluble lignin did not differ among the most species but showed to have a moderate and negative association with stemflow. The combination of wetting properties with stemflow, allowed to classify the species as highly wettable and non-wettable barks and then, the relations between bark traits and the stemflow dynamic became clearer. Highly wettable barks showed a strong and positive correlation between bark absorbability and stemflow, whereas for non-wettable barks the absorbability had a negative and moderate relation. Thus, the classification of wettability had a substantial effect on stemflow yield and proved to be an important variable to connect the laboratory and the field investigation, leading to a better understanding of the stemflow yield.
Data Availability Statement
The original contributions presented in the study are included in the article/Supplementary Material, further inquiries can be directed to the corresponding author.
Author Contributions
KT and AM contributed to conception and design of the experiment. KT, AM, and SM conducted the experiment and organized the database. KT, JB, and ML performed the statistical analyses and wrote the first draft of the manuscript. SC performed the physical analyses and part of the discussion. All authors contributed to manuscript revision, read, and approved the submitted version.
Conflict of Interest
The authors declare that the research was conducted in the absence of any commercial or financial relationships that could be construed as a potential conflict of interest.
Acknowledgments
The authors would like to thank the Brazilian National Council for Scientific and Technological Development (CNPq) and International Paper Co-Brazil.
Supplementary Material
The Supplementary Material for this article can be found online at: https://www.frontiersin.org/articles/10.3389/ffgc.2021.650665/full#supplementary-material
References
Ahmadi, M. T., Attarod, P., and Bayramzadeh, V. (2013). The role of rainfall size in canopy interception loss: an observational study in a typical beech forest. Middle-East J. Sci. Res. 13, 876–882. doi: 10.5829/idosi.mejsr.2013.13.7.2721
Aryal, B., and Neuner, G. (2010). Leaf wettability decreases along an extreme altitudinal gradient. Oecologia 162, 1–9. doi: 10.1007/s00442-009-1437-3
Astiani, D., Mujiman, M., and Curran, L. M. (2017). Trees of tropical peatland forest influence on variability of water and carbon input through stemflow. Biodiversitas 18, 383–388. doi: 10.13057/biodiv/d180150
Blow, F. E. (1985). Quantity and hydrologic characteristics of litter under upland oak forests in eastern Tennessee. J. For. 53, 190–195.
Butt, H. J., Golovko, D. S., and Bonaccurso, E. (2007). On the derivation of young’s equation for sessile drops: nonequilibrium effects due to evaporation. J. Phys. Chem. B 111, 5277–5283. doi: 10.1021/jp065348g
Corti, G., Agnelli, A., Cocco, S., Cardelli, V., Masse, J., and Courchesne, F. (2019). Soil affects throughfall and stemflow under Turkey oak (Quercus cerris L.). Geoderma 333, 43–56. doi: 10.1016/j.geoderma.2018.07.010
Crockford, R. H., and Richardson, D. P. (2000). Partitioning of rainfall into throughfall, stemslow∠d interception effect of forest type, ground cover and climate. Hydrol. Process. 14, 2903–2920.
de Leite, M. L. T. A., and Ribeiro, W. C. (2018). The Guarani Aquifer System (Gas) and the challenges for its management. J. Water Resour. Prot. 10, 1222–1241. doi: 10.4236/jwarp.2018.1012073
dos Santos, H. G., Jacomine, P. K. T., dos Anjos, L. H. C., de Oliveira, V. A., Lumbreras, J. F., Coelho, M. R., et al. (2018). Sistema Brasileiro de Classificação de Solos. Rio de Janeiro: Embrapa Solos.
Drelich, J. W. (2019). Contact angles: from past mistakes to new developments through liquid-solid adhesion measurements. Adv. Colloid Interface Sci. 267, 1–14. doi: 10.1016/j.cis.2019.02.002
Drelich, J. W., Boinovich, L., Chibowski, E., Della Volpe, C., Hołysz, L., Marmur, A., et al. (2020). Contact angles: history of over 200 years of open questions. Surf. Innov. 8, 3–27. doi: 10.1680/jsuin.19.00007
Dubreuil, V., Fante, K. P., Planchon, O., and Sant’Anna Neto, J. L. (2019). Climate change evidence in Brazil from Köppen’s climate annual types frequency. Int. J. Climatol. 39, 1446–1456. doi: 10.1002/joc.5893
Erbil, H. Y. (2014). The debate on the dependence of apparent contact angles on drop contact area or three-phase contact line: a review. Surf. Sci. Rep. 69, 325–365. doi: 10.1016/j.surfrep.2014.09.001
Feist, W. C. (1993). “Weathering and surface protection of wood,” in Proceeding of the Seventy-Ninth Annual Meeting of the American Wood-Preservers’ Association, (Stevensville, MD: American Wood- Preservers’ Association), 195–205. doi: 10.1016/B978-081551500-5.50016-1
Gordon, D. A. R., Coenders-Gerrits, M., Sellers, B. A., Sadeghi, S. M. M., and Van Stan, J. T. (2020). Rainfall interception and redistribution by a common North American understory and pasture forb, Eupatorium capillifolium (Lam. dogfennel). Hydrol. Earth Syst. Sci. 24, 4587–4599. doi: 10.5194/hess-24-4587-2020
Graves, S. J., Rifai, S. W., and Putz, F. E. (2014). Outer bark thickness decreases more with height on stems of fire-resistant than fire-sensitive Floridian oaks (Quercus spp. Fagaceae). Am. J. Bot. 101, 2183–2188. doi: 10.3732/ajb.1400412
Herwitz, S. R. (1986). Infiltration-excess caused by Stemflow in a cyclone-prone tropical rainforest. Earth Surf. Process. Landforms 11, 401–412. doi: 10.1002/esp.3290110406
Herwitz, S. R. (1987). Raindrop impact and water flow on the vegetative surfaces of trees and the effects on stemfow and throughfall generation. Earth Surf. Process. Landforms 12, 425–432. doi: 10.1002/esp.3290120408
Herwitz, S. R., and Slye, R. E. (1995). Three-dimensional modeling of canopy tree interception of wind-driven rainfall. J. Hydrol. 168, 205–226. doi: 10.1016/0022-1694(94)02643-P
Holder, C. D. (2020). “Advances and future research directions in the study of leaf water repellency,” in Forest-Water Interactions, ed. D. F. Levia (Cham: Springer), 261–278. doi: 10.1007/978-3-030-26086-6
Honda, E. A., Mendonça, A. H., and Durigan, G. (2015). Factors affecting the stemflow of trees in the Brazilian Cerrado. Ecohydrology 8, 1351–1362. doi: 10.1002/eco.1587
Huang, X., Kocaefe, D., Kocaefe, Y., Boluk, Y., and Pichette, A. (2012). Changes in wettability of heat-treated wood due to artificial weathering. Wood Sci. Technol. 46, 1215–1237. doi: 10.1007/s00226-012-0479-6
Hubbe, M. A., Gardner, D. J., and Shen, W. (2015). Contact angles and wettability of cellulosic surfaces: a review of proposed mechanisms and test strategies. BioResources 10, 8657–8749.
Ilek, A., and Jarosław, K. (2014). Hydrological properties of bark of selected forest tree species. Part I: the coefficient of development of the interception surface of bark. Trees - Struct. Funct. 28, 831–839. doi: 10.1007/s00468-014-0995-0
Ilek, A., Kucza, J., and Morkisz, K. (2017a). Hygroscopicity of the bark of selected forest tree species. IForest - Biogeosci. Forestry 10, 220–226. doi: 10.3832/ifor1979-009
Ilek, A., Kucza, J., and Morkisz, K. (2017b). Hydrological properties of bark of selected forest tree species. Part 2: interspecific variability of bark water storage capacity. Folia For. Pol. Ser. A 59, 110–122. doi: 10.1515/ffp-2017-0011
Ilek, A., Kucza, J., and Szostek, M. (2015). The effect of stand species composition on water storage capacity of the organic layers of forest soils. Eur. J. For. Res. 134, 187–197. doi: 10.1007/s10342-014-0842-2
Johnson, M. S., and Lehmann, J. (2006). Double-funneling of trees: stemflow and root-induced preferential flow. Écoscience 13, 324–333. doi: 10.2980/i1195-6860-13-3-324.1
Klamerus-Iwan, A., and Błońska, E. (2018). Canopy storage capacity and wettability of leaves and needles: the effect of water temperature changes. J. Hydrol. 559, 534–540. doi: 10.1016/j.jhydrol.2018.02.032
Klamerus-Iwan, A., Łagan, S., Zarek, M., Słowik-Opoka, E., and Wojtan, B. (2020a). Variability of leaf wetting and water storage capacity of branches of 12 deciduous tree species. Forests 11:1158. doi: 10.3390/f11111158
Klamerus-Iwan, A., Lasota, J., and Blonska, E. (2020b). Interspecific variability of water storage capacity and absorbability of deadwood. Forests 11:575. doi: 10.3390/f11050575
Klamerus-Iwan, A., Link, T. E., Klein, R. F., and Van Stan, J. T. (2020c). “Storage and routing of precipitation through canopies,” in Precipitation Partitioning by Vegetation: A Global Synthesis, eds T. John, Van Stan and J. F. Ethan Gutmann (Berlin: Springer Nature Switzerland), 17–34.
Kozlowski, R., Józwiak, M. A., and Borowska, E. (2010). “Porównanie wybranych metod do obliczania wysokosci opadu splywajacego po pniach [Comparison of selected methods for calculation of stemflow volume],” Monitoring Srodowiska Przyrodniczego, (Kielce: Kieleckie Towarzystwo Naukowe, 11: 25–33.
Levia, D. F. (2003). Winter stemflow leaching of nutrient-ions from deciduous canopy trees in relation to meteorological conditions. Agric. For. Meteorol. 117, 39–51. doi: 10.1016/S0168-1923(03)00040-6
Levia, D. F., and Frost, E. E. (2003). A review and evaluation of stemflow literature in the hydrologic and biogeochemical cycles of forested and agricultural ecosystems. J. Hydrol. 274, 1–29. doi: 10.1016/S0022-1694(02)00399-2
Levia, D. F., and Germer, S. (2015). A review of stemflow generation dynamics and stemflow-environment interactions in forests and shrublands. Rev. Geophys. 53, 673–714.
Levia, D. F., and Herwitz, S. R. (2005). Interspecific variation of bark water storage capacity of three deciduous tree species in relation to stemflow yield and solute flux to forest soils. Catena 64, 117–137. doi: 10.1016/j.catena.2005.08.001
Levia, D. F., Van Stan, J. T., Mage, S. M., and Kelley-Hauske, P. W. (2010). Temporal variability of stemflow volume in a beech-yellow poplar forest in relation to tree species and size. J. Hydrol. 380, 112–120. doi: 10.1016/j.jhydrol.2009.10.028
Levia, D. F., and Wubbena, N. P. (2006). Vertical variation of bark water storage capacity of Pinus strobus L. (Eastern White Pine) in Southern Illinois. Northeast. Nat. 13, 131–137.
Liu, G., Du, S., Peng, S., and Wang, G. (2014). Rainfall interception in two contrasting forest types in the mount gongga area of eastern tibet. China. Hydrol. Curr. Res. 4, 1–6. doi: 10.4172/2157-7587.1000161
Magyar, D., Van Stan, J. T., and Sridhar, K. R. (2021). Hypothesis and theory: fungal spores in stemflow and potential bark sources. Frontiers in Forests and Global Change 4 4: 1–18. doi: 10.3389/ffgc.2021.623758
Meijer, M. (2004). “A review of interfacial aspects in wood coatings: wetting, surface energy, substrate penetration and adhesion,” in Proceedings of the COST E18, (Paris), 1–16.
Metzger, J. C., Schumacher, J., Lange, M., and Hildebrandt, A. (2019). Neighbourhood and stand structure affect stemflow generation in a heterogeneous deciduous temperate forest. Hydrol. Earth Syst. Sci. 23, 4433–4452. doi: 10.5194/hess-23-4433-2019
Mitchell, R. J., Truscot, A. M., Leith, I. D., Cape, J. N., Van Dijk, N., Tang, Y. S., et al. (2005). A study of the epiphytic communities of Atlantic oak woods along an atmospheric nitrogen deposition gradient. J. Ecol. 93, 482–492. doi: 10.1111/j.1365-2745.2005.00967.x
Molnar, I. L., O’Carroll, D. M., and Gerhard, J. I. (2011). Impact of surfactant-induced wettability alterations on DNAPL invasion in quartz and iron oxide-coated sand systems. J. Contam. Hydrol. 119, 1–12. doi: 10.1016/j.jconhyd.2010.08.004
Neiva, D. M., Rencoret, J., Marques, G., Gutiérrez, A., Gominho, J., Pereira, H., et al. (2020). Lignin from tree barks: chemical structure and valorization. ChemSusChem 13, 4537–4547. doi: 10.1002/cssc.202000431
Nogalska, A., Trojanowska, A., Tylkowski, B., and Garcia-Valls, R. (2019). Surface characterization by optical contact angle measuring system. Phys. Sci. Rev. 5, 1–13. doi: 10.1515/psr-2019-0083
Notley, S. M., and Norgren, M. (2010). Surface energy and wettability of spin-coated thin films of lignin isolated from wood. Langmuir 26, 5484–5490. doi: 10.1021/la1003337
Papierowska, E., Szporak-Wasilewska, S., Szewińska, J., Szatyłowicz, J., Debaene, G., and Utratna, M. (2018). Contact angle measurements and water drop behavior on leaf surface for several deciduous shrub and tree species from a temperate zone. Trees - Struct. Funct. 32, 1253–1266. doi: 10.1007/s00468-018-1707-y
Pereira, L. C., Balbinot, L., Matus, G. N., Dias, H. C. T., and Tonello, K. C. (2021). Aspects of forest restoration and hydrology: linking passive restoration and soil – water recovery in Brazilian Cerrado. J. For. Res. doi: 10.1007/s11676-021-01301-3
Prakash, C. G. J., Clement Raj, C., and Prasanth, R. (2017). Fabrication of zero contact angle ultra-super hydrophilic surfaces. J. Colloid Interface Sci. 496, 300–310. doi: 10.1016/j.jcis.2017.01.007
Ptatscheck, C., Milne, P. C., and Traunspurger, W. (2018). Is stemflow a vector for the transport of small metazoans from tree surfaces down to soil? BMC Ecol. 18:43. doi: 10.1186/s12898-018-0198-4
Pypker, T. G., Levia, D. F., Staelens, J., and Van Stan, J. T. (2011). “Canopy structure in relation to hydrological and biogeochemical fluxes,” in Forest Hydrology and Biogeochemistry, Synthesis of Past Research and Future Directions, eds D. F. Levia, D. Carlyle-Moses, and T. Tanaka (Dordrecht: Springer), doi: 10.1007/978-94-007-1363-5
Rosado, B. H. P., and Holder, C. D. (2013). The significance of leaf water repellency in ecohydrological research: a review. Ecohydrology 6, 150–161. doi: 10.1002/eco.1340
Sadeghi, S. M. M., Gordon, D. A., Van Stan, J. T. (2020). “A global synthesis of throughfall and stemflow hydrometeorology,” in Precipitation Partitioning by Vegetation: A Global Synthesis, eds T. John, J. F. Van Stan, E. Gutmann, and J. Friesen (Berlin: Springer Nature Switzerland), 49–70. doi: 10.1007/978-3-030-29702-2
Siegert, C. M., and Levia, D. F. (2014). Seasonal and meteorological effects on differential stemflow funneling ratios for two deciduous tree species. J. Hydrol. 519, 446–454. doi: 10.1016/j.jhydrol.2014.07.038
Sinderski, L. G. Z. (2020). Ângulo de contato e rugosidade de madeiras, uma breve revisão. Rev. Ciência da Madeira 11, 1–11. doi: 10.12953/2177-6830/rcm.v11n1p1-11
Spencer, S. A., and van Meerveld, H. J. (2016). Double funnelling in a mature coastal British Columbia forest: spatial patterns of stemflow after infiltration. Hydrol. Process. 30, 4185–4201. doi: 10.1002/hyp.10936
Valová, M., and Bieleszová, S. (2008). Interspecific variations of bark’s water storage capacity of chosen types of trees and the dependance on occurance of epiphytic mosses. Geosci. Eng. LIV 4, 45–51.
Van Stan, J. T., and Allen, S. T. (2020). What we know about stemflow’s infiltration area. Front. For. Glob. Chang. 3:61. doi: 10.3389/ffgc.2020.00061
Van Stan, J. T., and Gordon, D. A. (2018). Mini-Review: stemflow as a resource limitation to near-stem soils. Front. Plant Sci. 9:248. doi: 10.3389/fpls.2018.00248
Van Stan, J. T., and Levia, D. F. (2010). Inter- and intraspecific variation of stemflow production from Fagus grandifolia ehrh. (American beech) and Liriodendron tulipifera L. (yellow poplar) in relation to bark microrelief in the eastern United States. Ecohydrology 3, 11–19. doi: 10.1002/eco
Van Stan, J. T., Lewis, E. S., Hildebrandt, A., Rebmann, C., and Friesen, J. (2016). Impact of interacting bark structure and rainfall conditions on stemflow variability in a temperate beech-oak forest, central Germany. Hydrol. Sci. J. 61, 2071–2083. doi: 10.1080/02626667.2015.1083104
Van Stan, J. T., and Pypker, T. G. (2015). A review and evaluation of forest canopy epiphyte roles in the partitioning and chemical alteration of precipitation. Sci. Total Environ. 536, 813–824. doi: 10.1016/j.scitotenv.2015.07.134
Van Stan, J. T., Siegert, C. M., Levia, D. F., and Scheick, C. E. (2011). Effects of wind-driven rainfall on stemflow generation between codominant tree species with differing crown characteristics. Agric. For. Meteorol. 151, 1277–1286. doi: 10.1016/j.agrformet.2011.05.008
Van Stan, J. T., Hildebrandt, A., Friesen, J., Metzger, J. C., and Yankine, S. A. (2020). “Spatial variability and temporal stability of local net precipitation patterns,” in Precipitation Partitioning by Vegetation, eds T. John, J. F. Van Stan, E. Gutmann, and J. Friesen (Cham: Springer), 89–104.
Voigt, G. K., and Zwolinski, M. J. (1964). Absorption of stem ow by bark of young red and white pines sign in oxford academic account sign in via society site sign in via your institution short-term access. For. Sci. 10, 277–282. doi: 10.1093/forestscience/10.3.277
Whyman, G., Bormashenko, E., and Stein, T. (2008). The rigorous derivation of young, cassie-baxter and wenzel equations and the analysis of the contact angle hysteresis phenomenon. Chem. Phys. Lett. 450, 355–359. doi: 10.1016/j.cplett.2007.11.033
Yuan, Y., and Lee, T. R. (2013). “Contact angle and wetting properties,” in Surface Science Techniques, eds G. Bracco and B. Holst (Berlin: Springer-Verlag), 1–32. doi: 10.1007/978-3-642-34243-1
Keywords: surface tension, water drop, water repellency, water storage capacity, insoluble lignin
Citation: Tonello KC, Campos SD, Menezes AJ, Bramorski J, Mathias SL and Lima MT (2021) How Is Bark Absorbability and Wettability Related to Stemflow Yield? Observations From Isolated Trees in the Brazilian Cerrado. Front. For. Glob. Change 4:650665. doi: 10.3389/ffgc.2021.650665
Received: 07 January 2021; Accepted: 07 April 2021;
Published: 12 May 2021.
Edited by:
Anna Klamerus-Iwan, University of Agriculture in Krakow, PolandReviewed by:
Luiza Maria Teophilo Aparecido, Arizona State University, United StatesAnna Ilek, Poznań University of Life Sciences, Poland
Copyright © 2021 Tonello, Campos, de Menezes, Bramorski, Mathias and Lima. This is an open-access article distributed under the terms of the Creative Commons Attribution License (CC BY). The use, distribution or reproduction in other forums is permitted, provided the original author(s) and the copyright owner(s) are credited and that the original publication in this journal is cited, in accordance with accepted academic practice. No use, distribution or reproduction is permitted which does not comply with these terms.
*Correspondence: Kelly Cristina Tonello, kellytonello@ufscar.br