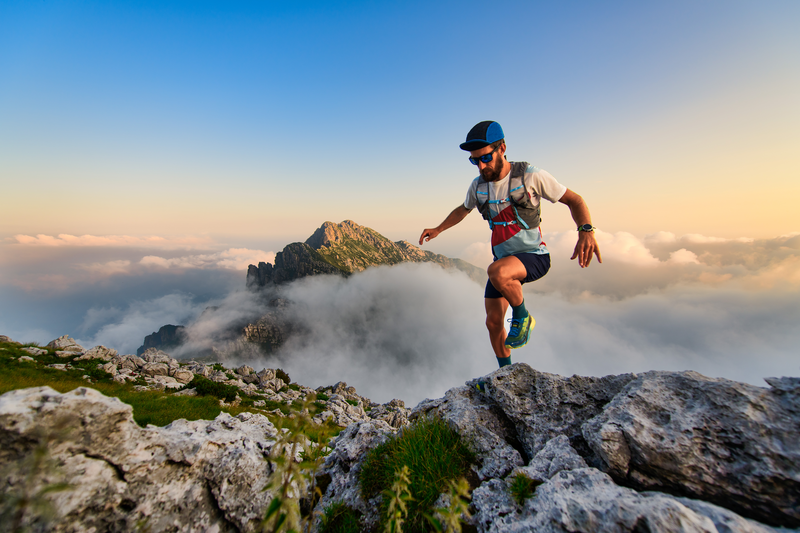
95% of researchers rate our articles as excellent or good
Learn more about the work of our research integrity team to safeguard the quality of each article we publish.
Find out more
ORIGINAL RESEARCH article
Front. For. Glob. Change , 21 April 2021
Sec. Forest Ecophysiology
Volume 4 - 2021 | https://doi.org/10.3389/ffgc.2021.647360
Continuous nitrogen (N) deposition has raised concerns that temperate forest ecosystems shift from N limitation to progressing phosphorus (P) limitation under global change. According to the multiple resource limitation theory, this will not only influence P economy, but also reduce N uptake and use efficiencies of trees such that growth is equally limited by N and P. We used different global change scenarios including summer drought to test the prediction of this hypothesis for the N economy of European beech (Fagus sylvatica L.) saplings. Our study demonstrates that the N uptake efficiency (NUptakeE) of beech adjusts to soil N availability and stabilizes leaf and fine root N concentrations. By contrast, both efficiencies of N use were curtailed by increasing P limitation under elevated soil N. The photosynthetic N use efficiency (PNUE) was serially reduced with decreasing soil P availability and increasing foliar N:P ratios, while the N use efficiency (NUE) decreased with increasing fine root N:P ratios. Soil drought induced relative P deficiency alike and reduced NUptakeE, PNUE, and NUE independent from the soil N:P ratios. We conclude that not only N deposition but also increasing summer droughts might affect N:P ratios, thereby inducing P imbalances and affecting the N economy of European beech saplings under global change.
The net primary production of temperate forests is primarily limited by N availability (LeBauer and Treseder, 2008). In recent decades, N availability has rapidly increased in most areas of the globe due to increased N deposition from fossil fuel combustion and intensive agriculture (Galloway et al., 2008). Increased N availability changes nutrient stoichiometry, i.e., leads to rises in the N:P ratio of ecosystems (Peñuelas et al., 2013). As a result, the degree of relative P deficiency increases and potentially shifts temperate forests from N limitation to P limitation (Talkner et al., 2015; Sardans et al., 2016; Hedwall et al., 2017).
According to co-limitation hypothesis, N acquisition and use efficiencies depend on P availability and vice versa (Ågren et al., 2012). According to this theory, P deficiency will increase P uptake efficiency (PUptakeE, that is the P content in recent biomass relative to the amount of available soil P; Fujita et al., 2010), but simultaneously may also reduce N uptake efficiency (NUptakeE, that is the N content in recent biomass relative to the amount of soil N) and N use efficiency (NUE, that is the increment in biomass for a given increase in biomass N). Yet there is currently no mechanistic understanding if (at all) plants achieve simultaneous co-limitation by N and P via modifications in root uptake kinetics (Rothstein et al., 2000) or via changes in root morphology and biomass partitioning (Zhang et al., 2018) or both.
Mycorrhizal symbiosis plays a major role in the P acquisition (Ferlian et al., 2018; Raven et al., 2018) and (to some extent) in the N acquisition of plants (Gobert and Plassard, 2008; Pena and Polle, 2014). Ectomycorrhizal fungi (ECMF) species are functionally diverse and vary in their influence on N acquisition (Pena and Polle, 2014), which makes the ECMF community composition an important factor for nutrient acquisition. In addition, the degree of mycorrhizal colonization affects the portion of mycorrhizal control on resource acquisition. Yet mycorrhizal control may greatly differ between P and N acquisition: low mobility of orthophosphates causes depletion zones around roots and may raise the importance of ECMF hyphae for P access beyond the root depletion zone, while this effect seems less important for highly mobile nitrate.
Nutrient availability also depends on water availability (Gessler et al., 2017). In dry soil, nutrient transport to roots through mass flow and diffusion is slowed down, which can induce edaphic N limitation and reduce the N uptake capacity of trees even in N-rich soil. Soil drought impairs soil microbes and can shift the mycorrhizal community composition with potential consequences for the nutrient uptake of the host tree (Leberecht et al., 2016). This is likely the case in severely dry soil in which the allocation of photosynthates to belowground structures is inadequate (Ruehr et al., 2009). Finally, drought can also decrease root vitality (Burton et al., 1998; Huang et al., 2005; Köhler et al., 2018) and induce fine root dieback (Meier and Leuschner, 2008), which reduces the active root surface area for nutrient uptake. However, the dependence of nutrient stoichiometry and nutrient co-limitation of important temperate forest trees on soil drought as expected under global change are not well understood (Korell et al., 2019).
In previous research, we examined the consequences of different global change scenarios (i.e., elevated temperature, N deposition, reduced soil moisture, and P availability) for P uptake and use efficiencies of European beech saplings (Köhler et al., 2018). We demonstrated that P uptake efficiency of beech in dry soils significantly decreased with decreasing ECMF α diversity (i.e., the Shannon-Weaver index based on the total number of ECMF species of individual beech sapling), whereas the P use efficiency was negatively related to soil P availability. In the related current study, we aimed to investigate the effect of global change on the N economy of European beech saplings in a four-factorial climate-chamber experiment that simulated the expected conditions for Central Europe. We hypothesized that (H1) beech achieves co-limitation by N and P under increasing soil N availability since NUptakeE and NUE decrease, (H2) increasing ECMF colonization rates and diversity increase NUptakeE, and (H3) summer drought strongly interferes with the acclimation of NUptakeE of beech to soil N availability and leads to shifts in the N:P stoichiometry and decreases in PNUE and NUE.
The present study uses the same provenances and experimental set-up as the previous work on the effect of global change on the composition of ECMF communities and the consequences for P uptake and use efficiencies of beech trees (Köhler et al., 2018). Briefly, the experiment was conducted with 2 year old European beech (Fagus sylvatica L.) saplings that originated from the 2012 tree masting of a mature beech forest in northwest Germany (N 52° 50′, E 10° 19′). The forest site was located on Pleistocene fluvioglacial sandy deposits with relatively low water storage capacity (79 mm 120 cm–1) at a mean annual precipitation of 816 mm and with a mean annual temperature of 8.7°C (for the period 1981–2010; climate data were provided by Deutscher Wetterdienst and were corrected for altitude; cf. Knutzen et al., 2017). At this forest site, adult beech trees showed on average a fungal colonization rate of 75% (Hertel et al., 2013) and had comparably species poor ECMF communities with, on average, nine ECMF species in adult trees (Zavišić et al., 2016) and three to six ECMF species in beech saplings (Leberecht et al., 2016). Excavated beech seedlings and their associated indigenous ECMF communities were cultivated in a sterilized 2:1 sand:peat mixture outdoors for one year in the Botanical Garden Göttingen, Central Germany. In May 2014, beech saplings were planted in 1.5–l pots filled with sieved and homogenized sandy mineral topsoil from the provenance, which contained the indigenous ECMF community. Thus, we aimed for maximizing the ECMF colonization rate of the beech saplings. Saplings of similar size (mean shoot height 20 cm, thirteen leaves) were arranged in a randomized array in a greenhouse and were kept well-watered.
In July 2014, we established a full factorial experiment with four factors (two temperature × three soil moisture × two N supply × three P supply levels; 36 treatments in total; n = eight replicates per treatment; see Supplementary Table S1). We simulated a gradient from current environmental conditions to the expected future global change conditions (elevated temperature and N deposition and reduced soil moisture and P availability): we established (i) ambient (T1; 18/12°C day/night) and elevated temperature conditions (T2; 22/16°C) in two separate climate chambers. Furthermore, we set-up (ii) three soil moisture levels an ambient, well-watered (M3; 90% field capacity (FC); 32% soil water content (SWC); v/v), a moderately moist (M2; 60% FC; 21% SWC), and a drought treatment (M1; 30% FC; 11% SWC). The field capacity of the sandy soil used in the experiment was determined according to Naeth et al. (1991). Water loss by evapotranspiration was replaced every second day by watering the pots to their target weight. We established (iii) three P availability levels, a high (P3; 0.69 mg P kg–1), a moderate (P2; 0.35 mg P kg–1), and a low P supply treatment (P1; 0.17 mg P kg–1), by adding biweekly NaH2PO4 as component of the Hoagland solution. Finally, we simulated (iv) ambient (N1; 3.9 mg N pot–1 growing season–1, representing 8.5 kg N ha–1 year–1) and elevated N deposition (N2; 7.8 mg N pot–1 growing season–1, representing 17 kg N ha–1 year–1), estimated on the basis of the current N deposition rates occurring in deciduous forests of Northern Germany (Schaap et al., 2018). N was added biweekly as NH4NO3 as component of the Hoagland solution. Our fertilization regime led to six N:P treatments: relative P availability decreased from N1P3 (0.69 mg P; N:P 5.6 g g–1) to N2P3 (0.69 mg P; N:P 11.3 g g–1), N1P2 (0.35 mg P; N:P 11.3 g g–1), N2P2 (0.35 mg P; N:P 22.6 g g–1), N1P1 (0.17 mg P; N:P 22.6 g g–1), and N2P1 (0.17 mg P; N:P 45.2 g g–1). Beech saplings were randomly assigned to these experimental treatments, positioned in a randomized design in two highly controllable walk-in climate chambers (York, Johnson Control Systems, Essen, Germany), and re-randomized biweekly for two growing seasons. During the non-growing season 2014/2015 (i.e., November 2014–March 2015) temperatures were reduced to 10/8°C day/night, the day length from 14 to 7.5 h, and photosynthetic photon flux density from 190 to 30 μmol photons m–2 s–1, while drought and fertilization treatments were paused. All conditions were brought back to the experimental conditions in April 2015 and maintained until the harvest of the experiment in September 2015. In total, we simulated two subsequent growing seasons with altered climatic and nutrient conditions for a total of 456 days.
At the end of June 2015, leaf gas exchange measurements were conducted for saplings grown at ambient and elevated temperature, well-watered and dry soil conditions, high and low P availability, and ambient and elevated N availability; each treatment combination being replicated five times (n = 80 saplings). Measurements were conducted on one canopy leaf per plant with an infrared CO2 analyzer (LI-6400; LI-COR Biosciences, Lincoln, NE, United States) during the midday hours. We measured the photosynthetic capacity (Amax; μmol CO2 m–2 s–1) at high photosynthetically active radiation (PAR: 1,500 μmol m–2 s–1; see Supplementary Table S2). Saturating light conditions were previously determined from light curves measured for a subset of plants. During the measurements, average leaf temperature was 18.2°C (T1) and 22.1°C (T2), relative humidity 61.3% (T1) and 56.2% (T2), vapor pressure deficit 9.6 hPa (T1) and 13.9 hPa (T2), flow rate 400.03 μmol s–1 (T1) and 400.1 μmol s–1 (T2), block temperature 15.0°C (T1) and 18.6°C (T2) and CO2 concentrations were ambient (390 μmol CO2 mol–1 air). We calculated total C assimilation per plant (in μmol C h–1) by multiplying Amax with total leaf area, which was determined from the product of specific leaf area (SLA in m2 kg–1; measured by using a flat-bed scanner and the computer program WinFOLIA 2014c; Régent Instruments Inc., Canada) and total dry biomass of green leaves. Photosynthetic N use efficiency (PNUE) was calculated according to PNUE = Amax∗SLA/NLeaves (in μmol CO2 g–1 N s–1), where NLeaves is the foliar N concentration (cf. Li et al., 2012).
All beech saplings were divided into senescent leaves, green leaves, shoots, coarse roots (diameter > 2 mm), and fine roots (diameter ≤ 2 mm) in mid-September 2015. For each sapling, we selected one representative, carefully washed, intact fine root section, transferred it to 5% glycerol solution, and kept it frozen at −18°C for subsequent analysis of the mycorrhizal colonization rate (in %; n = eight replicates per treatment). Fine root tips were counted (n = 85,327) and the ECMF morphotypes characterized (as per Agerer, 1987–2012). ECMF colonization rate was calculated as the percentage of the number of ECMF root tips in all vital root tips (see Supplementary Table S2). Root vitality was calculated as the percentage of vital root tips in all analyzed fine root tips (in %). Ten to twenty tips of each distinct morphotype were collected and used for molecular identification of fungal species (cf. Köhler et al., 2018). To describe ECMF diversity, we calculated the Shannon-Weaver index (Shannon and Weaver, 1949) from the relative proportion of an ECMF species in the total number of ECMF species per individual beech saplings (ECMF α diversity). Root length and root surface area were determined with WinRHIZO 2013e (Régent Instruments Inc., Canada; n = eight replicates per treatment). Subsequently, all leaves, stems, coarse roots, and fine roots were dried (48 h, 70°C) and weighed (see Supplementary Table S3). Specific root area (SRA, in cm2 g–1) and specific root length (SRL, in m g–1) were calculated.
All dried plant compartments were ground with a vibratory disc mill and were analyzed for total C and N content with an elemental analyzer (Vario EL III, elementar, Hanau, Germany). N uptake efficiency (NUptakeE; in g NDW g–1 Nadded) was calculated by dividing the N content in recent biomass, i.e., in fine roots, green and senescent leaves, by its amount added by fertilization during the 2015 growing season (n = eight replicates per treatment). N use efficiency (NUE, in g DW g–1 NDW) was calculated from the increment in total dry biomass for a given increase in biomass N (cf. Koide, 1991).
Statistical analyses were conducted with R 3.3.1 (R Development Core Team, 2017). Means and SE were calculated from eight replicates per treatment (Amax: n = 5). Residuals of the models were tested for normal distribution and homogeneity of variances by Shapiro-Wilk tests. The effects of N availability, P availability, temperature, soil moisture, and their interactions (fixed effects) on the response variables NUptakeE, PNUE, NUE, and plant nutrient concentrations (N concentration, C:N ratio and N:P ratio in leaves, fine roots and coarse roots) were analyzed by four-way ANOVAs type III using the car package (Fox and Weisberg, 2011). Tukey’s honest significant difference post hoc tests were conducted to identify significant differences between individual treatments. We calculated Pearson correlations for the relationships between NUptakeE, PNUE, or NUE with root vitality, ECMF colonization rate, ECMF species richness, ECMF α diversity, Amax, C assimilation, leaf and root morphology, and plant nutrient concentrations. For significant correlations, we conducted linear regression analyses and tested the significances of the slopes and intercepts as well as of the entire correlation models. The slopes of all significant correlation models were significant as well, while the intercepts were significant in most cases (exceptions: the intercept of the relationships between NUptakeE and fine root C:N ratio and between PNUE and coarse root C:N). For the relationship between NUptakeE and fine root biomass or fine root C:N ratio, pairwise comparisons of the slopes of N1 and N2 were performed using the “lstrends” function in the lsmeans R package (Lenth, 2016). We used SAS v 9.3 (SAS Institute Inc., Cary, NC, US) to conduct multiple regression analyses with backward variable elimination to test for significant independent predictors of NUptakeE, NUE, and PNUE. At each elimination step, the variable showing the smallest contribution to a model was deleted until all the variables remaining in the model produced significant F statistics even if their significant contribution was only small. Multicollinearity among variables was diagnosed: when (i) the significance of t-tests for individual slopes differed from the F-test of the model, (ii) pairs of predictor variables were highly correlated (r > 0.7), and (iii) collinearity diagnostics were critical (Belsley et al., 1980).
Despite the extended gradients in soil N availabilities (from 3.9 to 7.8 mg N kg–1) and soil N:P ratios (from 5.6 to 45.2 g g–1), N concentrations in beech leaves, fine roots, and coarse roots remained comparably constant across the range of soil nutrient conditions (see Supplementary Figures S1A,C,E). The average N concentration of leaves was 15 mg N g–1 and the average N concentration of fine roots 10 mg N g–1 at ambient climate conditions. With elevated temperature and reduced soil moisture, N concentrations increased to 19 mg N g–1 in leaves and to 15 mg N g–1 in fine roots. Leaf and fine root C concentrations were similar and not influenced by any of the experimental treatments (leaves and fine roots: 0.5 g C g–1). Due to the increase in N concentrations with elevated temperature and drought, fine root and foliar C:N ratios significantly decreased from ambient to climate change conditions (fine roots: from 48 to 33 g g–1; leaves: from 31 to 25 g g–1; see Supplementary Figures S1B,F). Similarly, drought also strongly increased coarse root N concentrations by 83% (from 6 to 11 mg N g–1; see Supplementary Figure S1C), which led to a decrease in the coarse root C:N ratio by about a half (see Supplementary Figure S1D).
NUptakeE related negatively to soil N availability (Figure 1A, see Table 2 and Supplementary Table S4). Elevated soil N availability reduced NUptakeE of beech saplings by almost half (from 9.5 to 4.9 g NDW g–1 Nadded). Elevated temperature slightly increased NUptakeE by 13% (from 6.8 to 7.7 g NDW g–1 Nadded), while soil drought reduced NUptakeE by 20% (from 7.7 to 6.2 g NDW g–1 Nadded). Soil P availability or the soil N:P ratio had no effect on NUptakeE.
Table 1. Three independent multiple regression analyses on the effects of multiple predictor plant traits on N uptake efficiency (NUptakeE), photosynthetic N use efficiency (PNUE), or N use efficiency (NUE).
Table 2. Four-factorial ANOVAs (type III SS) on the effects of N availability (N), P availability (P), temperature (T), soil moisture (M), and their interactions on the variance of N uptake efficiency (NUptakeE), photosynthetic N use efficiency (PNUE), and N use efficiency (NUE) of European beech saplings grown at increasing soil N:P ratios and climate change.
Figure 1. Means and SE of (A) N uptake efficiency (NUptakeE), (B), photosynthetic N use efficiency (PNUE), and (C) N use efficiency (NUE) of European beech saplings grown at increasing soil N:P ratios and ambient (dark bars; ambient temperature and soil moisture, AmbT + AmbM; treatment T1M3) or climate change conditions (light bars; elevated temperature and reduced soil moisture, ElevT + RedM; treatment T2M1) in climate chambers. The associated tables show the results of four-factorial ANOVAs and post hoc multiple comparisons according to Tukey for all experimental treatments (*P < 0.05, **P < 0.01, ***P < 0.001; ns, not significant; n = eight replicates per treatment for NUptakeE and NUE; n = five replicates per treatment for PNUE). Asterisks indicate significant differences between ambient and climate change conditions (*P < 0.05). N supply: N1, ambient; N2, elevated. P supply: P1, low; P2, moderate; P3, high. Temperature: T1, ambient; T2, elevated. Soil moisture: M1, drought; M2, moderately moist; M3, well-watered; see also Supplementary Table S1.
The main intrinsic influence on NUptakeE among the investigated influences had fine root and leaf biomass; that is, the larger the beech sapling and its fine root biomass the more efficient N uptake was (R2 = 0.42; Tables 1, 3). The relationship between fine root biomass and NUptakeE differed between soil N availabilities, though. The relationship had a greater intercept and slope at reduced soil N availability (difference significant at P < 0.001 between the slopes of N1 and N2; Figure 2A). In contrast to fine root biomass, fine root morphology had no effect on NUptakeE (see Table 3). A secondary intrinsic influence on NUptakeE was exerted by root C:N stoichiometry, where NUptakeE increased with the C:N ratio in fine roots and coarse roots (Figure 2B and Table 3), but with increased scatter of data points around the regression lines (R2 = 0.39–0.41) and non-significant intercepts. The relationship between NUptakeE and the fine root C:N ratio differed between soil N availability and had a greater intercept and slope at reduced soil N availability (Figure 2B). However, the slopes of N1 and N2 were only significantly different when single data points were compared (P = 0.004; not shown), and not in a comparison of treatment means (P = 0.21).
Table 3. Pearson’s correlations between N uptake efficiency (NUptakeE), photosynthetic N use efficiency (PNUE), and N use efficiency (NUE) with plant biomass, leaf and root morphology, ectomycorrhizal fungal (ECMF) community, photosynthetic capacity, nutrient concentrations, and nutrient ratios of European beech saplings grown at increasing soil N:P ratios and climate change.
Figure 2. Linear regressions between N uptake efficiency (NUptakeE) and (A) fine root biomass and (B) fine root C:N ratio of European beech saplings grown at increasing soil N:P ratios and climate change conditions (n = 36 treatments); (A) N1: NUptakeE = 4.1 + 2.50 × fine root biomass; N2: NUptakeE = 2.3 + 1.18 × fine root biomass; (B) N1: NUptakeE = 3.2 + 0.15 × fine root C:N ratio; N2: NUptakeE = 1.5 + 0.08 × fine root C:N ratio). All slopes are significant, as well as the intercepts for the relationships between NUptakeE and fine root biomass. The intercepts for the relationships between NUptakeE and fine root C:N ratio are not significant (N1: P = 0.13, N2: P = 0.16). Slopes of N1 and N2 were significantly different only for the relationship between NUptakeE and fine root biomass (P < 0.001), but not for the relationship between NUptakeE and fine root C:N ratio (P = 0.21). N supply: N1, ambient; N2, elevated.
Surprisingly, PNUE and NUE of the investigated beech saplings were not influenced by soil N availability, but by soil P availability and drought (Figures 1B,C and see Table 2). PNUE decreased by 45% with a reduction in P availability (from 5.5 to 3.8 μmol CO2 g–1 N s–1 from high to low P availability) and by 57% with soil drought (from 5.4 to 3.4 μmol CO2 g–1 N s–1 from the well-watered to the drought treatment when both temperature treatments were considered; Figure 1B and see Supplementary Table S5). Hence, there was a significant interaction of P availability and soil moisture on PNUE, which increased with increasing P availability and increasing soil moisture (Figure 1B). PNUE declined with decreasing coarse and fine root C:N ratios and increased with fine root N:P ratios (intercept for the relationship between PNUE and coarse root C:N not significant; Table 1, Figure 3D, see Table 3 and Supplementary Tables S4, S6), i.e., it was reduced with stoichiometric imbalance and relative deficiency of fine root P. Larger plants with greater and more vital root biomass had higher PNUE (see Table 3). Yet the low coefficient of determination in the multiple regression analysis (R2 = 0.25) also indicates additional non-predicted influences on PNUE which did not enter our regression model.
Figure 3. (A) Linear regressions between N use efficiency (NUE) and fine root C:N ratio, (B) between NUE and ECMF colonization rate, (C) between fine root C:N ratio and ECMF colonization rate, and (D) between photosynthetic N use efficiency (PNUE) and coarse root C:N ratio of European beech saplings grown at increasing soil N:P ratios and climate change conditions (n = 36 treatments; (A) NUE = -77.7 + 3.72 × fine root C:N ratio; (B) NUE = 41.5 + 0.63 × ECMF colonization rate; (C) Fine root C:N ratio = 32.5 + 0.16 × ECMF colonization rate; (D) PNUE = 0.8 + 0.06 × coarse root C:N ratio). Shown are the means of all 36 treatments (for PNUE only 16 treatments). All slopes are significant, as well as the intercepts for the relationships between NUE and fine root C:N ratio, between NUE and ECMF colonization rate, and between fine root C:N ratio and ECMF colonization rate. The intercept for the relationship between PNUE and coarse root C:N is not significant (P = 0.46).
Soil drought had a major impact on NUE (decrease by half from 102 to 53 g DW g–1 NDW from the well-watered to the drought treatment when both temperature treatments were considered; Figure 1C and see Supplementary Table S5). Further, NUE decreased with an increase in temperature (from 88 to 79 g DW g–1 NDW from ambient to elevated temperature) and at limiting soil P availability (from 91 to 78 g DW g–1 NDW from moderate to low P availability; see Supplementary Table S5). Next to these extrinsic influences, the C:N ratios in fine and coarse roots or the degree of relative N deficiency strongly increased NUE of saplings (Figure 3A and Tables 1, 3). The larger the leaf biomass of beech saplings was (and the higher the ECM colonization rate tended to be) the more efficient was their N use (Table 1 and Figure 3B). NUE was also significantly enhanced by increasing ECMF species richness and ECMF diversity (see Table 3). When all experimental treatments were considered, it appeared that ECMF colonization rate related negatively to N concentrations in leaves, coarse roots, and fine roots (see Supplementary Table S7), and positively to the corresponding C:N ratios (but with increased scatter of data points around the regression lines: R2 = 0.23; Figure 3C).
Elevated N deposition and summer droughts in the course of global change are assumed to affect nutrient stoichiometry (Vitousek et al., 2010; Peñuelas et al., 2013; Sardans et al., 2016), but it remains unclear to what degree plants counteract these changes by adjustments of the uptake efficiencies of individual nutrients. Here we demonstrate that European beech saplings respond to an increase in N availability by sufficient adjustments of NUptakeE (Figure 1A), with the consequence that tissue N concentrations and NUE remained comparably constant across the range of soil N availabilities and soil N:P ratios (Figure 1C and Supplementary Figure S1) but at the cost of a major tissue N:P stoichiometric change (Supplementary Table S6).
The capacity for N acquisition can be adjusted to N availability by changes in biomass partitioning, shifts in morphological or physiological traits, or by alterations at the cellular level (Kraiser et al., 2011) such as switching from low to high affinity nitrogen uptake (Gan et al., 2016). Previous fertilization experiments or N deposition studies showed that increases in N availability enhance total plant productivity, but they decrease fine root biomass partitioning (Nadelhoffer, 2000; Corrêa et al., 2011) and shift the ECMF community to nitrophilic taxa or even decrease ECMF colonization (Kjøller et al., 2012; de Witte et al., 2017). Yet in our fertilization study which simulated a naturally low to moderate N range, fine root biomass partitioning, ECMF colonization rate, and root morphology were unaffected by soil N availability (see also Köhler et al., 2018). A closer inspection of the regression between NUptakeE and fine root biomass illustrates that the relationship has similar coefficient of determination and is shifted to almost half the slope and to only a fraction of the intercept at elevated N availability. Theoretically, this bisection of the relationship can be caused by either a categorical shift in root architecture and functioning or by alterations in the membrane structure and transporter activity at elevated N availability. Plants can curtail NUptakeE by alterations in N uptake. Under high N availability, they exhibit a lower density and substrate affinity of nitrate transporters (Kraiser et al., 2011; Castro-Rodríguez et al., 2017). This shift in the relationship between NUptakeE and fine root biomass can stabilize tissue N at a given concentration optimal for plant functions and growth, as it was the case in the investigated European beech saplings.
The reduction of NUptakeE of beech by half when soil N availability was doubled reveals the negative relationship between NUptakeE and soil N availability. At the same time, the plant N:P ratio increased with increasing soil N:P, even though at a lower rate, which points at metastable tissue P concentrations and rising relative P limitation under elevated soil N:P. This rise in relative P limitation was attenuated in the investigated beech saplings by an increase in PUptakeE (Köhler et al., 2018), but not to the extent that no rise in tissue N:P occurred. Theoretically, plants may counteract an increase in tissue N:P ratio by both a (costly) increase in PUptakeE or a (cheaper) further restriction of NUptakeE when soil P is increasingly limiting. In our investigation the adjustment of NUptakeE was unresponsive to the availability of soil P and merely a function of N availability and fine root biomass. It seems that European beech saplings are only able to adjust their NUptakeE to the availability of N, but not to the availability of P. Biochemically dependent co-limitation (as defined by Saito et al., 2008) does not appear to play a role for N uptake.
In contrast to previous studies which focused on aboveground NUE in mature forests (e.g., Vitousek, 1982; Aerts and Chapin, 1999; Tateno et al., 2017), PNUE and whole-plant NUE of beech saplings were not influenced by soil N availability but were related to soil P availability instead (Figures 1B,C and Table 2). This points at stoichiometric constraints in PNUE and NUE, either from P or C limitation (Blanes et al., 2013). PNUE decreased with increasing P deficiency and decreasing Amax at high leaf N:P ratios. At a closer inspection it becomes clear that a response of PNUE to an increase in soil N availability occurs only after soil P availability increases (in the control climate; Figure 1B). This is an example of serially linked nutrient limitation as defined by Ågren et al. (2012), where the plant response to a second nutrient depends on the prior addition of another limiting resource. In the case of PNUE of beech, this response was synergistic and super-additive when both nutrients were added, but it was also dependent on the order of their addition. Serially linked nutrient limitation links to Liebig’s law of the minimum (Von Liebig, 1855) and indicates no strict co-limitation of PNUE by P and N. As a consequence of this serial limitation, PNUE of beech will probably not respond to N deposition as long as soil P continues to be increasingly limiting for plants (Talkner et al., 2015; Sardans et al., 2016; Hedwall et al., 2017).
Soil drought reduced PNUE by more than one third and had an even stronger effect at high soil N availability, which emphasizes the essential and overriding role of C limitation in decreasing PNUE. At the same time, foliar N increased in dry soil independent from soil N availability or the soil N:P ratio while the foliar C:N ratio decreased. Increased leaf N contents with drought have previously been observed in plants around the world (Sardans et al., 2017). Assimilation under drought can be optimized by increased leaf N contents to sustain the photosynthetic capacity (Farquhar et al., 2002). Increased leaf N contents with drought were associated with better stress adaptation from cellular membrane stability, lower osmotic potentials, reduced lipid peroxidation, and increased osmoprotectant (e.g., proline) content (Ashraf and Foolad, 2007). In our study, the increase in absolute and relative leaf N content under drought was not a consequence of greater availability and uptake of soil N. Rather, it was probably related to missing investment opportunities of N when drought is leading to reductions in stomatal opening width and consequently in photosynthetic C assimilation, i.e., to stronger drought effect on biomass production than on N to a stronger drought effect. More N is then stored in vacuoles and not used for growth, which leads to a decrease in the foliar C:N ratio. Similarly, the N concentration of absorptive foraging fine roots of mature beech trees increased during a severe summer drought while their C:N ratio decreased, which was probably consequence of a relatively high proportion of ephemeral roots with a high N concentration (Nikolova et al., 2020). Nevertheless, even if this increase in foliar N in our study may have counteracted to some extent the most severe drought stress, it was not sufficient to avoid any stomatal closing and decreases in photosynthetic capacity in drought-exposed beech saplings.
In dry soil, photosynthetic C assimilation of beech saplings was also limited by rising foliar N:P ratios and relative P limitation (Köhler et al., 2018). The factorial addition of P and soil moisture revealed a super-additive independent co-limitation (as defined by Harpole et al., 2011) by the two factors on PNUE, yet with a dominating effect exerted by soil drought and a subordinate effect by P deficiency (see Supplementary Figure S2). Previously, we have demonstrated that drought reduces the P uptake efficiency of beech from reductions in ECMF diversity and in the complementarity of P uptake by ECMF species (Köhler et al., 2018; see also Kreuzwieser and Gessler, 2010; Pena et al., 2010; Zavišić et al., 2016). This drought-induced P limitation probably also establishes the secondary role of P deficiency on PNUE. Limitations in plant P are reducing the ATP supply and thus the amount, regeneration, and specific activity of Rubisco (Reich et al., 2009; Yang et al., 2016), which decreases photosynthetic C assimilation and PNUE in addition to the direct stomatal control from soil drought. The observed significant decrease in PNUE in P-limited beech is in accordance with the results obtained from other tree species (Reich et al., 2009; Gan et al., 2016). Yet the dominating influence of soil moisture on PNUE will leave the response of European beech to P deficiency strongly dependent on future precipitation patterns.
NUE decreased with decreasing fine and coarse root C:N ratios and decreasing leaf biomass, i.e., when less photosynthates were available for beech growth. This decrease in NUE occurred at both high and low soil P availabilities (that is NUE is, when all experimental treatments are considered, significantly higher at P2 than at P1 and P3, as shown in the four-factorial ANOVA of Figure 1C) probably due to different reasons: the decrease in NUE with low soil P availability can be a consequence of a decrease in photosynthetic capacity and a reduction in PNUE from P limitation, as described above. The decrease in NUE of beech at high soil P can be explained by a reduction of photosynthetic P use efficiency when P is excessively supplied (cf. Lambers et al., 2012; Hidaka and Kitayama, 2013; Köhler et al., 2018). More P is then invested in non-photosynthetic membrane structures (Lambers et al., 2012) or accumulated in vacuoles (Elser et al., 2010; Gan et al., 2016) when surplus P cannot be used for growth. Previous studies found a decrease in NUE with increasing N availability in different deciduous tree species (e.g., Gan et al., 2016; Leberecht et al., 2016), but did not report on a decrease in NUE when the P supply was high. The observed close relationship of both PNUE and NUE with soil P availability in our study emphasizes the important role of the N:P stoichiometry for energy-dependent metabolic processes and, thus, beech NUE and growth.
The negative drought effect on the efficiencies for N uptake and N use intensified from NUptakeE (−20%) to PNUE (−37%) and NUE (−48%) (Supplementary Table S5); that is downstream its metabolic pathway from uptake to photosynthetic processing and biomass production in beech saplings. In addition, drought adjusted the different levels of PNUE and NUE to a similar, low level across the range of soil N:P ratios (Figure 1). At the same time, tissue N concentrations increased under drought (Supplementary Figure S1). The constant NUE with increasing tissue N in dry soil points at a counteracting effect by nutrient imbalances and P limitation (Supplementary Table S6), which are causing growth declines of beech saplings. In a comparison of the nutrient uptake efficiencies, drought had an even stronger effect on PUptakeE (as reported in Köhler et al., 2018) than on NUptakeE (cf. Dirks et al., 2019). Drought strongly decreased PUptakeE of beech saplings as a consequence of decreased ECMF colonization rate and the loss of ECMF with long-distance exploration. Soil exploration is more important in P uptake than in N uptake, since soil P occurs mostly in adsorbed, undissolved forms and diffuses very slowly (Lambers et al., 2008). Due to the loss in soil exploration by ECMF in dry soil, the negative drought effect was three times higher for PUptakeE than for NUptakeE and led to a shift in uptake stoichiometry. In our multiple regression analysis, ECMF colonization rate was identified as a secondary influence on beech NUE. This likely reflects the indirect role of hampered ECMF colonization rate on NUE through decreases in PUptakeE and increases in P deficiency, which decreases PNUE (as described above) and beech sapling growth from deteriorated N:P stoichiometry. As a consequence of these water limitations and nutrient imbalances, leaf and coarse root biomass and NUE strongly decreased while the root:shoot biomass ratio increased in beech saplings exposed to drought. It seems that soil drought has the potential to disturb the adjustment of plant stoichiometry to elevated N deposition.
We conclude that elevated N availability aggravates growth reductions from nutrient imbalances and summer droughts in European beech saplings. Notably, the direct negative effect of experimental drought on N uptake efficiency of European beech saplings intensifies downstream the metabolic pathway (that is from uptake to use) from additional limitations by P and C. Decreases in the ECMF colonization rate and ECMF diversity reduce P uptake efficiency in dry soil, leading to reductions in PNUE. Therefore, N use efficiency in dry soil strongly decreases from a combination of limited P uptake efficiency (and N uptake efficiency) and reduced photosynthetic C fixation leading to deteriorated nutrient stoichiometry and reduced beech sapling growth when exposed to experimental global change conditions. If such an effect also occurs in European beech saplings under natural conditions will have to be tested in subsequent field trials.
The original contributions presented in the study are included in the article/Supplementary Material, further inquiries can be directed to the corresponding author/s.
IM conceived of and designed the study and obtained the funding. JK and NY performed the experiments and collected the data. JK and IM analyzed and interpreted the data. IM, JK, AP, and RP wrote the manuscript. All authors approved the final version of the manuscript.
This work was supported by the Volkswagen Foundation [11-76251-99-34/13(ZN 2928) to IM], Natural Science Foundation of Jiangsu Province (BK20180767 to NY), and Deutsche Forschungsgemeinschaft (PE 2256/1-1 to RP).
The authors declare that the research was conducted in the absence of any commercial or financial relationships that could be construed as a potential conflict of interest.
We wish to thank I. Dirks, V. Raghavan, and J. Saetherø Steen for help with lab analyses, U. Nüsse-Hahne for help with ICP-OES analyses, and G. Kuchenbuch, R. Helbig, and K. Rapp for help with plant nurture.
The Supplementary Material for this article can be found online at: https://www.frontiersin.org/articles/10.3389/ffgc.2021.647360/full#supplementary-material
Aerts, R., and Chapin, F. S. III (1999). The mineral nutrition of wild plants revisited: A re-evaluation of processes and patterns. Adv. Ecol. Res. 30, 1–67. doi: 10.1016/S0065-2504(08)60016-1
Ågren, G. I., Wetterstedt, J. ÅM., and Billberger, M. F. K. (2012). Nutrient limitation on terrestrial plant growth – modeling the interaction between nitrogen and phosphorus. New Phytol. 194, 953–960. doi: 10.1111/J.1469-8137.2012.04116.X
Ashraf, M., and Foolad, M. R. (2007). Roles of glycine betaine and proline in improving plant abiotic stress resistance. Environ. Exp. Bot. 59, 206–216. doi: 10.1016/j.envexpbot.2005.12.006
Belsley, D. A., Koh, E., and Welsch, R. E. (1980). Regression diagnostics: Identifying influential data and sources of collinearity. New York: Wiley.
Blanes, M. C., Viñegla, B., Merino, J., and Carreira, J. A. (2013). Nutritional status of Abies pinsapo forests along a nitrogen deposition gradient: do C/N/P stoichiometric shifts modify photosynthetic nutrient use efficiency? Oecologia 171, 797–808. doi: 10.1007/s00442-012-2454-1
Burton, A. J., Pregitzer, K. S., Zogg, G. P., and Zak, D. R. (1998). Drought reduces root respiration in sugar maple forests. Ecol. Appl. 8, 771–778. doi: 10.1890/1051-07611998008[0771:DRRRIS]2.0.CO;2
Castro-Rodríguez, V., Cañas, R. A., de la Torre, F. N., Pascual, M. B., Avila, C., and Cánovas, F. M. (2017). Molecular fundamentals of nitrogen uptake and transport in trees. J. Exp. Bot. 68, 2489–2500. doi: 10.1093/jxb/erx037
Corrêa, A., Hampp, R., Magel, E., and Martins-Loução, M. A. (2011). Carbon allocation in ectomycorrhizal plants at limited and optimal N supply: An attempt at unraveling conflicting theories. Mycorrhiza 21, 35–51. doi: 10.1007/s00572-010-0309-3
de Witte, L. C., Rosenstock, N. P., van der Linde, S., and Braun, S. (2017). Nitrogen deposition changes ectomycorrhizal communities in Swiss beech forests. Sci. Total Environ. 605–606, 1083–1096. doi: 10.1016/j.scitotenv.2017.06.142
Dirks, I., Köhler, J., Rachmilevitch, S., and Meier, I. C. (2019). The phosphorus economy of Mediterranean oak saplings under global change. Front. Plant Sci. 10:405. doi: 10.3389/fpls.2019.00405
Elser, J. J., Fagan, W. F., Kerkhoff, A. J., Swenson, N. G., and Enquist, B. J. (2010). Biological stoichiometry of plant production: metabolism, scaling and ecological response to global change. New Phytol. 186, 593–608.
Farquhar, G. D., Buckley, T. N., and Miller, J. M. (2002). Optimal stomatal control in relation to leaf area and nitrogen content. Silva Fenn. 36, 625–637. doi: 10.14214/sf.530
Ferlian, O., Biere, A., Bonfante, P., Buscot, F., Eisenhauer, N., Fernandez, I., et al. (2018). Growing research networks on mycorrhizae for mutual benefits. Trends Plant Sci. 23, 975–984. doi: 10.1016/j.tplants.2018.08.008
Fox, J., and Weisberg, S. (2011). An R Companion to Applied Regression. california: Sage Publication.
Fujita, Y., Robroek, B. J. M., De Ruiter, P. C., Heil, G. W., and Wassen, M. J. (2010). Increased N affects P uptake of eight grassland species: the role of root surface phosphatase activity. Oikos 119, 1665–1673. doi: 10.1111/j.1600-0706.2010.18427.x
Galloway, J. N., Townsend, A. R., Erisman, J. W., Bekunda, M., Cai, Z., Freney, J. R., et al. (2008). Transformation of the nitrogen cycle: Recent trends, questions, and potential solutions. Science 320, 889–892. doi: 10.2307/20054730
Gan, H., Jiao, Y., Jia, J., Wang, X., Li, H., Shi, W., et al. (2016). Phosphorus and nitrogen physiology of two contrasting poplar genotypes when exposed to phosphorus and/or nitrogen starvation. Tree Physiol. 36, 22–38. doi: 10.1093/treephys/tpv093
Gessler, A., Schaub, M., and McDowell, N. G. (2017). The role of nutrients in drought-induced tree mortality and recovery. New Phytol. 214, 513–520. doi: 10.1111/nph.14340
Gobert, A., and Plassard, C. (2008). “The beneficial effect of mycorrhizae on N utilization by the host-plant: Myth or reality?,” in Mycorrhiza, ed. A. Varma, (Berlin: Springer), 209–240. doi: 10.1007/978-3-540-78826-3_11
Harpole, W. S., Ngai, J. T., Cleland, E. E., Seabloom, E. W., Borer, E. T., Bracken, M. E. S., et al. (2011). Nutrient co-limitation of primary producer communities. Ecol. Lett. 14, 852–862. doi: 10.1111/j.1461-0248.2011.01651.x
Hedwall, P.-O., Bergh, J., and Brunet, J. (2017). Phosphorus and nitrogen co-limitation of forest ground vegetation under elevated anthropogenic nitrogen deposition. Oecologia 185, 317–326. doi: 10.1007/s00442-017-3945-x
Hertel, D., Strecker, T., Müller-Haubold, H., and Leuschner, C. (2013). Fine root biomass and dynamics in beech forests across a precipitation gradient - Is optimal resource partitioning theory applicable to water-limited mature trees? J. Ecol. 101, 1183–1200. doi: 10.1111/1365-2745.12124
Hidaka, A., and Kitayama, K. (2013). Relationship between photosynthetic phosphorus-use efficiency and foliar phosphorus fractions in tropical tree species. Ecol. Evol. 3, 4872–4880. doi: 10.1002/ece3.861
Huang, X., Lakso, A. N., and Eissenstat, D. M. (2005). Interactive effects of soil temperature and moisture on Concord grape root respiration. J. Exp. Bot. 56, 2651–2660. doi: 10.1093/jxb/eri258
Kjøller, R., Nilsson, L. O., Hansen, K., Schmidt, I. K., Vesterdal, L., and Gundersen, P. (2012). Dramatic changes in ectomycorrhizal community composition, root tip abundance and mycelial production along a stand-scale nitrogen deposition gradient. New Phytol. 194, 278–286. doi: 10.1111/j.1469-8137.2011.04041.x
Knutzen, F., Dulamsuren, C., Meier, I. C., and Leuschner, C. (2017). Recent climate warming-related growth decline impairs European beech in the center of its distribution range. Ecosystems 20, 1494–1511. doi: 10.1007/s10021-017-0128-x
Köhler, J., Yang, N., Pena, R., Raghavan, V., Polle, A., and Meier, I. C. (2018). Ectomycorrhizal fungal diversity increases phosphorus uptake efficiency of European beech. New Phytol. 220, 1200–1210. doi: 10.1111/nph.15208
Koide, R. T. (1991). Nutrient supply, nutrient demand and plant response to mycorrhizal infection. New Phytol. 117, 365–386. doi: 10.1111/j.1469-8137.1991.tb00001.x
Korell, L., Auge, H., Chase, J. M., Harpole, S., and Knight, T. M. (2019). We need more realistic climate change experiments for understanding ecosystems of the future. Glob. Chang. Biol. 26:14797. doi: 10.1111/gcb.14797
Kraiser, T., Gras, D. E., Gutierrez, A. G., Gonzalez, B., and Gutierrez, R. A. (2011). A holistic view of nitrogen acquisition in plants. J. Exp. Bot. 62, 1455–1466. doi: 10.1093/jxb/erq425
Kreuzwieser, J., and Gessler, A. (2010). Global climate change and tree nutrition: influence of water availability. Tree Physiol. 30, 1221–1234. doi: 10.1093/treephys/tpq055
Lambers, H., Cawthray, G. R., Giavalisco, P., Kuo, J., Laliberté, E., Pearse, S. J., et al. (2012). Proteaceae from severely phosphorus-impoverished soils extensively replace phospholipids with galactolipids and sulfolipids during leaf development to achieve a high photosynthetic phosphorus-use-efficiency. New Phytol. 196, 1098–1108. doi: 10.1111/j.1469-8137.2012.04285.x
Lambers, H., Raven, J. A., Shaver, G. R., and Smith, S. E. (2008). Plant nutrient-acquisition strategies change with soil age. Trends Ecol. Evol. 23, 95–103. doi: 10.1016/j.tree.2007.10.008
LeBauer, D. S., and Treseder, K. K. (2008). Nitrogen limitation of net primary productivity in terrestrial ecosystems is globally distributed. Ecology 89, 371–379. doi: 10.1890/06-2057.1
Leberecht, M., Tu, J., and Polle, A. (2016). Acid and calcareous soils affect nitrogen nutrition and organic nitrogen uptake by beech seedlings (Fagus sylvatica L.) under drought, and their ectomycorrhizal community structure. Plant Soil 409, 143–157. doi: 10.1007/s11104-016-2956-4
Li, H., Li, M., Luo, J., Cao, X., Qu, L., Gai, Y., et al. (2012). N-fertilization has different effects on the growth, carbon and nitrogen physiology, and wood properties of slow- and fast-growing Populus species. J. Exp. Bot. 63, 6173–6185. doi: 10.1093/jxb/ers271
Liebig, J. (1855). Die Grundsätze der Agrikultur-Chemie mit Rücksicht auf die in England angestellten Untersuchungen. Braunschweig: Friedrich Vieweg und Sohn.
Meier, I. C., and Leuschner, C. (2008). Belowground drought response of European beech: Fine root biomass and carbon partitioning in 14 mature stands across a precipitation gradient. Glob. Chang. Biol. 14, 2081–2095. doi: 10.1111/j.1365-2486.2008.01634.x
Nadelhoffer, K. J. (2000). The potential effects of nitrogen deposition on fine-root production in forest ecosystems. New Phytol. 147, 131–139. doi: 10.1046/j.1469-8137.2000.00677.x
Naeth, M. A., Bailey, A. W., Chanasyk, D. S., and Pluth, D. J. (1991). Water holding capacity of litter and soil organic matter in mixed prairie and fescue grassland ecosystems of Alberta. J. Range Manag. 44:13. doi: 10.2307/4002630
Nikolova, P. S., Bauerle, T. L., Häberle, K. H., Blaschke, H., Brunner, I., and Matyssek, R. (2020). Fine-root traits reveal contrasting ecological strategies in European beech and Norway spruce during extreme drought. Front. Plant Sci. 11:1–18. doi: 10.3389/fpls.2020.01211
Pena, R., and Polle, A. (2014). Attributing functions to ectomycorrhizal fungal identities in assemblages for nitrogen acquisition under stress. ISME J. 8, 321–330. doi: 10.1038/ismej.2013.158
Pena, R., Offermann, C., Simon, J., Naumann, P. S., Gessler, A., Holst, J., et al. (2010). Girdling affects ectomycorrhizal fungal (EMF) diversity and reveals functional differences in EMF community composition in a beech forest. Appl. Environ. Microbiol. 76, 1831–1841. doi: 10.1128/AEM.01703-09
Peñuelas, J., Poulter, B., Sardans, J., Ciais, P., van der Velde, M., Bopp, L., et al. (2013). Human-induced nitrogen–phosphorus imbalances alter natural and managed ecosystems across the globe. Nat. Commun. 4:2934. doi: 10.1038/ncomms3934
R Development Core Team, (2017). R: A language and environment for statistical computing. Vienna: R foundation for statistical computing.
Raven, J. A., Lambers, H., Smith, S. E., and Westoby, M. (2018). Costs of acquiring phosphorus by vascular land plants: patterns and implications for plant coexistence. New Phytol. 217, 1420–1427. doi: 10.1111/nph.14967
Reich, P. B., Oleksyn, J., and Wright, I. J. (2009). Leaf phosphorus influences the photosynthesis–nitrogen relation: a cross-biome analysis of 314 species. Oecologia 160, 207–212. doi: 10.1007/s00442-009-1291-3
Rothstein, D. E., Zak, D. R., Pregitzer, K. S., and Curtis, P. S. (2000). Kinetics of nitrogen uptake by Populus tremuloides in relation to atmospheric CO2 and soil nitrogen availability. Tree Physiol. 20, 265–270. doi: 10.1093/treephys/20.4.265
Ruehr, N. K., Offermann, C. A., Gessler, A., Winkler, J. B., Ferrio, J. P., Buchmann, N., et al. (2009). Drought effects on allocation of recent carbon: from beech leaves to soil CO2 efflux. New Phytol. 184, 950–961. doi: 10.1111/j.1469-8137.2009.03044.x
Saito, M. A., Goepfert, T. J., and Ritt, J. T. (2008). Some thoughts on the concept of colimitation: Three definitions and the importance of bioavailability. Limnol. Oceanogr. 53, 276–290. doi: 10.4319/lo.2008.53.1.0276
Sardans, J., Alonso, R., Janssens, I. A., Carnicer, J., Vereseglou, S., Rillig, M. C., et al. (2016). Foliar and soil concentrations and stoichiometry of nitrogen and phosphorous across European Pinus sylvestris forests: Relationships with climate, N deposition and tree growth. Funct. Ecol. 30, 676–689. doi: 10.1111/1365-2435.12541
Sardans, J., Grau, O., Chen, H. Y. H., Janssens, I. A., Ciais, P., Piao, S., et al. (2017). Changes in nutrient concentrations of leaves and roots in response to global change factors. Glob. Chang. Biol. 23, 3849–3856. doi: 10.1111/gcb.13721
Schaap, M., Banzaf, S., Hendriks, C., Kranenburg, R., Kuenen, J., Nagel, H.-D., et al. (2018). PINETI-3: Modellierung atmosphärischer Stoffeinträge von 2000 bis 2015 zur Bewertung der ökosystem-spezifischen Gefährdung von Biodiversität durch Luftschadstoffe in Deutschland. Dessau-Roßlau: Umweltbundesamt.
Shannon, C. E., and Weaver, W. (1949). The mathematical theory of communication. Illinois: The University of Illinois Press.
Talkner, U., Meiwes, K. J., Potoèiæ, N., Seletkoviæ, I., Cools, N., De Vos, B., et al. (2015). Phosphorus nutrition of beech (Fagus sylvatica L.) is decreasing in Europe. Ann. For. Sci. 72, 919–928. doi: 10.1007/s13595-015-0459-8
Tateno, R., Taniguchi, T., Zhang, J., Shi, W. Y., Zhang, J. G., Du, S., et al. (2017). Net primary production, nitrogen cycling, biomass allocation, and resource use efficiency along a topographical soil water and nitrogen gradient in a semi-arid forest near an arid boundary. Plant Soil 420, 209–222. doi: 10.1007/s11104-017-3390-y
Vitousek, P. (1982). Nutrient Cycling and Nutrient Use Efficiency. Am. Nat. 119, 553–572. doi: 10.1086/283931
Vitousek, P. M., Porder, S., Houlton, B. Z., Chadwick, O. A., and Houlton, Z. (2010). Terrestrial phosphorus limitation: mechanisms, implications, and nitrogen — phosphorus interactions. Ecol. Appl. 20, 5–15. doi: 10.1890/08-0127.1
Yang, N., Zavišić, A., Pena, R., and Polle, A. (2016). Phenology, photosynthesis, and phosphorus in European beech (Fagus sylvatica L.) in two forest soils with contrasting P contents. J. Plant Nutr. Soil Sci. 179, 151–158. doi: 10.1002/jpln.201500539
Zavišić, A., Nassal, P., Yang, N., Heuck, C., Spohn, M., Marhan, S., et al. (2016). Phosphorus availabilities in beech (Fagus sylvatica L.) forests impose habitat filtering on ectomycorrhizal communities and impact tree nutrition. Soil Biol. Biochem. 98, 127–137. doi: 10.1016/j.soilbio.2016.04.006
Keywords: drought, Fagus sylvatica, nitrogen deposition, phosphorus limitation, uptake efficiency, use efficiency
Citation: Köhler J, Yang N, Pena R, Polle A and Meier IC (2021) Drought Deteriorates the N Stoichiometry of Biomass Production in European Beech Saplings Under Global Change. Front. For. Glob. Change 4:647360. doi: 10.3389/ffgc.2021.647360
Received: 29 December 2020; Accepted: 24 March 2021;
Published: 21 April 2021.
Edited by:
Kouki Hikosaka, Tohoku University, JapanReviewed by:
Petia Simeonova Nikolova, Snow and Landscape Research (WSL), SwitzerlandCopyright © 2021 Köhler, Yang, Pena, Polle and Meier. This is an open-access article distributed under the terms of the Creative Commons Attribution License (CC BY). The use, distribution or reproduction in other forums is permitted, provided the original author(s) and the copyright owner(s) are credited and that the original publication in this journal is cited, in accordance with accepted academic practice. No use, distribution or reproduction is permitted which does not comply with these terms.
*Correspondence: Julia Köhler, anVsaWEua29laGxlckBiaW9sb2dpZS51bmktZ29ldHRpbmdlbi5kZQ==
†Present address: Nan Yang, College of Biology and the Environment, Nanjing Forestry University, Nanjing, China; Rodica Pena, Department of Sustainable Land Management, School of Agriculture, Policy and Development, University of Reading, Reading, United Kingdom; Ina C. Meier, Functional Forest Ecology, Department of Biology, University of Hamburg, Hamburg, Germany
Disclaimer: All claims expressed in this article are solely those of the authors and do not necessarily represent those of their affiliated organizations, or those of the publisher, the editors and the reviewers. Any product that may be evaluated in this article or claim that may be made by its manufacturer is not guaranteed or endorsed by the publisher.
Research integrity at Frontiers
Learn more about the work of our research integrity team to safeguard the quality of each article we publish.