- 1Departamento de Ciências Florestais, Escola Superior de Agricultura “Luiz de Queiroz,” Universidade de São Paulo, Piracicaba, Brazil
- 2Departamento de Ingeniería Forestal y Medio Ambiente, Facultad de Ingeniería, Universidad Nacional Amazónica de Madre de Dios, Puerto Maldonado, Peru
- 3Carrera Profesional de Ingeniería Forestal y Ambiental, Universidad Nacional de Jaén, Jaén, Peru
- 4Departamento de Ecologia, Instituto de Biociências, Universidade de São Paulo, São Paulo, Brazil
Context: Gold mining is the most destructive activity in the natural forests of the Madre de Dios region in the southeastern Peruvian Amazon. Understanding the natural regeneration process of these degraded areas is necessary to develop forest restoration projects in such conditions.
Aims: We aimed to evaluate forest recovery and identify the successional and structure patterns of vegetation governing natural regeneration over time.
Methods: Structure, composition, richness, diversity, and successional status were evaluated in abandoned artisanal gold mine areas in Madre de Dios, southeastern Peru. Vegetation data were recorded in 61 plots of 250 m2 established in five sites varying from 1 to 19 years of abandonment. Vegetation in abandoned areas was compared with six undisturbed forests evaluated in previous inventories.
Results: In the mining lands, tree density and basal area recovered quickly, while species richness and composition were slow. Forest recovery is an initial stage of transition from pioneer to early secondary species until at least 19 years after abandonment. The most abundant and frequent species were the fast-growing species Ochroma pyramidale and Cecropia engleriana. These species could be considered potential candidates to promote restoration plans. Pioneer species represented 63% of the number of species in plots of 1–4 years, 57% in plots of 5–7 years, and 50% in plots of 8–19 years. Early and late secondary species represented 34 and 16%, respectively, of the number of species in plots of 8–19 years. Abandoned mining and reference plots present less than 5% of species in common.
Conclusion: Our results highlight a slow natural regeneration process in areas for up to 19 years after gold mining. Species from different successional statuses were identified as potential candidates for recovering vegetation in such areas. Our findings may have important implications for further research focusing on the ecological restoration in tropical forests severely degraded by gold mining.
Introduction
The southeastern region of Peru comprises one of the largest areas of preserved Amazon forest encompassing almost 30% of the total protected areas of the country (SERNANP, 2019). This region is recognized worldwide as part of the Tropical Andes Biodiversity Hotspot due to its high species diversity (Rodrigues, 2013). However, artisanal gold mining in the region is the principal driver of deforestation (Joshi et al., 2015). In the Madre de Dios region, gold mining-related deforestation was approximately 1,000 km2 between 1984 and 2017 (Swenson et al., 2011; Asner et al., 2013; Caballero Espejo et al., 2018), and it was responsible for the highest annual deforestation rate since 2011, 80 km2 year–1 on average (Caballero Espejo et al., 2018). Forest loss appears as extensive wastelands with superficial soil layers removed, large mounds of sand, and artificial bodies of water (Mosquera et al., 2009; Kalamandeen et al., 2020). Long-lasting impacts also include mercury-polluted air, soil, and water (Scullion et al., 2014) that severely limits forest recovery (Mosquera et al., 2009).
Forest recovery strongly depends on the initial degree of degradation (Chazdon, 2008). In tropical forests, results from chronosequences analyzed in abandoned sites impacted by agricultural and pasture activities showed that regenerating forests can attain many of the structural characteristics and woody species richness comparable with old-growth forests within a few decades (Guariguata and Ostertag, 2001). In contrast, in gold mining areas, the recovery is slower and more complex and may not even occur because the impacts not only alter the vegetation but also soil characteristics (Peterson and Heemskerk, 2001; Lamb et al., 2005; Ren et al., 2007; Kalamandeen et al., 2020).
Old-growth or secondary forests surrounding the degraded areas function as important seed sources that facilitate forest recovery following mining (Rodrigues et al., 2004). Their spatial distribution as well as their proximity to degraded areas usually defines the composition and structure of natural regeneration (Pereira et al., 2013; de Rezende et al., 2015; Sloan et al., 2016). Nevertheless, management strategies are needed to promote forest recovery in highly degraded areas. Active restoration accelerates forest recovery in less resilient areas (Stanturf, 2005; Chazdon, 2008; Rodrigues et al., 2009).
In the Peruvian Amazon, most of the areas degraded by gold mining are surrounded by old-growth forests (Joshi et al., 2015), suggesting favorable landscape-scale conditions for natural regeneration (Chazdon and Guariguata, 2016). Although variation in secondary forest structure and composition following agriculture and pasture abandonment has been widely studied (Chazdon et al., 2007; Feldpausch et al., 2007; Norden et al., 2015), quantifying the structural and compositional parameters and the time of recovery in abandoned gold mining areas remains poorly known. Considering the urgent need to restore landscapes across the tropics, it is important to understand how vegetation recovery in such lands occurs over time.
In this study, we aimed to evaluate vegetation recovery to help develop restoration strategies of tropical forests under gold mining impacts. We analyzed (i) the relationship between vegetation structure status with the age of abandonment and (ii) the species richness, diversity, and successional patterns as a function of site age; and (iii) compared the floristic composition between abandoned and reference forests evaluated in previous inventories. We also sought to identify pioneer and secondary forest species to enrich or accelerate natural regeneration in abandoned gold mining areas.
Materials and Methods
Study Areas
The study was conducted along the mining corridor of Madre de Dios Department, southeastern Peruvian Amazon (Figure 1). The corridor extends along the Madre de Dios River and Interoceanic Highway (PE-30). The forest is classified as seasonal tropical moist (Román-Dañobeytia et al., 2015; Dueñas and Garate, 2018). Mean annual rainfall is 2,300 mm and mean annual temperature is 27°C (SENAMHI, 2008).
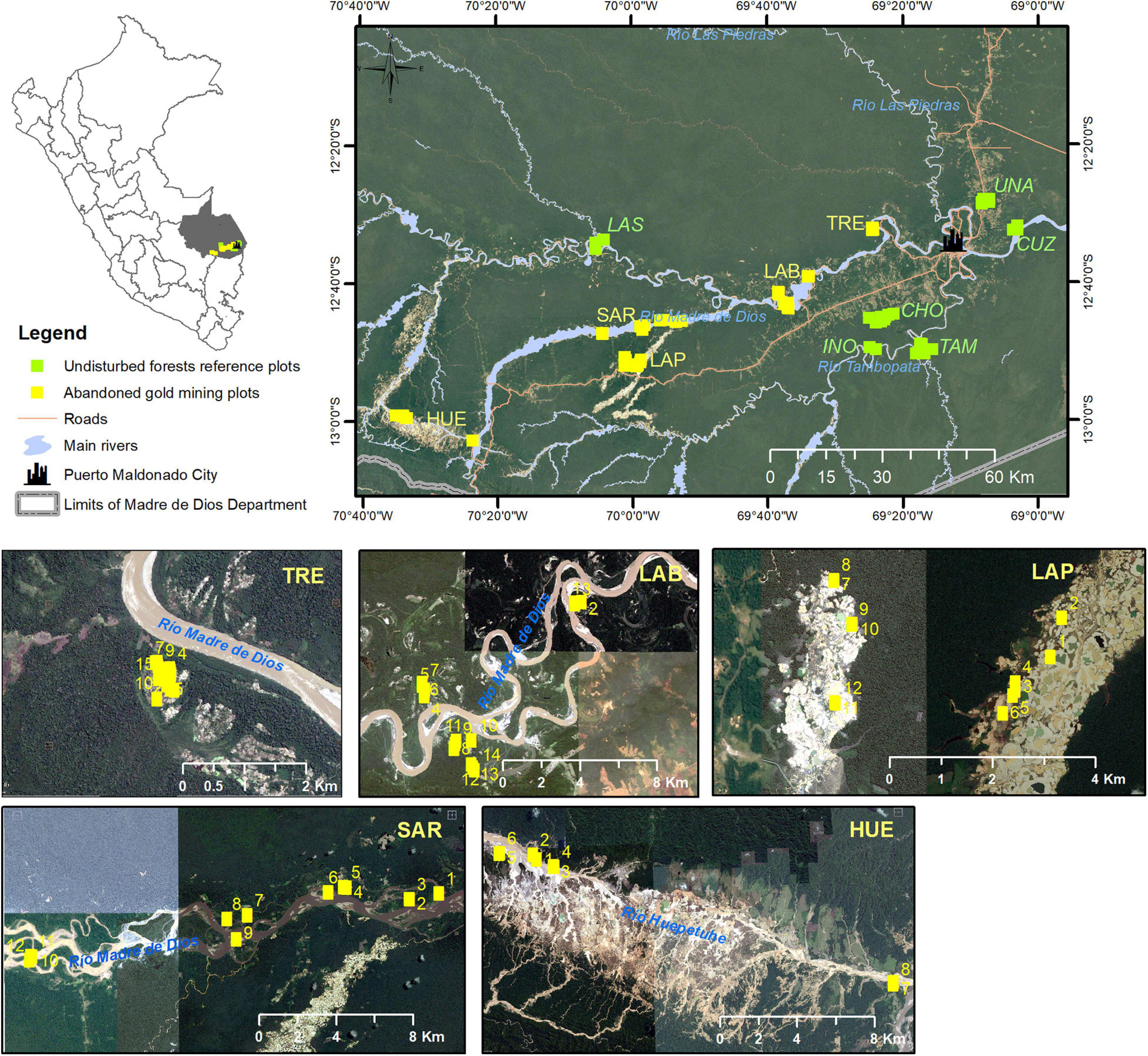
Figure 1. Location of the study region including the abandoned gold mining plots (TRE, Tres Islas; LAB, Laberinto; SAR, Sarayacu; LAP, La Pampa; HUE, Huepetuhe) and undisturbed forest reference plots (UNA, Forest of the Universidad Nacional Amazonica de Madre de Dios; INO, Inotawa; CHO, Chonta; TAM, Tambopata plot zero; LAS, Jacaralia Los Amigos; CUZ, Cuzco Amazonico). The numbers within the images indicate the plot number.
Most of the artisanal gold miners are informal and/or illegal because they either lack the proper permits to operate or work outside authorized mining concessions (Scullion et al., 2014; Daley, 2016; EJAtlas, 2018). Miners primarily exploit riverbanks and primary forestlands (Damonte et al., 2013). Widespread tree mortality is observed in the abandoned mining areas, possibly due to sediment mobilization and anoxic or desiccating conditions and perhaps because of heavy metal contamination (Asner et al., 2013).
Five abandoned sites were located: “Tres Islas,” “Laberinto,” “La Pampa,” “Sarayacu,” and “Huepetuhe” of 220, 900, 2,000, 1,200, and 2,200 ha in area, respectively (Figure 1). Reference forests included the Universidad Nacional Amazonica de Madre de Dios (UNA) (Escalante, 2017; Carhuarupay, 2018), Inotawa (INO) (Mamani, 2012; Báez, 2014), Chonta (author’s database), and three plot metadata collected by Forest Plots (ForestPlots, 2014): Tambopata plot zero (TAM), Jacaralia Los Amigos (LAS), and Cuzco Amazonico (CUZ).
Data Collection
Abandoned Mining Sites
Sixty-one plots of 250 m2 (10 × 25 m) were randomly established in five mining sites (between 8 and 15 plots per site). The plot ages were determined by interviews and confirmed by examining satellite images between 1990 and 2016. In each plot, three size classes of woody species (all trees and shrubs) were recorded, identified, and measured, and botanical material was collected: small trees (10 cm diameter-at-breast-height, dbh), saplings (5–10 cm dbh), and seedlings (≥ 1 m height and < 5 cm dbh). The identification and classification was performed by botanists at the Alwyn Gentry Herbarium (Universidad Nacional Amazonica de Madre de Dios) and the San Marcos Herbarium (Universidad Nacional Mayor de San Marcos). The classification was based on the Angiosperm Phylogeny Group (Chase et al., 2016), and the scientific names were updated and standardized based on the data from Missouri Botanical Garden1.
Reference Forest
Table 1 shows the number and characteristics of plots in reference sites. In each plot, all trees ≥ 10 cm dbh were identified at the species level, and their height and dbh were measured.
The species of abandoned mining and reference plots were also classified by their successional status (pioneer, early secondary, late secondary, or climax) based on a literature review of ecological studies in the Amazon rainforests (e.g., Gandolfi et al., 1995; Guariguata and Ostertag, 2001; Pitman et al., 2001; Gama et al., 2002; Souza et al., 2002; Da Silva et al., 2003; da Santos et al., 2004; do Amaral et al., 2009; Orrego and Zevallos, 2014; Dueñas and Garate, 2018).
Data Analysis
We classified plots into three groups by age of abandonment: 1–4, 5–7, and 8–19 years, with 22, 19, and 20 plots, respectively, based on a principal component analysis of mean diameter and height of seedlings, saplings, and small trees and the number of seedlings, saplings, small trees, pioneer, early secondary, and late secondary species by plot (Supplementary Figure 1). Three plots of La Pampa (two of 1 year and one of 2 years) did not have any individuals.
Linear regression analysis, plotting the age of site abandonment against species richness, basal area, and density of plants, for each successional status, was used to describe the pace of forest regeneration. Data correspond to 61 plot observations including pioneer, early secondary, and late secondary species. Analyses were performed in SAS Software, Version 9.4 (SAS Institute Inc, 2013).
Rank-abundance plots (Daly et al., 2018) were used to graphically depict the abundance distributions of species in each age group after mining, for each reference forest. For these analyses, log 10 data transformation was performed. The relative abundance of species (%) by successional status was calculated. Shannon diversity index (H′) (Magurran, 1988) and the effective number of species (ENS) calculated as ENS = exp(H′) (Jost, 2006; Daly et al., 2018) were determined to compare plant communities among age groups and reference forests.
Rarefied species richness curves (Chazdon et al., 1998) were constructed to compare expected species for each age group after mining and reference old-growth forests. Ninety-five percent confidence intervals derived from 100 permutations were also calculated. Rarefaction curves are robust to estimate species richness, and they are recommended for unbalanced sampling design (Magurran, 1988).
The Importance Value Index (IVI) was also calculated (Lamprecht, 1990). The ranking of the highest 10 species according to their IVI was graphed for each site. Analyses were performed in Past 3.2.1 (Ryan et al., 2001) and Origin 2018 (OriginLab Corporation, 2018).
Results
Forest Community Overview
We recorded 3,129 stems from 71 species of woody plants. Three plots (1 and 2 years since abandonment) from La Pampa did not have any individuals. In addition, 35 species were classified as pioneers, 23 as early secondary, and 13 as late secondary. Seventy percent of individuals corresponded to seedlings (61 species), 20% to saplings (43 species), and 10% to small trees (21 species).
In the reference plots, we recorded a total of 11,067 stems from 948 species of trees. Ninety-eight species were classified as pioneers, 314 as early secondary, 475 as late secondary, and 61 as climax.
Forest Structure, Species Richness, Diversity, and Successional Status Over Time
Total stem density was 2,155, 2,200, and 1,798 ind. ha–1 for 1–4, 5–7, and 8–19 years after mining, respectively. Pioneers had the highest stem density in all age groups, representing 96, 89, and 69% of total stem density in plots of 1–4, 5–7, and 8–19 years post-abandonment, respectively. The relative density of early and late secondary species increased with abandonment age, from 4.3% of total density in recently abandoned mines to 31% in the oldest plots. Small trees increased from 4 to 17% in plots of 1–4 and 8–19 years post-abandonment, respectively.
The pioneers Ochroma pyramidale and Cecropia engleriana showed the highest stem density across all ages. The stem density of O. pyramidale decreased with site age, from 1,235 ind. ha–1 (1–4 years) and 998 ind. ha–1 (5–7 years) to 328 ind. ha–1 (8–19 years). The plots of 5–7 years presented the highest density of C. engleriana, 364 ind. ha–1, while plots of 8–19 years presented the lowest, 132 ind. ha–1.
Reference plots presented an average stem density of 551 ind. ha–1 only for trees and small trees (≥10 cm dbh). The species with the highest density were Iriartea deltoidea, Pseudolmedia laevis, and Euterpe precatoria.
Stem density, basal area, and species richness increased positively and significantly with increasing age of abandonment, and this pattern was consistent with the successional status. The exceptions were the density of pioneer and late secondary that did not vary by increasing the abandonment age (Figure 2).
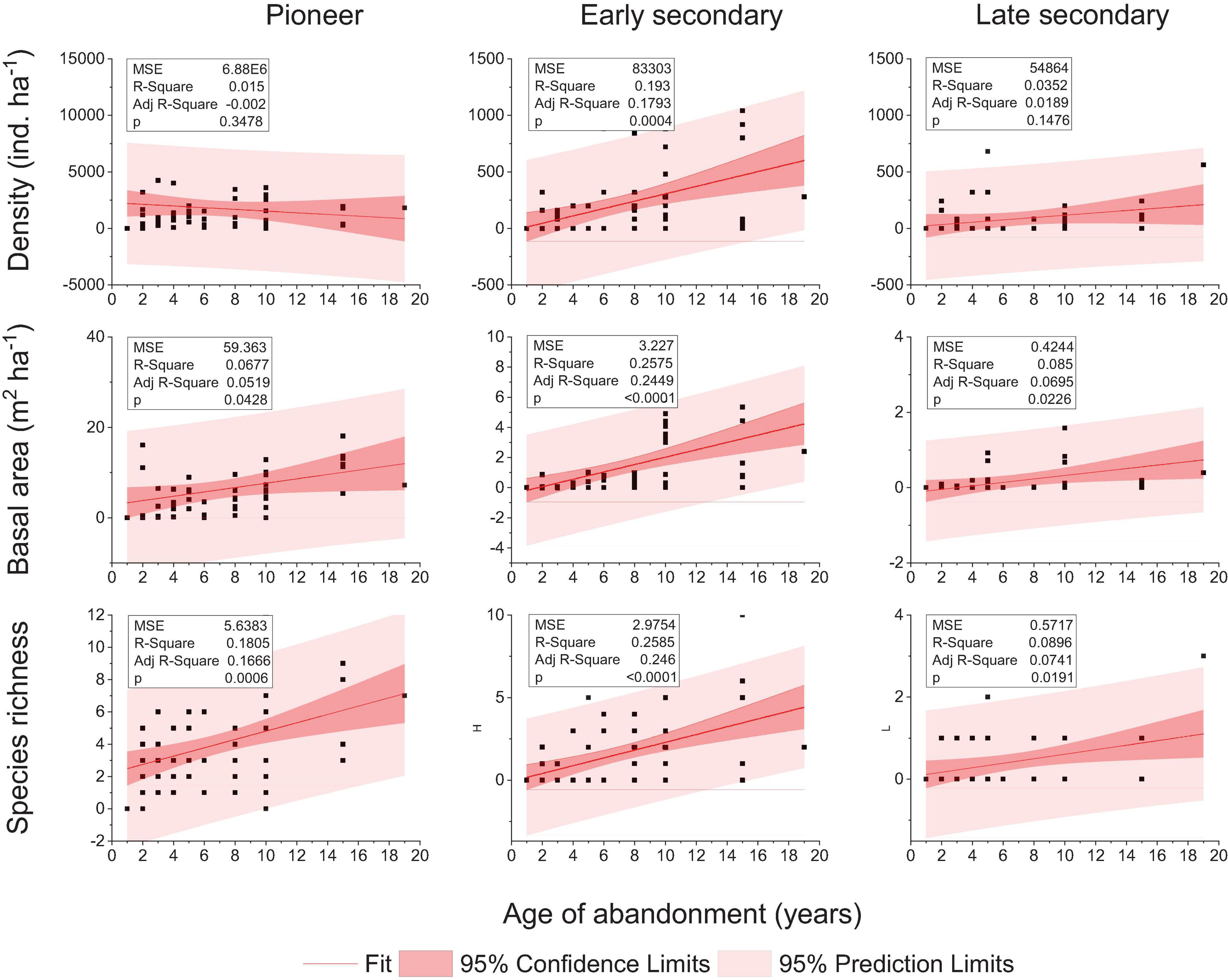
Figure 2. Relationships observed between the age of abandoned mining plots and density, basal area, and species richness for each successional status. Data correspond to 61 plot observations including pioneer, early secondary, and late secondary species. Linear regressions and correlation statistics are also shown.
Steep curves for small trees, saplings, and seedlings at 1–4 years in the dominance–diversity curves graph indicate communities with high dominance of very few species in recently abandoned areas. Similar patterns were observed for saplings and small trees at 5–7 years (Supplementary Figure 2A). On the other hand, curves for seedlings at 5–7 and 8–19 years show a relative uniformity in the distribution of individuals among the species of the community. These results indicate a more diverse species assemblage for seedlings and saplings.
Rarefied species richness (30 individuals) ranged from 8 to 10 species in abandonment plots (Supplementary Figure 2C) and 23–25 species in reference plots (Supplementary Figure 2D). The ENS and H′ show that the diversity in all size classes increased positively with abandonment age, although diversity is lower compared with the reference plots (Table 2).
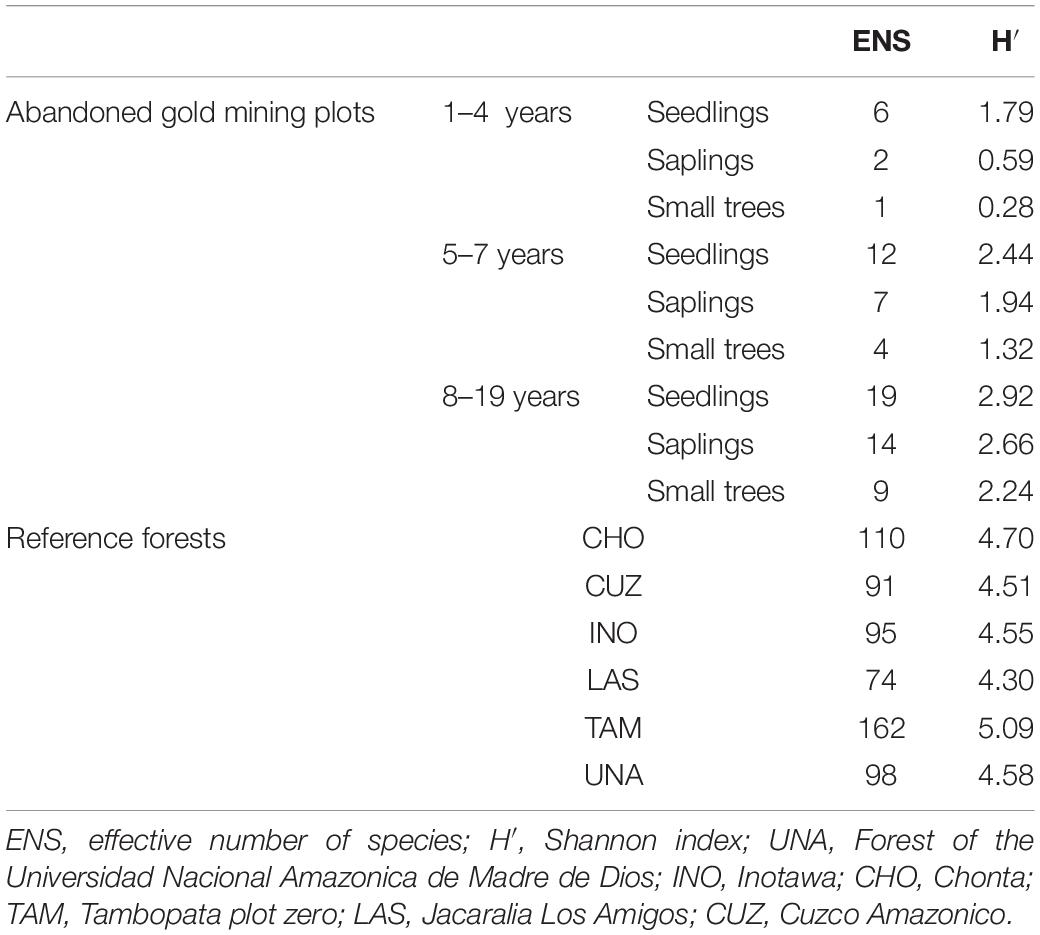
Table 2. Richness and diversity for the abandoned gold mining and reference forests plots in Madre de Dios, southeastern Peru.
The plant community across abandoned plots was dominated by pioneer species followed by early secondary species. Late secondary species were slightly proportionally higher in plots of 8–19 years of abandonment compared with the other plot ages (Figure 3A). The plant community in reference plots was dominated by early and late secondary species and a small set of pioneer and climax species (Figure 3B).
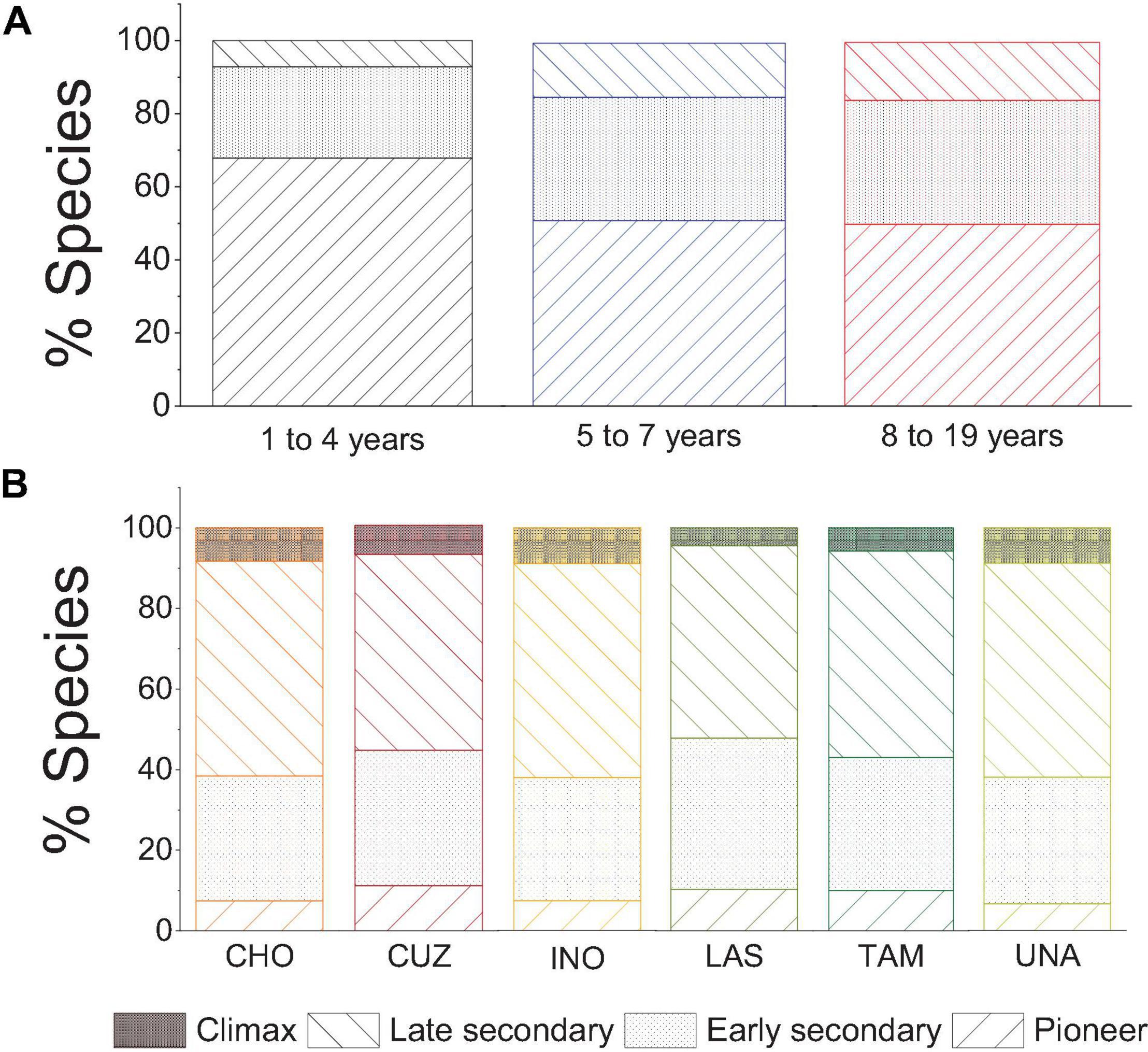
Figure 3. Percentage of species by successional status in abandoned mining plots (A) and reference plots (B). UNA, Forest of the Universidad Nacional Amazonica de Madre de Dios; INO, Inotawa; CHO, Chonta; TAM, Tambopata plot zero; LAS, Jacaralia Los Amigos; CUZ, Cuzco Amazonico.
Forest Composition
The IVI shows that O. pyramidale and C. engleriana are the dominant species across all abandoned plots, ages, and size classes (Supplementary Figure 3A). Furthermore, other pioneer species such as Piper sp. (seedlings), Schizolobium parahyba (saplings), Guazuma crinita, and Senna silvestris (small trees) presented high IVI in plots of 8–19 years of abandonment. Inga thibaudiana was the only non-pioneer species ranked among the 10 highest IVI in areas 5–7 and 8–19 years of abandonment.
In reference plots, the IVI shows that I. deltoidea, E. precatoria, P. laevis, and Bertholletia excelsa are the most important species in almost all areas. Forty-five, 40, 10, and 2% of the species in the reference plots corresponded to late secondary, early secondary, climax, and pioneer, respectively (Supplementary Figure 3B).
Shared Taxa
Plots younger than 7 years post-abandonment shared 29% of similarity (Supplementary Figure 3). This pattern was observed in both La Pampa and Huepetuhe plots. The oldest sites, Laberinto and Tres Islas, shared 35% of the species. Abandoned mining and reference plots presented less than 5% of species in common. Ficus maxima, Jacaranda copaia, S. parahyba, Terminalia oblonga, Trema micrantha, G. crinita, O. pyramidale, Cecropia sp., Inga sp., Piper sp., and Triplaris sp. were the main species observed in both abandoned and reference plots. Some of them were recorded in the oldest abandoned mining plots (Laberinto and Tres Islas) as seedlings and saplings.
Discussion
Forest Recovery Following Gold Mining
Considering the concerns about the recovery of vegetation in abandoned gold mining lands, we show that structural and compositional parameters presented different patterns of recovery, likely reflecting the biotic and abiotic local variations in the sites and historical land-use changes. Our results point out faster recovery of forest structure than recovery of species composition and species diversity. This is a relevant finding since in some cases the recovery of forest structure following gold mining in Amazonian forests is close to zero or extremely slow, as in Suriname (Peterson and Heemskerk, 2001) or Guyana (Kalamandeen et al., 2020).
The density and basal area of early secondary species increased with age since abandonment. Contrary to expected, the stem density of pioneers and late secondary species did not vary with plot age. Considering that basal area represents a conservative estimate of the total biomass (Gilman et al., 2016), this has accumulated rapidly in most plots and showed a positive increase as the plot age increased. These results highlight the value of naturally regenerating sites for promoting the provision of ecosystem services related to carbon storage and sequestration (Rozendaal et al., 2019). Other studies argue that carbon storage from regenerating forests may not reach levels of old-growth forests until many decades later (Denslow and Guzman, 2000; Mascaro et al., 2012).
Although species richness increased positively with abandonment age, it represented only 9–19% of that reported in reference forests. This result has been also described in other studies across the Amazon Basin (e.g., Lozano Baez, 2013; Valois-Cuesta and Martínez-Ruiz, 2017; Kalamandeen et al., 2020). Changes promoted by mining activities are even more intense than other human-induced land conversions such as agriculture and pastures (Fujisaka et al., 2000; Guariguata and Ostertag, 2001).
In the oldest abandoned plots, pioneer species had four times the stem density and almost twice the richness relative to early secondary species. These values were even higher when compared with late secondary species. Despite the dominance of the pioneers, the richness, basal area, and stem density of the early and late secondary species increased over time. Consistent with previous studies (Peterson and Heemskerk, 2001; Rozendaal et al., 2019), these floristic and structural changes indicate a transition from pioneer to early secondary species at least until 19 years after abandonment. The dominance of early–late secondary species can take a few more decades (Rodrigues et al., 2004; Van Breugel et al., 2013), whereas the progression of secondary into old-growth forest can take centuries (Peterson and Heemskerk, 2001; Sharma and Chaudhry, 2018; Rozendaal et al., 2019).
Factors Influencing Forest Recovery Patterns
The high abundance of pioneer species can be related to their seed dispersal strategies (Rozendaal et al., 2019) and their fast growth (Kalamandeen et al., 2020), while the low abundance of early and late secondary species could be due to soil removal (e.g., tailing ponds and mining pits) (Peterson and Heemskerk, 2001; Román-Dañobeytia et al., 2015; Kalamandeen et al., 2020).
However, we observed improvements in some soil characteristics over time (% sand and nutrients) (Supplementary Table 1), although the soil quality remained below that of reference forests. Despite the severe impacts on soil conditions, the complexity of the vegetation increased slightly with the abandonment age. The surrounding old-growth forests can probably function as a continuous source of seeds and contribute to increasing diversity over time (Fujisaka et al., 2000; Rodrigues et al., 2004).
Forest Composition in Abandoned Gold Mining Areas
Plant composition in almost all abandoned plots and plant size classes show that the dominant species are the fast-growing O. pyramidale and C. engleriana. Similarly, abandoned fallows in central-eastern Peru are dominated by T. micrantha and Cecropia membranacea (Fujisaka et al., 2000). In many other degraded lands across the Amazon basin, the presence of Cecropia spp. is common in natural regeneration but O. pyramidale (Mesquita, 2000; Mesquita et al., 2001; Rodrigues et al., 2004; Kalamandeen et al., 2020) is not, even though O. pyramidale is naturally distributed in these areas (ITTO, 2019).
The composition of the surrounding forests plays an important factor in driving the floristic composition of the plots. O. pyramidale and Cecropia spp. are abundant in riverbanks in the Madre de Dios region, and G. crinita, S. parahyba, and Calycophyllum spruceanum are common species in secondary forests and even in primary forests of this region (Gentry, 1982; Phillips and Gentry, 1993; Reynel et al., 2003; Dueñas and Garate, 2018).
Implications to Forest Restoration
The slow pace of natural regeneration observed after 19 years of mining abandonment suggests the need for active restoration strategies with soil reclamation efforts to accelerate forest recovery. The combination of strategies can assist the restocking of transitional succession species speeding up the succession process (Evans et al., 2013; Román-Dañobeytia et al., 2015; Orozco-Aceves et al., 2017).
In the southeastern Peruvian Amazon, however, considering that active restoration with soil reclamation costs 2,100–3,500 US$ ha–1 during the first year of activity (Román-Dañobeytia et al., 2015) and that the areas degraded by gold mining sum up to 80,000 ha, only during 2007–2017 (Caballero Espejo et al., 2018), this strategy imposes financial challenges. Therefore, it is necessary to evaluate the relative effectiveness of different restoration strategies, ranging from passive (e.g., natural regeneration) to more intensive ones (e.g., mixed plantations of native species with soil reclamation). In older sites, natural regeneration followed by enrichment with late-successional species could further accelerate the successional process.
Despite not formally testing the tolerance of specific species to soil conditions, we observed that several tree taxa were found continuously at high densities despite the extensive soil disturbance. O. pyramidale and C. engleriana colonized and dominated areas 19 years after abandonment, which could have created a favorable structure for the establishment of early secondary species in older areas, providing shade and contributing to organic matter (Mesquita et al., 2001). Both could be key species in facilitating forest succession in recently abandoned mining lands. G. crinita, S. parahyba, and C. spruceanum, pioneer species co-dominating the oldest areas, could also be considered as potential species to be used in restoration strategies, as they have timber value (Reynel et al., 2003). Tree plantations using timber species have been recognized as a cost-effective alternative to convince landowners to recover or catalyze successional processes in degraded lands (Lamb et al., 2005; Rodrigues et al., 2009). I. thibaudiana, a non-pioneer species found in abandoned 5-year-old plots (Supplementary Figure 3A), commonly grows under broad environmental conditions (Schierenbeck et al., 1997), and similar to other Inga spp., it recovers and enriches soils through nitrogen fixation (do Vale et al., 2014; UICN, 2019).
Conclusion
After gold mining in Madre de Dios, forests regenerating demonstrated a high potential for biomass recovery throughout the regeneration process and a high conservation value in degraded landscapes. Stimulating their natural regeneration and testing some restoration strategies (e.g., soil reclamation, species enrichment) to accelerate forest recovery in such areas are fundamental to complement the biodiversity conservation provided by surrounding reference forests.
Data Availability Statement
The datasets used and/or analyzed during the current study are available from the corresponding author on reasonable request.
Author Contributions
RC-L, DOR, FF, JPV, PZP JM-P, and DR conceived the research idea. RC-L, JPV, and PZP collected data. RC-L, DOR, and DR performed statistical analyses and wrote the manuscript, with contributions from FF, JPV, PZP, and JM-P. JM-P and PZP made the determination of successional status of all plant species of the areas. All authors discussed the results, commented, and approved the manuscript.
Funding
Funding for the field work was provided by the Madre de Dios Consortium-USAID and IIAP (Instituto de Investigaciones de la Amazonía Peruana).
Conflict of Interest
The authors declare that the research was conducted in the absence of any commercial or financial relationships that could be construed as a potential conflict of interest.
Acknowledgments
We gratefully acknowledge Laura Cutire, Laura Ramirez, Javier F. Valles, and Erika Sajami for the field work. We also thank the staff of Alwyn Gentry Herbarium of Universidad Nacional Amazónica de Madre de Dios and of San Marcos Herbarium of Universidad Nacional Mayor de San Marcos for their technical support in the taxonomic identification. We also thank FONDECYT-CONCYTEC (Fondo Nacional de Desarrollo Científico, Tecnológico y de Innovación Tecnológica, Perú, award #239-2018) for the fellowship grant to RC-L; CAPES (Coordenação de Aperfeiçoamento de Pessoal de Nível Superior do Governo do Brasil, Finance Code 001 and #88882.305844/2018-01), FAPESP (Fundação de Amparo à Pesquisa do Estado de São Paulo, grant #2012/24118-8), and The Royal Society for the fellowship granted to DR; and also FAPESP (grant #2018/22914-8) for the fellowship grant to DOR.
Supplementary Material
The Supplementary Material for this article can be found online at: https://www.frontiersin.org/articles/10.3389/ffgc.2021.594627/full#supplementary-material
Footnotes
References
Asner, G. P., Llactayo, W., Tupayachi, R., and Ráez Luna, E. (2013). Elevated rates of gold mining in the Amazon revealed through high-resolution monitoring. Proc. Nat. Acad. Sci. U S A. 110, 18454–18459. doi: 10.1073/pnas.1318271110
Báez, S. M. Q. (2014). Evaluación Dendrológica de Especies Forestales en un Bosque de Tierra firme en la Concesión de Conservación Gallocunca, Sector Baltimore, Distrito Tambopata, Provincia Tambopata – Departamento Madre de Dios. Tesis de Pregrado, Universidad Nacional Amazónica de Madre de Dios. Available online at: http://repositorio.unamad.edu.pe/handle/UNAMAD/101
Caballero Espejo, J., Messinger, M., Román-Dañobeytia, F., Ascorra, C., Fernandez, L. E., and Silman, M. (2018). Deforestation and forest degradation due to gold mining in the Peruvian Amazon: a 34-year perspective. Remote Sens. 10, 1–17. doi: 10.3390/rs10121903
Carhuarupay, S. L. (2018). Estructura, Diversidad y Composición Florística Arbórea de un Bosque de Terraza Alta del Sector Loboyoc, Distrito de Las Piedras, provincia de Tambopata, Madre de Dios. Tesis de Pregrado, Universidad Nacional Amazónica de Madre de Dios. Available online at: http://repositorio.unamad.edu.pe/handle/UNAMAD/334
Chase, M. W., Christenhusz, M. J. M., Fay, M. F., Byng, J. W., Judd, W. S., Soltis, D. E., et al. (2016). An update of the Angiosperm Phylogeny Group classification for the orders and families of flowering plants: APG IV. Bot. J. Linn. Soc. 181, 1–20. doi: 10.1111/boj.12385
Chazdon, R. L. (2008). Beyond deforestation: restoring forests and ecosystem services on degraded lands. Science 320, 1458–1460. doi: 10.1126/science.1155365
Chazdon, R. L., Colwell, R. K., Denslow, J. S., and Guariguata, M. R. (1998). “Statistical methods for estimating species richness of woody regeneration in primary and secondary rainforests of northeastern costa rica,” in Forest Biodiversity Research, Monitoring and Modeling: Conceptual Background and Old World Case Studies, eds F. Dallmeier and J. A. Comiskey (Paris: Parthenon Publishing Group), 285–309.
Chazdon, R. L., and Guariguata, M. R. (2016). Natural regeneration as a tool for large-scale forest restoration in the tropics: prospects and challenges. Biotropica 48, 716–730. doi: 10.1111/btp.12381
Chazdon, R. L., Letcher, S. G., Van Breugel, M., Martínez-Ramos, M., Bongers, F., and Finegan, B. (2007). Rates of change in tree communities of secondary neotropical forests following major disturbances. Philos. Trans. R. Soc. B Biol. Sci. 362, 273–289. doi: 10.1098/rstb.2006.1990
Cutire, L., and Ramirez, L. (2017). Caracterización Ecológica De Bosques Secundarios Regenerados En Áreas Degradadas Producto De La Actividad Aurífera En La Comunidad De Tres Islas. Madre De Dios, Perú. Tesis de Pregrado, Universidad Nacional Amazónica de Madre de Dios. Available online at: http://repositorio.unamad.edu.pe/handle/UNAMAD/347
da Santos, J. H. S., Ferreira, R. L. C., Da Silva, J. A. A., De Souza, A. L., Santos, E. D. S., and Meunier, I. M. J. (2004). Distinção de grupos ecológicos de espécies florestais por meio de técnicas multivariadas. Rev. Árvore 28, 387–396. doi: 10.1590/S0100-67622004000300010
Da Silva, A. F., Oliveira, R. V., De, Santos, N. R. L., and De Paula, A. (2003). Composição florística e grupos ecológicos das espécies de um trecho de floresta semidecídua submontana da Fazenda São Geraldo, Viçosa-MG. Rev. Árvore 27, 311–319. doi: 10.1590/S0100-67622003000300006
Daley, S. (2016). Peru Scrambles to Drive Out Illegal Gold Mining and Save Precious Land. New York, NY: New York Times.
Daly, A., Baetens, J., and De Baets, B. (2018). Ecological diversity: measuring the unmeasurable. Mathematics 6:119. doi: 10.3390/math6070119
Damonte, R., de Bueno, M. M., Pachas, V. H., Chávez Quijada, M., Flores, A., and De Echave, J. C. (2013). “Small-scale gold mining and social and environmental conflict en the Peruvian Amazon,” in Small-Scale Gold Mining in the Amazon. The cases of Bolivia, Brazil, Colombia, Peru and Suriname, eds L. Cremers, J. Kolen, and M. de Theije (Amsterdam: CEDLA), 68–84.
de Rezende, C. L., Uezu, A., Scarano, F. R., and Araujo, D. S. D. (2015). Atlantic Forest spontaneous regeneration at landscape scale. Biodivers. Conserv. 24, 2255–2272. doi: 10.1007/s10531-015-0980-y
Denslow, J. S., and Guzman, G. S. (2000). Variation in stand structure, light and seedling abundance across a tropical moist forest chronosequence. Panama. J. Veg. Sci. 11, 201–212. doi: 10.2307/3236800
do Amaral, D. D., Vieira, I. C. G., de Almeida, S. S., de Salomão, R. P., da Silva, A. S. L., and Jardim, M. A. G. (2009). Checklist da flora arbórea de remanescentes florestais da região metropolitana de Belém e valor histórico dos fragmentos. Pará, Brasil. Bol. do Mus. Para. Emílio Goeldi Ciências Nat. 4, 231–289.
do Vale, I., Costa, L. G. S., Miranda, I. S., do Vale, I., Costa, L. G. S., and Miranda, I. S. (2014). Indicated species to restoration of riparian forests in subwatershed of Peixe-Boi river, Pará State. Ciência Florest. 24, 573–582. doi: 10.1590/1980-509820142403006
Dueñas, H., and Garate, J. (2018). Diversidad, dominancia y distribución arbórea en Madre de Dios. Perú. Rev. For. del Perú 33, 4–23. doi: 10.21704/rfp.v33i1.1152
EJAtlas (2018). Illegal Gold Mining in La Pampa and Tambopata National Reserve, Peru. Available online at: https://ejatlas.org/conflict/illegal-mining-in-la-pampa-tambopata-peru (Accessed July 20, 2020).
Escalante, A. V. (2017). Riqueza, Dominancia, Diversidad Y Composición Florística de Árboles en la Localidad de Loboyoc, Tambopata- Madre de Dios. Tesis de Pregrado. Universidad Nacional Amazónica de Madre de Dios. Available online at: http://repositorio.unamad.edu.pe/handle/UNAMAD/417
Evans, D. M., Zipper, C. E., Burger, J. A., Strahm, B. D., and Villamagna, A. M. (2013). Reforestation practice for enhancement of ecosystem services on a compacted surface mine: path toward ecosystem recovery. Ecol. Eng. 51, 16–23. doi: 10.1016/j.ecoleng.2012.12.065
Feldpausch, T. R., da Conceicao, Prates-Clark, C., Fernandes, E. C. M., and Riha, S. J. (2007). Secondary forest growth deviation from chronosequence predictions in central Amazonia. Glob. Chang. Biol. 13, 967–979. doi: 10.1111/j.1365-2486.2007.01344.x
ForestPlots (2014). ForestPlots.Net: Helping To Measure, Monitor, And Analyse The World’s Tropical Forests. Available online at: http://www.forestplots.net/ (Accessed July 21, 2020)
Fujisaka, S., Escobar, G., and Veneklaas, E. J. (2000). Weedy fields and forests: interactions between land use and the composition of plant communities in the Peruvian Amazon. Agric. Ecosyst. Environ. 78, 175–186. doi: 10.1016/S0167-8809(99)00122-X
Gama, J. R. V., Botelho, S. A., and Bentes-Gama, M. D. M. (2002). Composição florística e estrutura da regeneração natural de floresta secundária de várzea baixa no estuário amazônico. Rev. Árvore 26, 559–566. doi: 10.1590/S0100-67622002000500005
Gandolfi, S., Leitão Filho, H. F., and Bezerra, C. L. F. (1995). Levantamento florístico e caráter sucessional das espécies arbustivo-arbóreas de uma floresta mesófila semidecídua no município de Guarulhos. SP. Rev. Bras. Biol. 55, 753–767.
Gentry, A. H. (1982). Neotropical floristic diversity: phytogeographical connections between central and south america, pleistocene climatic fluctuations, or an accident of the andean orogeny? Ann. Missouri Bot. Gard. 69:557. doi: 10.2307/2399084
Gilman, A. C., Letcher, S. G., Fincher, R. M., Perez, A. I., Madell, T. W., Finkelstein, A. L., et al. (2016). Recovery of floristic diversity and basal area in natural forest regeneration and planted plots in a Costa Rican wet forest. Biotropica 48, 798–808. doi: 10.1111/btp.12361
Guariguata, M. R., and Ostertag, R. (2001). Neotropical secondary forest succession: changes in structural and functional characteristics. For. Ecol. Manage 148, 185–206. doi: 10.1016/s0378-1127(00)00535-1
Joshi, N., Mitchard, E. T., Woo, N., Torres, J., Moll-Rocek, J., Ehammer, A., et al. (2015). Mapping dynamics of deforestation and forest degradation in tropical forests using radar satellite data. Environ. Res. Lett. 10:34014. doi: 10.1088/1748-9326/10/3/034014
Kalamandeen, M., Gloor, E., Johnson, I., Agard, S., Katow, M., Vanbrooke, A., et al. (2020). Limited biomass recovery from gold mining in Amazonian forests. J. Appl. Ecol. 57, 1730–1740. doi: 10.1111/1365-2664.13669
Lamb, D., Erskine, P. D., and Parrotta, J. A. (2005). Restoration of degraded tropical forest landscapes. Science 310, 1628–1632. doi: 10.1126/science.1111773
Lamprecht, H. (1990). Silvicultura en los Trópicos: Los Ecosistemas Forestales en los Bosques Tropicales y sus Especies Arbóreas; Posibilidades y Métodos Para un Aprovechamiento Sostenido. GmbH Eschborn: TZ-Verlag-Ges.
Lopez-Gonzalez, G., Lewis, S. L., Burkitt, M., and Phillips, O. L. (2011). ForestPlots.net: a web application and research tool to manage and analyse tropical forest plot data. J. Veg. Sci. 22, 610–613. doi: 10.1111/j.1654-1103.2011.01312.x
Lozano Baez, S. E. (2013). Restauración de la Cobertura Vegetal en Áreas Previamente Afectadas por la Minería Aluvial de oro en el Nordeste de Antioquia, Colombia. Colombia: Pontificia Universidad Javeriana.
Magurran, A. E. (1988). Ecological Diversity and Its Measurement. Dordrecht: Springer. doi: 10.1007/978-94-015-7358-7350
Mamani, J. L. (2012). Stock del Carbono Aéreo en un Bosque con Paca de Terrazas Altas de la Concesión de Conservación Gallocunca, Sector Baltimore, Distrito Tambopata Madre de Dios - Perú. Tesis de Pregrado. Universidad Nacional Amazónica de Madre de Dios. Available online at: http://repositorio.unamad.edu.pe/handle/UNAMAD/91
Mascaro, J., Asner, G. P., Dent, D. H., DeWalt, S. J., and Denslow, J. S. (2012). Scale-dependence of aboveground carbon accumulation in secondary forests of Panama: a test of the intermediate peak hypothesis. For. Ecol. Manage. 276, 62–70. doi: 10.1016/j.foreco.2012.03.032
Mesquita, R. D. C. G. (2000). Management of advanced regeneration in secondary forests of the Brazilian Amazon. For. Ecol. Manage. 130, 131–140. doi: 10.1016/S0378-1127(99)00174-177
Mesquita, R. D. C. G., Ickes, K., Ganade, G., and Williamson, G. B. (2001). Alternative succesional pathways in the amazon basin. J. Ecol. 89, 528–537. doi: 10.1046/j.1365-2745.2001.00583.x
Mosquera, C., Chávez, M. C., Pachas, V. H., and Moschella, P. (2009). Estudio Diagnóstico de la Actividad Minera Artesanal en Madre de Dios. Perú: Tarea Gráfica Educativa.
Norden, N., Angarita, H. A., Bongers, F., Martínez-Ramos, M., Cerda, I. G. D., La, et al. (2015). Successional dynamics in Neotropical forests are as uncertain as they are predictable. Proc. Natl. Acad. Sci. U S A. 112, 8013–8018. doi: 10.1073/pnas.1500403112
Orozco-Aceves, M., Tibbett, M., and Standish, R. J. (2017). Correlation between soil development and native plant growth in forest restoration after surface mining. Ecol. Eng. 106, 209–218. doi: 10.1016/j.ecoleng.2017.06.004
Orrego, J. A. R., and Zevallos, P. (2014). Estudio de la diversidad florística estructural de un bosque de terraza alta de la provincia de Tambopata, Madre de Dios, Perú. Biodivers. Amaz. 4, 34–45.
Pereira, L. C., Oliveira, C., and Torezan, J. (2013). Woody species regeneration in atlantic forest restoration sites depends on surrounding landscape. Brazilian J. Nat. Conserv. Res. Lett. Nat. Conserv. 11, 138–144. doi: 10.4322/natcon.2013.022
Peterson, G. D., and Heemskerk, M. (2001). Deforestation and forest regeneration following small-scale gold mining in the Amazon: the case of Suriname. Environ. Conserv. 28, 117–126. doi: 10.1017/S0376892901000121
Phillips, O., and Gentry, A. H. (1993). The useful plants of tambopata, Peru: i. statistical hypotheses tests with a new quantitative technique. Econ. Bot. 47, 15–32. doi: 10.1007/BF02862203
Pitman, N. C. A., Terborgh, J. W., Silman, M. R., Núñez, P. V., Neill, D. A., Cerón, C. E., et al. (2001). Dominance and distribution of tree species in upper Amazonian terra firme forests. Ecology 82, 2101–2117. doi: 10.1890/0012-9658(2001)082[2101:dadots]2.0.co;2
Ren, H., Li, Z., Shen, W., Yu, Z., Peng, S., Liao, C., et al. (2007). Changes in biodiversity and ecosystem function during the restoration of a tropical forest in south China. Sci. China Ser. C Life Sci. 50, 277–284. doi: 10.1007/s11427-007-0028-y
Reynel, C., Pennington, T. D, Pennington, R. T., Flores, C., and Daza, A. (2003). Arboles útiles de la Amazonía Peruana: un Manual con Apuntes de Identificación, Ecología y Propagación de las Especies. Perú: Tarea Gráfica Educativa, 509.
Rodrigues, A. S. L. (2013). “Hotspots,” in Encyclopedia of Biodiversity, 2nd Edn, ed. S. A. Levin (Amsterdam: Elsevier), 127–136. doi: 10.1016/B978-0-12-384719-5.00410-X
Rodrigues, R. R., Lima, R. A. F., Gandolfi, S., and Nave, A. G. (2009). On the restoration of high diversity forests: 30 years of experience in the Brazilian Atlantic Forest. Biol. Conserv. 142, 1242–1251. doi: 10.1016/J.BIOCON.2008.12.008
Rodrigues, R. R., Martins, S. V., and De Barros, L. C. (2004). Tropical Rain Forest regeneration in an area degraded by mining in Mato Grosso State. Brazil. For. Ecol. Manage. 190, 323–333. doi: 10.1016/j.foreco.2003.10.023
Román-Dañobeytia, F., Huayllani, M., Michi, A., Ibarra, F., Loayza-Muro, R., Vázquez, T., et al. (2015). Reforestation with four native tree species after abandoned gold mining in the Peruvian Amazon. Ecol. Eng. 85, 39–46. doi: 10.1016/j.ecoleng.2015.09.075
Rozendaal, D. M. A., Bongers, F., Aide, T. M., Alvarez-Dávila, E., Ascarrunz, N., Balvanera, P., et al. (2019). Biodiversity recovery of neotropical secondary forests. Sci. Adv. 5:eaau3114. doi: 10.1126/sciadv.aau3114
Ryan, P. D., Hammer, Ø, Harper, D. A., and Paul Ryan, D. D. (2001). PAST: paleontological statistic software package for education and data analysis. Palaeontol. Electron 4, 1–9.
Sajami, E. Q. (2017). Evaluación de la Regeneración Natural en Áreas Degradadas por la Minería Aurífera en el Distrito de Laberinto, Tambopata-Madre de Dios. Universidad Nacional Amazónica de Madre de Dios. Available online at: http://repositorio.unamad.edu.pe/handle/UNAMAD/246
SAS Institute Inc., (2013). SAS/ACCESS® 9.4 Interface to ADABAS: Reference. Cary, NC: SAS Institute Inc.
Schierenbeck, K. A., Skupski, M., Lieberman, D., and Lieberman, M. (1997). Population structure and genetic diversity in four tropical tree species in Costa Rica. Mol. Ecol. 6, 137–144. doi: 10.1046/j.1365-294X.1997.00166.x
Scullion, J. J., Vogt, K. A., Sienkiewicz, A., Gmur, S. J., and Trujillo, C. (2014). Assessing the influence of land-cover change and conflicting land-use authorizations on ecosystem conversion on the forest frontier of Madre de Dios, Peru. Biol. Conserv. 171, 247–258. doi: 10.1016/j.biocon.2014.01.036
Sharma, V., and Chaudhry, S. (2018). Vegetation composition and plant diversity in mining disturbed tropical thorn forest of Asola-Bhatti Wildlife Sanctuary. Northern India. Taiwania 63, 267–280. doi: 10.6165/tai.2018.63.267
Sloan, S., Goosem, M., and Laurance, S. G. (2016). Tropical forest regeneration following land abandonment is driven by primary rainforest distribution in an old pastoral region. Landsc. Ecol. 31, 601–618. doi: 10.1007/s10980-015-0267-264
Souza, A., Schettino, S., Jesus, R., and Vale, A. (2002). Dinâmica da regeneração natural em uma floresta ombrófila densa secundária, após corte de cipós, reserva natural da companhia vale do rio doce S.A., estado do Espírito Santo. Brasil. Árvore 26, 411–419. doi: 10.1590/S0100-67622002000400003
Stanturf, J. A. (2005). ““What is forest restoration?,”,” in Restoration of Boreal and Temperate Forests, eds J. A. Stanturf and P. Madsen (Boca Raton, FL: CRC Press), 3–11. doi: 10.1007/978-94-007-5326-6_1
Swenson, J. J., Carter, C. E., Domec, J. C., and Delgado, C. I. (2011). Gold mining in the peruvian amazon: global prices, deforestation, and mercury imports. PLoS One 6:e18875. doi: 10.1371/journal.pone.0018875
Valois-Cuesta, H., and Martínez-Ruiz, C. (2017). Especies vegetales colonizadoras de áreas perturbadas por la minería en bosques pluviales del Chocó. Colombia. Biota Colomb. 18, 88–105.
Keywords: degraded area, forest disturbance, forest succession, Madre de Dios Region, natural regenaration, artisanal gold mining
Citation: Chambi-Legoas R, Ortega Rodriguez DR, Figueiredo FdMd, Peña Valdeiglesias J, Zevallos Pollito PA, Marcelo-Peña JL and Rother DC (2021) Natural Regeneration After Gold Mining in the Peruvian Amazon: Implications for Restoration of Tropical Forests. Front. For. Glob. Change 4:594627. doi: 10.3389/ffgc.2021.594627
Received: 13 August 2020; Accepted: 11 May 2021;
Published: 01 July 2021.
Edited by:
Manuel R. Guariguata, Center for International Forestry Research (CIFOR), IndonesiaReviewed by:
Francisco Roman, CONDESAN, PeruJess K. Zimmerman, University of Puerto Rico, Puerto Rico
Copyright © 2021 Chambi-Legoas, Ortega Rodriguez, Figueiredo, Peña Valdeiglesias, Zevallos Pollito, Marcelo-Peña and Rother. This is an open-access article distributed under the terms of the Creative Commons Attribution License (CC BY). The use, distribution or reproduction in other forums is permitted, provided the original author(s) and the copyright owner(s) are credited and that the original publication in this journal is cited, in accordance with accepted academic practice. No use, distribution or reproduction is permitted which does not comply with these terms.
*Correspondence: Daigard Ricardo Ortega Rodriguez, dai.ricardo.or@gmail.com; dai.ricardo.or@usp.br