- 1Phytophthora Science and Management, Centre for Climate Impacted Terrestrial Ecosystems, Harry Butler Institute, Murdoch University, Murdoch, WA, Australia
- 2School of Biological Sciences, University of Canterbury, Christchurch, New Zealand
- 3Department of Biochemistry, Genetics and Microbiology, Forestry and Agricultural Biotechnology Institute, University of Pretoria, Pretoria, South Africa
In forest ecosystems, habitat fragmentation negatively impacts stand structure and biodiversity; the resulting fragmented patches of forest have distinct, disturbed edge habitats that experience different environmental conditions than the interiors of the fragments. In southwest Western Australia, there is a large-scale decline of the keystone tree species Corymbia calophylla following fragmentation and land use change. These changes have altered stand structure and increased their susceptibility to an endemic fungal pathogen, Quambalaria coyrecup, which causes chronic canker disease especially along disturbed forest habitats. However, the impacts of fragmentation on belowground processes in this system are not well-understood. We examined the effects of fragmentation on abiotic soil properties and ectomycorrhizal (ECM) and arbuscular mycorrhizal (AM) fungal communities, and whether these belowground changes were drivers of disease incidence. We collected soil from 17 sites across the distribution range of C. calophylla. Soils were collected across a gradient from disturbed, diseased areas to undisturbed, disease-free areas. We analysed soil nutrients and grew C. calophylla plants as a bioassay host. Plants were harvested and roots collected after 6 months of growth. DNA was extracted from the roots, amplified using fungal specific primers and sequenced using Illumina MiSeq. Concentrations of key soil nutrients such as nitrogen, phosphorus and potassium were much higher along the disturbed, diseased edges in comparison to undisturbed areas. Disturbance altered the community composition of ECM and AM fungi; however, only ECM fungal communities had lower rarefied richness and diversity along the disturbed, diseased areas compared to undisturbed areas. Accounting for effects of disturbance, ECM fungal diversity and leaf litter depth were highly correlated with increased disease incidence in C. calophylla. In the face of global change, increased virulence of an endemic pathogen has emerged in this Mediterranean-type forest.
Introduction
Globally, forest health is threatened by multiple factors including invasive forest pests and pathogens and global change (i.e., climate change and anthropogenic disturbance). Anthropogenic disturbances such as habitat fragmentation and land use change are occurring at accelerated rates around the world and are some of the leading causes of biodiversity loss (Jung et al., 2019). For forested ecosystems, habitat loss results in small, isolated fragments interspersed among a matrix of human-modified land (Zambrano et al., 2019). Fragmented forests have distinct edge habitats that experience different environmental conditions than the interior of the fragments, including greater fluctuations in temperature and moisture, increased windthrow and evaporation, and increased access for people, invasive alien species including pathogens, and fire (Arroyo-Rodríguez et al., 2017; Latimer and Zuckerberg, 2017; Watson et al., 2018). Such changes in environmental conditions can cause increases in tree mortality, damage and turnover (Santo-Silva et al., 2016). The loss of plant diversity and changes in overall forest structure can, as a result, cause changes in litter return which can alter soil nutrient cycling (Xiao et al., 2017). The magnitude of the effects of fragmentation is also dependent on the type of land use bordering forest fragments. For example, fertilisation is common in horticultural and agricultural land, thus there is an increased probability of deposition by nitrogen and other nutrients and pollutants into edge habitats (Crockatt, 2012; Department of Primary Industries Regional Development, 2016). Edges adjacent to roads experience higher concentrations of salt and heavy metals than forest interiors (Laurance et al., 2009; Neher et al., 2017). Furthermore, highly fragmented forests may be exposed to multiple types of land use (i.e., one edge of a forest fragment is adjacent to a road and a second edge adjacent to agricultural land). The effects of exposure to multiple land uses may result in additive or interactive effects on tree health.
The distinct microclimates along edge habitats of fragmented forests are not only aboveground, but also belowground. Changes in soil microclimates can further impact belowground processes and communities. However, the implications of fragmentation belowground are not as well-understood as the effects aboveground. This has severe repercussions for the future of forest health, as many soil fungi are beneficial mutualists of trees. For example, mycorrhizal fungi (ectomycorrhizal (ECM) and arbuscular mycorrhizal (AM) fungi) provide their hosts with minerals and water through nutrient exchange, provide increased resistance to root pathogens and improve soil structure (Sapsford et al., 2017; Neuenkamp et al., 2019). Mycorrhizal fungi are highly sensitive to changes in abiotic soil properties (e.g., moisture, temperature, nutrients) and consequently, edge habitats may become inhospitable. In addition, changes in abiotic soil properties can alter processes these fungi are involved in including leaf litter decomposition, fruit body production and spore release (Crockatt, 2012). Nitrogen deposition alters ECM fungal community structure and reduces ECM root tip abundance and mycelial production (Kjøller et al., 2012). These alterations can result in decreased uptake of nitrogen by roots and fungi and increased losses of nitrogen by leaching (Kjøller et al., 2012). ECM and AM fungi might also react differently to fragmentation and land use change; for example, edges along a white spruce and aspen forest had decreased ECM richness but increased AM fungi in comparison to undisturbed forests (Ramsfield et al., 2020). The combined effects of abiotic and biotic changes in the soil can affect future tree establishment (Tonn and Ibáñez, 2016; Sapsford et al., 2017) and disruptions of these host-mutualist interactions will have cascading effects, threatening the health and function of forest ecosystems.
The southwest of Western Australia (SWWA) consists of a Mediterranean-type ecosystem and is a recognized biodiversity hotspot (Klausmeyer and Shaw, 2009; Mittermeier et al., 2011). However, this region is under threat by multiple disturbances; extensive land clearing has led to a highly fragmented landscape (Shepherd et al., 2002), the rainfall has declined and temperatures increased since the 1970's (Bates et al., 2008), and invasive pathogens such as Phytophthora cinnamomi have spread throughout the region (Burgess et al., 2017). The combination of these disturbances has negatively impacted forest health in SWWA. Specifically, aboveground, these disturbances have impacted forest stand structure, with highly disturbed fragmented areas having different overstory composition in comparison to less disturbed areas (Paap et al., 2018). These changes have led to the decline in health of marri (Corymbia calophylla), a keystone species in SWWA forests. The seeds of marri are an important food source for many species of parrots including the endangered Carnaby's black cockatoo (Calyptorhynchus latirostris) (Cooper et al., 2003), and these trees are common nesting locations for these birds. The effects of anthropogenic disturbances (i.e., fragmentation, climate change, Phytophthora spp.) have also increased the incidence and severity of an endemic pathogen, Quambalaria coyrecup, that causes a fatal canker disease in C. calophylla (Paap et al., 2017, 2018).
Increased incidence of Q. coyrecup in C. calophylla in disturbed areas can be influenced by multiple factors. Highly fragmented areas have resulted in remnant tree stands dominated by C. calophylla. Originally, these areas consisted of mixed Eucalyptus marginata and C. calophylla forest stands; however, E. marginata may be less tolerant to soil moisture variability and soil pathogens (e.g., Phytophthora cinnamomi) present in these fragmented areas than the more tolerant C. calophylla (Paap et al., 2018). In addition, E. marginata is also removed by selective logging practices. This loss of tree diversity may have led to levels of pathogen pressure above those which C. calophylla is adapted to in their naturally occurring forest setting (Ennos, 2015). Incidence of disease in C. calophylla can also be influenced by changes in belowground. Fragmented remnant stands of C. calophylla are surrounded by two types of land use: roads and land used for horticulture, agriculture, viticulture or grazing. Increased deposition of nitrogen and other fertilisers, pesticides and other pollutants are likely to be higher in these fragmented areas. These changes in abiotic soil properties can then alter communities of mycorrhizal fungi (Sapsford et al., 2017). Overall, disruptions in soil and mycorrhizal communities can alter nutrient and water acquisition by the host; this increase in stress increases the hosts susceptibility to pathogens such as Q. coyrecup (Manion, 1991). While the impacts of habitat fragmentation and land use change on aboveground processes in C. calophylla forest communities have been investigated, the effects on belowground processes are not well-understood. Corymbia calophylla forms associations with two types of mycorrhizal fungi: ECM and AM fungi (Sapsford et al., 2020). In the complex decline of C. calophylla, mycorrhizal fungal communities have yet to be considered. Therefore, our aim was to determine how abiotic soil properties, and ECM and AM fungal communities respond to edge habitats of fragmented forests exposed to various types of land use (road, agricultural/horticultural/viticultural/grazing or both). We hypothesized fragmented forests exposed to multiple land uses would have (1) increased concentrations of soil nutrients and greater soil moisture, (2) decreased richness and diversity of mycorrhizal fungi, and (3) increased richness and diversity of pathogens in comparison to fragmented forests exposed to a single land use change and undisturbed forests. Furthermore, the combination of changes in abiotic and biotic soil variables would drive canker incidence along disturbed edges exposed to multiple land uses.
Materials and Methods
Study Species and Area
Corymbia calophylla (Myrtaceae) is a bloodwood tree endemic to southwest Western Australia (SWWA) (Figure 1). This forest and woodland keystone species occurs in areas of 360–1,150 mm annual rainfall and average annual temperatures between 14 and 21°C (Raupach et al., 2009).
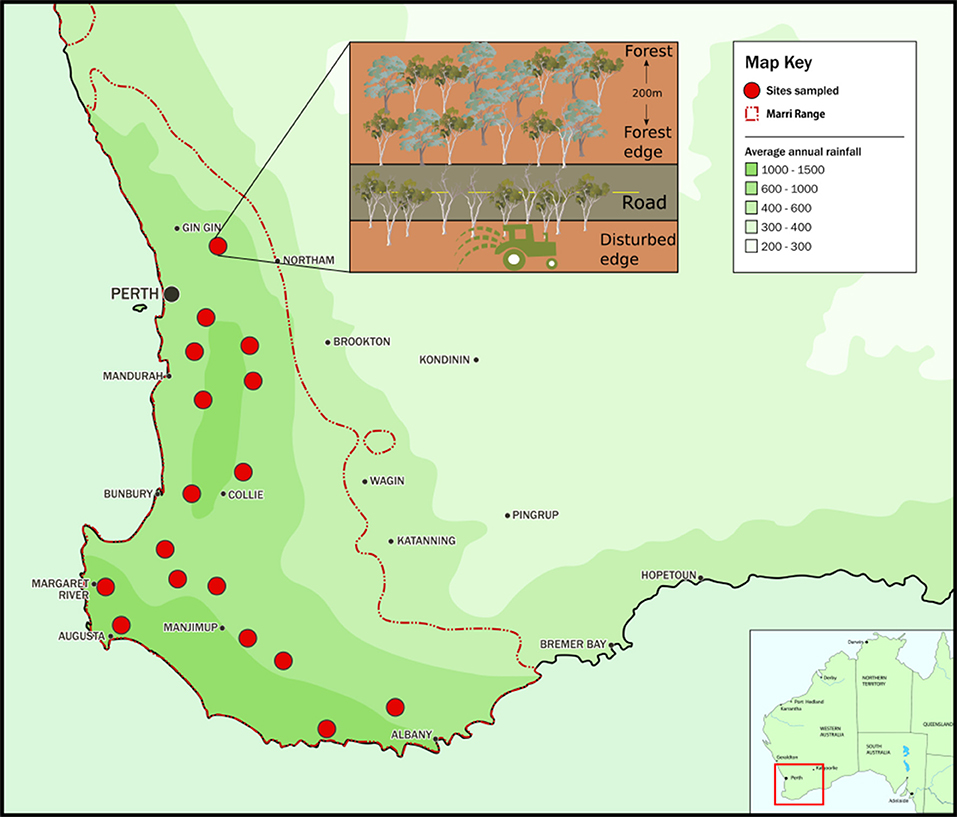
Figure 1. Map of the distribution range of Corymbia calophylla in southwest Western Australia and the 17 study sites. Inset: Each site consisted of three lateral transects perpendicular to the disturbance gradient. The disturbed edge consisted of a remnant stand of C. calophylla bordering agricultural land and a road; the forest edge was adjacent to the road; and the intact forest block was 200 m into intact forest.
Field Survey and Study Sites
Seventeen sites described by Paap et al. (2018) were selected throughout the C. calophylla range in SWWA (Figure 1). In brief, each site consisted of a gradient from a highly disturbed area (fragment exposed to two types of land use) to healthy forest (opposite side of road). The gradient included three lateral transects that were perpendicular to the disturbance gradient. The transect along the highly disturbed area (henceforth disturbed edge) consisted of a dominant, remnant stand of C. calophylla with many cankered trees (mean incidence of canker = 0.44). This transect had edges exposed to two types of land use: a road and land designated for agricultural, horticultural, viticultural, or grazing purposes (Inset of Figure 1). This remnant stand had few other tree and shrub species. The remaining two transects were part of an intact forest: one along the road edge (fragment exposed to only one type of land use), opposite the disturbed edge (~10 m from the disturbed edge), with few cankered trees (mean incidence of canker = 0.21), the other 200 m into the forest block (mean incidence of canker = 0.03). Each transect was 100 m in length and 3 m in width and thus an area of 300 m2. This gradient of disturbance allowed the comparison of impacts of multiple disturbances (e.g., the road and other land use along disturbed edges), a single disturbance (e.g., road along the forest edge) and no disturbance (e.g., healthy forest with no edge habitats). Assessment of canker presence was based on the presence of symptoms and signs associated with Q. coyrecup infection, as previously confirmed by Paap et al. (2018). For each transect, the number of C. calophylla trees with cankers was converted to a proportion by dividing it by the total number of surveyed C. calophylla trees present along the transect. All C. calophylla with a diameter at breast height of > 5 cm within each 300 m2 transect were assessed for canker. While floristically diverse, the dominant species in the forest block were C. calophylla, Eucalyptus marginata (Myrtaceae), and the mid-story species Xanthorrhoea preissii (Asphodelaceae) and Banksia grandis (Proteaceae).
Soil and Litter Collection
At each site, 30 C. calophylla trees were randomly selected, 10 from each transect (we walked along each transect and, at 10 m intervals, soil was collected from the nearest C. calophylla). One soil sample was collected 30 cm from the base of each tree to a depth of 20 cm using an auger (10 cm dia.). Samples were collected in June 2015 (austral winter) and bulked for each transect (51 bulked samples from the 17 sites). Bulked samples were mixed thoroughly using a cement mixer and then divided for soil nutrient analysis and a glasshouse bioassay. In addition, one handful of leaf litter around each tree was collected and bulked for each transect, for use in the glasshouse bioassay experiment, to mimic natural conditions along each transect at each site. This addition accounted for effects of leaf litter on mycorrhizal communities and soil abiotic variables. Leaf litter depth was recorded at each transect by taking the average of four measurements around each tree to the nearest 0.1 cm.
Abiotic Soil Properties and Nutrient Analysis
Soil temperature was recorded every 2 h for 12 months (October 2015 – October 2016) by placing a Thermochron iButton datalogger (model DS1921G, precision: 0.5°, accuracy: 1°) 10 cm below the surface of the soil at each transect. Dataloggers were placed ~1 m from a C. calophylla tree at the mid-point of each transect. If there was a layer of leaf litter, the litter was removed and the iButton placed 10 cm below the soil, and the leaf litter replaced.
Soil moisture was measured in March 2016 (end of austral summer and the driest time of year) and September 2016 (end of austral winter and the wettest season) by collecting soil from each transect at each site, weighing the soil, allowing the soil to dry (at a constant 37° for 2 weeks), and weighing the dried soil. The percentage difference in weight was used as a proxy for soil moisture throughout the analyses. Soil (500 g) from each transect, at each site, was sent for nutrient analysis (i.e., macro- and micronutrients, conductivity and pH; CSBP Soil and Plant Laboratory, Bibra Lake, Western Australia) (Supplementary Table 1).
Community Composition of Mycorrhizal Fungi
To determine the mycorrhizal communities associated with C. calophylla, plants were grown in soil collected from each transect (Sapsford et al., 2020), divided between five replicate (2.8 L) free-draining polyurethane pots (P175STK, 2.8L, Garden City Plastics, Canning Vale, Western Australia, 6155) for a total of 255 pots. Three C. calophylla seeds (provenance Plantagenet collected from 34°39'11.8512”S 117°30'5.8356”E) were placed into pots and each pot was covered in leaf litter collected (~1 cm depth) from the respective site and transect. An additional ten pots filled with pasteurized river sand (steamed at 65° for 3 h) acted as controls to account for potential glasshouse fungal contaminants present in glasshouse environments, giving a total of 265 pots. Control pots were covered in high density polyethylene beads (Qenos Pty Ltd, Altona, Victoria, Australia) to replicate leaf litter (~1 cm depth). Once germinated, seedlings were thinned to one per pot. Pots were watered to container capacity every 3 days. Due to the river sand being nutrient poor, the controls received one-quarter of the recommended rate of Thrive® (nitrogen: phosphorus = 5: 1; all-purpose soluble fertiliser; Yates Australia, Padstow, New South Wales, 2211) twice during the 6 months of the experiment to prevent plant death. Pots were placed in a randomized plot design, with each pot moved randomly every 2 weeks.
Plants were harvested after 6 months and the roots were rinsed of soil and stripped of fine roots (Sapsford et al., 2020) which were then separated from larger lateral roots using a sieve. To prevent contamination between samples, new gloves were worn for each sample. Fine roots (50 mg) were stored in 2 mL vials at −20°.
DNA Extraction and High-Throughput Sequencing
DNA was extracted from all roots using a PowerPlant Pro DNA isolation kit as per the manufacturer's protocol (now DNeasyPowerPlant Pro Kit from Qiagen). Amplicon library preparation was performed according to recommended protocols (Illumina Demonstrated Protocol: 16S Metagenomic Sequencing Library Preparation) with exceptions. eDNA was amplified using the fungal specific primer fITS7 (Integrated DNA Technologies, Baulkham Hills, New South Wales, 2153) and eukaryotic primer ITS4 as the reverse primer (Integrated DNA Technologies, Baulkham Hills, New South Wales, 2153) which amplified the ITS2 region (universal genetic barcode for fungi) (Ihrmark et al., 2012). Twelve extraction controls (i.e., no root sample included in the extraction) and 11 PCR controls (i.e., water used in place of DNA) were negative. PCR products were visualized on 2% agarose gels, and pooled based on DNA concentrations determined using the Qubit Flourometric Quantification (ThermoFisher). Some glasshouse controls only produced faint bands, but this was equalized at the pooling stage. Uniquely indexed libraries (265) were pooled for the sequencing run, which was performed on an Illumina MiSeq using 600-cycle V3 chemistry (300 bp paired-end reads) following the manufacturer's recommendations.
Bioinformatic Analysis
Paired-end reads were merged using USEARCH v8.0.1623 (Edgar, 2010) with a minimum overlap length of 50 bp with no gaps allowed in the merged alignments. Sequence deconvolution such as quality control and clustering was carried out using the bioinformatics platform Sequence Clustering and Analysis of Tagged Amplicons (SCATA; scata.mykopat.slu.se), developed and maintained by the Swedish University of Agricultural Sciences in Uppsala [parameters were set as per Clemmensen et al. (2015)]. Reads with low mean quality (<20) and sequences with missing primers were removed. Primer sequences were removed and sequences passing quality control were then clustered into operational taxonomic units (OTUs). The threshold distance for clustering was set at 0.015 [corresponding approximately to species level for ITS (Nguyen et al., 2017)] and minimum alignment for clusters (i.e., minimum length of pairwise alignment in the clustering process required to consider a sequence pair for clustering) was 0.85. Taxonomy of OTUs were identified by searching against the internally curated SCATA database (UNITE version 7.1) (Kõljalg et al., 2013; Clemmensen et al., 2015) and through BLAST searches in GenBank (https://www.ncbi.nlm.nih.gov/genbank/) using Geneious (version R9 http://www.geneious.com/). All identifications provided by SCATA were confirmed in Genbank. Positive identifications and phylogenetic analysis were conducted following methods outline by Sapsford et al. (2020).
Ecological information of each phylotype was based primarily on FUNGuild (https://github.com/UMNFuN/FUNGuild), following the user's manual where assignments were made on ranks of “probable” and “highly probable” (Nguyen et al., 2016a). Where there was no match or a phylotype was ranked as “possible,” literature searches were conducted and phylotypes assigned to a guild based on published literature. If more than one guild was applicable to a phylotype, then assignment was based on the most likely guild that would be found on tree roots. If no information was found than the phylotype was classified in an “unidentified fungus” guild.
Through SCATA, 76.5% of reads (total 14,035,861) passed quality control resulting in 1704 OTU's. OTUs that represented at least 0.001% of the reads (most dominant) were retained for analysis, and OTUs that were present in the glasshouse control pots were removed. This resulted in a library of 1665 OTU's. Of these, 255 were identified as mycorrhizal fungi through the library developed in a pilot study (Sapsford et al., 2020), literature and FUNGuild (Nguyen et al., 2016a) (Supplementary Table 2). Only OTUs that were classified as ectomycorrhizal (ECM), arbuscular mycorrhizal (AM) fungi or putative plant pathogens were retained in the following analyses, resulting in three separate OTU tables, one for each group. ECM fungi consisted of 191 OTUs, AM fungi consisted of 64 OTUs and putative plant pathogens consisted of 37 OTUs (Supplementary Table 2).
Statistical Analysis
All analyses were performed using R version 3.6.3 (R Core Team, 2020). Data exploration was carried out according to Zuur et al. (2010). Many soil nutrients and properties were collinear, and those with high variance inflation factor (VIF) were removed as collinearity can bias results (Zuur et al., 2010) (Supplementary Table 3). After accounting for collinearity, we finally included macronutrients: nitrate, nitrogen, phosphorus, potassium and sulfur; micronutrients: copper, iron, manganese and zinc; and soil properties: conductivity, leaf litter depth, mean soil temperature of wettest quarter, and winter soil moisture in the remaining analyses.
Soil Nutrients and Properties
Our first aim was to determine the effect of disturbance (i.e., habitat fragmentation and exposure to multiple land use) on abiotic soil properties; therefore, we used linear mixed models (LMM) to determine whether the concentration of soil nutrients differed across the three transects. Concentration (of each nutrient) was the response variable and the factor transect as the predictor variable. To incorporate the dependency among sites, site was used as a random intercept. Model assumptions were verified by visually inspecting residuals for assumptions of normality and homoscedasticity (Zuur and Ieno, 2016).
Community Composition of Soil Fungal Communities
To evaluate whether changes in the composition of communities of ectomycorrhizal (ECM) fungi were related to abiotic soil nutrients and properties, a distance-based redundancy analysis (dbRDA) was performed using function “capscale” in package vegan (Oksanen et al., 2015). We used the Bray-Curtis dissimilarity index to calculate dissimilarity values from the ECM fungal OTU relative abundance data. The dissimilarity matrix was used as the response variable and abiotic soil nutrients and properties (i.e., those that were significantly different across the disturbance gradient; Table 1) and transect (i.e., disturbance) were the explanatory variables. Transect was included to determine whether any abiotic soil nutrients or properties improve predictions or simply co-vary with disturbance. Relevant explanatory variables were selected by permutation tests with forward model selection using function ordiR2step in the vegan package (Oksanen et al., 2015). To test the significance of the model, we ran a permutation test (permutations = 1,000) using function ANOVA. We repeated this using Jaccard dissimilarity index to calculate dissimilarity values from the ECM fungal OTU presence-absence data. To evaluate changes in the composition of arbuscular mycorrhizal (AM) fungi and putative plant pathogens, we repeated the above dbRDA analysis on the AM fungal OTU data and plant pathogen OTU data.
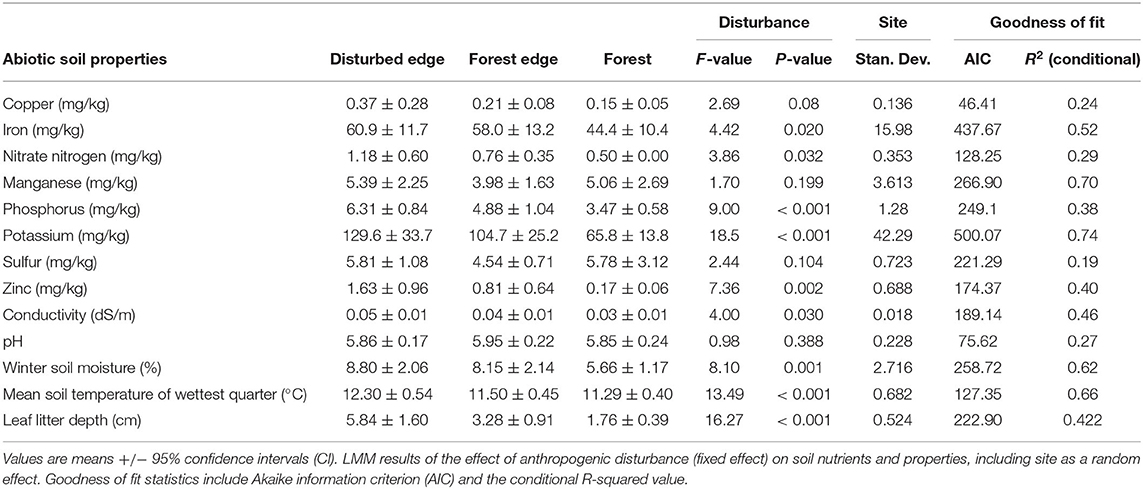
Table 1. Abiotic soil properties across seventeen sites in southwest Western Australia along a disturbance gradient.
We used linear mixed models (LMM) to determine whether rarefied richness and diversity of ECM, AM fungi and putative plant pathogens differed across the three transects; site was used as a random intercept. Model assumptions were verified by visually inspecting residuals for assumptions of normality and homoscedasticity (Zuur and Ieno, 2016). To determine richness (number of phylotypes) of each sample, OTUs were rarefied based on the minimum number of sequences (i.e., 1,000 for ECM fungi, 50 for AM fungi and 50 for putative plant pathogens) observed per sample using the rarefy function in the vegan package (Oksanen et al., 2015). The function diversity in the vegan package was used to calculate Shannon diversity and the exponent of Shannon diversity was calculated to obtain the effective number of species (Jost, 2006). To test the relationship between ECM fungi and plant pathogens, we also compared whether the richness or diversity of ECM fungi influenced the richness and diversity of plant pathogens irrespective of disturbance.
Canker Incidence
To investigate belowground drivers of canker presence, we modeled canker presence against belowground soil and fungal community factors. We used a binomial generalised linear mixed model with function glmer in the package lme4. Canker incidence of C. calophylla was the response variable. Fixed covariates included transect, soil nutrients (nitrate, phosphorus, potassium, copper, iron, zinc) and abiotic and biotic soil properties (ECM fungal diversity, ECM fungal richness, conductivity, winter soil moisture, leaf litter depth, and mean soil temperature of wettest quarter). All covariates were standardized (i.e., subtracting the mean and dividing by the standard deviation) before fitting the model, to ease the comparison of model coefficients. To incorporate the dependency among sites, site was used as a random intercept. Model assumptions were verified by visually inspecting residuals for assumptions of normality and homoscedasticity (Zuur and Ieno, 2016). The best model was based on Akaike's Information Criterion (AIC) (Burnham and Anderson, 2004). Models with lower AIC values are considered more parsimonious explanations of the data than models with higher AIC values. To tease out specific soil drivers within each transect, we also repeated the above analysis on each transect separately.
Results
The concentrations of most of the nutrients were significantly different (Table 1) across the disturbance gradient, with concentrations higher along disturbed edges (Supplementary Table 1). Copper, manganese and sulfur were the only nutrients that did not differ significantly across the disturbance gradient. Winter soil moisture and mean soil temperature of the wettest quarter were both significantly higher along disturbed edges. Disturbance had no effect on pH. Leaf litter depth was significantly higher along disturbed edges in comparison to forest transects (Table 1, Supplementary Table 1).
The most parsimonious distance- based redundancy analysis (dbRDA) model for ectomycorrhizal (ECM) fungi using Bray-Curtis dissimilarity suggested disturbance, moisture and zinc were the main drivers (F4,45 = 0.2.753, p = 0.001, R2 = 0.13) explaining the variation among the communities of ECM fungi (Figure 2A). The presence-absence of ECM fungi (Jaccard dissimilarity) suggested nitrate, potassium and soil moisture improved predictions of species presence-absence in addition to the effect of disturbance (F5,44 = 2.016, p = 0.001, R2 = 0.09) (Figure 2D). The most parsimonious dbRDA model for arbuscular mycorrhizal (AM) fungi (using Bray-Curtis dissimilarity) suggested disturbance, soil moisture and potassium were the main drivers of AM fungal communities (F4,45 = 2.594, p = 0.001, R2 = 0.12) (Figure 2B). Nitrate and iron were additional abiotic soil properties explaining the variation in the presence-absence of AM fungi (F6,43 = 2.859, p = 0.001, R2 = 0.20) (Figure 2E). The most parsimonious dbRDA model for putative plant pathogens (using Bray-Curtis dissimilarity) suggested leaf litter depth and soil moisture were the main drivers explaining the variation among these communities (F2,47 = 2.824, p = 0.001, R2 = 0.08) (Figure 2C). There was no effect of disturbance on community composition of plant pathogens based on relative abundance. Alternatively, disturbance, along with potassium and soil moisture were drivers of the presence-absence of plant pathogens (F4,45 = 1.898, p = 0.001, R2 = 0.09) (Figure 2F).
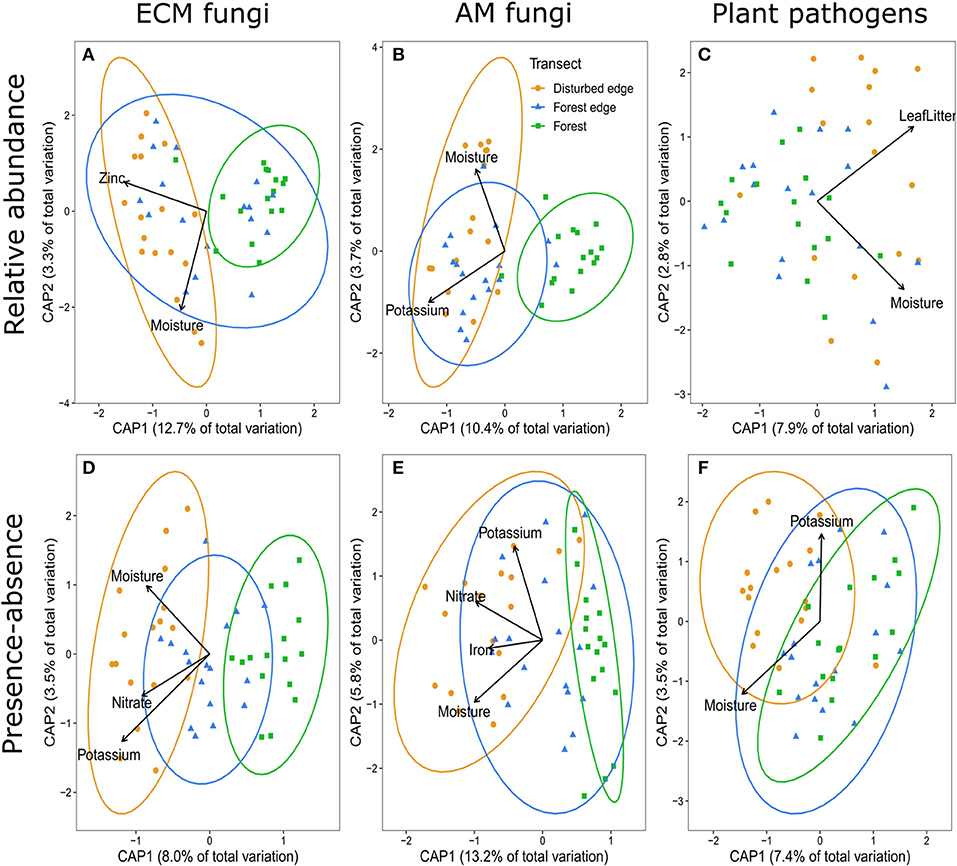
Figure 2. Distance-based redundancy analysis (dpRDA) demonstrating the communities of (A,D) ectomycorrhizal (ECM) fungi, (B,E) arbuscular mycorrhizal (AM) fungi, and (C,F) putative fungal plant pathogens associated with Corymbia calophylla grown in soils collected across a disturbance gradient: filled orange circles represent the disturbed edge [i.e., remnant stand of C. calophylla bordering a road with anthropogenic disturbance (agricultural, horticultural, viticultural or grazing purposes)], filled blue triangles indicate forest edge, and filled green sqaures indicate 200 m within a forest. Ellipses were included when disturbance significantly affected community composition. (A–C) represent the relative abundance (Bray-Curtis dissimilarity) of the communities and (D–F) represent presence-absence (Jaccard dissimilarity) of the communities.
Both rarefied richness (F = 10.001, P < 0.001) and diversity (F = 7.192, P = 0.003) of ECM fungi significantly differed across the disturbance gradient with communities from intact forests with the highest richness and diversity (Figures 3A,B). The diversity (p = 0.854) and richness of AM fungi (p = 0.936) did not differ across the disturbance gradient. The diversity (p = 0.300) and richness of plant pathogens (p = 0.100) did not differ across the disturbance gradient. No abiotic soil properties influenced ECM, AM fungi and pathogen richness and diversity. Neither ECM fungal richness (p = 0.436) nor diversity (p = 0.594) influenced the richness or diversity of pathogens.
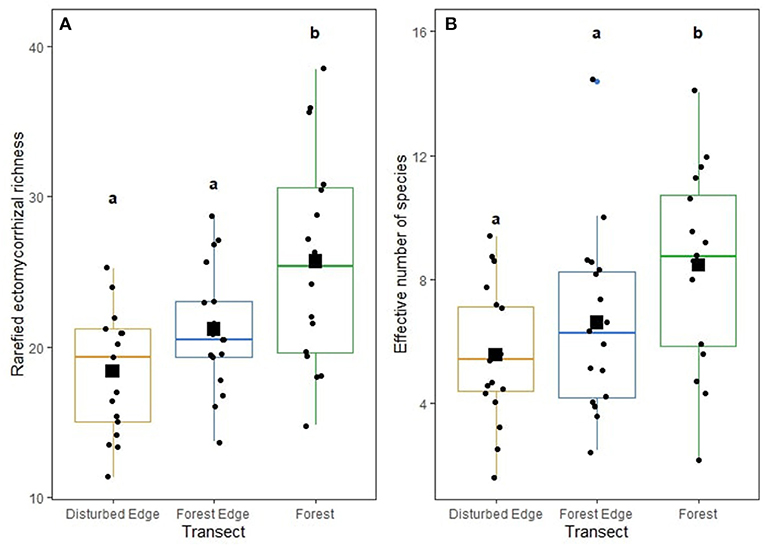
Figure 3. (A) Rarefied ectomycorrhizal (ECM) fungal richness and (B) effective number of species (exponent of Shannon diversity) of ectomycorrhizal (ECM) fungi across the disturbance gradient: disturbed edge [i.e., remnant stand of Corymbia calophylla bordering a road with anthropogenic disturbance (agriculture, horticulture, viticulture or grazing)], forest edge, and 200 m within a forest. For each boxplot, the median value is represented by a horizontal line, the first and third quartiles by boxes and values outside the quartiles by whiskers. Outliers are values >1.5 times the interquartile range. Squares indicate mean values and circles indicate range of data. Lower case letters that differ are significantly (p < 0.003) different from each other.
Based on the most parsimonious binomial GLMM, the proportion of trees with canker (canker incidence) was driven by leaf litter depth, ECM fungal diversity (exponent of Shannon diversity) and disturbance (Table 2; Figure 4). These results are corroborated by our most parsimonious binomial GLMM looking at disturbed edges only. Both leaf litter depth (estimate = 0.415, z = 5.163, p < 0.001) and ECM fungal diversity (estimate = −0.350, z = −2.804, p = 0.005) were significant drivers of canker incidence along disturbed edges only. By looking at the disturbed edges separately, we determined that leaf litter depth and ECM fungal diversity improved predictions of canker incidence (and did not co-vary with disturbance). There were no significant drivers of canker incidence along the forest edges or forest transect.
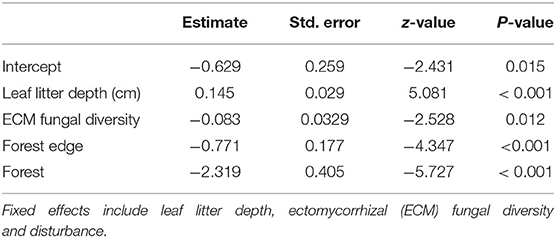
Table 2. Binomial GLMM results of the effects of soil nutrients and properties on the proportion of canker incidence.
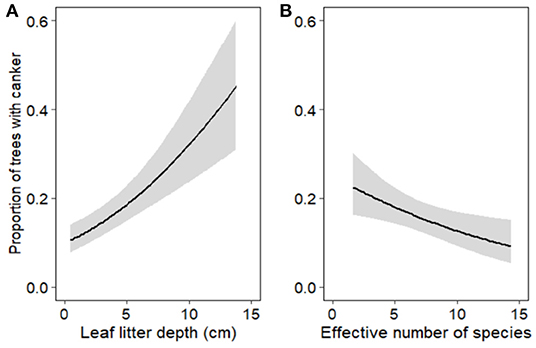
Figure 4. Canker incidence in Corymbia calophylla as a function of (A) leaf litter depth (cm) and (B) effective number of species (exponent of Shannon diversity) of ectomycorrhizal (ECM) fungi. Lines indicate mean fitted values extracted from the best fit model and gray ribbons represent 95% confidence intervals about the means.
Discussion
Drivers of Canker Incidence
Leaf litter depth and diversity of ectomycorrhizal (ECM) fungi were the two main drivers (along with disturbance) of canker incidence. Specifically, deeper leaf litter was found around trees with high incidence of canker along highly disturbed areas. It is most likely leaf litter is not a direct driver of canker incidence, but is imposing indirect effects on soil conditions which are resulting in the increase of C. calophylla's susceptibility to canker disease. For example, the increase in leaf litter along disturbed edges could be a by-product of tree mortality as a result of canker disease. Infection by Quambalaria coyrecup can result in fatal canker lesions on the stem and/or branches (Trudy Paap et al., 2017). As trees and limbs die, there is greater accumulation of leaf litter. Increased leaf litter could be acting as an insulator, thus preventing evaporation of water and preventing changes in soil temperatures along disturbed edges (Sydes and Grime, 1981). Along these disturbed, diseased edges we observed higher levels of soil moisture and less variation in soil temperatures. Therefore, this by-product of disease could be promoting a positive feedback loop (i.e., increased canker incidence results in increased leaf litter which results in excess soil moisture and thus increased susceptibility of C. calophylla to canker disease). However, over time, as the decline progresses, this feedback could be disrupted and there could be less litter along these disturbed edges. Long-term monitoring combined with leaf litter removal experiments at these disturbed sites could disentangle the direct and indirect effects of leaf litter and soil conditions on driving canker disease.
ECM fungal diversity was a biotic driver of canker incidence in C. calophylla, but not as strongly correlated as leaf litter depth. Low ECM fungal diversity was associated with disturbed, diseased edges in comparison to undisturbed areas. ECM fungi are important for tree health as they provide nutrients for their host and produce a mantle around root tips which is thought to provide protection against root pathogens (Albornoz et al., 2017); however, Q. coyrecup most likely spreads aerially, but epidemiology of this pathogen in relatively unknown (Paap et al., 2016). Therefore, communities of ECM fungi are not directly protecting against Q. coyrecup. However, it has been demonstrated in various ecosystems that healthy trees have a greater diversity of ECM fungi in comparison to disease-induced declining trees (Corcobado et al., 2014, 2020; Chu et al., 2016; Bzdyk et al., 2019). Greater diversity increases nutrient uptake efficiency which increases host productivity (Köhler et al., 2018). The changes in diversity we observed may be influencing the ability of their hosts to combat Q. coyrecup, but as ECM fungi are sensitive to edaphic changes, disentangling the role of these fungi in tree declines is challenging (Sapsford et al., 2017).
Abiotic Soil Properties
In our study, disturbed edges had higher concentrations of many soil nutrients (e.g., nitrate nitrogen, phosphorus and potassium) and had higher temperature (in austral winter) and moisture (both austral winter and summer) than forest edges. The magnitude of edge effects on abiotic soil properties were dependent on the type of land use bordering forest edges: the response of soil nutrients and properties along disturbed edges seem to be enhanced by the dual effects of the two types of land use change at our sites (i.e., road and farming). In comparison, our forest edges only bordered roads and thus soil conditions were more similar to conditions in undisturbed forests. The additive effect of exposure to multiple land uses could be due to the differences in how the two types of land uses alter abiotic soil properties. Roads are usually constructed using a cut-and-fill approach which can impede drainage and can also be a large source for nutrient and pollutant run-offs, which can be intensified by the increased water collected along these edges (Laurance et al., 2009). In comparison, nutrient fertilisation is common on farming land (Bolan et al., 1990; Lockie, 1997; Chudleigh and Simpson, 2001) and can have effects on the chemical properties of the rhizosphere such as changes in pH (although there was no significant change across the disturbance gradient in this study) and the presence and availability of soil nutrients (Jacobs and Timmer, 2005).
In addition to disturbance effects, concentrations of nutrients along disturbed edges could also be higher (than undisturbed areas) due to the increased amount of leaf litter. The amount and composition of leaf litter can influence soil organic matter, other nutrient concentrations and pH (De Groote et al., 2018). Tree species also differ in their chemical litter characteristics and thus can affect soil abiotic properties in a species-specific way (Grossman et al., 2020). Therefore, the disturbed edge dominated by C. calophylla is influencing soil conditions through its dominant leaf litter in comparison to the more diverse healthy forest stand. Comparing litter decomposition and the resulting changes in biogeochemical cycles across the disturbance gradient would provide insight into understanding the impacts of disturbance on abiotic soil properties and processes.
Soil Fungal Communities
Disturbance was also the main factor behind changes in community composition of ECM, AM fungi and putative plant pathogens. Both the relative abundance and presence-absence of species within each of these communities (besides pathogen relative abundance) was affected by disturbance. The changes in soil nutrients and properties as a result of habitat fragmentation can alter soil fungal communities: mycorrhizal fungi are sensitive to changes in soil moisture, temperature and nutrients availability (Ge et al., 2017; McBurney et al., 2017). Specifically, soil moisture along disturbed edges drove both abundance and presence-absence of these communities. ECM, AM fungi and pathogens are sensitive to changes in soil conditions, especially soil moisture. For example, plants in flooded soils have lower AM fungal diversity than plants exposed to soil moistures between 20 and 50% (Deepika and Kothamasi, 2015). ECM fungal species are also sensitive to changes in soil moisture, where certain species like to occupy soils of low moisture and others of high moisture (McBurney et al., 2017). Similarly, fungal pathogens tend to survive better in moist environments where the negative effects of pathogens are more prolific (Domínguez-Begines et al., 2020). Changes in soil nutrients, such as potassium and nitrate, had a greater influence on the presence-absence of soil fungal communities than the relative abundance. Both ECM (Morrison et al., 2016) and AM fungi (Jiang et al., 2018) community composition are sensitive to long-term nitrogen addition; however, certain ECM fungal genera such as Laccaria and Paxillus are nitrophilic and thus this sensitivity is species specific (Fransson, 2012).
Community compositional changes were reflected in changes of richness and diversity, but only in ECM fungi. The interior forest sites had higher ECM fungal species richness and diversity than the disturbed edges. Disturbance was the main predictor of changes in richness and diversity suggesting abiotic soil properties co-varied with disturbance (rather than improved predictions of richness and diversity). Interestingly, richness and diversity of ECM fungi did not differ between disturbed edges and forest edges, suggesting no additive effect of multiple disturbances. A single disturbance is enough to disrupt the richness and diversity of ECM fungi. We observed no effect of disturbance or abiotic soil properties on AM fungi or plant pathogen communities. In this case, however, an absence of an effect does not necessarily mean there is no effect. In our study, we used fungi-specific primers and these primers do not amplify AM fungi or root pathogens such as Phytophthora spp. well. Interestingly, in Eucalyptus gomphcephela seedlings, colonisation by AM fungi was greater in roots from sites with declining canopies than from sites with healthy canopies (Ishaq et al., 2018). Therefore, to fully understand AM fungi and oomycetes, further work is needed. In addition, root infection of ECM, AM fungi and plant pathogens could also be impacted by disturbance and abiotic soil properties. It has been demonstrated ECM fungal infection of root tips in healthy trees is between 57 and 64% greater than in Phytophthora-induced declining trees (Corcobado et al., 2020). Understanding how AM and ECM fungi and oomycetes interact at the root level is not well-understood and is an avenue for future research (Sapsford and Waller, 2020).
Overstory diversity can also influence communities of soil fungi; ECM fungal species richness is positively influenced by plant phylogenetic diversity (Nguyen et al., 2016b). In our study, disturbed edges had low tree species diversity as they were dominated by C. calophylla. In comparison, our forest transects had greater tree diversity (Paap et al., 2018). Increased plant diversity may result in more heterogeneous litter inputs which provides a variety of different niches for mycorrhizal fungi (Dickie, 2007). This may partially explain the increased ECM fungal species richness, and thus the increase in abundance of certain taxa found within the forest. Even though we only measured colonisation in C. calophylla, our community represents what was available as propagules in the soil and thus the forest interiors potentially provided more diverse communities of propagules for C. calophylla to associate with. This host diversity, in combination with low anthropogenic disturbance, within the healthy forest transects may be promoting an increase in ECM fungal species richness.
The complex decline of C. calophylla represents an example of, in the face of global change, how endemic pathogens may become more prevalent and pose as great a threat as invasive alien pathogens in forest ecosystems. Habitat fragmentation and land use change are altering abiotic soil properities and beneficial mycorrhizal communities. In addition, climate and drought events are playing a major role in the development of cankers in C. calophylla: incidence of canker is higher in wetter, cooler environments (Paap et al., 2017), and the timing of droughts within these environments are important in disease development (Hossain et al., 2018). Pathogens such as Phytophthora spp. (Paap et al., 2017; Croeser et al., 2018) in combination with Q. coyrecup are further contributing to the overall decline of this endemic tree species. By highlighting the complexity of tree decline, we shed light on the importance of considering both abiotic and biotic factors above and belowground in understanding tree declines.
Data Availability Statement
The datasets presented in this study can be found in online repositories. The names of the repository/repositories and accession number(s) can be found at: https://datadryad.org/stash/share/FxJVn8lpHtmCG5UI28_2bRgjIZ-YXGAKubCzQFErYRs.
Author Contributions
SS, GH, TP, and TB conceived and designed the methodology. SS performed the fieldwork, glasshouse work, molecular work, statistical analyses, and wrote the first draft of the manuscript. All authors discussed the results and contributed to the final version of the manuscript.
Funding
Funding was provided by the Holsworth Wildlife Research Endowment, the Australasian Mycological Society and the Australian Research Council Linkage LP120200581.
Conflict of Interest
The authors declare that the research was conducted in the absence of any commercial or financial relationships that could be construed as a potential conflict of interest.
Acknowledgments
We thank Briony Williams, Chris Shaw, Emma Steel, Claire Maltby, Clarissa Rowell, and Lou-an Crous for volunteering their time to help with field and glasshouse work, and Diane White for assistance in the laboratory. The project was carried out under permit SW016347, issued by the Western Australian Department of Biodiversity, Conservation and Attractions.
Supplementary Material
The Supplementary Material for this article can be found online at: https://www.frontiersin.org/articles/10.3389/ffgc.2020.593243/full#supplementary-material
References
Albornoz, F. E., Burgess, T. I., Lambers, H., Etchells, H., and Laliberté, E. (2017). Native soilborne pathogens equalize differences in competitive ability between plants of contrasting nutrient-acquisition strategies. J. Ecol. 105, 549–557. doi: 10.1111/1365-2745.12638
Arroyo-Rodríguez, V., Saldaña-Vázquez, R. A., Fahrig, L., and Santos, B. A. (2017). Does forest fragmentation cause an increase in forest temperature? Ecol. Res. 32, 81–88. doi: 10.1007/s11284-016-1411-6
Bates, B. C., Hope, P., Ryan, B., Smith, I., and Charles, S. (2008). Key findings from the Indian Ocean climate initiative and their impact on policy development in Australia. Clim. Change 89, 339–354. doi: 10.1007/s10584-007-9390-9
Bolan, N., White, R., and Hedley, M. (1990). A review of the use of phosphate rocks as fertilizers for direct application in Australia and New Zealand. Aust. J. Experi. Agricult. 30, 297–313. doi: 10.1071/EA9900297
Burgess, T. I., Scott, J. K., McDougall, K. L., Stukely, M. J. C., Crane, C., Dunstan, W. A., et al. (2017). Current and projected global distribution of Phytophthora cinnamomi, one of the world's worst plant pathogens. Glob. Change Biol. 23, 1661–1674. doi: 10.1111/gcb.13492
Burnham, K. P., and Anderson, D. R. (2004). Multimodel inference - understanding AIC and BIC in model selection. Sociol. Methods Res. 33, 261–304. doi: 10.1007/b97636
Bzdyk, R. M., Olchowik, J., Studnicki, M., Nowakowska, J. A., Oszako, T., Urban, A., et al. (2019). Ectomycorrhizal colonisation in declining oak stands on the Krotoszyn Plateau, Poland. Forests 10:30. doi: 10.3390/f10010030
Chu, H., Wang, C., Wang, H., Chen, H., and Tang, M. (2016). Pine wilt disease alters soil properties and root-associated fungal communities in Pinus tabulaeformis forest. Plant Soil 404, 237–249. doi: 10.1007/s11104-016-2845-x
Chudleigh, P., and Simpson, S. (2001). The Contribution of Fertilizers to Agricultural Production in Australia. Kingston: ACT.
Clemmensen, K. E., Finlay, R. D., Dahlberg, A., Stenlid, J., Wardle, D. A., and Lindahl, B. D. (2015). Carbon sequestration is related to mycorrhizal fungal community shifts during long-term succession in boreal forests. New Phytol. 205, 1525–1536. doi: 10.1111/nph.13208
Cooper, C. E., Withers, P. C., Mawson, P. R., Johnstone, R., Kirkby, T., Prince, J., et al. (2003). Characteristics of marri (Corymbia calophylla) fruits in relation to the foraging behaviour of the forest red-tailed black cockatoo (Calyptorhynchus banksii naso). J. R. Soc. Western Aust. 86, 139–142.
Corcobado, T., Cech, T. L., Brandstetter, M., Daxer, A., Hüttler, C., Kudláĉek, T., et al. (2020). Decline of European Beech in Austria: involvement of Phytophthora spp. and contributing biotic and abiotic factors. Forests 11:895. doi: 10.3390/f11080895
Corcobado, T., Vivas, M., Moreno, G., and Solla, A. (2014). Ectomycorrhizal symbiosis in declining and non-declining Quercus ilex trees infected with or free of Phytophthora cinnamomi. For. Ecol. Manage. 324, 72–80. doi: 10.1016/j.foreco.2014.03.040
Crockatt, M. E. (2012). Are there edge effects on forest fungi and if so do they matter? Fungal Biol. Rev. 26, 94–101. doi: 10.1016/j.fbr.2012.08.002
Croeser, L., Paap, T., Calver, M. C., Andrew, M. E., Hardy, G. E. S. J., and Burgess, T. I. (2018). Field survey, isolation, identification and pathogenicity of Phytophthora species associated with a Mediterranean-type tree species. Forest Pathol. 48:e12424. doi: 10.1111/efp.12424
De Groote, S. R. E., Vanhellemont, M., Baeten, L., De Schrijver, A., Martel, A., Bonte, D., et al. (2018). Tree species diversity indirectly affects nutrient cycling through the shrub layer and its high-quality litter. Plant Soil 427, 335–350. doi: 10.1007/s11104-018-3654-1
Deepika, S., and Kothamasi, D. (2015). Soil moisture-a regulator of arbuscular mycorrhizal fungal community assembly and symbiotic phosphorus uptake. Mycorrhiza 25, 67–75. doi: 10.1007/s00572-014-0596-1
Department of Primary Industries and Regional Development (2016). Fertiliser Partnership Overview. Perth, WA: Government of Western Australia.
Dickie, I. A. (2007). Host preference, niches and fungal diversity. New Phytol. 174, 230–233. doi: 10.1111/j.1469-8137.2007.02055.x
Domínguez-Begines, J., Ávila, J. M., García, L. V., and Gómez-Aparicio, L. (2020). Soil-borne pathogens as determinants of regeneration patterns at community level in Mediterranean forests. New Phytol. 227, 588–600. doi: 10.1111/nph.16467
Edgar, R. C. (2010). Search and clustering orders of magnitude faster than BLAST. Bioinformatics 26, 2460–2461. doi: 10.1093/bioinformatics/btq461
Ennos, R. A. (2015). Resilience of forests to pathogens: an evolutionary ecology perspective. Forestry 88, 41–52. doi: 10.1093/forestry/cpu048
Fransson, P. (2012). Elevated CO2 impacts ectomycorrhiza-mediated forest soil carbon flow: fungal biomass production, respiration and exudation. Fungal Ecol. 5, 85–98. doi: 10.1016/j.funeco.2011.10.001
Ge, Z.-W., Brenneman, T., Bonito, G., and Smith, M. E. (2017). Soil pH and mineral nutrients strongly influence truffles and other ectomycorrhizal fungi associated with commercial pecans (Carya illinoinensis). Plant Soil 418, 493–505. doi: 10.1007/s11104-017-3312-z
Grossman, J. J., Cavender-Bares, J., and Hobbie, S. E. (2020). Functional diversity of leaf litter mixtures slows decomposition of labile but not recalcitrant carbon over two years. Ecol. Monogr. 90:e01407. doi: 10.1002/ecm.1407
Hossain, M., Veneklaas, E. J., Hardy, G. E. S. J., and Poot, P. (2018). Tree host–pathogen interactions as influenced by drought timing: linking physiological performance, biochemical defence and disease severity. Tree Physiol. 39, 6–18. doi: 10.1093/treephys/tpy113
Ihrmark, K., Bödeker, I. T. M., Cruz-Martinez, K., Friberg, H., Kubartova, A., Schenck, J., et al. (2012). New primers to amplify the fungal ITS2 region – evaluation by 454-sequencing of artificial and natural communities. FEMS Microbiol. Ecol. 82, 666–677. doi: 10.1111/j.1574-6941.2012.01437.x
Ishaq, L., Barber, P. A., Hardy, G. E. St. J., Calver, M., and Dell, B. (2018). Seedling mycorrhizal type and soil chemistry are related to canopy condition of Eucalyptus gomphocephela. Mycorrhiza 23, 359–371. doi: 10.1007/s00572-012-0476-5
Jacobs, F. D., and Timmer, R. V. (2005). Fertilizer-induced changes in rhizosphere electrical conductivity: relation to forest tree seedling root system growth and function. New Forests 30, 147–166. doi: 10.1007/s11056-005-6572-z
Jiang, S., Liu, Y., Luo, J., Qin, M., Johnson, N. C., Öpik, M., et al. (2018). Dynamics of arbuscular mycorrhizal fungal community structure and functioning along a nitrogen enrichment gradient in an alpine meadow ecosystem. New Phytol. 220, 1222–1235. doi: 10.1111/nph.15112
Jung, M., Rowhani, P., and Scharlemann, J. P. W. (2019). Impacts of past abrupt land change on local biodiversity globally. Nat. Commun. 10:5474. doi: 10.1038/s41467-019-13452-3
Kjøller, R., Nilsson, L.-O., Hansen, K., Schmidt, I. K., Vesterdal, L., and Gundersen, P. (2012). Dramatic changes in ectomycorrhizal community composition, root tip abundance and mycelial production along a stand-scale nitrogen deposition gradient. New Phytol. 194, 278–286. doi: 10.1111/j.1469-8137.2011.04041.x
Klausmeyer, K. R., and Shaw, M. R. (2009). Climate change, habitat loss, protected areas and the climate adaptation potential of species in mediterranean ecosystems worldwide. PLoS ONE 4:e6392. doi: 10.1371/journal.pone.0006392
Köhler, J., Yang, N., Pena, R., Raghavan, V., Polle, A., and Meier, I. C. (2018). Ectomycorrhizal fungal diversity increases phosphorus uptake efficiency of European beech. New Phytol. 220, 1200–1210. doi: 10.1111/nph.15208
Kõljalg, U., Nilsson, R. H., Abarenkov, K., Tedersoo, L., Taylor, A. F. S., Bahram, M., et al. (2013). Towards a unified paradigm for sequence-based identification of fungi. Mol. Ecol. 22, 5271–5277. doi: 10.1111/mec.12481
Latimer, C. E., and Zuckerberg, B. (2017). Forest fragmentation alters winter microclimates and microrefugia in human-modified landscapes. Ecography 40, 158–170. doi: 10.1111/ecog.02551
Laurance, W. F., Goosem, M., and Laurance, S. G. W. (2009). Impacts of roads and linear clearings on tropical forests. Trends Ecol. Evol. 24, 659–669. doi: 10.1016/j.tree.2009.06.009
Lockie, S. (1997). Chemical risk and the self-calculating farmer: diffuse chemical use in Australian broadacre farming systems. Curr. Sociol. 45, 81–97. doi: 10.1177/001139297045003005
McBurney, K. G., Cline, E. T., Bakker, J. D., and Ettl, G. J. (2017). Ectomycorrhizal community composition and structure of a mature red alder (Alnus rubra) stand. Fungal Ecol. 27, 47–58. doi: 10.1016/j.funeco.2017.02.006
Mittermeier, R. A., Turner, W. R., Larsen, F. W., Brooks, T. M., and Gascon, C. (2011). “Global biodiversity conservation: the critical role of hotspots,” in: Biodiversity Hotspots: Distribution and Protection of Conservation Priority Areas, eds F. E. Zachos and J. C. Habel (Berlin: Springer Berlin Heidelberg), 3–22. doi: 10.1007/978-3-642-20992-5_1
Morrison, E. W., Frey, S. D., Sadowsky, J. J., van Diepen, L. T. A., Thomas, W. K., and Pringle, A. (2016). Chronic nitrogen additions fundamentally restructure the soil fungal community in a temperate forest. Fungal Ecol. 23, 48–57. doi: 10.1016/j.funeco.2016.05.011
Neher, D. A., Williams, K. M., and Lovell, S. T. (2017). Environmental indicators reflective of road design in a forested landscape. Ecosphere 8:e01734. doi: 10.1002/ecs2.1734
Neuenkamp, L., Prober, S. M., Price, J. N., Zobel, M., and Standish, R. J. (2019). Benefits of mycorrhizal inoculation to ecological restoration depend on plant functional type, restoration context and time. Fungal Ecol. 40, 140–149. doi: 10.1016/j.funeco.2018.05.004
Nguyen, D., Boberg, J., Cleary, M., Bruelheide, H., Hönig, L., Koricheva, J., et al. (2017). Foliar fungi of Betula pendula: impact of tree species mixtures and assessment methods. Sci. Rep. 7:41801. doi: 10.1038/srep41801
Nguyen, N. H., Song, Z., Bates, S. T., Branco, S., Tedersoo, L., Menke, J., et al. (2016a). FUNGuild: An open annotation tool for parsing fungal community datasets by ecological guild. Fungal Ecol. 20, 241–248. doi: 10.1016/j.funeco.2015.06.006
Nguyen, N. H., Williams, L. J., Vincent, J. B., Stefanski, A., Cavender-Bares, J., Messier, C., et al. (2016b). Ectomycorrhizal fungal diversity and saprotrophic fungal diversity are linked to different tree community attributes in a field-based tree experiment. Mol. Ecol. 25, 4032–4046. doi: 10.1111/mec.13719
Oksanen, J., Blanchet, F. G., Kindt, R., Legendre, P., Minchin, P. R., Ohara, R. B., et al. (2015). Vegan: Community Ecology Package. Available online at: http://CRAN.R-project.org/package=vegan (accessed March 4, 2020).
Paap, T., Brouwers, N. C., Burgess, T. I., and Hardy, G. E. S. J. (2017). Importance of climate, anthropogenic disturbance and pathogens (Quambalaria coyrecup and Phytophthora spp.) on marri (Corymbia calophylla) tree health in southwest Western Australia. Ann. For. Sci. 74:62. doi: 10.1007/s13595-017-0658-6
Paap, T., Burgess, T. I., Calver, M., McComb, J. A., Shearer, B. L., and Hardy, G. E. S. (2016). A thirteen-year study on the impact of a severe canker disease of Corymbia calophylla, a keystone tree in Mediterranean-type forests. For. Pathol. 47:e12292. doi: 10.1111/efp.12292
Paap, T., Burgess, T. I., Rolo, V., Steel, E., and Hardy, G. E. S. J. (2018). Anthropogenic disturbance impacts stand structure and susceptibility of an iconic tree species to an endemic canker pathogen. For. Ecol. Manage. 425, 145–153. doi: 10.1016/j.foreco.2018.05.055
R Core Team (2020). R: A Language and Environment for Statistical Computing. Vienna: R Foundation for Statistical Computing.
Ramsfield, T., Shay, P.-E., Trofymow, T., Myrholm, C., Tomm, B., Gagné, P., et al. (2020). Distance from the forest edge influences soil fungal communities colonizing a reclaimed soil borrow site in boreal mixedwood forest. Forests 11:427. doi: 10.3390/f11040427
Raupach, M. R., Briggs, P. R., Haverd, V., King, E. A., Paget, M., and Trudinger, C. M. (2009). Australian Water Availability Project (AWAP): CSIRO Marine and Atmospheric Component: Final report for Phase 3. Canberra, ACT: Centre for Australian Weather and Climate Research, Bureau of Meteorology and CSIRO.
Santo-Silva, E. E., Almeida, W. R., Tabarelli, M., and Peres, C. A. (2016). Habitat fragmentation and the future structure of tree assemblages in a fragmented Atlantic forest landscape. Plant Ecol. 217, 1129–1140. doi: 10.1007/s11258-016-0638-1
Sapsford, S. J., Paap, T., Hardy, G. E. S. J., and Burgess, T. I. (2017). The chicken or the egg: which comes first, forest tree decline or loss of mycorrhizae? Plant Ecol. 218, 1093–1106. doi: 10.1007/s11258-017-0754-6
Sapsford, S. J., Paap, T., Hopkins, A. J. M., Hardy, G. E. S. J., and Burgess, T. I. (2020). Habitat fragmentation in a Mediterranean-type forest alters resident and propagule mycorrhizal fungal communities. Pedobiologia 78:150611. doi: 10.1016/j.pedobi.2019.150611
Sapsford, S. J., and Waller, L. P. (2020). Seeing the forest not just for its trees: exotic pathogens shift forest communities aboveground and belowground. New Phytol. 227, 283–285. doi: 10.1111/nph.16612
Shepherd, D. P., Beeston, G. R., and Hopkins, A. J. (2002). Department of Agriculture and Food. Perth, WA: Native vegetation in Western Australia: extent, type and status.
Sydes, C., and Grime, J. P. (1981). Effects of tree leaf litter on herbaceous vegetation in deciduous woodland: II. An experimental investigation. J. Ecol. 69, 249–262. doi: 10.2307/2259829
Tonn, N., and Ibáñez, I. (2016). Plant-mycorrhizal fungi associations along an urbanization gradient: implications for tree seedling survival. Urban Ecosyst. 20, 823–837. doi: 10.1007/s11252-016-0630-5
Watson, J. E. M., Evans, T., Venter, O., Williams, B., Tulloch, A., Stewart, C., et al. (2018). The exceptional value of intact forest ecosystems. Nat. Ecol. Evolut. 2, 599–610. doi: 10.1038/s41559-018-0490-x
Xiao, S., Zhang, W., Ye, Y., Zhao, J., and Wang, K. (2017). Soil aggregate mediates the impacts of land uses on organic carbon, total nitrogen, and microbial activity in a Karst ecosystem. Sci. Rep. 7, 1–10. doi: 10.1038/srep41402
Zambrano, J., Garzon-Lopez, C. X., Yeager, L., Fortunel, C., Cordeiro, N. J., and Beckman, N. G. (2019). The effects of habitat loss and fragmentation on plant functional traits and functional diversity: what do we know so far? Oecologia 191, 505–518. doi: 10.1007/s00442-019-04505-x
Zuur, A. F., and Ieno, E. N. (2016). A protocol for conducting and presenting results of regression-type analyses. Methods Ecol. Evolut. 7, 636–645. doi: 10.1111/2041-210X.12577
Keywords: Quambalaria coyrecup, land use change, habitat fragmentation, emerging forest disease, eDNA, disturbance, Corymbia calophylla, canker pathogen
Citation: Sapsford SJ, Paap T, Hardy GESJ and Burgess TI (2021) Anthropogenic Disturbance Impacts Mycorrhizal Communities and Abiotic Soil Properties: Implications for an Endemic Forest Disease. Front. For. Glob. Change 3:593243. doi: 10.3389/ffgc.2020.593243
Received: 10 August 2020; Accepted: 31 December 2020;
Published: 29 January 2021.
Edited by:
Joanna Mucha, Institute of Dendrology (PAN), PolandReviewed by:
Yongjun Liu, Lanzhou University, ChinaBinwei Wu, Chinese Academy of Sciences (CAS), China
Copyright © 2021 Sapsford, Paap, Hardy and Burgess. This is an open-access article distributed under the terms of the Creative Commons Attribution License (CC BY). The use, distribution or reproduction in other forums is permitted, provided the original author(s) and the copyright owner(s) are credited and that the original publication in this journal is cited, in accordance with accepted academic practice. No use, distribution or reproduction is permitted which does not comply with these terms.
*Correspondence: Sarah J. Sapsford, c2FyYWguc2Fwc2ZvcmQmI3gwMDA0MDtnbWFpbC5jb20=