- 1Centre for Tropical Environmental and Sustainability Science (TESS), James Cook University, Cairns, QLD, Australia
- 2Centro de Investigaciones Ecológicas La Macarena (CIEM), Universidad de Los Andes, Bogotá, Colombia
Dispersal, recruitment and establishment limitations are crucial processes shaping forest composition. In secondary forests these mechanisms may operate differently than in mature forests, because young and isolated secondary forests may suffer stronger limitations due to a lack of suitable dispersers and harsh environmental conditions—such as the elevated competition of exotic grasses. To assess establishment limitations in these forests, we undertook transplant experiments involving seeds and seedlings along a chronosequence of secondary forests in tropical Australia. The experiments included six species that varied in seed size (6–50 mm in length) and successional status (early to late successional). Seeds of five species were placed in one of three treatments: (1) exposed seeds, (2) fenced seeds, and (3) buried seeds, and multiple seedlings of six species were transplanted in block treatments. After 14–17 months, seed from all plant species germinated across all ages of secondary forests. However, in young secondary forest sites (4–12 years) fewer germinated seeds survived. The highest survival rates were observed for buried seeds (27.2%) compared to the low survival of exposed seeds (6.0%). Planted seedlings (6 spp) had the greatest overall survival (63.1%) and the highest growth rates in older secondary forests. We found that species identity was important for growth and survival in both experiments, but detected no effect of successional status or seed size. A crucial finding of this study was that the buried seeds of all species germinated and had relatively high survival irrespective of variation in site conditions or successional status, suggesting that seed availability may be a greater barrier to recovery of secondary forests in the region than the establishment limitations imposed by environmental conditions.
Introduction
Rapid transformation of mature tropical forests puts at risk the high biodiversity and the varied ecosystem services these forests provide. Approximately 70% of the tropical rainforest biome has been modified into a matrix of mature forest remnants, agricultural fields, and secondary forests of different ages (Dent et al., 2013; van Breugel et al., 2013). Secondary forests are essential to biodiversity conservation, carbon sequestration and landscape connectivity, and understanding limitations to their recovery is vital to ensure best-practice management in the future (Faria et al., 2009; Dent et al., 2013; Chazdon, 2014; Rozendaal et al., 2019).
Models of forest succession explain and predict changes in species composition specifying interactions among species, and how plant traits affect such interactions through time (Pickett et al., 1987; Meiners et al., 2015; Pulsford et al., 2016). Some models focus on the physiological and morphological traits of the first colonists (e.g., Egler, 1954; Connell and Slatyer, 1977) and highlight that most of the species that will dominate later in succession are present since the onset of succession (Egler, 1954). These species will either prevent or facilitate the colonization of other species according to their life-spans and stress tolerances (Drury and Nisbet, 1973; Connell and Slatyer, 1977). Other models propose that changes in species composition depend on tradeoffs between life history traits, that differentiate species in their ability to acquire resources (Pulsford et al., 2016). For example, the tolerance-fecundity tradeoff model (Muller-Landau, 2010) that also explains tropical succession assumes that species vary inversely in fecundity and stress-tolerance; species with high fecundity and small seeds are unable to tolerate environmental stress (e.g., low soil moisture) whereas species with low fecundity and large seeds are able to tolerate environmental stress. Nonetheless, small seeded species tend to dominate early in succession because they are highly fecund and can be dispersed widely (Coomes and Grubb, 2003) and long-lived pioneers (usually small-seeded) are an important component of tropical forests (Rüger et al., 2020). However, species with larger seeds present at the onset of succession should outcompete small-seeded plants because larger seed reserves provide higher per capita recruitment and survival (Moles and Westoby, 2004) increase resource provisioning (Westoby et al., 1996), stress tolerance (Muller-Landau, 2010) and improve seedling performance (Moles and Westoby, 2002, 2004).
Multiple mechanisms affect and shape forest regeneration in both mature and altered ecosystems (Benitez-Malvido and Martinez-Ramos, 2013). Dispersal and establishment limitations are two key processes that shape forest composition. Dispersal limitation is the “failure of the propagules of a given species to arrive at an available site” (Terborgh et al., 2011), whereas establishment limitation is the failure of a species to be present in all sites favorable for its growth and survival (Hubbell et al., 1999; Muller-Landau et al., 2002; Schupp et al., 2002). Therefore, although overcoming dispersal allows species to reach different sites, establishment limitations may prevent the germination and/or survival of the species. Both mechanisms not only play a fundamental role in maintaining the high biodiversity of mature tropical forests (Terborgh, 2012; Vargas and Stevenson, 2013), but also affect the recovery of regenerating forests, where long distances to seed sources and harsh or unfavorable environmental conditions may delay forest recovery (Guariguata and Ostertag, 2001; Norden et al., 2009). Although recruitment cannot occur without seeds, seeds are no guarantee of recruitment (Clark et al., 2013). Therefore, studying recruitment limitations in tropical secondary forests can provide insights into the barriers for the recovery of these ecosystems.
Although multiple factors preventing forest recovery are well known such as: lack of nutrients and limited water (Carrasco-Carballido et al., 2019), competition with grasses and adverse microhabitats (González-Tokman D.M. et al., 2018) and the recovery of taxonomic and functional diversity of indicator groups (González-Tokman D. et al., 2018), increased knowledge regarding the strength of the processes that influence recovery in different landscapes is required (Rozendaal et al., 2019). Experimental research may assist in providing the necessary information to understand natural regeneration in secondary forests and develop management strategies to aid their recovery (Bentos et al., 2013).
Direct seeding and planting of seedlings have been widely investigated in different ecosystems to examine the potential of species in forest restoration, their potential economic value to forestry, their use by wildlife or to investigate how functional traits (e.g., seed size) influence performance (Palma and Laurance, 2015). In this study, we used direct seeding and planting of seedlings to investigate whether establishment limitation is a major barrier to forest succession and recovery.
Our experiments tested one main hypothesis establishment limitation plays a major role in slowing down succession in secondary forests. We predicted that germination, survival and growth rates would vary among seeds and seedlings in a successional forest chronosequence because they may experience different establishment limitations. We expected lower germination, survival and growth rates in young secondary forests compared to older forests. We used large and small-seeded species and predicted that if secondary forest age and/or seed size does not affect establishment then there would be no difference in germination, survival and growth rates among treatments. The different seed treatments (exposed, fenced, buried) helped us understand establishment limitations in the different sites. If predation and/or desiccation were limiting establishment, buried seeds would be more successful in all age categories. Alternatively, if these establishment limitations do not constraint the germination of exposed or fenced seeds, all treatments would have equal germination, and just placing seeds directly in the soil would be a fast and easy approach to enhance biodiversity recovery in these regenerating forests.
Planted seedlings of the same species provided insights on how the environment could limit growth and survival. If high rates of herbivory, mortality, and slow growth were found in all forests, establishment limitations could be strongly influencing the recovery of these tropical forests. Experiments like these ones that evaluate establishment limitations could provide guidance for successful management strategies (de la Peña-Domene and Martínez-Garza, 2018).
Materials and Methods
Study Area and Site Selection
The study was carried out on the southern Atherton Tableland (17°23.3′S 145°35.8′E–17°27.0′S 145°38.2′E), a highly fragmented landscape in north-eastern Australia. The climate is tropical, with mean annual rainfall ranging from 1700 to 2600 mm, and a distinct dry season from July to October (BOM 2019). Mean monthly temperatures range from a mean minimum of 10°C in the drier months to a mean maximum of 29°C during the wet season.
In the 1920s much of the region was cleared of continuous rainforest and converted to pasture for the dairy industry (Frawley, 1987; Gilmore, 2005). Some pastures were abandoned in the 1940s and others in the 1980s as economic conditions changed (Gilmore, 2005). Following pasture abandonment, forest regenerated and now secondary forests of different ages dominate the landscape. Interpreting a time-series of aerial photographs and satellite imagery, we selected different sites that would provide us with a chronosequence of secondary forest age from 4 to 34 years since canopy formation. This study encompassed 12 secondary forest sites that were not directly adjacent to large tracts of mature rainforest (Figure 1). Our study sites varied in size from 0.7 to 4.6 ha, with an average size of 2.6 ± 0.92 ha, and are embedded in a matrix of secondary forests, active pastures and forest remnants. Sites were >100 m from primary forest remnants with an average distance of 2.8 ± 1.3 km (range 1.1–4.8 km) from continuous primary forest.
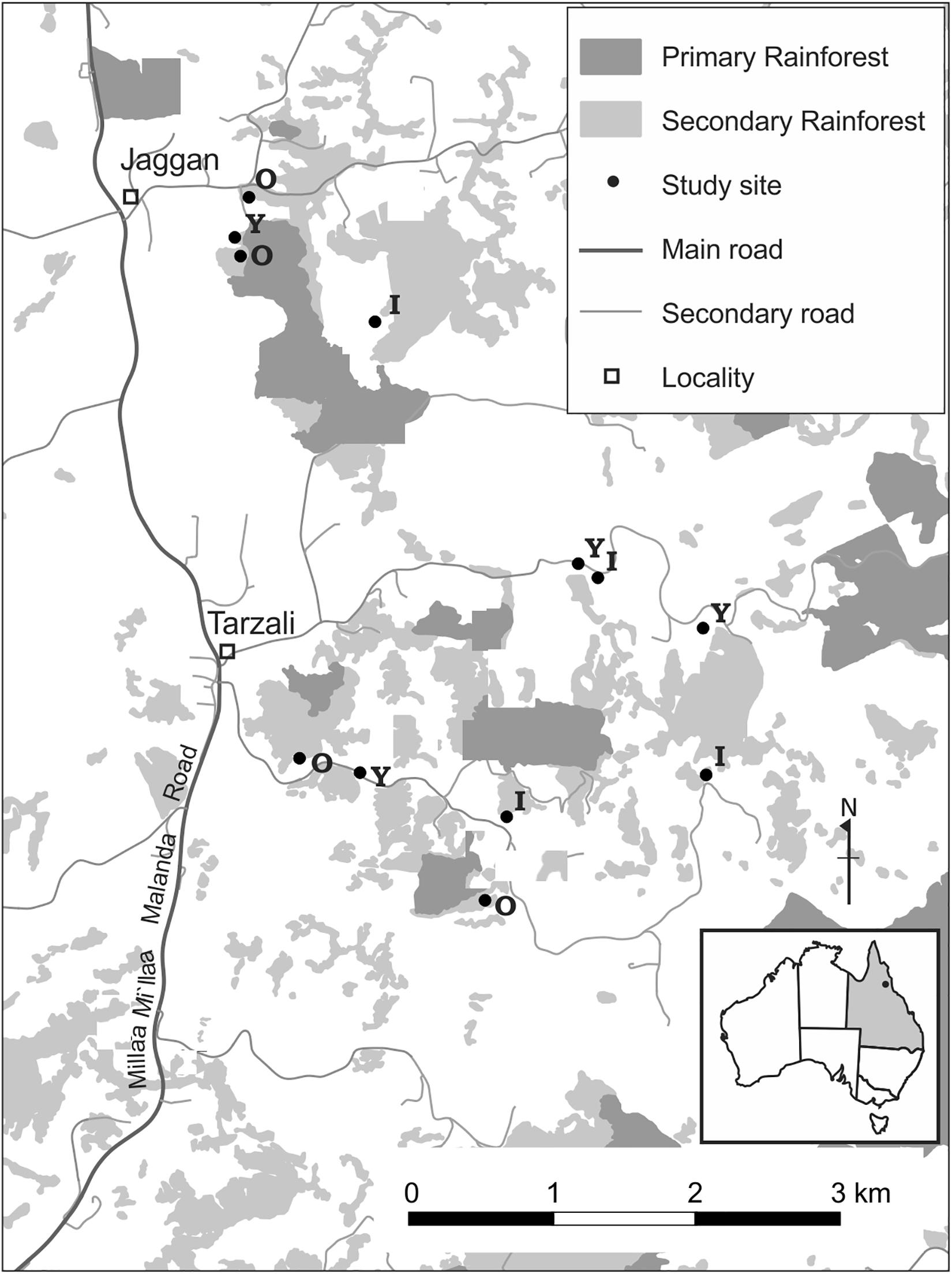
Figure 1. Map of the study area, southern Atherton Tableland, North Queensland, Australia. Letters represent the age of secondary sites. Y, Young secondary forests (4–12 years); I, Intermediate-aged (16–20 years); O, Old (23–34 years).
The study sites are located on soils derived from the two dominant soil types in the region: granite (Tully Granite) and basalt (Atherton Basalt). In our study area, basalt soils have higher clay content, total N and total P compared to sites in granite. Nonetheless, clay content, total N and total P are unrelated to soil pH and soil C:N and soil acidity is not strongly associated with nutrient concentration (Pandolfo Paz et al., 2016).
Chronosequence studies have been criticized because calculating age since abandonment and determining previous land uses are major challenges (Chazdon, 2014), and their explanatory power may depend on the scale of study design (Chazdon, 2008; Lebrija-Trejos et al., 2008; Dupuy et al., 2012). We have addressed these challenges through extensive aerial photography interpretation, replication of independent sites that are within the same climatic region and share similar past land use. Additionally, we used age since canopy formation instead of age since abandonment as a proxy of forest age to limit variation between the initial conditions of different sites.
Site Ages and Categories
We selected our studies sites using a range of Queensland State Government aerial photography, satellite images from Google Earth (©2014 Google Image, ©2014 DigitalGlobe), and imagery from Queensland Globe (©State of Queensland 2013, ©CNES 2012, Spot Image S.A. France, ©2013 Pitney Bowes), detailed methods in Goosem et al. (2016). Secondary forests were classified into three age categories using the number of years since canopy formation as a proxy of forest age: Young: forests of 4–12 years; Intermediate: forests of 16–20 years; and Old: forests of 23–34 years. We used four sites for each age category.
Species Selection
Six rainforest tree species were selected, based on previous information regarding the species composition of secondary and mature forest sites in this region (Goosem et al., 2016; Palma et al., in review). For the direct seeding experiments, we chose three large-seeded (> 20 mm in length) rainforest tree species (Beilschmiedia tooram, Cryptocarya oblata, and Endiandra bessaphila), which were absent from our regenerating forest but present in mature forest sites in the region; and two small-seeded (<15 mm in length) rainforest tree species (Litsea leefeana and Guioa lasioneura) that are present in both secondary and mature forest sites. For the planting experiment an additional small-seeded late successional species (Synima cordierorum) was included. This species is present in both secondary and mature forest sites in the region, but was unavailable as seed for the seed experiment. We collected seeds of C. oblata, E. bessaphila, L. leefeana, and G. lasioneura from at least four rainforest remnants and parental trees scattered within the region and purchased seeds of B. tooram and seedlings of S. cordierorum from local nurseries.
Seed Experiments
We established the seed experiments during the wet season (November 2013–February 2014). After collection, seeds were soaked in water for 48 h, the pulp removed and placed in the secondary forests within 60–72 h. The three seed treatments included: (1) exposed seeds (litter removed and seeds placed on the soil); (2) fenced seeds (litter removed, seeds placed on the soil within a plastic fence, 50 cm in height; and (3) buried seeds (litter removed, seeds sown to a depth of 2 cm). We used five species and 20 seeds per treatment per site for a total of 3,600 seeds. In each site, we established a seed experimental plot (3 m × 2.5 m) divided into 15 (1 m × 0.5 m) quadrats, randomly allocating one of the three different direct seeding treatments and species to each quadrat.
Seedling Experiments
All seedlings were germinated and grown for 1–3 months in a greenhouse, with 1 month of “hardening” outdoors with no extra watering (except S. cordierorum, bought at 8 months of age). Seedlings were planted during the wet season (March of 2014) at the same sites used for the direct seeding experiments. We planted six species and 30 seedlings per species per site (except C. oblata, 24 seedlings per site) for a total of 2,088 seedlings. Species were randomly allocated into 6 quadrats (3 m × 3 m) within an experimental planting plot (9 m × 6 m) at each site.
Data Collection and Analysis
Seed Experiments
All seeds were monitored weekly during the initial 5–10 weeks after placement in the secondary forest sites. During each census, we recorded seed number, status (five categories: eaten, removed, desiccated, rotten, germinated) and height of each uniquely tagged germinated seedling. After week 10, seeds were monitored once a month for 3 months and then every 3 months until April 2015. We calculated the relative growth rate of seedlings (RGR) in mm per day from the difference between initial and final heights of each seedling divided by the growth period [(ln (height final)−ln (height initial)/(growth period in days)]. The total height of each seedling was measured from the ground to the most distant part of the main stem.
Seedling Experiments
Planted seedlings were monitored 2 weeks after planting and all individual seedlings tagged with a unique number. Subsequently, seedlings were monitored every 3 months for 13 months. In each census, we recorded for each seedling status (four categories: eaten, removed, dry, dead) and height. We calculated relative growth rate (RGR) using the same method described above.
Statistical Analyses
We assessed the effects of secondary forest age and planting method (seed or seedling) and their interactions on survival, growth and species identity using nested analysis of variance models (ANOVA), with site nested within forest age. Our data met the assumptions of the nested ANOVA. Tukey’s tests were used for post hoc analysis. We analyzed survivorship for both experiments using the “survival” (Therneau, 2020) and “survminer” (Kassambra et al., 2019) packages and pairwise comparisons using Log-Rank Test (p-value adjustment method for multiple comparisons: BH). Analyses were performed using R (R Development Core Team, Version 1.1.1335) and IBM SPSS Statistics Version 21.
Results
Seed Experiments: Survival and Growth
Seeds that survived, were those seeds that germinated, grew into seedlings and were alive at our last census. We transplanted a total of 3,600 seeds, out of these 872 germinated (24.2%) and by the end of the experiment only 516 (14.3%) survived (i.e., remained as seedlings). Seed survival was very low in young forests compared to intermediate and old secondary forest sites (Figure 2A), with significant differences between young and intermediate forests (p < 0.0001) and between young and old forest sites (p < 0.0001). Seeding treatments also had a significant effect on seed survival (Figure 2B), with the greatest survival rates recorded when seeds were buried compared to both exposed and fence treatments (p < 0.0001 for both comparisons).
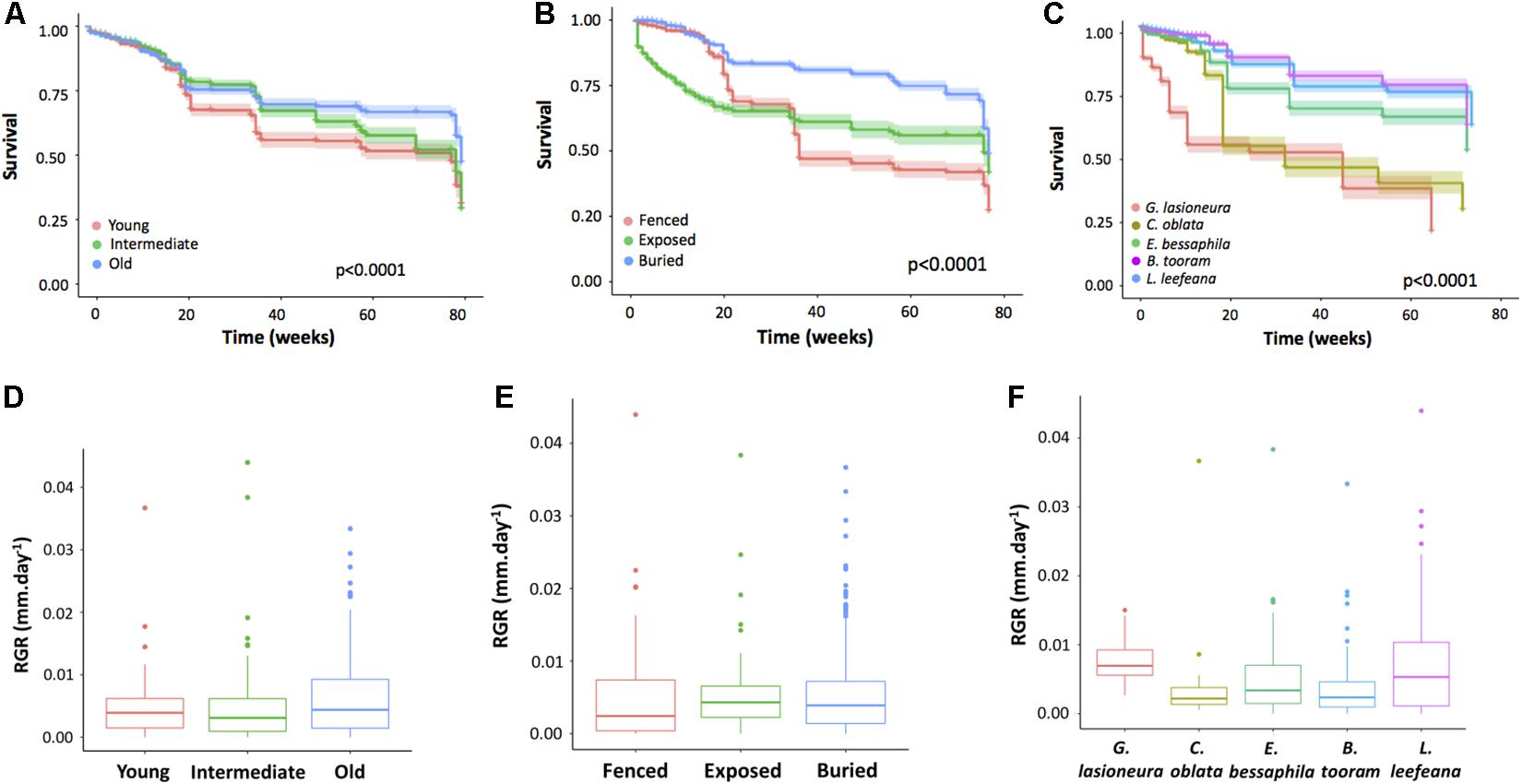
Figure 2. Survival and growth of seeding experiments in three secondary forest ages. (A) Secondary forest age had a significant effect on seed survival, with younger forests loosing significantly more seeds than old and intermediate forests (p < 0.0001 for both comparisons). (B) Direct seeding treatment affected seed survival, buried seeds had highest survival than both exposed and fenced seeds (p < 0.0001 for both comparisons). (C) Species identity was important for seed survival, B. tooram and L. leefeana had the highest survival and both differed significantly from C. oblata and G. lasioneura (p < 0.0001 for all comparisons); seeds of L. leefeana also survived better than seeds of E. bessaphila (p = 0.029). (D) Secondary forest age did not affect the growth of germinated seeds [F(2, 17) = 1.49, p = 0.25]. (E) Seeding treatment did not affect growth either [F(2, 23) = 0.12, p = 0.88]. (F) Species identity was important [F(4, 23) = 6.69, p = 0.001], with highest growth in G. lasioneura and L. leefeana; G. lasioneura grew more than B. tooram (p = 0.013) and L. leefeana showed higher growth when compared to B. tooram, C. oblata, and E. bessaphila (p < 0.0001; p = 0.006, and p = 0.015, respectively). For all box plot graphs, the tick line represents the median, the outer limits of the box the first and third quartiles. Whiskers extend to cover any data point <1.5 times the interquartile range. Circles represent outliers.
Species identity was also important for seed survival (Figure 2C). The highest survival was recorded for B. tooram and L. leefeana both of which differed significantly from all other species (p < 0.0001). We found interaction effects between secondary forest age, direct seeding method, and species identity on seed survival [F(16, 126) = 1.82, p = 0.034]. Overall, seed survival was greater in old secondary forests than in young forest for all species and treatments. Survival for B. tooram, E. bessaphila and L. leefeana was greater when seeds were buried in old secondary forests (43.75 ± 27.51, 33.75 ± 16.52, and 53.75 ± 19.31, respectively), whilst C. oblata and G. lasioneura were more successful if sown in intermediate-aged forests (12.5 ± 15.54 and 8.75 ± 6.29, respectively). We did not find a clear pattern relating to functional traits such as seed size or successional status.
Growth of germinated seeds was not significantly affected by secondary forest age, or direct seeding method [F(2, 17) = 1.49, p = 0.25, and F(2, 23) = 0.12, p = 0.88, respectively, Figures 2D,E]. Species identity was important [F(4, 23) = 6.69, p = 0.001], with highest growth in G. lasioneura and L. leefeana. G. lasioneura grew significantly more than B. tooram (p = 0.013) and L. leefeana grew more than B. tooram, C. oblata and E. bessaphila (p < 0.0001; p = 0.006, and p = 0.015, respectively, Figure 2F). We did not find interaction effects between secondary forest age, direct seeding method, and species identity on growth of the germinated seeds [F(2, 23) = 0.57, p = 0.57].
Across all age categories and treatments (Table 1) the highest loss of seeds was due to seed removal (seeds that were no longer found, average: 65.1%); followed by seeds that were rotten, eaten, desiccated and finally seeds with visible fungal damage. For the germinated seedlings, the highest cause of death was also removal (seedlings that were no longer found, average: 63.1%), followed by seedlings that were desiccated, showed visible fungi damage, suffered from stem breakage, showed clear signs of herbivory, and finally unearthed seedlings. Out of the 3,600 seeds only 872 germinated and at the time of our last census only 516 had established as seedlings (14.3%).
Seedling Experiments: Survival and Growth
Secondary forest age had a significant effect on the survival of transplanted seedlings (Figure 3A). Survivorship was significantly higher in old forest compared to young and intermediate secondary forests (p < 0.0001 for both comparisons). Species identity also influenced seedling survival (Figure 3B), with S. cordierorum showing the highest survivorship and surviving better than all other species (p < 0.0001, for all comparisons). Seedlings of G. lasioneura had the lowest survivorship and differed from all other species (p < 0.0001, for all comparisons). We did not find interaction effects between secondary forest age and species identity on seedling survival [F(10, 45) = 1.13, p = 0.35].
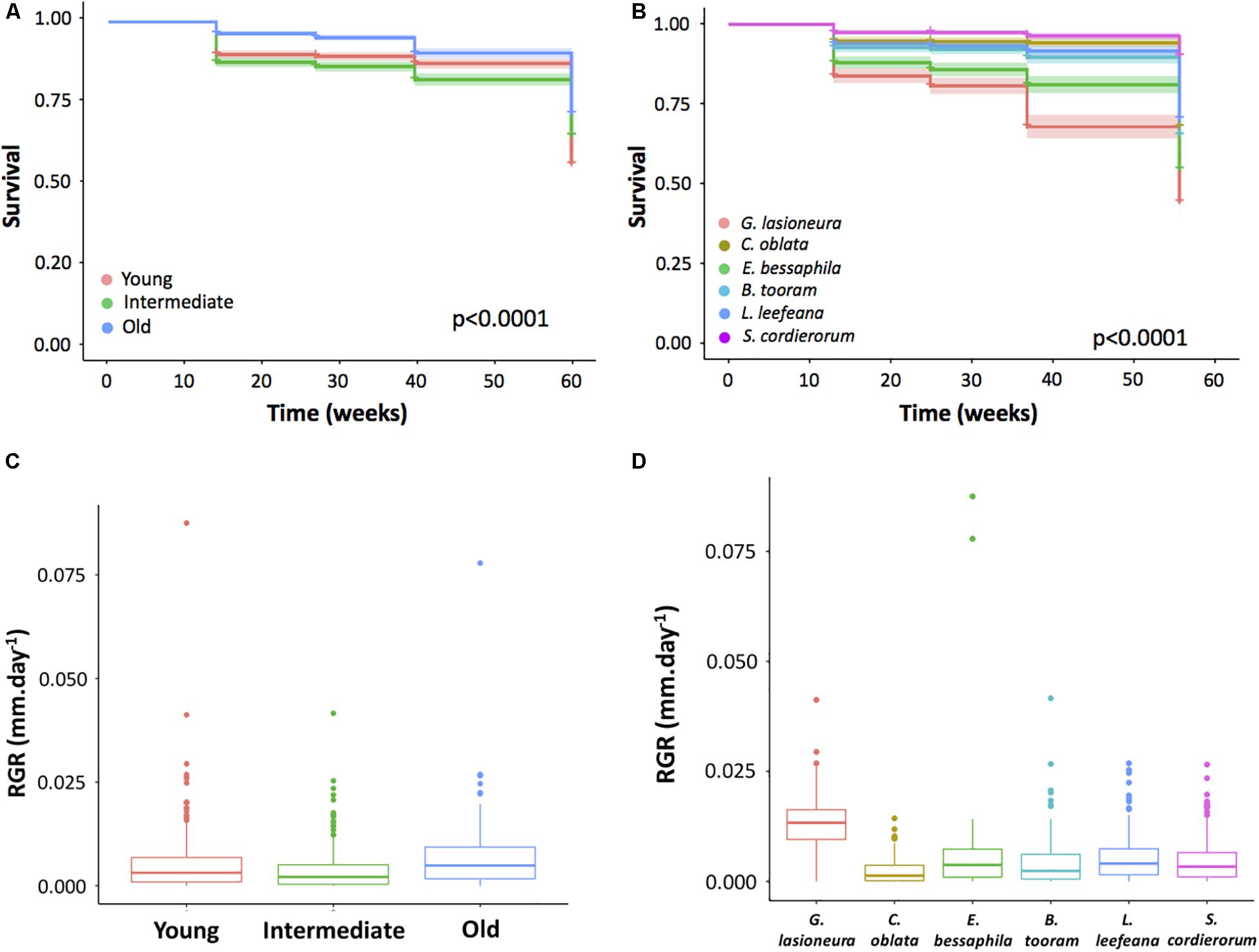
Figure 3. Survival and growth of planted seedlings in secondary forest of three different ages. (A) Secondary forest age had a significant effect on seedling survival, seedlings planted in old forests had the greatest survival. (B) Species identity influenced seedling survival, S. cordierorum had the highest survival, differing from B. tooram, E. bessaphila, and G. lasioneura (p < 0.0001 for all comparisons). Seedlings of G. lasioneura had the lowest survivorship and differed from all other species. (C) Secondary forest age did not influence seedlings growth [F(2, 13) = 2.32, p = 0.13]. (D) Species identity had a significant effect on seedlings growth [F(5, 35) = 17.51, p < 0.0001] with G. lasioneura growing more than all other species (p < 0.0001 for all comparisons. C. oblata showed the lowest growth rate and differed from E. bessaphila, L. leefeana, and S. cordierorum (p < 0.001, p = 0.005, and p = 0.026, respectively). For all box plot graphs, the tick line represents the median, the outer limits of the box the first and third quartiles. Whiskers extend to cover any data point <1.5 times the interquartile range. Circles represent outliers.
Secondary forest age did not influence growth in the planted seedlings [F(2, 13) = 2.32, p = 0.13, Figure 3C]. Species identity had a significant effect on seedlings growth [F(5, 35) = 17.51, p < 0.0001] with G. lasioneura growing more than all other species (p < 0.0001 for all comparisons, Figure 3D). In contrast, C. oblata showed the lowest growth rate and differed significantly from E. bessaphila, L. leefeana, and S. cordierorum (p = 0.00, p = 0.005, and p = 0.026, respectively). We did not find interaction effects between secondary forest age and species identity on seedling growth [F(10, 35) = 1.68, p = 0.12]. We did not find a clear pattern relating to functional traits such as seed size or successional status.
Across all age categories, removed seedlings (seedlings that were no longer found) accounted for the highest amount of mortality (71.69%), followed by desiccation, signs of herbivory, unearthed seedlings, and finally broken stems (Table 1).
Discussion
Along a chronosequence of secondary forests, seed and seedling experiments provided important insight into processes that may delay forest succession and recovery. We found that seeds from all study plant species germinated in all sites from our secondary forest chronosequence, regardless of forest age. This result highlights the importance of seed availability in these recovering forests.
We found that seeds of three late successional species of mature rainforest, that were naturally absent from secondary forest germinated and grew when placed in these recovering forests. These results provide evidence that the absence of these species is due in part to lack or limited seed supply of large-seeded species in this highly fragmented landscape. Additionally, we observed that introduced seeds and seedlings suffered from very high removal rates which we assume is due to predation or herbivory, and demonstrates the constraints to establishment in tropical forests.
Our results show that species identity was as important as forest age for growth and survival, but we did not find a clear pattern relating to functional traits such as seed size or successional status. For example, L. leefeana and B. tooram showed the greatest survival for the direct seeding experiment. L. leefeana is a small-seeded early successional species that is common in all sites, whereas B. tooram is a large-seeded late successional species rarely encountered in secondary forests. Alternatively, in the same experiment survival was lowest for C. oblata, a big-seeded late successional species and G. lasioneura a small-seeded early successional species. Although our sample size is small and multiple mechanisms limit recruitment, our data shows that seed size might not be enough to predict seed predation or establishment. Species with low dispersal limitation, generally small seeds, may have high recruitment limitations (de la Peña-Domene et al., 2018).
When planted as seedlings, S. cordierorum, a small-seeded late successional species survived the best. However, these seedlings were bought from a local nursery and they were 8 months older than all other seedlings. It is possible that S. cordierorum might actually survive better at these sites, or that their higher survival might just be an artifact of their greater age increasing their resilience (Vandendriessche, 1992; Herman et al., 2012; Palma and Laurance, 2015). Since over 20% of dead seedlings showed signs of desiccation, these older seedlings may have had a greater advantage establishing in these secondary forests. Since small seeds can be drought tolerant (dormant for longer) (Westoby et al., 1992), but small-seeded seedlings are vulnerable to drought because they lack reserves from the large cotyledons (Westoby et al., 1992; Harrison and LaForgia, 2019), using older seedlings from small-seeded species may be an alternative to overcome these limitations.
The small-seeded, early successional species G. lasioneura and L. leefeana showed the highest growth in seed and seedling experiments. These species are very common in these secondary forests and such fast growth is a typical characteristic of early successional species (Finegan, 1996), while large-seeded, late successional species such as B. tooram and C. oblata have relatively slower growth rates. Large seed size might not confer an advantage in the rate of seedling growth yet it has been demonstrated that large-seeded species can resprout more often after plant loss compared to small-seeded species (Green and Juniper, 2004).
Buried seeds had the greatest survivorship (average: 27.25%) with a three to fourfold higher persistence than exposed (6.0%) and fenced (9.75%) seeds. Burial appears to protect seeds from predators, desiccation, heavy rains or movement of leaf-litter by foraging wildlife which may be important to increase germination in these forests. We assumed that removed seeds were eaten or damaged and did not germinate, but it is possible that they were cached by small mammals such as white-tailed rats (Harrington et al., 1997). Overall, the direct seeding experiments showed a ca. 14% survival of seeds which is a very similar result to other direct seeding experiments in different ecosystems which average an 18% survival (Palma and Laurance, 2015).
Planting experiments provided further insight into recruitment limitations. We found the highest survival of transplanted seedlings in old secondary forest sites (average: 63.08%) where fewer grasses and exotic species may favor establishment (Chazdon, 2014). As with the direct seeding experiment, the main cause of mortality was seedling removal. These removed seedlings could have been eaten whole or unearthed and then washed away during rainstorms. Desiccation was the next most common cause of mortality. Droughts and desiccation are a commonly cited cause of death in planting experiments (Palma and Laurance, 2015).
Previous studies on seed germination and seedling survival of species planted into secondary forests have also found that species identity is more important for survival than variations in habitat (Camargo et al., 2002; Cole et al., 2011). However, early growth and survival are not always an indicator of the species persistence in the long-term (Benitez-Malvido and Martinez-Ramos, 2013). Extended monitoring of these experiments could provide more information on the success of each species and whether their relative success in establishment and growth changes over time and in response to changing biotic and abiotic environments (e.g., increased competition and decreasing light).
Our study demonstrated that some of the missing plant species in secondary forests of tropical Australia will germinate and grow when they are introduced as seeds and/or seedlings. With seed removal rates so high, direct seeding without burial is not recommended. Sowing seeds might be an effective way to reintroduce much of the plant diversity that is currently missing from secondary forests while avoiding potential seed predation by rodents (e.g., white-tailed rat, and the fawn-footed melomys), possums (i.e., common brushtail possum) and birds (i.e., common emerald dove and the Australian brush turkey) (Osunkoya, 1994; Doust, 2011). Planting of seedlings yields better results and older seedlings are more successful, however with the higher costs involved (e.g., greenhouses, transportation, and more labor) this approach should be used for highly isolated sites, for sites with high conservation value such as wildlife corridors (Tucker, 2000), with arrested succession or for the expansion of rare habitats and connectivity between fragments.
Restoration efforts are important to accelerate biodiversity gains in isolated sites, or to reintroduce endangered plant species. However, we need to keep in mind that even if restoration efforts are successful, 25 years after restoration plantings key features of mature rainforest are still lacking (Shoo et al., 2015). Although dispersal limitation lessens over the first years of recovery, with seed rain including more species, mature forest species continue to be limited (Reid et al., 2015), stressing the importance of actively introducing these species when possible.
In our experiments, forest age had a significant effect on seeds and seedling survival, with old secondary forest showing higher survival rates, nevertheless seeds and seedlings of all species were present at all sites until the end of the study. This shows that once succession has started and some canopy has formed, reintroduction of different species to accelerate forest recovery can be successful even in young secondary forests. Since species identity was important, testing different species at small scales before embarking in large-scale restoration projects is necessary. Hence we recommend, direct seeding experiments and planting of seedlings as an effective way to assess recruitment limitations in different habitats and for different species.
Our results highlight the significance of establishment limitations for the recovery of tropical secondary forests. We found that all the species included in the study germinated and grew even in young secondary forest sites. This shows that establishment limitation is not a major barrier for the recovery of these secondary forests, but that seed availability might be the biggest obstacle to forest recovery in this region. Our study sites are small in size (average 2.6 ha), embedded in an agricultural matrix and relatively far from seed sources (average distance to continuous forest: 2.8 Km). Enrichment planting in sites with similar conditions may accelerate forest recovery by providing food sources for seed dispersers and improving habitat conditions.
Planting clusters of different species has been a successful technique that enhances seed rain and seedling establishment with lower costs than tree plantations (Zahawi and Augspurger, 2006; Cole et al., 2010; Zahawi et al., 2013). These small clusters can also be used to plant different species, include a broader range of functional traits and test their resilience to desiccation or heavy rains. These types of experiments are important as they may help us to plan restoration efforts for future climatic scenarios (Palma and Laurance, 2015).
Data Availability Statement
The raw data supporting the conclusions of this article will be made available by the authors, without undue reservation.
Author Contributions
AP developed the idea, conducted fieldwork, analyzed the data, and wrote the manuscript. MG helped with fieldwork and editing the manuscript. PS helped editing the document and providing ecological advice. SL helped with fieldwork, developing the idea, writing the manuscript, and statistical advice. All authors contributed to the article and approved the submitted version.
Funding
Fellowships and field support were provided by the Colciencias (Colombian Department for the Administration of Science, Technology and Innovation) grant No. 529 awarded to AP; Wet Tropics Management Authority Student Research Grant Scheme awarded to AP; and the ARC Linkage Grant LP110201093 awarded to SL from the Australian Research Council and Queensland Herbarium in collaboration with the in-kind support from Wet Tropics Management Authority and Biome5 Pty Ltd.
Conflict of Interest
The authors declare that this study received funding from Biome5 Pty Ltd. The funder was not involved in the study design, collection, analysis, interpretation of data, the writing of this article or the decision to submit it for publication.
Acknowledgments
This contribution is part of the first author’s Doctoral thesis undertaken at James Cook University, with fellowships and field support provided by Colciencias (Colombian Department for the Administration of Science, Technology and Innovation), Wet Tropics Management Authority Student Research Grant Scheme awarded to ACP and the ARC Linkage Grant LP110201093 awarded to SGWL from the Australian Research Council and Queensland Herbarium in collaboration with the in kind support from Wet Tropics Management Authority and Biome5 Pty Ltd. We would like to thank Stephen McKenna for his assistance collecting seeds, and M. Salas, M. Cazar, and A. Cheesman for their help in setting up the planting experiments. Numerous volunteers helped in the greenhouse and the field, making this study possible. We also thank the landholders and owners of the different properties for granting access and allowing continuous fieldwork. We thank D. Apgaua and D. Tng for their statistical advice. The content of this manuscript has been published partially as part of the doctoral thesis of AP (Palma, 2016).
References
Benitez-Malvido, J., and Martinez-Ramos, M. (2013). Long-term performance and herbivory of tree seedlings planted into primary and secondary forests of Central Amazonia. J. Trop. Ecol. 29, 301–311. doi: 10.1017/s026646741300031x
Bentos, T. V., Nascimento, H. E. M., and Williamson, G. B. (2013). Tree seedling recruitment in Amazon secondary forest: importance of topography and gap micro-site conditions. For. Ecol. Manag. 287, 140–146. doi: 10.1016/j.foreco.2012.09.016
Camargo, J. L. C., Ferraz, I. D. K., and Imakawa, A. M. (2002). Rehabilitation of degraded areas of central Amazonia using direct sowing of forest tree seeds. Restor. Ecol. 10, 636–644. doi: 10.1046/j.1526-100X.2002.01044.x
Carrasco-Carballido, V., Martínez-Garza, C., Jiménez-Hernández, H., Márquez-Torres, F., and Campo, J. (2019). Effects of initial soil properties on three-year performance of six tree species in tropical dry forest restoration plantings forests. Biol. Open 10:428. doi: 10.3390/f10050428
Chazdon, R. (2014). Second Growth, the Promise of Tropical Forest Regeneration in an Age of Deforestation. Chicago: The University of Chicago Press.
Chazdon, R. L. (2008). Beyond deforestation: restoring forests and ecosystem services on degraded lands. Science 320, 1458–1460. doi: 10.1126/science.1155365
Clark, C. J., Poulsen, J. R., and Levey, D. J. (2013). Roles of seed and establishment limitation in determining patterns of afrotropical tree recruitment. PLoS One 8:e63330. doi: 10.1371/journal.pone.0063330
Cole, R. J., Holl, K. D., Keene, C. L., and Zahawi, R. A. (2011). Direct seeding of late-successional trees to restore tropical montane forest. For. Ecol. Manag. 261, 1590–1597. doi: 10.1016/j.foreco.2010.06.038
Cole, R. J., Holl, K. D., and Zahawi, R. A. (2010). Seed rain under tree islands planted to restore degraded lands in a tropical agricultural landscape. Ecol. Appl. 20, 1255–1269. doi: 10.1890/09-0714.1
Connell, J. H., and Slatyer, R. O. (1977). Mechanisms of succession in natural communities and their role in community stability and organization. Am. Naturalist 111, 1119–1144. doi: 10.1086/283241
Coomes, D., and Grubb, P. J. (2003). Colonization, tolerance, competition and seed-size variation within functional groups. Trends Ecol. Evol. 18, 283–291. doi: 10.1016/S0169-5347(03)00072-7
de la Peña-Domene, M., and Martínez-Garza, C. (2018). Integrating density into dispersal and establishment limitation equations in tropical forests. Forests 9:570. doi: 10.3390/f9090570
de la Peña-Domene, M., Martínez-Garza, C., Ayestarán-Hernández, L. M., and Howe, H. F. (2018). Plant attributes that drive dispersal and establishment limitation in tropical agricultural landscapes. Forests 9:620. doi: 10.3390/f9100620
Dent, D. H., DeWalt, S. J., and Denslow, J. S. (2013). Secondary forests of central Panama increase in similarity to old-growth forest over time in shade tolerance but not species composition. J. Veg. Sci. 24, 530–542. doi: 10.1111/j.1654-1103.2012.01482.x
Doust, S. J. (2011). Seed removal and predation as factors affecting seed availability of tree species in degraded habitats and restoration plantings in rainforest areas of queensland, Australia. Restorat. Ecol. 19, 617–626. doi: 10.1111/j.1526-100x.2010.00681.x
Dupuy, J. M., Hernández-Stefanoni, J. L., Hernández-Juárez, R. A., Tetetla-Rangel, E., López-Martínez, J. O., Leyequién-Abarca, E., et al. (2012). Patterns and correlates of tropical dry forest structure and composition in a highly replicated chronosequence in Yucatan, Mexico. Biotropica 44, 151–162. doi: 10.1111/j.1744-7429.2011.00783.x
Egler, F. E. (1954). Vegetation science concepts I. Initial floristic composition, a factor in old-field vegetation development with 2 figs. Veg. Acta Geob. 4, 412–417. doi: 10.1007/BF00275587
Faria, D., Mariano-Neto, E., Martini, A. M., Ortiz, J. V., Montingelli, R., Rosso, S., et al. (2009). Forest structure in a mosaic of rainforest sites: the effect of fragmentation and recovery after clear cut. For. Ecol. Manag. 257, 2226–2234. doi: 10.1016/j.foreco.2009.02.032
Finegan, B. (1996). Pattern and process in neotropical secondary rain forests: the first 100 years of succession. Trends Ecol. Evol. 11, 119–124. doi: 10.1016/0169-5347(96)81090-1
Frawley, K. J. (1987). The Maalan Group Settlement, North Queensland, 1954: An Historical Geography. Canberra: The University of New South Wales, Australian Defence Force Academy.
Gilmore, M. A. (2005). Kill, Cure or Strangle: The History of Government Intervention in Three Agricultural Industries on the Atherton Tablelands, 1895- 2005. Ph. D thesis, James Cook University, Cairns.
González-Tokman, D. M., Barradas, V. L., Boege, K., Domínguez, C. A., del-Val, E., Saucedo, E., et al. (2018). Performance of 11 tree species under different management treatments in restoration plantings in a tropical dry forest. Restorat. Ecol. 26, 642–649. doi: 10.1111/rec.12617
González-Tokman, D., Cultid-Medina, C., Díaz, A., Escobar, F., Ocampo-Palacio, L., and Martínez-Garza, C. (2018). Success or failure: the role of ecological restoration on the recovery of dung beetle diversity and function in a tropical rainforest. Rev. Mex. Biodiver. 89, 232–242.
Goosem, M., Paz, C., Fensham, R., Preece, N., Goosem, S., and Laurance, S. G. W. (2016). Forest age and isolation affect the rate of recovery of plant species diversity and community composition in secondary rain forest in tropical Australia. J. Veg. Sci. 27, 504–514. doi: 10.1111/jvs.12376
Green, P. T., and Juniper, P. A. (2004). Seed mass, seedling herbivory and reserve effects in tropical rainforest seedlings. Funct. Ecol. 18, 539–547. doi: 10.1111/j.0269-8463.2004.00881.x
Guariguata, M. R., and Ostertag, R. (2001). Neotropical secondary forest succession: changes in structural and functional characteristics. For. Ecol. Manag. 148, 185–206. doi: 10.1016/s0378-1127(00)00535-1
Harrington, G. N., Irvine, A. K., Crome, F. H. J., and Moore, L. A. (1997). “Regeneration of large-seeded trees in Australian rainforest fragments: a study of higher order interactions,” in Tropical Forest Remnants. Ecology, Management, and Conservation of Fragmented Communities, eds W. F. Laurance and R. O. Bierregaard (Chicago: University of Chicago Press), 292–303.
Harrison, S., and LaForgia, M. (2019). Seedling traits predict drought-induced mortality linked to diversity loss. Proc. Natl. Acad. Sci. U.S.A. 116, 5576–5581. doi: 10.1073/pnas.1818543116
Herman, J. J., Sultan, S. E., Horgan-Kobelski, T., and Riggs, C. (2012). Adaptive transgenerational plasticity in an annual plant: grandparental and parental drought stress enhance performance of seedlings in dry soil. Integr. Comp. Biol. 52, 77–88. doi: 10.1093/icb/ics041
Hubbell, S. P., Foster, R. B., O’Brien, S. T., Harms, K. E., Condit, R., and Wechsler, B. (1999). Light-gap disturbances, recruitment limitation, and tree diversity in a neotropical forest. Science 283, 554–557. doi: 10.1126/science.283.5401.554
Kassambra, A., Kosinski, M., and Biecek, P. (2019). Survminer: Drawing Survival Curves Using ‘ggplot2’. R Package Version 0.4.5. Available online at: https://cran.r-project.org/web/packages/survminer (accessed May, 2020). doi: 10.1126/science.283.5401.554
Lebrija-Trejos, E., Bongers, F., Perez-Garcia, E. A., and Meave, J. A. (2008). Successional change and resilience of a very dry tropical deciduous forest following shifting agriculture. Biotropica 40, 422–431. doi: 10.1111/j.1744-7429.2008.00398.x
Meiners, S. J., Cadotte, M. W., Fridley, J. D., Pickett, S. T. A., and Walker, L. R. (2015). Is successional research nearing its climax? New approaches for understanding dynamic communities. Funct. Zecol. 29, 154–164. doi: 10.1111/1365-2435.12391
Moles, A. T., and Westoby, M. (2002). Seed addition experiments are more likely to increase recruitment in larger-seeded species. Oikos 99, 241–248. doi: 10.1034/j.1600-0706.2002.990204.x
Moles, A. T., and Westoby, M. (2004). Seedling survival and seed size: a synthesis of the literature. J. Ecol. 92, 372–383. doi: 10.1111/j.0022-0477.2004.00884.x
Muller-Landau, H. (2010). The tolerance-fecundity trade-off and the maintenance of diversity in seed size. Proc. Natl. Acad. Sci. U.S.A. 107, 4242–4247. doi: 10.1073/pnas.0911637107
Muller-Landau, H. C., Wright, S. J., Calderon, O., Hubbell, S. P., and Foster, R. B. (2002). “Assessing recruitment limitation: concepts, methods and case-studies from a tropical forest,” in Seed Dispersal and Frugivory: Ecology, Evolution and Conservation, eds D. J. Levey, W. R. Silva, and M. Galleti (Wallingford: CAB International), 35–53. doi: 10.1079/9780851995250.0035
Norden, N., Chazdon, R. L., Chao, A., Jiang, Y. H., and Vilchez-Alvarado, B. (2009). Resilience of tropical rain forests: tree community reassembly in secondary forests. Ecol. Lett. 12, 385–394. doi: 10.1111/j.1461-0248.2009.01292.x
Osunkoya, O. O. (1994). Postdispersal survivorship of north Queensland rainforest seeds and fruit: effects of forest, habitat and species. Austr. J. Ecol. 19, 52–64. doi: 10.1111/j.1442-9993.1994.tb01543.x
Palma, A. C. (2016). Recruitment and Dispersal Limitations in a Chronosequence of Secondary Forests in Tropical Australia. Ph. D thesis, James Cook University, Townsville.
Palma, A. C., and Laurance, S. G. W. (2015). A review of the use of direct seeding and seedling plantings in restoration: what do we know and where should we go? Appl. Veg. Sci. 18, 561–568. doi: 10.1111/avsc.12173
Pandolfo Paz, C., Goosem, M., Bird, M., Preece, N., Goosem, S., Fensham, R., et al. (2016). Soil types influence predictions of soil carbon stock recovery in tropical secondary forests. For. Ecol. Manag. 376, 74–83. doi: 10.1016/j.foreco.2016.06.007
Pickett, S. L., Collins, J., and Armesto, J. (1987). Models, mechanisms and pathways of succession. Bot. Rev. 53, 335–371. doi: 10.1007/bf02858321
Pulsford, S. A., Lindenmayer, D. B., and Driscoll, D. A. (2016). A succession of theories: purging redundancy from disturbance theory. Biol. Rev. Camb. Philos. Soc. 91, 148–167. doi: 10.1111/brv.12163
Reid, J. L., Holl, K. D., and Zahawi, R. A. (2015). Seed dispersal limitations shift over time in tropical forest restoration. Ecol. Appl. 25, 1072–1082. doi: 10.1890/14-1399.1
Rozendaal, D. M. A., Bongers, F., Aide, T. M., Alvarez-Dávila, E., Ascarrunz, N., Balvanera, P., et al. (2019). Biodiversity recovery of Neotropical secondary forests. Sci. Adv. 5:eaau3114.
Rüger, N., Condit, R., Dent, D. H., DeWalt, S. J., Hubbell, S. P., Lichstein, J. W., et al. (2020). Demographic trade-offs predict tropical forest dynamics. Science 368, 165–168. doi: 10.1126/science.aaz4797
Schupp, E. W., Milleron, T., and Russo, S. E. (2002). “Dissemination limitation and the origin and maintanance of species-rich tropical forets,” in Seed Dispersal and Frugivory: Ecology, Evolution and Conservation, eds D. J. Levey, W. R. Silva, and M. Galleti (Wallingford: CAB International), 19–33. doi: 10.1079/9780851995250.0019
Shoo, L. P., Freebody, K., Kanowski, J., and Catterall, C. P. (2015). Slow recovery of tropical old-field rainforest regrowth and the value and limitations of active restoration. Conserv. Biol. 30, 121–132. doi: 10.1111/cobi.12606
Terborgh, J. (2012). Enemies maintain hyperdiverse tropical forests. Am. Nat. 179, 303–314. doi: 10.1086/664183
Terborgh, J., Alvarez-Loayza, P., Dexter, K., Cornejo, F., and Carrasco, C. (2011). Decomposing dispersal limitation: limits on fecundity or seed distribution? J. Ecol. 99, 935–944. doi: 10.1111/j.1365-2745.2011.01836.x
Tucker, N. I. J. (2000). Monitoring and evaluation at Donaghy’s wildlife corridor: Measures used and questions asked. Ecol. Manage. Restor. 1, 61–62.
van Breugel, M., Hall, J. S., Craven, D., Bailon, M., Hernandez, A., Abbene, M., et al. (2013). Succession of ephemeral secondary forests and their limited role for the conservation of floristic diversity in a human-modified tropical landscape. PLoS One 8:e082433. doi: 10.1371/journal.pone.0082433
Vandendriessche, R. (1992). Changes in drought resistance and root-growth capacity of container seedlings in response to nursery drought, nitrogen, and potassium treatments. Can. J. Forest Res. 22, 740–749. doi: 10.1139/x92-100
Vargas, I. N., and Stevenson, P. R. (2013). Seed and establishment limitation: effects on plant diversity in an amazonian rain forest. Biotropica 45, 737–746. doi: 10.1111/btp.12063
Westoby, M., Jurado, E., and Leishman, M. (1992). Comparative evolutionary ecology of seed size. Trends Ecol. Evol. 7, 368–372. doi: 10.1016/0169-5347(92)90006-w
Westoby, M., Leishman, M., and Lord, J. (1996). Comparative ecology of seed size and dispersal. Philos. Trans. R. Soc. Ser. B Biol. Sci. 315, 1309–1318. doi: 10.1098/rstb.1996.0114
Zahawi, R. A., and Augspurger, C. K. (2006). Tropical forest restoration: tree islands as recruitment foci in degraded lands of Honduras. Ecol. Appl. 16, 464–478. doi: 10.1890/1051-0761(2006)016[0464:tfrtia]2.0.co;2
Keywords: chronosequence, dispersal limitations, establishment, forest succession, seeding experiments, seedling plantings, secondary forest
Citation: Palma AC, Goosem M, Stevenson PR and Laurance SGW (2020) Enhancing Plant Diversity in Secondary Forests. Front. For. Glob. Change 3:571352. doi: 10.3389/ffgc.2020.571352
Received: 10 June 2020; Accepted: 12 October 2020;
Published: 03 November 2020.
Edited by:
Madelon Lohbeck, World Agroforestry Centre, KenyaReviewed by:
Marinés De La Peña-Domene, Western Institute of Technology and Higher Education, MexicoLucy Amissah, Council for Scientific and Industrial Research, Ghana
Copyright © 2020 Palma, Goosem, Stevenson and Laurance. This is an open-access article distributed under the terms of the Creative Commons Attribution License (CC BY). The use, distribution or reproduction in other forums is permitted, provided the original author(s) and the copyright owner(s) are credited and that the original publication in this journal is cited, in accordance with accepted academic practice. No use, distribution or reproduction is permitted which does not comply with these terms.
*Correspondence: Ana C. Palma, YW5hY3BhbG1hQGdtYWlsLmNvbQ==