- 1College of Forest Resources and Environmental Science, Michigan Technological University, Houghton, MI, United States
- 2Rocky Mountain Research Station, United States Department of Agriculture, U.S. Forest Service, Moscow, ID, United States
Severe wildfire can affect many soil processes, especially organic matter (OM) decomposition. Organic mulches are often applied on steep slopes to mitigate soil erosion, but little is known about how these surface organic additions affect subsequent soil OM decomposition. In 2003 the Cedar Fire burned 110,000 ha in southern California chaparral shrubland, after which hydromulch was aerially applied to reduce soil erosion. Subsequently, we established a 5-year study to assess the effect of hydromulch on OM decomposition at the burned soil surface and in the mineral soil using aspen (Populus tremuloides Michx.) and pine (Pinus taeda L.) wood stakes as standard substrates. Mass loss of both aspen and pine stakes in this dry Mediterranean ecosystem was lowest on the soil surface and increased with mineral soil depth. Decomposition was less in the hydromulched soil than in the untreated control, but the large loss of hydromulch from the soil surface within the first year after application make this result questionable. Subsequent analysis showed that subterranean termites had a major role in wood decomposition, but their variable activity in study replicates confounded the separation of hydromulch impacts on decomposition from other soil variables. Little is known on the role of termites in OM decomposition after wildfire, and they should be considered when designing studies in soils where termites are present. Our study results suggest that termite activity in mineral soil could also be a factor in root decomposition after a fire and affect soil stability on steep slopes.
Introduction
High-severity wildfires on steep terrain increase soil erosion and negatively impact water quality in adjacent streams and lakes (Robichaud, 2000; Spigel and Robichaud, 2007; Neary et al., 2012). In the post-fire landscape, the loss of surface organic matter (OM) and vegetation increases both dry season erosion (e.g., ravel) and wet season erosion (e.g., raindrop splash, sheetflow, and rilling; Rice, 1974; Geddes and Dunkerley, 1999; Li et al., 2014). Development of soil hydrophobicity after wildfire also can alter water-flow paths and increase surface water flow (Ritsema and Dekker, 1996). Consequently, property damage from debris flows and large erosive events is more common after wildfires in areas with steep slopes (Radeloff et al., 2001; Bautista et al., 2009; Bento-Gonçalves et al., 2012).
Such high-severity wildfires also remove large amounts of OM from the litter layer and surface mineral soil horizons (Doerr and Cerdà, 2005; Mataix-Solera et al., 2009, 2011), which cause many changes in soil chemical and physical properties (e.g., Baird et al., 1999; Gray and Dighton, 2006; Page-Dumroese and Jurgensen, 2006; Homann et al., 2011). However, these fires generally have short-term impacts on soil microbial populations but can alter microbial diversity and community structure that affect soil OM decomposition (e.g., Guerrero et al., 2005; Hebel et al., 2009; Page-Dumroese et al., 2019).
Organic mulches are often applied to the soil surface to reduce post-fire erosion by improving water-holding capacity and infiltration (Foltz and Wagenbrenner, 2010; Robichaud et al., 2013; Lucas-Borja et al., 2018a), and to help reestablish vegetation by lowering soil temperature, reducing surface water evaporation, and increasing soil OM content (Berryman et al., 2014a; Prats et al., 2016). Agricultural residues (e.g., wheat straw and hay) are often used, but they are susceptible to wind erosion and often contain seeds of non-native vegetation (Beyers, 2004; Bautista et al., 2009). Hydromulch, a combination of short organic fibers (e.g., wood strands, chips, and shreds), a tackifier, a suspension agent, and plant seeds, are also used on steep soils to reduce post-fire erosion and avoid some of the disadvantages of agricultural residues (Robichaud et al., 2013; Berryman et al., 2014b; Prats et al., 2016). The resulting organic-water slurry is wind-resistant and generally decomposes on the soil surface within a year of application, but some portion of the OM from the hydromulch moves into the surface mineral soil (Hubbert et al., 2012).
By adding OM and improving soil temperature and water holding conditions, organic mulches should increase soil microbial activity in burned soils, but mulching studies have given mixed results. Berryman et al. (2014a) found a positive, but variable, effect of different soil mulches on wood stake mass loss after a high-severity wildfire in Colorado. In contrast, Fontúrbel et al. (2012) and Lombao et al. (2013) reported that applying a straw mulch after a prescribed low-severity fire in Spain had no impact on soil microbial biomass, microbial diversity, and soil respiration rates.
In December 2003 a study was established by the United States Department of Agriculture (USDA) Forest Service on the Cleveland National Forest in southern California to investigate the impact of hydromulch applications on soil erosion, watershed sediment yields, and vegetative response after the high-severity Cedar Fire burned 110,000 hectares of chaparral shrublands (Wohlegemuth and Robichaud, 2007). In May 2004 we joined this study to assess the effect of the hydromulch treatment on soil microbial activity using trembling aspen (Populus tremuloides Michx.) and loblolly pine (Pinus taeda L.) wood stakes placed on the surface of the burned soil and inserted vertically to a mineral soil depth of 20 cm as an index of soil OM decomposition (mass loss). Wood is a normal soil component, and its slow decomposition rate integrates soil abiotic and biotic conditions over longer time periods (Harmon et al., 1986; Chen et al., 2005). These two tree species also have different wood properties (e.g., lignin type, lignin to cellulose ratio, and N content), which would favor the development of different wood-decomposing microbial communities (Blanchette, 1984).
However, as the study progressed, it became apparent that subterranean termites were an important invertebrate component in this soil. Termites are very efficient decomposers of dead wood (Sugimoto et al., 2000) and their impact on manmade structures has been well documented (e.g., Tsunoda, 2001; Kartal et al., 2013). Subterranean termites are found in a wide variety of United States soils and forest types (e.g., Wang and Powell, 2001; Bradford et al., 2014; Jurgensen et al., 2019) and are present in many areas of California (Haverty et al., 1999; Tripodi et al., 2006). However, the relatively few studies on termites in forest ecosystems have focused on wood decomposition at the soil surface (e.g., Ulyshen et al., 2014, 2016), and little is known on their activity in California chaparral or how they are impacted by wildfire. Therefore, our study protocols were changed so the effect of the surface hydromulch application on wood decomposition by both soil microorganisms and termites was assessed. This entailed separating all wood stakes collected from research plots into two groups for subsequent laboratory and statistical analyses based on the presence or absence of termite attack. Our study hypotheses are:
(1) Wood decomposition (mass loss) would be greater in hydromulched soil, as the surface mulch likely created more favorable soil conditions for both microbial and termite activity.
(2) Soil microorganisms are more important for wood decay at the soil surface than termites in this dry Mediterranean-type climate, but termites would dominate the decomposition process in the mineral soil.
Materials and Methods
Study Site
After the Cedar Fire burned over 110,000 ha in October 2003, a study was established on the Cleveland National Forest and Capitan Grande Indian Reservation ∼10 km from Alpine, California (Figure 1). This dry, Mediterranean-type site is located at 865 m on 18–20% west-facing slopes (32° 87′N, -116° 76′W), with a mean annual precipitation of 398 mm occurring primarily in the winter and occasional summer thunderstorms. Pre-fire overstory vegetation was chamise (Adenostoma fasciculatum Hook. & Arn.) and desert ceanothus (Ceanothus greggii A. Gray) growing on Cieneba loamy sand (loamy, mixed superactive, non-acid, and thermic shallow Typic Xerorthents). In December 2003, 2.0 Mg ha–1 (dry mulch weight) of a commercial hydromulch (wood and paper fiber, a non-water soluble tackifier, and water) was aerially applied to high fire-severity areas over the entire catchment (Robichaud et al., 2013). However, much of the hydromulch was lost from the soil surface during several large rainfall events that occurred the first year after application (Hubbert et al., 2012). For example, a large rainfall event was recorded in April 2004 at 29 mm h–1 totaling 60 mm of rain, and in October 2004 the site received approximately 160 mm of precipitation (Hubbert et al., 2012).
Wood Stakes
Two surface (2.5 cm × 2.5 cm × 15 cm) and two field mineral soil (2.5 cm × 2.5 cm × 20 cm) stakes were cut from a longer, kiln-dried, knot-free, sapwood stake of each tree species (Figure 2). The remaining 10 cm center section was used as a laboratory control (time = 0) to determine mass loss of the two surface and mineral soil stakes after they were sampled during the study. The top of each mineral soil stake was treated with a wood sealer to reduce moisture loss after installation. For additional details on wood stake properties and fabrication, see Jurgensen et al. (2006).
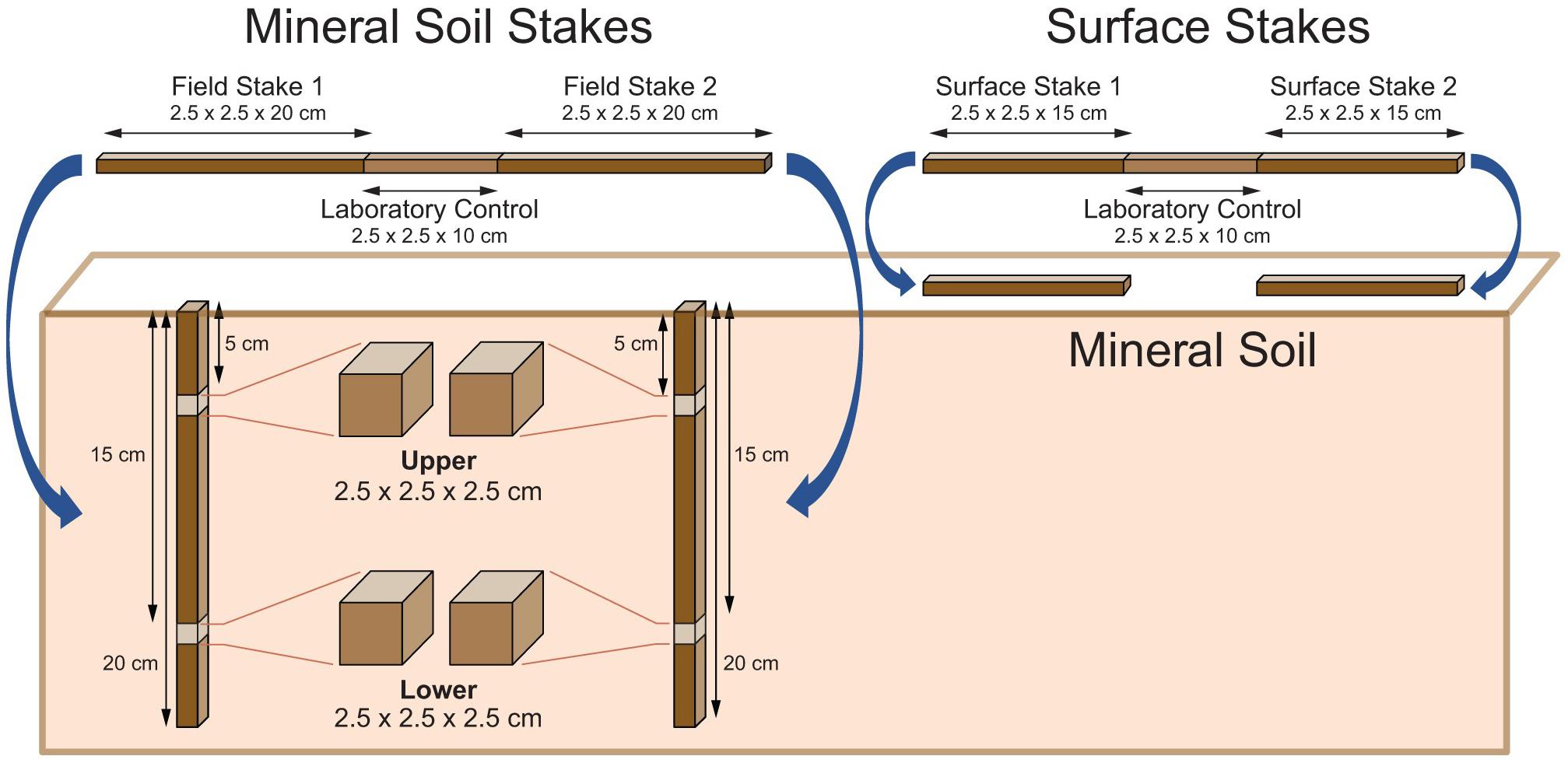
Figure 2. Fabrication, cutting, and placement of surface and mineral soil stakes used in the Cedar Fire study.
Experimental Design
Wildfires are difficult to assess because of their extent, intensity, and lack of replication. We wanted to include an unburned soil in our study to assess the effect of this high severity fire on OM decomposition, but all unburned sites were so distant from the hydromulch study location that their soil properties or physiographic conditions were too different to be used as an unburned control. However, pseudo-replication was avoided because wood stakes were randomly assigned to each treatment plot (Van Mantgem et al., 2001). Therefore, we established a 10 m × 10 m plot on three hydromulched soil areas and on unmulched soil areas adjacent to each mulched plot as controls. Since fire removed all of the litter layer, surface stakes (15 cm long) were placed horizontally on the mineral soil surface (Figure 2). Twenty-five aspen and 25 pine stakes were placed on the mineral soil surface of the hydromulch and control plot in replicate 1. However, due to an error during installation, only 10 surface stakes of each species were placed on the treatment plots in replicate 2 and in replicate 3. In addition, 25 stakes were inserted vertically to a mineral soil depth of 20 cm in each plot, so that the top of the stakes were level with the soil surface (Figure 2). To minimize soil compaction at the wood-soil interface, stakes were placed into holes 2.5 cm on a side, ∼30 cm apart, made by a square 2.5 cm soil coring tool. A total of 540 wood stakes were used in the study: 2 treatments (hydromulch and control) × 3 replicates × 2 tree species (aspen and pine) × 2 stake locations (surface and mineral soil) × individual stakes (25 for mineral soil; 15 for surface).
As noted above, much of the hydromulch was removed from the soil surface during the first year after application, and so the surface mulch had a relatively short time to affect underlying mineral soil properties. Consequently, the impact of hydromulch on subsequent soil OM decomposition could be quite small (Berryman et al., 2014b). However, we decided to continue the stake study, whether or not there would be a hydromulch-treatment effect, as there is very little information in the literature on wood decomposition after wildfire. Therefore, beginning in January 2005 and annually for the next 4 years, we randomly sampled five surface and five mineral soil stakes of both tree species from each treatment plot. All stakes were weighed in the field to determine wood moisture content at extraction and sent to the School of Forest Resources and Environmental Science, Michigan Technological University, Houghton, MI, United States for processing.
Laboratory Analyses
In the laboratory, all stakes were cleaned of adhering soil, dried at 105°C for 48 h, and weighed. Stakes were then separated into two groups: (1) microbial decay—stake shows no evidence of termite attack. All mass loss is from microbial activity; and (2) termite-damaged—stake has been attacked by termites (e.g., feeding on wood surface, holes into wood) or is missing. Mass loss is from termite activity and any microbial activity that might have occurred before, during, or after the termite attack. Stakes with termite damage were also checked for soil moved into the wood by termites, and if present, it was physically removed with small scrapers. Wood blocks (2.5 cm long) were cut from all mineral soil stakes without termite damage at the 5 and 15 cm stake length to assess the effect of soil depth on decomposition (Figure 2).
Decomposition (mass loss) of both microbial decay and termite-damaged stakes was measured by subtracting the dry weight of individual surface and field stakes from the dry weight of its corresponding laboratory control (t0). Mass loss averages for each replicate (n = 5) were used as treatment observations in the statistical analyses. The impact of termites on stake decomposition was assessed by comparing mass loss of termite-damaged stakes to mass loss of microbial-decayed stakes taken from the same treatment plot and sample date.
Soil Sampling and Analyses
Three soil samples were collected from the 0–30 cm depth in each hydromulch and control plot replicate using the soil excavation/foam core method (Page-Dumroese et al., 1999). All samples were taken to USFS Soil Analytical Laboratory in Moscow, Idaho, to determine soil physical properties and bulk density. Soil samples were dried at 105°C for 24 h, weighed, and sieved through a 2-mm mesh screen to remove rocks, which were weighed to calculate soil fine-fraction bulk density (Page-Dumroese et al., 1999). The sieved soil was analyzed for OM by weight loss on ignition at 375°C for 16 h and pH on a 2:1 water to soil paste (Table 1).
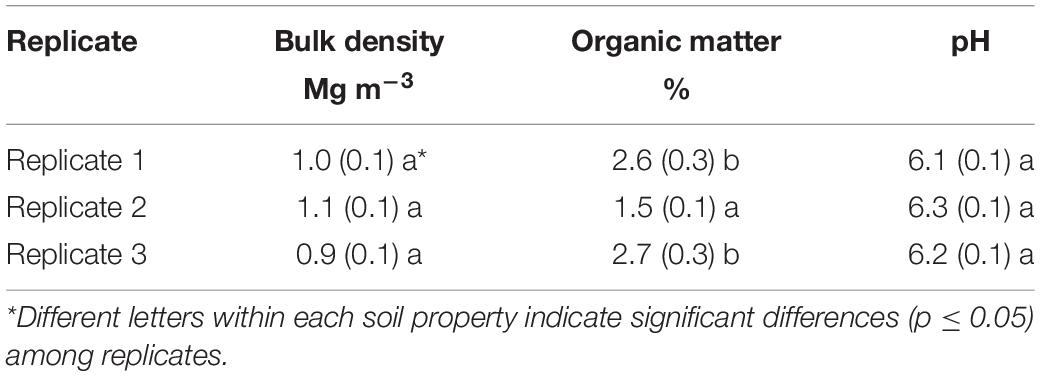
Table 1. Fine-fraction soil bulk density, organic matter concentration, and pH in each replicate of the Cedar Fire study site averaged (±SE) across hydromulch-treated and control soil.
Statistical Analysis
Analyses were conducted using SAS version 9.4 (SAS Institute, Cary, NC, United States) and assessed at α ≤ 0.05 significance level. SAS PROC GLM was utilized for this factorial design in conducting an analysis of variance (ANOVA). Factors in this analysis were sampling date (2005, 2006, 2007, 2008, and 2009), treatment (hydromulch application and control), stake location (surface or mineral), mineral soil depth (5 and 15 cm), and replicates (3 of each sampling date-treatment combination). The response variable was stake mass loss as a proportion of original mass, and each factor and all possible interactions were considered in the model. The arcsine square root transformation was used to homogenize the mass loss error term, as described in Steel and Torrie (1980). Post hoc assessments of significant effects and interactions were conducted with SAS PROC MEANS utilizing Tukey’s range test. Assessments of significant replication effects were conducted with the LSMEANS procedure. Results of these tests were considered for either pooled or un-pooled variances, as indicated by preliminary F-tests for homogeneity of variance.
Stake mass loss was averaged by replicate and used for each treatment × sampling date combination. As indicated earlier, two wood stake datasets were created to assess the effect of termites on decomposition: (1) mass loss from only microbial decay and (2) mass loss from microbial decay + termite damage. After this, a third “all stake” dataset was created by combining dataset 1 and 2. To further assess the effect of termites on wood mass loss, a categorical covariant for each stake was introduced to the model: “0” for only microbial decay, and “1” for termite damage. The frequency of stake termite attack between treatments, between wood species, and among plot replicates were compared with Fisher’s Exact test using SAS PROC FREQ.
Results
As expected, the full ANOVA model shows the strong impact of incubation time, stake location (surface or mineral soil), and tree species (aspen or pine) on wood mass loss (Supplementary Table S1), but we were surprised by the highly significant (p < 0.0001) treatment effect, since much of the hydromulch was lost early in the study. However, the explanatory power of tree species and stake location in the ANOVA (F-value) was very large (Table 2), which may have confounded the separation of the treatment from the other study variables in the model. Therefore, the impact of hydromulch on wood decomposition was analyzed separately by stake location and by tree species. Separate models, while still statistically valid, reduced the overall power of the test but increased the clarity of the treatment terms. Another surprising result was the highly significant (p < 0.0001) difference in stake mass loss among treatment replicates, which will be addressed in greater detail in the following sections.
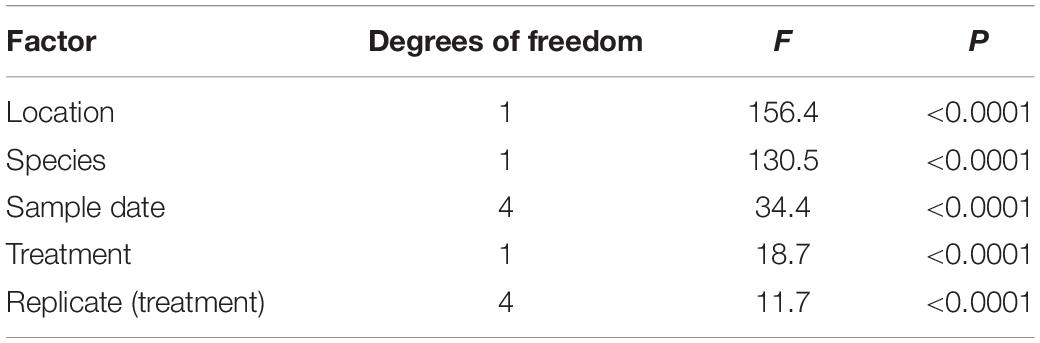
Table 2. Full model ANOVA main effects in the Cedar Fire study with wood stake mass loss as the dependent factor and hydromulch treatment, incubation time, species, and stake location as the independent factors.
The main effect results shown in Table 2 also reflect the activity of both soil microorganisms and termites in the wood decay process. By separating the mass loss of stakes decayed only by soil microorganisms to stakes decomposed by both microorganisms and termites in our analyses, the relative impact of each group on wood decomposition in this soil was estimated (Supplementary Table S2).
Surface Stakes
Aspen surface stakes decayed much faster than pine when placed on both the hydromulch and the untreated control soil (p < 0.0001). Contrary to our hypothesis, the hydromulch application did not increase microbial decomposition of aspen or pine stakes at any sampling date (Figure 3) and, when averaged over the 5-year study, aspen mass loss was significantly less on the surface of hydromulched soil than on the burned control soil (p < 0.0001). Termites were not active at the surface in either soil treatment, as only three of 45 aspen surface stakes had termite damage: one stake from the burned control in 2006 and 2008, and one stake in 2009 from the hydromulch treatment. While mass loss of termite-damaged stakes ranged from 29.1 to 56.8%, their overall effect on surface decomposition was minimal: an increase of 4% in 2006 and 8% in 2008 on the burned soil, and less than 2% on the hydromulched soil in 2009. Only one termite-damaged pine stake was found on hydromulched soil in 2009, which increased total treatment mass loss by 8%.
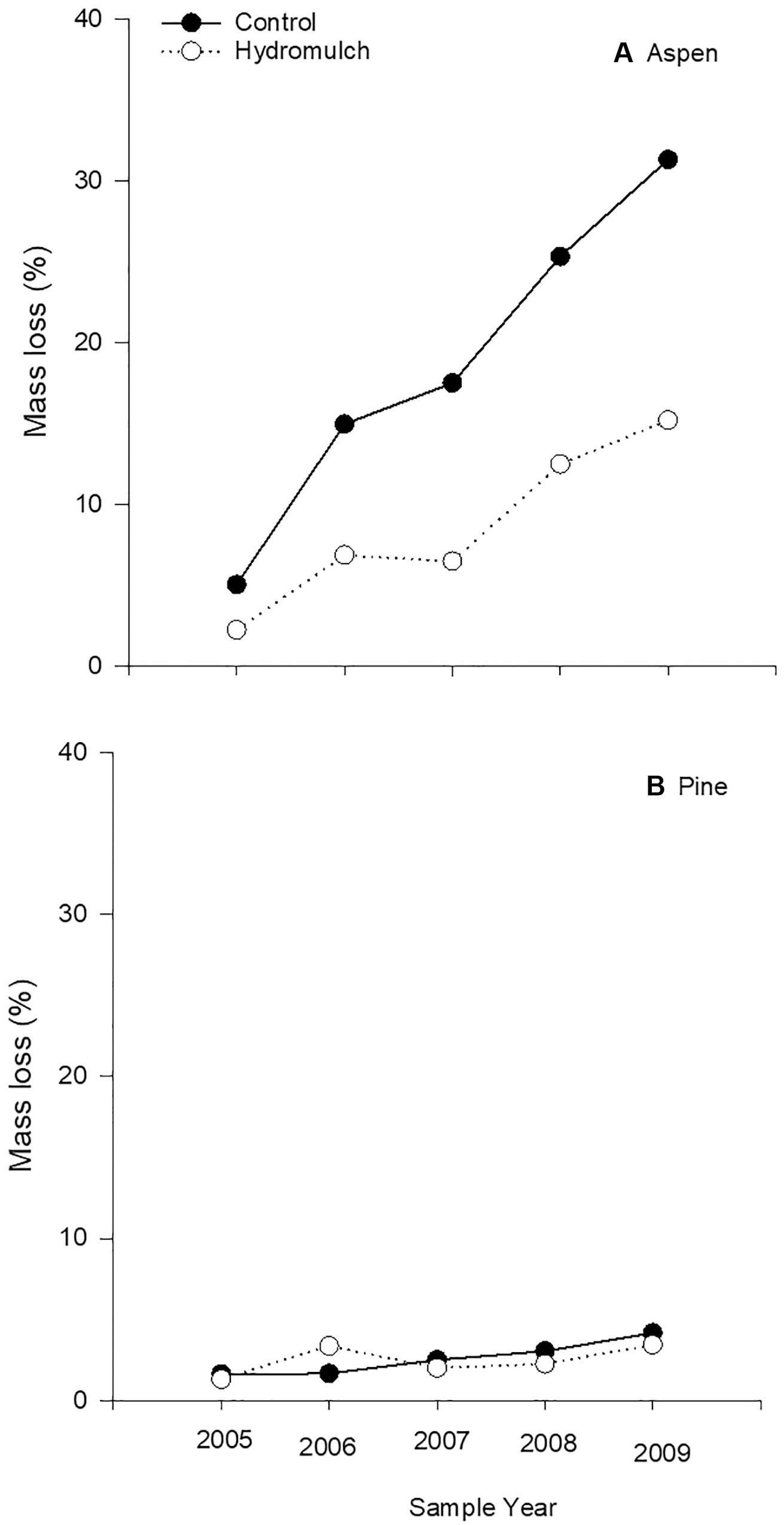
Figure 3. Mass loss of (A) aspen and (B) pine surface stakes from microbial decay following a hydromulch application after the Cedar Fire near Alpine, California.
Mineral Stakes
Aspen
Aspen stake mass loss from microbial decay was significantly less in the mineral soil of hydromulch-treated soil than in the control soil only at the 2007 sampling (Table 3), and also when averaged across the five sample dates (p = 0.0008). Termites dominated wood stake decomposition in the mineral soil. Of the 150 aspen stakes sampled from the hydromulch and control mineral soil, 79 (52.7%) had termite damage or were totally consumed or excavated by termites. The incidence of termite attacks on control soil stakes sampled in 2006 was significantly greater than on stakes from the hydromulched soil (p = 0.030), but the difference of stake numbers attacked by termites between the two treatments decreased in subsequent samplings. When averaged over the 5 years, termites attacked more stakes in the control than in the hydromulched soil (p = 0.051), but mass loss from termite-damaged stakes was similar (p = 0.228).
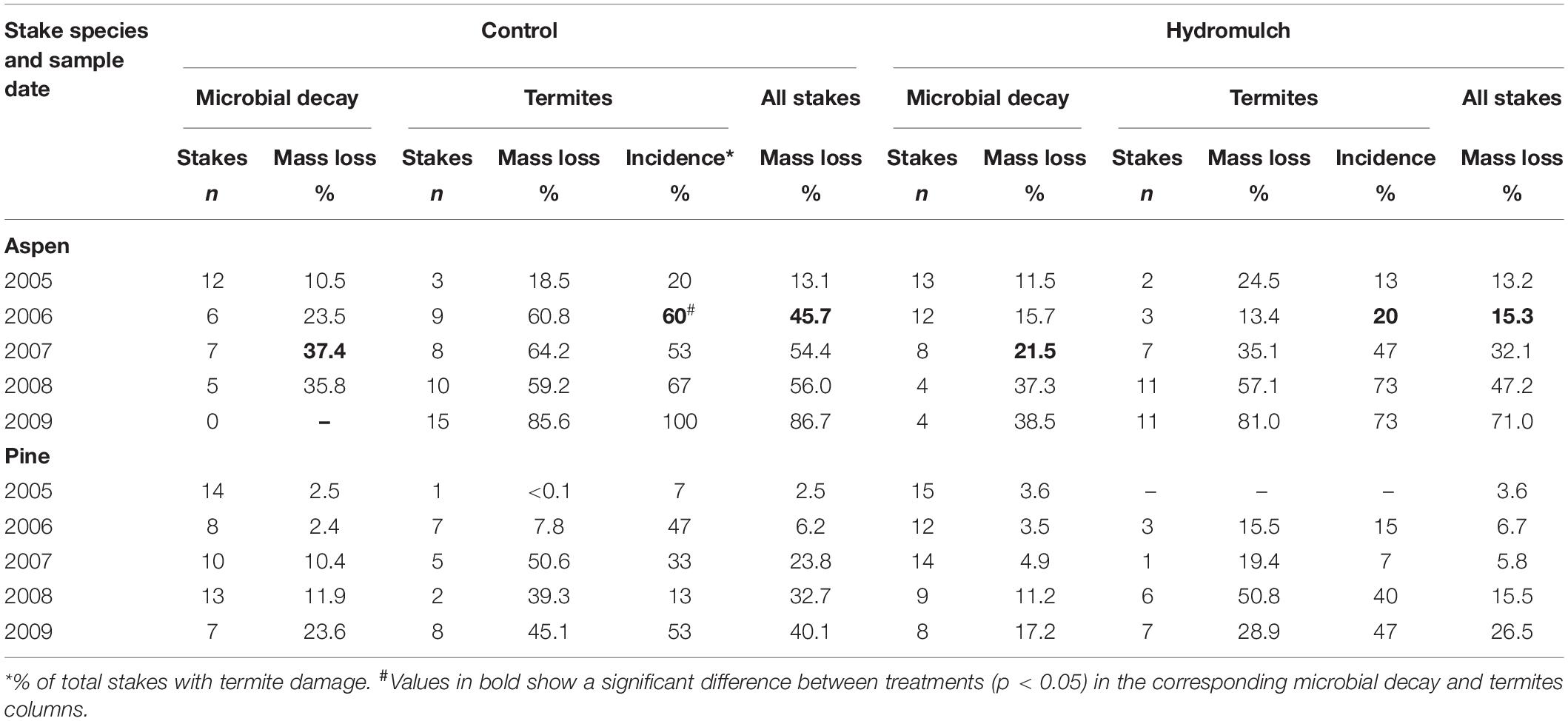
Table 3. Mass loss of aspen and pine stakes from microbial decay and termites in mineral soil following a hydromulch application after the Cedar Fire near Alpine, California.
As expected, mass loss of aspen stakes attacked by termites was greater than from microbial decay in both the hydromulched (p = 0.002) and control soil (p = 0.005). When aspen mineral soil stakes (microbial decay and termite-damaged) were combined by treatment, mass loss at the second (2006) sampling was significantly less in hydromulched soil than in the control soil (p = 0.004). As more stakes were attacked by termites, mass loss differences between the hydromulch and control soil became non-significant (Table 3); therefore, by the end of the 5-year study aspen mass loss in the mineral soil of both treatments was greater than 70% (p = 0.345). However, when averaged over all sample dates, aspen decomposition was significantly less in the hydromulch-treated soil than in the control soil (p = 0.002).
Pine
The temporal pattern of pine stake decomposition in mineral soil was similar to aspen (Table 3), but pine mass loss from microbial decay (p < 0.0001) and the incidence of termite attack (p = 0.0006) was significantly less than aspen stakes. Compared to the untreated burned control soil, the application of hydromulch had little effect on microbial decomposition of pine stakes in any sampled year, or when averaged over the study (p = 0.598). Only 40 of the 150 mineral soil pine stakes were attacked by termites (27%), and the incidence of termite attack was not significantly different between treatments in any sample year. However, when averaged over all sample dates, termites attacked more pine stakes in the control soil than in the hydromulched soil (p = 0.001), but the mass loss of termite-damaged stakes was similar (p = 0.389). When all pine mineral soil stakes (microbial decay and termite-damaged) were combined, the difference in mass loss between treatments was not significant in any sample year, but when averaged over the 5 years, pine stakes decomposed less in the hydromulched soil than in the control soil (p = 0.050).
Soil Depth
Since many mineral soil stakes of both species were fragmented, incomplete, or missing as a result of termite activity, the impact of soil depth on wood decomposition was assessed using only stakes with microbial decay, which totaled 58 aspen (38.7%) and 73 pine (48.7%) stakes. The hydromulch application had little effect on wood decomposition at both the 5 and 15 cm soil depths (aspen: p = 0.989; pine: p = 0.501), but averaged across both treatments, mass loss of aspen (p = 0.0068) and pine (p = 0.0014) stakes was highest at the 15 cm soil depth. The proportion of total stake mass loss at each soil depth was similar between species, averaging 38.8% at 5 cm and 61.2% at 15 cm.
Stake Moisture
Unfortunately, we were unable to obtain adequate information on soil water content during the study, but stake moisture content would reflect recent precipitation events and soil water conditions in this Mediterranean-type ecosystem (Table 4). As expected, stakes of both species were drier on the soil surface than in the mineral soil (p < 0.0001), but there was no significant difference in stake water content between the hydromulch treatment and the burned control for either surface (p = 0.076) or mineral soil (p = 0.227) stakes. The dry soil conditions on this site were indicated by many stakes having water contents near or below the wood fiber saturation point (∼30%), which limits microbial wood decay (Wood Handbook, 2010). Stake water content increased as wood porosity increased during the decomposition process, but it is not clear if this added wood water-holding capacity increased the rate of decay.
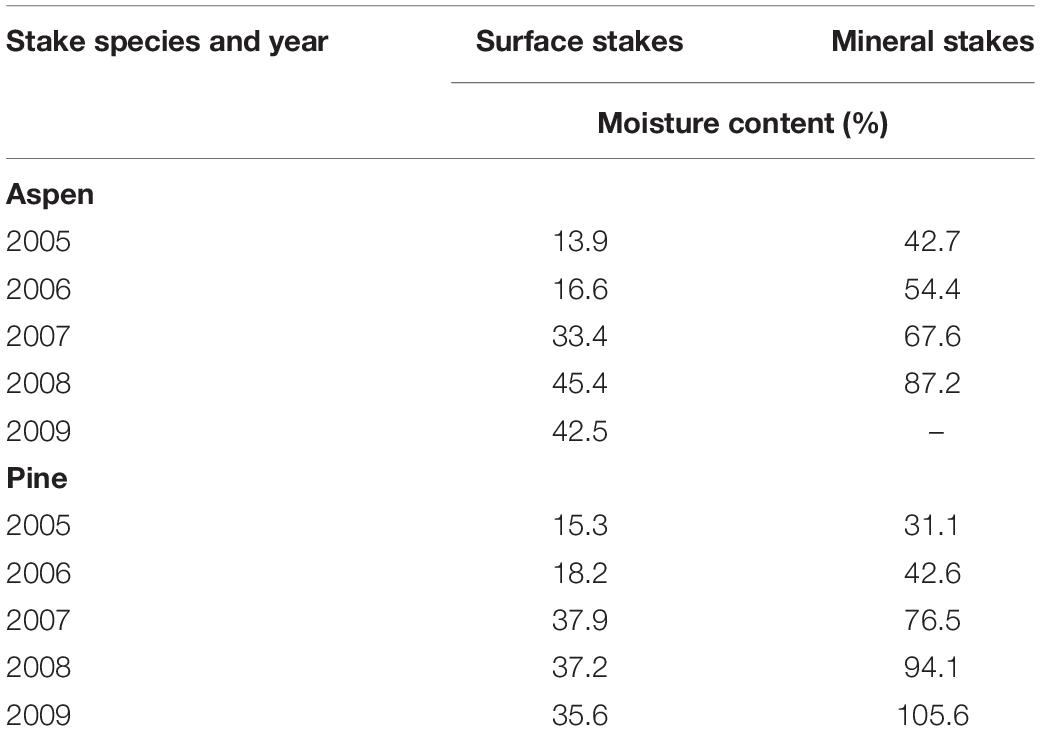
Table 4. Water content of surface and mineral soil stakes with microbial decay after a hydromulch application on the Cedar Fire near Alpine, California.
Replicate Variability
As shown in Table 2, wood stake mass loss among treatment replicates was highly significant, which reflects the variability of stakes in the mineral soil, but not on the soil surface. Large differences in stake mass loss among treatment replicates would likely decrease the precision of our analysis of hydromulch impacts on wood stake decomposition. Therefore, we used all stakes (microbial decay and termite-damaged combined) sampled during the study to assess mass loss differences among treatment replicates, which showed that mass loss of both aspen (p = 0.003) and pine (p = 0.003) stakes was significantly higher in the control soil of replicate 3 than in the corresponding hydromulch-treated soil (Figure 4).
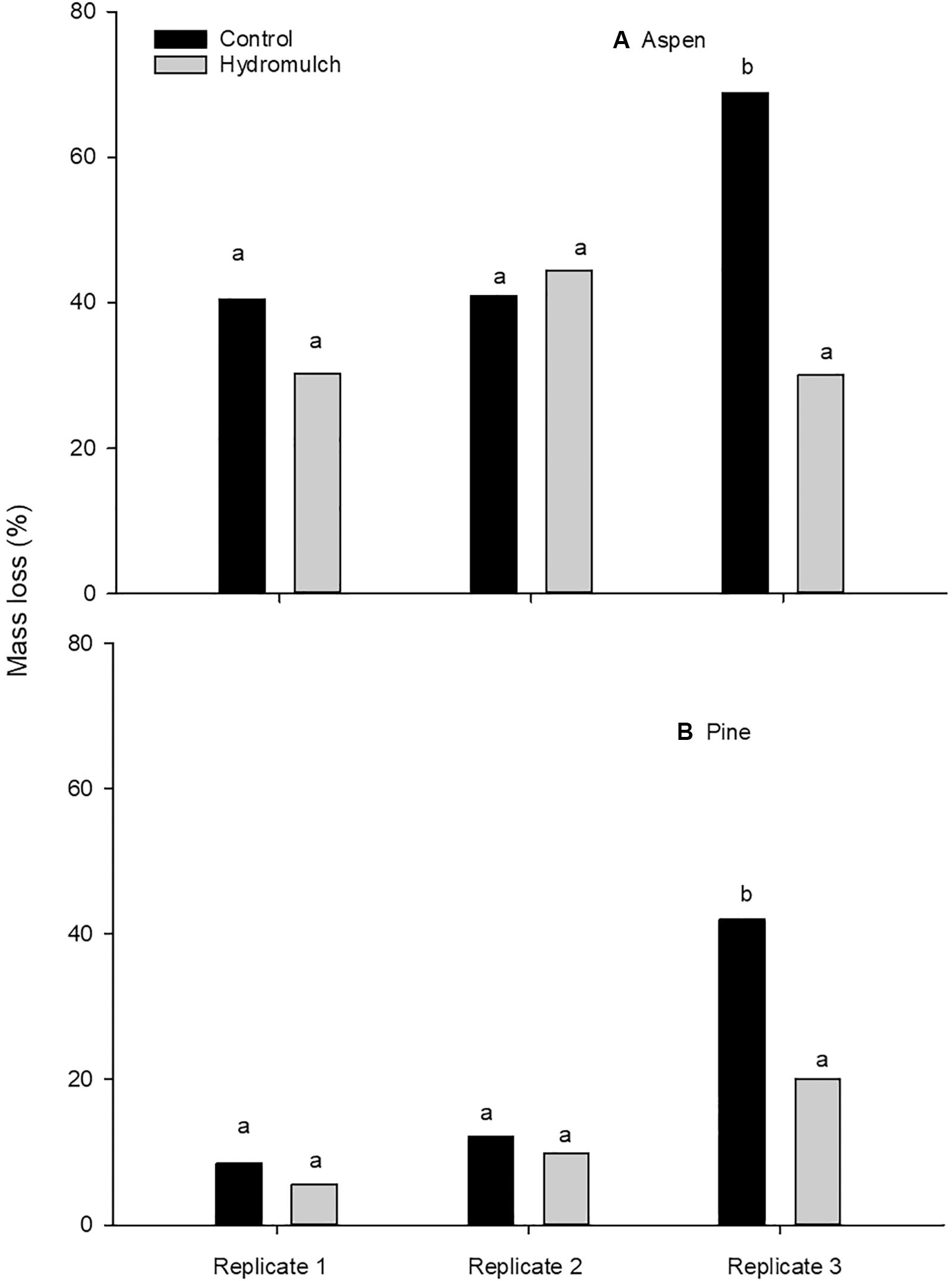
Figure 4. Mass loss of (A) aspen and (B) pine stakes in the mineral soil of three treatment replicates averaged across sampling dates on the Cedar Fire near Alpine, California. Different letters within species indicate a significant treatment difference (p ≤ 0.05) within replicates.
The high mass loss of mineral soil stakes in this one control replicate was due to a greater incidence of termite attack and not from increased microbial decay (Table 5). Therefore, we tried to separate the impact of the variable termite distribution from the effect of hydromulch on stake mass loss by using a categorical covariant for each stake: “0” for only microbial decay, and “1” for microbial decay + termite damage. While the adjusted means were still highly significant (p < 0.0001), this procedure did not decrease the error term or improve the F-statistic for the model. However, pine stake mass loss differences among replicates (p = 0.088) and between treatments were no longer significant (p = 0.313), but aspen mass loss was still lower in the hydromulch soil than in the burned control (p = 0.008).
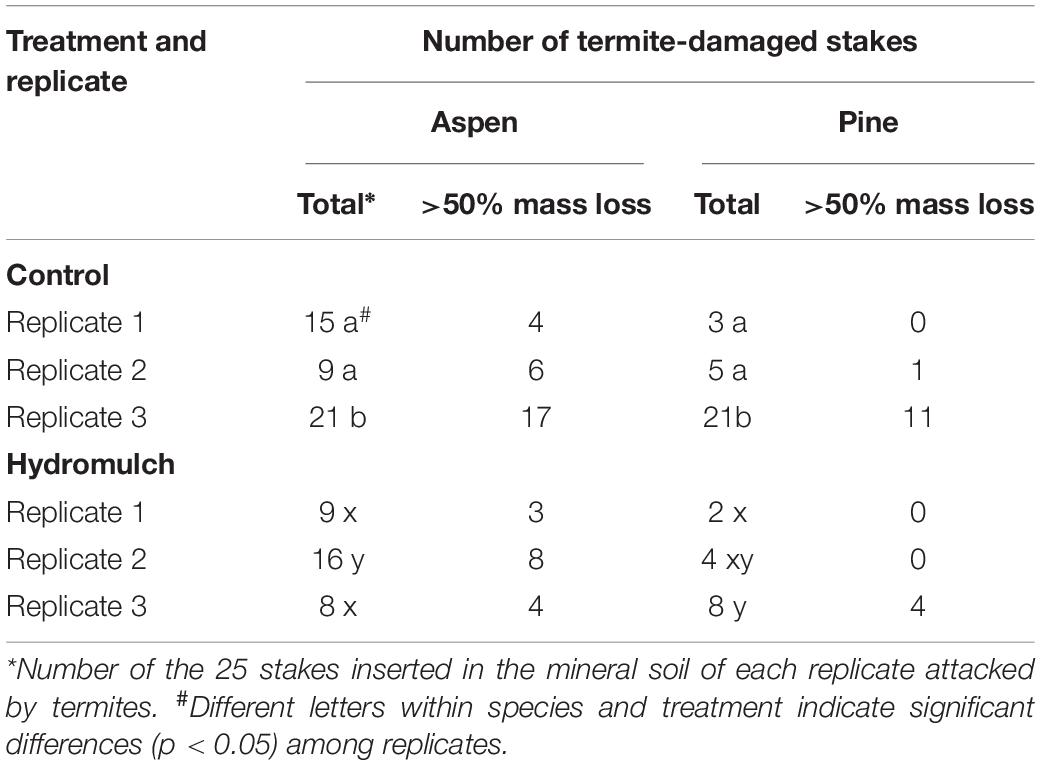
Table 5. Effect of treatment replicate on the incidence of subterranean termite attack and resulting mass loss of mineral soil aspen and pine stakes after the Cedar Fire near Alpine, California.
Discussion
Many studies in the United States and in Europe have shown that surface organic mulches generally improve soil chemical and physical properties and moderate soil microclimate after high-severity wildfires (e.g., Dodson and Peterson, 2010; Berryman et al., 2014a, b; Prats et al., 2016; Lucas-Borja et al., 2018b), leading us to hypothesize that hydromulch applied after the Cedar Fire would increase OM decomposition. However, a soil erosion study conducted near our study site indicated that much of the hydromulch was lost from the soil surface during several large storm events the first year after application (Hubbert et al., 2012). An early loss of a surface soil mulch also occurred after a wildfire on a similar coastal California shrubland (McCullough and Endress, 2012). Therefore, the hydromulch likely had little impact on mineral soil properties and subsequent OM decomposition, so we were surprised that stake mass loss was significantly less in the mulched soil than in the untreated control. This unexpected result was not due to the hydromulch application, but from greater termite activity in the untreated control plot of one replicate, in which the stakes were likely placed in soil with greater termite activity (e.g., near a nest or preferred food source) than in the other two replicates. We did not identify the termites on our study site, but seven species of Reticulitermes have been shown to occur in the California Southwestern Ecological Zone, where the Cedar Fire is located, and two likely species are Reticulitermes okanaganensis and Reticulitermes flavipes (Tripodi et al., 2006).
As we hypothesized, termites dominated wood stake decomposition in the mineral soil. Our results also showed that termites attacked aspen stakes more often than pine stakes. Since termites would have an equal probability of finding aspen and pine stakes as they forage through soil (Su et al., 1984), the higher attack frequency suggests that termites are attracted to aspen wood (Grace and Campora, 2005). Such an attractant could be a metabolite from microbial activity, or a chemical released from aspen wood during the decomposition process (Cornelius et al., 2003). Another possibility is that termites find pine stakes as often as aspen but decide to allocate their feeding efforts on a better food source (Oi et al., 1996; Lee and Forschler, 2016). Aspen has a higher cellulose to lignin ratio and a lower wood density than pine, which makes it more favorable to termite attack (e.g., Judd and Corbin, 2009; Shanbhag and Sundararaj, 2013).
In retrospect, we should not have been surprised about finding subterranean termites at our study site. They are found in a wide variety of United States soils that do not freeze in the winter (Wang and Powell, 2001; King et al., 2013), are common in semi-arid and arid woodlands (De Souza et al., 2003), and are present in many areas of California (Haverty et al., 1999; Tripodi et al., 2006). Globally, subterranean termites are found in many fire-prone ecosystems, in which they are buffered from the direct effect of fire by nesting underground (Avitabile et al., 2015), and may benefit from the increased availability of dead wood, as charred woody residue has little effect on termite-feeding preference (Peterson et al., 2008).
Subterranean termites increase the decomposition rate of surface woody residue in temperate forests (e.g., Ulyshen et al., 2016), but relatively little is known on how they impact wood decomposition in mineral soil, especially coarse roots. Tree roots usually comprise 25–30% of total tree biomass and are critical for maintaining soil stability on steep slopes (Ekanayake and Phillips, 1999). Root decay is a parameter in landslide susceptibility models, which assume dead roots are slowly decomposed by soil microorganisms (Sidle et al., 1985; Sakals and Sidle, 2004). The high incidence of termite attack on wood stakes in the mineral soil of our study site indicates that termites could also be a factor in root decomposition and slope stability after wildfire, but we could find no study in the literature that addressed this question.
The low mass loss of surface aspen and pine stakes from microbial decay and termite activity reflects the dry soil conditions in this Mediterranean-type ecosystem. Organic matter on the soil surface is prone to various cycles of wetting and drying coupled with extremes in temperature, and some species of termites and fungi are particularly sensitive to wood and soil moisture conditions less than 30% (Green et al., 2005; Wood Handbook, 2010). Unfortunately, we were unable to obtain information on soil water contents during the study, but higher stake mass loss deeper in the mineral soil indicated the predominance of low water conditions near the soil surface throughout the year. Increased mass loss at greater soil depth was also found in a wood stake decomposition study conducted after several wildfires in Montana forests (Page-Dumroese et al., 2019).
The high incidence of termite attack on wood stakes in the mineral soil clearly show that termites could have a major role in belowground wood decomposition in dry California soils, and could also be a factor in root decomposition and soil stability on steep slopes after wildfire (Ammann et al., 2009). However, variable termite activity among study replicates confounded the statistical assessment of the soil mulch and other study variables on OM decomposition, so termites should be considered in the experimental design and sampling protocols of decomposition studies in soils where they are present or suspected.
Management Implications
Due to the early loss of the hydromulch after application, our study gives little insight on the effect of this surface mulch on soil OM decomposition. However, greater wood stake decomposition with increasing soil depth reflects the impact of dry soil conditions on microbial activity in this Mediterranean-type ecosystem. It is critical to obtain better estimates of the contribution of arthropods to wood decay in chaparral and forest ecosystems to enhance our ability to model C and predict decay rates. Land managers can use this information to estimate how much wood or coarse roots may be consumed by termites. Further, data on wood decay in the mineral soil after wildfire can be used in slope stability models.
Data Availability Statement
The datasets generated for this study are available on request to the corresponding author.
Author Contributions
MJ and DP-D contributed to study design and field plot establishment. CM provided the statistical analyses. MJ wrote the first draft of the manuscript. CM and DP-D wrote sections of the manuscript. All authors contributed to manuscript revision, read, and approved the submitted versions.
Conflict of Interest
The authors declare that the research was conducted in the absence of any commercial or financial relationships that could be construed as a potential conflict of interest.
The reviewer PR declared a shared affiliation, with no collaboration, with one of the authors DP-D, to the handling editor at the time of review.
Acknowledgments
We gratefully acknowledge Dr. Pete Robichaud and Bob Brown (USDA Forest Service) and his team for establishing the hydromulched plots within the Cedar Fire and for graphic work. We also thank Jim Marin Graphics for Figure 2, the USDA Forest Service and Michigan Technological University field and laboratory crews that helped inventory, install, extract, and analyze the wood stakes.
Supplementary Material
The Supplementary Material for this article can be found online at: https://www.frontiersin.org/articles/10.3389/ffgc.2020.00093/full#supplementary-material
References
Ammann, M., Böll, A., Rickli, C., Speck, T., and Holdenrieder, O. (2009). Significance of tree root decomposition for shallow landslides. For. Snow Landsc. Res. 82, 79–94.
Avitabile, S. C., Nimmo, D. G., Bennett, A. F., and Clarke, M. F. (2015). Termites are resistant to the effects of fire at multiple spatial scales. PLoS One 10:e0140114. doi: 10.1371/journal.pone.0140114
Baird, M., Zabowski, D., and Everett, R. L. (1999). Wildfire effects on carbon and nitrogen in inland coniferous forests. Plant Soil 209, 233–243. doi: 10.1023/A:1004602408717
Bautista, S., Robichaud, P. R., and Blade, C. (2009). “Post-fire mulching,” in Fire Effects on Soils and Restoration Strategies, eds A. Cerdà and P. R. Robichaud (Boca Raton, FL: CRC press), 353–373.
Bento-Gonçalves, B., Vieira, V., Úbeda, X., and Martin, D. (2012). Fire and soils: key concepts and recent advances. Geoderma 191, 3–13. doi: 10.1016/j.geoderma.2012.01.004
Berryman, E. M., Morgan, P., Robichaud, P. R., and Page-Dumroese, D. (2014a). Post-Fire Erosion Control Mulches Alter Belowground Processes and Nitrate Reductase Activity of a Perennial Forb, Heartleaf Arnica (Arnica cordifolia). Res. Note RMRS-RN-69. Fort Collins, CO: US Department of Agriculture, Forest Service, Rocky Mountain Research Station.
Berryman, E., Page-Dumroese, D., Jurgensen, M., and Robichaud, P. (2014b). “Post fire erosion control mulch effects on soil organic matter turnover,” in Advances in Forest Fire Research, Chap. 6, ed. D. X. Viegas (Portugal: Universidade de Coimbra), 1749–1751. doi: 10.14195/958-989-26-0884-6_192
Beyers, J. L. (2004). Postfire seeding for erosion control: effectiveness and impacts on native plant communities. Conserv. Biol. 18, 947–956. doi: 10.1111/j.1523-1739.2004.00523.x
Blanchette, R. A. (1984). Screening wood decayed by white rot fungi for preferential lignin degradation. Appl. Environ. Microbiol. 48, 647–653. doi: 10.1128/aem.48.3.647-653.1984
Bradford, M. A., Warren, R. J. II, Baldrian, P., Crowther, T. W., Maynard, D. S., et al. (2014). Climate fails to predict wood decomposition at regional scales. Nat. Clim. Change 4, 625–630. doi: 10.1038/nclimate2251
Chen, X., Wei, X., and Scherer, R. (2005). Influence of wildfire and harvest on biomass, carbon pool, and decomposition of large woody debris in forested streams of southern interior British Columbia. For. Ecol. Manage. 208, 101–114. doi: 10.1016/j.foreco.2004.11.018
Cornelius, M. L., Daigle, D. J., Connick, W. J. Jr., Williams, K. S., and Lovisa, M. P. (2003). Responses of the Formosan subterranean termite (Isoptera: Rhinotermitidae) to wood blocks inoculated with lignin-degrading fungi. Sociobiology 41, 513–525.
De Souza, O., Albuquerque, L. B., Tonello, V. M., Pinto, L. P., and Reis, R. Jr. (2003). Effects of fire on termite generic richness in a savanna-like ecosystem (‘cerrado’) of central Brazil. Sociobiology 42, 639–649.
Dodson, E. K., and Peterson, D. W. (2010). Mulching effects on vegetation recovery following high severity wildfire in north-central Washington State. USA. For. Ecol. Manage. 260, 1816–1823. doi: 10.1016/j.foreco.2010.08.026
Doerr, S. H., and Cerdà, A. (2005). Fire effects on soil system functioning: new insights and future challenges. Inter. J. Wildland Fire. 14, 339–342.
Ekanayake, J. C., and Phillips, C. J. (1999). A method for stability analysis of vegetated hillslopes: an energy approach. Can. Geotech. J. 36, 1172–1184. doi: 10.1139/t99-060
Foltz, R. B., and Wagenbrenner, N. S. (2010). An evaluation of three wood shred blends for post-fire erosion control using indoor simulated rain events on small plots. Catena. 80, 86–94. doi: 10.1016/j.catena.2009.09.003
Fontúrbel, M. T., Barreiro, A., Vega, J. A., Martín, A., Jiménez, E., Carballas, T., et al. (2012). Effects of an experimental fire and post-fire stabilization treatments on soil microbial communities. Geoderma 191, 51–60. doi: 10.1016/j.geoderma.2012.01.037
Geddes, N., and Dunkerley, D. (1999). The influence of organic litter on the erosive effects of raindrops and of gravity drops released from desert shrubs. Catena 36, 303–313. doi: 10.1016/s0341-8162(99)00050-8
Grace, J. K., and Campora, C. E. (2005). “Food location and discrimination by subterranean termites (Isoptera: Rhinotermitidae),” in Proceedings of the 5th International Conference Urban Pests, eds C.-Y. Lee and W. H. Robinson (Singapore: ICUP), 437–441.
Gray, D. M., and Dighton, J. (2006). Mineralization of forest litter nutrients by heat and combustion. Soil Biol. Biochem. 38, 1469–1477. doi: 10.1016/j.soilbio.2005.11.003
Green, J. M., Scharf, M. E., and Bennett, G. W. (2005). Impacts of soil moisture level on consumption and movement of three sympatric subterranean termites (Isoptera: Rhinotermitidae) in a laboratory assay. J. Econ. Ento. 98, 933–937.
Guerrero, C., Mataix-Solera, J., Gómez, I., García-Orenes, F., and Jordán, M. M. (2005). Microbial recolonization and chemical changes in a soil heated at different temperatures. Inter. J. Wildland Fire 14, 385–400.
Harmon, M. E., Franklin, J. F., Swanson, F. J., Sollins, P., Gregory, S. V., Lattin, J. D., et al. (1986). Ecology of coarse woody debris in temperate ecosystems. Adv. Ecol. Res. 15, 133–302. doi: 10.1016/s0065-2504(08)60121-x
Haverty, M. I., Getty, G. M., Copren, K. A., and Lewis, V. R. (1999). Seasonal foraging and feeding behavior of Reticulitermes spp. (Isoptera: Rhinotermitidae) in a wildland and a residential location in northern California. Environ. Ento. 28, 1077–1084. doi: 10.1093/ee/28.6.1077
Hebel, C. L., Smith, J. E., and Cromack, K. Jr. (2009). Invasive plant species and soil microbial response to wildfire burn severity in the Cascade Range of Oregon. Appl. Soil Ecol. 42, 150–159. doi: 10.1016/j.apsoil.2009.03.004
Homann, P. S., Bormann, B. T., Darbyshire, R. L., and Morrissette, B. A. (2011). Forest soil carbon and nitrogen losses associated with wildfire and prescribed fire. Soil Sci. Soc. Am. J. 75, 1926–1934. doi: 10.2136/sssaj2010-0429
Hubbert, K. R., Wohlgemuth, P. M., and Beyers, J. L. (2012). Effects of hydromulch on post-fire erosion and plant recovery in chaparral shrublands of southern California. Inter. J. Wildland Fire. 14, 385–400.
Judd, T. M., and Corbin, C. C. (2009). Effect of cellulose concentration on the feeding preferences of the termite Reticulitermes flavipes (Isoptera: Rhinotermitidae). Sociobiology 53, 775–784.
Jurgensen, M. F., Miller, C. A., Trettin, C. T., and Page-Dumroese, D. S. (2019). Bedding of wetland soil: Effects of bed height and termite activity on wood decomposition. Soil Sci. Soc. Am. J. 83, 218–227.
Jurgensen, M. F., Reed, D. D., Page-Dumroese, D. S., Laks, P., Collins, A., Mroz, G., et al. (2006). Wood strength loss as a measure of decomposition in the northern forest mineral soil. Europ. J. Soil Sci. 42, 23–31. doi: 10.1016/j.ejsobi.2005.09.001
Kartal, S. N., Aysal, S., Terzi, E., Yilgör, N., Yoshimura, T., and Tsunoda, K. (2013). Wood and bamboo-PP composites: fungal and termite resistance, water absorption, and FT-IR analyses. BioResources 8, 1222–1244.
King, J. R., Warren, R. J., and Bradford, M. A. (2013). Social insects dominate eastern US temperate hardwood forest macroinvertebrate communities in warmer regions. PLoS One 8:e75843. doi: 10.1371/journal.pone.0075843
Lee, T. Y., and Forschler, B. T. (2016). Wood preference of Reticulitermes virginicus (Blattodea: Rhinotermitidae) using no-, two-, and four-choice designs and seven different measures of wood consumption. J. Econ. Entomol. 109, 785–791. doi: 10.1093/jee/tov391
Li, X., Niu, J., and Xie, B. (2014). The effect of leaf litter cover on surface runoff and soil erosion in Northern China. PLoS One 9:e107789. doi: 10.1371/journal.pone.0107789
Lombao, A., Martín, A., Barreiro, A., Fontúrbel, M. T., Carballas, T., and Vega, J. A. (2013). Microbial biomass estimated by phospholipid fatty acids (PLFA pattern) in a soil with different post-fire treatments (seeding, mulching) one year after the experimental fire. Flamma 4, 9–12.
Lucas-Borja, M. E., González-Romero, J., Plaza-Álvarez, P. A., Sagra, J., Gómez, M. E., et al. (2018a). The impact of straw mulching and salvage logging on post-fire runoff and soil erosion generation under Mediterranean climate conditions. Sci. Total Envir. 654, 441–451. doi: 10.1016/j.scitotenv.2018.11.161
Lucas-Borja, M. E., Zema, D., Carra, G. B., Cerdá, A., Plaza-Alvarez, P. A., et al. (2018b). Short-term changes in infiltration between straw mulched and non-mulched soils after wildfire in Mediterranean forest ecosystems. Ecol. Eng. 112, 27–31. doi: 10.1016/j.ecoleng.2018.07.018
Mataix-Solera, J., Cerdà, A., Arcenegui, V., Jordán, A., and Zavala, L. M. (2011). Fire effects on soil aggregation: a review. Earth-Sci. Rev. 109, 44–60. doi: 10.1016/j.earscirev.2011.08.002
Mataix-Solera, J., Guerrero, C., García-Orenes, F., Bárcenas, G. M., Torres, M. P., and Barcenas, M. (2009). “Forest fire effects on soil microbiology,” in Fire Effects on Soils and Restoration Strategies, eds A. Cerdà and P. R. Robichaud (Boca Raton, FL: CRC press), 133–175. doi: 10.1201/9781439843338-c5
McCullough, S. A., and Endress, B. A. (2012). Do postfire mulching treatments affect plant community recovery in California coastal sage scrub lands? Environ. Manage. 49, 142–150. doi: 10.1007/s00267-011-9777-2
Neary, D. G., Koestner, K. A., Youberg, A., and Koestner, P. E. (2012). Post-fire rill and gully formation, Schultz Fire 2010, Arizona, USA. Geoderma 191, 97–104. doi: 10.1016/j.geoderma.2012.01.016
Oi, F. M., Su, N.-Y., Koehler, P. G., and Slansky, F. (1996). Laboratory evaluation of food placement and food types on feeding preference of Reticulitermes virginicus (Isoptera: Rhinotermitidae). J. Econ. Entomol. 89, 915–921. doi: 10.1093/jee/89.4.915
Page-Dumroese, D. S., Brown, R. E., Jurgensen, M. F., and Mroz, G. D. (1999). Comparison of methods for determining bulk densities of rocky forest soils. Soil Sci. Soc. Am. J. 63, 379–383. doi: 10.2136/sssaj1999.03615995006300020016x
Page-Dumroese, D. S., and Jurgensen, M. F. (2006). Soil carbon and nitrogen pools in mid- to late-successional forest stands of the northwestern United States: Potential impact of fire. Can. J. Forest Res.. 36, 2270–2284. doi: 10.1139/x06-125
Page-Dumroese, D. S., Jurgensen, M. F., Miller, C. A., Pickens, J. B., and Tirocke, J. M. (2019). Wildfire alters belowground and surface wood decomposition on two national forests in Montana. USA. Intern. J. Wildland Fire 28, 456–469.
Peterson, C. J., Gerard, P. D., and Wagner, T. L. (2008). Charring does not affect wood infestation by subterranean termites. Ento. Exper. Appl. 126, 78–84.
Prats, S. A., Wagenbrenner, J. W., Martins, M. A., Malvar, M. C., and Keizer, J. J. (2016). Hydrologic implications of post-fire mulching across different spatial scales. Land Degrad. Dev. 27, 1440–1452. doi: 10.1002/ldr.2422
Radeloff, V. C., Hammer, R. B., Voss, P. R., Hagen, A. E., Field, D. R., and Mladenoff, D. J. (2001). Human demographic trends and landscape level forest management in the northwest Wisconsin Pine Barrens. For. Sci. 47, 229–241.
Rice, R. M. (1974). “The hydrology of chaparral watersheds,” in Proceedings of the Symposium on Living with the Chaparral, Riverside, CA, ed. M. Rosenthal (San Francisco, CA: Sierra Club), 27–34.
Ritsema, C. J., and Dekker, L. W. (1996). Water repellency and its role in forming preferred flow paths in soils. Soil Res. 34, 475–487.
Robichaud, P. R. (2000). Fire effects on infiltration rates after prescribed fire in northern Rocky Mountain forests, USA. J. Hydrol. 231-32, 220–229. doi: 10.1016/s0022-1694(00)00196-7
Robichaud, P. R., Lewis, S. A., Wagenbrenner, J. W., Ashmun, L. E., and Brown, R. E. (2013). Post-fire mulching for runoff and erosion mitigation: Part I: Effectiveness at reducing hillslope erosion rates. Catena 105, 75–92. doi: 10.1016/j.catena.2012.11.015
Sakals, M. E., and Sidle, R. C. (2004). A spatial and temporal model of root cohesion in forest soils. Can. J. For. Res. 34, 950–958. doi: 10.1139/x03-268
Shanbhag, R. R., and Sundararaj, R. (2013). Physical and chemical properties of some imported woods and their degradation by termites. J. Insect Sci. 13, 63.
Sidle, R. C., Pearce, A. J., and O’Loughlin, C. L. (1985). Hillslope stability and land use. Am. Geophys. Union Water Resource Monography 11. Washington, D.C: American Geophysical Union.
Spigel, K. M., and Robichaud, P. R. (2007). First-year post-fire erosion rates in Bitterroot National Forest, Montana. Hydrol. Process. 21, 998–1005. doi: 10.1002/hyp.6295
Steel, R. G., and Torrie, J. H. (1980). Principles and Procedures of Statistics, A Biometrical Approach (No. Ed. 2). New York, NY: McGraw-Hill Kogakusha, Ltd.
Su, N., Tamashiro, M., Yates, J. R., and Haverty, M. I. (1984). Foraging behavior of the Formosan subterranean termite (Isoptera: Rhinotermitidae). Environ. Entomol. 13, 1466–1470. doi: 10.1093/ee/13.6.1466
Sugimoto, A., Bignell, D. E., and McDonald, J. A. (2000). “Global impact of termites on the carbon cycle and atmospheric trace gases,” in Termites: Evolution, Sociality, Symbiosis, Ecology, eds T. Abe, D. E. Bignell, and M. Higashi (Dordrecht: Springer), 409–435. doi: 10.1007/978-94-017-3223-9_19
Tripodi, A. D., Austin, J. W., Szalanski, A. L., McKern, J., Carroll, M. K., et al. (2006). Phylogeography of Reticulitermes termites (Isoptera: Rhinotermitidae) in California inferred from mitochondrial DNA sequences. Ann. Ento. Soc. Am. 99, 697–706. doi: 10.1603/0013-8746(2006)99[697:portir]2.0.co;2
Tsunoda, K. (2001). Preservative properties of vapor-boron-treated wood and wood-based composites. J. Wood Sci. 47, 149–153. doi: 10.1007/bf00780565
Ulyshen, M. D., Diehl, S. V., and Jeremic, D. (2016). Termites and flooding affect microbial communities in decomposing wood. Intern. Biodeterioration Biodeg. 115, 83–89. doi: 10.1016/j.ibiod.2016.07.017
Ulyshen, M. D., Wagner, T. L., and Mulrooney, J. E. (2014). Contrasting effects of insect exclusion on wood loss in a temperate forest. Ecosphere 4, 1–15.
Van Mantgem, P., Schwartz, M., and Keifer, M. (2001). Monitoring fire effects for managed burns and wildfires: coming to terms with pseudoreplication. Nat. Areas J. 21, 266–273.
Wang, C., and Powell, J. (2001). Survey of termites in the Delta Experimental Forest of Mississippi. Florida Ento. 84:222. doi: 10.2307/3496170
Wohlegemuth, P. M., and Robichaud, P. R. (2007). “The effects of selected post-fire emergency rehabilitation techniques on small watershed sediment yields in southern California,” in Advancing the fundamental sciences: Proceedings of the Forest Service National Earth Sciences Conference, eds M. Furniss, C. Clifton, and K. Ronnenberg (Portland, OR: U.S. Department of Agriculture, Forest Service, Pacific Northwest Research Station), 36–42.
Keywords: wildfire, organic matter, hydromulching, Pinus taeda, Populus tremuloides, Cedar Fire, termites
Citation: Jurgensen MF, Miller CA and Page-Dumroese DS (2020) Wood Decomposition After an Aerial Application of Hydromulch Following Wildfire in a Southern California Chaparral Shrubland. Front. For. Glob. Change 3:93. doi: 10.3389/ffgc.2020.00093
Received: 22 December 2019; Accepted: 07 July 2020;
Published: 30 July 2020.
Edited by:
Peter Fule, Northern Arizona University, United StatesReviewed by:
Daniel Moya, University of Castilla-La Mancha, SpainPete Robichaud, United States Forest Service (USDA), United States
Copyright © 2020 Jurgensen, Miller and Page-Dumroese. This is an open-access article distributed under the terms of the Creative Commons Attribution License (CC BY). The use, distribution or reproduction in other forums is permitted, provided the original author(s) and the copyright owner(s) are credited and that the original publication in this journal is cited, in accordance with accepted academic practice. No use, distribution or reproduction is permitted which does not comply with these terms.
*Correspondence: Deborah S. Page-Dumroese, ZGViYmllLmR1bXJvZXNlQHVzZGEuZ292