- 1Forest Health and Biotic Interactions, Swiss Federal Institute for Forest, Snow and Landscape Research WSL, Birmensdorf, Switzerland
- 2Forest Protection and Forest Health Section, Federal Office for the Environment FOEN, Ittigen, Switzerland
Invasions by non-native pathogens represent a major threat to managed and natural ecosystems worldwide. Although necessary for adopting preventive strategies, the identification of invasive species before they are introduced is particularly difficult. Indeed, most pathogenic species that have become established in the last decades were first described only after they became invasive. To prevent further biological invasions, not only the early identification of potential new invasive plant pathogens is crucial, but also the assessment of their potential host range. In this study, we determined the pathogenicity and the saprotrophic ability of three Cryphonectria species toward three potential hosts in the family Fagaceae. For this, seedlings and dormant stems of European chestnut (Castanea sativa), pedunculate oak (Quercus robur) and European beech (Fagus sylvatica) were inoculated with different genotypes of C. parasitica (Asian species, invasive in Europe), C. naterciae (European species), and C. japonica (Asian species, not present in Europe). Lesion growth was measured and mortality assessed for 4 months. The highest damage was caused by C. parasitica on European chestnut, while C. japonica and C. naterciae induced significantly smaller lesions on this host species. All three Cryphonectria species did not grow saprophytically on F. sylvatica and Q. robur, but successfully colonized dormant stems of C. sativa. In the context of biological invasions, our study shows that the Asian C. japonica most likely represents a much less severe threat than C. parasitica for the tested European host species. Nonetheless, the ability of C. naterciae and C. japonica to saprotrophically colonize fresh chestnut wood may suggest that they could become established in chestnut forests and eventually infect weakened chestnut trees or other hosts not tested in this study.
Introduction
The risk of accidentally moving plant pathogens outside their native range is constantly increasing because of global trade and other anthropogenic activities (Santini et al., 2013). Climatic changes, which allow pests to survive in formerly climatic adverse regions, further contribute to range expansions (Stenlid and Oliva, 2016). In the new range, harmful organisms frequently encounter new plant species, some of which may be highly susceptible. In this case, the introduced pest can become invasive (Ghelardini et al., 2017). Detecting invasive species before they are introduced is particularly difficult. Indeed, most pathogenic species that have become established in the last decades were first described only after they became invasive (Brasier, 2008). Assessing the pathogenicity of related species of already present invasive pathogens may be a valuable strategy to spot potentially invasive species before they are introduced (Gilbert et al., 2015).
Although plant pathogens in the introduced range generally attack species which are closely related to the primary host in the natural range (Gilbert and Webb, 2007; De Vienne et al., 2009; Gilbert et al., 2015), in some cases drastic host changes are observed. For example, the rust fungus Puccinia psidii Winter considerably expanded its host range when introduced to Australia (Carnegie and Lidbetter, 2012). The bark pathogen Chrysoporthe cubensis Bruner Gryzenhout causes severe damage on introduced Eucalyptus spp. and native Myrtaceae and Melostomataceae in Southeast Asia, South America, Africa and Australia (Rodas et al., 2005; Nakabonge et al., 2007; Pegg et al., 2010). Therefore, to prevent further biological invasions not only the early detection of potential new invasive plant pathogens is crucial, but also the assessment of its potential host range.
Cryphonectria parasitica, (Murr.) Barr. the causal agent of chestnut blight, is one of the most damaging invasive pathogens in forest ecosystems. Native to Asia (China, Japan, Korea), it was introduced to North America at the beginning of the 20th century and then from there to Europe in the 1930’s (Rigling and Prospero, 2018). Additional introductions to Europe as well as to the Caucasus region took place later directly from Asia (Dutech et al., 2012; Prospero and Cleary, 2017). The pathogen spread rapidly in the introduced ranges where it encountered susceptible Castanea species (Anagnostakis, 1992) on which it causes lethal bark lesions (so-called cankers). In North America, American chestnut (C. dentata) has virtually become extinct because of chestnut blight (Anagnostakis, 1987). By contrast, in Europe the C. parasitica epidemic on European chestnut (Castanea sativa) showed a milder course due to the appearance and spread in the C. parasitica population of a hyperparasitic mycovirus (CHV-1) that acts as biological control agent, and to higher resistance of C. castanea compared to C. dentata (Rigling and Prospero, 2018). The pathogen can also survive and readily sporulate on fresh dead wood, which is considered to have an important epidemiological role (Prospero et al., 2006; Meyer et al., 2019). Although C. parasitica is considered a primary pathogen only on C. dentata and C. sativa (Anagnostakis, 1987; Bragança et al., 2011), it has also been occasionally detected on various Quercus species in different European countries, such as Greece (Tziros et al., 2015), the Czech Republic (Haltofová et al., 2005), Italy (Biraghi, 1950; Dallavalle and Zambonelli, 1999) and Slovakia (Adamčíková et al., 2010).
Recently, two other species of the genus Cryphonectria have been officially detected and described, namely C. naterciae in Europe (Bragança et al., 2011), and C. japonica in Asia (Myburg et al., 2004). C. naterciae was isolated in Portugal from bark cankers on C. sativa and orange bark lesions on cork oaks (Quercus suber). Its closest relatives inferred by partial sequencing of the ITS region are C. radicalis and C. parasitica (Bragança et al., 2011). C. japonica (previously named C. nitschkei) was isolated from Castanea crenata in Japan (Liu et al., 2003; Gryzenhout et al., 2009) and from Quercus spp. in China, on which it causes bark cankers with orange fruiting structures (Jiang et al., 2019). The pathogenicity of C. naterciae and C. japonica on C. sativa and other European species in the family Fagaceae is, however, still unknown.
In this study, we aimed to compare the parasitic and saprotrophic ability of the Asian C. japonica with that of an invasive species already established in Europe (C. parasitica) and a native European species (C. naterciae). As hosts we selected three species of the Fagaceae, i.e., C. sativa, the main host of C. parasitica, and pedunculate oak (Quercus robur) and European beech (Fagus sylvatica), two widespread species in Central Europe. The results of this study will allow a better understanding of the risk posed by Cryphonectria species to common European Fagaceae species.
Materials and Methods
Cryphonectria spp. Isolates
The study was conducted using three isolates each of C. parasitica, C. naterciae and C. japonica (Table 1). Isolates of C. parasitica were selected to represent two frequent vegetative compatibility (vc) types (EU-1 and EU-2) and one rare vc type (EU-12) in Switzerland (Prospero and Rigling, 2012). Genotyping at 10 microsatellite loci (Prospero and Rigling, 2012) showed that each of them belonged to a different multilocus genotype (M2372–CpMG15, M2671–CpMG37, and M4023–CpMG21). Isolates of C. naterciae and C. japonica originated from Portugal and Japan, respectively, and showed no amplification with the C. parasitica specific microsatellite assay.
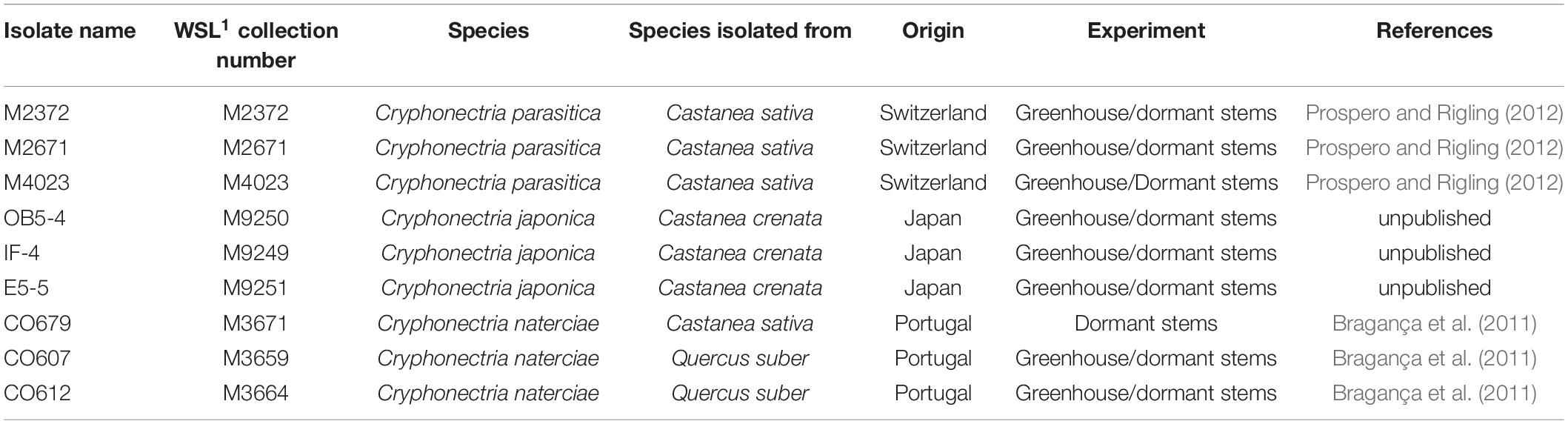
Table 1. Cryphonectria spp. isolates used in this study. 1: WSL: Swiss Federal Institute for Forest, Snow and Landscape Research, Birmensdorf, Switzerland.
Seedling Experiment
Inoculations were performed on two-year-old seedlings of C. parasitica, Q. robur, and F. sylvatica obtained from natural populations (i.e., no clonal material). The plants were placed on charts in a chamber of the WSL biosafety greenhouse, which was set at a temperature of 22 C. A random block design was used for the experiment with weekly rotations of the blocks within the chamber to avoid chamber effects. The Cryphonectria spp. isolates were grown on Potato Dextrose Agar (PDA; 39g/L, Difco Laboratories, Detroit United States) for 5 days at 25°C in the dark and then inoculated on the stems of the seedlings, as described in Dennert et al. (2019). Briefly, holes of 0.5 cm diameter were made in the bark using a cork borer, and an agar plug of the same size was inserted with the mycelium toward the stem. A total of five seedlings were inoculated with each isolate and five seedlings of each tree species with an agar plug as negative control. The inoculation points were sealed with tape and the developing lesions were measured every 2 weeks for 4 months. The length and the width were recorded, and lesion area was calculated with the formula for the ellipse area, since lesions had an approximately elliptical shape (Dennert et al., 2019; Meyer et al., 2019). At the end of the experiment, the final lesion area was recorded and used for further analysis. For the seedlings that died during the experiment, the lesion area at the time point of death was used for further analysis.
At the end of the experiment or at the time point of seedling death, all lesions were sampled to re-isolate the inoculated Cryphonectria species. The outer part of the bark was removed with a sterile scalpel in a sterile bench and small pieces (approx. 4 × 4 mm) of the necrotic tissue were placed on 1.5% water agar with 40 mg/L streptomycin. The plates were incubated at room temperature in the dark and after 5 days, growing colonies were transferred on PDA plates. The identity of the Cryphonectria species grown on the PDA plates was assessed visually from the culture morphology using reference cultures for each species.
Dormant Stem Experiment
Dormant stems of C. sativa, Q. robur, and F. sylvatica (3–5 cm diameter) were obtained from Swiss forests. The stems were cut in 50 cm long segments and ends were sealed with paraffin to avoid desiccation. The Cryphonectria spp. isolates were cultured and inoculated on the dormant stems with the same method as described for the seedling experiment. To avoid a stem effect on the experiment, on each stem, one randomly selected isolate of each Cryphonectria species was inoculated. Five replicate inoculations were performed for each isolate on each tree species. The stems were placed in plastic boxes on plastic supports and the boxes filled with 4L demineralized water to avoid desiccation of the stems (Bryner et al., 2012). The boxes were placed at 25°C in the dark for 2 weeks. Afterward, the bark around the inoculation point was removed using a scalpel and the length and width of the lesions that had formed was measured. Since the lesions were approximately elliptical, their area was calculated by using the formula for the ellipse area.
Data Analysis
Data were analyzed with R version 3.4.1 (R Core Team, 2014). Both in the seedling experiment and in the dormant stem experiment, differences between host-pathogen combinations were analyzed with a linear model with tree species and Cryphonectria species as factors. The isolates were nested within the Cryphonectria species. In the greenhouse experiment, one C. naterciae isolate (CO679) had to be excluded from the analyses because it was mixed up with a C. parasitica isolate. In the dormant stem experiment, this error was corrected and the measurements for C. naterciae CO679 could be included in the analysis.
Results
Seedling Experiment
Mortality was only observed on chestnut seedlings inoculated with C. parasitica (13 of 15 seedlings died before the end of the experiment). Moreover, this host-pathogen combination resulted in significantly greater lesion areas (average area for single isolates ranged from 26.0 to 34.4 cm2) at the end of the experiment than all other combinations (Figures 1, 2). On oak and beech, no mortality was observed and lesion growth was limited, with the smallest lesions measured on beech for all three Cryphonectria species (Figure 2). Although C. naterciae and C. japonica produced significantly smaller bark lesions on chestnut than C. parasitica (Figure 1), they could establish on the seedlings and at the end of the experiment were successfully re-isolated from 80–100% of the lesions (Figure 3). The re-isolations were successful from 20–60% of the lesions on oak for C. parasitica, from 40–60% of the lesions for C. naterciae and from 0–20% of the lesions for C. japonica (Figure 3). From beech, the proportion of re-isolations was smaller than those from oak, varying from 0 to 40% of lesions for all three Cryphonectria species.
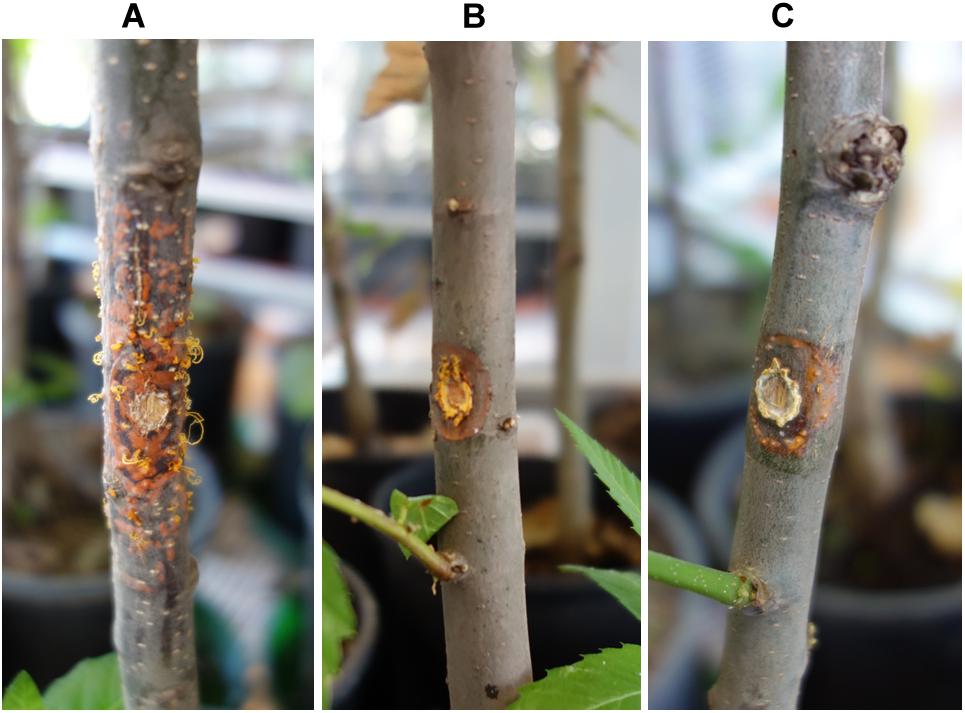
Figure 1. Bark lesions on the stems of two-year-old Castanea sativa seedlings caused by Cryphonectria parasitica (A), C. naterciae (B), and C. japonica (C) 1.5 months after inoculation. Abundant asexual sporulation is visible on the seedling inoculated with C. parasitica (A). Stems were artificially inoculated with one disk (5 mm diameter) taken from a pure culture of each Cryphonectria species grown on PDA.
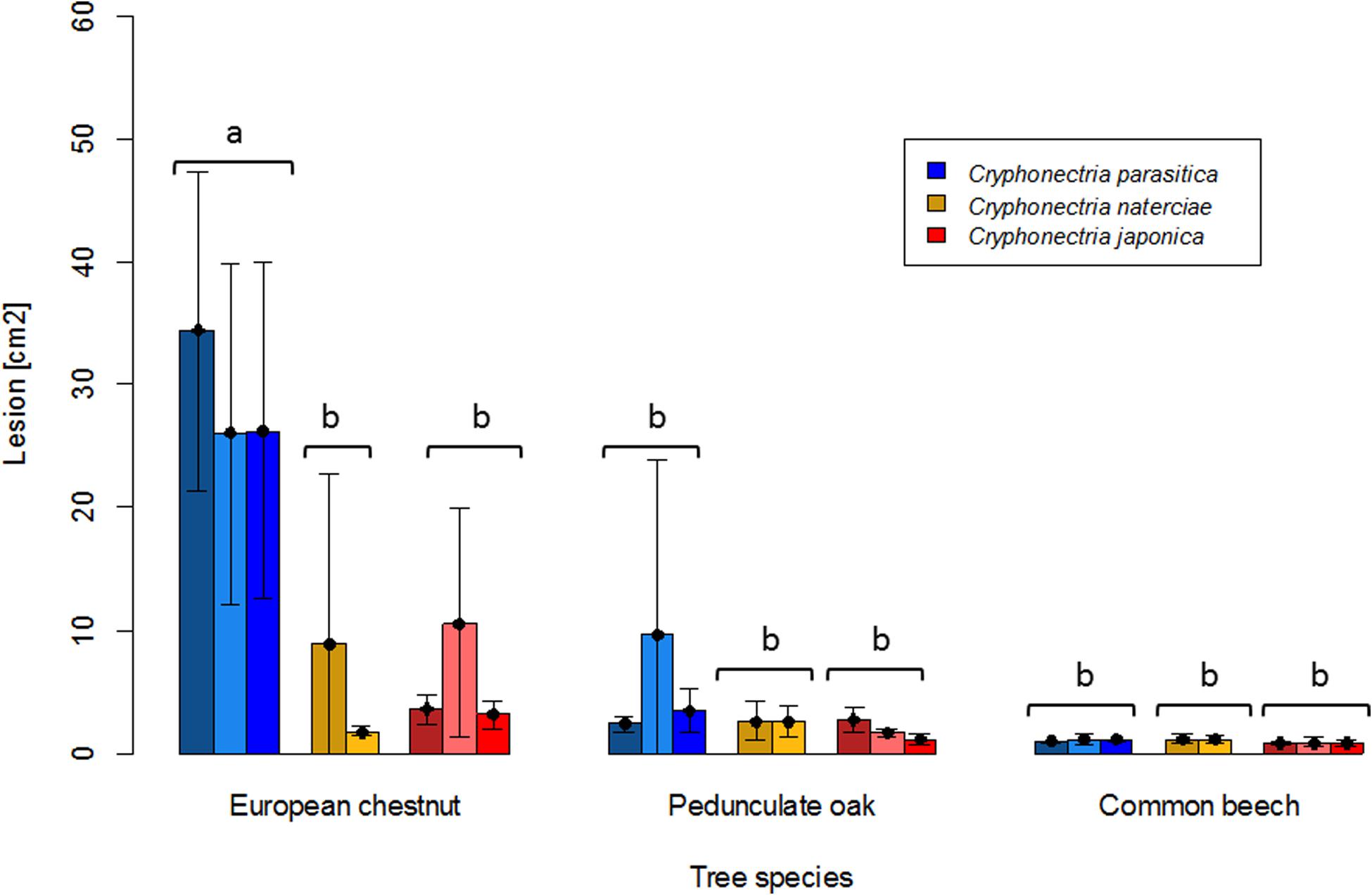
Figure 2. Barplots of lesion sizes caused by Cryphonectria parasitica (blue), C. naterciae (yellow), and C. japonica (red) on the bark of European chestnut (Castanea sativa), pedunculate oak (Quercus robur) and common beech (Fagus sylvatica). Three isolates of Cryphonectria parasitica and C. japonica and two isolates of C. naterciae were tested in the experiment. Each bar shows the average value for one isolate (five seedlings per isolate were inoculated). Black lines show standard deviations. Letters show significant differences between combinations of Cryphonectria species and tree species (Linear model with Tukey post hoc test, p < 0.05). Data for the single isolates are shown in Supplementary Table S1
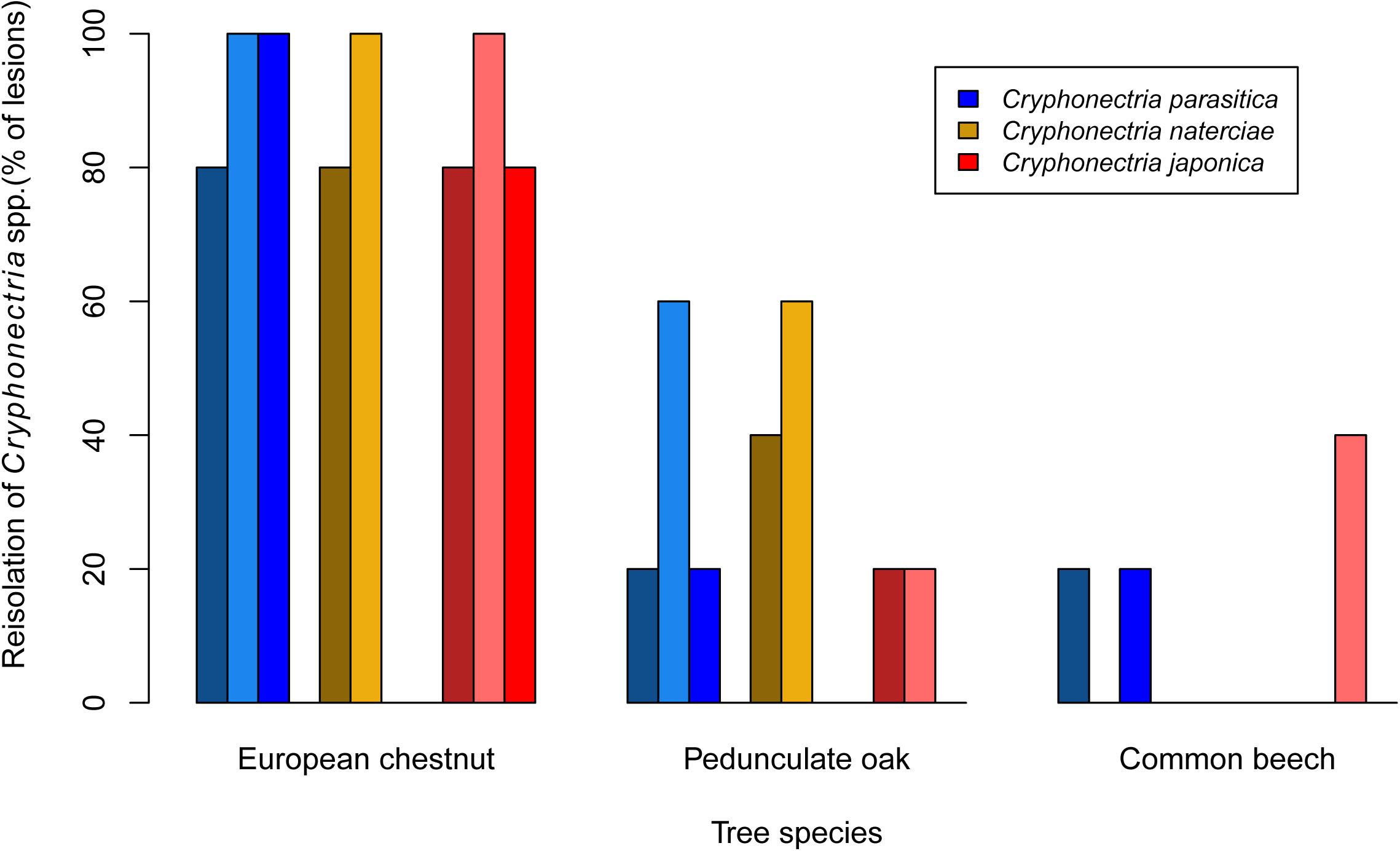
Figure 3. Barplots showing the proportion of bark lesions from which Cryphonectria parasitica (blue), C. naterciae (yellow), and C. japonica (red) could be re-isolated 4 months after artificial inoculation. Each bar represents one isolate (five seedlings per isolate were used, i.e., the proportion of lesions from which re-isolations were successful is 100% if Cryphonectria sp. could be re-isolated from all five lesions).
Dormant Stem Experiment
In the dormant stem experiment, where the three Cryphonectria species were inoculated on chestnut, oak and beech stems to assess saprophytic growth, the results were similar to the seedling experiment for oak and beech. None of the three Cryphonectria species could form lesions on these two tree species (Figures 4, 5). For chestnut, however, the results of the stem experiment differed from those of the seedling experiment, with all three Cryphonectria species forming bark lesions on the inoculated stems (Figures 4, 5). The lesions caused by C. parasitica and C. naterciae were of similar size (C. parasitica average: 47.1 cm2, C. naterciae average: 52.0 cm2), while the lesions caused by C. japonica were significantly smaller (average: 22.2 cm2), but were nonetheless significantly greater than the lesions induced by all three species on oak and beech (Figure 5). Considering the single isolates, in all the three Cryphonectria species there seemed to be no clear correlation between the growth on living seedlings and on dormant stems. However, this could not be statistically analyzed since the number of isolates per species was too low.
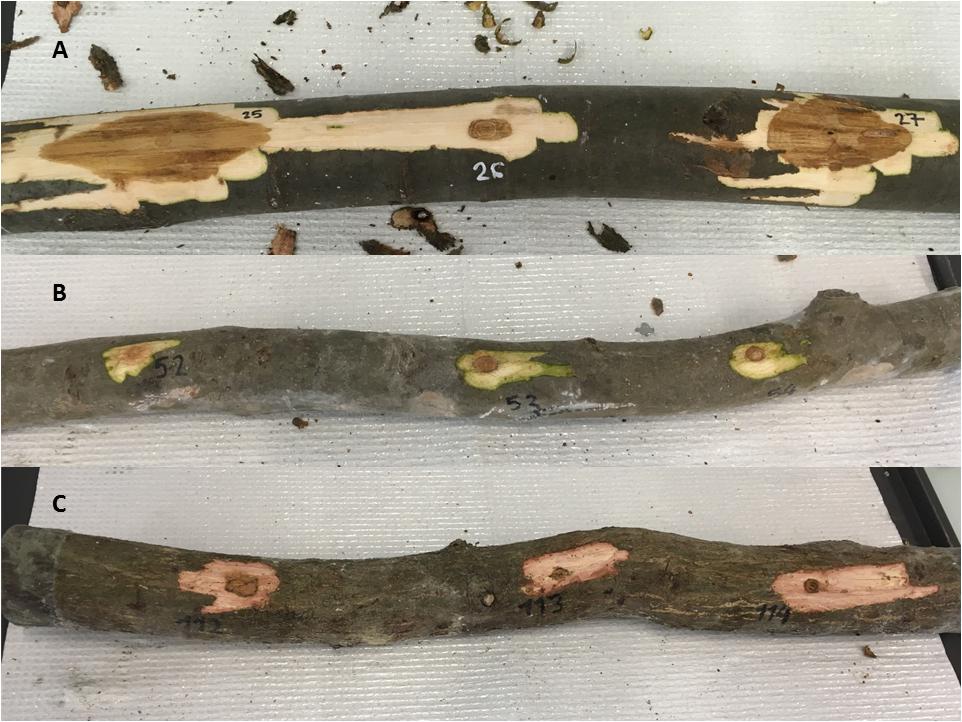
Figure 4. Bark lesions on dormant Castanea sativa (A), Fagus sylvatica (B) and Quercus robur (C) stems obtained after artificial inoculation of Cryphonectria parasitica, C. naterciae and C. japonica. To avoid a stem effect, each stem was inoculated with one randomly selected isolate of each Cryphonectria species. For example on stem A, lesion 25 was produced by C. parasitica, lesion 26 by C. japonica, and lesion 27 by C. naterciae.
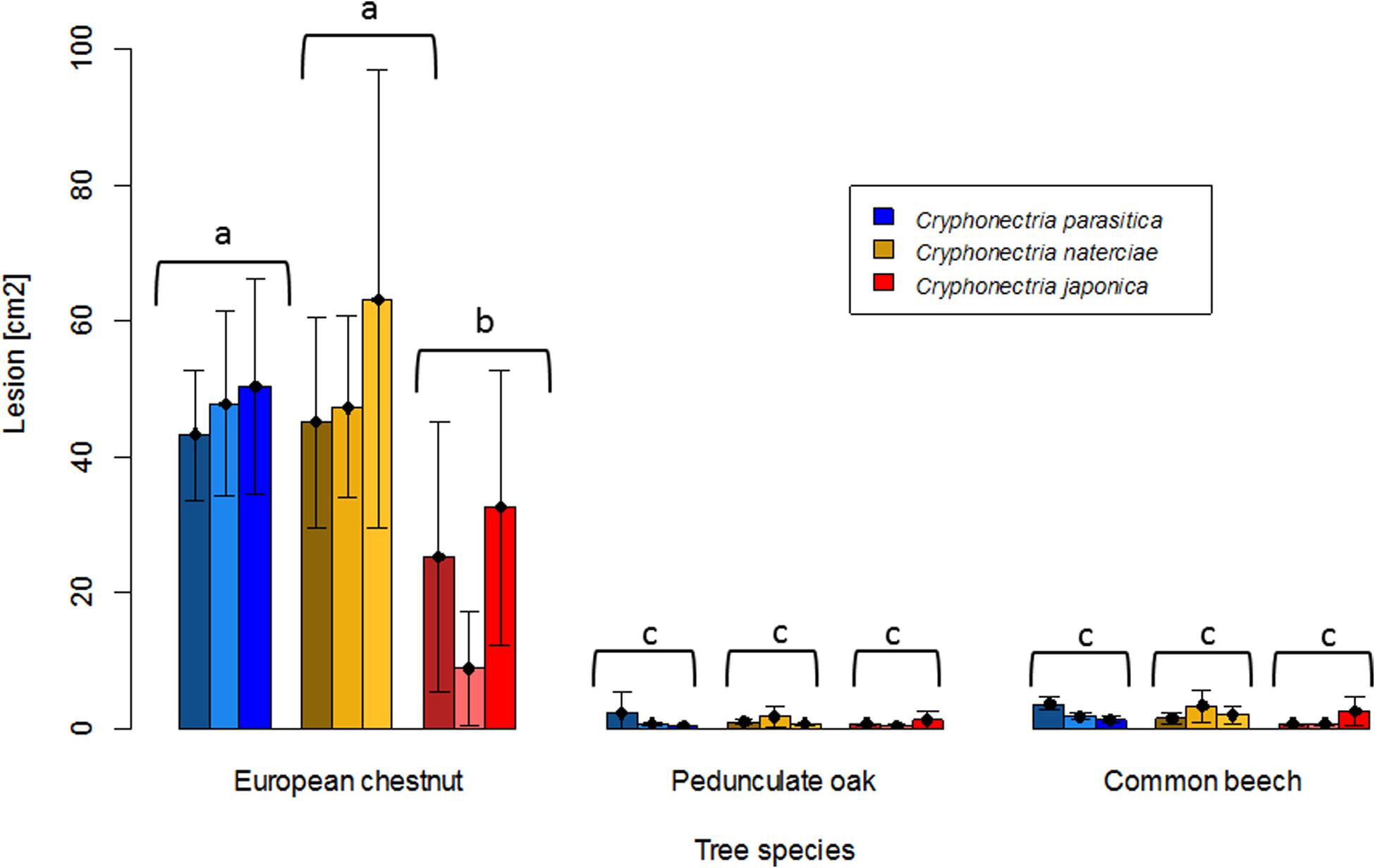
Figure 5. Barplot of lesion sizes obtained on dormant stems of European chestnut (Castanea sativa), pedunculate oak (Quercus robur), and common beech (Fagus sylvatica) after artificial inoculation of Cryphonectria parasitica (blue), C. naterciae (yellow), and C. japonica (red). Each bar represents the mean lesion size obtained for one isolate (five replicates). Black bars represent standard deviations. Letters show significant differences between combinations of Cryphonectria species and tree species (Linear model with Tukey post hoc test, p < 0.05). Data for the single isolates are shown in Supplementary Table S1
Discussion
To avoid the introduction of new invasive pests, including pathogens, preventive strategies have to be adopted, like border controls and regulated plant trade (Klapwijk et al., 2016). The implementation of such measures, however, implies the identification of potential invasive pests before they become invasive, i.e., before they are moved to a new range. This is particularly challenging because many invasive pests in their native range are harmless endophytes not causing any visible symptoms on their host (e.g., the ash dieback fungus Hymenoscyphus fraxineus Baral, Queloz, Hosoya in Asia; Cleary et al., 2016). Here, we used inoculation experiments to test the pathogenicity of two Cryphonectria species closely related to one of the most lethal invasive forest pathogens, namely Cryphonectria parasitica. One species (C. japonica) is still not present in Europe, whereas the other (C. naterciae) seems to be currently restricted to Portugal and some areas in Italy (Bragança et al., 2011; Pinna et al., 2019). Inoculation experiments have previously been used to identify potential new host species of other invasive plant pathogens. For example, Tooley and Kyde (2007) tested the susceptibility to Phytophthora ramorum Werres, De Cock, Man of different potential new host species in the eastern United States. Similarly, Carnegie and Lidbetter (2012), assessed the pathogenicity of an invasive rust species in Australia by inoculating a selection of potential host trees.
Our results show large differences in pathogenicity among the three Cryphonectria species included in the study. Differences in lifestyles among congeneric species were previously reported in other fungal genera, including Alternaria (Thomma, 2003), Diaporthe (Gomes et al., 2013), and Fusarium (Kuldau and Yates, 2000; Padhi et al., 2016). Although the Asian species C. parasitica is an aggressive pathogen on non-Asian Castanea species (Rigling and Prospero, 2018), C. japonica, which is also native to Asia, is likely not pathogenic on European chestnut, nor on European beech and pedunculate oak. Indeed, inoculations of C. japonica on these three tree species did only result in very small bark lesions. Moreover, fruiting bodies were predominantly observed from C. parasitica on chestnut. Thus, even if this species would be accidentally introduced into Europe, it would most likely not result in severe damage on the above mentioned tree species. Similarly, C. naterciae, a European species present in Portugal and Italy, does not seem to represent a real threat for chestnut, beech and oak, in the case it would be moved outside of its current distribution range.
Although C. naterciae and C. japonica were not pathogenic on seedlings of chestnut and of the other two Fagaceae species, they were able to colonize the bark of freshly cut dormant C. sativa stems, and could be successfully re-isolated from the majority of chestnut seedlings in the greenhouse experiment. This suggests that both species may be able to behave as secondary pathogens on C. sativa trees already weakened, for example, by abiotic stresses like drought (Desprez-Loustau et al., 2006). Considering the climatic changes predicted for European chestnut growing regions, like an increase of extreme weather events and of drought periods (Giorgi and Lionello, 2008; Mariotti et al., 2015), it would be worth to further investigate the effect of such tree stressors on the pathogenicity of these two Cryphonectria species. As observed in C. parasitica (Prospero et al., 2006; Meyer et al., 2019), the ability of C. naterciae and C. japonica to colonize and saprophytically grow on recently dead chestnut stems could contribute in building up a reservoir of inoculum. This could help both species to become successfully established and spread in European forests.
Our study confirms the potential of artificial inoculation experiments to assess the pathogenicity of plant-associated fungi toward selected host species. The tested fungi can include known pathogens, but also saprotrophs or endophytes, which may spread with plants for planting and represent a threat for non-native hosts. This approach is particularly helpful in combination with sentinel plantings, where host species are grown outside their natural distribution range to detect potential new pathogens (Morales-Rodríguez et al., 2019). Once such pathogens have been identified, their host range can be determined by conducting inoculation experiments with living plants in the greenhouse. However, invasiveness of a fungal pathogen (i.e., the ability to arrive, spread beyond its introduction site and become established in a new location; Desprez-Loustau et al., 2007) is not only a function of its pathogenicity. Other phenotypic traits, such as sporulation and saprotrophic ability may play an important role. Moreover, environmental conditions strongly influence plant diseases, therefore climate changes can also be considered drivers of disease outbreaks (Sturrock et al., 2011). Thus, to obtain a full view it is necessary to experimentally assess as many traits as possible. In our specific case, artificial inoculations showed that an accidental introduction to Europe of C. japonica or the spread within Europe of C. naterciae would most likely have much less devastating consequences than the invasion by C. parasitica. Nonetheless, the ability of both species to saprotrophically colonize fresh chestnut wood may suggest that they could become established in chestnut forests and eventually infect weakened chestnut trees or other hosts not tested in this study.
Data Availability Statement
The datasets generated for this study are available on request to the corresponding author.
Author Contributions
FD collected and analyzed the data of the dormant stem experiment and wrote the first version of the manuscript, DR planned the seedling experiment together with JM and SP and performed the re-isolations from the inoculated seedlings, JM performed the seedling experiment with SP, CS analyzed the data of the seedling experiment, EA performed the dormant stem experiment, SP planned the experiments and supervised the study. All authors contributed to the final version of the manuscript.
Funding
This project was funded by the Swiss Federal Institute for Forest, Snow and Landscape Research (WSL).
Conflict of Interest
The authors declare that the research was conducted in the absence of any commercial or financial relationships that could be construed as a potential conflict of interest.
Acknowledgments
We thank Helena Bragança for providing the C. naterciae isolates and Michael Milgroom for providing the C. japonica isolates used in this study. We also thank Hélène Blauenstein for technical support in the WSL biosafety facility.
Supplementary Material
The Supplementary Material for this article can be found online at: https://www.frontiersin.org/articles/10.3389/ffgc.2020.00052/full#supplementary-material
References
Adamčíková, K., Kobza, M., and Juhásová, G. (2010). Characteristics of the Cryphonectria parasitica isolated from Quercus in Slovakia. For. Pathol. 40, 443–449. doi: 10.1111/j.1439-0329.2009.00618.x
Anagnostakis, S. L. (1987). Chestnut blight: the classical problem of an introduced pathogen. Mycologia 79, 23–37. doi: 10.2307/3807741
Anagnostakis, S. L. (1992). Measuring resistance of chestnut trees to chestnut blight. Can. J. For. Res. 22, 568–571. doi: 10.1139/x92-075
Biraghi, A. (1950). Il cancro della corteccia può colpire anche le querce? Monti e boschi 3, 129–132.
Bragança, H., Rigling, D., Diogo, E., Capelo, J., Phillips, A., and Tenreiro, R. (2011). Cryphonectria naterciae: a new species in the Cryphonectria-Endothia complex and diagnostic molecular markers based on microsatellite-primed PCR. Fungal Biol. 115, 852–861. doi: 10.1016/j.funbio.2011.06.014
Brasier, C. M. (2008). The biosecurity threat to the UK and global environment from international trade in plants. Plant Pathol. 57, 792–808. doi: 10.1111/j.1365-3059.2008.01886.x
Bryner, S. F., Rigling, D., and Brunner, P. C. (2012). Invasion history and demographic pattern of Cryphonectria hypovirus 1 across European populations of the chestnut blight fungus. Ecol. Evol. 2, 3227–3241. doi: 10.1002/ece3.429
Carnegie, A. J., and Lidbetter, J. R. (2012). Rapidly expanding host range for Puccinia psidii sensu lato in Australia. Australas Plant Pathol. 41, 13–29. doi: 10.1007/s13313-011-0082-6
Cleary, M., Nguyen, D., Marčiulynienë, D., Berlin, A., Vasaitis, R., and Stenlid, J. (2016). Friend or foe? Biological and ecological traits of the European ash dieback pathogen Hymenoscyphus fraxineus in its native environment. Sci. Rep. 6:21895. doi: 10.1038/srep21895
Dallavalle, E., and Zambonelli, A. (1999). Epidemiological role of strains of Cryphonectria parasitica isolated from hosts other than chestnut. Eur. J. For. Pathol. 29, 97–102. doi: 10.1046/j.1439-0329.1999.00140.x
De Vienne, D. M., Hood, M. E., and Giraud, T. (2009). Phylogenetic determinants of potential host shifts in fungal pathogens. J. Evol. Biol. 22, 2532–2541. doi: 10.1111/j.1420-9101.2009.01878.x
Dennert, F., Meyer, J. B., Rigling, D., and Prospero, S. (2019). Assessing the phytosanitary risk posed by an intraspecific invasion of Cryphonectria parasitica in Europe. Phytopathology 109, 2055–2063. doi: 10.1094/phyto-06-19-0197-r
Desprez-Loustau, M.-L., Marçais, B., Nageleisen, L.-M., Piou, D., and Vannini, A. (2006). Interactive effects of drought and pathogens in forest trees. Ann. For. Sci. 63, 597–612. doi: 10.1051/forest:2006040
Desprez-Loustau, M.-L., Robin, C., Buée, M., Courtecuisse, R., Garbaye, J., Suffert, F., et al. (2007). The fungal dimension of biological invasions. Trends Ecol. Evol. 22, 472–480. doi: 10.1016/j.tree.2007.04.005
Dutech, C., Barrčs, B., Bridier, J., Robin, C., Milgroom, M. G., and Ravigné, V. (2012). The chestnut blight fungus world tour: successive introduction events from diverse origins in an invasive plant fungal pathogen. Mol. Ecol. 21, 3931–3946. doi: 10.1111/j.1365-294X.2012.05575.x
Ghelardini, L., Luchi, N., Pecori, F., Pepori, A. L., Danti, R., Della Rocca, G., et al. (2017). Ecology of invasive forest pathogens. Biol. Invasions 19, 3183–3200. doi: 10.1007/s10530-017-1487-0
Gilbert, G. S., Briggs, H. M., and Magarey, R. (2015). The impact of plant enemies shows a phylogenetic signal. PLoS One 10:e0123758. doi: 10.1371/journal.pone.0123758
Gilbert, G. S., and Webb, C. O. (2007). Phylogenetic signal in plant pathogen–host range. Proc. Natl. Acad. Sci. U.S.A. 104, 4979–4983. doi: 10.1073/pnas.0607968104
Giorgi, F., and Lionello, P. (2008). Climate change projections for the Mediterranean region. Glob. Planet Chang. 63, 90–104. doi: 10.1016/j.gloplacha.2007.09.005
Gomes, R. R., Glienke, C., Videira, S. I. R., Lombard, L., Groenewald, J. Z., and Crous, P. W. (2013). Diaporthe: a genus of endophytic, saprobic and plant pathogenic fungi. Persoonia 31, 1–41. doi: 10.3767/003158513X666844
Gryzenhout, M., Wingfield, B., and Wingfield, M. (2009). Taxonomy, Phylogeny and Ecology of Bark-Infecting and Tree Killing Fungi in the Cryphonectriaceae. Saint Paul, MI: American Phytopathological Society.
Haltofová, P., Jankovský, L., and Palovčíková, D. (2005). New finds of Cryphonectria parasitica and the first record of chestnut blight on red oak Quercus rubra L. in the Czech Republic. J. For. Sci. 51, 256–258.
Jiang, N., Fan, X. L., and Tian, C. M. (2019). Identification and pathogenicity of Cryphonectriaceae species associated with chestnut canker in China. Plant Pathol. 68, 1132–1145. doi: 10.1111/ppa.13033
Klapwijk, M. J., Hopkins, A. J. M., Eriksson, L., Pettersson, M., Schroeder, M., Lindelöw, Å., et al. (2016). Reducing the risk of invasive forest pests and pathogens: combining legislation, targeted management and public awareness. Ambio 45, 223–234. doi: 10.1007/s13280-015-0748-3
Kuldau, G. A., and Yates, I. E. (2000). “Evidence for Fusarium endophytes,” in Microbial Endophytes, eds C. W. Bacon and J. F. White (New York, NY: Marcel Dekker), 85.
Liu, Y. C., Linder-Basso, D., Hillman, B. I., Kaneko, S., and Milgroom, M. G. (2003). Evidence for interspecies transmission of viruses in natural populations of filamentous fungi in the genus Cryphonectria. Mol. Ecol. 12, 1619–1628. doi: 10.1046/j.1365-294x.2003.01847.x
Mariotti, A., Pan, Y., Zeng, N., and Alessandri, A. (2015). Long-term climate change in the Mediterranean region in the midst of decadal variability. Clim. Dyn. 44, 1437–1456. doi: 10.1007/s00382-015-2487-3
Meyer, J. B., Chalmandrier, L., Fässler, F., Schefer, C., Rigling, D., and Prospero, S. (2019). Role of fresh dead wood in the epidemiology and the biological control of the chestnut blight fungus. Plant Dis. 103, 430–438. doi: 10.1094/pdis-05-18-0796-re
Morales-Rodríguez, C., Anslan, S., Auger-Rozenberg, M.-A., Augustin, S., Baranchikov, Y., Bellahirech, A., et al. (2019). Forewarned is forearmed: harmonized approaches for early detection of potentially invasive pests and pathogens in sentinel plantings. NeoBiota 47, 95–123. doi: 10.3897/neobiota.47.34276
Myburg, H., Gryzenhout, M., Wingfield, B. D., Milgroom, M. G., Kaneko, S., and Wingfield, M. J. (2004). DNA sequence data and morphology define Cryphonectria species in Europe, China, and Japan. Can. J. Bot. 82, 1730–1743. doi: 10.1139/b04-135
Nakabonge, G., Gryzenhout, M., Wingfield, B. D., Wingfield, M. J., and Roux, J. (2007). Genetic diversity of Chrysoporthe cubensis in eastern and southern Africa. S. Afr. J. Sci. 103, 261–264.
Padhi, S., Panda, M. K., Das, D., and Tayung, K. (2016). ITS2 RNA secondary structure analysis reveals close affinity between endophytic and pathogenic fungi: a case study in Fusarium species. Ann. Microbiol. 66, 625–633. doi: 10.1007/s13213-015-1142-x
Pegg, G. S., Gryzenhout, M., O’dwyer, C., Drenth, A., and Wingfield, M. J. (2010). The Eucalyptus canker pathogen Chrysoporthe cubensis discovered in eastern Australia. Australas Plant Pathol. 39, 343–349. doi: 10.1071/AP10004
Pinna, C., Linaldeddu, B. T., Deiana, V., Maddau, L., Montecchio, L., and Lentini, A. (2019). Plant pathogenic fungi associated with Coraebus florentinus (Coleoptera: Buprestidae) attacks in declining oak forests. Forests 10:488. doi: 10.3390/f10060488
Prospero, S., and Cleary, M. (2017). Effects of host variability on the spread of invasive forest diseases. Forests 8:80. doi: 10.3390/f8030080
Prospero, S., Conedera, M., Heiniger, U., and Rigling, D. (2006). Saprophytic activity and sporulation of Cryphonectria parasitica on dead chestnut wood in forests with naturally established hypovirulence. Phytopathology 96, 1337–1344. doi: 10.1094/phyto-96-1337
Prospero, S., and Rigling, D. (2012). Invasion genetics of the chestnut blight fungus Cryphonectria parasitica in Switzerland. Phytopathology 102, 73–82. doi: 10.1094/PHYTO-02-11-0055
R Core Team (2014). R: A Language and Environment for Statistical Computing. Vienna: R Foundation for Statistical Computing.
Rigling, D., and Prospero, S. (2018). Cryphonectria parasitica, the causal agent of chestnut blight: invasion history, population biology and disease control. Mol. Plant Pathol. 19, 7–20. doi: 10.1111/mpp.12542
Rodas, C. A., Gryzenhout, M., Myburg, H., Wingfield, B. D., and Wingfield, M. J. (2005). Discovery of the Eucalyptus canker pathogen Chrysoporthe cubensis on native Miconia (Melastomataceae) in Colombia. Plant Pathol. 54, 460–470. doi: 10.1111/j.1365-3059.2005.01223.x
Santini, A., Ghelardini, L., De Pace, C., Desprez-Loustau, M. L., Capretti, P., Chandelier, A., et al. (2013). Biogeographical patterns and determinants of invasion by forest pathogens in Europe. New Phytol. 197, 238–250. doi: 10.1111/j.1469-8137.2012.04364.x
Stenlid, J., and Oliva, J. (2016). Phenotypic interactions between tree hosts and invasive forest pathogens in the light of globalization and climate change. Philos. Trans. R. Soc. B 371:20150455. doi: 10.1098/rstb.2015.0455
Sturrock, R. N., Frankel, S. J., Brown, A. V., Hennon, P. E., Kliejunas, J. T., Lewis, K. J., et al. (2011). Climate change and forest diseases. Plant Pathol. 60, 133–149. doi: 10.1111/j.1365-3059.2010.02406.x
Thomma, B. P. H. J. (2003). Alternaria spp.: from general saprophyte to specific parasite. Mol. Plant Pathol. 4, 225–236. doi: 10.1046/j.1364-3703.2003.00173.x
Tooley, P. W., and Kyde, K. L. (2007). Susceptibility of some eastern forest species to Phytophthora ramorum. Plant Dis. 91, 435–438. doi: 10.1094/pdis-91-4-0435
Keywords: Castanea sativa, Fagus sylvatica, Quercus robur, invasive pathogen, phenotyping, inoculation experiments, virulence
Citation: Dennert F, Rigling D, Meyer JB, Schefer C, Augustiny E and Prospero S (2020) Testing the Pathogenic Potential of Cryphonectria parasitica and Related Species on Three Common European Fagaceae. Front. For. Glob. Change 3:52. doi: 10.3389/ffgc.2020.00052
Received: 06 February 2020; Accepted: 09 April 2020;
Published: 05 May 2020.
Edited by:
Alberto Santini, Institute for Sustainable Plant Protection (CNR), ItalyReviewed by:
Giorgio Maresi, Fondazione Edmund Mach, ItalyJennifer Gene Klutsch, University of Alberta, Canada
Copyright © 2020 Dennert, Rigling, Meyer, Schefer, Augustiny and Prospero. This is an open-access article distributed under the terms of the Creative Commons Attribution License (CC BY). The use, distribution or reproduction in other forums is permitted, provided the original author(s) and the copyright owner(s) are credited and that the original publication in this journal is cited, in accordance with accepted academic practice. No use, distribution or reproduction is permitted which does not comply with these terms.
*Correspondence: Francesca Dennert, ZnJhbmNlc2NhLmRlbm5lcnRAd3NsLmNo