- 1IRET-CNR, Firenze, Italy
- 2Institute of Ecology, Key Laboratory of Agrometeorology of Jiangsu Province, School of Applied Meteorology, Nanjing, China
- 3Research Faculty of Agriculture, School of Agriculture, Hokkaido University, Sapporo, Japan
Ozone (O3) risk assessment for the protection of forests requires species-specific critical levels (CLs), based on either O3 concentrations (AOT40) or stomatal uptake (PODY) accumulation over the growing season. Larch (Larix sp.) is a genus with O3-susceptible species, widely distributed in the northern hemisphere and with global economic importance. We analyzed published and unpublished data of Japanese larch (Larix kaempferi) and its hybrid F1 (Larix gmelinii var. japonica × L. kaempferi) stomatal responses for developing a parameterization of stomatal conductance model and estimating PODY-based CLs with two Y thresholds, that is, 0 and 1 nmol m–2 s–1 projected leaf area (PLA). In parallel, we estimated AOT40-based CLs. The results show that the AOT40-based CLs for a 2% and 4% biomass loss in Japanese larch were 5.79 and 11.59 ppm h, that is, higher than those in hybrid larch F1 (2.18 and 4.36 ppm h AOT40), suggesting a higher O3 susceptibility of the hybrid. However, the use of PODY reconciled the species-specific differences, because the CLs were similar, that is, 9.40 and 12.00 mmol m–2 POD0 and 2.21 and 4.31 mmol m–2 POD1 in Japanese larch versus 10.44 and 12.38 mmol m–2 POD0 and 2.45 and 4.19 mmol m–2 POD1 in the hybrid, for 2% and 4% biomass loss, respectively. Overall, the CLs were lower than those in other forest species, which suggests a relatively high susceptibility of these larches. These results will inform environmental policy-makers and modelers about larch susceptibility to O3.
Introduction
Tropospheric ozone (O3) is the most widespread phytotoxic air pollutant (Mills et al., 2018). In the period 1995–2014, control measures were effective in North America and Europe, as indicated by a decrease of O3 concentrations, while a significant increase in O3 concentrations occurred in East Asia (Chang et al., 2017; Mills et al., 2018). Ozone has a strong oxidative capacity and may cause severe injury to forests (Paoletti, 2007; Li et al., 2017). To assess O3 risk to forests, different metrics have been developed (Lefohn et al., 2018). One of the most common metrics is AOT40, that is, the accumulated exposure over an hourly threshold of 40 ppb during the growing season, although there is a general consensus that the accumulated stomatal O3 flux – or phytotoxic ozone dose (POD) – is more biologically meaningful as it estimates the amount of O3 actually entering the plants through the stomata (Paoletti and Manning, 2007). A flux threshold Y below which O3 uptake is not expected to be injurious to plants has been postulated. For all tree species, a uniform threshold of Y = 1 nmol m–2 s–1 projected leaf area (PLA) was recommended by the Convention on Long-Range Transboundary Air Pollution (CLRTAP, 2017) based on Büker et al. (2015). For easier calculation, a Y threshold of 0 nmol m–2 s–1 PLA was also recommended, if we assume that all O3 molecules induce a physiological reaction after uptake (De Marco et al., 2015, 2016; Anav et al., 2016), which is a plausible assumption in the light of low-dose adaptive responses (Agathokleous et al., 2019).
For the protection of susceptible vegetation from O3, critical levels (CLs) are recommended, defined as the “concentration, cumulative exposure or cumulative stomatal flux of atmospheric pollutants above which direct adverse effects on susceptible vegetation may occur according to present knowledge” (CLRTAP, 2017). CLs are derived for either a 2% (Norway spruce) reduction or a 4% (beech/birch, Mediterranean deciduous and evergreen species) reduction in annual new growth (based on aboveground, root, or whole-tree biomass) of young trees up to 10 years old. AOT40-based CLs for tree biomass loss (5%) are available for Fagus sylvatica and Betula pendula in a previous version of the ICP Vegetation manual (CLRTAP, 2014; AOT40-based CLs are not included in the latest version) and for some other species in the literature (e.g., 18 Japanese species including two larch species, Yamaguchi et al., 2011; Populus deltoides cv. “55/56” × P. deltoides cv. “Imperial” and Populus euramericana cv. “74/76,” Shang et al., 2017). Stomatal flux-based CLs are available for F. sylvatica, B. pendula, Picea abies, Quercus faginea, Quercus pyrenaica, Quercus robur, Quercus ilex, Ceratonia siliqua, and Pinus halepensis in the ICP Vegetation manual (CLRTAP, 2017) and for few other species in the literature (Zelkova serrata, Hoshika et al., 2012; Quercus pubescens, Hoshika et al., 2018b; Pinus pinea, Hoshika et al., 2017; hybrid poplars, Zhang et al., 2018; Feng et al., 2019b; Fagus crenata, Quercus serrata, Quercus mongolica var. crispula, and Betula platyphylla var. japonica, Yamaguchi et al., 2019). For estimating PODY (phytotoxic ozone dose above a threshold Y nmol m–2 s–1)-based CLs, a species-specific parameterization of the stomatal flux or DO3SE model is required (Emberson et al., 2000; Büker et al., 2012). There is a need of more species-specific CLs for biomass loss in forest species, especially for forest species in Asia, where elevated O3 pollution levels are a serious risk for forests at present (Li et al., 2017; Mills et al., 2018; Feng et al., 2019a).
Larch (Larix sp.) is a widely distributed genus (Pinaceae family) with global economic importance, which includes some of the few deciduous conifer species. Larch is among the dominant tree species of northern hemisphere boreal forests. Hence, its natural distribution range is very wide and spans from Siberia to Canada, passing through Europe, mountainous China, and Japan. Larches provide high-quality wood and are commercially valuable (Bardak et al., 2019). As any pioneer species, larches have a relatively high growth rate and stomatal conductance (Streit et al., 2014; Agathokleous et al., 2017; Hoshika et al., 2018c). Although their susceptibility to O3 has been investigated in several papers (Wieser and Havranek, 1996; Matsumura, 2001; Watanabe et al., 2006; Koike et al., 2012; Agathokleous et al., 2017; Sugai et al., 2018, 2019), a comprehensive risk assessment including parameterization of the stomatal conductance model and definition of CLs for biomass losses is missing. Previous studies focused on the biomass responses to O3 of Japanese larch (Larix kaempferi) and its hybrid F1 (Larix gmelinii var. japonica × L. kaempferi). Hybrid F1 displays heterosis and is important for timber production and afforestation due to more desirable characteristics compared to its parents, with a significant superiority in terms of growth rates (Ryu et al., 2009; Kita et al., 2009; Agathokleous et al., 2017; Sugai et al., 2018). A question arises whether hybrid clones, selected for fast-growing capacities, are representative of natural forest responses to O3 when used in manipulative experiments (e.g., Di Baccio et al., 2008; Hu et al., 2015; Dusart et al., 2019; Podda et al., 2019).
Our aim was to collate published and unpublished data from previous experiments for developing a parameterization of the DO3SE model for Japanese larch and its hybrid F1 and estimating the CLs not to be exceeded for the protection of these larch species from O3. Based on published research documenting a higher O3 susceptibility of the faster-growing hybrid F1 than the slower-growing Japanese larch (Agathokleous et al., 2017; Sugai et al., 2018), we hypothesized that the CLs of hybrid F1 have a lower susceptibility than that of the wild Japanese larch.
Materials and Methods
A literature survey was conducted in Web of Science (9 December 2019), with the keywords “ozone” and “larch” or “larix” (search method: Topic). All the identified papers (n = 33 and 36 for each combination; most were duplicates) were reviewed for relevance, including whether they reported O3 and biomass data. Finally, data on O3 concentrations, exposure duration, and total biomass were collected from six published experiments carried out in open-top chambers (OTCs) (Table 1: Matsumura, 2001; Watanabe et al., 2006; Koike et al., 2012; Wang et al., 2015; Sugai et al., 2018, 2019) and used to calculate AOT40 and percentage losses of biomass relative to controls in low-O3 air. Data from combined experiments, such as O3 with either fertilization or CO2, were not included. Data of Dahurian larch (L. gmelinii var. japonica) from the same experiments were not included because of scarcity, thus being insufficient for analysis.
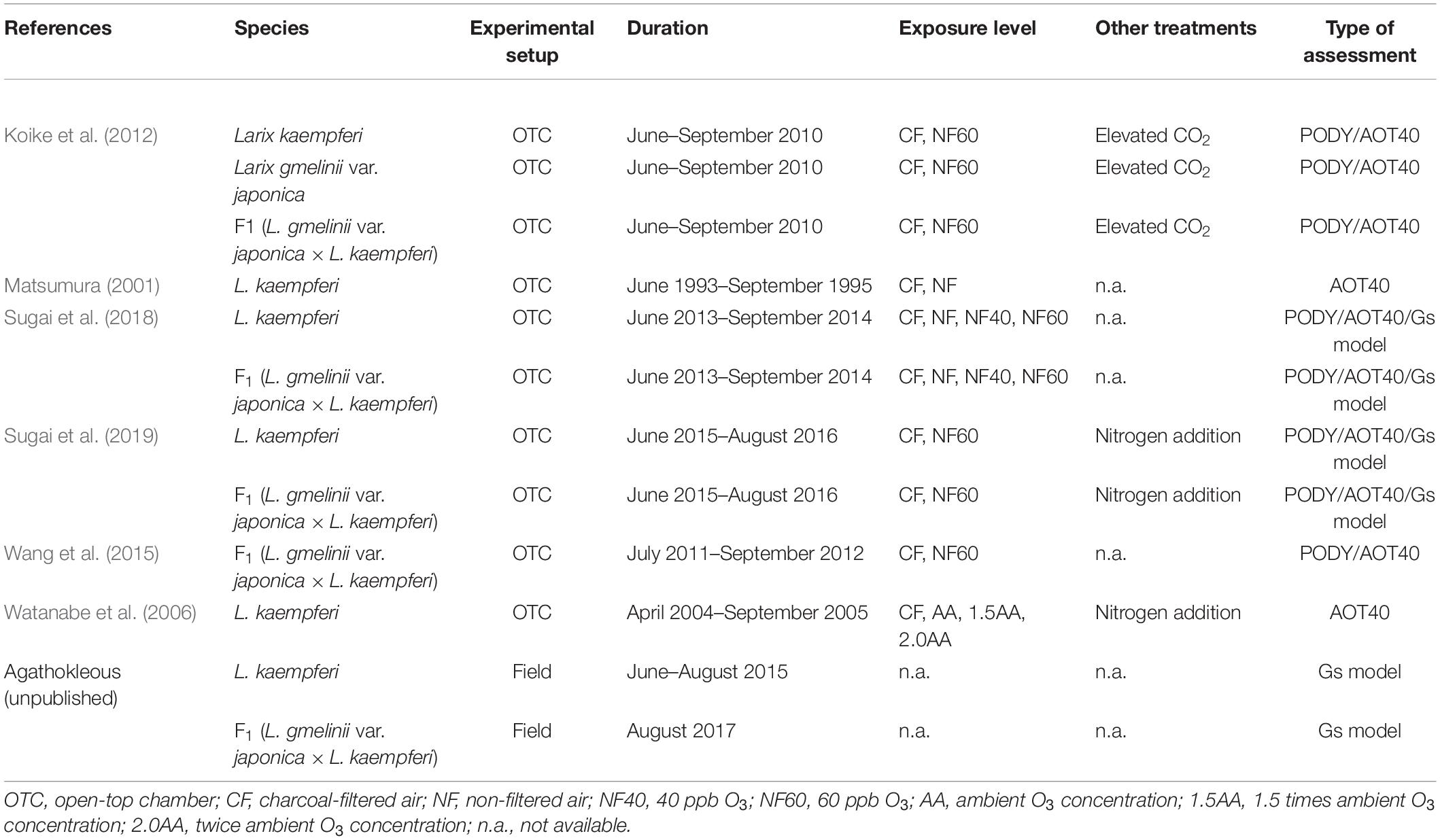
Table 1. Details of experiments from which data were obtained for the analysis (PODY, AOT40, and Gs model).
Individual measurements of stomatal conductance across a range of environmental conditions were obtained from the authors Sugai et al. (2018, 2019) and Agathokleous (unpublished). Measurements by Agathokleous (unpublished) were carried out in field-grown 2-year-old larch seedlings at the Sapporo experimental forest, Hokkaido University, in Japan (Table 1). All measurements were carried out by means of Li-Cor 6400 gas analyzers (Li-Cor Inc., Lincoln, NE, United States). As soil water content measurements were missing, we used the following simplified formula for the estimation of the stomatal conductance gsto in the DO3SE model (CLRTAP, 2017):
where gmax is the maximum stomatal conductance of either Japanese larch or its hybrid F1, fmin is the species-specific minimum stomatal conductance, and flight, ftemp, and fVPD account for the effects of photosynthetic photon flux density (PPFD), air temperature (T), and vapor pressure deficit (VPD), respectively, on stomata. Parameterization was carried out using a boundary line analysis (Alonso et al., 2008; Braun et al., 2010; Hoshika et al., 2012). First, the gsto data were divided into classes with the following stepwise increases for each variable: 200 μmol photons m–2 s–1 for PPFD (when the values were less than 200 μmol photons m–2 s–1, PPFD classes at 50 μmol photons m–2 s–1 steps were adopted), 2°C for T, and 0.2 kPa for VPD. A function was fitted against each model variable based on 95th percentile values per class of environmental factors. Values of gmax and fmin were calculated as the 95th percentile and 5th percentile, respectively (Hoshika et al., 2012; Bičárová et al., 2019). For details of flight, ftemp, and fVPD, see CLRTAP (2017).
Stomatal O3 uptake (Fst; nmol m–2 s–1) was calculated as follows:
where rc is the leaf surface resistance [= 1/(gsto + gext); s m–1] and gext is the external leaf or cuticular conductance (= 0.0004 m s–1, CLRTAP, 2017). The standard DO3SE model considers the leaf boundary layer resistance (rb):
where the factor 1.3 accounts for the difference in diffusivity between heat and O3, 150 is the empirical constant, Ld is the cross-wind leaf dimension (0.008 m for conifers, CLRTAP, 2017), and u is the wind speed. The wind speed data were not available in collected literatures. However, in OTCs, since a constant ventilation from the blowers is realized, rb is less important compared with stomatal resistance (rsto) (Unsworth et al., 1984; Uddling et al., 2004; Tuovinen et al., 2009). This is supported by the fact that the rb/rc ratio was small in the present study when assuming that rsto was rsto_min (= 1/gmax) and wind speed was constant inside a chamber (rb/rc = 0.07 and 0.06 at 1 m s–1 and 0.05 and 0.04 at 2 m s–1 of wind speed in hybrid and Japanese larch, respectively). Here, we assumed that rb was negligible for the calculation of Fst.
PODY (mmol m–2) was estimated from hourly data as follows:
where Y is a species-specific threshold of stomatal O3 uptake (nmol m–2 s–1) and Δt = 1 h is the averaging period. Fst,i is the ith hourly stomatal O3 uptake (nmol m–2 s–1), and n is the number of hours included in the calculation period. Y is subtracted from each Fst,i when Fst,i > Y. PODY was then estimated based on hourly data of air temperature, solar photosynthetic active radiation, and VPD as registered locally and accumulated over the duration of the experiments from the six papers (Table 1). Data from Matsumura (2001) and Watanabe et al. (2006) were excluded from this analysis because of missing meteorological data.
To establish PODY-based dose–response relationships, two representative values of Y (= 0 or 1 nmol m–2 s–1) were tested. This is because CLRTAP (2017) suggested POD1 to be suitable for biomass assessment in elevated O3 while several studies reported a better performance of POD0 rather than POD1 for O3 risk assessment (e.g., Sicard et al., 2016). CLs were estimated for a total biomass reduction of both 2% as suggested for deciduous species and 4% as suggested for non-Mediterranean conifer species (CLRTAP, 2017). In addition, since CLRTAP (2017) provided an AOT40-based CL corresponding to a 5% biomass reduction for forests, the CLs for the 5% biomass reduction were also shown. For PODY, CLs were calculated, referring to a “REF10” PODY calculated at a constant O3 concentration of 10 ppb referring to a “pre-industrial” O3 concentration, as recommended by CLRTAP (2017).
Simple linear regression analyses were used to assess the relationships between O3 indices (AOT40, POD0, and POD1) and relative biomass. In addition, to compare the gmax values between the two larches, Student’s t-test was performed on values within the top five percentile in gsto data. Results were considered significant at p < 0.05. All the analyses were performed using R 3.5.1 (R Core Team, 2018).
Results
The parameterization of the stomatal conductance model (Figure 1) resulted in very similar values for Japanese larch and its hybrid F1 (Table 2). The gmax in hybrid larch was slightly higher than that in Japanese larch although gmax values in the two larches were not statistically different (p = 0.48, Student’s t-test for the values within the top five percentile in gsto, data not shown). On the other hand, fmin was slightly higher in Japanese larch than in hybrid larch F1.
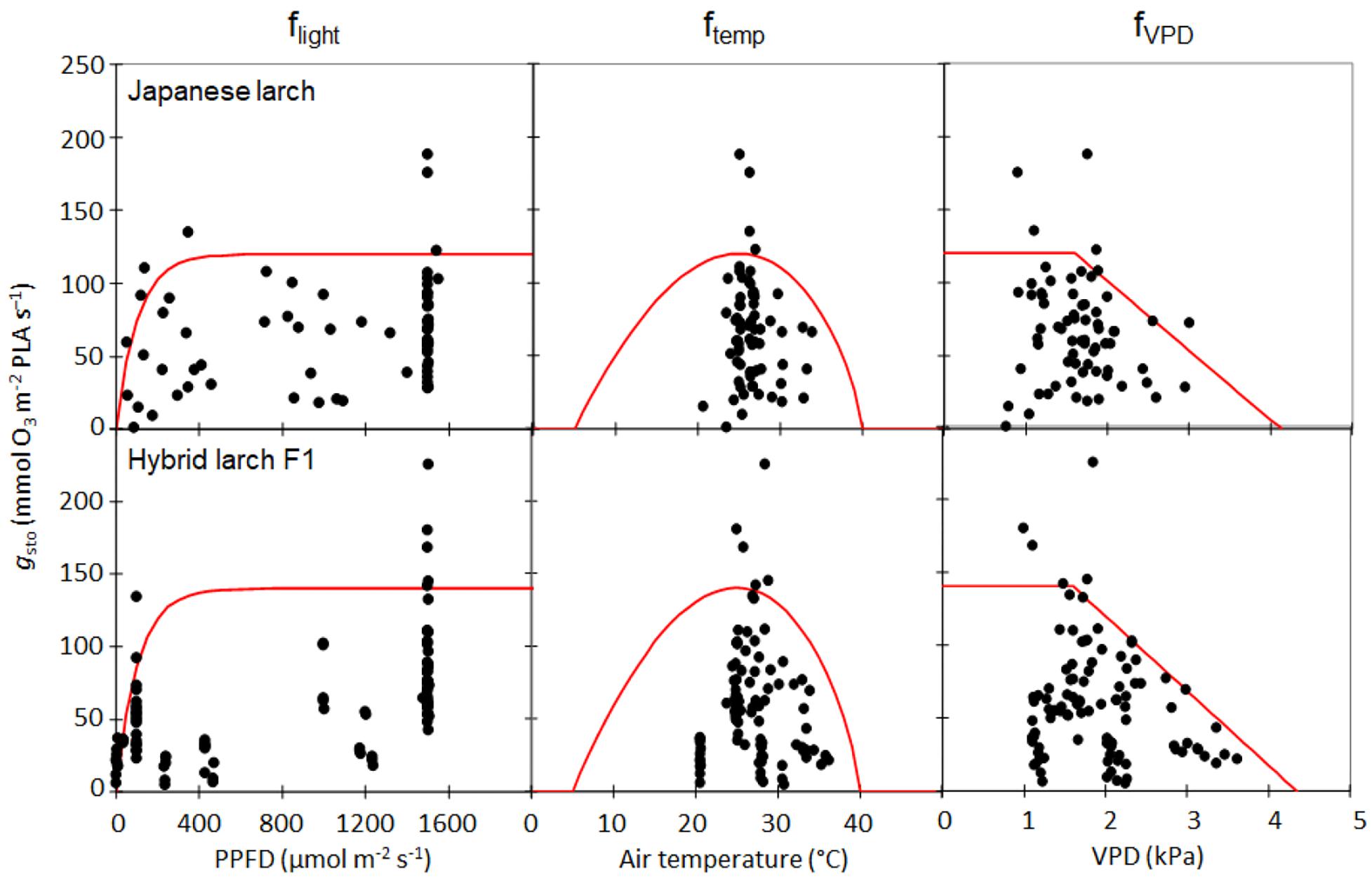
Figure 1. Parameterization of the stomatal conductance (gsto) model for Japanese larch (above) and its hybrid F1 (below), where flight, ftemp, and fVPD are functions of photosynthetically photon flux density (μmol photons m− 2 s− 1), air temperature (T, °C), and vapor pressure deficit (VPD, kPa), respectively. The results of the boundary line analysis are shown in red.
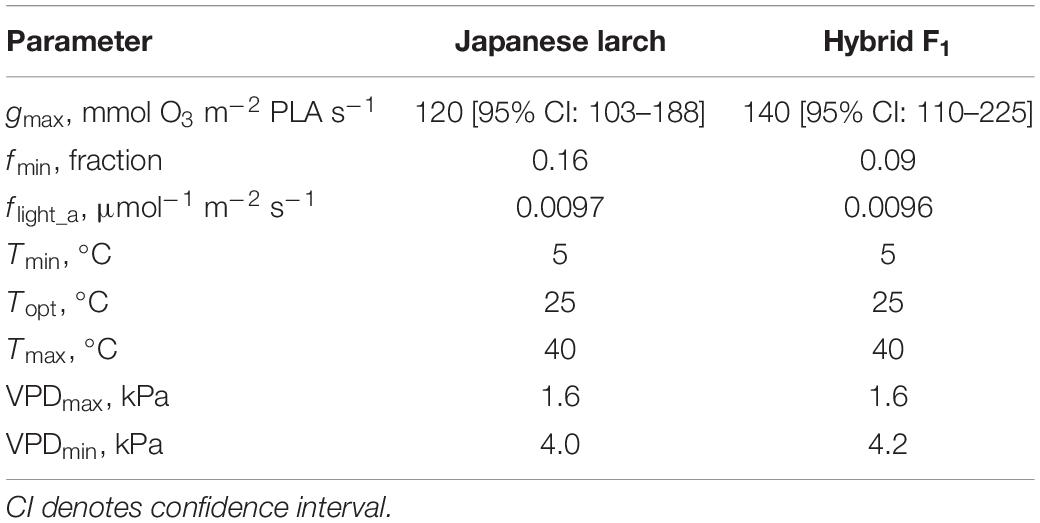
Table 2. DO3SE model parameters for Japanese larch and hybrid F1, where gmax is maximum stomatal conductance; fmin is minimum stomatal conductance; flight_a is a parameter determining the shape of the hyperbolic relationship of stomatal response to light; Tmax, Topt, and Tmin are the maximum, optimal, and minimum temperatures, respectively, for calculating the function ftemp that expresses the variation of gsto with temperature; VPDmin and VPDmax are the vapor pressure deficit for attaining minimum and maximum stomatal aperture, respectively (fVPD).
All the dose–response relationships were significant. When AOT40 was applied, in particular, a higher slope was found for hybrid larch F1 than for Japanese larch (Figure 2).
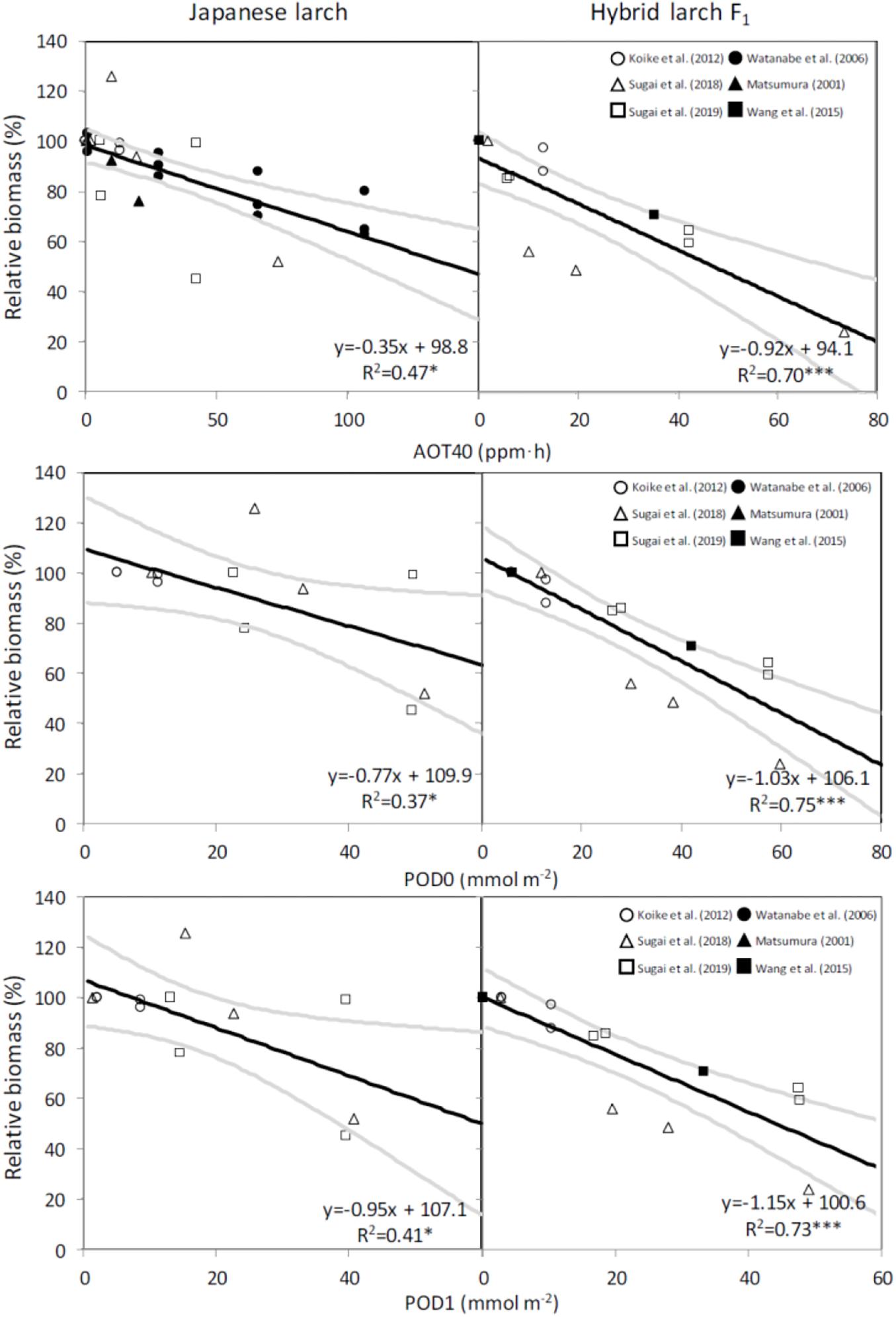
Figure 2. Dose–response relationships for total biomass losses in two species of larch seedlings on the basis of AOT40, POD0, or POD1 in different experiments. Black lines denote the regressions, and gray lines denote the 95% confidence intervals. Asterisks show the level of significance: ***p ≤ 0.001; *p ≤ 0.05.
The CLs calculated on the basis of these dose–response relationships were 2.7 times higher in Japanese larch than in its hybrid F1 when AOT40 was used, while PODY-based CLs were similar between the two species when using either no Y threshold or a Y threshold of 1 nmol m–2 s–1 PLA (Table 3).
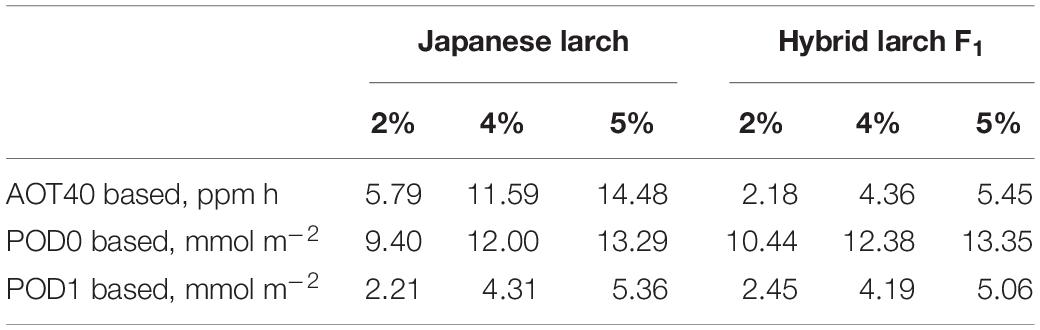
Table 3. Critical levels for larch protection from ozone corresponding to a total biomass loss of 2%, 4%, or 5% and based on the dose–response relationships in Figure 2.
Discussion
The boreal area in the northern hemisphere where larches are widely distributed is at risk of changes due to the potential O3 impact on photosynthetic carbon assimilation (Sicard et al., 2017), as estimated by several global atmospheric chemistry transport models and representative concentration pathways emission scenarios. For a realistic estimate of O3 risks to forests, CLs should be developed for the major forest species or types. Even though natural areas and plantations for larch trees are very wide and larch is a major genus of the forest category defined as boreal deciduous species, PODY-based CLs were not yet available for larch and are suggested here for the first time.
Organismic “sensitivity” may be defined as “the response of an organism (i.e., biological deviation) above or below a homeostatic state (control) of a set of biological traits, after sensing some environmental stress-inducing agents” (Agathokleous and Saitanis, 2020). However, “the organismal predisposition to be inhibited or adversely affected by or die of a xenobiotic,” as expressed by “negative (inhibitory or adverse) effects induced by diseases or environmental challenges,” is termed susceptibility (Agathokleous and Saitanis, 2020). Hence, organismic susceptibility can be assessed by studying dose/exposure–response relationships and, in particular, by comparing CLs among organisms (Agathokleous and Saitanis, 2020). Since the CLs are affected by the O3 metric used to develop dose/exposure–response relationships, susceptibility rankings can be different depending on the O3 metric used (Agathokleous et al., 2019).
So far, CLs have been estimated for a total biomass reduction in either deciduous broadleaf and Mediterranean conifer species (recommended biomass loss: 2%) or non-Mediterranean evergreen conifer species (recommended biomass loss: 4%) (CLRTAP, 2017). As larch is both a deciduous species and a non-Mediterranean conifer species, we decided to calculate the CLs for both the loss thresholds of 2% and 4%. We decided also to calculate the CLs for AOT40, although this metric is known for not being able to assess how much O3 enters the leaf through the stomata (Paoletti and Manning, 2007). However, it is still the legislative standard in Europe (Directive 2008/50), is used in many other continents (e.g., Agathokleous et al., 2018; Pleijel et al., 2019) because it is simple to calculate, and helps in the comparison with other results in the literature. The AOT40-based CL suggested so far for O3-susceptible deciduous broadleaves (F. sylvatica and B. pendula, 5 ppm h for a 5% biomass loss; CLRTAP, 2014, 2017) is similar to that of hybrid larch F1 (5.45 ppm h for 5% biomass loss), while Japanese larch showed a markedly higher AOT40-based CL corresponding to 5% loss (i.e., 14.48 ppm h). Based on a reanalysis of only two of the papers investigated here (Matsumura, 2001; Watanabe et al., 2006), Yamaguchi et al. (2011) had already suggested high O3 susceptibility for Japanese larch. In fact, the AOT40-based CLs that they recommended were consistent with those found in our work (i.e., 8–15 ppm h). In addition, our results would suggest a higher susceptibility to O3 of the hybrid and confirm previous studies where ecophysiological responses of the hybrid were more severely affected by O3 exposure than those of Japanese larch (Koike et al., 2012; Sugai et al., 2019).
An accurate parameterization of stomatal conductance model is essential for the flux-based O3 risk assessments (Emberson et al., 2000). For larch, the information of leaf-level gsto parameters was limited, although some studies tried to estimate O3 uptake at stand level by sap-flow measurements (Nunn et al., 2007) and at forest level by eddy covariance (Finco et al., 2017). Wieser and Havranek (1995) previously reported just stomatal VPD responses to estimate stomatal O3 uptake in European larch (Larix decidua). Our study is the first one to achieve a proper leaf-level parameterization (gmax, fmin, flight, ftemp, and fVPD) in larch trees to develop a flux-based approach. The maximum value of gsto in European larch by Wieser and Havranek (1995) was 150 mmol O3 m–2 PLA s–1, which was comparable to the gmax values in our findings. Interestingly, hybrid larch F1 showed a slightly higher gmax (140 vs. 120 mmol O3 m–2 PLA s–1 in Japanese larch). As gmax is known to play the most important role in determining PODY (Tuovinen et al., 2007), the small difference in gmax between the two species translated into a higher stomatal uptake of O3 by the hybrid at similar AOT40 levels; that is, the higher susceptibility of the hybrid under similar O3 exposures was due to a higher stomatal uptake. It is well known that fast-growing species with high stomatal conductance are susceptible to O3 because of an elevated stomatal uptake (Feng et al., 2018; Hoshika et al., 2018a). When the CLs are calculated on a PODY basis, in fact, the two species showed surprisingly similar CLs: 9.40 and 12.00 mmol m–2 POD0 and 2.21 and 4.31 mmol m–2 POD1 in Japanese larch versus 10.44 and 12.38 mmol m–2 POD0 and 2.45 and 4.19 mmol m–2 POD1 in the hybrid, for 2% and 4% biomass loss, respectively. These POD1-based values are below the CL recommended for non-Mediterranean trees (5.7 mmol m–2; CLRTAP, 2017), suggesting that these larches are more susceptible to O3 even when evaluated on the basis of stomatal flux. Different susceptibilities to O3 injury in the two larch species may be also due to different antioxidant capacities (Di Baccio et al., 2008). Although monoterpene emissions from leaves were preliminarily studied (Mochizuki et al., 2017), the role of antioxidants, secondary metabolites, and other leaf defensive molecules in the response of these two species to O3 remains elusive.
Conclusion
Based on a reanalysis of literature results and new measurements, we conclude that Japanese larch and its hybrid F1 should be classified as species with considerable O3 susceptibility as compared to the CLs available so far for other forest species. We also found that AOT40 and PODY can give very different results when assessing a species’ susceptibility to O3. While AOT40 suggested a higher susceptibility of hybrid F1, PODY did not highlight marked differences between the two species. Future research should clarify the O3 susceptibility of hybrid clones versus their wild forest species and increase the number of forest species with a species-specific parameterization and PODY-based CLs, especially in the Asian continent. This kind of information is needed for improving our modeling capacities, assessing O3 risks to local-to-global forests, and transferring this knowledge to environmental policy-makers.
Data Availability Statement
Basic raw data are available with YH (Italy) or TK (Japan).
Author Contributions
EP conceptualized the work and wrote the manuscript. EA, TS, and TK provided the data. YH analyzed the data. All authors reviewed the manuscript.
Conflict of Interest
The authors declare that the research was conducted in the absence of any commercial or financial relationships that could be construed as a potential conflict of interest.
Acknowledgments
This study is partly supported by the LIFE15 ENV/IT/000183 project MOTTLES and JST-2019 (Grant No. JPMJSC18HB).
References
Agathokleous, E., Belz, R. G., Calatayud, V., De Marco, A., Hoshika, Y., Kitao, M., et al. (2019). Predicting the effect of ozone on vegetation via the linear non-threshold (LNT), threshold and hormetic dose-response models. Sci. Total Environ. 649, 61–74. doi: 10.1016/j.scitotenv.2018.08.264
Agathokleous, E., Kitao, M., and Kinose, Y. (2018). A review study on O3 phytotoxicity metrics for setting critical levels in Asia. Asian J. Atmos. Environ. 12, 1–16. doi: 10.5572/ajae.2018.12.1.001
Agathokleous, E., and Saitanis, C. J. (2020). Plant susceptibility to ozone: a tower of babel? Sci. Total Environ. 703:134962. doi: 10.1016/j.scitotenv.2019.134962
Agathokleous, E., Vanderstock, A., Kita, K., and Koike, T. (2017). Stem and crown growth of Japanese larch and its hybrid F1 grown in two soils and exposed to two free-air O3 regimes. Environ. Sci. Pollut. Res. 24, 6634–6647. doi: 10.1007/s11356-017-8401-2
Alonso, R., Elvira, S., Sanz, M. J., Gerosa, G., Emberson, L. D., Bermejo, B., et al. (2008). Sensitivity analysis of a parameterization of the stomatal component of the DO3SE model for Quercus ilex to estimate ozone fluxes. Environ. Pollut. 155, 473–480. doi: 10.1016/j.envpol.2008.01.032
Anav, A., De Marco, A., Proietti, C., Alessandri, A., Dell’Aquila, A., Cionni, I., et al. (2016). Comparing concentration-based (AOT40) and stomatal uptake (PODy) metrics for ozone risk assessment to European forests. Glob. Change Biol. 22, 1608–1627. doi: 10.1111/gcb.13138
Bardak, S., Nemli, G., and Bardak, T. (2019). The quality comparison of particleboards produced from heartwood and sapwood of European Larch. Maderas 21:19.
Bičárová, S., Sitková, Z., Pavlendová, H., Fleisher, P. Jr., Fleisher, P. Sr., and Bytnerowicz, A. (2019). The role of environmental factors in ozone uptake of Pinus mugo Turra. Atmos. Pollut. Res. 10, 283–293. doi: 10.1016/j.apr.2018.08.003
Braun, S., Schindler, C., and Leuzinger, S. (2010). Use of sap flow measurements to validate stomatal functions for mature beech (Fagus sylvatica) in view of ozone uptake calculations. Environ. Pollut. 158, 2954–2963. doi: 10.1016/j.envpol.2010.05.028
Büker, P., Feng, Z., Uddling, J., Briolat, A., Alonso, R., Braun, S., et al. (2015). New flux based dose-response relationships for ozone for European forest tree species. Environ. Pollut. 206, 163–174. doi: 10.1016/j.envpol.2015.06.033
Büker, P., Morrissey, T., Briolat, A., Falk, R., Simpson, D., Tuovinen, J.-P., et al. (2012). DO3SE modelling of soil moisture to determine ozone effects to forest trees. Atmos. Chem. Phys. 12, 5537–5562. doi: 10.5194/acp-12-5537-2012
Chang, K.-L., Petropavlovskikh, I., Cooper, O. R., Schultz, M. G., and Wang, T. (2017). Regional trend analysis of surface ozone observations from monitoring networks in eastern North America, Europe and East Asia. Elementa Sci. Anthropocene 5:50. doi: 10.1525/elementa.243
CLRTAP, (2014). Mapping Critical Levels for Vegetation, Chapter III of Manual on Methodologies and Criteria for Modelling and Mapping Critical Loads and Levels and Air Pollution Effects, Risks and Trends. Geneva: UNECE.
CLRTAP, (2017). Mapping Critical Levels for Vegetation, Chapter III of Manual on Methodologies and Criteria for Modelling and Mapping Critical Loads and Levels and Air Pollution Effects, Risks and Trends. Geneva: UNECE.
De Marco, A., Sicard, P., Fares, S., Tuovinen, J.-P., Anav, A., and Paoletti, E. (2016). Assessing the role of soil water limitation in determining the Phytotoxic Ozone Dose (PODY) thresholds. Atmos. Environ. 147, 88–97. doi: 10.1016/j.atmosenv.2016.09.066
De Marco, A., Sicard, P., Vitale, M., Carriero, G., Renou, C., and Paoletti, E. (2015). Metrics of ozone risk assessment for Southern European forests: canopy moisture content as a potential plant response indicator. Atmos. Environ. 120, 182–190. doi: 10.1016/j.atmosenv.2015.08.071
Di Baccio, D., Castagna, A., Paoletti, E., Sebastiani, L., and Ranieri, A. (2008). Could the differences in O3 sensitivity between two poplar clones be related to a difference in antioxidant defense and secondary metabolic response to O3 influx? Tree Physiol. 28, 1761–1772. doi: 10.1093/treephys/28.12.1761
Dusart, N., Gérard, J., Le Thiec, D., Collignon, C., Jolivet, Y., and Vaultier, M. N. (2019). Integrated analysis of the detoxification responses of two Euramerican poplar genotypes exposed to ozone and water deficit: focus on the ascorbate-glutathione cycle. Sci. Total Environ. 651, 2365–2379. doi: 10.1016/j.scitotenv.2018.09.367
Emberson, L. D., Ashmore, M. R., Cambridge, H. M., Simpson, D., and Tuovinen, J. P. (2000). Modelling stomatal ozone flux across Europe. Environ. Pollut. 109, 403–413.
Feng, Z., Büker, P., Pleijel, H., Emberson, L., Karlsson, P. E., and Uddling, J. (2018). A unifying explanation for variation in ozone sensitivity among woody plants. Glob. Change Biol. 24, 78–84. doi: 10.1111/gcb.13824
Feng, Z., De Marco, A., Anav, A., Gualtieri, M., Sicard, P., Tian, H., et al. (2019a). Economic losses due to ozone impacts on human health, forest productivity and crop yield across China. Environ. Int. 131:104966. doi: 10.1016/j.envint.2019.104966
Feng, Z., Shang, B., Gao, F., and Calatayud, V. (2019b). Current ambient and elevated ozone effects on poplar: a global meta-analysis and response relationships. Sci. Total Environ. 654, 832–840. doi: 10.1016/j.scitotenv.2018.11.179
Finco, A., Marzuoli, R., Chiesa, M., and Gerosa, G. (2017). Ozone risk assessment for an Alpine larch forest in two vegetative seasons with different approaches: comparison of POD1 and AOT40. Environ. Sci. Pollut. Res. 24, 26238–26248. doi: 10.1007/s11356-017-9301-1
Hoshika, Y., Carrari, E., Zhang, L., Carriero, G., Pignatelli, S., Fasano, G., et al. (2018a). Testing a ratio of photosynthesis to O3 uptake as an index for assessing O3-induced foliar visible injury in poplar trees. Environ. Sci. Pollut. Res. 25, 8113–8124. doi: 10.1007/s11356-017-9475-6
Hoshika, Y., Fares, S., Gruening, C., Goded, I., De Marco, A., Sicard, P., et al. (2017). Stomatal conductance models for ozone risk assessment at canopy level in two Mediterranean evergreen forests. Agric. Forest Meteorol. 234, 212–221. doi: 10.1016/j.agrformet.2017.01.005
Hoshika, Y., Moura, B. B., and Paoletti, E. (2018b). Ozone risk assessment in three oak species as affected by soil water availability. Environ. Sci. Pollut. Res. 25, 8125–8136. doi: 10.1007/s11356-017-9786-7
Hoshika, Y., Osada, Y., De Marco, A., Penuelas, J., and Paoletti, E. (2018c). Global diurnal and nocturnal parameters of stomatal conductance in woody plants and major crops. Glob. Ecol. Biogeogr. 27, 257–275. doi: 10.1111/geb.12681
Hoshika, Y., Paoletti, E., and Omasa, K. (2012). Parameterization of Zelkova serrata stomatal conductance model to estimate stomatal ozone uptake in Japan. Atmos. Environ. 55, 271–278.
Hu, E., Gao, F., Xin, Y., Jia, H., Li, K., Hu, J., et al. (2015). Concentration- and flux-based ozone dose-response relationships for five poplar clones grown in North China. Environ. Pollut. 207, 21–30. doi: 10.1016/j.envpol.2015.08.034
Kita, K., Fujimoto, T., Uchiyama, K., Kuromaru, M., and Akutsu, H. (2009). Estimated amount of carbon accumulation of hybrid larch in three 31-year-old progeny test plantations. J. Wood Sci. 55, 425–434. doi: 10.1007/s10086-009-1064-y
Koike, T., Mao, Q., Inada, N., Kawaguchi, K., Hoshika, Y., Kita, K., et al. (2012). Growth and photosynthetic responses of cuttings of a hybrid larch (Larix gmelinii var. japonica x L. kaempferi) to elevated ozone and/or carbon dioxide. Asian J. Atmos. Environ. 2, 104–110.
Lefohn, A. S., Malley, C. S., Smith, L., Wells, B., Hazucha, M., Simon, H., et al. (2018). Tropospheric ozone assessment report: global ozone metrics for climate change, human health, and crop/ecosystem research. Elementa 6:28. doi: 10.1525/elementa.279
Li, P., Feng, Z., Catalayud, V., Yuan, X., Xu, Y., and Paoletti, E. (2017). A meta-analysis on growth, physiological, and biochemical responses of woody species to ground-level ozone highlights the role of plant functional types. Plant Cell Environ. 40, 2369–2380. doi: 10.1111/pce.13043
Matsumura, H. (2001). Impacts of ambient ozone and/or acid mist on the growth of 14 tree species: an open-top chamber study conducted in Japan. Water Air Soil Pollut. 130, 959–964. doi: 10.1007/978-94-007-0810-5_7
Mills, G., Pleijel, H., Malley, C. S., Sinha, B., Cooper, O. R., Schultz, M. G., et al. (2018). Tropospheric ozone assessment report: present-day tropospheric ozone distribution and trends relevant to vegetation. Elementa 6:47. doi: 10.1525/elementa.302
Mochizuki, T., Watanabe, M., Koike, T., and Tani, A. (2017). Monoterpene emissions from needles of hybrid larch F1 (Larix gmelinii var. japonica × Larix kaempferi) grown under elevated carbon dioxide and ozone. Atmos. Environ. 148, 197–202. doi: 10.1016/j.atmosenv.2016.10.041
Nunn, A. J., Wieser, G., Metzger, U., Löw, M., Wipfler, P., Häberle, K. H., et al. (2007). Exemplifying whole-plant ozone uptake in adult forest trees of contrasting species and site conditions. Environ. Pollut. 146, 629–639. doi: 10.1016/j.envpol.2006.06.015
Paoletti, E., and Manning, W. J. (2007). Toward a biologically significant and usable standard for ozone that will also protect plants. Environ. Pollut. 150, 85–95. doi: 10.1016/j.envpol.2007.06.037
Pleijel, H., Broberg, M. C., and Uddling, J. (2019). Ozone impact on wheat in Europe, Asia and North America – A comparison. Sci. Total Environ. 664, 908–914. doi: 10.1016/j.scitotenv.2019.02.089
Podda, A., Pisuttu, C., Hoshika, Y., Pellegrini, E., Carrari, E., Lorenzini, G., et al. (2019). Can nutrient fertilization mitigate the effects of ozone exposure on an ozone-sensitive poplar clone? Sci. Total Environ. 657, 340–350. doi: 10.1016/j.scitotenv.2018.11.459
R Core Team (2018). R: A Language and Environment for Statistical Computing. Vienna: R Foundation for Statistical Computing. Available online at: https://www.R-project.org/
Ryu, K., Watanabe, M., Shibata, H., Takagi, K., Nomura, M., and Koike, T. (2009). Ecophysiological responses of the larch species in northern Japan to environmental changes as a base of afforestation. Landsc. Ecol. Eng. 5, 99–106. doi: 10.1007/s11355-009-0063-x
Shang, B., Feng, Z., Li, P., Yuan, X., Xu, Y., and Calatayud, V. (2017). Ozone exposure- and flux-based response relationships with photosynthesis, leaf morphology and biomass in two poplar clones. Sci. Total Environ. 603-604, 185–195. doi: 10.1016/j.scitotenv.2017.06.083
Sicard, P., Anav, A., De Marco, A., and Paoletti, E. (2017). Projected global tropospheric ozone impacts on vegetation under different emission and climate scenarios. Atmos. Chem. Phys. 17, 12177–12196. doi: 10.5194/acp-17-12177-2017
Sicard, P., De Marco, A., Dalstein-Richier, L., Tagliaferro, F., Renou, C., and Paoletti, E. (2016). An epidemiological assessment of stomatal ozone flux-based critical levels for visible ozone injury in Southern European forests. Sci. Total Environ. 541, 729–741. doi: 10.1016/j.scitotenv.2015.09.113
Streit, K., Siegwolf, R. T. W., Hagedorn, F., Schaub, M., and Buchman, N. (2014). Lack of photosynthetic or stomatal regulation after 9 years of elevated [CO2] and 4 years of soil warming in two conifer species at the alpine treeline. Plant Cell Environ. 37, 315–326. doi: 10.1111/pce.12197
Sugai, T., Kam, D.-G., Agathokleous, E., Watanabe, M., Kita, K., and Koike, T. (2018). Growth and photosynthetic response of two larches exposed to O3 mixing ratios ranging from preindustrial to near future. Photosynthetica 56, 901–910. doi: 10.1007/s11099-017-0747-7
Sugai, T., Watanabe, T., Kita, K., and Koike, T. (2019). Nitrogen loading increases the ozone sensitivity of larch seedlings with higher sensitivity to nitrogen loading. Sci. Total Environ. 663, 587–595. doi: 10.1016/j.scitotenv.2019.01.292
Tuovinen, J.-P., Emberson, L., and Simpson, D. (2009). Modelling ozone fluxes to forests for risk assessment: status and prospects. Ann. For. Sci. 66:401. doi: 10.1051/forest/2009024
Tuovinen, J.-P., Simpson, D., Emberson, L., Ashmore, M., and Gerosa, G. (2007). Robustness of modelled ozone exposures and doses. Environ. Pollut. 146, 578–586. doi: 10.1016/j.envpol.2006.03.011
Uddling, J., Günthardt-Goerg, M. S., Matyssek, R., Oksanen, E., Pleijel, H., Selldén, G., et al. (2004). Biomass reduction of juvenile birch is more strongly related to stomatal uptake of ozone than to indices based on external exposure. Atmos. Environ. 38, 4709–4719. doi: 10.1016/j.atmosenv.2004.05.026
Unsworth, M. H., Heagle, A. S., and Heck, W. W. (1984). Gas exchange in open-top field chambers—II. Resistances to ozone uptake by soybeans. Atmos. Environ. 18, 381–385. doi: 10.1016/0004-6981(84)90112-4
Wang, X., Qu, L., Mao, Q., Watanabe, M., Hoshika, Y., Koyama, A., et al. (2015). Ectomycorrhizal colonization and growth of the hybrid larch F1 under elevated CO2 and O3. Environ. Pollut. 197, 116–126. doi: 10.1016/j.envpol.2014.11.031
Watanabe, M., Yamaguchi, M., Iwasaki, M., Matsuo, N., Naba, J., Tabe, C., et al. (2006). Effects of ozone and/or nitrogen load on the growth of Larix kaempferi, Pinus densiflora and Cryptomeria japonica seedlings. J. Jpn. Soc. Atmos. Environ. 41, 320–334.
Wieser, G., and Havranek, W. M. (1995). Environmental control of ozone uptake in Larix decidua Mill.: a comparison between different altitudes. Tree Physiol. 15, 253–258. doi: 10.1093/treephys/15.4.253
Wieser, G., and Havranek, W. M. (1996). Evaluation of ozone impact on mature spruce and larch in the field. J. Plant Physiol. 148, 189–194. doi: 10.1016/S0176-1617(96)80313-0
Yamaguchi, M., Kinose, Y., Matsumura, H., and Izuta, T. (2019). Evaluation of O3 effects on cumulative photosynthetic CO2 uptake in seedlings of four Japanese deciduous broad-leaved forest tree species based on stomatal O3 uptake. Forests 10:556. doi: 10.3390/f10070556
Yamaguchi, M., Watanabe, M., Matsumura, H., Kohno, Y., and Izuta, T. (2011). Experimental studies on the effects of ozone on growth and photosynthetic activity of Japanese forest tree species. Asian J. Atmos. Environ. 5, 65–78. doi: 10.5572/ajae.2011.5.2.065
Keywords: critical level, DO3SE model, forest, larch, parameterization, tropospheric ozone
Citation: Hoshika Y, Paoletti E, Agathokleous E, Sugai T and Koike T (2020) Developing Ozone Risk Assessment for Larch Species. Front. For. Glob. Change 3:45. doi: 10.3389/ffgc.2020.00045
Received: 19 December 2019; Accepted: 25 March 2020;
Published: 12 May 2020.
Edited by:
Armin Hansel, University of Innsbruck, AustriaReviewed by:
Juha-Pekka Tuovinen, Finnish Meteorological Institute, FinlandSteffen M. Noe, Estonian University of Life Sciences, Estonia
Rüdiger Grote, Karlsruhe Institute of Technology (KIT), Germany
Copyright © 2020 Hoshika, Paoletti, Agathokleous, Sugai and Koike. This is an open-access article distributed under the terms of the Creative Commons Attribution License (CC BY). The use, distribution or reproduction in other forums is permitted, provided the original author(s) and the copyright owner(s) are credited and that the original publication in this journal is cited, in accordance with accepted academic practice. No use, distribution or reproduction is permitted which does not comply with these terms.
*Correspondence: Elena Paoletti, elena.paoletti@cnr.it; Evgenios Agathokleous, evgenios_ag@hotmail.com