- 1Department of Earth Sciences, Montana State University, Bozeman, MT, United States
- 2Department of Environmental Sciences, Informatics and Statistics, Ca’ Foscari University of Venice, Venice, Italy
- 3Natural Resources Department, Salish Kootenai College, Pablo, MT, United States
- 4U.S. Geological Survey, Northern Rocky Mountain Science Center, Bozeman, MT, United States
Warm summer temperatures and longer fire seasons are promoting larger, and in some cases, more fires that are severe in low- and mid-elevation, dry mixed-conifer forests of the Northern Rocky Mountains (NRM). Long-term historical fire conditions and human influence on past fire activity are not well understood for these topographically and biophysically heterogeneous forests. We developed reconstructions of millennial-scale fire activity, vegetation change, and human presence at Black Lake, a small closed-basin lake on the Flathead Indian Reservation in the Mission Valley, Northwestern Montana, United States. Fossil pollen, charcoal, and biomarkers associated with human presence were used to evaluate the interaction between climate variability, fire activity, vegetation change and human activity for the past 7000 years. Comparisons among multiple proxies suggest climate variability acted as the primary control on fire activity and vegetation change from the early Holocene until the late Holocene when records suggest fire activity and climate variability decoupled. Specific biomarkers (5β-stanols including coprostanol and epi-coprostanol) associated with human presence indicate humans were present within the Black Lake watershed for thousands of years, although the inferred intensity of human presence is highly variable. A strong relationship between climate variability and fire activity during the early and mid-Holocene weakens during the last few thousand years, suggesting possible increased influence of humans in mediating fire activity in recent millennia, and/or a shift in the interaction between the distribution and abundance of woody fuel and fire severity. Human-set fires during the cooler and wetter late Holocene may have been aimed at maintaining important cultural resources associated with the heterogeneous mosaic of mixed conifer forests within the Black Lake watershed. The paleoenvironmental reconstruction at Black Lake corroborates archeological records that show humans were present within the Black Lake watershed for over 7000 years. Further research is needed to evaluate the evidence for this continuous presence and the possible role that people played in shaping fire regimes and vegetation within low- to mid-elevation mixed-conifer ecosystems of the NRM.
Introduction
Across western North America, rapid changes in disturbance regimes are altering the structure and function of forest ecosystems. In the Northern Rocky Mountains (NRM), multiple disturbances including insect activity, increased stand-replacing wildfires, and prolonged drought stress are combining to influence large areas of mixed-conifer forests (Heyerdahl et al., 2008; Raffa et al., 2008). Wildfire suppression costs in the western US have risen to over two billion dollars annually and now comprise nearly half of the US Forest Service budget (Balch et al., 2018). While the intensity and extent of these changes in forest dynamics are linked to synergies among land use, higher temperatures, shifts in fire regimes, and insect outbreaks, the relative importance of these drivers is unclear. In the NRM, century-long trends suggest the recent four decades of warming has occurred at 2–3 times the rate of the global average resulting in reduced snowpack, early snowmelt runoff, early onset of spring, reduced summer streamflow, increased frequency of high-temperature extremes, and reduced frequency of extreme winter cold events (Pederson et al., 2010; Klos et al., 2014). It is likely that these changes have affected disturbance dynamics, but this idea has not been well tested because data on historical forest dynamics is lacking from this region, especially from dry, low- to mid-elevation mixed conifer forests of the NRM.
Recent changes to the structure and function of forest ecosystems and fire-mediated forest transitions are calling new attention to the interactions and feedbacks among climate, human activities, and fire (Odion et al., 2010; Tepley Alan et al., 2017). Historical fire regimes are well characterized in some forest types, such as the dry, ponderosa pine forests in the southwestern and interior northwestern US. These forests are dominated by high-frequency, low-severity fire, yet this research is being used to guide management of many other forest types, even the fuel-rich, low- to mid-elevation forests characterized by highly variable fire regimes (Halofsky et al., 2011). Low- to mid-elevation forests in the NRM, including those in Montana, are among the most affected by structural changes resulting from the legacy of 20th century land management and changing climatic conditions (Dodge, 1972; Dennison et al., 2014). These forests are widespread and include large areas dominated by varying mixtures of Pseudotsuga menziesii (Douglas-fir), Pinus ponderosa (ponderosa pine), Abies grandis (grand fir), and Larix occidentalis (western larch). Historical fire regimes for these forests are thought to be of intermediate frequency (35–200 years) and low- to mixed-severity, although little well-dated quantitative data exists to test these assumptions (Arno et al., 1997). Despite the fact that forests broadly classified as mixed-severity compose nearly half (46%) the forests of the western US, and 43% of the 11 million ha treated under a national plan to reduce hazardous fuels (Schoennagel and Nelson, 2011), there is little information on the appropriateness of thinning treatments for heterogenous mixed-severity forests of the NRM and their potential unintended ecological consequences (e.g., inhibiting regeneration and nutrient cycling; Donato et al., 2006).
Recent efforts to better understand how ancient and modern humans altered forest ecosystems and fire regimes are underway in fire-prone systems in the southwestern US, motivated by the need to evaluate how land-use and climate interact to promote transitions to new alternate stable states (Miller et al., 2019), but again these efforts focus on dry, ponderosa pine ecosystems. The lack of multi-scale reconstructions for mixed-severity forests of the NRM highlights the need to better understand the historical variability in fire activity and the role of climate versus people in shaping the conditions that influence fire activity. Conditions that include changes to the composition, structure continuity, and availability of fuels and the frequency, spatial distribution, and timing of ignitions (Whitlock et al., 2010).
Humans have used fire as a tool to manage landscapes for millennia; to enhance the quality and quantity of desired resources, improve hunting, facilitate travel, reduce fire risk and hazard, clear land, and for ceremonial and other purposes (Lake et al., 2017). Disentangling the relative role of human versus climate in driving fire regimes remains one of the great challenges in paleofire science (Bowman et al., 2011; Swetnam Thomas et al., 2016). While climatic conditions serve as fundamental controls on the factors that shape fire regimes, humans have magnified or reduced climatic controls by igniting fires when and where they were naturally rare, modifying fuel composition and structure, and through suppression or elimination of fires (McWethy et al., 2013). Comparing past records of fire activity with climatic reconstructions and robust and continuous archeological records offers an opportunity to identify time periods where humans may have enhanced or reduced climatic impacts on fire activity (Roos et al., 2018), but few locations offer robust multi-millennial archeological records. Where archeological data is discontinuous and/or sparse, changes in charcoal accumulation from wetland sediment records are often used as indirect evidence for anthropogenic burning yet other factors may also be responsible. As a result, linking past changes in vegetation and fire with human activity is largely inferential. Organic markers, or biomarkers, are stable molecular compounds that provide new opportunities to reconstruct past human presence (e.g., 5β-stanols such as coprostanol and epi-coprostanol), changes in biomass burning from anthropogenic and natural origins (e.g., polycyclic aromatic hydrocarbons; PAHs), and shifts in vegetation communities (e.g., n-alkanes). Fecal biomarker data can be particularly useful in evaluating the relative role of humans and climate in shaping fire activity and vegetation change over multi-millennial time scales (Bull et al., 2002; Battistel et al., 2016; Argiriadis et al., 2018).
Radiocarbon dated cultural materials from western Montana and the Mission Valley indicate Indigenous populations were present in western Montana throughout the Holocene (Gajewski et al., 2011). Radiocarbon dated cultural materials for the Mission Valley and the Black Lake watershed are spatially and temporally discontinuous, however, limiting the extent to which we can infer changes in human activity at the Black Lake site and the potential influence of human-set fires on fire regimes and ecosystem dynamics over time. Coupling archeological records with reconstructions of human presence from fecal biomarkers derived from lake sediments can begin to address these limitations by providing temporally continuous information on variations in human activity over time within individual watersheds.
Here we reconstruct past environmental conditions, human presence, and fire activity through analysis of multiple proxies (pollen, macroscopic charcoal, biomarkers, and lithological and geochemical changes) found in lake sediments. We ask: How spatiotemporally variable were fire regimes in low- to mid-elevation mixed-conifer forests? How has human presence varied over the last ∼7000 years? Is there a relationship between reconstructions of human presence and changes in fire activity and vegetation? Based on a database of radiocarbon dated cultural materials from western Montana, we expect that fecal biomarker records will indicate long-term, yet intermittent, human presence throughout the last ∼7000 years, with increasing presence and influence on fire regimes and vegetation during the last two millennia when archeological records suggest Indigenous populations of the NRM were greatest (Peros et al., 2010; Gajewski et al., 2011; MacDonald, 2012).
Site Description
Black Lake (47°51.7′N, 114°19.3′W; 985 m elevation; 25 ha) is located in the Mission Valley in Lake County, Montana (Figure 1). The site lies in forest that is dominated by Pseudotsuga menziesii (56%) and Pinus ponderosa (38%), with a small amount of Larix occidentalis (6%). Alnus spp., Salix spp., Rosaceae, and various species of Poaceae grow in the understory and around the lake margins. The conifer trees on the east side of the lake are mostly uniform in age and approximately 90 years old. On the west side of the lake is a mosaic of uneven-aged stands ranging from 30 to 300 years old with substantive cohort establishments ca. 1600, 1890, and 1920.
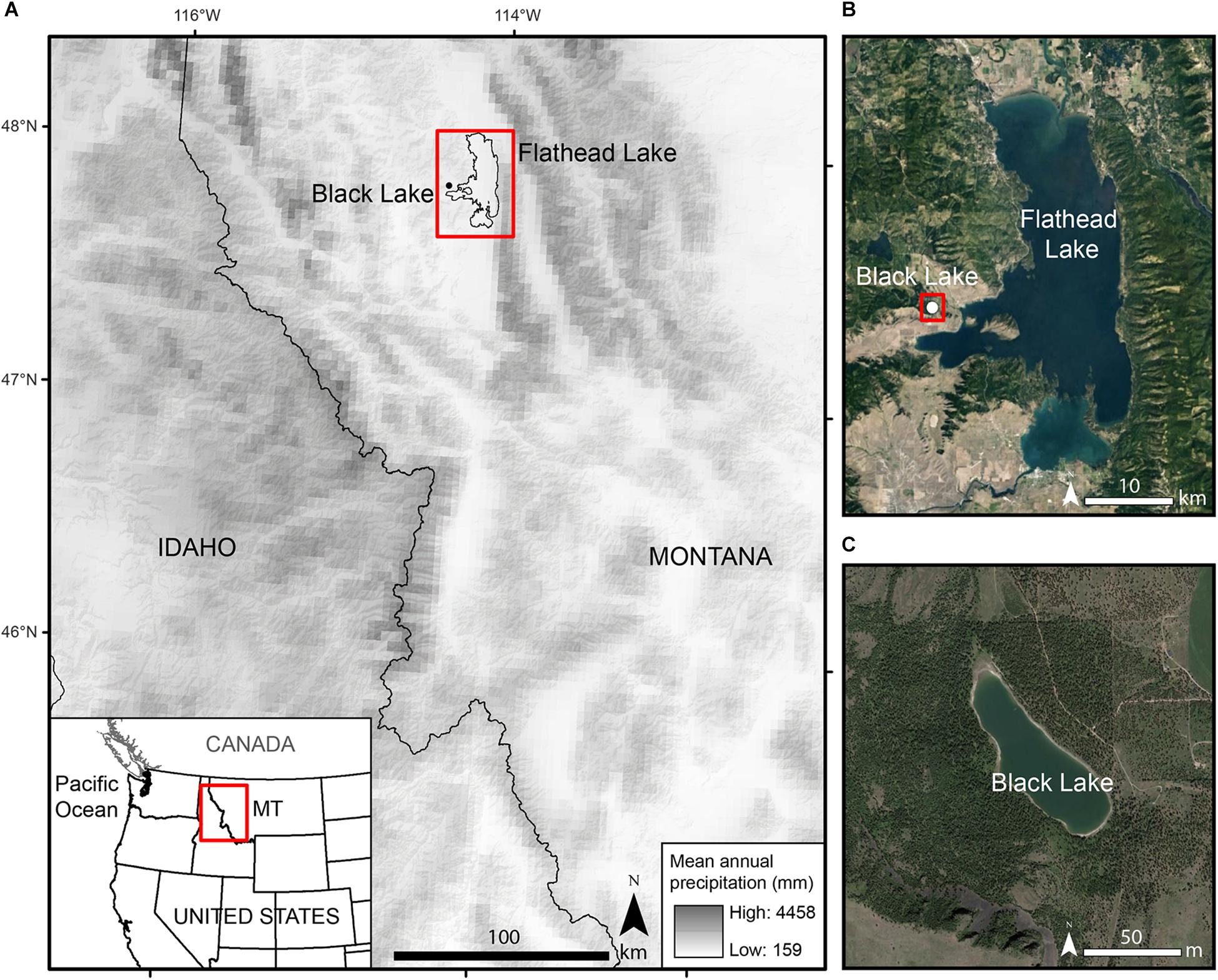
Figure 1. (A) Location of Black Lake in the Northern Rocky Mountains over a base map showing the mean annual precipitation from 1981 to 2010 (PRISM Climate Group, 2015; map source: ESRI). (B) Location of Black Lake in Lake County, MT (map source, Google Earth). (C) Close up of Black Lake (map source, Google Earth).
Most of the precipitation in the region occurs in the form of snow brought by frontal Pacific storm systems during the winter at higher elevations; however the majority of the precipitation at Black Lake itself comes from convectional storms in the spring and summer (June-August average: 130 mm; September-November average: 110 mm December-February average: 90 mm; March-May average: 113 mm). Mean annual temperature is 7°C; mean maximum and minimum summer temperatures (June–August) are 25 and 9°C respectively; mean maximum and minimum winter temperatures (December–February) are 1°C and −7°C respectively. All climate data represent 30 year averages from 1981 to 2010 (Prism Climate Group, 2019 accessed May 1, 2019).
Materials and Methods
Two sediment cores were collected from the deepest part of Black Lake (water depth = 8 m) from an anchored platform in 2013 and 2014: one 121 cm long core (core 13A obtained in 2013) was taken with a polycarbonate tube (Klein corer) to capture the mud-water interface, and one 464 cm long core (core 14A obtained in 2014) was taken with a modified Livingstone square-rod piston coring device (Wright et al., 1984). Core 13A was contained within the polycarbonate tube and sealed with Zorbitrol, the segments of core 14A were wrapped with plastic wrap and aluminum foil, and all cores were transported to Montana State University where they were refrigerated until analysis. In the laboratory they were cut longitudinally and inspected for macrofossils, lithological changes were described, and magnetic susceptibility readings were taken every 0.5 cm. Samples of bulk sediment and woody material were taken at approximate 20–40 cm intervals and submitted for accelerator mass spectrometry (AMS) radiocarbon dating.
Pollen Analysis
Samples of 1 cm3 volume were processed following procedures outlined by Bennett and Willis (2002), and a Lycopodium tablet of known concentration was added to each sample in order to calculate pollen accumulation rates (PAR; grains cm–2 yr–1). A minimum of 300 terrestrial pollen grains and spores were counted per sample at magnifications of 400 and 1000x. Pollen reference slides and keys (McAndrews et al., 1973; Faegri and Iversen, 1975; Kapp et al., 2000) were used to identify the grains. Terrestrial pollen counts were converted to percentages based on the terrestrial pollen sum, and aquatic pollen counts were converted to percentages based on the combined sum of terrestrial and aquatic pollen. Cyperaceae was considered an aquatic species at this site and included in the aquatic pollen sum. Arboreal pollen (AP) is the sum of all tree and shrub species excluding Artemisia, and non-arboreal pollen (NAP) is the sum of Artemisia, herbs, and grasses. The AP:NAP ratio was calculated with the formula (AP-NAP)/(AP + NAP). Tilia software was used to create a pollen diagram, and the record was divided into pollen zones using CONISS cluster analysis (Grimm, 1987).
Pinus grains were categorized as Pinus subgenus Pinus-type (P. ponderosa or P. contorta) and Pinus subgenus Strobus-type (P. albicaulis, P. monticola, or P. flexilis) based on the presence of verrucae on the distal membrane. Those with missing or destroyed distal membranes were classified as undifferentiated Pinus. Picea grains were attributed to P. engelmannii, Abies grains to A. grandis or A. lasiocarpa, and Cupressaceae pollen was assigned to Juniperus communis, J. scopulorum and/or T. plicata. Pseudotsuga menziesii, Larix occidentalis, and L. lyallii all grow in the region of Black Lake and their pollen grains are undistinguishable; therefore their pollen grains were counted as Pseudotsuga/Larix.
Charcoal Analysis
The top 50 cm of core 13A was subsampled at 0.5 cm contiguous intervals, and the remainder of core 13A and all of core 14A were subsampled at 1 cm contiguous intervals for charcoal analysis. Samples of 2 cm3 were taken from each interval and processed following the procedure outlined by Whitlock and Larsen (2001). A 125 μm sieve was used to collect charcoal particles, because charcoal particles larger than 125 μm diameter typically reflect local fire events (<2 km) (Whitlock and Larsen, 2001; Higuera et al., 2010). Macroscopic charcoal particles were counted and identified as wood or grass under a stereomicroscope.
CharAnalysis1 was used to perform a statistical analysis of the charcoal counts and reconstruct a fire history according to the methods described by Higuera et al. (2009). Charcoal counts were converted to charcoal accumulation rates (CHAR; particles cm–2 yr–1) and the time series was interpolated to 14 years time steps (the median deposition time per sample) to minimize differences in charcoal accumulation due to changes in deposition rate. A 500 years lowess smoother, robust to outliers, was used to distinguish the long-term background trends (BCHAR). After applying a Poisson minimum count test, charcoal peaks that exceeded a 95% threshold were identified as fire episodes (one or more fires occurring during the time span of the peak), and the time in between fire episodes was smoothed with a 1000 years window to produce a fire-episode frequency record (episodes 1000 yrs–1).
Validation studies show that fire reconstructions from macroscopic charcoal influx record high-severity fire events that occurred near coring sites (0.1–2 km) as well as trends in regional biomass burning (from background charcoal accumulation) at millennial scales, but often underestimate or fail to record mixed and low-severity fires that occurred locally (e.g., Higuera et al., 2005; Allen et al., 2008; Beaty and Taylor, 2009; Swetnam et al., 2009). We interpret fire peaks identified through analysis of macroscopic charcoal influx to represent high-severity fire events within the sampling resolution time step (14 year times step) derived from the age-depth chronology for Black Lake. While low-severity fires likely contributed to the charcoal influx record, individual low-severity events cannot be well resolved in our record.
Biomarker Analysis
We analyzed lake sediments for specific molecular markers to provide a continuous record of human presence to compare to vegetation and fire reconstructions obtained from pollen and charcoal (Supplementary Tables S1, S2). We determined polycyclic aromatic hydrocarbons (PAHs), n-alkanes (NAs), and fecal sterols (FSs) in 66 samples from the Black Lake 13A and 14A cores. Wet samples were dried in the desiccator with silica gel until they maintained a constant weight and were then hand milled and homogenized in a ceramic mortar. Samples were stored at room temperature in sealed vials until extraction, which was performed with an ASE 300 (Accelerated Solvent Extraction, Dionex Thermo Fisher Scientific). Each sample was dispersed with diatomaceous earth and spiked with a known amount of internal standard solutions for the quantification of the analytes (13C6-Acenaphtylene, 13C6-Phenanthrene, 13C4-Benzo(a)pyrene for PAHs, Hexatriacontane for NA and 13C6-Cholesterol for FS) and extracted twice at 150°C and 1500 psi with dichloromethane (DCM).
Extracts were concentrated under a gentle stream of nitrogen (Turbovap, Caliper Life Sciences) up to ∼0.5 mL and purified onto disposable solid phase extraction silica tubes (Supelco DSC-Si 12 mL, 2 g bed weight), previously conditioned with 40 mL of n-hexane: DCM (1:1, v/v). The clean-up and fractionation of samples were achieved by eluting the samples with 15 mL of n-hexane:DCM (1:1, v/v) followed by 70 mL of DCM (Kirchgeorg et al., 2014; Battistel et al., 2015, 2016). The two fractions (F1 and F2) were collected separately. F1 was concentrated to 100–200 μL and PAHs and n-alkanes were analyzed through gas chromatography-mass spectrometry (GC-MS). F2 was evaporated to dryness and redissolved in 100 μL of DCM, then 100 μL of BSTFA + 1% TMCS (N,O-Bis(trimethylsilyl)trifluoroacetamide + 1% Trimethylchlorosilane) were added to the samples to allow derivatization at 70°C for 1 h. After a stabilization period of 24 h at room temperature, FS were analyzed by GC-MS. The GC-MS methods, along with instrumental setup are reported elsewhere (Piazza et al., 2013; Kirchgeorg et al., 2014; Battistel et al., 2015). Analytes were quantified based on internal standards (solutions at known concentration) that were distributed as a spike within the sample matrix during extraction in order to correctly quantify concentrations of the analytes. 13C-Cholesterol (200 absolute ng) was used for FS, hexatriacontane (2.5 abs μg) for alkanes, 13C-phenanthrene (50 abs ng), 13C-acenaphthylene (50 abs ng) and 13C-benzo(a)pyrene for PAHs. Results were calculated and corrected by the instrumental response factors, obtained by the repeated analysis of standard solutions containing all analytes and 13C internal standards at 100 pg μL–1 for PAHs, 10 ng μL–1 for NA and 1 ng μL–1 for FS.
Several procedural blanks were also analyzed in order to quantify possible contamination from the laboratory equipment. Absolute quantities of cholesterol and n-alkanes were corrected for the blank plus three times the standard deviation of the blanks and divided by the dry weight of samples to obtain concentrations. Coprostanol and epi-coprostanol were not present in blanks and therefore no correction was applied. The average blank was 95 abs ng for cholesterol (values consistent with what would naturally occur), 61 for β-sitosterol and 29 for stigmastanol. As for PAHs, acenaphthylene, benzo(b)fluoranthene, benzo(k)fluoranthene, benzo(e)pyrene, perylene, benzo(ghi)perylene, indeno(1,2,3-cd)pyrene and dibenzo(ah)anthracene did not occur in blanks, while the other compounds ranged between 0.2 and 6.8 abs ng, except for naphthalene that had a higher value (13.4 ng) (values consistent with values that would naturally occur). Fluxes were calculated using the accumulation rate and the dry density (Menounos, 1997). The average accuracy was 89 ± 10% for PAH, 87 ± 8% for FPS and 84 ± 10% for NA. Precision ranged between 2 and 5% for PAH, 1–4% for FPS 7–24% for NA.
n-Alkanes
n-Alkanes (NAs) were used in conjunction with pollen data to evaluate changes in the composition and structure of vegetation. Long-chain n-alkanes are widely used in palaeoenvironmental studies from lake sediments to obtain information about the vegetation input (Bush and McInerney, 2013). These compounds are contained in the epicuticular waxes of terrestrial plants, especially in leaves, and have the potential to provide useful diagnostic ratios due to the fact that certain n-alkane chain lengths are predominant in specific plant groups. Although several indices have been proposed for reconstructing the vegetation input preserved in lake sediments, their use should be carefully evaluated. Since sediments collect a multisource plant wax input, an unambiguous discrimination is oftentimes very challenging, further complicated by the influence of the productivity season and latitude. Though indices should be prudently used, it has been widely demonstrated that the abundance of long-chain n-alkanes is generally higher in angiosperms than in gymnosperms (Bush and McInerney, 2013; Diefendorf and Freimuth, 2017). Therefore, in this paper we propose the use of the sum of C22-C33 (∑(C22–33)) as an indicator of the angiosperm versus gymnosperm relative abundance, where higher ∑C22–33 values indicate the predominance of angiosperms. Here, the indicator ∑C22–33 is reported in terms of fluxes in order to take into account the sedimentation rate.
Polycyclic Aromatic Hydrocarbons (PAHs)
Polycyclic aromatic hydrocarbons are organic compounds produced by a wide range of combustion processes. The chemical structure, composed by > 2 aromatic rings fused together, ensures a high resistance to degradation. Therefore, PAHs are used in pre-industrial sedimentary archives as tracers of past combustion processes and several diagnostic ratios are employed to infer the source of burning and combustion temperatures (Page et al., 1999; Yunker et al., 2002). Among PAHs, retene is regarded as a direct proxy for softwood combustion and degradation of dehydroabietic acid (Ramdahl, 1983; Vicente et al., 2012; Ahad et al., 2015).
Fecal Sterols
Fecal sterols primarily reach lake sediments through runoff (Meyers, 1997), and thus describe inputs from the local watershed. Cholesterol and cholestanol are produced by vertebrates, especially mammals including humans (Leeming et al., 1996) and are widely used as normalization parameters. The evidence of human presence within the Black Lake catchment is provided by coprostanol, the most abundant sterol in human feces (Bull et al., 2002), and epi-coprostanol resulting from the post-depositional epimerization of coprostanol (Birk et al., 2011). While omnivores such as dogs (and potentially bears and wolves), can also produce coprostanol, previous research indicates that coprostanol concentrations for humans are much more abundant than other ominivores (often > 10 times more abundant) (Leeming et al., 1996); therefore we interpret humans to be the primary source of coprosanol in the sediments.
As coprostanol is highly abundant in human feces and considering that atmospheric transport is very limited for this proxy, in situ degradation reaction is the main factor that can affect the coprostanol signal. Therefore, in order to account for potential variations due to degradation processes, we used a ratio (hereon referred to as the coprostanol threshold ratio or CTR), defined as follows:
Based on validation studies (Takada et al., 1994; Carreira et al., 2015) we consider the value CTR = 0.2 as a threshold to distinguish a signal due to a human contribution versus natural variability (i.e., CTR values higher than 0.2 indicate human presence), and CTR values greater than 1.0 to indicate intensive use of the site by humans. In general, we interpreted higher/lower CTR values as indicative of higher/lower presence of human populations, and values below 0.2 as absence of humans (Carreira et al., 2015).
Results
Chronology
The age-depth chronology was derived from nineteen accelerator mass-spectrometry 14C dates from 17 bulk sediment samples and two wood fragments (Table 1). The 14C dates were converted to calibrated ages using the IntCal13 calibration curve (Reimer et al., 2013), and the final age-depth model was constructed using Bayesian accumulation histories for deposits (Bacon) software for modeling (Blaauw and Christen, 2011) in R (R Core Team, 2015; Figure 2). Bulk sediment dated from depth 440 cm represents an age reversal but was included in the age-depth model. The sedimentation rate was highest at the base of the core, averaging 24 yr cm–1 between 464 and 415 cm. From 415–44 cm depth, sedimentation rate averaged 15 yr cm–1 other than between 99 and 91 cm depth when it increased to an average of 32 yr cm–1. The rate increased at the top of the core with an average of 20 yr cm–1 from 44 and 0 cm depth.
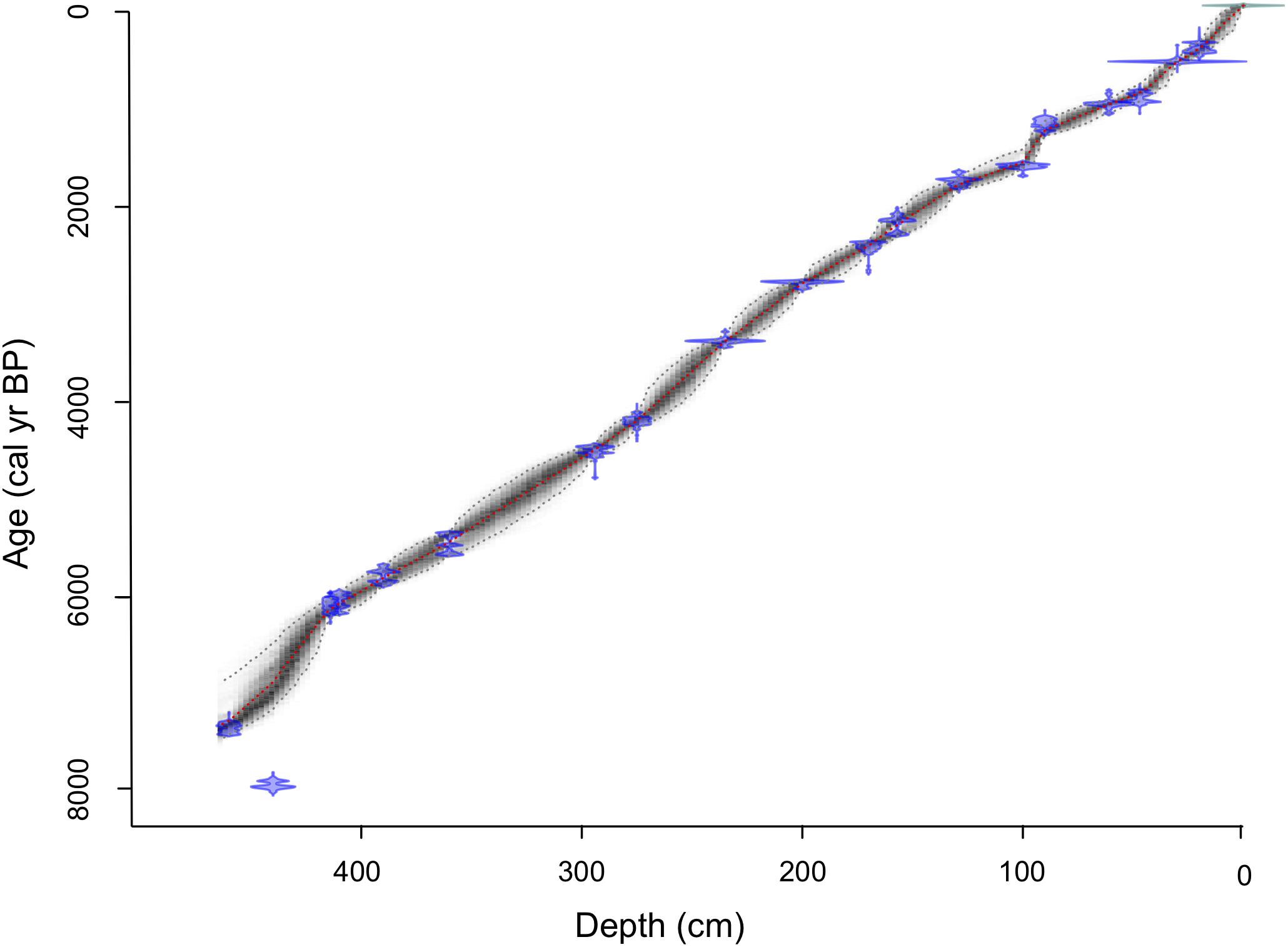
Figure 2. Age-depth model for Black Lake using Bacon age-depth modeling software (Blaauw and Christen, 2011) in R (R Core Team, 2015).
Lithology
The 14A core was divided into two lithologic units (Figure 3). Unit 1 (464–415 cm depth) consisted of dark gray inorganic clay and had the highest average magnetic susceptibility values at the base (average of 5 SI units from 464 to 455 cm). Unit 2 (415–0 cm depth) consisted of fine-detritus gyttja with laminations of pink, red, brown, and gray. Both of the cores had a section of thin red and dark brown laminations at 140 cm depth that was used to correlate the cores. Core 14A additionally had sections of very dark brown to black bands of fine-detritus gyttja at 175–176, 339–340, 374–375, and 410–414 cm depth. Magnetic susceptibility values were generally stable throughout the record. Magnetic susceptibility was low in Unit 2 (average of -1 SI units) other than two small peaks that corresponded with bands of dark brown to black fine-detritus gyttja at 374–375 and 410–414 cm depth, and four other peaks at 116, 215, 303, and 320 cm depth that do not correspond with lithological changes or tephras.
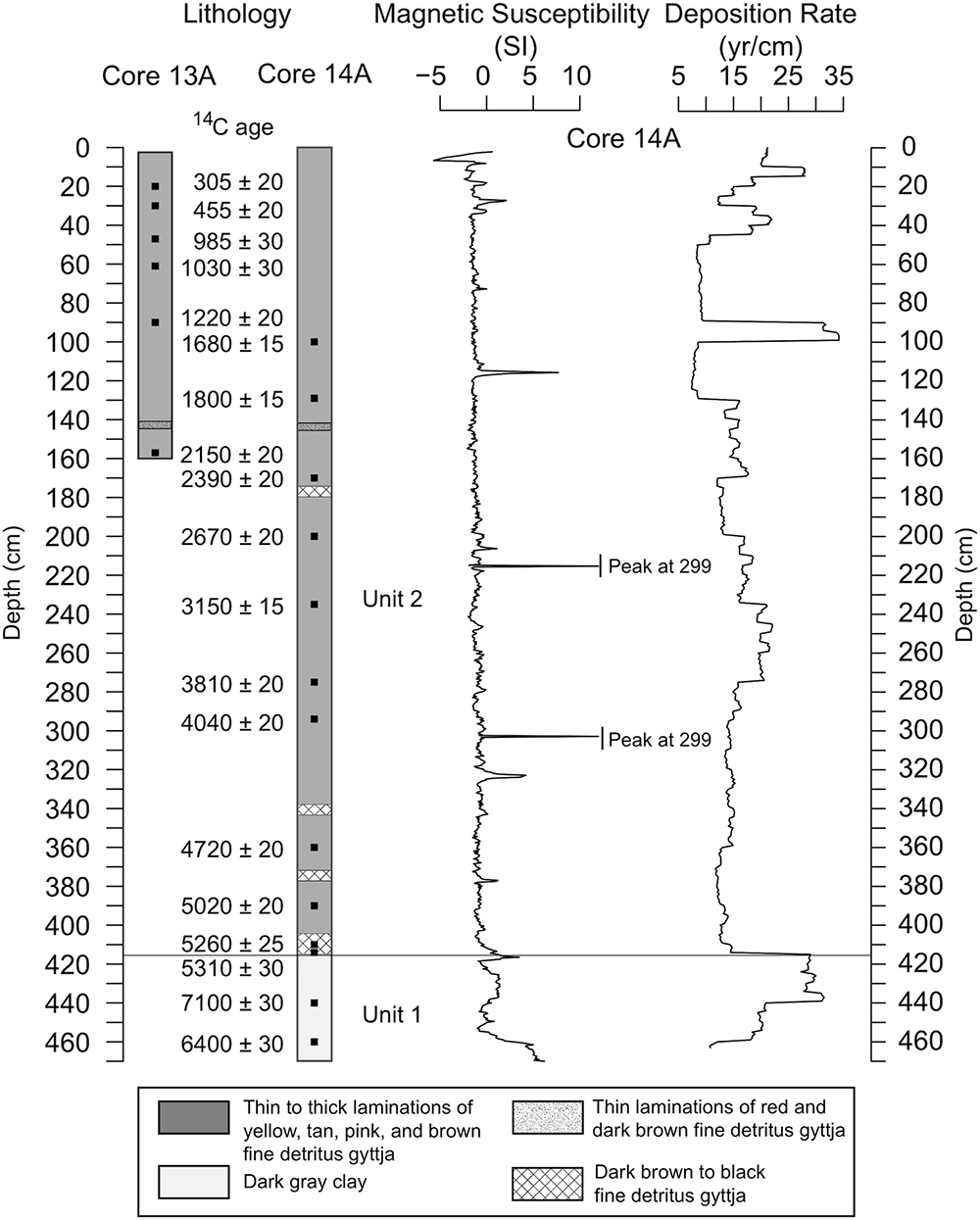
Figure 3. Lithology, uncalibrated radiocarbon ages, magnetic susceptibility data, and deposition rate for the Black Lake sediment cores.
Pollen and Charcoal
Based on CONISS results, the pollen record was divided into three zones, BL1-BL3 (Figure 4). Zone BL1 (464–380 cm depth; 7300–5600 cal yr BP) was characterized by high levels of Poaceae (12–23%) and Artemisia (5–17%) pollen, and low values of conifer pollen (total Pinus: 45–60%). Although not very prominent, pollen from shrubs and herbs, such as Amaranthaceae (1–4%), Asteraceae subfamily Asteroideae (1–3%), and Rosaceae (<1–2%), had the highest levels of the record in this zone. Alnus spp. increased (from <1 to 6%) at ca. 6000 cal yr BP, concurrently with an increase in Cyperaceae pollen (from <1 to 8%). The arboreal to non-arboreal pollen ratio (AP:NAP) slowly increased through the zone, and the PAR was low (average: 3060 grains cm–2 yr–1). The Pinus pollen percentages are consistent with those found in modern steppe/parkland or steppe environments (Iglesias et al., 2018) and the low values of PAR are also consistent with an open forest (Davis, 1976).
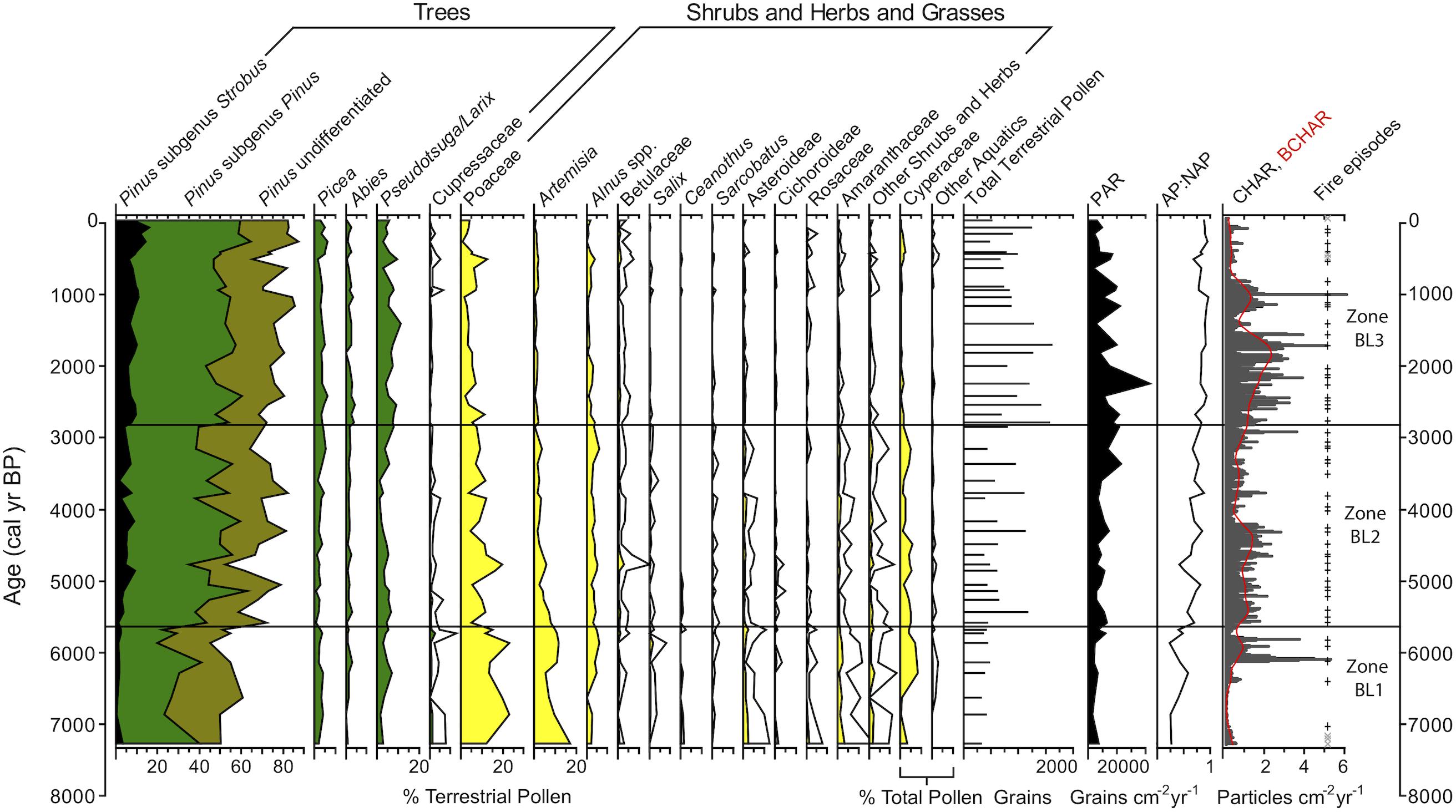
Figure 4. Pollen percentage data for select taxa, arboreal/non-arboreal pollen ratio (AP:NAP), and charcoal accumulation data from Black Lake sediments. Pinus pollen data are stacked percentages. White outlines represent 5x exaggeration.
CHAR and BCHAR were very low at the bottom of Zone BL-1 but increased significantly at 420 cm depth (6000 cal yr BP), coincident with a peak in magnetic susceptibility. Fire frequency increased from 1 to 7 episodes 1000 yrs–1 over the range of the zone (Figure 5).
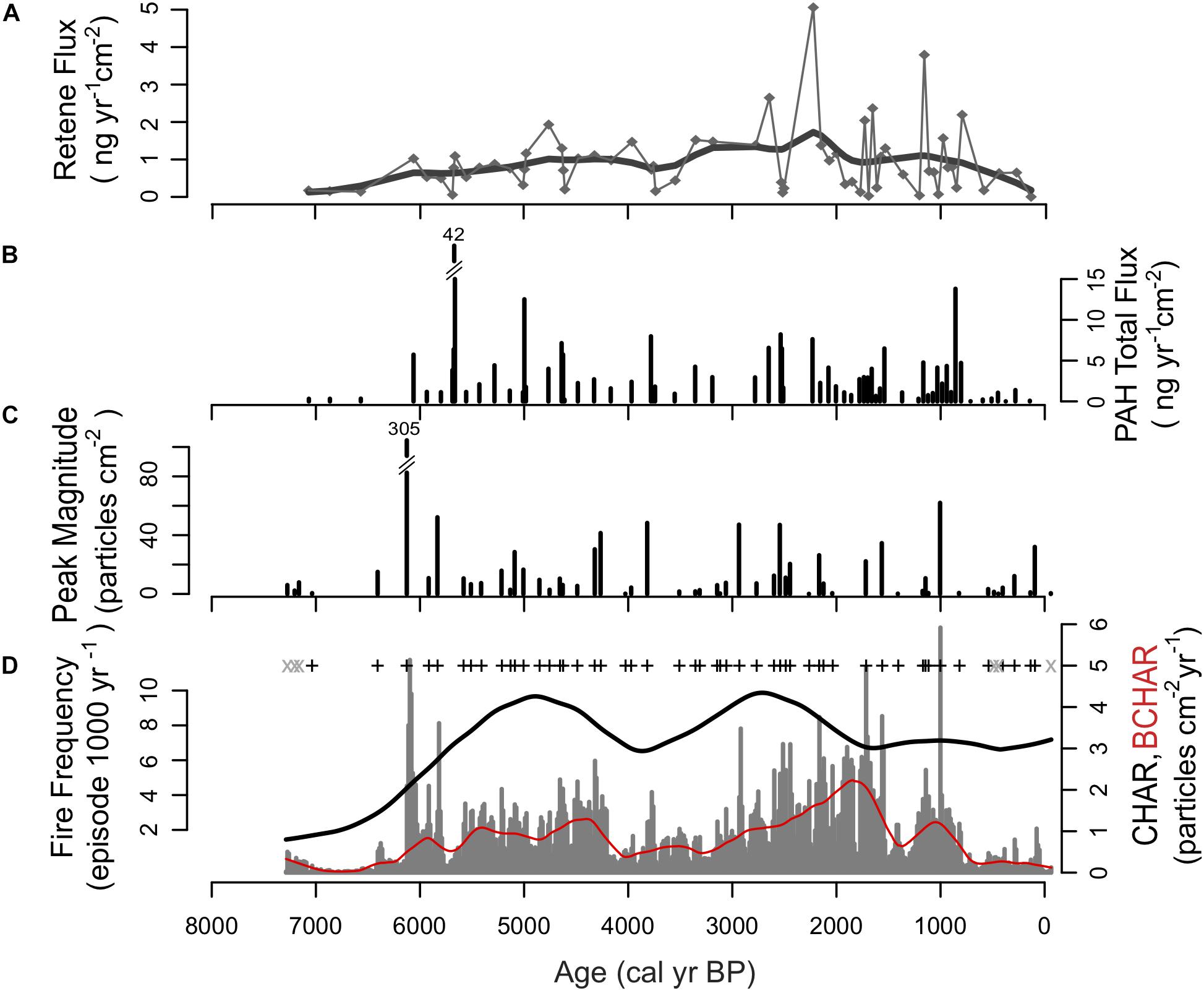
Figure 5. Reconstruction of fire activity from multiple proxies from Black Lake sediments. (A) PAH flux. (B) Retene flux. (C) Charcoal peak magnitude. (D) Charcoal influx and fire frequency data; gray “x” represent insignificant fire peaks; black “+” represent significant fire peaks; red line represents background charcoal component (BCHAR).
Zone BL2 (380–204 cm depth; 5600–2800 cal yr BP) was characterized by decreasing Artemisia (<1–8%) and Poaceae (5–20%) values, and an increase in total Pinus pollen (52–81%). Alnus (2–6%) and Cyperaceae values were steady (1–5%), with the exception of a period of low values (1–2%) from ca. 4300–3600 cal yr BP. Picea and Pseudotsuga/Larix pollen percentages increased in the end of this zone at 3150 cal yr BP (to a high of 8 and 6% respectively) suggesting a mixed conifer forest. PAR increased to an average of 5630 grains cm–2 yr–1, and AP:NAP increased throughout the zone. The higher AP:NAP ratio and Pinus pollen values over 70% suggest a forest environment (Iglesias et al., 2018).
CHAR and BCHAR were high from ca. 5600–4200 cal yr BP and then dropped until ca. 3000 cal yr BP. Fire frequency decreased from a high of 10 episodes 1000 yrs–1 to a low of 6 episodes 1000 yrs–1 during the period of lower CHAR values.
Zone BL3 (204–0 cm depth, 2800 cal yr BP-present day) had the lowest values of Poaceae (<1–13%), Artemisia (<1–2), total shrubs and herbs, and Cyperaceae. Pinus (65–87%) increased, as did Pseudotsuga/Larix (3–10%). PAR increased to an average of 7000 grains cm–2 yr–1, and AP:NAP was the highest of the record, all suggesting a closed forest environment.
CHAR and BCHAR reached their highest levels at ca. 2000 cal yr BP then decreased toward present day with the exception of one large peak at ca. 1000 cal yr BP. After an initial rise to 10 episodes 1000 yrs–1 at ca. 2500 cal yr BP, fire frequency declined to 6–7 episodes 1000 yrs–1, with an average fire return interval of 125 years to present day. The most recent fire episode occurred ca. 90 cal yr BP.
Biomarkers
n-Alkanes
Total n-alkane sum values (∑C22–33) were generally high between 6000 and 3000 cal yr BP and decreased to the present (Figure 6). In the period between 6000 and 3000 cal yr BP, ∑C22–33 was approximately 5 times higher than the more recent part of the record, suggesting that after 3000 cal yr BP angiosperm contribution to the n-alkane composite sum was diminished relative to gymnosperms within the Black Lake watershed (Figure 6).
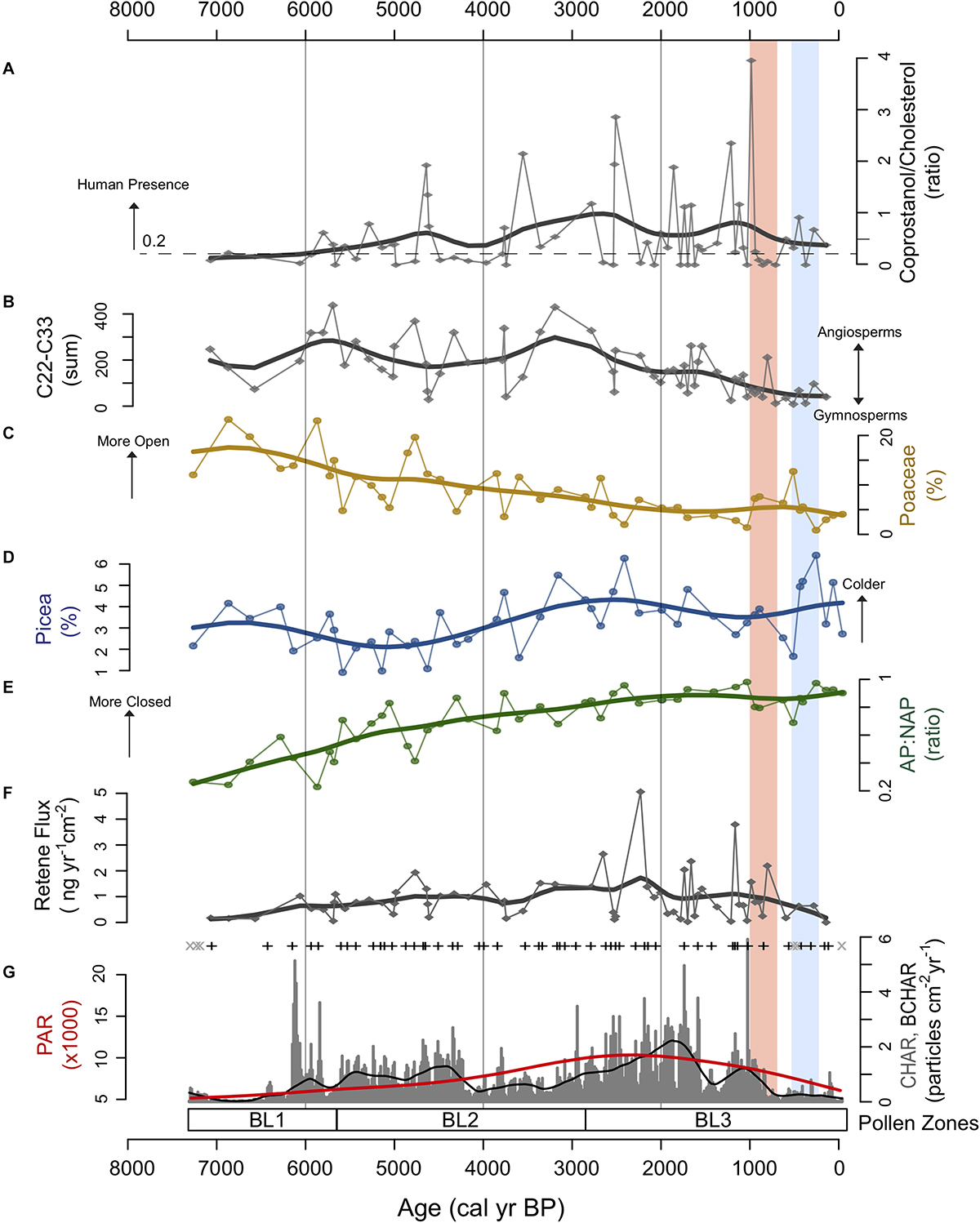
Figure 6. Multi-proxy comparison from Black Lake sediments. (A) Fecal sterol ratio. (B) Total n-alkane sum. (C–E) Pollen percentage data for select taxa and arboreal/non-arboreal pollen ratio (AP:NAP). (F) Retene flux. (G) Charcoal accumulation data and pollen accumulation rate (PAR); gray “x” represent insignificant fire peaks, black “+” represent significant fire peaks, black line represents background charcoal component (BCHAR), red line represents PAR. Red shading represents the Medieval Climate Anomaly (MCA, 1000–750 cal yr BP) and blue shading represents the Little Ice Age (LIA, 550–250 cal yr BP) (Mann et al., 2009).
Polycyclic Aromatic Hydrocarbons
The record shows a low PAH signal prior to ca. 5800 cal yr BP, indicating very low fire activity in this period, similar to the charcoal record (Figure 5). Between ca. 5800 and 2500 cal yr BP, PAHs peak every 500–1000 years. In this period, the largest peak at ca. 5800 cal yr BP is consistent with the charcoal record. High levels of PAHs were detected between ca. 3000 and 700 cal yr BP, in contrast with the period between ca. 700 cal yr BP to present, where the PAH signal was significantly lower. The lower PAH signal recorded in the most recent part of the record indicates that woody biomass burning in the Black Lake watershed during the last few centuries declined substantially. Retene values generally correspond to trends in macroscopic charcoal levels throughout the time series, recording peaks ca. 3000–2000 cal yr BP (BL3, Figure 5) when forest taxa became more dense and the availability of woody biomass increased as indicated by arboreal to non-arboreal ratios and pollen accumulation data.
Fecal Sterols
Low coprostanol threshold ratio (CTR) values indicate a potential absence of humans from ca. 7500 to 6000 cal yr BP, although a number of factors could result in low coprostanol values including poor preservation in lake sediments, low or intermittent human use of the Black Lake watershed and/or highly variable coprostanol deposition within and around Black Lake among others. Beginning ca. 5800 cal yr BP, values increased above the CTR of 0.2 and generally remained above this threshold until present. While variable, high CTR values during the mid- and late Holocene (eleven peaks > 1.0) provide strong evidence that human populations were present within the Black Lake watershed.
Discussion
Millennial-scale paleoenvironmental reconstructions from Black Lake sediments suggest climatic variability was the dominant control on broad-scale changes in the environment and fire activity during the early and mid-Holocene until the relationship between climate and fire activity decoupled during the cooler and wetter late Holocene. Biomarkers associated with human presence indicate long-term, yet episodic, human activity within the Black Lake watershed for over 6000 years. While climatic controls correspond with changes in fire activity during the early and mid-Holocene, the persistence of high levels of biomass burning during the generally cool and wet late-Holocene may signal human-set fires were used to maintain high levels of biomass burning to manage ecosystems within the Black Lake watershed.
Early to Middle Holocene – 7500–5500 cal yr BP
The Black Lake vegetation and fire record begins ca. 7500 cal yr BP during the early to middle Holocene, when summer insolation levels were ∼7% higher than present day (Berger and Loutre, 1991; Kutzbach et al., 1998). During this period, pollen data suggest an open forest of predominantly Pinus subgenus Pinus with Poaceae and Artemisia surrounding the lake. Pseudotsuga/larix was present, but not in high abundance. Based on the current amounts of Pinus ponderosa at low elevations in the Mission Valley as opposed to Pinus flexilis, the predominant species was likely Pinus ponderosa. Low AP:NAP ratios suggest a landscape with lower tree cover than present. The open landscape and xerophytic pollen taxa such as Poaceae and Artemisia agree with models that summers were warmer and drier than present (Braconnot et al., 2019). Despite a period of generally warmer and drier summers, there was minimal fire activity. The lack of macroscopic charcoal in the record could be the result of few fires on the landscape, or low-severity grass fires that did not input macroscopic charcoal into the lake basin (Allen et al., 2008; Swetnam et al., 2009).
Between ca. 6000 and 5500 cal yr BP, Poaceae and Artemisia decreased, and there was an increase in conifer trees, namely Pinus. Pseudotsuga menziessii increased slightly, but high levels of Pinus pollen suggest a forest of primarily pine. The decline of non-arboreal pollen coincident with the increase in arboreal pollen suggests that as summer insolation levels decreased into the middle Holocene, low-elevation forests expanded and became denser. Cyperaceae increased just prior to 6000 cal yr BP, likely a result of increased precipitation and higher lake water levels. A large charcoal peak (peak magnitude = 305 particles cm–2, Figure 5) occurred ca. 6000 cal yr BP and was followed by a substantial increase in CHAR, BCHAR, and PAH. This large fire and increase in charcoal accumulation and PAH was likely related to an increase in the density of conifer trees and associated increase in the availability of woody biomass for burning.
While pollen influx from conifers was likely dominated by forest canopy taxa, n-alkane data suggest that angiosperm shrub taxa (deciduous species such as Alnus, Betula, Salix, and Ceanothus) were abundant during this period. This suggests that there was an increase in available moisture toward the end of this period when both shrubs and conifer trees filled in the watershed that had been dominated by Poaceae and Artemisia from ca. 7500 to 6000 cal yr BP.
During the warm and dry early Holocene, biomarker data indicate humans were absent or present in low numbers. The lower elevations and the valley floor would have been very warm and dry and it is possible that during the height of early Holocene warming Black Lake may have dried. This is consistent with the basal clays found at the bottom of our record before ca. 7500 cal yr BP. As sediment accumulation initiated and increased, signs of human presence increased, coinciding with forest expansion and generally cooler and wetter conditions. Hence, the early absence of humans coincides with the initiation of sediment accumulation at Black Lake and may be related to climatic conditions (e.g., hot and dry) that were unfavorable for human activity prior to 6000 years ago.
Mid- to Late Holocene – 5500–2000 cal yr BP
Pinus ponderosa continued to expand throughout the mid- to late Holocene, and Picea, Abies, and Pseudotsuga/Larix increased. From 4000 to 3000 cal yr BP, the increasing PAR of Picea, Abies, and Pseudotsuga/Larix suggest further forest densification coincided with colder and wetter conditions as indicated by increased lake levels recorded in the region (Shuman and Serravezza, 2017) and glacial readvances in the Canadian Rockies (Solomina et al., 2015). A ratio of warm/dry (WD) associated taxa (Poaceae + Artemisia + Ameranthaceae + Juniperus) to cool/wet (CW) associated taxa (Picea + Abies + Alnus) indicate a prolonged interval of cool and wet conditions during this neoglacial period (Figure 7). A decrease in CHAR and BCHAR shown at the same time that forests expanded and became more dense, suggests cool and wet conditions likely suppressed fire activity.
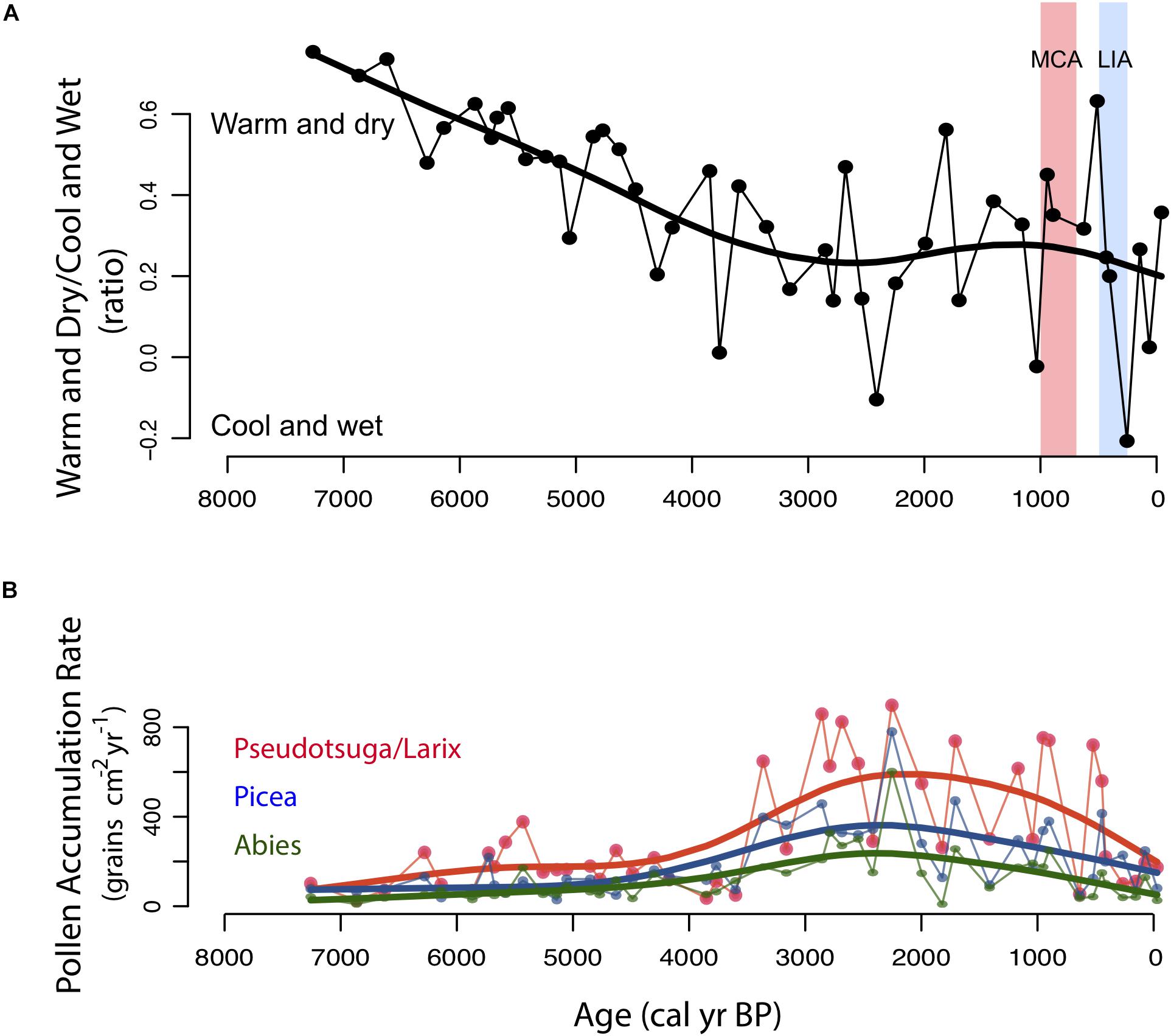
Figure 7. Pollen data from select taxa from Black Lake sediments. (A) Ratio of warm and dry pollen taxa versus cool and wet pollen taxa calculated with the equation: (WD-CW)/(WD + CW). Red shading represents the Medieval Climate Anomaly (MCA, 1000–750 cal yr BP) and blue shading represents the Little Ice Age (LIA, 550–250 cal yr BP) (Mann et al., 2009). (B) PAR of Pseudotsuga/Larix, Picea, and Abies.
Modest increases in Pseudotsuga/Larix and Pinus at the expense of Picea and Abies began ca. 3000 cal yr BP, as forest structure and composition became most similar to present day mixed-conifer forests. Fire activity increased in the late Holocene after 3000 cal yr BP, coinciding with the highest PAR levels, suggesting the density of forests may have peaked during this time period. The lack of fire activity and cool wet conditions from 4000 to 3000 cal yr BP could have allowed for the lower elevation forests to become more dense, providing high levels of available biomass to burn when conditions were conducive for fire spread.
Biomarkers indicate human presence increased after ca. 4000 cal yrs BP, during a period of cool and wet conditions. The signal of human presence is generally high, although intermittent, in this period. Due to the very local nature of the fecal biomarker signature, the absence/lower presence of humans in the watershed may be related to episodic, yet possibly intensive human use of the Black lake watershed throughout the mid- to late Holocene until biomarkers suggest limited use of the site in recent centuries.
Late Holocene to Present – 2000 cal yr BP-Present
Following the onset of generally cool and wet conditions ca. 4000–3000 cal yr BP, forest composition remained relatively stable with the exception of an increase in taxa associated with warm/dry conditions during the Medieval Climate Anomaly (MCA; 1000–750 cal yr BP) and an increase in taxa associated with cool/wet conditions during the Little Ice Age (LIA; 550–250 cal yr BP) (Mann et al., 1999; Cook et al., 2010; Pederson et al., 2011). During the MCA, the increase in levels of pollen of Poaceae (Figure 6), and decline in the WD:CW ratio (Figure 7), suggests that prolonged warm (and dry) conditions evident in climatic reconstructions for the NRM (Cook et al., 2010; Pederson et al., 2011) led to a decline in the density and abundance of forest. During the LIA, the increase in forest taxa pollen (notably Picea, and Pinus spp. including Pinus subgenus Strobus-type) at the expense of open vegetation taxa as indicated by the AP:NAP ratio (Figure 6) and WD:CW ratio (Figure 7), suggests forest expansion and infill.
Fire activity (CHAR) increased during the warmer and drier MCA and decreased during the cooler and wetter LIA. The largest fire peak of the CHAR record and increased PAH fluxes occur at the onset of the MCA, ca. 1000 cal yr BP. Fire frequency and background charcoal decrease slightly after the MCA from ca. 1000 cal yr BP to present, similar to other low elevation sites in the NRM (Carter et al., 2018). Low fire activity persisted during the LIA, possibly because cool and wet conditions limited fire spread within the Black Lake watershed. While fire activity decreased following the MCA, the persistence of abundant fire activity during the cool and wet conditions of the late-Holocene prior to the MCA suggest a possible role for human influence on fire management.
Evidence for human presence at Black Lake peaked during the late Holocene until ca. 700 cal yr BP when fecal biomarker data suggest humans were absent or infrequently using the Black Lake site. Increases in biomass burning coincide with biomarker data recording increased human presence and decline when humans appear to have abandoned or used the site infrequently.
Human Presence and Influence
Oral traditions from the Confederated Salish and Kootenai Tribes detail a rich relationship between Indigenous populations and the land of northwestern Montana that began thousands of years ago (CSKT, 2005). Fire was a critical part of this relationship and one of the most powerful tools used by Indigenous peoples of the northwestern US (and worldwide) to maintain important lifeways and to manage landscapes to promote culturally significant plants and animals (Bowman et al., 2011; Trauernicht et al., 2015). Native peoples historically used fire for a number of purposes, including: as a means of communication, for ceremonial activities, as well as to aid in hunting, promote the growth of valuable plant and animal resources, and facilitate travel (Barrett and Arno, 1999; Boyd, 1999; Lake et al., 2017). Archeological records (MacDonald, 2012) and analyses of a suite of radiocarbon dated cultural materials from the NRM suggest Native American populations were increasing in the NRM during the late Holocene (Gajewski et al., 2011). These data are consistent with population density estimates based on radiocarbon dated archeological materials and population growth models that suggest that human populations in the NRM increased from 0.1 person km–2 prior to 3000 cal yr BP to nearly 1 person km–2 after 2000 cal yr BP (Peros et al., 2010; Chaput et al., 2015).
Biomarker values from the Black Lake sediments corroborate archeological data from the NRM, indicating humans were using the Black Lake watershed for over 6000 years. CTR values exceed thresholds used to identify human presence (Carreira et al., 2015), and far exceed these thresholds numerous times throughout the record. These high CTR values suggest populations were intensively using the watershed episodically throughout the middle and late Holocene. Evidence for human presence continue to increase with the expansion of forests and cooler and wetter conditions, peaking between 4000 and 1000 years ago until ca. 700 cal yr BP when biomarkers associated with human presence decline. Despite the onset of generally cool and wet conditions in the late Holocene, fire activity remained high, pointing to a possible influence of humans in maintaining fire activity under conditions that would have otherwise maintained lower levels of fire activity. The seemingly-anomalous persistent high levels of biomass burning at Black Lake despite cool wet conditions in the late Holocene is consistent with paleoenvironmental reconstructions from a number of other sites in Washington, Oregon, and British Columbia which attribute this pattern to be a result of increased fuel availability, anthropogenic fires and/or increased interannual climate variability (Walsh et al., 2015; Alt et al., 2018). During the last millennium, CTR values from Black Lake decline, suggesting human presence decreased prior to widespread depopulation that occurred in western North America resulting from the introduction of European diseases (Boyd, 1990; Prentiss et al., 2005). Roos et al. (2018) document intensified communal bison hunting and trade in grassland systems northeast of Black Lake (ca. 50 km) during the last millennium. While lower CTR values recorded in Black Lake sediments from ca. 1000 cal yrs BP to present could result from a number of factors influencing the demography of Mission Valley Indigenous populations, the intensification of regional hunting and economic activity in adjacent NRM grasslands may have attracted populations in the Mission Valley to migrate east to participate. During the last two centuries European contact and subsequent regional depopulation from disease may have then further contributed to a persistent absence of human activity in the Black Lake watershed.
An evaluation of biomarkers associated with human presence provides several important findings: first, that humans were using the Black Lake watershed throughout the mid- and late Holocene; second, that consistent with records from throughout the inland northwestern US, human-set fires may explain persistently high biomass burning during a period when a cooling and wetting climate would have, without anthropogenic burning, limited biomass burning in mid-elevation forests of western Montana; and third, that a decline in biomarker levels during the last millennium is likely related to changes in demographic and land-use patterns that may be linked to changes in regional economic and demographic activity in adjacent grassland systems (Roos et al., 2018). In summary, this new information from fecal biomarkers corroborates previous research pointing to an important role of humans in managing fire regimes and vegetation during the last several millennia.
Conclusion
Low sedimentation rates, increased dominance of clays and pollen associated with arid taxa suggest Black Lake may have been dry for centuries to millennia prior to the basal dates for this record, ca. 7000 cal yr BP. Vegetation surrounding Black Lake during the warm and dry early to mid-Holocene was characterized by an open grassland, patchy forest mosaic. The onset of wetter and cooler conditions after ca. 6000 cal yr BP promoted an increase in arboreal pollen indicating an expansion and densification of mixed-conifer forests and an increase in biomass burning. Pollen and biomarker data indicate the infill/densification of forest taxa increased ca. 4000 cal yr BP with cooler and wetter conditions, a trend which persisted until present with the exception of a short pause during the warmer and drier MCA. Fire episodes were frequent within the Black Lake watershed, occurring every 100 years throughout most of the record. Frequent fire activity persisted after a period of cold and wet conditions ca. 4000–3000 cal yr BP. Elevated fire activity during this interval of generally cool and wet conditions could have resulted from an increase in available woody biomass and seasonality allowing for fuel drying and/or an increase in human-set fires used to manage these forest ecosystems. Fire activity and indicators of fire intensity increase during the warm and dry MCA then decrease during the LIA. Biomarker data and high CTR values suggest humans were present within the Black Lake watershed for most of the 7500 years record. Low fire activity during the last several hundred years was possibly related to first, changes in regional land-use patterns linked to regional economic intensification and, later, to eventual depopulation of the region of Indigenous peoples following the introduction of European diseases.
Oral traditions from the Confederated Salish and Kootenai tribes describe thousands of years of management of western Montana landscapes with fire. The reconstruction of human presence from organic biomarkers found in Black Lake sediments corroborates this long-term presence of humans and highlights a possible increased human influence on fire activity during the last 2–3 millennia. Coupling new records of human activity from organic biomarkers with pollen and charcoal records provides important new insights into the role of human-environment interactions in the NRM. The development of a network of organic biomarker records from a suite of sites could further advance our understanding of how Indigenous populations adapted and responded to climate variability through management of NRM ecosystems.
Data Availability Statement
The datasets generated for this study are available on request to the corresponding author.
Author Contributions
DM, MA, RE, and GP designed the research. DM and RE carried out paleoecological fieldwork. DM and MA carried out pollen, charcoal, geochemistry and magnetic susceptibility analyses. DB and EA carried out organic biomarker analyses. DM and MA led the writing of the manuscript with inputs from all other authors.
Funding
Funding support for this research came from the National Science Foundation awards BCS-1539820 and BCS-1832486 to DM, MA, RE, GP, and EH and USDA National Institute of Food and Agriculture (NIFA; 2013-38424-20992) RE, DM, MA, GP, and EH. Additional funding was provided by the European Research Council under the European Union’s Seventh Framework Programme (FP7/2007–2013)/ERC Grant agreement No. 267696 – EARLYhumanIMPACT.
Conflict of Interest
The authors declare that the research was conducted in the absence of any commercial or financial relationships that could be construed as a potential conflict of interest.
Acknowledgments
Valued support was provided by the Confederated Salish and Kootenai Tribes, specifically Mike Durglo of CSKT Cultural Preservation, Rich Jansen of the Department of Natural Resources and Jim Durglo of CSKT Tribal Forestry, which allowed us to conduct research on tribal lands. The Salish Cultural Committee, and Salish Kootenai College provided additional support. We thank Emily Heyerdahl at the U.S. Forest Service, Rocky Mountain Research Station, Missoula, MT for supporting fieldwork efforts and providing helpful comments that were useful in revising drafts of this paper. Any use of trade, firm, or product names is for descriptive purposes only and does not imply endorsement by the U.S. Government.
Supplementary Material
The Supplementary Material for this article can be found online at: https://www.frontiersin.org/articles/10.3389/ffgc.2020.00044/full#supplementary-material
Footnotes
References
Ahad, J. M. E., Jautzy, J. J., Cumming, B. F., Das, B., Laird, K. R., and Sanei, H. (2015). Sources of polycyclic aromatic hydrocarbons (PAHs) to northwestern Saskatchewan lakes east of the Athabasca oil sands. Org. Geochem. 80, 35–45. doi: 10.1016/j.orggeochem.2015.01.001
Allen, C. D., Anderson, R. S., Jass, R. B., Toney, J. L., and Baisan, C. H. (2008). Paired charcoal and tree-ring records of high-frequency Holocene fire from two New Mexico bog sites. Int. J. Wildl. Fire 17, 115–130.
Alt, M., McWethy, D. B., Everett, R., and Whitlock, C. (2018). Millennial scale climate-fire-vegetation interactions in a mid-elevation mixed coniferous forest, Mission Range, northwestern Montana, USA. Quat. Int. 90, 66–82. doi: 10.1017/qua.2018.25
Argiriadis, E., Battistel, D., McWethy, D. B., Vecchiato, M., Kirchgeorg, T., Kehrwald, N. M., et al. (2018). Lake sediment fecal and biomass burning biomarkers provide direct evidence for prehistoric human-lit fires in New Zealand. Sci. Rep. 8:12113. doi: 10.1038/s41598-018-30606-3
Arno, S. F., Smith, H. Y., and Krebs, M. A. (1997). “Old Growth ponderosa pine and western larch stand structures: influences of pre-1900 fires and fire exclusion,” in F.S. U.S. Department of Agriculture, (Ogden, UT: Intermountain Research Station).
Balch, J., Schoennagel, T., Williams, A., Abatzoglou, J., Cattau, M., Mietkiewicz, N., et al. (2018). Switching on the Big Burn of 2017. Fire 1:17. doi: 10.3390/fire1010017
Barrett, S., and Arno, S. (1999). “Indian fires in the Northern Rockies,” in Indians, Fire and the Land in the Pacific Northwest, ed. R. Boyd (Corvallis: Oregon State University Press), 50–64.
Battistel, D., Argiriadis, E., Kehrwald, N., Spigariol, M., Russell, J. M., and Barbante, C. (2016). Fire and human record at Lake Victoria, East Africa, during the early iron age: did humans or climate cause massive ecosystem changes? Holocene 27, 997–1007. doi: 10.1177/0959683616678466
Battistel, D., Piazza, R., Argiriadis, E., Marchiori, E., Radaelli, M., and Barbante, C. (2015). GC-MS method for determining faecal sterols as biomarkers of human and pastoral animal presence in freshwater sediments. Anal. Bioanal. Chem. 407, 8505–8514. doi: 10.1007/s00216-015-8998-2
Beaty, R. M., and Taylor, A. H. (2009). A 14 000 year sedimentary charcoal record of fire from the northern Sierra Nevada. Lake Tahoe Basin, California, USA. Holocene 19, 347–358. doi: 10.1177/0959683608101386
Bennett, K. D., and Willis, K. J. (2002). “Pollen,” in Tracking Environmental Change Using Lake Sediments. Terrestrial, Algal, and Siliceous Indicators, Vol. 3, eds J. P. Smol, H. J. B. Birks, and W. M. Last (Dordrecht: Kluwer Academic Publishers), 5–32.
Berger, A., and Loutre, M. F. (1991). Insolation values for the climate of the last 10 million of years. Q. Sci. Rev. 10, 297–317. doi: 10.1016/0277-3791(91)90033-q
Birk, J. J., Teixeira, W. G., Neves, E. G., and Glaser, B. (2011). Faeces deposition on Amazonian Anthrosols as assessed from 5β-stanols. J. Archaeol. Sci. 38, 1209–1220. doi: 10.1016/j.jas.2010.12.015
Blaauw, M., and Christen, J. A. (2011). Flexible paleoclimate age-depth models using an autoregressive gamma process. Bayesian Anal. 6, 457–474. doi: 10.1214/ba/1339616472
Bowman, D. M. J. S., Balch, J., Artaxo, P., Bond, W. J., Cochrane, M. A., D’Antonio, C. M., et al. (2011). The human dimension of fire regimes on Earth. J. Biogeogr. 38, 2223–2236. doi: 10.1111/j.1365-2699.2011.02595.x
Boyd, R. (1999). Indians, fire and the Land in the Pacific Northwest. Corvallis: Oregon State University Press.
Boyd, R. T. (1990). “Demographic history, 1774-1874,” in Handbook of North American Indians: Vol 7. Northwest Coast, ed. W. P. Suttles (Washington D.C: Smithsonian Institution), 135–147.
Braconnot, P., Zhu, D., Marti, O., and Servonnat, J. (2019). Strengths and challenges for transient Mid- to Late Holocene simulations with dynamical vegetation. Clim. Past 15, 997–1024. doi: 10.5194/cp-15-997-2019
Bull, I. D., Lockheart, M. J., Elhmmali, M. M., Roberts, D. J., and Evershed, R. P. (2002). The origin of faeces by means of biomarker detection. Environ. Int. 27, 647–654. doi: 10.1016/S0160-4120(01)00124-6
Bush, R. T., and McInerney, F. A. (2013). Leaf wax n-alkane distributions in and across modern plants: implications for paleoecology and chemotaxonomy. Geochim. Cosmochim. Acta 117, 161–179. doi: 10.1016/j.gca.2013.04.016
Carreira, R. S., Albergaria-Barbosa, A. C. R., Arguelho, M. L. P. M., and Garcia, C. A. B. (2015). Evidence of sewage input to inner shelf sediments in the NE coast of Brazil obtained by molecular markers distribution. Mar. Pollut. Bull. 90, 312–316. doi: 10.1016/j.marpolbul.2014.11.011
Carter, V. A., Power, M. J., Lundeen, Z. J., Morris, J. L., Petersen, K. L., Brunelle, A., et al. (2018). A 1,500-year synthesis of wildfire activity stratified by elevation from the U.S. Rocky Mountains. Q. Int. 488, 107–119. doi: 10.1016/j.quaint.2017.06.051
Chaput, M. A., Kriesche, B., Betts, M., Martindale, A., Kulik, R., Schmidt, V., et al. (2015). Spatiotemporal distribution of Holocene populations in North America. Proc. Natl. Acad. Sci. U.S.A. 112, 12127–12132. doi: 10.1073/pnas.1505657112
Cook, E. R., Seager, R., Heim, R. R. Jr., Vose, R. S., Herweijer, C., and Woodhouse, C. (2010). Megadroughts in North America: placing IPCC projections of hydroclimatic change in a long-term palaeoclimate context. J. Q. Sci. 25, 48–61. doi: 10.1002/jqs.1303
CSKT (2005). Confederated Salish & Kootenai Tribes - Forest Management Plan - An Ecosystem Approach to Tribal Forest Management: Flathead Reservation Comprehensive Resources. Pablo: CSKT.
Davis, M. B. (1976). Pleistocene biogeography of temperate deciduous forests. Geosci. Man 13, 13–26.
Dennison, P. E., Brewer, S. C., Arnold, J. D., and Moritz, M. A. (2014). Large wildfire trends in the western United States, 1984-2011. Geophys. Res. Lett. 41, 2928–2933. doi: 10.1002/2014gl059576
Diefendorf, A. F., and Freimuth, E. J. (2017). Extracting the most from terrestrial plant-derived n-alkyl lipids and their carbon isotopes from the sedimentary record: a review. Org. Geochem. 103, 1–21. doi: 10.1016/j.orggeochem.2016.10.016
Dodge, M. (1972). Forest fuel accumulation—a growing problem. Science 177:139. doi: 10.1126/science.177.4044.139
Donato, D. C., Fontaine, J. B., Campbell, J. L., Robinson, W. D., Kauffman, J. B., and Law, B. E. (2006). Post-wildfire logging hinders regeneration and increases fire risk. Science 311, 352–352. doi: 10.1126/science.1122855
Gajewski, K., Munoz, S., Peros, M., Viau, A., Morlan, R., and Betts, M. (2011). The Canadian Archaeological Radiocarbon Database (Card): archaeological 14C Dates in North America and their paleoenvironmental context. Radiocarbon 53, 371–394. doi: 10.1017/S0033822200056630
Grimm, E. C. (1987). CONISS: a FORTRAN 77 program for stratigraphically constrained cluster analysis by the method of incremental sum of squares. Comput. Geosci. 13, 13–35. doi: 10.1016/0098-3004(87)90022-7
Halofsky, J. E., Donato, D. C., Hibbs, D. E., Campbell, J. L., Cannon, M. D., Fontaine, J. B., et al. (2011). Mixed-severity fire regimes: lessons and hypotheses from the Klamath-Siskiyou Ecoregion. Ecosphere 2:art40. doi: 10.1890/es10-00184.1
Heyerdahl, E. K., Morgan, P., and Riser, J. P. II (2008). Multi-season climate synchronized widespread historical fires in dry forests (1650-1900), Northern Rockies, USA. Ecology 89, 705–716. doi: 10.1890/06-2047.1
Higuera, P. E., Brubaker, L. B., Anderson, P. M., Hu, F. S., and Brown, T. A. (2009). Vegetation mediated the impacts of postglacial climate change on fire regimes in the south-central Brooks Range, Alaska. Ecol. Monogr. 79, 201–219. doi: 10.1890/07-2019.1
Higuera, P. E., Sprugel, D. G., and Brubaker, L. B. (2005). Reconstructing fire regimes with charcoal from small-hollow sediments: a calibration with tree-ring records of fire. Holocene 15, 238–251. doi: 10.1191/0959683605hl789rp
Higuera, P. E., Whitlock, C., and Gage, J. A. (2010). Linking tree-ring and sediment-charcoal records to reconstruct fire occurrence and area burned in subalpine forests of Yellowstone National Park USA. Holocene 21, 327–341. doi: 10.1177/0959683610374882
Iglesias, V., Whitlock, C., Krause, T. R., and Baker, R. G. (2018). Past vegetation dynamics in the Yellowstone region highlight the vulnerability of mountain systems to climate change. J. Biogeogr. 45, 1768–1780. doi: 10.1111/jbi.13364
Kapp, R. O., Davis, O. K., and King, J. E. (2000). Pollen and Spores. Dallas, TX: American Association of Stratigraphic Palynologists Foundation.
Kirchgeorg, T., Schüpbach, S., Kehrwald, N., McWethy, D. B., and Barbante, C. (2014). Method for the determination of specific molecular markers of biomass burning in lake sediments. Org. Geochem. 71, 1–6. doi: 10.1016/j.orggeochem.2014.02.014
Klos, P. Z., Link, T. E., and Abatzoglou, J. T. (2014). Extent of the rain-snow transition zone in the western U.S. under historic and projected climate. Geophys. Res. Lett. 41:2014GL060500. doi: 10.1002/2014GL060500
Kutzbach, J., Gallimore, R., Harrison, S., Behling, P., Selin, R., and Laarif, F. (1998). Climate and biome simulations for the past 21,000 years. Q. Sci. Rev. 17, 473–506. doi: 10.1016/s0277-3791(98)00009-2
Lake, F. K., Wright, V., Morgan, P., McFadzen, M., McWethy, D., and Stevens-Rumann, C. (2017). Returning fire to the land—celebrating traditional knowledge and fire. J. Forest. 115, 343–353. doi: 10.5849/jof.2016-043R2
Leeming, R., Ball, A., Ashbolt, N., and Nichols, P. (1996). Using faecal sterols from humans and animals to distinguish faecal pollution in receiving waters. Water Res. 30, 2893–2900. doi: 10.1016/S0043-1354(96)00011-5
MacDonald, D. H. (2012). Montana Before History: 11,000 Years of Hunter-Gatherers in the Rockies and Plains. Missoula: Mountain press Publishing Company.
Mann, M. E., Bradley, R. S., and Hughes, M. K. (1999). Northern hemisphere temperatures during the past millennium: inferences, uncertainties, and limitations. Geophys. Res. Lett. 26, 759–762. doi: 10.1029/1999gl900070
Mann, M. E., Zhang, Z., Rutherford, S., Bradley, R. S., Hughes, M. K., Shindell, D., et al. (2009). Global signatures and dynamical origins of the little ice age and medieval climate anomaly. Science 326, 1256–1260. doi: 10.1126/science.1177303
McAndrews, J. H., Berti, A. A., and Norris, G. N. (1973). Key to Quaternary Pollen and Spores of the Great Lakes Region. Toronto: Royal Ontario Museum.
McWethy, D. B., Higuera, P. E., Whitlock, C., Veblen, T. T., Bowman, D. M. J. S., Cary, G. J., et al. (2013). A conceptual framework for predicting temperate ecosystem sensitivity to human impacts on fire regimes. Glob. Ecol. Biogeogr. 22, 900–912. doi: 10.1111/geb.12038
Menounos, B. (1997). The water content of lake sediments and its relationship to other physical parameters: an alpine case study. Holocene 7, 207–212. doi: 10.1177/095968369700700208
Meyers, P. A. (1997). Organic geochemical proxies of paleoceanographic, paleolimnologic, and paleoclimatic processes. Org. Geochem. 27, 213–250. doi: 10.1016/S0146-6380(97)00049-1
Miller, A. D., Thompson, J. R., Tepley, A. J., and Anderson-Teixeira, K. J. (2019). Alternative stable equilibria and critical thresholds created by fire regimes and plant responses in a fire-prone community. Ecography 42, 55–66. doi: 10.1111/ecog.03491
Odion, D. C., Moritz, M. A., and DellaSala, D. A. (2010). Alternative community states maintained by fire in the Klamath Mountains USA. J. Ecolo. 98, 96–105. doi: 10.1111/j.1365-2745.2009.01597.x
Page, D. S., Boehm, P. D., Douglas, G. S., Bence, A. E., Burns, W. A., and Mankiewicz, P. J. (1999). Pyrogenic polycyclic aromatic hydrocarbons in sediments record past human activity: a case study in prince william sound alaska. Mar. Pollut. Bull. 38, 247–260. doi: 10.1016/S0025-326X(98)00142-8
Pederson, G. T., Gray, S. T., Ault, T., Marsh, W., Fagre, D. B., Bunn, A. G., et al. (2010). Climatic controls on the snowmelt hydrology of the Northern Rocky mountains. J. Clim. 24, 1666–1687. doi: 10.1175/2010jcli3729.1
Pederson, G. T., Gray, S. T., Woodhouse, C. A., Betancourt, J. L., Fagre, D. B., Littell, J. S., et al. (2011). The unusual nature of recent snowpack declines in the North American Cordillera. Science 333, 332–335. doi: 10.1126/science.1201570
Peros, M. C., Munoz, S. E., Gajewski, K., and Viau, A. E. (2010). Prehistoric demography of North America inferred from radiocarbon data. J. Archaeol. Sci. 37, 656–664. doi: 10.1016/j.jas.2009.10.029
Piazza, R., Gambaro, A., Argiriadis, E., Vecchiato, M., Zambon, S., Cescon, P., et al. (2013). Development of a method for simultaneous analysis of PCDDs, PCDFs, PCBs, PBDEs, PCNs and PAHs in Antarctic air. Anal. Bioanal. Chem. 405, 917–932. doi: 10.1007/s00216-012-6464-y
Prentiss, W. C., Chatters, J. C., Lenert, M., Clarke, D. S., and O’Boyle, R. C. (2005). The archaeology of the plateau of Northwestern North America during the late prehistoric period (3500–200 B.P.): evolution of hunting and gathering societies. J. World Prehist. 19, 47–118. doi: 10.1007/s10963-005-9001-5
PRISM Climate Group (2015). Oregon State University. Available online at: http://prism.oregonstate.edu (accessed May 1, 2019).
Prism Climate Group (2019). PRISM Climate Group. Available online at: http://prism.oregonstate.edu (accessed May 1, 2019).
R Core Team (2015). R: A Language and Environment for Statistical Computing. Vienna: R Foundation for Statistical Computing. Available online at: https://www.R-project.org/
Raffa, K. F., Aukema, B. H., Bentz, B. J., Carroll, A. L., Hicke, J. A., Turner, M. G., et al. (2008). Cross-scale drivers of natural disturbances prone to anthropogenic amplification: the dynamics of bark beetle eruptions. Bioscience 58, 501–517. doi: 10.1641/b580607
Ramdahl, T. (1983). Retene—a molecular marker of wood combustion in ambient air. Nature 306, 580–582. doi: 10.1038/306580a0
Reimer, P. J., Bard, E., Bayliss, A., Beck, J. W., Blackwell, P. G., Ramsey, C. B., et al. (2013). IntCal13 and Marine13 Radiocarbon Age Calibration Curves 0–50,000 Years cal BP. Radiocarbon 55, 1869–1887. doi: 10.2458/azu_js_rc.55.16947
Roos, C. I., Zedeño, M. N., Hollenback, K. L., and Erlick, M. M. H. (2018). Indigenous impacts on North American Great Plains fire regimes of the past millennium. Proc. Natl. Acad. Sci. U.S.A. 115, 8143. doi: 10.1073/pnas.1805259115
Schoennagel, T., and Nelson, C. R. (2011). Restoration relevance of recent National Fire Plan treatments in forests of the western United States. Front. Ecol. Environ. 9:271–277. doi: 10.1890/090199
Shuman, B. N., and Serravezza, M. (2017). Patterns of hydroclimatic change in the Rocky Mountains and surrounding regions since the last glacial maximum. Q. Sci. Rev. 173, 58–77. doi: 10.1016/j.quascirev.2017.08.012
Solomina, O. N., Bradley, R. S., Hodgson, D. A., Ivy-Ochs, S., Jomelli, V., Mackintosh, A. N., et al. (2015). Holocene glacier fluctuations. Q. Sci. Rev. 111, 9–34. doi: 10.1016/j.quascirev.2014.11.018
Swetnam, T. W., Baisan, C. H., Caprio, A. C., Brown, P. M., Touchan, R., Anderson, R. S., et al. (2009). Multi-millennial Fire History of the Giant Forest, Sequoia National Park, California, USA. Fire Ecol. 5, 120–150. doi: 10.4996/fireecology.0503120
Swetnam Thomas, W., Farella, J., Roos Christopher, I., Liebmann Matthew, J., Falk Donald, A., and Allen Craig, D. (2016). Multiscale perspectives of fire, climate and humans in western North America and the Jemez Mountains USA. Philos. Trans. R. Soc. B 371:20150168. doi: 10.1098/rstb.2015.0168
Takada, H., Farrington, J. W., Bothner, M. H., Johnson, C. G., and Tripp, B. W. (1994). Transport of sludge-derived organic pollutants to deep-sea sediments at deep water dump site 106. Environ. Sci. Technol. 28, 1062–1072. doi: 10.1021/es00055a015
Tepley Alan, J., Jonathan, R., Epstein Howard, E., and Anderson-Teixeira Kristina, J. (2017). Vulnerability to forest loss through altered postfire recovery dynamics in a warming climate in the Klamath Mountains. Glob. Change Biol. 23, 4117–4132. doi: 10.1111/gcb.13704
Trauernicht, C., Brook, B. W., Murphy, B. P., Williamson, G. J., and Bowman, D. M. J. S. (2015). Local and global pyrogeographic evidence that indigenous fire management creates pyrodiversity. Ecol. Evol. 5, 1908–1918. doi: 10.1002/ece3.1494
Vicente, A., Alves, C., Monteiro, C., Nunes, T., Mirante, F., Cerqueira, M., et al. (2012). Organic speciation of aerosols from wildfires in central Portugal during summer 2009. Atmosph. Environ. 57, 186–196. doi: 10.1016/j.atmosenv.2012.04.030
Walsh, M. K., Marlon, J. R., Goring, S. J., Brown, K. J., and Gavin, D. G. (2015). A Regional Perspective on Holocene Fire–Climate–Human Interactions in the Pacific Northwest of North America. Ann. Assoc. Am. Geogr. 105, 1135–1157. doi: 10.1080/00045608.2015.1064457
Whitlock, C., Higuera, P. E., McWethy, D. B., and Briles, C. E. (2010). Paleoecological perspective on fire ecology: revisiting the fire regime concept. Open Ecol. J. 3, 6–23. doi: 10.2174/1874213001003020006
Whitlock, C., and Larsen, C. (2001). “Charcoal as a fire proxy,” in Tracking Environmental Change Using Lake Sediments: Volume 3 Terrestrial, Algal, and Siliceous indicators, eds J. P. Smol, H. J. B. Birks, and W. M. Last (Dordrecht: Kluwer Academic Publishers), 75–97. doi: 10.1007/0-306-47668-1_5
Wright, H. E., Mann, D. H., and Glaser, P. H. (1984). Piston corers for peat and lake sediments. Ecology 65, 657–659. doi: 10.2307/1941430
Keywords: anthropogenic fire, pollen, charcoal, sterols, biomarkers, mixed-conifer forest, fire
Citation: McWethy DB, Alt M, Argiriadis E, Battistel D, Everett R and Pederson GT (2020) Millennial-Scale Climate and Human Drivers of Environmental Change and Fire Activity in a Dry, Mixed-Conifer Forest of Northwestern Montana. Front. For. Glob. Change 3:44. doi: 10.3389/ffgc.2020.00044
Received: 04 November 2019; Accepted: 23 March 2020;
Published: 17 April 2020.
Edited by:
Rachel Loehman, United States Geological Survey, United StatesReviewed by:
Christopher Izaak Roos, Southern Methodist University, United StatesPatrick John Bartlein, University of Oregon, United States
Copyright © 2020 McWethy, Alt, Argiriadis, Battistel, Everett and Pederson. This is an open-access article distributed under the terms of the Creative Commons Attribution License (CC BY). The use, distribution or reproduction in other forums is permitted, provided the original author(s) and the copyright owner(s) are credited and that the original publication in this journal is cited, in accordance with accepted academic practice. No use, distribution or reproduction is permitted which does not comply with these terms.
*Correspondence: David B. McWethy, ZGF2ZW1jd2V0aHlAZ21haWwuY29t
†ORCID: Gregory T. Pederson, orcid.org/0000-0002-6014-1425