- 1Department of Ecosystem Science and Management, Texas A&M University, College Station, TX, United States
- 2Department of Forest Resources, University of Minnesota, St. Paul, MN, United States
- 3School of Forest Resources and Conservation, University of Florida, Gainesville, FL, United States
In 2011, the state of Texas experienced its worst drought ever recorded, breaking statewide temperature, and precipitation records. With climate predictions suggesting increases in the severity and extent of future droughts in this region, forest managers will need to plan for such events to minimize tree mortality. In east Texas, pine species are economically and ecologically important and are often managed, providing an opportunity to examine silvicultural strategies for mitigating exceptional drought mortality. We used U.S. Forest Service Forest Inventory and Analysis data and Bayesian, logistic, mixed effects regression to model individual tree mortality and the effect of stand structure (i.e., tree size, relative density, and species dominance) on three major pine groups, planted (PL) and naturally-regenerated (NL) loblolly pine (Pinus taeda L.) and all shortleaf pine (SL, Pinus echinata Mill.), under pre-drought and drought periods in east Texas. These groups represent a spectrum of management intensity with PL generally intensively managed and NL and SL relatively unmanaged. Moreover, loblolly pine tends to be production-oriented while shortleaf pine has perceived drought tolerance. Surprisingly, pine mortality did not increase significantly from pre-drought to drought periods in spite of the record drought conditions. However, mortality differed between pine groups and in response to stand structure for loblolly pine. Planted loblolly was least affected as mortality rate increased 9.8%. In contrast, NL and SL pine mortality rates were significantly higher than PL and increased 26.3 and 20.0%, respectively. The smallest and largest stems experienced elevated mortality under both periods, notably PL under exceptional drought. As expected, higher densities of loblolly pine exacerbated exceptional drought mortality. Surprisingly, greater overstory diversity for NL reduced mortality under exceptional drought. Despite the unprecedented hot and dry conditions of the 2011 drought, our results suggest that current practices in PL that manage relative density and tree size for non-drought conditions confers mortality resistance under exceptional drought. In NL stands, mortality resistance could be increased through active thinning and promoting greater overstory diversity. These results offer critical knowledge for managers tasked with providing continued forest resources in the face of future exceptional droughts.
Introduction
Future climate is predicted to become hotter and increase the extent and severity of future droughts worldwide [Intergovernmental Panel on Climate Change (IPCC), 2013]. Forests may already be responding to climatic changes (van Mantgem et al., 2009; Peng et al., 2011) in part through increases in drought-related tree mortality (Allen et al., 2015). Elevated mortality and possible tree die-offs from future exceptional droughts could have profound ramifications on forested systems (Anderegg et al., 2013) and represent a major challenge to resource managers tasked with maintaining healthy, productive forests in an uncertain future (Clark et al., 2016; Vose et al., 2016). Manipulating stand structure and composition through silvicultural practices could mitigate stressful conditions and provide resistance to mortality from future disturbances (Puettmann, 2011). However, knowledge of whether such tools could be effective for increasing forest resistance to mortality from future exceptional droughts remains virtually non-existent.
Forests of the southeastern U.S. are highly productive and economically important generating more timber volume than any other region in the country (Oswalt et al., 2014). Nearly 20% of all pine-dominated forest in the southeastern U.S. is comprised of intensively managed plantations (Chen et al., 2017) often receiving competition control, fertilization, and planting of genetically improved seedlings at calculated densities (Fox et al., 2007). In east Texas, this paradigm holds true for loblolly pine (Pinus taeda L.) which occurs largely as both heavily managed planted pine and unmanaged or minimally-managed, naturally-regenerated pine (Edgar and Zehnder, 2017). This dichotomy in loblolly pine condition has led to questions about the functionality of plantations compared to naturally-regenerated stands of this species under extreme drought conditions. Evidence from a comparison of physiological characteristics (i.e., root hydraulic conductivity, root:shoot ratios) suggested that plantation loblolly pine should be more drought-sensitive than naturally-regenerated pine in terms of productivity (Domec et al., 2015) possibly driven by fertilization inputs affecting transpiration and root production (Ward et al., 2015). However, fertilized plantation pine at the western edge of its range increased water use efficiency and sustained productivity under water-limited conditions (Maggard et al., 2017; Bracho et al., 2018) suggesting intensively managed pine could better cope with drought. Still, these studies focused on productivity response under more moderate drought conditions. Knowledge of the mortality response of these pine groups to exceptional drought remains virtually non-existent and the implications could have cascading economic impacts throughout the most productive forests in the U.S.
Species selection for planting may play a critical role in the mortality response of forests to future exceptional droughts. In the southeastern U.S., shortleaf pine (Pinus echinata Mill.) has a perceived potential to withstand elevated water stress given its historical occurrence across a range of site conditions including xeric sites and rocky outcrops (Mattoon, 1915). Under non-drought conditions, mature shortleaf pine sustained higher mortality and lower productivity than loblolly pine in southeastern Oklahoma (Dipesh et al., 2015). However, no study has compared the mortality response of mature shortleaf pine to more commonly occurring southern pine species under drought conditions, moderate, or exceptional. Shortleaf pine has seen drastically reduced dominance because of logging and subsequent fire suppression (Barrett, 1995) leading to widespread restoration initiatives to increase its prevalence in the southeastern U.S. (e.g., Shortleaf Pine Initiative). East Texas is one region in which this species could be targeted for restoration efforts. Yet, very little information exists on growth and mortality responses of shortleaf pine to drought, and none under exceptional drought. Ultimately, this gap in knowledge hinders management efforts aimed at successfully restoring this declining species in an uncertain climate future.
Stand structure (e.g., tree size, stem density, and species composition) represents one set of conditions most easily manipulated by managers for mitigating negative drought effects (Clark et al., 2016). The smallest and largest trees tend to experience higher mortality rates under non-drought conditions, often termed “U-shaped” or “J-shaped” mortality curves (Lines et al., 2010; Dietze and Moorcroft, 2011). Small stems typically comprise the regenerating component of early-successional forests and drought-related increases in mortality in this group could alter future forest composition (Thrippleton et al., 2018). Large trees play important ecological roles in forested ecosystems (Lindenmayer et al., 2012) yet, recent evidence suggests that they may be most susceptible to extreme drought conditions and are suffering disproportionate mortality worldwide (Lindenmayer et al., 2012; Bennett et al., 2015). However, these patterns in large tree drought mortality have been variable and difficult to confirm (Floyd et al., 2009; Klos et al., 2009; Ganey and Vojta, 2011). Additionally, alleviating competition for limited resources by reducing stand density and basal area (cross-sectional stem area at 1.37 m height) has long been utilized by practitioners to improve growth and productivity. Recent evidence highlights that, reducing competition through silvicultural thinning has improved growth response to water stress (D'Amato et al., 2013; Bottero et al., 2017; Gleason et al., 2017). However, unprecedented exceptional drought conditions that drive very low soil water potentials may negate any benefits gained from reduced competition resulting in increased tree mortality regardless of density (Floyd et al., 2009). Finally, stand species composition can be an important factor in affecting drought mortality (Klos et al., 2009; Cavin et al., 2013) as interactions with water and nutrient pools may differ among species (Forrester, 2014). Neighboring trees of different species may show facilitation via hydraulic lift more than competition (Pretzsch et al., 2013) or access different resource pools alleviating stressful conditions (Kramer and Holscher, 2010) which may be exacerbated in single-species-dominated stands. However, despite the wealth of knowledge on controlling stand structure to achieve desired outcomes, critical knowledge gaps exist in understanding whether common management practices remain effective for increasing pine resistance to future exceptional drought mortality.
From October 2010 to September 2011, the state of Texas experienced its worst drought on record with over 80% of the land area under the most severe (i.e., exceptional) drought classification (Nielsen-Gammon, 2012). The heavily forested region of east Texas suffered similarly exceptional hot and dry temperature and precipitation patterns seen statewide, having the hottest summer temperature deviation (+3.1°C) and lowest 12-month precipitation (619 mm; 47% lower than twentieth century average of 1,162 mm) ever recorded [National Oceanic and Atmospheric Administration (NOAA), 2018] Within east Texas, Pinus mortality was lower than other common genera such as Quercus and Liquidambar yet still experienced elevated levels of mortality from the harsh conditions (Moore et al., 2016; Klockow et al., 2018). The record exceptional drought of 2011 provides a critical opportunity to examine more closely southern pine vulnerability to exceptional drought and to identify specific aspects of stand structure that could be manipulated to develop adaptive management strategies for increasing resistance to exceptional drought mortality. Using national forest inventory plots with complete and systematic coverage of east Texas, we addressed the following objectives: (1) examine mortality rates of three common pine species groups (i.e., planted loblolly pine, naturally-regenerated loblolly pine, and shortleaf pine) under exceptional drought conditions and pre-drought (i.e., non-exceptional drought) conditions, (2) determine how stand structure (i.e., tree size, stem density, and species composition) affected individual tree mortality in the same pine species groups under exceptional drought conditions and pre-drought conditions, and (3) provide targeted management suggestions based on predicted mortality trends for mitigating exceptional drought mortality in southern pine. We address these objectives at the individual tree scale using extensive re-measurements of pine throughout the region.
For objective 1, we hypothesized that pre-drought group mortality rates would be lowest in planted loblolly pine, given the extensive competition control and management actions in this group, and highest in the shortleaf pine group, given past evidence from Dipesh et al. (2015) under non-drought conditions. Regarding objective 2, we hypothesized for each group that, under pre-drought conditions, smaller trees would have higher mortality given their limited rooting depth and access to deeper water. Larger trees would have higher mortality potentially due to greater hydraulic stress, increased crown exposure, and preference by bark beetles (Bennett et al., 2015). Furthermore, under pre-drought conditions, the highest stem densities would show higher mortality following expected patterns of competition and the lowest stem densities would show higher mortality due to possible increased individual tree risk from maintenance of greater leaf area and root systems (Clark et al., 2016). Finally, pure species mixtures (i.e., plantations) would show higher mortality possibly through increased intra-specific competition (Klos et al., 2009). We generally expected that mortality increased from pre-drought to drought period for each group and each stand structure factor. However, given the dearth of knowledge regarding southern pine mortality to exceptional drought conditions, as experienced in Texas in 2011, it was difficult to speculate on the magnitude of the mortality response and whether any particular groups or aspects of stand structure fared better or worse than others.
Materials and Methods
Study Area
This study was located in eastern Texas (29° 17′ to 33° 57′ N and 93° 30′ to 96° 27′ W; Figure 1), comprising the western extent of West Gulf Coastal Plain forests. Forests in this region are composed of a diverse species mix yet are heavily dominated by pine species, namely loblolly pine (Pinus taeda L.) followed by shortleaf pine (Pinus echinata Mill.). Hardwood species tend to comprise much of the mid- and under-story and include a diverse mix of oaks (e.g., Quercus stellata Wangenh., Quercus nigra L., etc.), sweetgum (Liquidambar styraciflua L.), and elm species (e.g., Ulmus alata Michx.). Climate is generally humid sub-tropical with hot, humid summers and mild, wet winters. During the study period (2003–2016), mean annual precipitation and temperature ranged between 769–1737 mm and 18.1–20.1°C, respectively (twentieth century averages of 1,162 mm and 18.6°C, respectively) with the lowest precipitation (769 mm) and second highest temperature (19.9°C) during this period occurring in 2011 [National Oceanic and Atmospheric Administration (NOAA), 2018]. Variation in topography is minimal with flat to rolling elevation changes ranging from sea-level near the coast to nearly 200 m above sea level. Soils are variable, ranging from poorly-drained to well-drained conditions predominantly comprised of loamy to clayey Alfisols and Ultisols [US Department of Agriculture Natural Resources Conservation Service (USDANRCS), 2006].
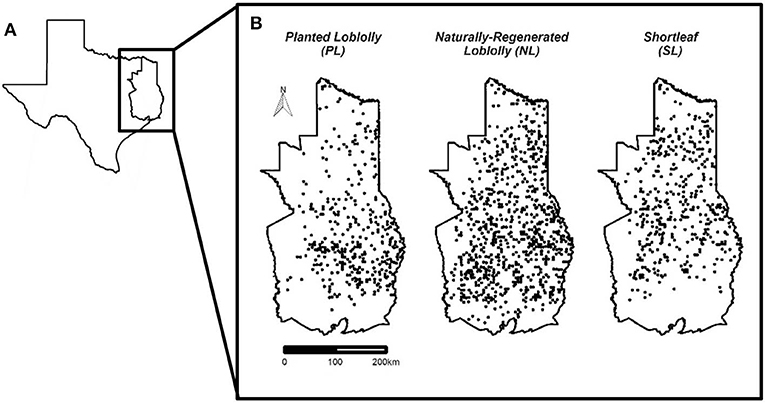
Figure 1. State of Texas map with (A) study area of east Texas as inset and (B) Forest Inventory and Analysis plot locations used for each pine group.
Dataset
Data were taken from the U.S. Forest Service Forest Inventory and Analysis (FIA) program for the region of east Texas. The full dataset consisted of 1,640 forested plots (>10% tree cover) measured across the range of ownerships and conditions existing within east Texas. A plot consists of four subplots each covering 168.1 m2 (~672.5 m2 total plot area) with one central subplot and the three remaining subplots oriented ~36.6 m distance (central subplot-center to outer subplot-center) at 0, 120, and 240 degrees. Trees were classified as stems ≥2.54 cm diameter at breast height (DBH; 1.37 m stem height). Trees with DBH ≥ 2.54 cm and <12.7 cm were measured on four microplots (13.5 m2 each, ~54.0 m2 total) located within subplots while trees with DBH > 12.7 cm were measured on each full subplot. Species, DBH, and status (live or dead) were all recorded at each plot measurement and used in this study.
We categorized trees within the dataset as planted loblolly pine (PL), naturally-regenerated loblolly pine (NL), and all shortleaf pine (SL). Planted loblolly was identified by selecting plots originating from planted seedlings of loblolly pine and NL was identified by selecting plots of non-planted origin. The FIA dataset does not include information on genetic source of planted seedlings given challenges with spatial and temporal scales of sampling and the variety of ownerships across the landscape. However, these groups (i.e., PL and NL) provide a regional, fundamental comparison between trees of differing origins and management paradigms. There existed a few instances where a plot straddled both PL and NL conditions. We excluded these plots from our dataset to avoid the confounding effects of active or non-management at the interface of PL and NL conditions. Given the rare occurrence of shortleaf pine plantations coupled with their relatively low numbers in the dataset, shortleaf pine was categorized as one group (i.e., comprising both naturally-regenerated and any planted stems). All harvested/salvaged trees were excluded from the dataset to avoid confounding effects of silvicultural activity on mortality.
We classified trees into two measurement periods, pre-drought and drought. The pre-drought period consisted of trees initially measured as being alive and subsequently re-measured as alive or dead prior to 2011 (i.e., 2003–2010). Thus, the mortality response of pre-drought trees was not affected by the exceptional conditions of the 2011 drought. Drought period trees were initially measured as alive prior to 2011 and subsequently re-measured as alive or dead after 2011 (i.e., re-measurements between 2012 and 2016). Thus, the mortality response of drought period trees reflect exposure to the 2011 drought conditions assuming any individual tree did not die after initial measurement and prior to the onset of the drought. All plots were re-measured over approximately a 5-year period and differences in plot re-measurement intervals were addressed in our modeling approach described below. Preliminary analyses for spatial autocorrelation of plot-level mortality via Mantel tests confirmed that no significant (α = 0.05) spatial autocorrelation existed among individual or all pine groups for both pre-drought and drought period plots.
We selected and calculated the following stand structural variables prior to any analysis: DBH to describe tree size, plot relative density (RD; calculated via DBH and wood specific gravity after Ducey and Knapp (2010), see their Equation 16) to describe competition among all trees within each plot, and plot species group dominance (SPD; basal area of a focal species group in a plot divided by total basal area of the plot) to describe the contribution of each pine group to the relative species diversity of plots. Variable selections were chosen based on their importance in describing individual tree size/age and local inter- and intra-specific competitive interactions. Moreover, the variables included in our analyses represent common metrics used by managers for manipulating forest conditions to achieve desired management objectives, offering operational relevance for potentially improving pine resistance to future exceptional droughts. All data were summarized and presented in Table 1.
Analysis
We analyzed the data for each objective using Bayesian, logistic, mixed-effects regression models. In all cases, the response variable was binary tree status at re-measurement (live = 1, dead = 0) modeled as a Bernoulli-distributed variable constrained by a probability of survival.
Where, yij is the response for tree i in plot j and pSij is the probability of survival for tree i in plot j. To account for variability in plot re-measurement time intervals, we used an approach first presented by Hamilton and Edwards (1976) and incorporated a random effect component.
Where, pSij is the same as described in Equation (1), is the transposed matrix of covariates for tree i in plot j, βk is the vector of length k of parameters to be estimated, uj is the random effect of plot j, and Lj is the re-measurement interval for plot j. Using this approach, the estimated βk's describe the annual log odds of survival for each tree as opposed to the log odds of survival for the specific re-measurement interval Lj. We included the random effect in each model to account for plot-level variability from site differences. Random effects were modeled as a normally-distributed variable with mean of zero and common variance.
Where, μj is the mean effect in log odds for plot j and σ2 is the variance of the distribution of plot mean effects. For objective 1, a single model was constructed and explanatory variables included the three pine groups (PG: PL, NL, SL), two measurement periods (MP: pre-drought, drought), and their interaction.
Where, X is the matrix of covariates from Equation (2). For objective 2, separate models were constructed for each pine group and measurement period (six total). Explanatory variables for each model included the three stand structural variables of DBH, RD, and SPD. All the explanatory variables were modeled as having a quadratic effect on predicted survival response to account for higher hypothesized mortality in the smallest and largest stems, lowest and highest density plots, etc.
Where, X is the matrix of covariates from Equation (2). Variables for objective 2 were mean-centered and standardized to allow for more meaningful comparison of the effect sizes of each variable on predicted mortality within each model. For the presentation of results, we converted survival probabilities to mortality probabilities via pMij = 1 − pSij.
All models were fit using Hamiltonian Monte Carlo simulations implemented in the RStan package (Stan Development Team, 2017) accessed via R software (R Core Team, 2016). Vague priors were chosen for the estimated parameters. Specifically, priors for βk followed a normal distribution of mean zero and standard deviation of 104 and μj followed a uniform distribution with mean zero and range 104. Use of vague priors meant that results of these analyses should be close to estimations from a maximum likelihood analysis. Chains were run for 100 k iterations with a 50 k warm-up and were thinned by 1/20 to reduce autocorrelation. Chain convergence to the posterior distribution was assessed visually using traceplots and by the R-hat statistic (Gelman and Rubin, 1992). To evaluate the performance of our models, we used a mixed posterior predictive assessment developed by Green et al. (2009) as employed by Masuda and Stone (2015). Broadly, posterior predictive model checking involves simulating replicated data under its modeled distribution using each MCMC simulated value of the estimated model parameters and comparing these new data with the observed data set (Hobbs and Hooten, 2015). In particular, the mixed posterior predictive assessment provides a more conservative assessment of model performance, being similar to the widely-accepted cross-validation technique, than a full posterior predictive assessment, particularly for hierarchical models (e.g., containing a random effect) (Green et al., 2009). This is accomplished by first drawing a new random effect for each group from its modeled distribution, adding the new mean effect to the estimated linear model component, and using the resulting value to draw a new observation from its modeled distribution. In contrast, the fixed posterior predictive assessment uses the estimated random effect rather than drawing a new one which consistently results in a deceptively better fit between observed data and replicated data (Green et al., 2009).
We used common management metrics to produce mortality curves from our resulting models and identified particular areas of concern where management actions could be implemented to potentially reduce exceptional drought mortality. Specifically, we produced mortality curves for 35 and 65% relative densities, which represent the range of fully-stocked stands, and for merchantable breast height stem diameters of 15, 25, and 35 cm, representing common pulpwood, small sawtimber, and large sawtimber stem sizes, respectively, in the study area.
Results
Pine Group Mortality
As expected, drought period mortality increased relative to pre-drought mortality for all pine groups increasing 9.8, 20.0, and 26.3% for PL, SL, and NL, respectively. Surprisingly, none of the drought period mortality responses differed significantly from the pre-drought period (Figure 2, Supplementary Table 1). Notably, PL had significantly lower overall group mortality for both periods than NL and SL. Naturally-regenerated loblolly pine had the greatest increase in mean mortality response between periods suggesting it was the most sensitive to the drought conditions of the three pine groups. Shortleaf pine had the highest mean mortality for both periods and the greatest variability in mortality response.
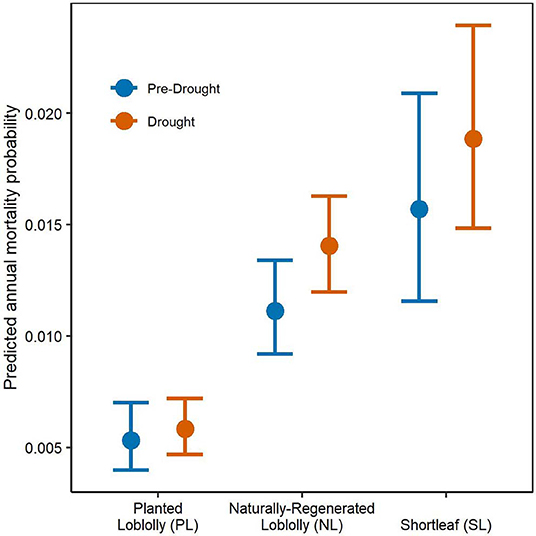
Figure 2. Mortality probabilities for each pine group and measurement period with 95% credible intervals. Pre-drought period trees were measured and re-measured from 2003 to 2010 while drought period trees were initially measured prior to 2011 and re-measured 2012–2016. The R2 for the mixed predictive assessment was 0.09. Prediction accuracy of live and dead trees was 0.999 and 0.132 for observed vs. predicted responses and 0.916 and 0.097 for replicated vs. predicted responses, respectively.
Stand Structure
Stand structure was most important for describing mortality in loblolly pine with differing effects across PL, NL, and measurement periods (Table 2). Interestingly, stand structure did not describe mortality in SL under either period. Diameter at breast height followed the hypothesized “U-shaped” or “J-shaped” pattern, reflecting higher mortality in the smallest and largest stems, when examined in relation to mortality for both PL and NL in both periods (Table 2). Notably, exceptional drought accentuated this mortality effect in the smallest and largest stems of PL but did not for NL. Plot relative density significantly increased mortality in loblolly pine primarily causing greater mortality with increasing density, being most pronounced in PL under drought (Table 2). The effects of relative density on pre-drought NL mortality leveled off at the highest densities but under exceptional drought, increased significantly at higher densities. In contrast to our hypothesis, lower relative densities did not result in elevated loblolly mortality for either period (Table 2). Interestingly, plot species dominance, describing the relative mixture of each pine group to all other species in a plot, significantly affected mortality among drought-period NL which experienced higher mortality with increasing NL dominance while pre-drought PL experienced lower mortality under increasing PL dominance (Table 2).
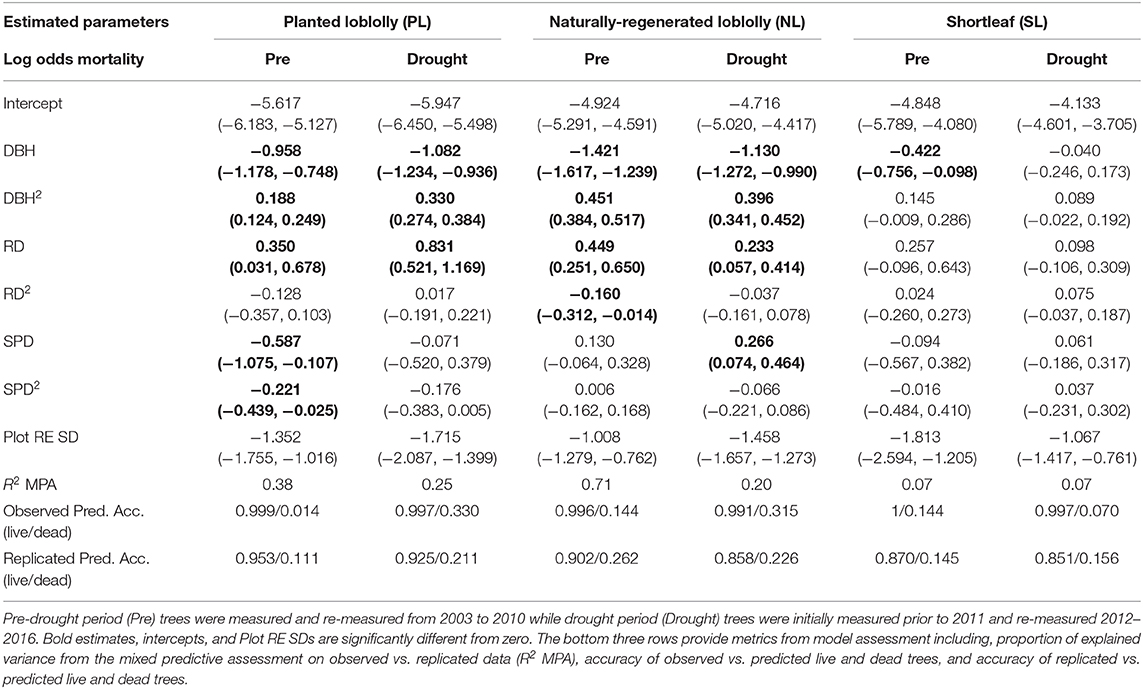
Table 2. Model results for the effects of stand structure on pine group mortality for each measurement period with 95% credible intervals (DBH, diameter at breast height; RD, plot relative density; SPD, plot species dominance; Plot RE SD, estimated standard deviation from the random effect of plots).
Management-Based Mortality Curves
Planted loblolly mortality curves for DBH highlight the increased vulnerability of the smallest and largest stem sizes exposed to the exceptional drought conditions (DBH of <20 cm and > 40 cm; Figures 3A,B). This effect was most pronounced in the largest stems at higher stand densities (65% RD; Figure 3B). Merchantable stems of PL had very low mortality with very low variability regardless of period (Figures 3A,B). Interestingly, smaller stems under pre-drought conditions did not significantly differ in mortality at 35 or 65% RD (Figure 3C). However, smaller stems under exceptional drought had significantly higher mortality at 65% RD than at 35% RD (Figure 3D). For reference, PL mortality curves for RD and SPD can be found in Supplementary Figures 1, 2, respectively but are not discussed here.
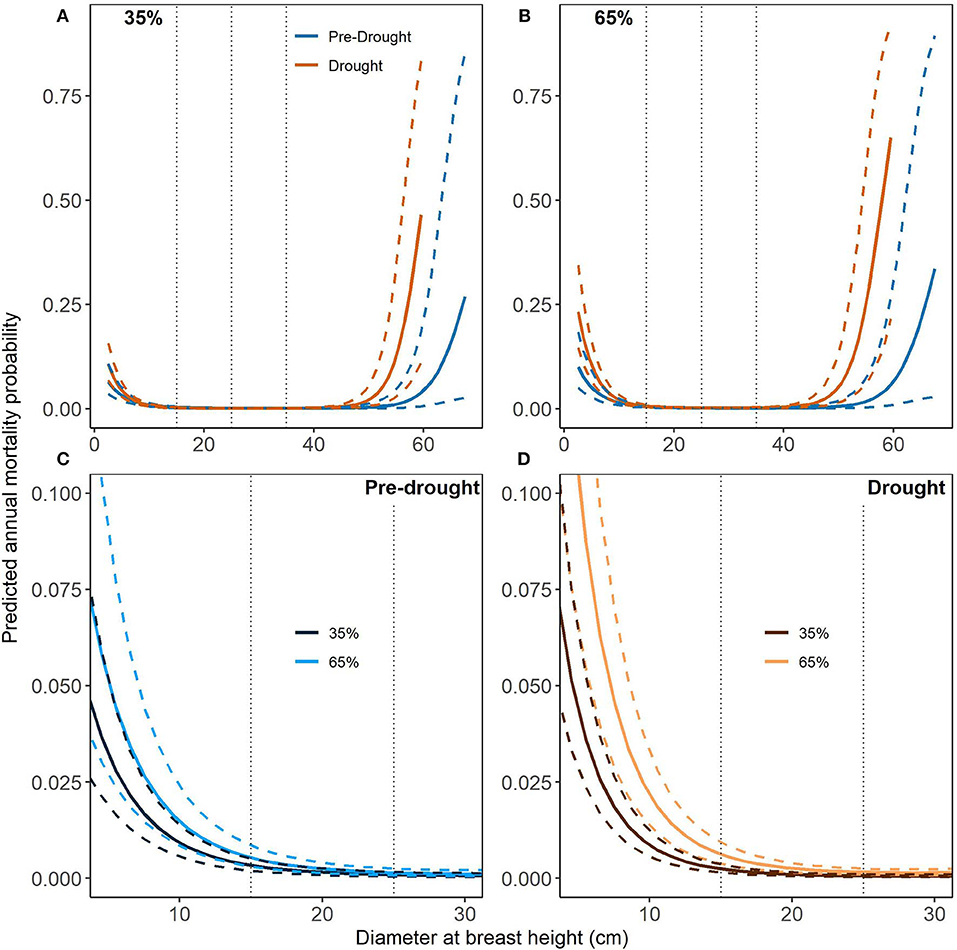
Figure 3. Mortality curves (solid lines) for planted loblolly pine (PL) and diameter at breast height (DBH) with 95% credible intervals (dashed lines). Relative density (RD) is held constant at the lower (35%) and upper (65%) limits of fully-stocked conditions while species dominance (SPD) is held constant at its median values (~90%, see Table 1). Pre-drought period trees were measured and re-measured from 2003 to 2010 while drought period trees were initially measured prior to 2011 and re-measured 2012–2016. Dotted vertical lines highlight merchantable size classes of 15, 25, and 35 cm DBH. (A,B) show mortality curves across the full range of DBH values while (C,D) display notable differences which occur at smaller DBH values.
Naturally-regenerated loblolly pine had higher mortality in the smallest and largest stems however, this effect did not differ between pre-drought and drought periods (Figures 4A,B). As with PL, merchantable stems of NL had very low mortality with very low variability (Figures 4A,B). However, merchantable stem mortality was significantly higher under exceptional drought at 35% RD (Figure 4C) whereas, at 65% RD, mortality did not differ between periods but was higher overall than at 35% RD (Figure 4D). Interestingly, SPD mortality curves for NL indicated that drought period mortality was significantly higher than pre-drought above ~50% NL dominance for all merchantable stem sizes (Figures 5A–C). However, mortality was lower overall and did not differ significantly when NL dominance was below ~50% (Figures 5A–C). For reference, the NL mortality curve for RD can be found in Supplementary Figure 3 but is not discussed here.
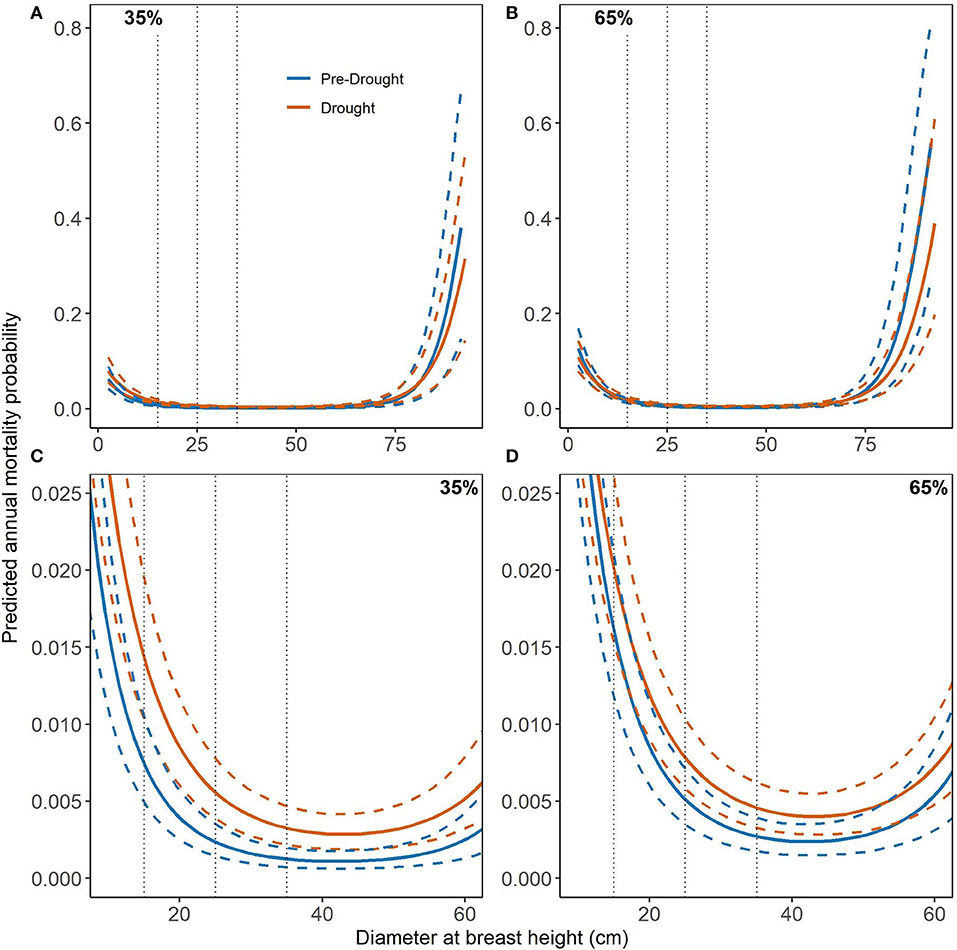
Figure 4. Mortality curves (solid lines) for naturally-regenerated loblolly pine (NL) and diameter at breast height (DBH) with 95% credible intervals (dashed lines). Relative density (RD) is held constant at the lower (35%) and upper (65%) limits of fully-stocked conditions while species dominance (SPD) is held constant at its median values (~60%, see Table 1). Pre-drought period trees were measured and re-measured from 2003 to 2010 while drought period trees were initially measured prior to 2011 and re-measured 2012–2016. Dotted lines highlight merchantable size classes of 15, 25, and 35 cm DBH. (A,B) show mortality curves across the full range of DBH values in the dataset while (C,D) display notable differences which occur at small and mid-range DBH values.
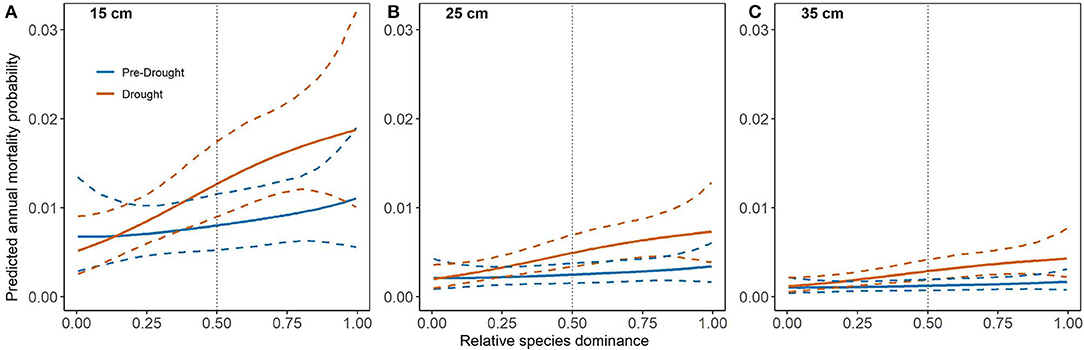
Figure 5. Mortality curves (solid lines) for naturally-regenerated loblolly pine (NL) and species dominance (SPD) with 95% credible intervals (dashed lines). Diameter at breast height (DBH) is held constant at merchantable size classes, (A) 15, (B) 25, and (C) 35 cm, while relative density (RD) is held constant at the lower limit of fully-stocked conditions (35%). Pre-drought period trees were measured and re-measured from 2003 to 2010 while drought period trees were initially measured prior to 2011 and re-measured 2012–2016. Dotted vertical lines highlight 50% dominance by naturally-regenerated loblolly pine (NL).
Model Assessment
The mixed predictive assessment for the model based on Equation (4) (i.e., pine groups and measurement periods) suggested that live trees were predicted accurately and mortality responses were not predicted as well (Table 2). This is likely attributable to the limited number of dead trees in the dataset for PL as it had low mortality during both periods and may also be attributable to the similarity in mortality estimates for NL and SL making differentiation between groups difficult. The mixed predictive assessment for the models based on Equation (5) (i.e., stand structure) shows that the PL and NL models performed fairly well while the SL models performed poorly (Table 2). In all cases, live trees were predicted well while dead trees were predicted fairly (NL, PL) to poorly (SL). Since essentially none of the stand structural variables for SL significantly differed from zero and most were different from zero for PL and NL, it is not surprising that the SL models performed poorly and the PL and NL models provided better explanatory power for describing pre-drought and drought period mortality.
Discussion
Pine Group Mortality
Climate projections indicate future increases in the extent and severity of droughts with possible substantial and widespread increases in tree mortality (Allen et al., 2015). Given the potential economic and ecological ramifications to the timber industry and forest ecosystem function, adaptive management strategies for coping with future exceptional droughts in forests need to be developed yet are critically lacking in the scientific literature (Kemp et al., 2015; Nagel et al., 2017). In this study, we provide an assessment of drought mortality in east Texas by examining the dominant species, loblolly pine, under its two primary silvicultural strategies, planted and naturally-regenerated, which broadly represent managed plantations and unmanaged/minimally-managed stands, respectively. These two conditions occur extensively throughout east Texas across a variety of ownerships (Edgar and Zehnder, 2017) and generally reflect conditions throughout the southeastern U.S., the most productive forested region in the country (Oswalt et al., 2014). Moreover, we examined shortleaf pine, currently a common species throughout the West Gulf Coastal Plain, which is targeted for restoration throughout its extensive historic range. Shortleaf pine is often perceived as drought-tolerant, given its wide historical range which included occurring on xeric sites (Mattoon, 1915), yet has received little attention in the literature regarding its drought mortality response. Here we show these predominant pine species groups are resistant to mortality from the historic exceptional drought conditions experienced in 2011 throughout east Texas.
Notably, we found that intensively managed stands (i.e., PL) appear to be most resistant to drought mortality. This finding was especially notable given that this region represents the westernmost extent of loblolly pine. Planted loblolly mortality was lowest of the three pine groups for both pre-drought and drought conditions, providing support for our hypothesis that pre-drought PL had the lowest mortality of the pine groups examined. Interestingly, exceptional drought exposure did not result in disproportionate mortality vulnerability in PL as has been hypothesized (Domec et al., 2015). Klos et al. (2009) observed higher drought sensitivity (growth and mortality) in pine species of Alabama, Georgia, and Virginia; however, they did not separate out PL from NL. Also, pines in the Klos et al. (2009) study occur in the central reaches of their geographical distribution, whereas east Texas represents the western range margin of loblolly pine. As mentioned in the methods, we were unable to explicitly account for PL seedling source. Yet in general, it is possible that loblolly pine genotypes in Texas are better adapted to drier, more variable climate than those genotypes found further east (Eckert et al., 2010; McNulty et al., 2014; Rehm et al., 2015). Recent examination of PL growth in the West Gulf Coastal Plain suggests that, even under water-limited conditions (albeit not as extreme as the 2011 drought), trees remained productive particularly when given fertilizer inputs (Maggard et al., 2017), suggesting positive response of water-stressed PL under management. However, that study addressed growth and not mortality response of PL to increased water stress. Critically, it appears the broad management actions associated with PL likely allowed these stands to resist mortality from the harsh, hot and dry conditions of the exceptional 2011 drought.
Of the groups examined, NL appeared the most vulnerable to exceptional drought having the highest increase in mean mortality response (26.3% increase). However, NL was still quite resistant given the lack of a significant increase in mortality between periods. A challenge with examining this group as a whole across the region of east Texas is disentangling the multiple factors driving this increased drought mortality response. Some NL stands remain unmanaged until harvest, however, many stands of NL have active competition control to improve productivity (Nelson and Bragg, 2016) providing an advantage when exposed to water stress. The existence of some management activity in a portion of NL stands may have muted the drought mortality response of unmanaged stands. Regardless, our data highlight that NL stands, as a whole, typically have higher densities across east Texas than PL (Table 1) suggesting that density-dependent competition may be the key factor driving the higher exceptional drought mortality response in this group.
Shortleaf pine maintained the highest mean group mortality rates under both pre-drought and drought periods. This result provides some support for the hypothesis that this species experienced the highest pre-drought mortality of the pine groups examined. This agrees with a recent study conducted in forests of southeastern Oklahoma, which reported higher mortality in SL compared to PL (Dipesh et al., 2015). The high variability in mortality estimates for SL can be attributed to the relatively small sample size in our dataset. Ultimately, SL is a relatively minor component of east Texas forests (~3% of all species measured by FIA) possibly occurring on sites less suitable for loblolly production. Thus, these higher mean group mortality rates may be more reflective of inherent site conditions than any particular physiological adaptations suited for drought.
Stand Structure
Despite the exceptional conditions of the 2011 drought, loblolly pine still followed hypothesized trends of mortality with tree size. Specifically, both PL and NL had low mortality in moderately-sized (e.g., merchantable) stems and higher mortality in the smallest and largest stems. Higher mortality in smaller stems is most likely driven by inter- and intra-specific competitive effects before reaching maturity. Increased mortality in larger stems could be driven by multiple effects including senescence, preference by pests (Pfeifer et al., 2011), windthrow (Harcombe et al., 2009), and increased susceptibility to hydraulic failure (Zhang et al., 2009). Interestingly, the exceptional drought conditions significantly elevated mortality in the smallest and largest PL stems compared to pre-drought conditions yet, did not have the same effect in NL. D'Amato et al. (2013) found that pine plantations thinned at a young age and maintained at a low density exhibited lower growth resistance and resilience to drought at later ages likely due to difficulty maintaining high leaf area-to-sapwood ratios developed over time in the low-density conditions. It is possible this effect is occurring in intensively managed loblolly pine plantations in east Texas which were thinned and maintained at low densities and slated for harvest beyond a typical rotation age (e.g., >25 years) but were later abandoned.
Density-dependent mortality in PL and NL still followed expected linear trends of increasing mortality with increasing density despite the historic drought conditions. A growing body of literature has found density-dependent mortality occurs in prevalent North American pine species under increasingly water-limited conditions across temperature and precipitation gradients (D'Amato et al., 2013; Bottero et al., 2017; Gleason et al., 2017). Resources inherently become limited as the number of trees occupying the potential growing space in a stand increases and this appeared to be exacerbated under exceptional drought conditions for PL. Interestingly, exceptional drought caused elevated mortality in lower densities of NL compared to the pre-drought period. Naturally-regenerated loblolly trees growing at low densities over time may have greater canopy area and root architecture than denser stands, given the increased growing space and access to resources (D'Amato et al., 2013), and may be more prone to hydraulic failure possibly causing the elevated drought mortality in low density NL stands compared to pre-drought.
Under exceptional drought, species dominance (i.e., relative species mixtures in a plot) became a significant factor in describing NL mortality. Interestingly, intra-specific competition significantly increased mortality in NL-dominated stands under exceptional drought compared to pre-drought. This suggests that more overstory diversity in NL stands (i.e., lower NL basal area) allows for resource partitioning or facilitative effects between mature NL and other species, an effect also noted by Klos et al. (2009). Species dominance affected PL differently, being important pre-drought but having no significant effect under exceptional drought. Planted loblolly pine predominantly occurs in monocultures and ~85% of all PL plots in this study had >50% of basal area as PL. In fact, mortality decreased as PL dominance increased under pre-drought conditions. This likely reflects that, as PL dominance reaches 100%, these plots occur in the most intensively managed plantations on the landscape where competition control was most prevalent. Thus, pure stands of PL may be more buffered against mortality if they are primarily occurring in actively managed plantations.
Management Implications
The exceptional 2011 drought that occurred in Texas was the worst ever recorded for the state and represents the type of event that could become commonplace in the next few decades (Klepzig et al., 2014). Given that over half of the land area in east Texas is economically productive timberland (Edgar and Zehnder, 2017), it is imperative to develop adaptive management strategies for coping with the stressful conditions of exceptional drought. A critical finding of this study was that, broadly, under current management practices PL stands were more resistant to mortality from exceptional drought stress than NL stands, a finding that, to our knowledge, has not been shown before. In general, PL stands were maintained at lower densities (i.e., within the range of fully-stocked conditions) and at smaller stem sizes compared to NL. These typical management-related effects may have provided the important buffer needed to keep exceptional drought mortality low in PL. Management suggestions for reducing exceptional drought mortality in NL stands are comparable to those for PL with relative density of NL stems being a key driver of mortality risk. Maintaining stands in fully-stocked conditions and even understocked conditions could reduce overall mortality, particularly from exceptional drought. Importantly, promoting other species (i.e., maintaining ≤50% NL basal area), where possible, could provide a crucial advantage for reducing exceptional drought mortality in NL stands, particularly at lower densities. Finally, it remains unclear from this study as to what management strategies could benefit SL under exceptional drought. Further research into the response of SL to exceptional drought conditions is warranted as initiatives continue to seek strategies to restore this species throughout the southeastern U.S. Overall, the suggestions presented here are based on broad-scale modeling results from West Gulf Coastal Plain forests. Reduction of mortality risk depends on local conditions and, critically, overarching management objectives. However, these suggestions provide straightforward management strategies that could be implemented relatively easily by resource managers concerned with exceptional drought mortality.
Data Availability Statement
The datasets analyzed for this study can be found in the U.S. Forest Service Forest Inventory and Analysis program FIA Datamart (https://apps.fs.usda.gov/fia/datamart/datamart.html).
Author Contributions
PK, CE, GM, and JV conceived the ideas. PK designed the methodology, analyzed the data, and led the writing of the manuscript. JV, GM, and CE revised the manuscript for important intellectual content. All authors contributed critically to the drafts and gave final approval for publication.
Funding
Funding for this study was provided in part by a Texas A&M University Merit Fellowship and the National Aeronautics and Space Administration Rapid Response and Novel Research in Earth Science program (Grant number NNX14AN99G). The contents of this study are solely the responsibility and creation of the authors and do not necessarily represent the official views of the National Aeronautics and Space Administration.
Conflict of Interest
The authors declare that the research was conducted in the absence of any commercial or financial relationships that could be construed as a potential conflict of interest.
Acknowledgments
We thank the U.S. Forest Service FIA program and the Texas A&M Forest Service for collecting, organizing, and making available data used in this study. We also thank Dr. Denis Valle for early assistance with the analysis and Dr. Michelle Masuda for providing valuable insight into the mixed posterior predictive model assessment.
Supplementary Material
The Supplementary Material for this article can be found online at: https://www.frontiersin.org/articles/10.3389/ffgc.2020.00023/full#supplementary-material
References
Allen, C. D., Breshears, D. D., and McDowell, N. G. (2015). On underestimation of global vulnerability to tree mortality and forest die-off from hotter drought in the Anthropocene. Ecosphere 6, 1–55. doi: 10.1890/ES15-00203.1
Anderegg, W. R. L., Kane, J. M., and Anderegg, L. D. L. (2013). Consequences of widespread tree mortality triggered by drought and temperature stress. Nat. Clim. Chang. 3, 30–36. doi: 10.1038/nclimate1635
Bennett, A. C., McDowell, N. G., Allen, C. D., and Anderson-Teixeira, K. J. (2015). Larger trees suffer most during drought in forests worldwide. Nat. Plants 1:15139. doi: 10.1038/nplants.2015.139
Bottero, A., D'Amato, A. W., Palik, B. J., Bradford, J. B., Fraver, S., Battaglia, M. A., et al. (2017). Density-dependent vulnerability of forest ecosystems to drought. J. Appl. Ecol. 54, 1605–1614. doi: 10.1111/1365-2664.12847
Bracho, R., Vogel, J. G., Will, R. E., Noormets, A., Samuelson, L. J., Jokela, E. J., et al. (2018). Carbon accumulation in loblolly pine plantations is increased by fertilization across a soil moisture availability gradient. For. Ecol. Manage. 424, 39–52. doi: 10.1016/j.foreco.2018.04.029
Cavin, L., Mountford, E. P., Peterken, G. F., and Jump, A. S. (2013). Extreme drought alters competitive dominance within and between tree species in a mixed forest stand. Funct. Ecol. 27, 1424–1435. doi: 10.1111/1365-2435.12126
Chen, G., Pan, S., Hayes, D. J., and Tian, H. (2017). Spatial and temporal patterns of plantation forests in the United States since the 1930s: an annual and gridded data set for regional Earth system modeling. Earth Syst.Sci. Data 9, 545–556. doi: 10.5194/essd-9-545-2017
Clark, J. S., Iverson, L., Woodall, C. W., Allen, C. D., Bell, D. M., Bragg, D. C., et al. (2016). The impacts of increasing drought on forest dynamics, structure, and biodiversity in the United States. Glob. Change Biol. 22, 1–24. doi: 10.1111/gcb.13160
D'Amato, A. W., Bradford, J. B., Fraver, S., and Palik, B. J. (2013). Effects of thinning on drought vulnerability and climate response in north temperate forest ecosystems. Ecol. Appl. 23, 1735–1742. doi: 10.1890/13-0677.1
Dietze, M. C., and Moorcroft, P. R. (2011). Tree mortality in the eastern and central United States: patterns and drivers. Glob. Chang. Biol. 17, 3312–3326. doi: 10.1111/j.1365-2486.2011.02477.x
Dipesh, K., Will, R. E., Lynch, T. B., Heinemann, R., and Holeman, R. (2015). Comparison of loblolly, shortleaf, and pitch X loblolly pine plantations growing in Oklahoma. For. Sci. 61, 540–547. doi: 10.5849/forsci.14-004
Domec, J.-C., King, J. S., Ward, E., Oishi, A. C., Palmroth, S., Radecki, A., et al. (2015). Conversion of natural forests to managed forest plantations decreases tree resistance to prolonged droughts. For. Ecol. Manage. 355, 58–71. doi: 10.1016/j.foreco.2015.04.012
Ducey, M. J., and Knapp, R. A. (2010). A stand density index for complex mixed species forests in the northeastern United States. For. Ecol. Manage. 260, 1613–1622. doi: 10.1016/j.foreco.2010.08.014
Eckert, A. J., van Heerwaarden, J., Wegrzyn, J. L., Nelson, C. D., Ross-Ibarra, J., Gonzalez-Martinez, S. C., et al. (2010). Patterns of population structure and environmental associations to aridity across the range of loblolly pine (Pinus taeda L., Pinaceae). Genetics 185, 969–982. doi: 10.1534/genetics.110.115543
Edgar, C. B., and Zehnder, R. (2017). “East Texas Forestlands, 2015,” in Forest Resource Reports (College Station, TX: Texas A&M Forest Service), 6.
Floyd, M. L., Clifford, M., Cobb, N. S., Hanna, D., Delph, R., Ford, P., et al. (2009). Relationship of stand characteristics to drought-induced mortality in three Southwestern piñon - Juniper woodlands. Ecol. Appl. 19, 1223–1230. doi: 10.1890/08-1265.1
Forrester, D. I. (2014). The spatial and temporal dynamics of species interactions in mixed-species forests: from pattern to process. For. Ecol. Manage. 312, 282–292. doi: 10.1016/j.foreco.2013.10.003
Fox, T. R., Jokela, E. J., and Allen, H. L. (2007). The development of pine plantation silviculture in the southern United States. J. For. 105, 337–347. doi: 10.1093/jof/105.7.337
Ganey, J. L., and Vojta, S. C. (2011). Tree mortality in drought-stressed mixed-conifer and ponderosa pine forests, Arizona, USA. For. Ecol. Manage. 261, 162–168. doi: 10.1016/j.foreco.2010.09.048
Gelman, A., and Rubin, D. B. (1992). Inference from iterative simulation using multiple sequences. Stat. Sci. 7, 457–472. doi: 10.1214/ss/1177011136
Gleason, K. E., Bradford, J. B., Bottero, A., D'Amato, A. W., Fraver, S., Palik, B. J., et al. (2017). Competition amplifies drought stress in forests across broad climatic and compositional gradients. Ecosphere 8, 1–16. doi: 10.1002/ecs2.1849
Green, M. J., Medley, G. F., and Browne, W. J. (2009). Use of posterior predictive assessments to evaluate model fit in multilevel logistic regression. Vet. Res. 40:10. doi: 10.1051/vetres/2009013
Hamilton, D. A. Jr., and Edwards, B. M. (1976). “Modeling the probability of individual tree mortality,” in USDA Forest Service Intermountain Research Station Research Paper INT-185 (Ogden, UT), 22. doi: 10.5962/bhl.title.68792
Harcombe, P. A., Leipzig, L. E. M., and Elsik, I. S. (2009). Effects of Hurricane Rita on three long-term forest study plots in east Texas, USA. Wetlands 29, 88–100. doi: 10.1672/08-64.1
Hobbs, N. T., and Hooten, M. B. (2015). Bayesian Models: A Statistical Primer for Ecologists. Princeton, NJ: Princeton University Press.
Intergovernmental Panel on Climate Change (IPCC) (2013). Change 2013: The Physical Science Basis. Cambridge, New York, NY: Contribution of Working Group I to the Fifth Assessment Report of the Intergovernmental Panel on Climate Change.
Kemp, K. B., Blades, J. J., Klos, P. Z., and Hall, T. E. (2015). Managing for climate change on federal lands of the western United States: perceived usefulness of climate science, effectiveness of adaptation strategies, and barriers to implementation. Ecol. Soc. 20:14. doi: 10.5751/ES-07522-200217
Klepzig, K., Shelfer, R., and Choice, Z. (2014). Outlook for Coastal Plain Forests: A Subregional Report From the Southern Forest Futures Project. Genral Technical Report SRS-196. U.S. Department of Agriculture Forest Service, Southern Research Station, Asheville, NC.
Klockow, P. A., Vogel, J. G., Edgar, C. B., and Moore, G. W. (2018). Lagged mortality among tree species four years after an exceptional drought in east Texas. Ecosphere 9:e02455. doi: 10.1002/ecs2.2455
Klos, R. J., Wang, G. G., Bauerle, W. L., and Rieck, J. R. (2009). Drought impact on forest growth and mortality in the southeast USA: an analysis using forest health and monitoring data. Ecol. Appl. 19, 699–708. doi: 10.1890/08-0330.1
Kramer, I., and Holscher, D. (2010). Soil water dynamics along a tree diversity gradient in a deciduous forest in Central Germany. Ecohydrology 3, 262–271. doi: 10.1002/eco.103
Lindenmayer, D. B., Laurance, W. F., and Franklin, J. F. (2012). Global decline in large old trees. Science 338, 1305–1306. doi: 10.1126/science.1231070
Lines, E. R., Coomes, D. A., and Purves, D. W. (2010). Influences of forest structure, climate and species composition on tree mortality across the eastern US. PLoS ONE 5:e13212. doi: 10.1371/journal.pone.0013212
Maggard, A. O., Will, R. E., Wilson, D. S., Meek, C. R., and Vogel, J. G. (2017). Fertilization can compensate for decreased water availability by increasing the efficiency of stem volume production per unit of leaf area for loblolly pine (Pinus taeda) stands. Can. J. For. Res. 47, 445–457. doi: 10.1139/cjfr-2016-0422
Masuda, M. M., and Stone, R. P. (2015). Bayesian logistic mixed-effects modelling of transect data: relating red tree coral presence to habitat characteristics. ICES J. Mar. Sci. 72, 2674–2683. doi: 10.1093/icesjms/fsv163
Mattoon, W. R. (1915). Life History of Shortleaf Pine. Washington, DC: US Department of Agriculture.
McNulty, S. G., Boggs, J. L., and Sun, G. (2014). The rise of the mediocre forest: why chronically stressed trees may better survive extreme episodic climate variability. New For. 45, 403–415. doi: 10.1007/s11056-014-9410-3
Moore, G. W., Edgar, C. B., Vogel, J. G., Washington-Allen, R. A., March, R. G., and Zehnder, R. (2016). Tree mortality from an exceptional drought spanning mesic to semiarid ecoregions. Ecol. Appl. 26, 602–611. doi: 10.1890/15-0330
Nagel, L. M., Palik, B. J., Battaglia, M. A., D'Amato, A. W., Guldin, J. M., Swanston, C. W., et al. (2017). Adaptive silviculture for climate change: a national experiment in manager-scientist partnerships to apply an adaptation framework. J. For. 115, 167–178. doi: 10.5849/jof.16-039
National Oceanic and Atmospheric Administration (NOAA) (2018). National Centers for Environmental Information, Climate at a Glance: Divisional Time Series. Available online at: https://www.ncdc.noaa.gov/cag/ (accessed July 26, 2018).
Nelson, A. S., and Bragg, D. C. (2016). Multidecadal response of naturally regenerated southern pine to early competition control and commercial thinning. For. Sci. 62, 115–124. doi: 10.5849/forsci.15-056
Oswalt, S. N., Smith, W. B., Miles, P. D., and Pugh, S. A. (2014). Forest Resources of the United States, 2012: A Technical Document Supporting the Forest Service 2010 Update of the RPA Assessment. Washington, DC: Gen. Tech. Rep. WO-91. U.S. Department of Agriculture, Forest Service. doi: 10.2737/WO-GTR-91
Peng, C., Ma, Z., Lei, X., Zhu, Q., Chen, H., Wang, W., et al. (2011). A drought-induced pervasive increase in tree mortality across Canada's boreal forests. Nat. Climate Change 1, 467–471. doi: 10.1038/nclimate1293
Pfeifer, E. M., Hicke, J. A., and Meddens, A. J. H. (2011). Observations and modeling of aboveground tree carbon stocks and fluxes following a bark beetle outbreak in the western United States. Global Change Biol. 17, 339–350. doi: 10.1111/j.1365-2486.2010.02226.x
Pretzsch, H., Schutze, G., and Uhl, E. (2013). Resistance of European tree species to drought stress in mixed versus pure forests: evidence of stress release by inter-specific facilitation. Plant Biol. 15, 483–495. doi: 10.1111/j.1438-8677.2012.00670.x
Puettmann, K. J. (2011). Silvicultural challenges and options in the context of global change: “simple” fixes and opportunities for new management approaches. J. For. 109, 321–331. doi: 10.1093/jof/109.6.321
R Core Team (2016). R: A Langauge and Environment for Statistical Computing. Vienna: R Foundation for Statistical Computing. Available online at: https://www.R-project.org/
Rehm, E. M., Olivas, P., Stroud, J., and Feeley, K. J. (2015). Losing your edge: climate change and the conservation value of range-edge populations. Ecol. Evol. 5, 4315–4326. doi: 10.1002/ece3.1645
Stan Development Team (2017). RStan: The R Interface to Stan. R package version 2.16.2. Available online at: http://mc-stan.org
Thrippleton, T., Bugmann, H., Folini, M., and Snell, R. S. (2018). Overstorey-understorey interactions intensify after drought-induced forest die-off: long-term effects for forest structure and composition. Ecosystems 21, 723–739. doi: 10.1007/s10021-017-0181-5
US Department of Agriculture Natural Resources Conservation Service (USDANRCS) (2006). Land Resource Regions and Major Land Resource Areas of the United States, the Caribbean, and the Pacific Basin. Washington, DC: U.S. Department of Agriculture Handbook 296.
van Mantgem, P. J., Stephenson, N. L., Byrne, J. C., Daniels, L. D., Franklin, J. F., Fulé, P. Z., et al. (2009). Widespread increase of tree mortality rates in the western United States. Science 323, 521–524. doi: 10.1126/science.1165000
Vose, J. M., Clark, J. S., Luce, C. H., and Patel-Weynand, T. (2016). Effects of Drought on Forests and Rangelands in the United States: A Comprehensive Science Synthesis. Genral Technical Report WO-93b. U.S. Department of Agriculture, Forest Service, Washington Office, Washington, DC.
Ward, E. J., Domec, J.-C., Laviner, M. A., Fox, T. R., Sun, G., McNulty, S., et al. (2015). Fertilization intensifies drought stress: water use and stomatal conductance of Pinus taeda in a midrotation fertilization and throughfall reduction experiment. For. Ecol. Manage. 355, 72–82. doi: 10.1016/j.foreco.2015.04.009
Zhang, Y. J., Meinzer, F. C., Hao, G. Y., Scholz, F. G., Bucci, S. J., Takahashi, F. S. C., et al. (2009). Size-dependent mortality in a Neotropical savanna tree: the role of height-related adjustments in hydraulic architecture and carbon allocation. Plant Cell Environ. 32, 1456–1466. doi: 10.1111/j.1365-3040.2009.02012.x
Keywords: Bayesian multi-level model, exceptional drought, Forest Inventory and Analysis (FIA), loblolly pine, natural regeneration, plantation, shortleaf pine, tree mortality
Citation: Klockow PA, Edgar CB, Moore GW and Vogel JG (2020) Southern Pines Are Resistant to Mortality From an Exceptional Drought in East Texas. Front. For. Glob. Change 3:23. doi: 10.3389/ffgc.2020.00023
Received: 31 October 2019; Accepted: 24 February 2020;
Published: 13 March 2020.
Edited by:
Matthew D. Hurteau, University of New Mexico, United StatesReviewed by:
Taehee Hwang, Indiana University Bloomington, United StatesLucy Penn Kerhoulas, Humboldt State University, United States
Copyright © 2020 Klockow, Edgar, Moore and Vogel. This is an open-access article distributed under the terms of the Creative Commons Attribution License (CC BY). The use, distribution or reproduction in other forums is permitted, provided the original author(s) and the copyright owner(s) are credited and that the original publication in this journal is cited, in accordance with accepted academic practice. No use, distribution or reproduction is permitted which does not comply with these terms.
*Correspondence: Paul A. Klockow, cGtsb2Nrb3dAdW1uLmVkdQ==