- 1Department of Zoology, University of Cambridge, Cambridge, United Kingdom
- 2SMART Research Institute, Pekanbaru, Indonesia
- 3School of Biological Sciences, University of Southampton, Southampton, United Kingdom
- 4School of Geography and Environmental Science, University of Southampton, Southampton, United Kingdom
The expansion of oil palm (Elaeis guineensis) plantations is a primary cause of land-use change and biodiversity loss in Southeast Asia. This has led to an increasing demand for the development of more sustainable agricultural management practices in plantations, such as Integrated Pest Management. Although populations of carnivorous mammals show declines when forest is converted to oil palm, some species, such as Leopard Cats (Prionailurus bengalensis) have been found to persist. They are often encouraged by plantation managers for their conservation value, and as agents of pest control to manage rat populations. Despite this, little is known about whether they reduce pest rat numbers, or whether plantation management affects how they use the oil palm habitat. This study was based at the Biodiversity and Ecosystem Function in Tropical Agriculture (BEFTA) Programme in mature oil palm plantations in Riau, Sumatra, where there are three management strategies altering understory vegetation structure. We quantified Leopard Cat activity, invasive rat abundance and rat damage using camera traps, live traps, and visual estimates, respectively. We collected data over a 4-year period, before and after the management strategies were applied. We recorded three species of wild mammals (Leopard Cats, Common Palm Civets, and Wild Pig) within the plantations, of which Leopard Cats made up 82% of the total number of observations. We found that Leopard Cat habitat use was higher with increased understory vegetation, but that there was no effect of the vegetation treatments on rat abundance or rat damage. There was also a trend for reduced rat abundance with increased Leopard Cat activity. These results show that management practices can significantly affect Leopard Cat habitat use, with potential benefits for pest control. They also highlight the value of large-scale long-term manipulative experiments for developing more sustainable management practices in oil palm.
Introduction
Oil palm is one of the five fastest expanding crops in the tropics (Phalan et al., 2013), with plantations covering over 21 million hectares of land (fao.org/faostat 2016). It is a highly productive (fao.org/faostat 2016) and versatile (Khosla, 2011) crop, and makes important contributions to growing global food requirements (Tilman et al., 2011; OECD Publishing, 2018). However, oil palm has replaced substantial areas of high conservation value land, including tropical forests and peat swamps (Wicke et al., 2011; Stibig et al., 2014), resulting in severe losses of biodiversity (Koh and Wilcove, 2008; Foster et al., 2011) and increases in carbon emissions (Reijnders and Huijbregts, 2008; Koh et al., 2011). As awareness of the environmental impacts of palm oil has grown, there has been an increasing demand for sustainably certified palm oil, such that oil palm now has the third highest proportion of certified land of any crop (Tayleur et al., 2017). Therefore, there is huge pressure on the oil palm industry to develop sustainable management practices within plantations, which include steps to reduce pollution and protect areas and species of high conservation value [Roundtable on Sustainable Palm Oil (RSPO), 2018].
An important aspect of sustainable plantation management is the use of Integrated Pest Management (IPM) techniques to control diseases, weeds, invasive introduced species, and pests [Roundtable on Sustainable Palm Oil (RSPO), 2018]. IPM, a term that was coined in the 1970s, can be defined as: “a decision support system for the selection and use of pest control tactics… based on cost/benefit analyses that take into account the interests of and impacts on producers, society, and the environment” (Kogan, 1998). For oil palm, this involves minimizing the use of chemicals and, where possible, using native species to control pests [Roundtable on Sustainable Palm Oil (RSPO), 2018]. The IPM strategy is a change from the early methods in oil palm cultivation, which centered around the use of pesticides, before issues such as pesticide resistance and secondary poisoning of predators were realized (Wood and Fee, 2003). IPM strategies are now used increasingly for the control of all major oil palm pests (Dislich et al., 2017). Biological control agents, primarily used to combat herbivorous insects, Ganoderma fungus and rats, range from parasitoids to carniverous insects, viruses to fungi, and barn owls to snakes (Dislich et al., 2017).
Invasive rats (Rattus tiomanicus and R. rattus diardii) are a major pest in oil palm plantations as they feed on oil palm fruit and flowers (Wood and Fee, 2003). Their abundance, which can reach 600 individuals/ha (Wood and Fee, 2003), is positively correlated with oil palm fruit damage, and reduces yield by an estimated 5% (Wood and Liau, 1984; Puan et al., 2011c). Alongside the use of baited rat traps, the IPM strategy of introducing and encouraging Barn Owls (Tyto alba), which feed almost exclusively on rats in oil palm plantations, has proven valuable for rat control (Wood, 2002; Wood and Fee, 2003; Puan et al., 2011b). Barn Owl population growth was initially limited by a lack of nesting sites, but with the introduction of nesting boxes in the 1970s they became widely established, and are now common in many plantations (Duckett, 1976). However, beyond this, there has been little research into the importance of other vertebrate predators in controlling pest rat populations in oil palm, or into the effects of plantation habitat management on rat or predator populations (Dislich et al., 2017).
Although the majority of carnivorous mammal species found in forest do not survive in the oil palm matrix, and the abundance of the remaining species is often low, a few species are present and can reach considerable densities in plantations (Maddox et al., 2007; Gamage et al., 2011; Yue et al., 2015; Wearn et al., 2016, 2017; Pardo et al., 2018). In Indonesia and Malaysia, Leopard Cats (Prionailurus bengalensis), which are listed under CITES (cites.org/eng/node/24193), and the Common Palm Civet (Paradoxurus hermaphroditus), can be highly abundant in oil palm (Maddox et al., 2007; Rajaratnam et al., 2007; Jennings et al., 2015; Yue et al., 2015; Chua et al., 2016; Wearn et al., 2017). Leopard Cats have adapted to the oil palm environment by becoming more nocturnal (Chua et al., 2016), possibly to avoid the hotter daily temperatures (Luskin and Potts, 2011) or human interference (Gaynor et al., 2018). Both Leopard Cats and Common Palm Civets have been shown to eat rats as a large part of their diet: 90% for Leopard Cats and 13% for Common Palm Civets (Rajaratnam et al., 2007; Nakashima et al., 2013; Chua et al., 2016), suggesting high potential as pest control agents. Local observations of Leopard Cat scat contents indicate that rats make up 75.1% of scat volume in oil palm plantations in Riau (Verwilghen, 2015). Common Palm Civets have also been shown to eat palm oil fruit as 44% of their diet (Nakashima et al., 2013), however, so it is not clear whether their net effect is positive or negative for plantation production.
Given the potential value of carnivorous mammals as pest control agents, as well as the intrinsic value of supporting native species, efforts should be made to manage plantations so as to increase their populations. Leaving patches of forest may be one way to do this, as the greater diversity of species within them can spill over into plantations, though many species are only found near forest edges (Maddox et al., 2007; Gamage et al., 2011; Yue et al., 2015; Mendes-Oliveira et al., 2017; Wearn et al., 2017; Pardo et al., 2018). Another strategy is to create a more favorable habitat within the plantations themselves, by reducing hunting pressure, reducing traffic-related deaths (Maddox et al., 2007; Azhar et al., 2013), and increasing habitat complexity (Pardo et al., 2018). The latter can be achieved by growing palms in polyculture (with either livestock or other plant crops) (Koh et al., 2009; Azhar et al., 2014b; Tohiran et al., 2019), or allowing understory vegetation to develop, through reduced application of herbicide (Ashton-Butt et al., 2018; Spear et al., 2018; Luke et al., 2019).
There is evidence to suggest that changes in plantation management can affect mammal communities, and habitat structure is an important determinant of mammal community composition in plantations (Wearn et al., 2019). Mammal species richness (rats and carnivores) (Pardo et al., 2018) and abundance (anteater, armadillo, and deer) (Pardo et al., 2019) in oil palm plantations in Columbia was positively correlated with the density and presence of understory vegetation, and smallholdings in Malaysia had a higher abundance of carnivorous mammals compared to large-scale plantations, probably due to a greater habitat complexity that could provide an important habitat for some species (Azhar et al., 2014a). For example, Common Palm Civets prefer to nest in oil palm with dense mats of ferns and many leaves (Nakashima et al., 2013). Greater habitat complexity can also provide shelter for carnivorous mammals, potentially hiding them from human activity, which is known to deter them (Azhar et al., 2014a). Conversely, in some cases having higher complexity could reduce effective pest control. For example, Leopard Cats are thought to prefer more open areas for hunting, as this increases catch success (Rajaratnam et al., 2007). Greater habitat complexity can also directly benefit pest species and therefore increase pest damage. Indeed, rats prefer areas with more vegetation as it provides cover (Puan et al., 2011a), and such habitats might have a greater diversity of food such as fruit and insects. Therefore, it is not clear how mammalian predators and pests are affected by different management strategies, nor the ultimate positive or negative effect of management on fruit damage and yield.
In this study, we make use of a large-scale, long-term understory vegetation manipulation experiment to investigate whether changing vegetation management techniques within mature oil palm plantations affects Leopard Cat activity, the abundance of pest rats, and the incidence of rat-damage to fruit bunches.
Methods
Study Sites
This study was conducted as part of the Biodiversity and Ecosystem Function in Tropical Agriculture (BEFTA) Programme [oilpalmbiodiversity.org, Luke et al. (under review)]. The BEFTA Programme is located in Riau Province, Sumatra, Indonesia (NO 55.559, E101 11.619), in oil palm estates owned and managed by PT Ivo Mas Tunggal, a subsidiary company of Golden Agri Resources (GAR), with technical advice from Sinar Mas Agro Resources and Technology Research Institute (SMARTRI, the research and development center of GAR). See Luke et al. (under review) for a site map. The vast majority of land in these estates and the surrounding area is used to cultivate oil palm. The region is managed as a large-scale industrial plantation and there are few other crops in the area. The nearest intact forest is Siak Kecil Forest, which is 60 km away, and the nearest degraded forest is 15 km away along river Mandau. Average monthly rainfall during the period of this experiment is shown in Figure S1, with the dates of data collection overlaid.
The BEFTA Understory Vegetation Project is a long-term ecological experiment within the BEFTA Programme that is investigating the effects of understory vegetation management on biodiversity, ecosystem functioning, and yield in mature oil palm plantations (planted 1987-1993) (Luke et al., under review). Three experimental treatments that varied in their level of herbicide application, and therefore understory vegetation complexity, were implemented in February 2014 (Figure 1). Herbicides used included Glyphosate (Rollup 480 SL), Paraquat Dichloride (Rolixone 276 SL), metsulfuron- methyl (Erkafuron 20 WG), and Fluroxypyr (Starane 290 EC). Experimental plots were replicated six times in triplets, with each experimental treatment represented once in each triplet. The order of the treatments within the triplets was randomized. Plots are located on flat ground, 10–30 m above sea level, and far from human settlements. Each plot is 150 × 150 m in size, with a central 50 × 50 m block from which data were collected. Rodenticides were not used in the estates during this study.
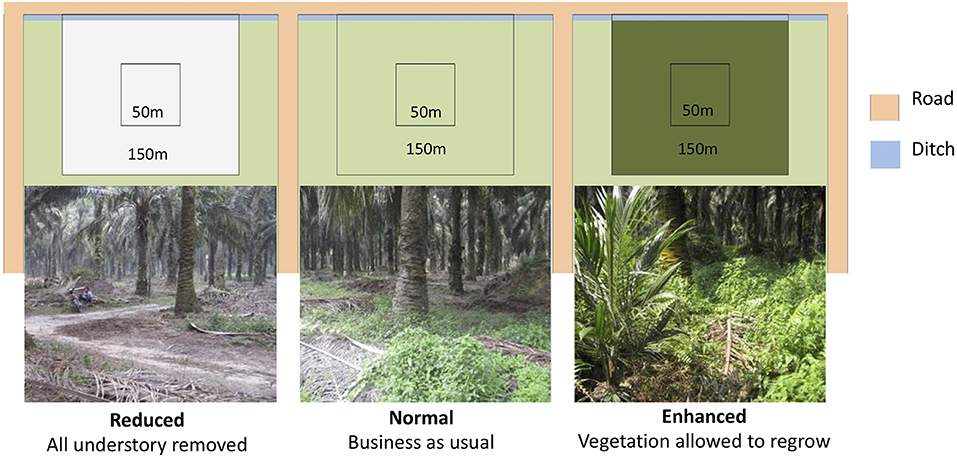
Figure 1. One triplet of plots showing the three experimental vegetation treatments. Photographs of the plantations were taken 2 years after the treatments were implemented. Experimental plots within the triplet are seperated by 150m of plantation and ~5m of road.
The three experimental treatments were as follows:
1. Reduced complexity: All understory vegetation was removed by spraying herbicide.
2. Normal complexity: Understory vegetation was removed from the harvesting paths and harvesting circle (an area around the base of each oil palm tree ~1.5 m in radius) using herbicide, and large woody vegetation was removed from all over the plots manually. All other vegetation was allowed to grow. This is standard industry practice in the region.
3. Enhanced complexity: No herbicide was used. Understory vegetation in the harvesting circle and harvesting paths was cleared manually using a strimmer. Large woody vegetation was removed manually.
Camera Trapping
Leopard Cat activity was recorded using camera traps Reconyx HC600 HyperFire and Bushnell Trophy Cam XLT. We use the term “Leopard Cat activity” instead of “Leopard Cat abundance” as their home range is 1.5–12.4 km2 (Chen et al., 2016), so likely extended beyond the area of the plots, which were 150 × 150 m. This means that we could only calculate differences in habitat use, not population trends. One camera trap was fixed to the base of an oil palm tree near the center of each plot in four of the six replicate triplets. This meant that in total there were twelve camera traps active at any time, with four in each vegetation treatment. Within the triplets, traps were 300 m apart. Between triplets, traps were 2–12 km apart (Luke et al., under review for a site map). Traps were positioned 30 cm above the ground, facing into harvesting paths, where understory vegetation is low. This minimized the bias created by any differences in openness between the three experimental treatments. The traps were active through the day and night and they were triggered by movement. Their field of vision was ~5–10 m. Camera traps were checked at every 1–2 weeks and replaced if they malfunctioned. Pre-treatment camera traps were active for 499 camera trap nights from 10 June to 5 August 2013. Post-treatment camera traps were active from 1 July to 13 August 2015 and 10 April to 2 June 2016 for 498 and 505 camera trap nights, respectively. The total number of camera trap nights active was 1,502, excluding camera trap failures.
All individuals triggering the cameras were identified to species level using local identification guides (Payne and Frances, 2007; MacKinnon and Phillipps, 2014). We considered individuals to be different if they were clearly different in appearance or if photos were taken at least 30 min apart. We also recorded all humans that triggered the traps and considered them as individuals using the same criteria.
Rat Trapping
We used a mark-release-recapture technique to estimate the population size of rats in the plots. Ninety rat traps, baited with oil palm fruit, were randomly placed in the vegetation in the 50 × 50 m central block in all six replicate triplets. Every trap was checked daily for 3 days, and every rat was marked according to the treatment of the plot it was found in, and then released. On the fourth sampling day, the number of marked and unmarked rats was recorded. We used Bailey's (Bailey, 1952) modification of the Lincoln Index to estimate the population size (N), following:
where:
N = population size
R = total number of individuals marked in the first three nights
S = total number of individuals captured on the fourth night
M = number of marked individuals captured on the fourth night
This gave us the population size per 50 × 50 m plot, and we scaled this up to per ha as it is a more intuitive metric. Rat populations were estimated at 6-month intervals from June 2013 to December 2016. Eight time points were measured in total, two of which were pre-treatment and six of which were post-treatment. No rats were killed during the course of this study and all were released after the final survey.
Fruit Damage
During harvesting of fruit, the number of palms in the 50 × 50 m plots with rat damage (Figure S2) was counted, and we calculated the percentage of damaged palms in each plot. Fruit damage was estimated at the same time as rat population estimations, i.e., eight times between June 2013 and December 2016.
Statistical Analysis
We analyzed data in software package R version 3.5.1 (R Core Team, 2018) with R Studio version 1.1.456 (RStudio Team, 2016). We used packages tidyverse (Wickham, 2017) and lattice (function: dotplot) (Sarkar, 2008) for data wrangling and plotting throughout the analysis. We ran a series of generalized linear mixed-effects models (GLMMs) to determine the relationship between vegetation treatment, Leopard Cat activity, rat abundance and rat damage. We used plot (Plot) as a random intercept to account for temporal dependency. When modeling rat abundance, we nested this in the random effect triplet (Triplet) to account for spatial dependency. We did not include it when modeling Leopard Cat activity as in that instance it would have only had four levels, or when modeling rat damage as it was estimated to zero and the model would not converge. We used packages lme4 (function: glmer) (Bates et al., 2015), glmmTMB (function: glmmTMB) (Brooks et al., 2017) and influence.ME (function: influence) (Nieuwenhuis et al., 2012) for constructing and validating models. Data exploration was conducted following Zuur et al. (2010), and model validation following Zuur and Ieno (2016). To verify the models, we plotted model residuals against fitted values, and against all covariates (whether included in the final model or not), and random effects. We simplified models with multiple explanatory variables by selecting those with the lowest Akaike's Information Criterion (AIC). Where Δ < 2 we applied the nesting rule, and discarded models that were more complicated versions of those that had a lower AIC value (Richards et al., 2011). To assess the completeness of sampling for all species recorded, we created a species accumulation curve using the method rarefaction with the R package vegan (Oksanen et al., 2018).
We ran a series of GLMMs to determine whether Leopard Cat activity was affected by the vegetation treatments (Equation 1 in Supplementary Information). We included the covariates vegetation treatment (Vegetation: categorical with three levels) and time (Time1: categorical with two levels). Due to the low sample size, we combined both post-treatment measurements as visual inspection of the data indicated that they had similar values. We used a Poisson distribution with a log link, as the data were counts. We tested for overdispersion by simulating 10,000 datasets and comparing their dispersion statistics to the observed one, and found that a Poisson model fit well. Data exploration revealed that human activity (as recorded by the camera traps) and Time1 were co-linear, such that human activity increased post-treatment in all vegetation treatments. We selected Time1 as the expected predictor, as we thought that the change in vegetation treatment was the more likely driver of any change in Leopard Cat activity. This assumption was validated by running a GLMM of Leopard Cat activity against human activity in the normal vegetation plots (removing the effects of treatment and time), which confirmed that there was no relationship (GLMM: Estimate: −0.120 ± 0.158, N: 36, Z: −0.758, P: 0.448). We used a negative binomial distribution, as the data were counts and a Poisson distributed model was overdispersed.
We ran a series of GLMMs to determine whether rat abundance differed immediately after the treatments were established, by comparing the years before and after the treatments were applied (Equation 2 in Supplementary Information). We included vegetation treatment, time (Time2: categorical with two levels), and the interaction between them. This indicated that there was no effect of the vegetation treatments on rat abundance in the year immediately post-treatment (Equation 2 in Table 2), so we then analyzed the post-treatment data independently from the pre-treatment data as the act of implementing the treatments had affected all treatments the same. We ran a GLMM to determine the effect of the vegetation treatments on rat abundance through time, including only post-treatment time points (Equation 3 in Supplementary Information). We included Vegetation, time (Time3: continuous with six time points), and the interaction between them. We included a first-order autoregressive (AR-1) autocorrelation structure to account for temporal autocorrelation, as the time points were equally spaced. We confirmed that there was no residual autocorrelation using autocorrelation function (ACF) plots. We ran a GLMM to determine whether rat abundance was a function of Leopard Cat activity (Equation 4 in Supplementary Information). We ran this separately from the model with Vegetation and Time3 as Leopard Cat activity was only measured at three time points, compared to six for the other variables. For all three models we used a gamma distribution and a log link as a Gaussian distributed model predicted negative fitted values.
We ran a series of GLMMs to determine whether rat damage was a function of rat abundance. We used a beta distribution and a logit link, as the response variable was a proportion (Equation 5 in Supplementary Information). As some values assumed the extremes of 0 or 1, we transformed the data using the equation (Cribari-Neto and Zeileis, 2010):
Where:
Y = rat damage
N = sample size.
Results
Camera Trap Data
Six species of mammals (excluding humans) (178 observations), five species of birds (45 observations), and monitor lizards (eight observations) were recorded using the camera traps (Table 1). Frogs (one observation), rats (six observations), and seven “Unknown” observations (i.e., where the image in the photograph was blurry) were excluded from analysis, as it was not possible to identify these to species from the camera trap photos. An accumulation curve plotted across all plots and species showed that sampling of species using this method was nearly complete (Figure S3). Humans (plantation workers and researchers) accounted for the vast majority of observations at all sites (79% of all observations). After humans, Leopard Cats were by far the most common species recorded (65.5% of mammal observations). Other wild mammals recorded were the Common Palm Civet (14% of mammal observations) and wild pig (0.4% of mammal observations). 87% of Leopard Cat observations and 97% of Common Palm Civet were at night (18:00–06:00). Domestic species (Domestic cats, Domestic dogs and Bali cattle) accounted for 20% of the total mammal records. Leopard Cat activity did not change in the enhanced plots after the treatment was applied, but became significantly lower in the normal and reduced plots (Figure 2, Equation 1 in Table 2).
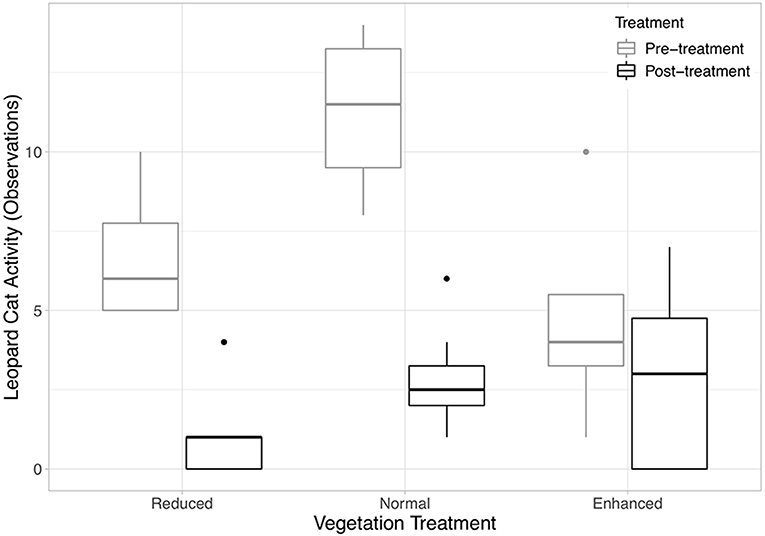
Figure 2. Boxplots showing the median and interquartile ranges (IQR) of Leopard Cat activity (number of observations) against vegetation treatment (Equation 1 in Supplementary Information, Equation 1 in Table 1). The legend codes the time period. Post-treatment data includes 2 and 3 years after the treatment was applied. The whiskers incorporate data that are 1.5*IQR. Data beyond the whiskers are plotted individually.
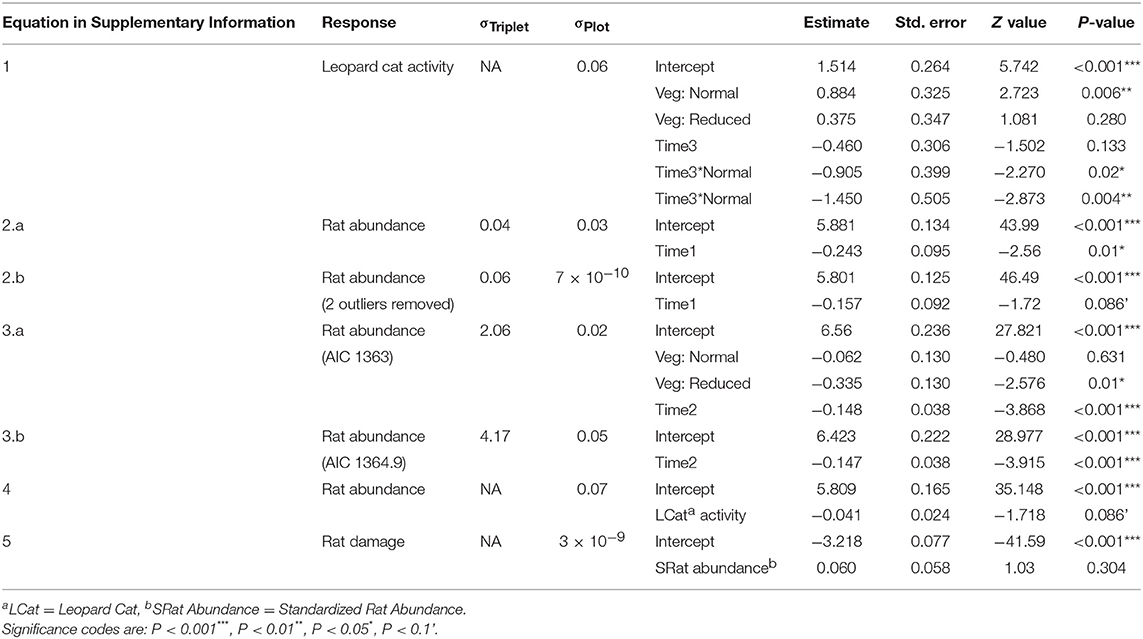
Table 2. Estimated regression parameters (unstandardized), standard errors, z-values and P-values for the GLMMs presented in Supplementary Information.
Rat Abundance and Damage
Rat abundance was high across all plots. It ranged from 52 to 992 individuals per ha, with an average of 300. Rat abundance was significantly lower in the year immediately post-treatment (Equation 2a in Table 2), but did not appear to be affected by vegetation treatment, as this variable was not included in the final model. However, this reduction in abundance was somewhat driven by two outliers, as when these were removed the relationship only tended toward significance (Equation 2b in Table 2). Having shown that there was no effect of the vegetation treatments on rat abundance in the year immediately post-treatment, we modeled rat abundance against vegetation treatment through time for post-treatment time points only and found two models of best fit (Equation 3a,b in Table 2). Both showed that rat abundance declined uniformly across all vegetation treatments after they were applied (Figure 3, Equation 3a in Table 2). The interaction between vegetation treatment and time was not included in the final models, showing that the vegetation treatments did not affect rat abundance. There was a trend for a reduction in rat abundance with greater activity of Leopard Cats (Figure 4, Equation 4 in Table 2). Levels of rat damage were low, from 0 to 11.7% with an average of 3.5% of palms showing damage. Rat damage did not have a significant relationship with rat abundance (Figure 5, Equation 5 in Table 2).
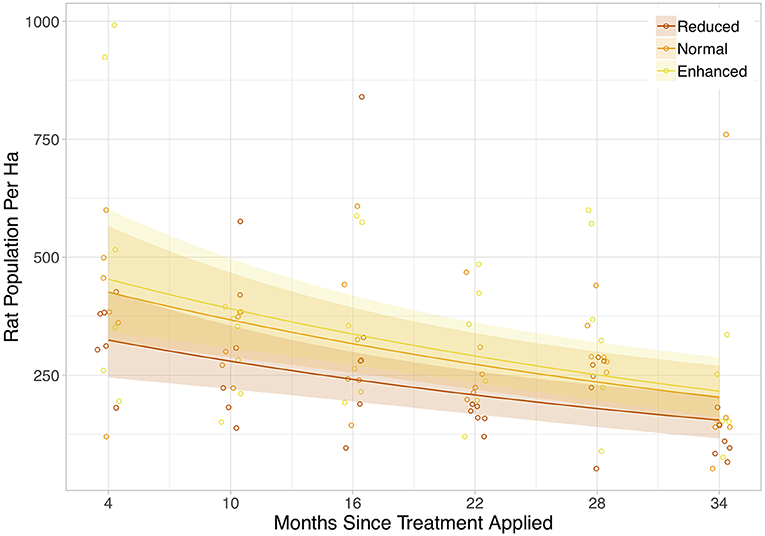
Figure 3. Rat population per ha against time since the vegetation treatment was applied. The colored lines are the lines of best fit (Equation 3 in Supplementary Information, Equation 3a in Table 1), and shaded areas show 95% confidence intervals. The legend codes the three vegetation treatments. Points are jittered slightly along the x-axis for clarity. Each individual point represents a single plot, and all post-treatment time points are included.
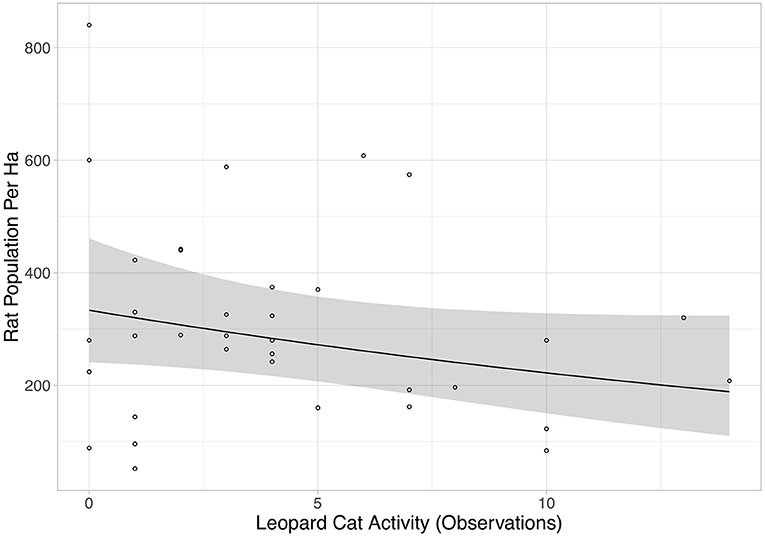
Figure 4. Rat population per ha against Leopard Cat activity (number of observations). Each individual point represents a single plot, and all treatments and time points when the camera traps were active are included. The black line is the line of best fit (Equation 4 in Supplementary Information, Equation 4 in Table 1), and shaded area shows 95% confidence intervals.
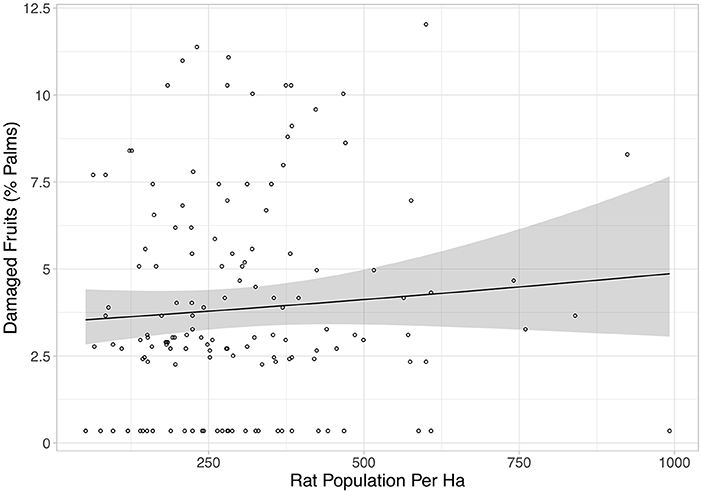
Figure 5. The percentage of damaged fruits against rat population per ha. Each individual point represents a single plot, and all treatments and time points are included. The black line is the line of best fit (Equation 5 in Supplementary Information, Equation 5 in Table 1), and the shaded area shows 95% confidence intervals. This relationship was not significant.
Discussion
We recorded only a limited number of mammal and bird species living in the plantations from our camera traps, with accumulation curves indicating that we sampled the majority of species present. All the wild species we recorded are commonly associated with more disturbed habitats (Payne and Frances, 2007; MacKinnon and Phillipps, 2014). We also recorded a high incidence of domestic species and a very high incidence of human activity, highlighting the high levels of disturbance and active management that take place in oil palm plantations. These findings are in line with other studies that have demonstrated a low persistence of forest species in oil palm plantations (e.g., Wearn et al., 2017). Of the native species recorded, Leopard Cats made up the vast majority of recordings, with 146 occurrences logged over the collection period. From trapping data, rat abundances were also high but very variable across our plots, with densities ranging from 52 to 992 individuals per hectare; a wider range than that reported from earlier studies in Malaysian estates that recorded between 106 and 578 individuals per hectare (Wood and Fee, 2003).
The high number of detections of Leopard Cats we recorded is a clear indicator that the species can reach high numbers in oil palm plantations, as has been recorded from other studies in the same area (Jennings et al., 2015). As the nearest intact forest is 60 km away, the nearest degraded forest is 15 km away, and the home range size for Leopard Cats is not known to extend past 13 km2 (Chen et al., 2016), the Leopard Cats must be resident within the oil palm matrix itself. A major reason for their persistence in the plantations is likely to be the high number of rats as prey. In addition, the wider plantation management around the BEFTA plots involves only targeted application of herbicides in a 1.5 m circle around oil palms or along harvesting paths (Luke et al., under review). Therefore, a considerable amount of understory vegetation is allowed to develop (Luke et al., 2019), providing Leopard Cats with shelter and potentially increasing numbers. Indeed, casual observations in the field included disturbing Leopard Cats from areas of dense vegetation during the day and juvenile Leopard Cats being observed in the plots, indicating that the species is using the areas to rest during the day and are reproducing within the plantations. Finally, Leopard Cats are actively protected from hunting in these plantations, owing to a belief that they are important pest control agents.
Both Leopard Cat activity and rat abundance declined over the course of the experiment. It is likely that this decline in both species is related to the drought caused by an El Niño Southern Oscillation in 2015 (Santoso et al., 2017). Lower rainfall levels are known to reduce fruit production in oil palm, with past El Niño episodes resulting in yield reductions of between 10 and 21% (Suresh, 2013). Therefore, severe drought may result in a poorer food supply for rats and potentially impact negatively on their population. This in turn could reduce Leopard Cat numbers and reproduction, as rats make up a large proportion of their diets (Rajaratnam et al., 2007; Chua et al., 2016). Local observations of Leopard Cat scat contents indicate that rats make up 75.1% of scat volume in oil palm plantations in Riau (Verwilghen, 2015). An alternative reason for the lower number of observations in 2015 and 2016 could be due to an increase in disturbance caused by human activity, but as we found no relationship between human and Leopard Cat activity drought may be the more likely explanation.
Despite overall declines in Leopard Cat activity in the normal and reduced complexity treatments, the number of observations remained constant in the enhanced complexity plots over the course of the experiment. This indicates that plots with higher vegetation cover are preferred by Leopard Cats and that this preference is enough to offset the overarching declines in habitat use observed. Given the relatively large home range size of Leopard Cats of 1.5–12.4 km2 (Chen et al., 2016), it is unlikely that individuals are living exclusively in the plots, but rather that they are using them as resting or hunting sites. Given the role of dense understory for daytime resting and breeding by Leopard Cats and their preference for more open habitats for hunting that is cited in the literature (Rajaratnam et al., 2007), it is likely that the importance of dense vegetation for resting during the day is the more likely reason for the relatively greater Leopard Cat activity observed in enhanced plots. In contrast to Leopard Cats, numbers of rats and damage to fruit did not differ between the three treatments over the timeframe of the experiment. This result goes against the results of other studies, which found higher densities of rats in areas with higher vegetation cover (Puan et al., 2011a). It is possible that the rats are benefitting from the greater vegetation complexity here too, but that the higher activity of Leopard Cats, and resultant predation, outweighs these benefits.
Overall, it is therefore clear that enhancing vegetation complexity in oil palm plantations increases Leopard Cat activity. In this study we also found that greater Leopard Cat activity was associated with reduced rat numbers. Therefore, managing for greater understory complexity not only helps to support a native carnivore population, but could also have a positive impact in reducing rat damage to oil palm fruits. In contrast to other studies (e.g., Puan et al., 2011c), we did not find that higher rat numbers were associated with greater crop damage. However, levels of damage in our plots were uniformly low (an average of only 3.5% of palms showing damage), indicating that rats were not causing significant crop damage at the densities present.
Here we experimentally show for the first time the value of increasing understory complexity for Leopard Cats. Other studies in oil palm have also demonstrated the value of increased understory complexity in supporting Lepidopteran pest predators, such as ants, beetles, and birds (Nájera and Simonetti, 2010; Ashton-Butt et al., 2018). There is, therefore, growing evidence that managers should aim to reduce spraying and management of the understory as far as possible, to benefit important predatory groups (Turner and Hinsch, 2018), with potential benefits for pest control. By enhancing the species richness and evenness of predators, including native ones, the ecosystem will be more resilient against pest outbreaks (Crowder et al., 2010; Bessou et al., 2017). The wider BEFTA Programme is continuing to measure whether allowing understory growth results in reduced yield of oil palms, through increased competition between understory plants and palms, but if this is not the case then encouraging understory regrowth represents a cheap and tractable solution for encouraging predators into oil palm plantations. These results are therefore directly relevant to Integrated Pest Management practices in plantations and could be implemented to reduce pesticide applications as part of sustainability guidelines for plantation management, such as the Principles and Criteria of the Roundtable on Sustainable Palm Oil (RSPO) [Roundtable on Sustainable Palm Oil (RSPO), 2018].
Data Availability
The datasets generated for this study are available on request to the corresponding author.
Ethics Statement
This study was exempt from ethics approval as according to Indonesian regulation Undang 440 undang no. 12. 1992 pest monitoring must be carried out in plantations. These data were collected as part of ongoing monitoring in the plantations, following standard industry operating procedures. No change in the monitoring procedure was made for this study. An ethics approval was not required for this study as per applicable institutional and national guidelines and regulations.
Author Contributions
AA, ADA, SP, JS, MN, WF, J-PC, and ET contributed conception and design of the study. AA, ADA, and MN led the collection of data. AA, AH, ET, DP, and RW wrote the first draft of the manuscript, with particular input from SL and WF. AA, AH, DP, and RW performed the statistical analysis. All authors contributed to manuscript revision, read, and approved the submitted version.
Funding
This work was funded by The Isaac Newton Trust Cambridge, Sinar Mas Agro Resources Technology Research Institute (SMARTRI), and the Natural Environment Research Council [grant number NE/P00458X/1]. AH was funded by the Claire Barnes Studentship from the Department of Zoology, University of Cambridge. Fieldwork was funded by SMARTRI.
Conflict of Interest Statement
Co-authors listed with a SMARTRI affiliation were employed by SMARTRI, a research division of Sinar Mas, at the time of the study. The wider BEFTA Programme is co-funded by Sinar Mas, with matched funds from the Isaac Newton Trust, Cambridge. However, there is an MOU in place that protects the intellectual freedom and data-use for all researchers working on the project. The Programme therefore represents a collaboration between the University of Cambridge and an oil palm company, ensuring that results are readily disseminated to inform best management practices, but maintaining academic independence.
The handling Editor declared a past co-authorship with several of the authors AH and SL.
Acknowledgments
We thank Pt. Ivo Mas Tunggal and Golden Agri Resources, and Sinar Mas Agro Resources Technology Research Institute (SMARTRI) for permission to work in their plantations. We are grateful to all the staff at SMARTRI for their hard work on this project, and to Ollie Wearn for his comments on the manuscript. We thank RISTEK for granting ECT research permission to establish the BEFTA Programme 426/SIP/FRP/SM/XI/2012, 72/EXT/SIP/FRP/SM/IX/2013, and 44/EXT/SIP/FRP/SM/IX/2014. We thank our two reviewers for their helpful comments.
Supplementary Material
The Supplementary Material for this article can be found online at: https://www.frontiersin.org/articles/10.3389/ffgc.2019.00051/full#supplementary-material
References
Ashton-Butt, A., Aryawan, A. A. K., Hood, A. S. C., Naim, M., Purnomo, D., Suhardi, et al. (2018). Understory vegetation in oil palm plantations benefits soil biodiversity and decomposition rates. Front. For. Glob. Chang. 1:10. doi: 10.3389/ffgc.2018.00010
Azhar, B., Lindenmayer, D., Wood, J., Fischer, J., Manning, A., McElhinny, C., et al. (2013). Contribution of illegal hunting, culling of pest species, road accidents and feral dogs to biodiversity loss in established oil-palm landscapes. Wildl. Res. 40, 1–9. doi: 10.1071/WR12036
Azhar, B., Lindenmayer, D. B., Wood, J., Fischer, J., and Zakaria, M. (2014a). Ecological impacts of oil palm agriculture on forest mammals in plantation estates and smallholdings. Biodivers. Conserv. 23, 1175–1191. doi: 10.1007/s10531-014-0656-z
Azhar, B., Puan, C. L., Zakaria, M., Hassan, N., and Arif, M. (2014b). Effects of monoculture and polyculture practices in oil palm smallholdings on tropical farmland birds. Basic Appl. Ecol. 15, 336–346. doi: 10.1016/j.baae.2014.06.001
Bailey, N. T. J. (1952). Improvements in the interpretation of recapture data. J. Anim. Ecol. 21, 120–127. doi: 10.2307/1913
Bates, D. M., Maechler, M., Bolker, B., and Walker, S. (2015). Fitting linear mixed-effects models using lme4. J. Stat. Softw. 67, 1–48. doi: 10.18637/jss.v067.i01
Bessou, C., Verwilghen, A., Beaudoin-Ollivier, L., Marichal, R., Ollivier, J., Baron, V., et al. (2017). Agroecological practices in oil palm plantations: examples from the field. Agro-ecology 24:D305. doi: 10.1051/ocl/2017024
Brooks, M. E., Kristensen, K., Benthem, K. J., van Magnusson, A., Berg, C. W., Nielsen, A., et al. (2017). glmmTMB balances speed and flexibility among packages for zero-inflated generalized linear mixed modeling. R J. 9, 378–400. doi: 10.32614/RJ-2017-066
Chen, M.-T., Liang, Y.-J., Kuo, C.-C., and Pei, K. J.-C. (2016). Home ranges, movements and activity patterns of Leopard Cats (Prionailurus bengalensis) and threats to them in Taiwan. Mammal Study 41, 77–86. doi: 10.3106/041.041.0205
Chua, M. A. H., Sivasothi, N., and Meier, R. (2016). Population density, spatiotemporal use and diet of the Leopard Cat (Prionailurus bengalensis) in a human-modified succession forest landscape of Singapore. Mammal Res. 61, 99–108. doi: 10.1007/s13364-015-0259-4
Cribari-Neto, F., and Zeileis, A. (2010). Beta regression in R. J. Stat. Softw. 34, 1–24. doi: 10.18637/jss.v034.i02
Crowder, D. W., Northfield, T. D., Strand, M. R., and Snyder, W. E. (2010). Organic agriculture promotes evenness and natural pest control. Nature 466, 109–112. doi: 10.1038/nature09183
Dislich, C., Keyel, A. C., Salecker, J., Kisel, Y., Meyer, K. M., Auliya, M., et al. (2017). A review of the ecosystem functions in oil palm plantations, using forests as a reference system. Biol. Rev. 49, 1539–1569. doi: 10.1111/brv.12295
Duckett, J. E. (1976). Owls as major predators of rats in oil palm estates with particular reference to the barn owl (Tyto alba). Plant. Malaysia 52, 4–15.
Foster, W. A., Snaddon, J. L., Turner, E. C., Fayle, T. M., Cockerill, T. D., Ellwood, M. D. F., et al. (2011). Establishing the evidence base for maintaining biodiversity and ecosystem function in the oil palm landscapes of South East Asia. Philos. Trans. R. Soc. B Biol. Sci. 366, 3277–3291. doi: 10.1098/rstb.2011.0041
Gamage, S. N., Weerakoon, D. K., and Gunawardena, A. (2011). Current status of vertebrate diversity in anthropogenic and natural ecosystems in south-western Sri Lanka. J. Natl. Sci. Found. Sri Lanka 39, 383–389. doi: 10.4038/jnsfsr.v39i4.3886
Gaynor, K. M., Hojnowski, C. E., Carter, N. H., and Brashares, J. S. (2018). The influence of human disturbance on wildlife nocturnality. Science 360, 1232–1235. doi: 10.1126/science.aar7121
Jennings, A. P., Naim, M., Advento, A. D., Aryawan, A. A. K., Ps, S., Caliman, J. P., et al. (2015). Diversity and occupancy of small carnivores within oil palm plantations in central Sumatra, Indonesia. Mammal Res. 60, 181–188. doi: 10.1007/s13364-015-0217-1
Khosla, P. (2011). ‘Nutritional characteristics of palm oil,’ in Reducing Saturated Fats in Foods (Elsevier), 112–127. doi: 10.1533/9780857092472.1.112
Kogan, M. (1998). Integrated pest management: historical perspectives and contemporary developments. Annu. Rev. Entomol. 43, 243–270. doi: 10.1146/annurev.ento.43.1.243
Koh, L. P., Levang, P., and Ghazoul, J. (2009). Designer landscapes for sustainable biofuels. Trends Ecol. Evol. 24, 431–438. doi: 10.1016/j.tree.2009.03.012
Koh, L. P., Miettinen, J., Liew, S. C., and Ghazoul, J. (2011). Remotely sensed evidence of tropical peatland conversion to oil palm. Proc. Natl. Acad. Sci. U.S.A. 108, 5127–5132. doi: 10.1073/pnas.1018776108
Koh, L. P., and Wilcove, D. S. (2008). Is oil palm agriculture really destroying tropical biodiversity? Conserv. Lett. 1, 60–64. doi: 10.1111/j.1755-263X.2008.00011.x
Luke, S. H., Purnomo, D., Advento, A. D., Aryawan, A. A. K., Naim, M., Pikstein, R. N., et al. (2019). Effects of understory vegetation management on plant communities in oil palm plantations in Sumatra, Indonesia. Front. For. Glob. Change 2:33. doi: 10.3389/ffgc.2019.00033
Luskin, M. S., and Potts, M. D. (2011). Microclimate and habitat heterogeneity through the oil palm lifecycle. Basic Appl. Ecol. 12, 540–551. doi: 10.1016/j.baae.2011.06.004
MacKinnon, J., and Phillipps, K. (2014). A Field Guide to the Birds of Borneo, Sumatra, Java, and Bali, 12th Edn. New York, NY: Oxford University Press.
Maddox, T., Priatna, D., Gemita, E., and Salampessy, A. (2007). The conservation of tigers and other wildlife in oil palm plantations, Jambi Province, Sumatra, Indonesia (October 2007). ZSL Conserv. Rep. 7, 1–66.
Mendes-Oliveira, A. C., Peres, C. A., Maués, P. C. R. D. A., Oliveira, G. L., Mineiro, I. G. B., Silva de Maria, S. L., et al. (2017). Oil palm monoculture induces drastic erosion of an Amazonian forest mammal fauna. PLoS ONE 12:e0187650. doi: 10.1371/journal.pone.0187650
Nájera, A., and Simonetti, J. A. (2010). Can oil palm plantations become bird friendly? Agroforest Syst. 80, 203–209. doi: 10.1007/s10457-010-9278-y
Nakashima, Y., Nakabayashi, M., and Sukor, J. A. (2013). Space use, habitat selection, and day-beds of the common palm civet (Paradoxurus hermaphroditus) in human-modified habitats in Sabah, Borneo. J. Mammal. 94, 1169–1178. doi: 10.1644/12-MAMM-A-140.1
Nieuwenhuis, R., te Grotenhuis, M., and Pelzer, B. (2012). influence.ME: tools for detecting influential data in mixed effects models. R. J. 4, 38–47. doi: 10.32614/RJ-2012-011
OECD Publishing (2018). ‘Oilseeds and oilseed products’, in OECD-FAO Agricultural Outlook 2018-2027 (Rome: Paris/Food and Agriculture Organization of the United Nations), 127–138. doi: 10.1787/agr_outlook-2018-7-en
Oksanen, J., Blanchet, F. G., Friendly, M., Kindt, R., Legendre, P., McGlinn, D., et al. (2018). vegan: Community Ecology Package. R package version 2.5-2. Available online at: https://CRAN.R-project.org/package=vegan
Pardo, L. E., Campbell, M. J., Cove, M. V., Edwards, W., Clements, G. R., and Laurance, W. F. (2019). Land management strategies can increase oil palm plantation use by some terrestrial mammals in Colombia. Sci. Rep. 9:7812. doi: 10.1038/s41598-019-44288-y
Pardo, L. E., Campbell, M. J., Edwards, W., Clements, G. R., and Laurance, W. F. (2018). Terrestrial mammal responses to oil palm dominated landscapes in Colombia. PLoS ONE 13:e0197539. doi: 10.1371/journal.pone.0197539
Payne, J., and Frances, C. M. (2007). A Field Guide to the Mammals of Borneo. Kota Kinabalu: The Sabah Society.
Phalan, B., Bertzky, M., Butchart, S. H. M., Donald, P. F., Scharlemann, J. P. W., Stattersfield, A. J., et al. (2013). Crop expansion and conservation priorities in tropical countries. PLoS ONE 8:e51759. doi: 10.1371/journal.pone.0051759
Puan, C. L., Goldizen, A. W., Zakaria, M., and Baxter, G. S. (2011a). Understanding of relationships between ground cover and rat abundances: an integrative approach for management of the oil palm agroecosystem. Crop Prot. 30, 1263–1268. doi: 10.1016/j.cropro.2011.05.025
Puan, C. L., Goldizen, A. W., Zakaria, M., Hafidzi, M. N., and Baxter, G. S. (2011b). Absence of differential predation on rats by Malaysian barn owls in oil palm plantations. J. Raptor Res. 45, 71–78. doi: 10.3356/JRR-10-18.1
Puan, C. L., Goldizen, A. W., Zakaria, M., Hafidzi, M. N., and Baxter, G. S. (2011c). Relationships among rat numbers, abundance of oil palm fruit and damage levels to fruit in an oil palm plantation. Integr. Zool. 6, 130–139. doi: 10.1111/j.1749-4877.2010.00231.x
R Core Team (2018). R: A Language and Environment for Statistical Computing. R Foundation for Statistical Computing. Available online at: https://www.r-project.org/
Rajaratnam, R., Sunquist, M., Rajaratnam, L., and Ambu, L. (2007). Diet and habitat selection of the Leopard Cat (Prionailurus bengalensis borneoensis) in an agricultural landscape in Sabah, Malaysian Borneo. J. Trop. Ecol. 23, 209–217. doi: 10.1017/S0266467406003841
Reijnders, L., and Huijbregts, M. A. J. (2008). Palm oil and the emission of carbon-based greenhouse gases. J. Clean. Prod. 16, 477–482. doi: 10.1016/j.jclepro.2006.07.054
Richards, S. A., Whittingham, M. J., and Stephens, P. A. (2011). Model selection and model averaging in behavioural ecology: the utility of the IT-AIC framework. Behav. Ecol. Sociobiol. 65, 77–89. doi: 10.1007/s00265-010-1035-8
Roundtable on Sustainable Palm Oil (RSPO) (2018). Principles and Criteria for the Production of Sustainable Palm Oil 2018.
RStudio Team (2016). RStudio: Integrated Development for R. RStudio, Inc. Available online at: http://www.rstudio.com/
Santoso, A., Mcphaden, M. J., and Cai, W. (2017). The defining characteristics of ENSO extremes and the strong 2015/2016 El Niño. Rev. Geophys. 55, 1079–1129. doi: 10.1002/2017RG000560
Spear, D. M., Foster, W. A., Advento, A. D., Naim, M., Caliman, J.-P., Luke, S. H., et al. (2018). Simplifying understory complexity in oil palm plantations is associated with a reduction in the density of a cleptoparasitic spider, Argyrodes miniaceus (Araneae: Theridiidae), in host (Araneae: Nephilinae) webs. Ecol. Evol. 8, 1595–1603. doi: 10.1002/ece3.3772
Stibig, H. J., Achard, F., Carboni, S., Raši, R., and Miettinen, J. (2014). Change in tropical forest cover of Southeast Asia from 1990 to 2010. Biogeosciences 11, 247–258. doi: 10.5194/bg-11-247-2014
Suresh, K. (2013). ‘Adaptation and mitigation strategies for climate-resilient oil palm,’ in Climate-Resilient Horticulture: Adaptation and Mitigation Strategies, eds H. C. P. Singh, N. K. S. Rao, and K. S. Shivashankar (Springer India), 199–211. doi: 10.1007/978-81-322-0974-4_18
Tayleur, C., Balmford, A., Buchanan, G. M., Butchart, S. H. M., Ducharme, H., Green, R. E., et al. (2017). Global coverage of agricultural sustainability standards, and their role in conserving biodiversity. Conserv. Lett. 10, 610–618. doi: 10.1111/conl.12314
Tilman, D., Balzer, C., Hill, J., and Befort, B. L. (2011). Global food demand and the sustainable intensification of agriculture. Proc. Natl. Acad. Sci. U.S.A. 108, 20260–20264. doi: 10.1073/pnas.1116437108
Tohiran, K. A., Nobilly, F., Zulkifli, R., Ashton-Butt, A., and Azhar, B. (2019). Cattle-grazing in oil palm plantations sustainably controls understory vegetation. Agric. Ecosyst. Environ. 278, 54–60. doi: 10.1016/j.agee.2019.03.021
Turner, E. C., and Hinsch, J. (2018). ‘Integrated pest management in sustainable palm oil production,’ in Achieving Sustainable Cultivation of Oil Palm- Volume 2: Diseases, Pests, Quality and Sustainability, ed A. Rival [Center for International Cooperation in Agricultural Research for Development (CIRAD)], Cambridge: Burleigh Dodds Science Publishing, 93–113.
Verwilghen, A. (2015). Rodent Pest Management And Predator Communities in Oil Palm Plantations in Indonesia: A Comparison of Two Contrasting Systems. France: Ph.D. Thesis, University of Franche-Comté, Besançon.
Wearn, O. R., Carbone, C., Rowcliffe, J. M., Bernard, H., and Ewers, R. M. (2016). Grain- dependent responses of mammalian diversity to land use and the implications for conservation set- aside. Ecol. Appl. 26, 1409–1420. doi: 10.1890/15-1363
Wearn, O. R., Carbone, C., Rowcliffe, J. M., Pfeifer, M., Bernard, H., and Ewers, R. M. (2019). Land-use change alters the mechanisms assembling rainforest mammal communities in Borneo. J. Anim. Ecol. 88, 125–137. doi: 10.1111/1365-2656.12903
Wearn, O. R., Rowcliffe, J. M., Carbone, C., Pfeifer, M., Bernard, H., and Ewers, R. M. (2017). Mammalian species abundance across a gradient of tropical land-use intensity: a hierarchical multi-species modelling approach. Biol. Conserv. 212, 162–171. doi: 10.1016/j.biocon.2017.05.007
Wicke, B., Sikkema, R., Dornburg, V., and Faaij, A. (2011). Exploring land use changes and the role of palm oil production in Indonesia and Malaysia. Land Use Policy 28, 193–206. doi: 10.1016/j.landusepol.2010.06.001
Wickham, H. (2017). tidyverse: Easily Install and Load the ‘Tidyverse’. R package version 1.2.1. Available online at: https://CRAN.R-project.org/package=tidyverse
Wood, B. J. (2002). Pest control in Malaysia's perennial crops: a half century perspective tracking the pathway to integrated pest management. Integr. Pest Manag. Rev. 7, 173–190. doi: 10.1023/B:IPMR.0000027501.91697.49
Wood, B. J., and Fee, C. G. (2003). A critical review of the development of rat control in Malaysian agriculture since the 1960s. Crop Prot. 22, 445–461. doi: 10.1016/S0261-2194(02)00207-7
Wood, B. J., and Liau, S. S. (1984). A long-term study of Rattus tiomanicus populations in an oil palm plantation in Johore, Malaysia: II. Recovery from control and economic aspects. J. Appl. Ecol. 21, 465–472. doi: 10.2307/2403422
Yue, S., Brodie, J. F., Zipkin, E. F., and Bernard, H. (2015). Oil palm plantations fail to support mammal diversity. Ecol. Appl. 25, 2285–2292. doi: 10.1890/14-1928.1
Zuur, A. F., and Ieno, E. N. (2016). A protocol for conducting and presenting results of regression-type analyses. Methods Ecol. Evol. 7, 636–645. doi: 10.1111/2041-210X.12577
Keywords: oil palm, Leopard Cat, Rattus, habitat heterogeneity, habitat complexity, understory vegetation, integrated pest management, herbicide
Citation: Hood ASC, Aryawan AAK, Advento AD, Purnomo D, Wahyuningsih R, Luke SH, Ps S, Snaddon JL, Foster WA, Caliman J-P, Turner EC and Naim M (2019) Understory Vegetation in Oil Palm Plantations Promotes Leopard Cat Activity, but Does Not Affect Rats or Rat Damage. Front. For. Glob. Change 2:51. doi: 10.3389/ffgc.2019.00051
Received: 22 February 2019; Accepted: 16 August 2019;
Published: 19 September 2019.
Edited by:
Rory Padfield, University of Leeds, United KingdomReviewed by:
Badrul Azhar, Putra Malaysia University, MalaysiaMarc Ancrenaz, HUTAN-Kinabatangan Orangutan Conservation Project, Malaysia
Copyright © 2019 Hood, Aryawan, Advento, Purnomo, Wahyuningsih, Luke, Ps, Snaddon, Foster, Caliman, Turner and Naim. This is an open-access article distributed under the terms of the Creative Commons Attribution License (CC BY). The use, distribution or reproduction in other forums is permitted, provided the original author(s) and the copyright owner(s) are credited and that the original publication in this journal is cited, in accordance with accepted academic practice. No use, distribution or reproduction is permitted which does not comply with these terms.
*Correspondence: Amelia S. C. Hood, amelia.hood3@gmail.com
†These authors have contributed equally to this work